- 1Laboratoire Universitaire de Biodiversité et Écologie Microbienne, ESIAB, Université de Brest, Plouzané, France
- 2Linking Landscape, Environment, Agriculture and Food, Instituto Superior de Agronomia, Universidade de Lisboa, Lisbon, Portugal
- 3Instituto Hispano-Luso de Investigaciones Agrarias, Department of Microbiology and Genetics, University of Salamanca, Salamanca, Spain
Colletotrichum spp. infect a wide diversity of hosts, causing plant diseases on many economically important crops worldwide. The genus contains approximately 189 species organized into at least 11 major phylogenetic lineages, also known as species complexes. The Colletotrichum acutatum species complex is a diverse yet relatively closely related group of plant pathogenic fungi within this genus. Within the species complex we find a wide diversity of important traits such as host range and host preference, mode of reproduction and differences in the strategy used to infect their hosts. Research on fungal comparative genomics have attempted to find correlations in these traits and patterns of gene family evolution but such studies typically compare fungi from different genera or even different fungal Orders. The C. acutatum species complex contains most of this diversity within a group of relatively closely related species. This Perspective article presents a review of the current knowledge on C. acutatum phylogeny, biology, and pathology. It also demonstrates the suitability of C. acutatum for the study of gene family evolution on a fine scale to uncover evolutionary events in the genome that are associated with the evolution of phenotypic characters important for host interactions.
Biology of the C. acutatum Species Complex
Many species belonging to the genus Colletotrichum are associated with plant diseases, commonly referred to as anthracnose. Colletotrichum spp. can affect a wide range of hosts and these pathogens are characterized by a global distribution. Virtually every botanic family cultivated is susceptible to one or more species of Colletotrichum and it is one of the most studied plant pathogenic fungi (Prusky et al., 2000; Latunde-Dada, 2001; Dean et al., 2012). Common hosts include many dicotyledonous plants such as strawberry, apple, citrus, and stone fruits, and major cereals such as maize and sorghum. Members of this genus produce crop losses up to 100% in many of these staple foods (Bailey and Jeger, 1992; Prusky et al., 2000; Talhinhas et al., 2005; Cannon et al., 2012).
Colletotrichum species employ a broad diversity of approaches to colonize and obtain nutrients from their hosts, ranging from biotrophs, nectrotrophs, hemibiotrophs to endophytes among others (Bailey and Jeger, 1992; Perfect et al., 1999; Rodriguez and Redman, 2008; Vargas et al., 2012; Prusky et al., 2013). Several Colletotrichum spp. are well-known as models for studying hemibiotrophy (Bergstrom and Nicholson, 1999; Perfect et al., 1999; Münch et al., 2008; O’Connell et al., 2012; Vargas et al., 2012). Hemibiotrophs initially infect through a brief biotrophic phase, which is associated with the production of large intracellular primary hyphae that can infect host cells without causing cell death. Later they switch to a necrotrophic phase during which narrower secondary hyphae are produced that spread throughout the host tissue causing necrotic lesions (Perfect et al., 1999). The scientific community has long studied the biomolecular processes that regulate this lifestyle, especially those related to the switch from biotrophy to necrotrophy (Dufresne et al., 2000; Thon et al., 2002; Wharton and Diéguez-Uribeondo, 2004; Peres et al., 2005; O’Connell et al., 2012).
The genus Colletotrichum has undergone frequent taxonomic changes in the past decades with the merging and addition of many species. Species concepts are still in a state of flux, however several major monophyletic clades, or species complexes, are now recognized (Cannon et al., 2012). A recent study provides an account of the 189 currently accepted species subdivided into 11 species complexes and 23 singleton species (Jayawardena et al., 2016).
Colletotrichum acutatum was identified by Simmonds (1965) and validated by the same author in Simmonds (1968). Since then, a growing number of fungi have been assigned to C. acutatum. This was either based on the identification of new species or on the reclassification of other species, mostly from C. gloeosporioides, that show a high morphological similarity and an overlapping spectrum of hosts (Peres et al., 2005; Sreenivasaprasad and Talhinhas, 2005). In fact, while approximately 7% of the scientific literature dealing with Colletotrichum during a 10-year period from 1991 to 2000 addressed C. acutatum, this proportion raised to 18% in the following decade (based on a ISI Web of Science search on 1839 publications during 1991–2010 containing “Colletotrichum” in the title), depicting C. acutatum as a “popular” entity.
Diversity among C. acutatum isolates has long been recognized (up to 4% variability in the rDNA-ITS sequences), but comparison to neighboring taxa clearly suggested this as a monophyletic group (Sreenivasaprasad et al., 1996; Damm et al., 2012; Baroncelli et al., 2016). Several intra-specific groupings were established within C. acutatum based on morphological, physiological, sexual, and molecular data (as revised by Sreenivasaprasad and Talhinhas, 2005), and these were compiled into eight groups (A1–A8) based on rDNA-ITS and β-tubulin 2 (TUB2) sequence analyses (Sreenivasaprasad and Talhinhas, 2005). To date 34 species are accepted (Damm et al., 2012; Huang et al., 2013; Crous et al., 2015; Bragança et al., 2016; Jayawardena et al., 2016; de Silva et al., 2017) and comprise what is now known as the C. acutatum species complex. Species complexes are informal designations widely used in the genus Colletotrichum to aggregate the huge and increasing (e.g., Pardo-de la Hoz et al., 2016) number of species described, recognizing monophyletic sub-generic groups. However, the number of species is expected to increase due to the high genetic variability of the system and to the increasing number of population studies unraveling strains that do not belong to any species described previously. The designation of infrageneric to accommodate what are now considered species complexes in the genus Colletotrichum would avoid confusions such as the one between C. acutatum s.l. and C. acutatum s.s. These 34 species cluster in five clades (Figure 1), two of which are of narrow diversity (clades 3 and 4), while the other three contain at least eight species each, with clades 2 and 5 encompassing the largest genetic diversity within the C. acutatum species complex.
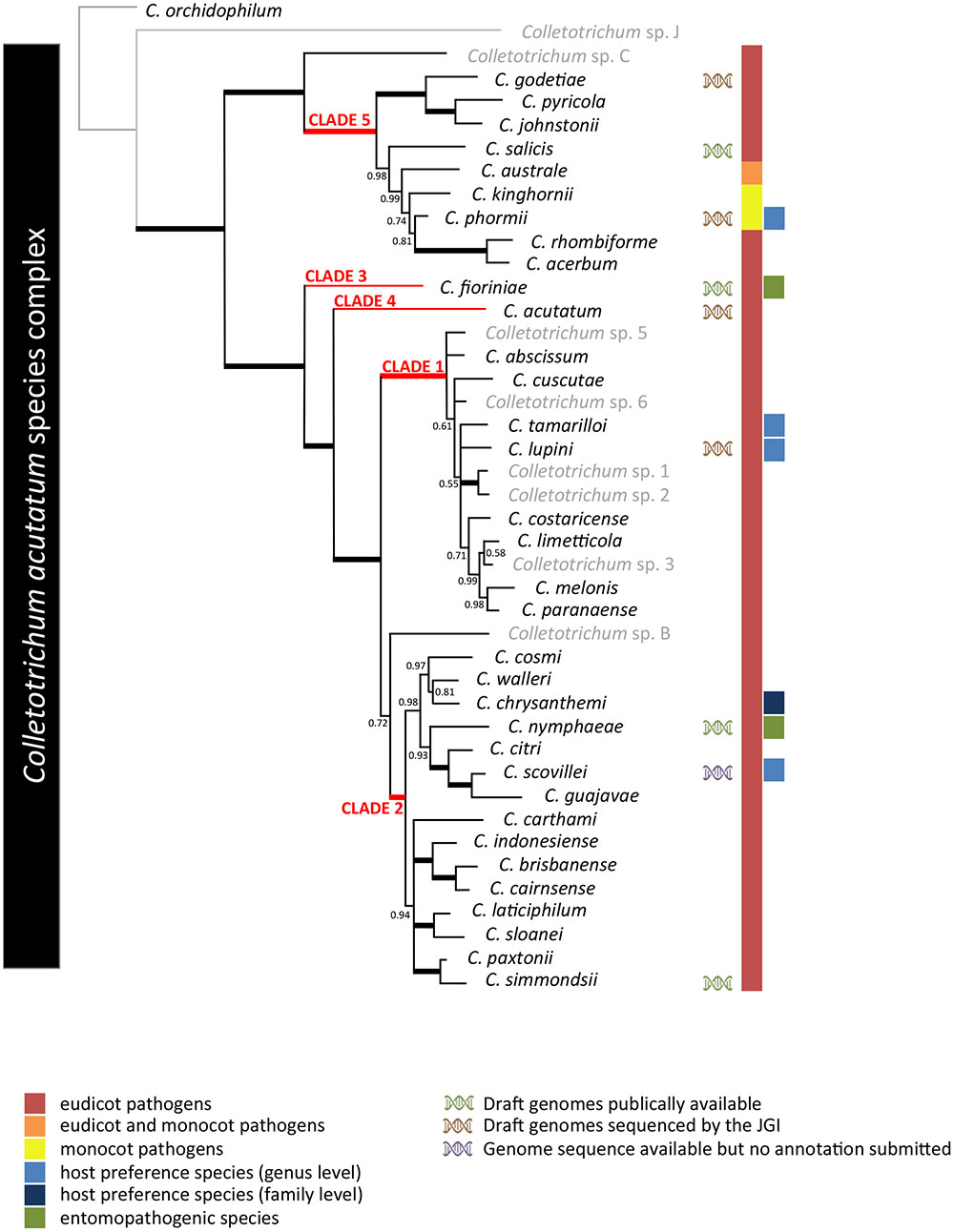
FIGURE 1. Phylogenetic analysis of the 42 Colletotrichum acutatum species complex strains listed in Supplementary Table S1 based on a multilocus concatenated alignment of the ITS, GAPDH, CHS-1, HIS3, ACT, and TUB2 genes. Accepted species (Jayawardena et al., 2016) are highlighted in black while isolates not fitting with those are reported in gray. Colletotrichum orchidophilum was used as an outgroup.
Members of the C. acutatum species complex have been well-documented from agricultural and natural hosts worldwide (Peres et al., 2005; Sreenivasaprasad and Talhinhas, 2005). The species complex has a very wide host range. Meta-analyses have revealed that C. acutatum s.l. strains have been associated with infection on 100s of species from more than 90 genera of plants (Magnoliophyta: monocotyledons and dicotyledons, Pinophyta and Pteridophyta), along with at least two insect species (Marcelino et al., 2008; Mascarin et al., 2016) and in a few cases also with marine ecosystems (Manire et al., 2002; Namikoshi et al., 2002). There appears to be little to no evidence of co-evolution of host and pathogen. This is especially true in cultivated fruit systems such as strawberry and olive that are susceptible to many members of the species complex (Baroncelli et al., 2015b; Talhinhas et al., 2015). Although the C. acutatum species complex is regarded as polyphagous, the lupin anthracnose pathogen has been shown to form a well-defined, homogeneous and host-specific group, unlike other heterogeneous and polyphagous groups (Talhinhas et al., 2016). A similar situation applies to safflower (Carthamus tinctorius), as only strains belonging to C. chrysanthemi were pathogenic to safflower. Strains genetically very close but belonging to different species were not pathogenic on the same host (Baroncelli et al., 2015a). Other members of the species complex that show a strong relationship with a specific host include C. phormii pathogenic to Phormium spp. a monocot native to New Zealand and C. tamarilloi the causal agent of tamarillo (Solanum betaceum) anthracnose (Baroncelli, 2012; Damm et al., 2012).
The lifestyles employed by members of the C. acutatum species complex depend on many factors, including the host, host tissue infected, and the environment (Redman et al., 2001; Dieguez-Uribeondo et al., 2005; Peres et al., 2005). For example, on apples (Malus domestica) members of the C. acutatum species complex cause necrotic lesions of fruits but does not cause any symptoms on leaves (Peres et al., 2005). On sweet orange, the pathogen acts as a necrotroph on flowers and as a biotroph on leaves (Peres et al., 2005). On olive, at least six Colletotrichum spp. are associated with anthracnose, most frequently C. nymphaeae, C. godetiae, and C. acutatum (Talhinhas et al., 2005, 2009). Upon penetration of mature fruits, these species have a short biotrophic phase that is represented by multi-lobed primary hyphae, followed by an extended necrotrophic stage, leading to disease symptoms. On olive leaves and branches, the fungus is most frequently asymptomatic but capable of epiphytic growth and sporulation (Talhinhas et al., 2011). Colletotrichum nymphaeae is also an entomopathogen infecting the citrus orthezia scale insect, Praelongorthezia praelonga (Mascarin et al., 2016) while C. fioriniae is pathogenic toward the insect Fiorinia externa (Marcelino et al., 2008).
On some fruits, such as apple and blueberry (Vaccinium spp.), members of the C. acutatum species complex cause both pre-harvest and post-harvest diseases (Milholland, 1995; Jones et al., 1996; Shi et al., 1996). On these plants, asymptomatic, quiescent infections occur on unripe fruit and begin to develop necrotic lesions as the fruit ripens (Alkan et al., 2015). There are many other examples of post-harvest decays on fruits such as peaches (Prunus persica), almonds (Prunus dulcis), avocado (Persea americana), mango (Mangifera indica), papaya (Carica papaya), and guava (Psidium guajava) that develop from quiescent infections that began in the field (Agostini et al., 1992; Freeman and Shabi, 1996; Smith, 1998; Afanador-Kafuri et al., 2003).
Sexual reproduction has been identified in some Colletotrichum species but for most of them only the anamorph is known (Carvajal and Edgerton, 1944; Politis, 1975; Guerber and Correll, 2001). In vitro analyses have demonstrated that some C. acutatum species are heterothallic, requiring two strains of opposite mating types to complete the sexual cycle (Guerber and Correll, 2001) while others are homothallic, capable of sexual reproduction without a second strain (Talgø et al., 2007; LoBuglio and Pfister, 2008; Baroncelli, 2012; Damm et al., 2012). Phylogenetic analyses also suggest a strong relationship between monophyletic lineages and mating behavior (Baroncelli, 2012). In this case, a hypothetical homothallic ancestor and two lineage-specific events: the first being the acquisition of heterothallic capability in specific lineages and the second being the complete loss of mating behavior in other lineages. Thus, C. acutatum s.l. is a suitable system for studying the genetic bases of fungal mating systems and the effect of sexual behavior in genome architecture, ecological adaptation, and host association patterns.
Colletotrichum acutatum s.l. also shows a worldwide geographic distribution. Strains belonging to these taxa are present in diverse climatic zones worldwide (Sreenivasaprasad and Talhinhas, 2005; Damm et al., 2012). Even if different geographic areas do show particular trends in population distribution any strong connection between genetic groups or populations and their distribution has not been shown yet. However, results from previous studies do suggest Oceania as the possible origin of the complex and particularly of clade 5 (Baroncelli, 2012). This region showed the highest level of variability and strains closely related to a hypothetical ancestral population are mainly distributed in Australia and New Zealand. The low genetic variability of clade 1 and the presence of all the strains that cannot be assigned to designed species might reflect an on going speciation process. Clade 1 probably originated in South America as this geographic origin shows the highest diversity within the clade and more genetic groups and species are recently being described from this area. Interestingly only C. lupini as member of Clade 1 spread across the globe (over the last few decades) while all others report are mainly confined in South America. It is tempting to speculate on a cause-effect relationship between the capacity of a specific population to infect lupins and its worldwide spread, especially considering that C. lupini is a seed-born pathogen (Talhinhas et al., 2016). Clade 4 is represented solely by C. acutatum sensu stricto [i.e., the fungus identified by Simmonds (1965) in Australia as the species holotype]. The populations clustering in this taxon show limited diversity, but they can nevertheless be found on a relatively large number of hosts predominantly in Oceania and Africa (Sreenivasaprasad and Talhinhas, 2005), although recently this fungus has emerged as a pathogen associated with olive anthracnose, along with C. nymphaeae and C. godetiae, in several Mediterranean countries (Talhinhas et al., 2009; Mosca et al., 2014; Chattaoui et al., 2016), suggesting shifts in pathogen populations at global scale.
The general evolutionary trend emerging in the C. acutatum species complex appears to be that of distinct populations undergoing clear changes in their host-association pattern. This also might suggest a role of switching in mating behavior and changes in host association patterns. The occurrence of heterothallism seems to have influenced host range diversity. In contrast, isolates capable of homothallism (C. salicis and C. phormii) have a narrow range of hosts. The evolutionary trend suggests that the capacity of populations to exchange genetic information lead to rearrangement at the genomic level increasing genome plasticity and the host spectrum of this pathogen.
Colletotrichum Genomics
Genome sequences for 28 species of Colletotrichum have been published in recent years, and comparative genomic studies have focused on genes associated with pathogenicity (Gan et al., 2013), host specialization (Baroncelli et al., 2016; Gan et al., 2016), transition in lifestyle between biotrophy and necrotrophy (O’Connell et al., 2012) and more recently between endophytic and parasitic lifestyles (Hacquard et al., 2016; Hiruma et al., 2016). Comparative analyses of secretomes have revealed that the majority of predicted secreted proteins have enzymatic activity, many of which are candidate effectors (O’Connell et al., 2012; Gan et al., 2013; Baroncelli et al., 2016; Sanz-Martín et al., 2016). The host range of Colletotrichum spp. appears to be associated with genes loss or gain in families such as those encoding carbohydrate-active enzymes (CAZymes) and proteases (Baroncelli et al., 2016). In particular, gene families encoding proteases and carbohydrate-degrading enzymes are highly expanded in Colletotrichum compared to other Sordariomycetes (Gan et al., 2013; Baroncelli et al., 2016), suggesting in increased importance of these gene families in Colletotrichum spp. infection processes. Transcriptional profiling experiments have also revealed that CAZymes, along with other classes of secreted proteins are highly modulated during the infection process, further implicating them as important players in pathogenicity (Kleemann et al., 2012; O’Connell et al., 2012).
Another important feature that has emerged from comparative studies is that lifestyle and host preference is not correlated with phylogenetic relationships (Baroncelli et al., 2016; Gan et al., 2016). A comparison of the genomes of the endophyte C. tofieldiae and the closely related pathogen C. incanum revealed that the transition to an endophytic lifestyle was associated with a reduction in the number of predicted effectors and an expansion in chitin-binding and secondary metabolism related proteins (Hacquard et al., 2016). In contrast, relatively distantly related members of the C. acutatum and C. gloeosporioides species complexes have strikingly similar repertoires of carbohydrate-active enzymes and secreted proteases (Baroncelli et al., 2016). This observation suggests that these gene families evolved recently and independently in these two phylogenetically separated lineages (Baroncelli et al., 2016; Gan et al., 2016). Further comparative analyses of the C. acutatum species complex genomes revealed that necrosis and ethylene-inducing peptide 1-like proteins were twice as abundant as the other fungi studied. The large number of these genes associated with leaf necrosis and immunity associated responses in Dicotyledonous plants would make the C. acutatum species complex a model system to study their evolution and biological role (Baroncelli et al., 2016).
The C. acutatum Species Complex as a Model to Study the Evolution of Plant Pathogens
Recently, the genomes of more than 20 Colletotrichum species have been sequenced (O’Connell et al., 2012; Alkan et al., 2013; Gan et al., 2013, 2016, 2017; Baroncelli et al., 2014a,b, 2016; Hacquard et al., 2016; Han et al., 2016; Hiruma et al., 2016; Queiroz et al., 2017), revealing a tremendous diversity in genome architecture and gene content. While most of the studies focused on comparisons of distantly related lineages they also demonstrate the need for higher resolution taxonomic sampling in order to better understand the evolution of fungal genomes and the possible association with phenotypic characters such as host range, pathogenic lifestyle and reproductive strategy. In this context, the C. acutatum species complex provides a good model system, offering a variety of evolutionary closely related lineages with different phenotypic characteristics. The switch between mono and dicotyledonous hosts occurred in clade 5 (Figure 1) gives an example. Comparative genomics and transcriptomics of late diverging species adapted to different hosts could help us to gain an understanding of the genomic bases involved in this host switch. Another example is provided by the host specialization of certain lineages to a specific host such as C. lupini. The biological diversity of the C. acutatum species complex and the presence of very closely related species with different characteristics such as host range and spectrum and mating behavior makes C. acutatum a suitable model to investigate genomic signatures associated with changes in important phenotypic characters of fungal plant pathogens.
Materials and Methods
Sequences for the genes used for phylogenetic analyses (ITS, GAPDH, CHS-1, HIS3, ACT, TUB2) were retrieved from public databases (Supplementary Table S1). Multiple sequence alignments were performed with MAFFT v. 7.304 (Katoh and Standley, 2013) were exported to MEGA7 (Kumar et al., 2016) where best-fit substitution models were calculated for each separate sequence dataset. The multilocus concatenated alignment was performed with Geneious 10.2.21 (Kearse et al., 2012). A Markov Chain Monte Carlo algorithm was used to generate phylogenetic trees with Bayesian probabilities using MrBayes 3.2.1 (Ronquist and Huelsenbeck, 2003) for the combined sequence alignment. Models of nucleotide substitution for each gene determined by MEGA7 were used for each locus. The analysis in MrBayes ran for 5∗106 of generations to reach a P-value lower than 0.01 with two parallel searches using three heated and one cold Markov chain sampled every 100 generations and 25% of the generations were discarded as burn-in.
Author Contributions
All authors listed have made substantial, direct and intellectual contribution to the work, and approved it for publication. RB and PT drafted the manuscript; FP, SS, GLF and MT critically revised and improved the manuscript.
Conflict of Interest Statement
The authors declare that the research was conducted in the absence of any commercial or financial relationships that could be construed as a potential conflict of interest.
Acknowledgments
This research was supported by grants AGL2012-34139 and AGL2015-66362-R from the Ministerio de Economia y Competitividad (MINECO) of Spain, by “The Prograilive” project (grant: RBRE160116CR0530019) funded by the regions of Bretagne and Pays de la Loire and FEADER grants, France, and by the Fundação para a Ciência e a Tecnologia (FCT), Portugal, concerning financial support to the research unit LEAF (UID/AGR/04129/2013).
Supplementary Material
The Supplementary Material for this article can be found online at: https://www.frontiersin.org/articles/10.3389/fmicb.2017.02001/full#supplementary-material
TABLE S1 | List of Colletotrichum acutatum reference strains used for the phylogeny shown in Figure 1, indicating species designation, strain ID, GenBank accession numbers of the sequences retrieved and used for phylogenetic analyses and references.
Footnotes
References
Afanador-Kafuri, L., Minz, D., Maymon, M., and Freeman, S. (2003). Characterization of Colletotrichum isolates from tamarillo, passiflora, and mango in Colombia and identification of a unique species from the genus. Phytopathology 93, 579–587. doi: 10.1094/PHYTO.2003.93.5.579
Agostini, J. P., Timmer, L. W., and Mitchell, D. J. (1992). Morphological and pathological characteristics of strains of Colletotrichum gloeosporioides from citrus. Phytopathology 82, 1377–1382. doi: 10.1094/Phyto-82-1377
Alkan, N., Friedlander, G., Ment, D., Prusky, D., and Fluhr, R. (2015). Simultaneous transcriptome analysis of Colletotrichum gloeosporioides and tomato fruit pathosystem reveals novel fungal pathogenicity and fruit defense strategies. New Phytol. 205, 801–815. doi: 10.1111/nph.13087
Alkan, N., Meng, X., Friedlander, G., Reuveni, E., Sukno, S., Sherman, A., et al. (2013). Global aspects of pacC regulation of pathogenicity genes in Colletotrichum gloeosporioides as revealed by transcriptome analysis. Mol. Plant Microbe Interact. 26, 1345–1358. doi: 10.1094/MPMI-03-13-0080-R
Bailey, J. A., and Jeger, M. J. (1992). Colletotrichum: Biology, Pathology and Control. Wallingford: CAB International.
Baroncelli, R. (2012). Colletotrichum acutatum sensu lato: From Diversity Study to Genome Analysis. Ph.D. dissertation, University of Warwick, Coventry.
Baroncelli, R., Amby, D. B., Zapparata, A., Sarrocco, S., Vannacci, G., Le Floch, G., et al. (2016). Gene family expansions and contractions are associated with host range in plant pathogens of the genus Colletotrichum. BMC Genomics 17:555. doi: 10.1186/s12864-016-2917-6
Baroncelli, R., Sanz-Martín, J. M., Rech, G. E., Sukno, S. A., and Thon, M. R. (2014a). Draft genome sequence of Colletotrichum sublineola, a destructive pathogen of cultivated sorghum. Genome Announc. 2, e00540-14. doi: 10.1128/genomeA.00540-14
Baroncelli, R., Sarrocco, S., Zapparata, A., Tavarini, S., Angelini, L. G., and Vannacci, G. (2015a). Characterization and epidemiology of Colletotrichum acutatum sensu lato (C. chrysanthemi) causing Carthamus tinctorius anthracnose. Plant Pathol. 64, 375–384. doi: 10.1111/ppa.12268
Baroncelli, R., Sreenivasaprasad, S., Sukno, S. A., Thon, M. R., and Holub, E. (2014b). Draft genome sequence of Colletotrichum acutatum sensu lato (Colletotrichum fioriniae). Genome Announc. 2, e00112-e00114. doi: 10.1128/genomeA.00112-14
Baroncelli, R., Zapparata, A., Sarrocco, S., Sukno, S. A., Lane, C. R., Thon, M. R., et al. (2015b). Molecular diversity of anthracnose pathogen populations associated with UK strawberry production suggests multiple introductions of three different Colletotrichum species. PLOS ONE 10:e0129140. doi: 10.1371/journal.pone.0129140
Bergstrom, G. C., and Nicholson, L. (1999). The biology of corn anthracnose: knowledge to exploit for improved management. Phytopathology 83, 596–608. doi: 10.1094/PDIS.1999.83.7.596
Bragança, C. A., Damm, U., Baroncelli, R., Massola Júnior, N. S., and Crous, P. W. (2016). Species of the Colletotrichum acutatum complex associated with anthracnose diseases of fruit in Brazil. Fungal Biol. 120, 547–561. doi: 10.1016/j.funbio.2016.01.011
Cannon, P. F., Damm, U., Johnston, P. R., and Weir, B. S. (2012). Colletotrichum – current status and future directions. Stud. Mycol. 73, 181–213. doi: 10.3114/sim0014
Carvajal, F., and Edgerton, C. W. (1944). The perfect stage of Colletotrichum falcatum. Phytopathology 34, 206–213.
Chattaoui, M., Raya, M. C., Bouri, M., Moral, J., Perez-Rodriguez, M., Trapero, A., et al. (2016). Characterization of a Colletotrichum population causing anthracnose disease on Olive in northern Tunisia. J. Appl. Microbiol. 120, 1368–1381. doi: 10.1111/jam.13096
Crous, P. W., Wingfield, M. J., Guarro, J., Hernández-Restrepo, M., Sutton, D. A., Acharya, K., et al. (2015). Fungal Planet description sheets: 320–370. Persoonia 34, 167–266. doi: 10.3767/003158515X688433
Damm, U., Cannon, P. F., Woudenberg, J. H., and Crous, P. W. (2012). The Colletotrichum acutatum species complex. Stud. Mycol. 73, 37–113. doi: 10.3114/sim0010
de Silva, D. D., Ades, P. K., Crous, P. W., and Taylor, P. W. J. (2017). Colletotrichum species associated with chili anthracnose in Australia. Plant Pathol. 66, 254–267. doi: 10.1111/ppa.12572
Dean, R., Van Kan, J. A., Pretorius, Z. A., Hammond-Kosack, K. E., Di Pietro, A., Spanu, P. D., et al. (2012). The Top 10 fungal pathogens in molecular plant pathology. Mol. Plant Pathol. 13, 414–430. doi: 10.1111/j.1364-3703.2011.00783.x
Dieguez-Uribeondo, J., Forster, H., Sotoestrada, A., and Adaskaveg, J. E. (2005). Subcuticular-intracellular hemibiotrophic and intercellular necrotrophic development of Colletotrichum acutatum on almond. Phytopathology 95, 751–758. doi: 10.1094/PHYTO-95-0751
Dufresne, M., Perfect, S., Pellier, A. L., Bailey, J. A., and Langin, T. (2000). A GAL4-like protein is involved in the switch between biotrophic and necrotrophic phases of the infection process of Colletotrichum lindemuthianum on common bean. Plant Cell 12, 1579–1590. doi: 10.1105/tpc.12.9.1579
Freeman, S., and Shabi, E. (1996). Cross-infection of subtropical and temperate fruits by Colletotrichum species from various hosts. Physiol. Mol. Plant Pathol. 49, 395–404. doi: 10.1006/pmpp.1996.0062
Gan, P., Ikeda, K., Irieda, H., Narusaka, M., O’Connell, R. J., Narusaka, Y., et al. (2013). Comparative genomic and transcriptomic analyses reveal the hemibiotrophic stage shift of Colletotrichum fungi. New Phytol. 197, 1236–1249. doi: 10.1111/nph.12085
Gan, P., Narusaka, M., Kumakura, N., Tsushima, A., Takano, Y., Narusaka, Y., et al. (2016). Genus-wide comparative genome analyses of Colletotrichum species reveal specific gene family losses and gains during adaptation to specific infection lifestyles. Genome Biol. Evol. 8, 1467–1481. doi: 10.1093/gbe/evw089
Gan, P., Narusaka, M., Tsushima, A., Narusaka, Y., Takano, Y., and Shirasu, K. (2017). Draft genome assembly of Colletotrichum chlorophyti, a pathogen of herbaceous plants. Genome Announc. 5, e01733-16. doi: 10.1128/genomeA.01733-16
Guerber, J. C., and Correll, J. C. (2001). Characterization of Glomerella acutata, the teleomorph of Colletotrichum acutatum. Mycologia 93, 216–219. doi: 10.2307/3761619
Hacquard, S., Kracher, B., Hiruma, K., Münch, P. C., Garrido-Oter, R., Thon, M. R., et al. (2016). Survival trade-offs in plant roots during colonization by closely related beneficial and pathogenic fungi. Nat. Commun. 7:11362. doi: 10.1038/ncomms11362
Han, J. H., Chon, J. K., Ahn, J. H., Choi, I. Y., Lee, Y. H., and Kim, K. S. (2016). Whole genome sequence and genome annotation of Colletotrichum acutatum, causal agent of anthracnose in pepper plants in South Korea. Genom. Data 8, 45–46. doi: 10.1016/j.gdata.2016.03.007
Hiruma, K., Gerlach, N., Sacristán, S., Nakano, R. T., Hacquard, S., Kracher, B., et al. (2016). Root endophyte Colletotrichum tofieldiae confers plant fitness benefits that are phosphate status dependent. Cell 165, 464–474. doi: 10.1016/j.cell.2016.02.028
Huang, F., Chen, G. Q., Hou, X., Fu, Y. S., Cai, L., Hyde, K. D., et al. (2013). Colletotrichum species associated with cultivated citrus in China. Fungal Divers. 61, 61–74. doi: 10.1007/s13225-013-0232-y
Jayawardena, R. S., Hyde, K. D., Damm, U., Cai, L., Liu, M., Li, X. H., et al. (2016). Notes on currently accepted species of Colletotrichum. Mycosphere 7, 1192–1260. doi: 10.5943/mycosphere/si/2c/9
Jones, A. L., Ehret, G. R., Meyer, M. P., and Shane, W. W. (1996). Occurrence of bitter rot on apple in Michigan. Plant Dis. 80, 1294–1297. doi: 10.1094/PD-80-1294
Katoh, K., and Standley, D. M. (2013). MAFFT multiple sequence alignment software version 7: improvements in performance and usability. Mol. Biol. Evol. 30, 772–780. doi: 10.1093/molbev/mst010
Kearse, M., Moir, R., Wilson, A., Stones-Havas, S., Cheung, M., Sturrock, S., et al. (2012). Geneious Basic: an integrated and extendable desktop software platform for the organization and analysis of sequence data. Bioinformatics 28, 1647–1649. doi: 10.1093/bioinformatics/bts199
Kleemann, J., Rincon-Rivera, L. J., Takahara, H., Neumann, U., Ver Loren van Themaat, E., van der Does, H. C., et al. (2012). Sequential delivery of host-induced virulence effectors by appressoria and intracellular hyphae of the phytopathogen Colletotrichum higginsianum. PLOS Pathog. 8:e1002643. doi: 10.1371/journal.ppat.1002643
Kumar, S., Stecher, G., and Tamura, K. (2016). MEGA7: Molecular Evolutionary Genetics Analysis version 7.0 for bigger datasets. Mol. Biol. Evol. 33, 1870–1874. doi: 10.1093/molbev/msw054
Latunde-Dada, A. O. (2001). Colletotrichum: tales of forcible entry, stealth, transient confinement and breakout. Mol. Plant Pathol. 2, 187–198. doi: 10.1046/j.1464-6722.2001.00069.x
LoBuglio, K. F., and Pfister, D. H. (2008). A Glomerella species phylogenetically related to Colletotrichum acutatum on Norway maple in Massachusetts. Mycologia 100, 710–715. doi: 10.3852/07-192
Manire, C. A., Rhinehart, H. L., Sutton, D. A., Thomson, E. H., Rinaldi, M. G., Buck, J. D., et al. (2002). Disseminated mycotic infection caused by Colletotrichum acutatum in a Kemp’s ridley sea turtle (Lepidochelys kempi). J. Clin. Microbiol. 40, 4273–4280. doi: 10.1128/JCM.40.11.4273-4280.2002
Marcelino, J., Giordano, R., Gouli, S., Gouli, V., Parker, B. L., Skinner, M., et al. (2008). Colletotrichum acutatum var. fioriniae (teleomorph: Glomerella acutata var. fioriniae var. nov.) infection of a scale insect. Mycologia 100, 353–374. doi: 10.3852/07-174R
Mascarin, G. M., Guarín-Molina, J. H., Arthurs, S. P., Humber, R. A., Moral, R. A., Demétrio, C. G., et al. (2016). Seasonal prevalence of the insect pathogenic fungus Colletotrichum nymphaeae in Brazilian citrus groves under different chemical pesticide regimes. Fungal Ecol. 22, 43–51. doi: 10.1016/j.funeco.2016.04.005
Milholland, R. D. (1995). “Anthracnose fruit rot (ripe rot),” in Compendium of Blueberry and Cranberry Diseases, eds L. Caruso and D. C. Ramsdell (St. Paul, MN: American Phytopathological Society).
Mosca, S., Li Destri Nicosia, M. G., Cacciola, S. O., and Schena, L. (2014). Molecular analysis of Colletotrichum species in the carposphere and phyllosphere of olive. PLOS ONE 9:e114031. doi: 10.1371/journal.pone.0114031
Münch, S., Lingner, U., Floss, D. S., Ludwig, N., Sauer, N., and Deising, H. B. (2008). The hemibiotrophic lifestyle of Colletotrichum species. J. Plant Physiol. 165, 41–51. doi: 10.1016/j.jplph.2007.06.008
Namikoshi, M., Akano, K., Kobayashi, H., Koike, Y., Kitazawa, A., Rondonuwu, A. B., et al. (2002). Distribution of marine filamentous fungi associated with marine sponges in coral reefs of Palau and Bunaken Island, Indonesia. J. Tokyo Univ. Fish. 88, 15–20.
O’Connell, R. J., Thon, M. R., Hacquard, S., Amyotte, S. G., Kleemann, J., Torres, M. F., et al. (2012). Lifestyle transitions in plant pathogenic Colletotrichum fungi deciphered by genome and transcriptome analyses. Nat. Genet. 44, 1060–1065. doi: 10.1038/ng.2372
Pardo-de la Hoz, C. J., Calderón, C., Rincón, A. M., Cárdenas, M., Danies, G., López-Kleine, L., et al. (2016). Species from the Colletotrichum acutatum, Colletotrichum boninense and Colletotrichum gloeosporioides species complexes associated with tree tomato and mango crops in Colombia. Plant Pathol. 65, 227–237. doi: 10.1111/ppa.12410
Peres, N. A., Timmer, L. W., Adaskaveg, J. E., and Correll, J. C. (2005). Life styles of Colletotrichum acutatum. Plant Dis. 89, 784–796. doi: 10.1094/PD-89-0784
Perfect, S. E., Hughes, H. B., O’Connell, R. J., and Green, J. R. (1999). Colletotrichum: a model genus for studies on pathology and fungal-plant interactions. Fungal Genet. Biol. 27, 186–198. doi: 10.1006/fgbi.1999.1143
Politis, D. J. (1975). The identity and perfect state of Colletotrichum graminicola. Mycologia 67, 56–62. doi: 10.2307/3758227
Prusky, D., Alkan, N., Mengiste, T., and Fluhr, R. (2013). Quiescent and necrotrophic lifestyle choice during postharvest disease development. Annu. Rev. Phytopathol. 51, 155–176. doi: 10.1146/annurev-phyto-082712-102349
Prusky, D., Freeman, S., and Dickman, M. B. (eds) (2000). Colletotrichum: Host Specificity, Pathology, and Host-Pathogen Interaction. St. Paul, MN: APS Press, 393.
Queiroz, C. B., de Correia, H. L. N., Menicucci, R. P., Vidigal, P. M. P., and de Queiroz, M. V. (2017). Draft genome sequences of two isolates of Colletotrichum lindemuthianum, the causal agent of anthracnose in common beans. Genome Announc. 5, e00214-17. doi: 10.1128/genomeA.00214-17
Redman, R. S., Dunigan, D. D., and Rodriguez, R. J. (2001). Fungal symbiosis: from mutualism to parasitism, who controls the outcome, host or invader? New Phytol. 151, 705–716. doi: 10.1046/j.0028-646x.2001.00210.x
Rodriguez, R., and Redman, R. (2008). More than 400 million years of evolution and some plants still can’t make it on their own: plant stress tolerance via fungal symbiosis. J. Exp. Bot. 59, 1109–1111. doi: 10.1093/jxb/erm342
Ronquist, F., and Huelsenbeck, J. P. (2003). MRBAYES 3: bayesian phylogenetic inference under mixed models. Bioinformatics 19, 1572–1574. doi: 10.1093/bioinformatics/btg180
Sanz-Martín, J. M., Pacheco-Arjona, J. R., Bello-Rico, V., Vargas, W. A., Monod, M., Díaz-Mínguez, J. M., et al. (2016). A highly conserved metalloprotease effector enhances virulence in the maize anthracnose fungus Colletotrichum graminicola. Mol. Plant Pathol. 17, 1048–1062. doi: 10.1111/mpp.12347
Sato, T., and Moriwaki, J. (2013). Re-classification of the Colletotrichum acutatum group A2 and A4 and re-identification of strains belonging to the groups in Japan. Plant Prot. 67, 367–372.
Shi, Y. L., Correll, J. C., and Guerber, J. C. (1996). Frequency of Colletotrichum species causing bitter rot of apple in the south-eastern United States. Plant Dis. 80, 692–696. doi: 10.1094/PD-80-0692
Simmonds, J. H. (1965). A study of the species of Colletotrichum causing ripe fruit rots in Queensland. Qld J. Agric. Anim. Sci. 22, 437–459.
Simmonds, J. H. (1968). Type specimens of Colletotrichum gloeosporioides var. minor and Colletotrichum acutatum. Qld. J. Agric. Anim. Sci. 25, 178A.
Smith, B. J. (1998). Compendium of Strawberry Diseases, ed. J. L. Mass (St. Paul, MN: American Phytopathological Society).
Sreenivasaprasad, S., Mills, P. R., Meehan, B., and Brown, A. E. (1996). Phylogeny and systematics of 18 Colletotrichum species based on ribosomal DNA sequences. Genome 39, 499–512. doi: 10.1139/g96-064
Sreenivasaprasad, S., and Talhinhas, P. (2005). Genotypic and phenotypic diversity in Colletotrichum acutatum a cosmopolitan pathogen causing anthracnose on a wide range of hosts. Mol. Plant Pathol. 6, 361–378. doi: 10.1111/j.1364-3703.2005.00291.x
Talgø, V., Aamot, H. U., Strømeng, G. M., Klemsdal, S. S., and Stensvand, A. (2007). Glomerella acutata on Highbush Blueberry (Vaccinium corymbosum L.) in Norway. Plant Health Prog. doi: 10.1094/PHP-2007-0509-01-RS
Talhinhas, P., Baroncelli, R., and Le Floch, G. (2016). Anthracnose of lupins caused by Colletotrichum lupini: a recent disease and a successful worldwide pathogen. J. Plant Pathol. 98, 5–14. doi: 10.4454/JPP.V98I1.040
Talhinhas, P., Gonçalves, E., Sreenivasaprasad, S., and Oliveira, H. (2015). Virulence diversity of anthracnose pathogens (Colletotrichum acutatum and C. gloeosporioides species complexes) on eight olive cultivars commonly grown in Portugal. Eur. J. Plant Pathol. 142, 73–83. doi: 10.1007/s10658-014-0590-7
Talhinhas, P., Mota-Capitão, C., Martins, S., Ramos, A. P., Neves-Martins, J., Guerra-Guimarães, L., et al. (2011). Epidemiology, histopathology and aetiology of olive anthracnose caused by Colletotrichum acutatum and C. gloeosporioides in Portugal. Plant Pathol. 60, 483–495. doi: 10.1111/j.1365-3059.2010.02397.x
Talhinhas, P., Neves-Martins, J., Oliveira, H., and Sreenivasaprasad, S. (2009). The distinctive population structure of Colletotrichum species associated with olive anthracnose in the Algarve region of Portugal reflects a host-pathogen diversity hot spot. FEMS Microbiol. Lett. 296, 31–38. doi: 10.1111/j.1574-6968.2009.01613.x
Talhinhas, P., Sreenivasaprasad, S., Neves-Martins, J., and Oliveira, H. (2005). Molecular and phenotypic analyses reveal the association of diverse Colletotrichum acutatum groups and a low level of C. gloeosporioides with olive anthracnose. Appl. Environ. Microbiol. 71, 2987–2998. doi: 10.1128/AEM.71.6.2987-2998.2005
Thon, M. R., Nuckles, E. M., Takach, J. E., and Vaillancourt, L. J. (2002). CPR1: a gene encoding a putative signal peptidase that functions in pathogenicity of Colletotrichum graminicola to maize. Mol. Plant Microbe Interact. 15, 120–128. doi: 10.1094/MPMI.2002.15.2.120
Vargas, W. A., Martín, J. M., Rech, G. E., Rivera, L. P., Benito, E. P., Díaz-Mínguez, J. M., et al. (2012). Plant defense mechanisms are activated during biotrophic and necrotrophic development of Colletotricum graminicola in maize. Plant Physiol. 158, 1342–1358. doi: 10.1104/pp.111.190397
Keywords: Colletotrichum acutatum, evolution, fungal lifestyle, pathogenicity, anthracnose
Citation: Baroncelli R, Talhinhas P, Pensec F, Sukno SA, Le Floch G and Thon MR (2017) The Colletotrichum acutatum Species Complex as a Model System to Study Evolution and Host Specialization in Plant Pathogens. Front. Microbiol. 8:2001. doi: 10.3389/fmicb.2017.02001
Received: 18 July 2017; Accepted: 28 September 2017;
Published: 11 October 2017.
Edited by:
John R. Battista, Louisiana State University, United StatesCopyright © 2017 Baroncelli, Talhinhas, Pensec, Sukno, Le Floch and Thon. This is an open-access article distributed under the terms of the Creative Commons Attribution License (CC BY). The use, distribution or reproduction in other forums is permitted, provided the original author(s) or licensor are credited and that the original publication in this journal is cited, in accordance with accepted academic practice. No use, distribution or reproduction is permitted which does not comply with these terms.
*Correspondence: Pedro Talhinhas, cHRhbGhpbmhhc0Bpc2EudWxpc2JvYS5wdA==