- 1Medical Research Council Centre for Medical Mycology at the University of Aberdeen, Aberdeen Fungal Group, Aberdeen, United Kingdom
- 2School of Biological Sciences, University of East Anglia, Norwich, United Kingdom
- 3Department of Microbial Pathogenicity Mechanisms, Leibniz Institute for Natural Product Research and Infection Biology – Hans Knöll Institute, Jena, Germany
- 4Center for Sepsis Control and Care, University Hospital, Jena, Germany
- 5Institute of Microbiology, Microbial Pathogenicity, Friedrich Schiller University, Jena, Germany
Pathogenic microorganisms often face acute micronutrient limitation during infection due to the action of host-mediated nutritional immunity. The human fungal pathogen Candida albicans is polymorphic and its morphological plasticity is one of its most widely recognized pathogenicity attributes. Here we investigated the effect of zinc, iron, manganese, and copper limitation on C. albicans morphology. Restriction of zinc specifically resulted in the formation of enlarged, spherical yeasts, a phenotype which we term Goliath cells. This cellular response to zinc restriction was conserved in C. albicans, C. dubliniensis and C. tropicalis, but not in C. parapsilosis, C. lusitaniae or Debaryomyces hansenii, suggesting that it may have emerged in the last common ancestor of these related pathogenic species. Cell wall analysis revealed proportionally more chitin exposure on the Goliath cell surface. Importantly, these cells were hyper-adherent, suggesting a possible role in pathogenicity. Interestingly, the zincophore-encoding gene PRA1 was expressed by Goliath cells in zinc limited media and lack of Pra1 inhibited both cellular enlargement and adhesion. Goliath cells represent a further layer of Candida phenotypic plasticity.
Introduction
In order to prevent the growth of invading microbes and restrict the progression of infection, the mammalian immune system has developed sophisticated mechanisms to actively withhold certain essential trace nutrients such as iron and zinc. This process is called nutritional immunity (Cassat and Skaar, 2012; Hood and Skaar, 2012). Therefore, in order for a pathogen to survive this nutritionally restrictive environment within its infected host, it must have evolved strategies to overcome nutritional immunity.
Transition metals are essential to life as approximately a third of all proteins interact with a metal cofactor (Waldron et al., 2009; Hood and Skaar, 2012) and within the context of nutritional immunity, the host and invading microbe battle for these micronutrients resulting in a ‘nutritional tug-of-war’ (Anzaldi and Skaar, 2010; Skaar, 2010). The importance of host-driven iron sequestration to control microbial infections is well established (Skaar, 2010). More recently, an emerging role for zinc in nutritional immunity has been recognized (Kehl-Fie and Skaar, 2010). Zinc is an essential trace metal crucial for various biological processes and 9% of eukaryotic proteins are predicted to be zinc metalloproteins (Andreini et al., 2009). Furthermore, eukaryotic cells rely on a significant number of zinc dependent transcription factors for gene regulation (Andreini et al., 2006; Hood and Skaar, 2012). Moreno et al. (2007) demonstrated the importance of zinc acquisition in pathogenicity of Aspergillus fumigatus. Similarly, deletion of zinc transporters in Cryptococcus gattii, Cryptococcus neoformans, and Histoplasma capsulatum has been shown to attenuate virulence in murine models of infection; thus providing evidence that the response of fungal pathogens to zinc limitation is important for virulence (Amich et al., 2014; Jung, 2015; Schneider et al., 2015; Dade et al., 2016; Do et al., 2016).
Polymorphism in several fungal pathogens is a well-recognized virulence factor (Boyce and Andrianopoulos, 2015). H. capsulatum, Blastomyces dermatitidis, Paracoccidioides brasiliensis, Talaromyces marneffei and most other pleomorphic fungal pathogens grow as filamentous forms in the environment, but transition to pathogenic yeast phases insides their infected hosts (Kane, 1984; Maresca and Kobayashi, 1989). C. neoformans also transitions to pathogenic yeast growth during infection and Okagaki et al. (2010), Zaragoza et al. (2010), Okagaki and Nielsen (2012) have demonstrated the formation of giant Titan cells, which were resistant to phagocytosis by macrophage-like cells.
Candida albicans is a polymorphic fungus and its morphological plasticity is recognized as a key virulence attribute (Liu, 2001; Sudbery et al., 2004; Whiteway and Bachewich, 2007). During infections, filamentous forms of C. albicans are known to penetrate epithelial and endothelial cells and mucosal barriers causing damage to host tissue (Sudbery, 2011; Tyc et al., 2014). Multiple studies have shown that morphological transitions play an important role in host–pathogen interactions for this fungus. However, the physiological response of C. albicans to nutritional immunity is poorly understood. C. albicans, although a member of the human gut microbiota, is frequently responsible for superficial as well as life threatening disseminated infections. Kullberg and Arendrup (2015) reported more than 250,000 people worldwide suffer from severe invasive Candidiasis each year with high mortality rates (Kullberg and Arendrup, 2015).
Here we describe the physiological response of C. albicans to zinc starvation. We found that zinc (but not iron, manganese, or copper) deprivation causes C. albicans to transform to a giant yeast cell phenotype. Combined phylogenetic-phenotypic analysis indicates that this cellular-enlargement response to zinc limitation is species-specific, arose in a common ancestor of C. albicans and C. tropicalis and was not observed in several other tested Candida species. Importantly, these cells exhibit enhanced adhesion – a property normally associated with the hyphal morphology. We propose the term “Goliath” cell for this giant, hyper-adherent C. albicans phenotype.
Results
Zinc Starvation Induces Cellular Enlargement in Candida albicans
To prevent infection, the host immune system withholds certain essential nutrients like iron and zinc in a process known as nutritional immunity. In order to understand the physiological responses of C. albicans to metal starvation, the laboratory wild type (WT) strain (BWP17+Clp30), was subjected to iron, manganese, copper or zinc starvation in vitro for 3 days. Following incubation in metal limiting media, cells were observed microscopically. Figure 1 shows that, of the metals tested, zinc starvation induced cellular enlargement in C. albicans.
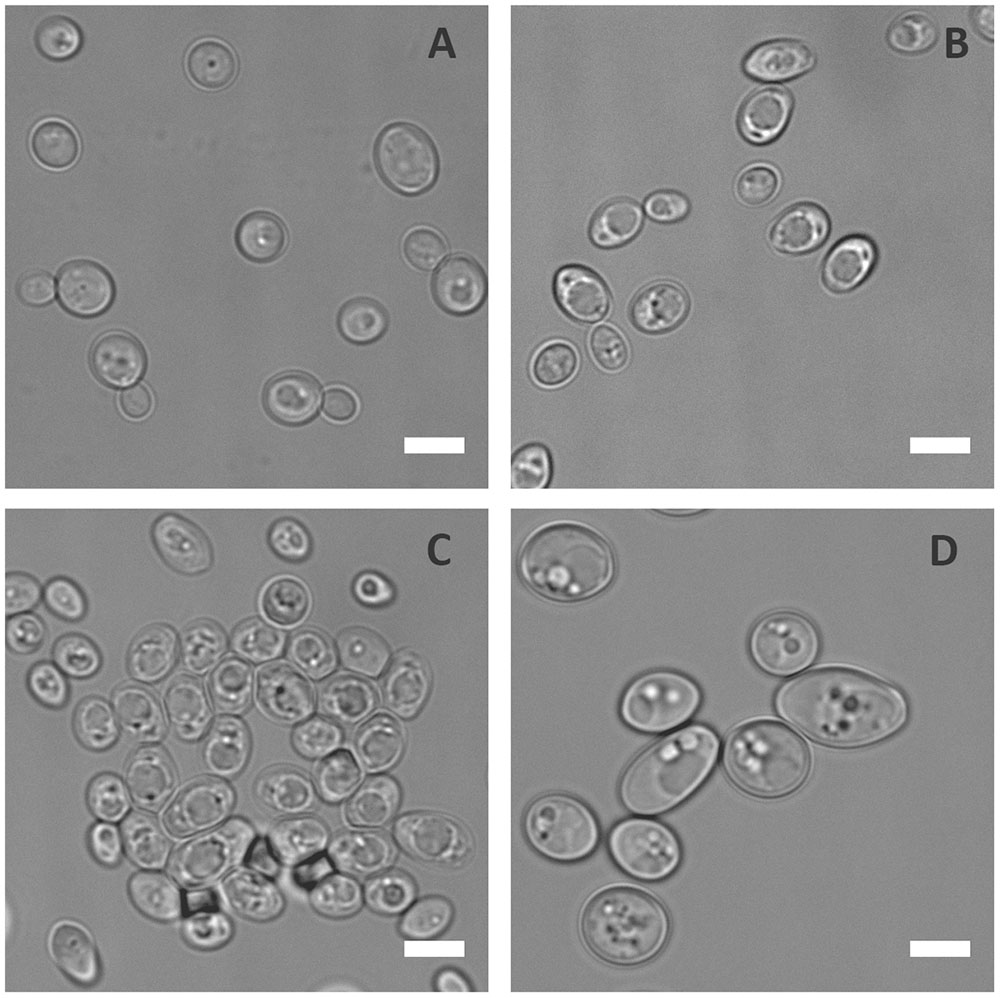
FIGURE 1. Physiological response of C. albicans to metal starvation. C. albicans (BWP17 + Clp30) cells subjected to copper (A), iron (B), manganese (C), and zinc (D) starvation by incubating in limiting medium independently lacking these metals at 30°C, 200 rpm for 3 days. Experiment performed twice. DIC images show that of the metals tested only zinc starvation resulted in cellular enlargement in C. albicans. Scale bar represents 5 μm.
Next, the kinetics of zinc limitation-induced cellular gigantism was assessed. C. albicans cells were incubated in limited zinc medium (LZM) and in medium containing zinc (LZM + Z). Cells were analysed microscopically daily for 3 days and cell volume determined. Figure 2A shows that significant cellular enlargement was observed as early as day 1 of zinc starvation, and an average cell volume of 146 μm3 (±43.6 μm3) was reached by day 3. This is in contrast to regular yeast cells which exhibit average cell volumes of 28–35 μm3. To confirm this was not a medium-specific response, C. albicans was incubated in another synthetic defined medium lacking zinc (YNB-zinc drop out – “SD0”). Again a similar cellular enlargement was observed in SD0 with cells reaching an average volume of 119 μm3 by day 3 and 198 μm3 by day 7 (Figure 2B). Figures 2C,D show that C. albicans growth was inhibited in a zinc-dependent manner in these experiments. To ensure that OD600 measurements did not represent dead cells, colony forming units (cfu) were determined. Yeast cells inoculated into LZM to a cell density of 3 × 106 cfu/ml on day 0 increased by day 1 to 1 × 107 cfu/ml. Viability (cfu/ml) then remained constant for up to 7 days.
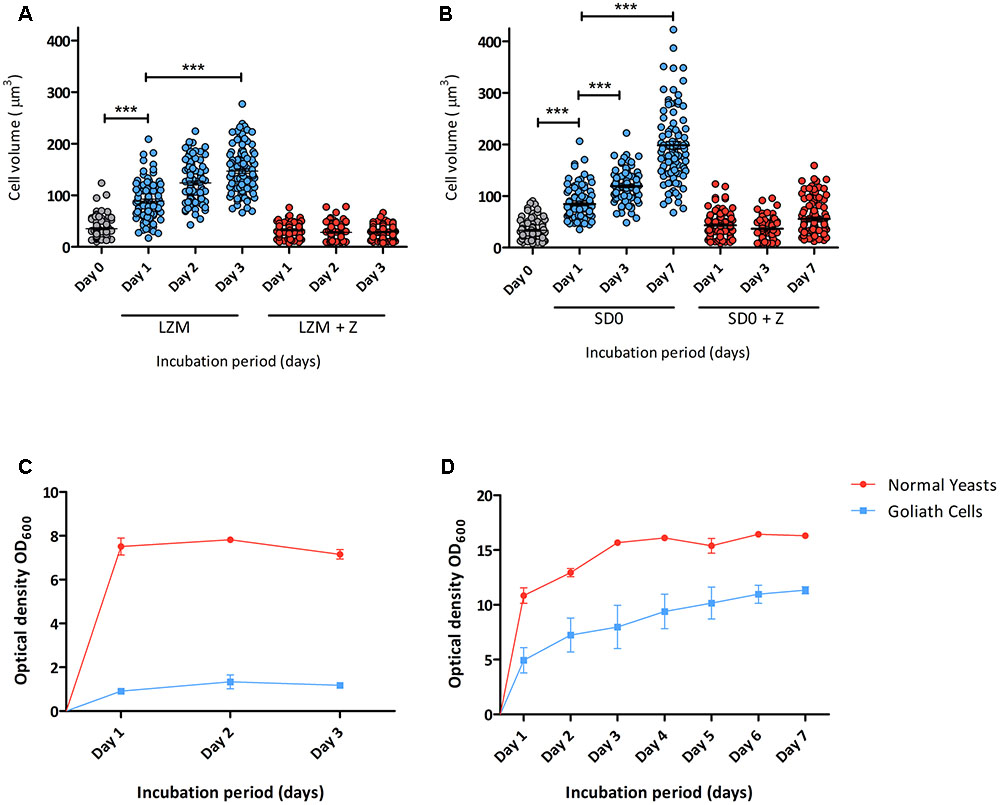
FIGURE 2. Developmental kinetics of C. albicans Goliath cell formation under zinc limitation. C. albicans cells pre-grown in SD medium were (A) incubated in LZM or LZM + Z over 3 days or (B) in SD0 or SD0 + Z over 7 days. Cells were imaged at indicated time points and axes diameters measured using ImageJ. Cell volumes were calculated by V = 4/3 π ab2. Each data column shows >100 cells from at least two independent experiments. Both zinc starvation media induced significant cellular enlargement (∗∗∗ < 0.001, Stat. test [ANOVA]). One data point (530 μm3) is omitted from SD0 Day 7 (B). Optical density (OD600) was determined for cultures incubated in (C) LZM (±zinc) every 24 h for 3 days and (D) SDw/oZ (±zinc) every 24 h for 7 days. Data are from three independent experiments. Error bars represent the standard error of the mean.
Enlarged yeast cells have previously been described for C. neoformans, where they are known as “Titan Cells” (Zaragoza et al., 2010). Here we propose the term C. albicans “Goliath cells” for this cellular enlargement observed upon zinc depletion.
Origin of Cellular Enlargement in Candida
BWP17+CIp30 used in this study is a laboratory strain. To determine if the observed cellular enlargement is a conserved response, multiple C. albicans clinical isolates spanning the four major clades of this species (Supplementary Table S1) (MacCallum et al., 2009) were incubated in LZM for 3 days and observed microscopically for cellular enlargement. All the tested clinical isolates enlarged to varying degrees upon zinc depletion (Figure 3), indicating that this response is a conserved feature of C. albicans biology.
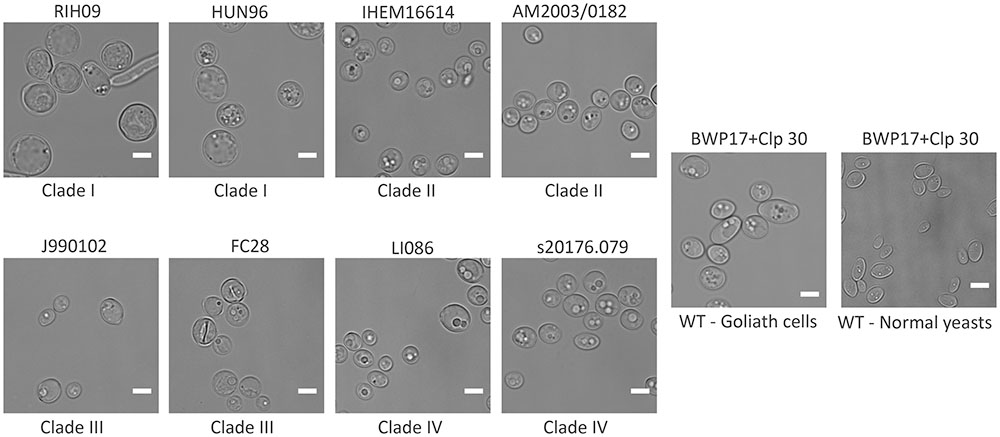
FIGURE 3. Cellular gigantism in multiple C. albicans clinical isolates. Indicated C. albicans clinical isolates pre-grown in SD medium were incubated in LZM for 3 days at 30°C, 200 rpm. DIC images show that all the tested clinical isolates can enlarge to varying degrees upon zinc depletion. Clinical isolates belonging to Clade 1 enlarged to a greater degree than other isolates. All the strains are distinctly bigger than the LZM + Z control. Scale bar represents 5 μm.
We next questioned when during fungal evolution this property emerged. C. albicans is a member of the CUG-Ser clade of yeasts, which uniquely translate the CUG codon as serine instead of leucine. We therefore incubated several clinical isolates and reference strains of C. dubliniensis, C. tropicalis, C. parapsilosis, C. lusitaniae, and Debaryomyces hansenii (also known as C. famata) under zinc depletion and monitored their morphology. Interestingly, all the tested strains of C. dubliniensis and C. tropicalis enlarged upon zinc starvation. However, the other tested species did not exhibit the Goliath cell phenotype upon zinc deprivation (Figure 4 and data not shown). This analysis suggests that cellular enlargement in response to zinc limitation is species-specific and may have arisen in the last common ancestor of C. albicans, C. dubliniensis, and C. tropicalis. All three species are pathogens of humans (Butler et al., 2009).
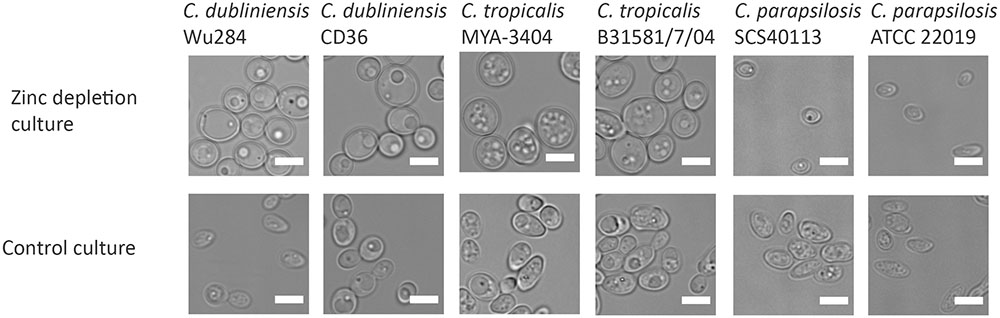
FIGURE 4. Zinc restriction-induced cellular enlargement is conserved in closely related Candida species. Multiple strains of C. dubliniensis, C. tropicalis, and C. parapsilosis were also tested for their capacity to enlarge by incubating SD-pre-grown cells in LZM (±zinc) for 3 days at 30°C, 200 rpm. All the tested strains of C. dubliniensis and C. tropicalis enlarged in response to zinc limitation, whereas C. parapsilosis strains did not enlarge upon zinc deprivation. Scale bar represents 5 μm.
The Impact of Zinc Restoration on Morphology
When C. albicans hyphae are switched to yeast culture medium, or if the hyphal maintenance program is genetically perturbed, the hyphal compartments themselves endure and produce yeast cells (Enjalbert and Whiteway, 2005; Polke et al., 2017). We therefore investigated the fate of Goliath cells and the morphology of daughter cells produced upon zinc restoration. The Goliath cell’s surface were stained with FITC, which is maintained on the stained cell during culture, but not transferred to daughter cells. These stained cells were inoculated into SD yeast growth medium at OD600 = 0.5 and incubated for 24 h. Following this culture period, cell density increased to OD600 10–12. Microscopic analysis revealed both large and regularly sized yeast cells in the culture, with only the large cells exhibiting FITC fluorescence (Figure 5). The ratio of regular-sized un-labeled to large FITC-labeled cells was 20:1 (4.7% FITC-labeled). Therefore, Goliath cells maintain their size, but generate regular-sized daughter cells in response to zinc re-supplementation.
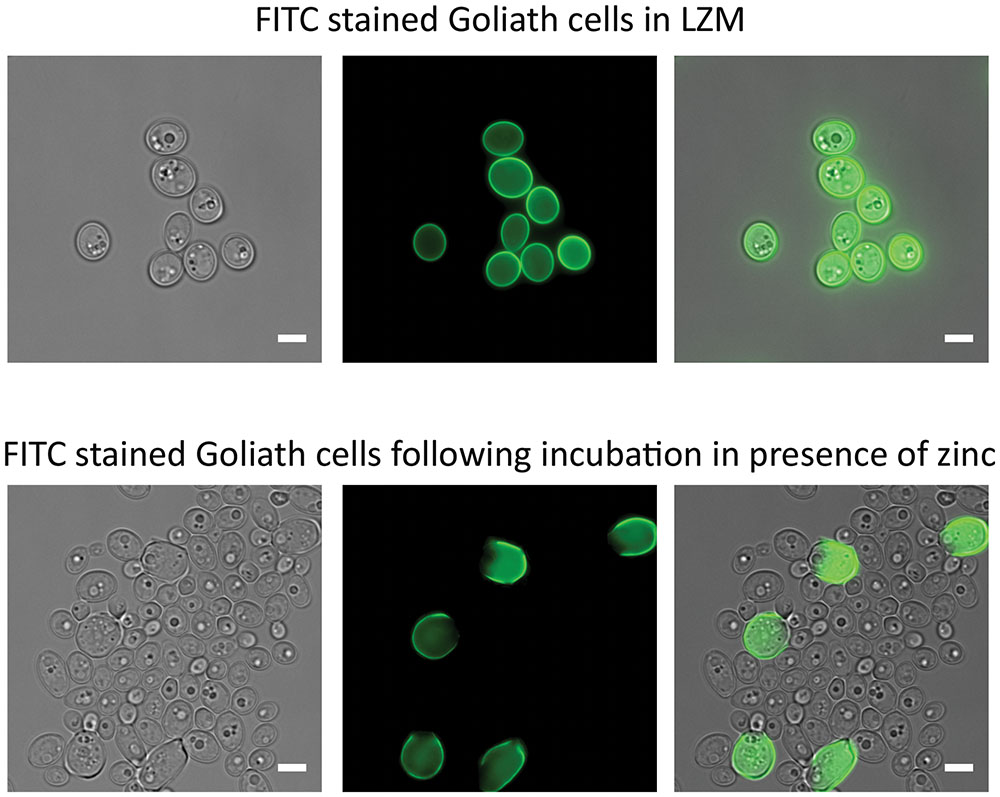
FIGURE 5. Effects of zinc restoration on Goliath cell morphology. C. albicans cells pre-grown in SD were incubated in LZM for 2 days to generate Goliath cells. These Goliath cells were FITC labeled (upper panel) and subsequently inoculated into SD (zinc-replete) medium to an OD600 = 0.5. Lower panel shows that non-labeled cells exhibit regular yeast morphology. Labeled cells remained large. Scale bar represents 5 μm.
We next tested whether Goliath cells can form hyphae. Goliath cells and control yeasts were inoculated into the wells of tissue culture plates containing RPMI and incubated at 37°C and 5% CO2 – a potent inducer of hyphae. Figure 6 shows that, as expected, the majority of control yeasts had germinated by 90 min and these hyphae continued to extend in length at 3 and 5 h post-inoculation. In contrast, Goliath cell germination was not observed until 3 h (Figure 6A). To test whether these filaments were true hyphae, the cells were stained with Calcofluor White. Figure 6B shows the presence of true hyphae formed by Goliath cells as indicated by the position of the primary septum and parallel cell wall around septa (Sudbery et al., 2004). Therefore, Goliath cells exhibit delayed filamentation in response to a strong inducer of hyphae.
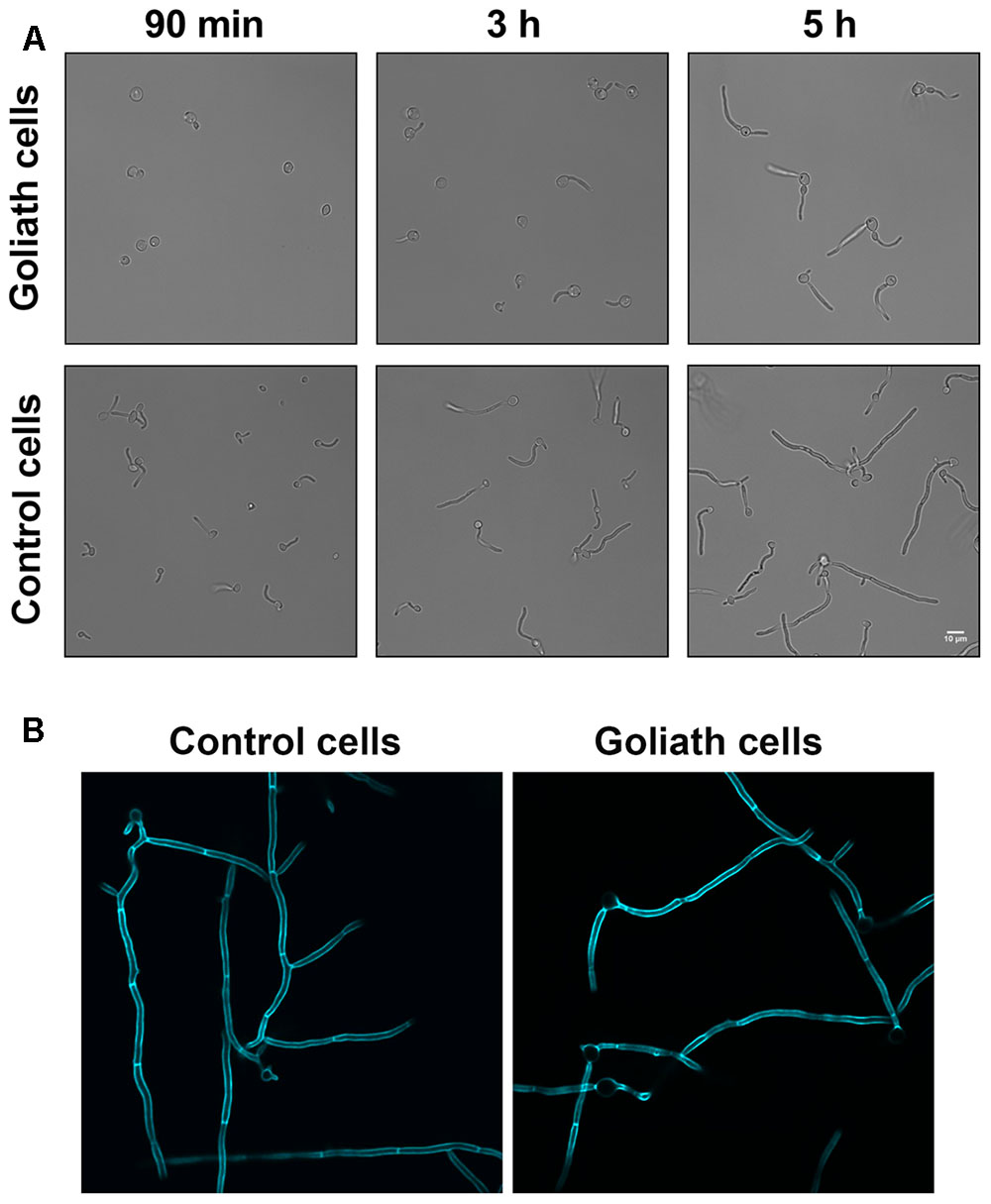
FIGURE 6. Hyphae induction. Goliath cells and normal yeast cells incubated in the presence of hyphae inducing media (RPMI) for up to 5 h (A) or 6 h at 37°C and stained with Calcofluor White (B). Goliath cells exhibit a delay in germination and hyphae formation as compared to normal yeasts. Scale bar represents 10 μm.
Cellular Enlargement Increases Chitin Exposure in C. albicans
Candida albicans yeast cells are known to remodel their cell wall in response to environmental changes and during morphological switches such as the yeast to hypha transition (Klis et al., 2001; Heilmann et al., 2011). We hypothesized that the cellular transformation to the Goliath phenotype may also be associated with cell wall remodeling. To investigate whether Goliath cells exhibit alterations in cell wall composition, cells were stained with dyes against the major cell wall components and analyzed by fluorescence microscopy (Figure 7A) and flow cytometry (Figure 7B). These were: concanavalin A (mannan), FC-dectin (β-glucan), Calcofluor White (total chitin) and FITC-conjugated wheat-germ agglutinin (exposed chitin).
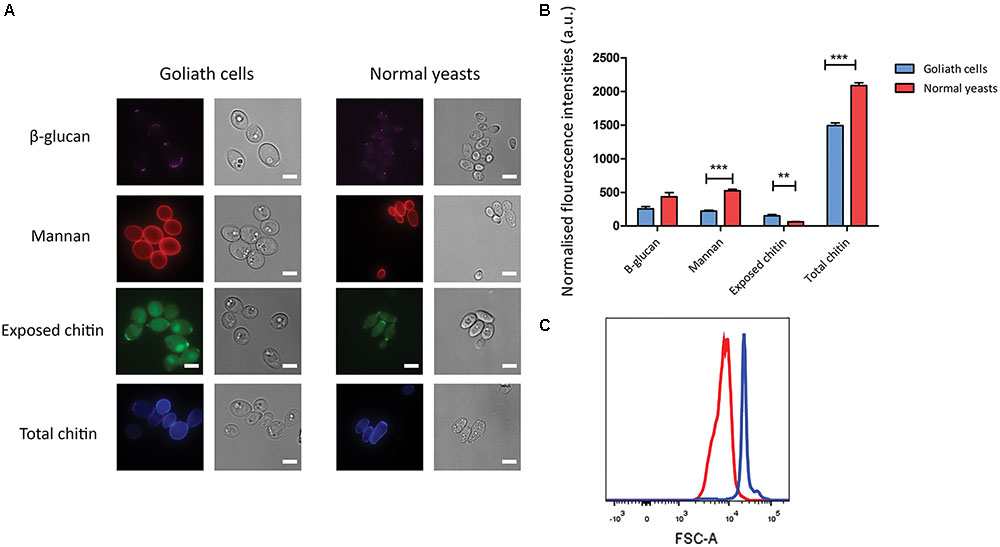
FIGURE 7. Cell wall analysis of Goliath cells. (A) Goliath cells stained for exposed β-glucan (Fc-Dectin), mannan (concanavalin A), exposed (WGA) and total (Calcofluor White) chitin and compared with normal yeast cells. Scale bar represents 5 μm. (B) Mean fluorescence intensities of respective cell wall components for Goliath cells and normal yeast cells as determined by flow cytometry represented as a bar graph. Data were obtained from three independent experiments and normalized by cell size (C), error bars represent standard deviation and p-values were calculated by t-test. A significant increase in mean fluorescence intensity of WGA was observed Goliath cells as compared to normal yeast cells. (∗P ≤ 0.05, ∗∗P ≤ 0.01, ∗∗∗P ≤ 0.0001). (C) Increase in cell size determined using flow cytometry by measuring forward scatter (FSC) intensities of Goliath cells (blue) and normal yeast cells (red) (n > 10000). A shift in FCS peak shows that Goliath cells are significantly larger than normal yeast cells. Mean FCS intensities were compared to determine fold increase in cell size. Based on the FCS intensities, C. albicans Goliath cells exhibit a 3.3-fold increase in size as compared to normal yeasts. Note that relative to cell size, only chitin exposure is relative higher for Goliath cells vs. control yeasts.
Flow cytometry measurements indicated increased mannan (1.4-fold), β-glucan (2-fold) and total chitin (3.8-fold) levels in Goliath cell walls compared to control yeast cells. However, the defining feature of Goliath cells is their size. In agreement with our microscopic measurements (Figure 2A), forward light scatter flow cytometry measurements (a proxy for particle size) of Goliath cells were increased by 3.3-fold compared to control yeasts (Figure 7C). This suggested that apparently increased mannan, β-glucan and total chitin may simply be due to the increased surface area of Goliath cells. Indeed microscopy revealed no obvious differences in Concanavalin A, FC-dectin or Calcofluor White fluorescence intensity between cell types (Figure 7A). Therefore, whilst Goliath cells may contain more chitin molecules per cell, this is unlikely to reflect an increased concentration of these molecules in the cell wall. Indeed, for mannan (1.4-fold increase) and β-glucan (2-fold increase), relative levels of these cell wall components in Goliath cell wall may be even lower than control yeasts. In contrast, chitin exposure on Goliath cells was 8-fold higher as determined by flow cytometry and when fluorescence was normalized to cell size, exposed chitin was the only component found to be higher on Goliath cells (Figure 7B). Moreover, fluorescence microscopy revealed an increase in the number and intensity of FITC-WGA positive puncta on the Goliath cell wall (Figure 7A). Therefore, Goliath cells do exhibit altered cell wall composition, associated with increased chitin exposure that occur in a non-uniform and punctate pattern across the cell wall.
C. albicans Goliath Cells Show an Increased Adhesion Capacity
The capacity of C. albicans to adhere to both biotic and abiotic surfaces is a key pathogenicity mechanism and is also likely important for commensal colonization. However, under standard laboratory growth conditions, yeast cells adhere poorly compared to hyphae (Wachtler et al., 2011). As Goliath cells exhibited cell wall alterations (Figure 7) and have an increased surface area compared to control yeasts, we reasoned that Goliath cells may exhibit altered adhesive properties.
We tested the capacity of both Goliath cells (LZM preculture) and control yeasts (LZM + Z preculture) to adhere to polystyrene surfaces in the presence of either LZM or in LZM + Z (Figures 8A,B).
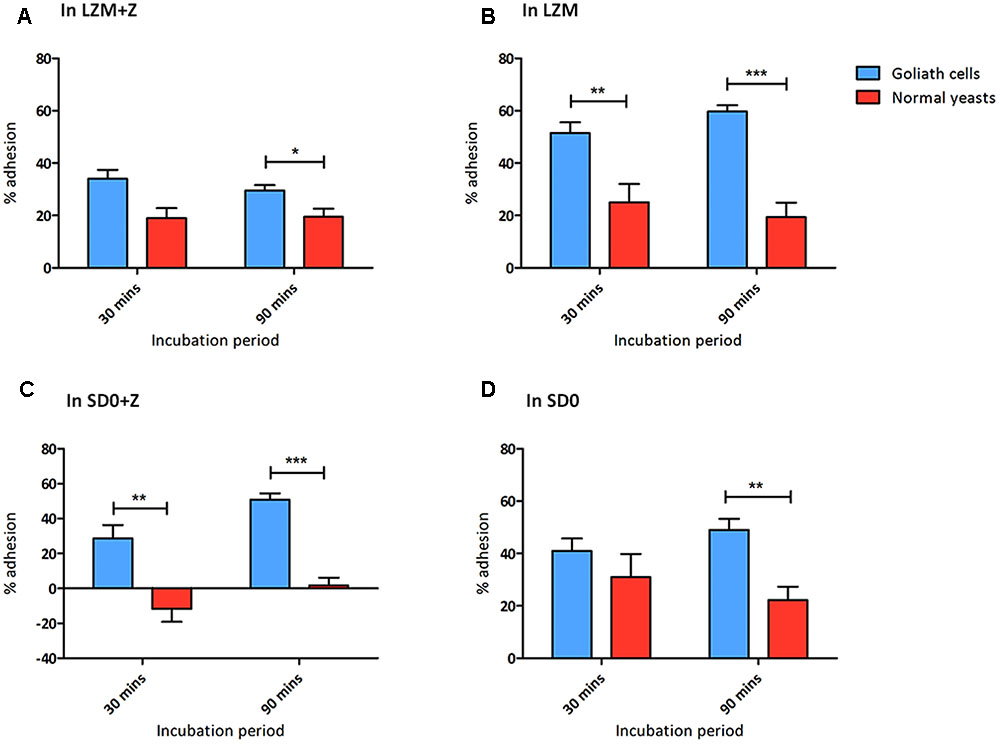
FIGURE 8. Goliath cells exhibit increased adhesion to plastic surfaces. Adherence of C. albicans Goliath cells formed in LZM (A,B) or in SD0 (C,D) to plastic in the presence (A,C) or absence (B,D) of zinc in the adhesion assay medium. Relevant control yeast cells formed in LZM + Z or SD0 + Z. Following incubation for 30 or 90 min, wells were washed three times with ultrapure water. CFUs in the initial supernatant and in all three wash steps were determined to calculate % adhesion. Error bars represent standard deviation and p-values were determined by t-test. Data are from three independent experiments (A,B); and two independent experiments (C,D). Goliath cells are more adherent than normal yeast cells in all the tested media type at both the time points. The adhesion capacity of Goliath cells is reduced upon incubation in LZM + Z (A vs. B). However, the presence of zinc during adhesion had a less pronounced effect on Goliath cell adhesion in SD0 + Z (C vs. D). Normal yeast cells show increased adhesion when incubated in the absence of zinc (D vs. C) (∗P ≤ 0.05, ∗∗P ≤ 0.01, ∗∗∗P ≤ 0.0001).
When assayed in either LZM + Z or LZM, Goliath cells adhered significantly better than control yeasts and this effect was far more pronounced when zinc was omitted from the assay medium (LZM). In fact, in LZM, Goliath cells reached 51.5 and 59.5% adhesion by 30 and 90 min, respectively.
We considered even 20% adhesion of control yeast cells in these assays surprising. In our experience C. albicans yeast cells do not adhere until they form hyphal germ tubes (Wilson and Hube, 2010; Wachtler et al., 2012) and in the current assay, all cells remained as either Goliath or yeast cells. The zinc-depletion medium used here (LZM/LZM + Z) contains 1 mM EDTA and only 0.2% glucose, which may already be stressful for C. albicans, even with zinc supplementation. It may be that LZM + Z control yeast cells themselves are already somewhat nutritionally stressed and it has previously been shown that altered carbon source, for example, can affect adhesion (Ene et al., 2012).
We therefore repeated the adhesion experiment using SD0 as the basal medium. When assayed for adhesion in SD0 + Z, control yeast cells did not adhere at all. In contrast, Goliath cells exhibited robust adhesion –41 and 50.5% by 30 and 90 min, respectively (Figure 8C). Interestingly, when we performed the adhesion assay itself in the absence of zinc (SD0), both Goliath and control yeast cells exhibited robust adhesion with Goliath cells being almost twice as adherent as control yeasts after 90 min.
In summary, Goliath cells are highly adherent to a polystyrene surface and, in SD0 basal medium, adhesion of control yeast cells is stimulated by short term exposure to zinc depletion (Figures 8C,D). Therefore both cellular and environmental zinc levels can significantly influence C. albicans adhesion.
Impact of Pra1 on the Goliath Phenotype
We have previously demonstrated a role for Pra1 in fungal adaption to zinc restriction at neutral pH (Citiulo et al., 2012; Wilson, 2015). However, the zinc limited media used in this study is acidic (∼pH 4.6–4.8) and, although responsive to zinc (Citiulo et al., 2012), it was unclear whether PRA1 would be expressed in acidic environments (Sentandreu et al., 1998; Bensen et al., 2004). We therefore incubated cells harboring either empty vector (negative control), PPRA1-GFP, or PACT1-GFP (positive control) in LZM and assessed GFP expression. Interestingly, at each time point analyzed, the majority of the PPRA1-GFP cell population expressed GFP at levels similar to the PACT1-GFP control (Figure 9A). Therefore, in addition to being a pH-regulated antigen, PRA1 can also be expressed at acidic pH when environmental zinc highly restricted.
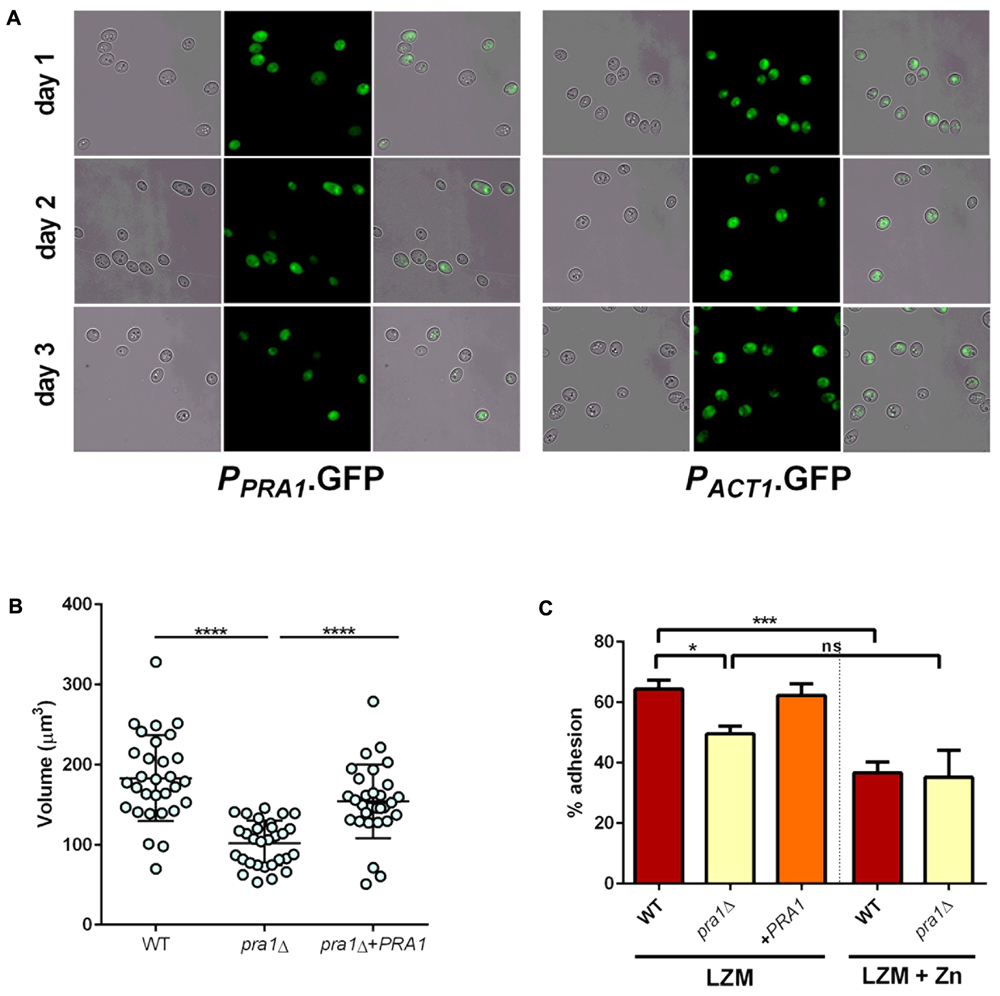
FIGURE 9. Pra1 contributes to enlargement and adhesion properties of Goliath cells. (A) PRA1 is expressed in limited zinc medium (LZM). Cells harboring PPRA1-GFP (Citiulo et al., 2012) or PACT1-GFP were analyzed at indicated time points. (B) C. albicans cells were cultured for 2 days in LZM and cell volumes were calculated by V = 4/3 π ab2. Data in each column represent at least 30 independent measurements from three independent experiments. pra1Δ cells were significantly smaller than wild type and pra1Δ+PRA1 cells (P < 0.0001, one-way ANOVA). (C) Adherence of indicated C. albicans cells cultured for 2 days in LZM to induce cellular enlargement or for 1 day in LZM + Z to generate yeast cells. Wild type, but not pra1Δ exhibited enhanced adhesion following incubation in LZM (∗P < 0.05, ∗∗P < 0.01, ∗∗∗P < 0.001, ∗∗∗∗ P < 0.0001).
Having established expression, we next tested whether deletion of PRA1 affected Goliath cell formation itself. Isogenic wild type, pra1Δ and pra1Δ+PRA1 cells were incubated and cell size determined as before. Although, pra1Δ cells did enlarge upon zinc restriction (reaching a mean cell volume of 102 μm3), these cells were significantly smaller than wild type Goliath cells (183 μm3) and complementation with a single copy of PRA1 significantly restored cell size to 154 μm3 (Figure 9B). Therefore, although not essential for cellular enlargement in response to zinc restriction (the volume of regular yeast cells is approximately 30 μm3), Pra1 does positively contribute to Goliath cell formation.
Given the observed defect in enlargement of pra1Δ, we next tested the impact of this on adhesion. Cells were cultured in LZM to induce enlargement and adhesion again assessed in the presence of LZM. Interestingly, PRA1 copy number strongly correlated with both cell size and adhesion, with wild type (PRA1/PRA1), pra1Δ and pra1Δ+PRA1 strains exhibiting 64, 49.6, and 62.3%, respectively (Figure 9C). In fact, the R2-value of the correlation between cell volume and adhesion potential was 0.948 (based on a linear equation of normal yeast, and enlarged cells of pra1Δ, pra1Δ+PRA1, wild type adhesion over volume). These data indicate that the size of Goliath cells themselves contributes to their increased adhesion.
The Effect of Environmental pH and Temperature on Goliath Cell Formation
Candida albicans morphogenesis is exquisitely sensitive to the cells’ ambient environment. In particular, temperature and pH are important morphogenic cues: low temperature and acidic pH promote the yeast morphology; whilst physiological temperature (37°C) and neutral-to mildly alkaline pH promote the hyphal morphology. We therefore tested the effect of zinc-, pH-, and temperature on C. albicans morphology. At 30°C, alkalinizing the medium to pH 7.3 had no obvious impact on C. albicans yeast morphology in zinc replete conditions (Figures 10A,B). In contrast, in the absence of zinc, cells initially enlarged, and then began to filament (Figure 10B). At these later time points, very little filamentation was observed in the presence of zinc (not shown).
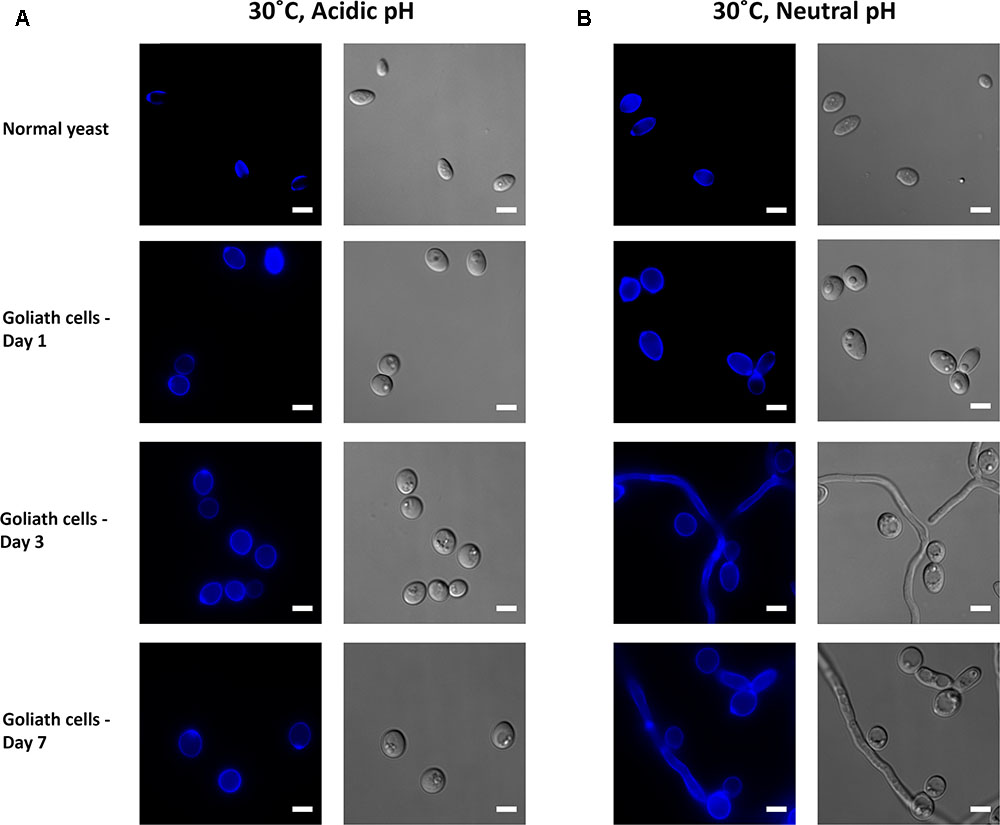
FIGURE 10. Impact of pH on Goliath morphology. C. albicans cells were incubated in limited zinc media (LZM) at 30°C at acidic (pH 4.6) or neutral-alkaline (pH 7.3) and morphology assessed. (A) In acidic medium zinc limitation induces cellular enlargement. (B) At pH 7.3 zinc limitation induces yeast cell enlargement and triggers filamentation. Experiment was performed twice.
In zinc replete acidic medium at 37°C, C. albicans cells grew as slightly elongated yeasts (Figure 11A). Under zinc limitation, Goliath cell formation was even more pronounced at 37°C than at 30°C (compare Figures 10A, 11A) and some filamentation was observed, however, invaginations at the septa indicated that these cells were mainly pseudohyphae. Therefore physiological temperature (37°C) stimulates Goliath cell formation. Finally in neutral medium at 37°C, all cells formed hyphae independent of zinc status (Figure 11B). This was unsurprising as pH 7.3, together with 37°C is a strong inducer of hypha formation (Sudbery, 2011).
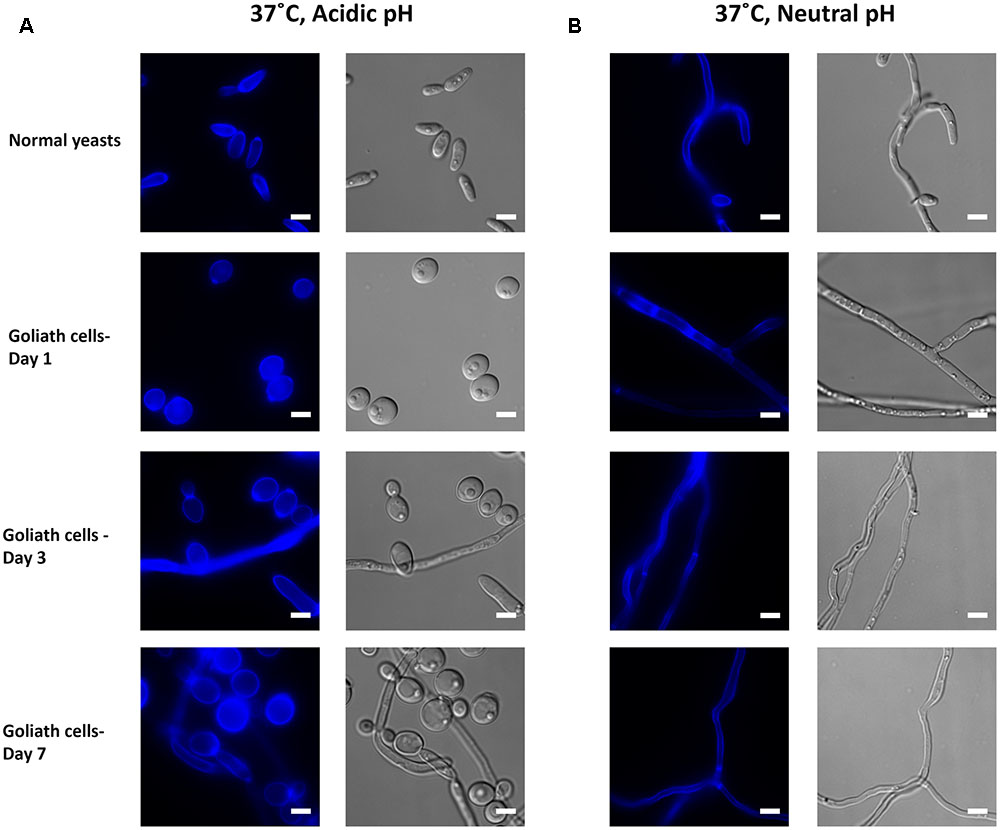
FIGURE 11. Impact of temperature on Goliath morphology. C. albicans cells were incubated in limited zinc media (LZM) at 37°C at acidic (pH 4.6) or neutral-alkaline (pH 7.3) and morphology assessed. (A) In acidic medium at 37°C zinc limitation induces a greater degree of cellular enlargement than at 30°C (compare Figure 10A) and pseudohypha development. (B) At pH 7.3 and 37°C filamentation occurs independent of media zinc status. Experiment was performed twice.
In summary environmental zinc restriction is a novel trigger for C. albicans adhesion, associated with a notable increase in cell size.
Discussion
Candida albicans polymorphism is one of the most widely recognized attributes of this important human fungal pathogen (Lu et al., 2014; Jacobsen and Hube, 2017; Noble et al., 2017). The most well studied morphologies are yeast and hyphae and the yeast to hypha dimorphic transition is thought to be a key virulence factor. This is because multiple virulence-associated genes which encode factors required for adhesion (e.g., Hwp1, Als3), invasion (Als3) and damage (Ece1) are hardwired into the hyphal morphogenetic program (Martin et al., 2013). Whilst hyphae are considered the dominant invasive morphology, yeast cells are thought to be important for commensal colonization, dissemination in the bloodstream and reseeding from biofilms during recurrent candidaemia (Kullberg and Arendrup, 2015). The role of other morphologies in commensalism and disease, including pseudohyphae and chlamydospores, are not as well understood. In addition to these morphological transitions, yeast cells can also undergo phenotypic switching. The most well studied of these is the white-opaque switch, which is important for mating in C. albicans (Hickman et al., 2013). More recently, Pande et al. (2013) described a novel yeast phenotype. The authors found that gastrointestinal passage of a strain over-expressing the transcription factor Wor1 resulted in a switch to an elongated yeast cell morphology. These GUT cells (gastrointestinal induced transition), which were distinct from the opaque phenotype, had increased gastrointestinal (GI) tract fitness and differentially regulated genes involved in iron acquisition, glucose metabolism and phosphate uptake.
Here we describe and characterize another C. albicans phenotype: the Goliath cell. This form of cellular enlargement occurred in response to zinc limitation, but not to iron-, manganese- or copper- restriction. The group of Soll has also observed C. albicans cellular enlargement in response to zinc limitation (Bedell and Soll, 1979) and this, combined with our own observations that all C. albicans clinical isolates tested underwent this transition, strongly suggests that this is a conserved morphological response in C. albicans. Enlarged yeast cells have been observed under other laboratory conditions such as during stationary phase growth of a genetic mutant lacking ECM33 (Gil-Bona et al., 2016), on IsoVitalex-supplemented chocolate agar (Bottone et al., 1999) or in response to xylitol (Ameglio et al., 1990). Importantly, enlarged yeast cells have also been observed from a patient suffering from systemic candidiasis (Alasio et al., 2003).
Zinc limitation is relevant to both Candida commensal colonization and pathogenicity. In the GI tract, active inflammation results in high levels of calprotectin in the gastrointestinal lumen. In fact, calprotectin is amongst the best studied markers of gastrointestinal inflammation (Walsham and Sherwood, 2016). Calprotectin is a heterodimeric protein composed of S100A8 and A9 subunits and has potent antimicrobial activity (Urban et al., 2009). Its antifungal activity is mediated by zinc chelation and calprotectin has high affinity for this metal, with a dissociation constant in the low nanomolar range (Kehl-Fie et al., 2011). However, C. albicans also produces a zinc binding (“zincophore”) protein, encoded by PRA1 (Citiulo et al., 2012). Like host calprotectin, Pra1 has low nanomolar affinity for zinc (Loboda and Rowinska-Zyrek, 2017), and this zincophore allows the fungus to scavenge for zinc from host tissue. Here we demonstrate a role for Pra1 in Goliath cell enlargement and adhesive potential. Therefore, in the context of its evolutionary history as GI tract commensal, C. albicans likely experiences zinc restriction during gastrointestinal inflammation. Superficial mucosal infections, such as vulvovaginal candidiasis, can also be associated with calprotectin and neutrophil influx (Yano et al., 2012). Moreover, when C. albicans enters normally sterile site, such as the bloodstream, it will likely face extreme zinc limitation due to the coordinate action of nutritional immunity (Crawford and Wilson, 2015).
Beyond their size, we observed three major Goliath cell phenotypes: (i) hyper-adherence, (ii) delayed hyphal morphogenesis and (iii) increased chitin exposure. (i) The high adhesive potential of Goliath cells was unexpected. In our experience (Wilson and Hube, 2010; Wachtler et al., 2011), C. albicans yeast cells adhere very poorly to both biotic and abiotic surfaces under laboratory conditions. At this stage, the molecular mechanism of zinc depletion-induced adhesion is unclear, but may involve Zap1. This transcription factor is responsive to changes in zinc availability and is the main regulator of zinc homeostasis in fungi (Ballou and Wilson, 2016; Wilson and Bird, 2016). Interestingly, as well as regulating genes involved in zinc assimilation and homeostasis, Zap1 in C. albicans also controls the expression of the Cell surface targets of adherence regulators (CSTAR) genes, a regulon composed of multiple putative and described adhesin genes (Nobile et al., 2009; Finkel et al., 2012). It is therefore likely that the increased adhesion phenotype of Goliath cells is the result of zinc-depletion activation of Zap1. Indeed, we observed that deletion of PRA1 (a major Zap1 target) inhibited both cellular enlargement and adhesion. Although Pra1 has been implicated in the binding of C. albicans to host cells and various immune mediators (Soloviev et al., 2007; Zipfel et al., 2011), we think it is unlikely that Pra1 is directly functioning as an adhesin on C. albicans Goliath cells. It is possible that impaired zinc homeostasis in the pra1Δ mutant inhibits cellular enlargement, and that it is simply this enlargement defect which results in reduced adhesion of the pra1Δ mutant. Indeed, the observed correlation between cell size and adhesive potential indicates that the larger surface area of Goliath cells may contribute to adhesion by providing a larger point of contact between the fungus and the substratum.
(ii) The hyphae-inducing medium used in this study (RPMI, 37°C, 5% CO2) is a potent inducer of hyphal morphogenesis, and yet Goliath cells exhibited a lengthy delay before the initiation of germ tube formation in this medium. Therefore, compared to normal yeast cells, Goliath cells appear to be less primed to form hyphae. In natural environments, C. albicans faces more complex environments, and its morphological output will depend on the integration of these multiple stimuli (Sudbery, 2011). As hypha formation may represent a commitment to invasive growth (Mech et al., 2014), and is intimately associated with host cell damage (Moyes et al., 2016), reduced hypha formation on host surfaces may be associated with reduced damage and immune activation. On the other hand, under conditions of elevated pH and low temperature, zinc restriction promoted filamentation. It would therefore appear that environmental zinc is set to take its place amongst the plethora of environmental cues which influence morphogenesis in C. albicans (Sudbery, 2011).
(iii) Finally, of the cell wall components tested in this study, only chitin exposure was increased relative to cell size on the Goliath cell wall. This observation may have implications for immune interactions (Wagener et al., 2014). As well as providing structural stability to the cell wall (Gow and Hube, 2012; Gow et al., 2017), chitin can also be recognized by the host immune system. Depending on the size of chitin biomolecule, it has been shown to trigger either a pro-inflammatory or an anti-inflammatory response. Mora-Montes et al. (2011) showed that ultrapure chitin from the cell wall of C. albicans failed to elicit a cytokine response in human peripheral blood monocytes (PBMCs) and that PBMCs pre-incubated with ultrapure chitin did not readily recognize live C. albicans cells, causing a significant reduction in cytokine production.
Together, these three traits may facilitate a non-damaging “persister” phenotype: the capacity of C. albicans Goliath cells to colonize host surfaces via adhesion, whist presenting increased chitin and greatly diminished hypha formation may allow the cells to avoid triggering damage and inflammation. We are now investigating the impact of this morphotype on host pathogen interactions and immune evasion.
Intriguingly, from an evolutionary perspective, cellular enlargement in response to zinc limitation was observed for C. albicans, C. dubliniensis, and C. tropicalis: the three most closely related commonly studied Candida species (Butler et al., 2009). Although all three species are common pathogens of humans, it is unlikely that this cellular response originated within the context of colonization of humans. The age of the CUG-Ser clade (to which these three species belong) has been estimated at 170 million years (Massey et al., 2003). The two more closely related species, C. albicans and C. dubliniensis appear to have diverged 20 million years ago (McManus and Coleman, 2014). It is unclear when the more distantly related C. tropicalis diverged from their common ancestor: based on these divergence estimates and branch length reported in Butler et al. (2009), this may have occurred between approximately 57 and 70 million years ago. Therefore, it would appear that cellular enlargement in response to zinc limitation has been maintained for tens of millions of years and remains a morphological property of at least three medically important extant species.
Materials and Methods
Strains and Growth Conditions
Strains (C. albicans clinical isolates and other Candida species) used in this study are listed in Supplementary Table S1. The isogenic wild type C. albicans strain (BWP17+CIp30) was used for most experiments.
All strains were maintained on YPD agar (1% yeast extract 2% mycological peptone, 2% D-glucose and 2% agar). Liquid overnight cultures were grown in SD (2% glucose, 2% YNB, 0.5% NH4SO4) medium in a shaking incubator at 30°C and 200 rpm.
Metal Limitation
To elicit acute zinc limitation, cells were precultured in SD media, washed three times with ultrapure water and inoculated at an OD600 0.2 in 4 ml LZM (media composition listed in Supplementary Table S2) in a universal tube. Tubes were incubated at 30°C, 200 rpm for 3 days. As an alternative method of zinc starvation, SD pre-grown cells were washed and inoculated at an OD600 0.05 in 4 ml SD0 (2% glucose without zinc, 2% YNB without zinc, Formedium). Tubes were incubated similarly for 7 days. Control cultures were supplemented with 25 μM ZnSO4 (LZM + Z/SD0 + Z) and incubated for 1 day.
To monitor increases in cell size, C. albicans (WT) Goliath cells (LZM/SD0) and normal yeasts (LZM + Z/SD0 + Z) were imaged and optical densities were measured at OD600. Imaged cells were analyzed using ImageJ v1.47and cell volume determined using the formula V = 4/3 π ab2 where a is the radius of the major axis and b is the radius of the minor axis of the cell.
The effect of zinc limitation was tested on C. albicans clinical isolates and Candida species listed in Supplementary Table S1 by incubating SD precultured cells in LZM and/or LZM + Z as described above. Following incubation, cells were imaged using Deltavision-RT at 100× magnification.
To induce iron, copper and manganese limitation, WT C. albicans cells precultured in SD media were washed and inoculated at an OD600 0.2 in 4 ml LIM, LCM, and LMM (media composition in Supplementary Table S2) in a universal tube. Tubes were incubated at 30°C, 200 rpm for 3 days. Control cultures were supplemented with FeSO4, CuSO4, and MnSO4, respectively, as described in Supplementary Table S2 and incubated for 1 day.
Hypha Induction
To assess the capacity of Goliath cells to form hyphae, C. albicans WT cells cultured for 3 days in LZM and normal yeasts cultured in LZM + Z for 1 day were washed three times with ultrapure water and 105 cells were inoculated in RPMI 1640 (Gibco) in a 12 well plate. Plates were incubated at 37°C and 5% CO2 for 90, 180, or 360 min, fixed and stained with stained with Calcofluor White. Cells were imaged using DeltaVision at 40× magnification.
Cell Wall Staining
To assess the effect of C. albicans Goliath cells exposure to zinc, WT cells incubated for 2 days in LZM were washed 3× with ultrapure water and stained with 10 μg/ml FITC in carbonate-bicarbonate buffer (pH 9.6) for 20 min in the dark at room temperature. Stained cells were washed with ultrapure water and inoculated in SD media at an OD600 0.2 in a universal tube. Tubes were incubated for 16 h as previously described. Following incubation cells were washed and fixed in Histofix 4% (Roth®) for 45 min at 30°C. Fixed cells were washed and imaged using FITC filter channel in Deltavision-RT at 100× magnification.
To determine potential differences in cell wall composition, 2.5 × 106 Goliath cells (cultured in LZM for 3 days) and normal yeasts (grown for 1 day in LZM + Z) were fixed and washed three times with ultrapure water. Fixed cells were stained independently with 25 μg/ml Calcofluor White (CFW), 100 μg/ml wheat germ agglutinin (WGA), 50 μg/ml concanavalin A (ConA) or 5 μg/ml FC-Dectin. Cells were incubated for 45 min to 1 h at room temperature in the dark. Following incubation, cells were washed twice with PBS containing 1% fetal calf serum and 0.5 mM EDTA. Stained cells were analyzed using flow cytometry and fluorescence microscopy. For imaging, cells were analyzed using appropriate filter channels (DAPI, Rh-TRITC, and FITC) in DeltaVision-RT at 100× magnification. Flow cytometry data was obtained using BD Fortessa and mean fluorescence intensities were measured using Alexa 350 and Ds-Red channels. Forward and side scatter were measured to determine difference in cell size.
Adhesion Assay
Candida albicans WT cells (105 cells/ml) pre-grown in LZM for 2 days and LZM + Z for 1 day were further incubated in LZM and LZM + Z in a 12 well FalconTM plate at 30°C for 0, 30, and 90 min. Following incubation, supernatant from wells was collected and wells were washed three times with sterile ultrapure water. Washes from each well were also collected. Serial dilutions were prepared for supernatant and washes that were then plated on to YPD agar. CFUs obtained following incubation were counted to determine the percentage of detached and adherent cells at each time-points. This protocol was repeated with WT C. albicans cells pre-grown in SD0 for 7 days and SD0 + Z for 1 day.
Statistical Analysis
All data were obtained from at least two independent experiments. At least 100 cells (and 35 cells for day 0) were measured for determination of cell size over three independent experiments. Statistical analysis was performed on GraphPad Prism5 using Students t-test and ANOVA as appropriate.
Author Contributions
DW, BH, and NG conceived the study. DM, LL-M, OA, EW, and DW acquired, analyzed, and interpreted the data. DM and DW drafted the manuscript. LL-M, OA, EW, NG, and BH critically revised the manuscript. All authors approved the final submitted version and agree to accountability for all aspects of the work.
Funding
DW is supported by a Sir Henry Dale Fellowship jointly funded by the Wellcome Trust and the Royal Society (102549/Z/13/Z), a Wellcome Trust ISSF seed corn grant (RG12723 14), and the Medical Research Council and University of Aberdeen (MR/N006364/1). DW and DM received support from a Wellcome Trust Strategic Award for Medical Mycology and Fungal Immunology (097377/Z/11/Z). NG is supported by a Wellcome Trust Investigator Award (101873). BH is supported by the Deutsche Forschungsgemeinschaft DFG SFB/TR 124 FungiNet, project C1. LL-M is supported by a Royal Society Dorothy Hodgkin Fellowship (DH150187). OA is supported by the Public Authority of Applied Education and Training, Kuwait.
Conflict of Interest Statement
The authors declare that the research was conducted in the absence of any commercial or financial relationships that could be construed as a potential conflict of interest.
Acknowledgments
The cytometry data presented herein were obtained at the Iain Fraser Cytometry Centre (IFCC) at the University of Aberdeen. We thank IFCC for providing the flow cytometry service and the Microscopy and Histology core facility for assistance with microscopy.
Supplementary Material
The Supplementary Material for this article can be found online at: https://www.frontiersin.org/articles/10.3389/fmicb.2017.02238/full#supplementary-material
References
Alasio, T. M., Lento, P. A., and Bottone, E. J. (2003). Giant blastoconidia of Candida albicans. A case report and review of the literature. Arch. Pathol. Lab. Med. 127, 868–871.
Ameglio, F., Di Giorgio, C., Terzaroli, P., and Gandolfo, G. M. (1990). Giant cell production by C. albicans cultured in xylitol. Microbiologica 13, 343–346.
Amich, J., Vicentefranqueira, R., Mellado, E., Ruiz-Carmuega, A., Leal, F., and Calera, J. A. (2014). The ZrfC alkaline zinc transporter is required for Aspergillus fumigatus virulence and its growth in the presence of the Zn/Mn-chelating protein calprotectin. Cell Microbiol. 16, 548–564. doi: 10.1111/cmi.12238
Andreini, C., Banci, L., Bertini, I., and Rosato, A. (2006). Zinc through the three domains of life. J. Proteome Res. 5, 3173–3178. doi: 10.1021/pr0603699
Andreini, C., Bertini, I., and Rosato, A. (2009). Metalloproteomes: a bioinformatic approach. Acc. Chem. Res. 42, 1471–1479. doi: 10.1021/ar900015x
Anzaldi, L. L., and Skaar, E. P. (2010). Overcoming the heme paradox: heme toxicity and tolerance in bacterial pathogens. Infect. Immun. 78, 4977–4989. doi: 10.1128/IAI.00613-10
Ballou, E. R., and Wilson, D. (2016). The roles of zinc and copper sensing in fungal pathogenesis. Curr. Opin. Microbiol. 32, 128–134. doi: 10.1016/j.mib.2016.05.013
Bedell, G. W., and Soll, D. R. (1979). Effects of low concentrations of zinc on the growth and dimorphism of Candida-Albicans - evidence for zinc-resistant and zinc-sensitive pathways for mycelium formation. Infect. Immun. 26, 348–354.
Bensen, E. S., Martin, S. J., Li, M., Berman, J., and Davis, D. A. (2004). Transcriptional profiling in Candida albicans reveals new adaptive responses to extracellular pH and functions for Rim101p. Mol. Microbiol. 54, 1335–1351. doi: 10.1111/j.1365-2958.2004.04350.x
Bottone, E. J., Horga, M., and Abrams, J. (1999). Giant blastoconidia of Candida albicans: morphologic presentation and concepts regarding their production. Diagn. Microbiol. Infect. Dis. 34, 27–32. doi: 10.1016/S0732-8893(99)00013-9
Boyce, K. J., and Andrianopoulos, A. (2015). Fungal dimorphism: the switch from hyphae to yeast is a specialized morphogenetic adaptation allowing colonization of a host. FEMS Microbiol. Rev. 39, 797–811. doi: 10.1093/femsre/fuv035
Butler, G., Rasmussen, M. D., Lin, M. F., Santos, M. A., Sakthikumar, S., Munro, C. A., et al. (2009). Evolution of pathogenicity and sexual reproduction in eight Candida genomes. Nature 459, 657–662. doi: 10.1038/nature08064
Cassat, J. E., and Skaar, E. P. (2012). Metal ion acquisition in Staphylococcus aureus: overcoming nutritional immunity. Semin. Immunopathol. 34, 215–235. doi: 10.1007/s00281-011-0294-4
Citiulo, F., Jacobsen, I. D., Miramon, P., Schild, L., Brunke, S., Zipfel, P., et al. (2012). Candida albicans scavenges host zinc via Pra1 during endothelial invasion. PLOS Pathog. 8:e1002777. doi: 10.1371/journal.ppat.1002777
Crawford, A., and Wilson, D. (2015). Essential metals at the host-pathogen interface: nutritional immunity and micronutrient assimilation by human fungal pathogens. FEMS Yeast Res. 15:fov071. doi: 10.1093/femsyr/fov071
Dade, J., DuBois, J. C., Pasula, R., Donnell, A. M., Caruso, J. A., Smulian, A. G., et al. (2016). HcZrt2, a zinc responsive gene, is indispensable for the survival of Histoplasma capsulatum in vivo. Med. Mycol. 54, 865–875. doi: 10.1093/mmy/myw045
Do, E., Hu, G., Caza, M., Kronstad, J. W., and Jung, W. H. (2016). The ZIP family zinc transporters support the virulence of Cryptococcus neoformans. Med. Mycol. 54, 605–615. doi: 10.1093/mmy/myw013
Ene, I. V., Heilmann, C. J., Sorgo, A. G., Walker, L. A., de Koster, C. G., Munro, C. A., et al. (2012). Carbon source-induced reprogramming of the cell wall proteome and secretome modulates the adherence and drug resistance of the fungal pathogen Candida albicans. Proteomics 12, 3164–3179. doi: 10.1002/pmic.201200228
Enjalbert, B., and Whiteway, M. (2005). Release from quorum-sensing molecules triggers hyphal formation during Candida albicans resumption of growth. Eukaryot. Cell 4, 1203–1210. doi: 10.1128/EC.4.7.1203-1210.2005
Finkel, J. S., Xu, W., Huang, D., Hill, E. M., Desai, J. V., Woolford, C. A., et al. (2012). Portrait of Candida albicans adherence regulators. PLOS Pathog. 8:e1002525. doi: 10.1371/journal.ppat.1002525
Gil-Bona, A., Reales-Calderon, J. A., Parra-Giraldo, C. M., Martinez-Lopez, R., Monteoliva, L., and Gil, C. (2016). The cell wall protein Ecm33 of Candida albicans is involved in chronological life span, morphogenesis, cell wall regeneration, stress tolerance, and host-cell interaction. Front. Microbiol. 7:64. doi: 10.3389/fmicb.2016.00064
Gow, N. A., and Hube, B. (2012). Importance of the Candida albicans cell wall during commensalism and infection. Curr. Opin. Microbiol. 15, 406–412. doi: 10.1016/j.mib.2012.04.005
Gow, N. A. R., Latge, J. P., and Munro, C. A. (2017). The fungal cell wall: structure, biosynthesis, and function. Microbiol. Spectr. 5, 1–25. doi: 10.1128/microbiolspec.FUNK-0035-2016
Heilmann, C. J., Sorgo, A. G., Siliakus, A. R., Dekker, H. L., Brul, S., de Koster, C. G., et al. (2011). Hyphal induction in the human fungal pathogen Candida albicans reveals a characteristic wall protein profile. Microbiology 157(Pt 8), 2297–2307. doi: 10.1099/mic.0.049395-0
Hickman, M. A., Zeng, G., Forche, A., Hirakawa, M. P., Abbey, D., Harrison, B. D., et al. (2013). The ’obligate diploid’ Candida albicans forms mating-competent haploids. Nature 494, 55–59. doi: 10.1038/nature11865
Hood, M. I., and Skaar, E. P. (2012). Nutritional immunity: transition metals at the pathogen-host interface. Nat. Rev. Microbiol. 10, 525–537. doi: 10.1038/nrmicro2836
Jacobsen, I. D., and Hube, B. (2017). Candida albicans morphology: still in focus. Expert Rev. Anti Infect. Ther. 15, 327–330. doi: 10.1080/14787210.2017.1290524
Jung, W. H. (2015). The zinc transport systems and their regulation in pathogenic fungi. Mycobiology 43, 179–183. doi: 10.5941/MYCO.2015.43.3.179
Kane, J. (1984). Conversion of blastomyces-dermatitidis to the yeast form at 37-degrees-C and 26-degrees-C. J. Clin. Microbiol. 20, 594–596.
Kehl-Fie, T. E., Chitayat, S., Hood, M. I., Damo, S., Restrepo, N., Garcia, C., et al. (2011). Nutrient metal sequestration by calprotectin inhibits bacterial superoxide defense, enhancing neutrophil killing of Staphylococcus aureus. Cell Host Microbe 10, 158–164. doi: 10.1016/j.chom.2011.07.004
Kehl-Fie, T. E., and Skaar, E. P. (2010). Nutritional immunity beyond iron: a role for manganese and zinc. Curr. Opin. Chem. Biol. 14, 218–224. doi: 10.1016/j.cbpa.2009.11.008
Klis, F. M., De Groot, P., and Hellingwerf, K. (2001). Molecular organization of the cell wall of Candida albicans. Med. Mycol. 39, 1–8. doi: 10.1080/mmy.39.1.1.8-0
Kullberg, B. J., and Arendrup, M. C. (2015). Invasive Candidiasis. N. Engl. J. Med. 373, 1445–1456. doi: 10.1056/NEJMra1315399
Liu, H. (2001). Transcriptional control of dimorphism in Candida albicans. Curr. Opin. Microbiol. 4, 728–735. doi: 10.1016/S1369-5274(01)00275-2
Loboda, D., and Rowinska-Zyrek, M. (2017). Zinc binding sites in Pra1, a zincophore from Candida albicans. Dalton Trans. 46, 13695–13703. doi: 10.1039/c7dt01675a
Lu, Y., Su, C., and Liu, H. P. (2014). Candida albicans hyphal initiation and elongation. Trends Microbiol. 22, 707–714. doi: 10.1016/j.tim.2014.09.001
MacCallum, D. M., Castillo, L., Nather, K., Munro, C. A., Brown, A. J. P., Gow, N. A., et al. (2009). Property differences among the four major Candida albicans strain clades. Eukaryot. Cell 8, 373–387. doi: 10.1128/EC.00387-08
Maresca, B., and Kobayashi, G. S. (1989). Dimorphism in histoplasma-capsulatum - a model for the study of cell-differentiation in pathogenic fungi. Microbiol. Rev. 53, 186–209.
Martin, R., Albrecht-Eckardt, D., Brunke, S., Hube, B., Hunniger, K., and Kurzai, O. (2013). A core filamentation response network in Candida albicans is restricted to eight genes. PLOS ONE 8:e58613. doi: 10.1371/journal.pone.0058613
Massey, S. E., Moura, G., Beltrao, P., Almeida, R., Garey, J. R., and Tuite, M. F. (2003). Comparative evolutionary genomics unveils the molecular mechanism of reassignment of the CTG codon in Candida spp. Genome Res. 13, 544–557. doi: 10.1101/gr.811003
McManus, B. A., and Coleman, D. C. (2014). Molecular epidemiology, phylogeny and evolution of Candida albicans. Infect. Genet. Evol. 21, 166–178. doi: 10.1016/j.meegid.2013.11.008
Mech, F., Wilson, D., Lehnert, T., Hube, B., and Thilo Figge, M. (2014). Epithelial invasion outcompetes hypha development during Candida albicans infection as revealed by an image-based systems biology approach. Cytometry A 85, 126–139. doi: 10.1002/cyto.a.22418
Mora-Montes, H. M., Netea, M. G., Ferwerda, G., Lenardon, M. D., Brown, G. D., Mistry, A. R., et al. (2011). Recognition and blocking of innate immunity cells by Candida albicans Chitin. Infect. Immun. 79, 1961–1970. doi: 10.1128/IAI.01282-10
Moreno, M. A., Ibrahim-Granet, O., Vicentefranqueira, R., Amich, J., Ave, P., Leal, F., et al. (2007). The regulation of zinc homeostasis by the ZafA transcriptional activator is essential for Aspergillus fumigatus virulence. Mol. Microbiol. 64, 1182–1197. doi: 10.1111/j.1365-2958.2007.05726.x
Moyes, D. L., Wilson, D., Richardson, J. P., Mogavero, S., Tang, S. X., Wernecke, J., et al. (2016). Candidalysin is a fungal peptide toxin critical for mucosal infection. Nature 532, 64–68. doi: 10.1038/nature17625
Nobile, C. J., Nett, J. E., Hernday, A. D., Homann, O. R., Deneault, J. S., Nantel, A., et al. (2009). Biofilm matrix regulation by Candida albicans Zap1. PLOS Biol. 7:e1000133. doi: 10.1371/journal.pbio.1000133
Noble, S. M., Gianetti, B. A., and Witchley, J. N. (2017). Candida albicans cell-type switching and functional plasticity in the mammalian host. Nat. Rev. Microbiol. 15, 96–108. doi: 10.1038/nrmicro.2016.157
Okagaki, L. H., and Nielsen, K. (2012). Titan cells confer protection from phagocytosis in Cryptococcus neoformans infections. Eukaryot. Cell 11, 820–826. doi: 10.1128/EC.00121-12
Okagaki, L. H., Strain, A. K., Nielsen, J. N., Charlier, C., Baltes, N. J., Chretien, F., et al. (2010). Cryptococcal cell morphology affects host cell interactions and pathogenicity. PLOS Pathog. 6:e1000953. doi: 10.1371/journal.ppat.1000953
Pande, K., Chen, C. B., and Noble, S. M. (2013). Passage through the mammalian gut triggers a phenotypic switch that promotes Candida albicans commensalism. Nat. Genet 45, 1088–1091. doi: 10.1038/ng.2710
Polke, M., Sprenger, M., Scherlach, K., Alban-Proano, M. C., Martin, R., Hertweck, C., et al. (2017). A functional link between hyphal maintenance and quorum sensing in Candida albicans. Mol. Microbiol. 103, 595–617. doi: 10.1111/mmi.13526
Schneider, R. D., Diehl, C., dos Santos, F. M., Piffer, A. C., Garcia, A. W. A., Kulmann, M. I. R., et al. (2015). Effects of zinc transporters on Cryptococcus gattii virulence. Sci. Rep. 5:10104. doi: 10.1038/srep10104
Sentandreu, M., Elorza, M. V., Sentandreu, R., and Fonzi, W. A. (1998). Cloning and characterization of PRA1, a gene encoding a novel pH-regulated antigen of Candida albicans. J. Bacteriol. 180, 282–289.
Skaar, E. P. (2010). The battle for iron between bacterial pathogens and their vertebrate hosts. PLOS Pathog. 6:e1000949. doi: 10.1371/journal.ppat.1000949
Soloviev, D. A., Fonzi, W. A., Sentandreu, R., Pluskota, E., Forsyth, C. B., Yadav, S., et al. (2007). Identification of pH-regulated antigen 1 released from Candida albicans as the major ligand for leukocyte integrin alphaMbeta2. J. Immunol. 178, 2038–2046. doi: 10.4049/jimmunol.178.4.2038
Sudbery, P., Gow, N., and Berman, J. (2004). The distinct morphogenic states of Candida albicans. Trends Microbiol. 12, 317–324. doi: 10.1016/j.tim.2004.05.008
Sudbery, P. E. (2011). Growth of Candida albicans hyphae. Nat. Rev. Microbiol. 9, 737–748. doi: 10.1038/nrmicro2636
Tyc, K. M., Kuhn, C., Wilson, D., and Klipp, E. (2014). Assessing the advantage of morphological changes in Candida albicans: a game theoretical study. Front. Microbiol. 5:41. doi: 10.3389/fmicb.2014.00041
Urban, C. F., Ermert, D., Schmid, M., Abu-Abed, U., Goosmann, C., Nacken, W., et al. (2009). Neutrophil extracellular traps contain calprotectin, a cytosolic protein complex involved in host defense against Candida albicans. PLOS Pathog. 5:e1000639. doi: 10.1371/journal.ppat.1000639
Wachtler, B., Citiulo, F., Jablonowski, N., Forster, S., Dalle, F., Schaller, M., et al. (2012). Candida albicans-epithelial interactions: dissecting the roles of active penetration, induced endocytosis and host factors on the infection process. PLOS ONE 7:e36952. doi: 10.1371/journal.pone.0036952
Wachtler, B., Wilson, D., Haedicke, K., Dalle, F., and Hube, B. (2011). From attachment to damage: defined genes of Candida albicans mediate adhesion, invasion and damage during interaction with oral epithelial cells. PLOS ONE 6:e17046. doi: 10.1371/journal.pone.0017046
Wagener, J., Malireddi, R. K. S., Lenardon, M. D., Koberle, M., Vautier, S., MacCallum, D. M., et al. (2014). Fungal chitin dampens inflammation through IL-10 induction mediated by NOD2 and TLR9 activation. PLOS Pathog. 10:e1004050. doi: 10.1371/journal.ppat.1004050
Waldron, K. J., Rutherford, J. C., Ford, D., and Robinson, N. J. (2009). Metalloproteins and metal sensing. Nature 460, 823–830. doi: 10.1038/nature08300
Walsham, N. E., and Sherwood, R. A. (2016). Fecal calprotectin in inflammatory bowel disease. Clin. Exp. Gastroenterol. 9, 21–29. doi: 10.2147/CEG.S51902
Whiteway, M., and Bachewich, C. (2007). Morphogenesis in Candida albicans. Annu. Rev. Microbiol. 61, 529–553. doi: 10.1146/annurev.micro.61.080706.093341
Wilson, D. (2015). An evolutionary perspective on zinc uptake by human fungal pathogens. Metallomics 7, 979–985. doi: 10.1039/c4mt00331d
Wilson, D., and Hube, B. (2010). Hgc1 mediates dynamic Candida albicans-endothelium adhesion events during circulation. Eukaryot. Cell 9, 278–287. doi: 10.1128/EC.00307-09
Wilson, S., and Bird, A. J. (2016). Zinc sensing and regulation in yeast model systems. Arch. Biochem. Biophys. 611, 30–36. doi: 10.1016/j.abb.2016.02.031
Yano, J., Noverr, M. C., and Fidel, P. L. Jr. (2012). Cytokines in the host response to Candida vaginitis: identifying a role for non-classical immune mediators, S100 alarmins. Cytokine 58, 118–128. doi: 10.1016/j.cyto.2011.11.021
Zaragoza, O., Garcia-Rodas, R., Nosanchuk, J. D., Cuenca-Estrella, M., Rodriguez-Tudela, J. L., and Casadevall, A. (2010). Fungal cell gigantism during mammalian infection. PLOS Pathog. 6:e1000945. doi: 10.1371/journal.ppat.1000945
Keywords: Candida albicans, fungal pathogen, morphology, morphogenesis, nutritional immunity, micronutrients, zinc, adhesion
Citation: Malavia D, Lehtovirta-Morley LE, Alamir O, Weiß E, Gow NAR, Hube B and Wilson D (2017) Zinc Limitation Induces a Hyper-Adherent Goliath Phenotype in Candida albicans. Front. Microbiol. 8:2238. doi: 10.3389/fmicb.2017.02238
Received: 10 August 2017; Accepted: 31 October 2017;
Published: 14 November 2017.
Edited by:
Oscar Zaragoza, Instituto de Salud Carlos III, SpainReviewed by:
Rocio Garcia-Rodas, University of Nice Sophia Antipolis, FranceMaria Rapala-Kozik, Jagiellonian University, Poland
Copyright © 2017 Malavia, Lehtovirta-Morley, Alamir, Weiß, Gow, Hube and Wilson. This is an open-access article distributed under the terms of the Creative Commons Attribution License (CC BY). The use, distribution or reproduction in other forums is permitted, provided the original author(s) or licensor are credited and that the original publication in this journal is cited, in accordance with accepted academic practice. No use, distribution or reproduction is permitted which does not comply with these terms.
*Correspondence: Duncan Wilson, duncan.wilson@abdn.ac.uk