- 1Infectious Diseases Research Centre-CRI, Research Center of the CHU de Québec, Université Laval, Quebec, QC, Canada
- 2Maisonneuve-Rosemont Hospital Research Center, Montreal, QC, Canada
- 3Department of Medicine, Université de Montréal, Montreal, QC, Canada
- 4Medical Microbiology and Infectious Diseases, Research Center of the CHU de Québec, Quebec, QC, Canada
- 5Department of Microbiology, Infectious Disease and Immunology, Faculty of Medicine, University Laval, Quebec, QC, Canada
Current antifungal drugs suffer from limitations including toxicity, the emergence of resistance and decreased efficacy at low pH that are typical of human vaginal surfaces. Here, we have shown that the antipsychotic drug valproic acid (VPA) exhibited a strong antifungal activity against both sensitive and resistant Candida albicans in pH condition similar to that encountered in vagina. VPA exerted a strong anti-biofilm activity and attenuated damage of vaginal epithelial cells caused by C. albicans. We also showed that VPA synergizes with the allylamine antifungal, Terbinafine. We undertook a chemogenetic screen to delineate biological processes that underlies VPA-sensitivity in C. albicans and found that vacuole-related genes were required to tolerate VPA. Confocal fluorescence live-cell imaging revealed that VPA alters vacuole integrity and support a model where alteration of vacuoles contributes to the antifungal activity. Taken together, this study suggests that VPA could be used as an effective antifungal against vulvovaginal candidiasis.
Introduction
Candida albicans is the major human fungal pathogens and also a component of the normal human flora, colonizing primarily mucosal surfaces, gastrointestinal and genitourinary tracts, and skin (Berman and Sudbery, 2002). Although many infections involve unpleasant but non-life-threatening colonization of various surface of mucosal membranes, immunosuppressed patients can fall prey to serious mucosal infections, such as oropharyngeal candidiasis in HIV patients and newborns, and lethal systemic infections (Odds, 1987). C. albicans followed by C. glabrata are natural components of the vaginal fungal microbiota and, opportunistically, the leading causative agents of vulvovaginal candidiasis (VVC). VVC affects 70–75% of childbearing women at least once, and 40–50% of them will experience recurrence (Sobel, 2007).
Topical azoles-based antifungal formulations (e.g., fluconazole, clotrimazole, miconazole, or butoconazole) such as vaginal suppositories, tablets, and cream are widely used to treat VVC. However, their efficiency is questioned especially for C. glabrata who is intrinsically resistant to azoles. Furthermore, VVC are often caused by C. albicans azole-resistant strains (Sobel, 2007; Marchaim et al., 2012). Importantly, antifungals used for VVC treatments had to fulfill the constraint of remaining effective at acidic pH (4–4.5), which is the normal pH of human vaginal surfaces. Recent studies had proven that the acidic pH increases the minimal inhibitory concentrations (MICs) of several antifungals including azoles, amphothericin B, ciclopirox olamine, flucytosine, and caspofungin for C. albicans (Danby et al., 2012). Pai and Jones reported a similar finding in C. glabrata where MICs of triazoles were increased in pH 6 as compared to pH 7.4 (Pai and Jones, 2004). Taken together, these data demonstrate that in addition to the complications related to the acquired or the intrinsic-resistance to conventional antifungals, reduction of antifungal potency at acidic pH can further complicate the treatments of VVC. Due to the fact that the antifungal discovery pipelines of pharmaceutical companies are almost dry, there is an urgent need to identify novel low pH-effective antifungal molecules for VVC therapeutic intervention.
Valproic acid (VPA), is a branched short-chain fatty acid well-known as a class I/II histone deacetylase inhibitor (HDACi) (Gottlicher et al., 2001; Phiel et al., 2001). VPA is widely prescribed as antipsychotic to treat epilepsy, bipolar disorder, and uncontrolled seizures (Privitera et al., 2006). The antifungal properties of VPA has been previously reported against different opportunistic fungi causing infections of the central nervous system (Galgoczy et al., 2012; Homa et al., 2015). Despite the growing interest on VPA as antifungal, its precise mechanism of action remains not clear. Recent investigations in the budding yeast Saccharomyces cerevisiae have shown that VPA induces apoptosis and inhibits both cell-cycle at the G1-S transition and the activation of the cell wall integrity pathway, Stl2 MAP kinase (Mitsui et al., 2005; Desfosses-Baron et al., 2016). VPA was also shown to cause inositol depletion which in turn led to vacuolar ATPase perturbation (Ju and Greenberg, 2003; Deranieh et al., 2015). In Schizosaccharomyce pombe, VPA acts as an HDACi and disturbs different cellular processes including calcium homeostasis, cell wall integrity, and membrane trafficking (Miyatake et al., 2007; Zhang et al., 2013).
We have recently shown that low pH strongly potentiates VPA antimicrobial activity against the model yeast S. cerevisiae (Desfosses-Baron et al., 2016). Here, we investigated the in vitro susceptibility of both planktonic and sessile cells of different sensitive and resistant clinical isolates of the opportunistic yeast C. albicans to VPA using conditions mimicking the vaginal environment. The effect of VPA on the ability of C. albicans to cause damage to vaginal epithelial cells were investigated. Drug synergy between VPA and 11 standard antifungal agents were also explored. In attempt to gain insight into the mechanism of action associated with the antifungal activity of VPA a genetic screen was undertaken to uncover mutations conferring hypersensitivity to VPA.
Materials and Methods
Fungal Strains, Media, and Chemicals
The fungal clinical and laboratory strains used in this study are listed in the Tables S1, S2, respectively. C. albicans and other yeast strains were routinely maintained at 30°C on YPD (1% yeast extract, 2% peptone, 2% dextrose, with 50 mg/ml uridine) or synthetic complete (SC; 0.67% yeast nitrogen base with ammonium sulfate, 2.0% glucose, and 0.079% complete supplement mixture) or RPMI (RPMI-1640 with 0.3 g/L-glutamine) media. Acidic pHs used for VPA susceptibility were obtained using hydrochloric acid.
Valproic acid (VPA; Sigma-P4543) was dissolved in sterile water (50 mg/ml). Standard antifungals used for VPA-synergy assessment are: Fluconazole (FCZ; Sigma-F8929), Caspofungin (CSP; Sigma-SML0425), Voriconazole (VCZ; Sigma-PZ0005), Amphothericin B (AMB; Sigma-A488), Itraconazole (ITZ; Sigma-I6657), Clotrimazole (CTZ; Sigma-C6019), Teroconazole (TCZ; Toronto Research Chemicals-T110600), Miconazole (MCZ; Sigma-PHR1618), Terbinafine (TRB; Sigma-T8826), Nystatin (NST; Sigma-N4014), and Micafungin (MCF; McKesson Canada-205666). Antifungals were prepared using DMSO for Amphothericin B (30 mg/ml), Fluconazole (300 mg/ml), Terbinafine (10 mg/ml), Clotrimazole (9 mg/ml), Nystatine (5 mg/ml), Miconazole (30 mg/ml), Terconazole (1 mg/ml); water for caspofungin (10 mg/ml), Voriconazole (10 mg/ml), Micafungin (10 mg/ml), and chloroform for Itraconazole (50 mg/ml).
VPA Susceptibility and Time-Kill Assays
The pH-dependant effect of VPA on C. albicans was evaluated as follows: The reference clinical strain SC5314 was grown overnight in YPD medium at 30°C in a shaking incubator. Cells were then resuspended in fresh SC at an optical density at 595 nm (OD595nm) of 0.05. The pHs of SC media were adjusted using sodium hydroxide or hydrochloric acid for alkaline and acidic pHs, respectively. A total volume of 99 μl C. albicans cells was added to each well of a flat-bottom 96-well plate in addition to 1 μl of the corresponding stock solution of VPA. Plates were incubated in a Sunrise-Tecan plate reader at 30°C with agitation and OD595nm readings were taken every 10 min over 24 h. Experiments were performed in triplicate, and average values were used for analysis. VPA effect on other fungal species at acidic pH was performed in a similar fashion.
The Minimal Inhibitor Concentration (MIC) was determined following the CLSI recommendations (CLSI, 2008). Briefly, 50 μl of VPA or standard antifungals at two-fold the final concentration prepared in RPMI was serially diluted in flat-bottom 96-well plates (Costar-Corning) and combined with 50 μl of an-overnight culture of C. albicans and other yeasts at 104 cell/ml. Plates were incubated at 30°C with shaking and OD595nm readings were taken after 24 h using the Sunrise-Tecan plate reader. The MIC was determined as the first well with growth reduction of >10% based on OD595nm values in the presence of VPA or conventional antifungals as compared to untreated control cells. Time-kill was performed as described by Sanglard et al. (2003). Briefly, C. albicans SC5314 strain cultures were grown in RPMI pH 4.5 at 30°C under shaking in the presence of different concentration of VPA for defined time periods (6, 24, and 48 h). Fractions of cultures were removed at each exposition time and the colony forming units (CFU) counts were ensured by serial dilution in YPD-agar.
Synergism Assay
Evaluation of synergistic interactions between VPA and standard antifungals was performed using RPMI-1640 medium buffered at pH 4.5. Synergism was assessed by calculating the fractional inhibitory concentration (FIC) index as described by Epp et al. (2010). The FIC index was calculated as follows: (MIC of VPA in combination/MIC of VPA alone) plus (MIC of a standard antifungal in combination/MIC of a standard antifungal alone).
Biofilm Formation and XTT Reduction Assay
Biofilm formation and XTT (2,3-bis(2-methoxy-4-nitro-5-sulfo- phenyl)-2H-tetrazolium-5-carboxanilide) assays were carried out as previously described by Askew et al. (2011). Overnight YPD cultures were washed three times with PBS and resuspended in fresh RPMI supplemented with L-glutamine (0.3 g/l) to an OD595nm of 1. C. albicans yeast cells were allowed to adhere to the surface of 96-well polystyrene plate for 3 h at 37°C in a rocking incubator. Non-attached cells were washed from each well three times with PBS and fresh RPMI supplemented with VPA was added for 24 h at 37°C for biofilm formation. The plates were then washed and fresh RPMI supplemented with 100 μl of XTT-menadione (0.5 mg/ml XTT in PBS and 1 mM menadione in acetone) was added. After 3 h incubation on the dark at 37°C, 80 μl of the resulting colored supernatants were used for colorimetric reading (OD490nm) to assess metabolic activity of biofilms. A minimum of four replicates were at least performed.
Vaginal Epithelial Cell Damage Assay
Damage of vaginal epithelial cells was assessed using the lactate dehydrogenase (LDH) cytoxicity detection kit (Sigma) based on the release of LDH in the surrounding medium following the manufacturer's protocol. VK2/E6E7 (ATCC- CRL-2616) vaginal epithelial cell line was grown on a keratinocyte-serum free medium (supplemented with 0.1 ng/ml recombinant epidermal growth factor and 50 μg/ml bovine pituitary extract) as a monolayer to 95% confluency on a 96-well culture plate and incubated at 37°C with 5% CO2. VK2/E6E7 cells were infected with 2 × 104 of C. albicans SC5314 blastospores for 24 h. A total of 100 μl supernatant was removed from each experiment and LDH activity in this supernatant was determined by measuring the absorbance at 490 nm (OD490nm). LDH activity were calculated as the mean of, at least, three independent biological replicates.
Genetic Screen for VPA-Sensitive Mutants
A total of 2371 mutants from the transcription factors (Homann et al., 2009) (365 strains), transcriptional regulators (Vandeputte et al., 2012) (509 strains), kinases (Blankenship et al., 2010) (165 strains), and generalist collections (Noble et al., 2010) (1,332 strains) were screened for VPA-sensitivity. These mutant libraries were obtained from the Fungal Genetics Stock Center (FGSC). With the exception of the kinase collection where genes were disrupted by transposon insertions, mutants of the other collections were created through gene deletion of the complete ORF. In most cases and for each gene, at least two independent transformants were screened. Mutant strains were grown overnight in SC with pH 4.5 on flat-bottom 96-well and were plated on SC-agar pH 4.5 medium with or without VPA (50 μg/ml) using a 96-well blot-replicator. Mutants exhibiting more than fold-fold growth reduction based on colony diameter were compiled together in a 96-well plate and their sensitivity were confirmed to different concentration of VPA (10, 50, and 100 μg/ml) following the same procedure. Mutant strain with established VPA sensitivity were individually reconfirmed by serial dilution spot assay. A complete listing of VPA-sensitive mutants is shown in Table S3. The overrepresentation of specific GO terms associated with the function of gene required for VPA tolerance was determined with GO Term Finder using a hypergeometric distribution with multiple hypothesis correction (http://www.candidagenome.org/cgi-bin/GO/goTermFinder) (Inglis et al., 2012). Descriptions related to gene function in Table S3 were extracted from CGD (Candida Genome Database) database (Inglis et al., 2012).
Confocal Microscopy and Vacuole Integrity
C. albicans vacuole integrity was assessed using the lipophilic vacuole membrane dye MDY-64 (Molecular probes, Fisher Scientific) following the manufacturer's recommended procedure. Briefly, cells were grown overnight on RPMI liquid medium with pH 4.5 at 30°C. Cells were pelleted and washed twice with fresh RPMI pH 4.5 and resuspended in the same medium at an OD595 of 0.1. VPA was added at different concentrations (10, 50, and 100 μg/ml). Cells were incubated for 2 h at 30°C under agitation. Aliquots were taken from VPA-treated and non-treated cultures and the MDY-64 was added at a final concentration of 10 μM. Cells were incubated at room temperature for 3 min prior to confocal microscopy visualization. Images were acquired with a 1.3-numerical-aperture (NA) 63x objective on a Leica DMI6000B inverted microscope connected to a Hamamatsu C9100-13 camera.
Pan1-green fluorescent protein (GFP), End3-GFP and LIFEACT-GFP (Epp et al., 2013) were visualized using confocal microscopy as follow: an overnight culture was diluted in SC supplemented with 10 or 50 μg/ml VPA to an OD595nm of 0.05 and grown for four generations at 30°C under agitation. Cells were imaged as described for the vacuole staining experiments.
Results
Antifungal Activity of VPA Is pH-Dependant
Antifungal activity of VPA on C. albicans was evaluated by monitoring OD595nm of cultures exposed for 24 h to increased concentration of VPA in SC media at different pHs. VPA exerted an inhibitory effect that was exaggerated in acidic pH (Figure 1A). Antifungal activity of VPA was also assessed in other clinically relevant Candida species including C. glabrata, C. tropicalis, C. parapsilosis, and C. krusei in addition to the yeast S. cerevisiae. The obtained data demonstrates that VPA inhibited the growth of all tested fungal species, with C. albicans exhibiting higher sensitivity at elevated VPA concentrations (>216 μg/ml) (Figure 1B). Thus, VPA is a potent antifungal compound against C. albicans at acidic pH.
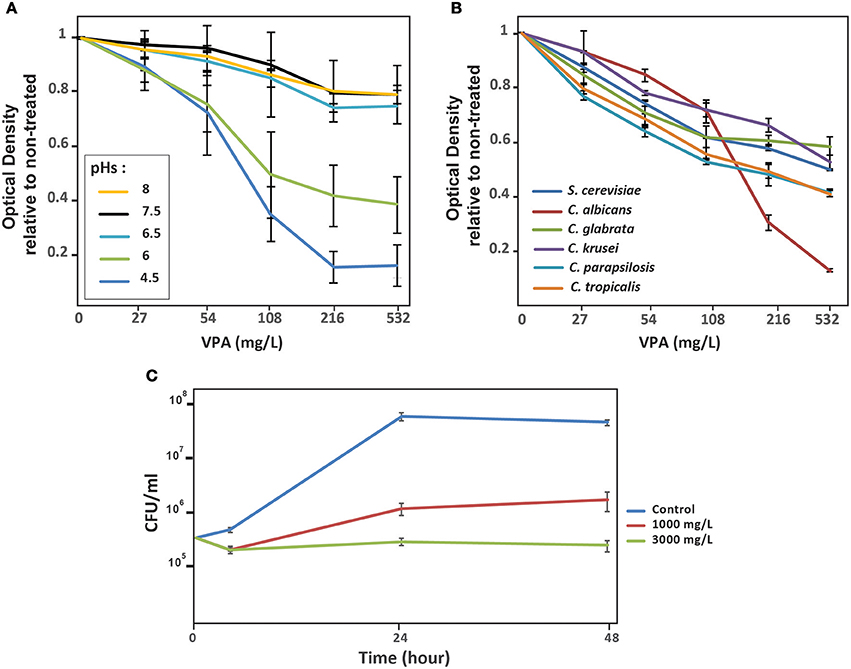
Figure 1. In vitro antifungal activity of valproic acid is pH-dependant. (A) Effect of different pHs on antifungal activity of VPA. The C. albicans SC5314 strain was grown in SC medium with different pH (4.5–8) supplemented with different concentration of VPA. SC5314 strain was grown at 30°C and OD595nm reading was taken after 24 h of incubation. ODs measurement for each VPA concentration is the mean of triplicate. (B) VPA inhibit the growth of non-albicans Candida species. C. glabrata, C. parapsilosis, C. tropicalis, C. krusei in addition to S. cerevisiae were grown in SC medium pH 4.5 with different concentration of VPA. OD595nm reading was taken after 24 h of incubation at 30°C under agitation. (C) Time-kill curve demonstrating the fungistatic activity of VPA. C. albicans SC5314 strain was exposed to two different concentrations (1,000 and 3,000 μg/ml) at different times (6, 24, and 48). CFUs were calculated as described in the method section.
To test whether VPA had fungistatic or fungicidal activity on C. albicans at acidic pH, time-kill curve assays were performed. Two high concentrations of VPA which correspond to 125x (1,000 μg/ml) and 375x (3,000 μg/ml) of the MIC for the C. albicans reference strain SC5314 (Table 1) were tested. VPA exhibited a concentration-independent fungistatic activity (Figure 1C). Lower VPA concentrations ranging from 7.8 (MIC for the SC5314 strain) and 500 μg/ml were also tested and the obtained results demonstrates a similar fungistatic activity (result not shown).
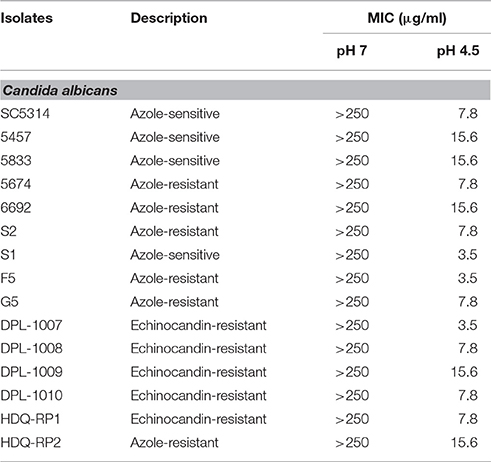
Table 1. In vitro activity of valproic acid (MIC) on C. albicans antifungal sensitive and resistant strains.
Antifungal Activity of VPA against Azole- and Echinocandin-Resistant Strains
Since VPA was highly potent against C. albicans, we wanted to test whether its antifungal activity can be expanded to other clinically sensitive and resistant strains of this yeast. Several azole-resistant strains with different resistant mechanisms, were selected (Table S1) in addition to echinocandin-resistant isolates. A total of four sensitive and 11 resistant strains (six azole- and five echinocandin-resistant strains) were examined using broth microdilution assay as specified by CLSI at both neutral or acidic pHs. The sensitivity of C. albicans isolates to VPA was pH-dependant and MICs ranged from 3.5 to 15.6 μg/mlfor both resistant and susceptible strains (Table 1). The range of MICs was also similar when comparing azole-resistant and echinocandin-resistant clinical strains separately (3.5–15.6 μg/ml). Overall, these results demonstrate that VPA may be of use to tackle therapeutic limitations related to acquired clinical resistance of C. albicans. Furthermore, comparable VPA-sensitivity in susceptible and resistant strains indicates that the mechanisms that confer resistance to azoles and echinocandins are distinct from those that may cause VPA resistance.
Valproic Acid Attenuate Damage of Vaginal Epithelial Cells Caused by C. albicans
To verify whether VPA exerts protective antifungal activity during host cell invasion, interaction of C. albicans with the human epithelial vaginal cell line VK2/E6E7 were performed as described in the method section. C. albicans-mediated damage of VK2/E6E7 cells were quantified based on the LDH release. Two different concentrations of VPA (7.8 and 78 μg/ml) corresponding to the MIC and 10x MIC for C. albicans SC5314 strain were used. In accordance with our in vitro data, the VPA had no significant protective effect at pH 7 (Figure 2). At pH 5, 7.8, and 78 μg/ml of VPA prevented 55 and 100% of VK2/E6E7 damage, respectively, as compared to the control. Intermediate protective activity was perceived at pH 6 where 28 and 52% damage reduction was obtained with 7.8 and 78 μg/ml of VPA, respectively. In support of in vitro data, these results demonstrate that VPA confers a protective antifungal activity during the invasion of vaginal epithelial cells.
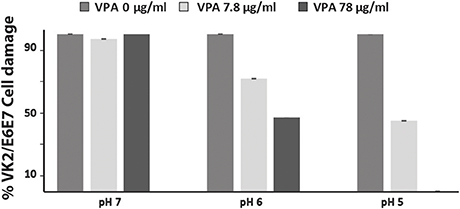
Figure 2. Valproic acid attenuate damage of vaginal epithelial cells caused by C. albicans. Damage of human epithelial vaginal cell line VK2/E6E7 infected by C. albicans SC5314 strain was assessed using LDH release assay. For each pH, cell damage was calculated as percentage of LDH activity of VPA-treated experiment relatively to that of the control experiment (C. albicans invading VK2/E6E7 cells in the absence of VPA). Results are the mean of three independent replicates.
VPA Acts Synergistically with Terbinafine in Both Susceptible and Resistant Strains
Different standard antifungals used against C. albicans and other human fungal pathogens were screened to identify drugs that could potentiate the anti-Candida activity of VPA. Interactions of VPA with other 11 antifungal agents including azoles (Fluconazole, Voriconazole, Itraconazole, clotrimazole, Terconazole, and Miconazole), polyenes (Amphothericin B and Nystatin), echinocandins (Caspofungin and Micafungin), and the allylamine, Terbinafine were tested. Based on the appreciation of the FIC index in the clinical strain SC5314, VPA was found to exhibit an apparent synergistic interaction with terbinafine (Table 2; FIC index < 0.5). VPA-terbinafine combinations were also synergistic in azole and echinocandin resistant clinical strains (Table 2).
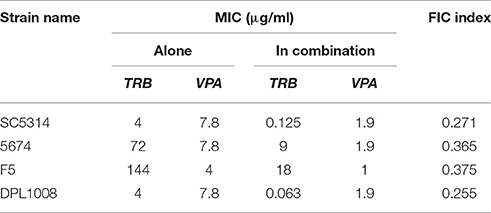
Table 2. Synergistic interaction of valproic acid with the allylamine antifungal, terbinafine on sensitive, and azole- and echinocandin-resistant strains.
VPA Inhibits Biofilm Formation in Both Susceptible and Resistant Strains
The effect of VPA on biofilm formation was evaluated using the metabolic colorimetric assay based on the reduction of XTT at acidic and neutral pHs. At neutral pH, no VPA anti-biofilm activity was noticed of all tested concentrations for the C. albicans SC5314 reference strain (not shown). In contrast, at pH 4.5, biofilm inhibition was apparent at 1.44 μg/ml of VPA with ~5% of inhibition as compared to the control (Figure 3). The MIC of VPA on the SC5314 strain was evaluated at 7.2 μg/ml. The effect of VPA on biofilm formation was also tested in two azole-resistant strains (S2 and F5) with different resistance mechanisms in addition to two echinocandin-resistant isolates (DPL-1008 and DPL-1010). As for the SC5314 sensitive strain, the four resistant strains exhibited a clear reduction in metabolic activity at 1.44 μg/ml of VPA (Figure 3). The MIC values for the azole-resistant strains were similar (2.88 μg/ml VPA) and slightly decreased as compared to the SC5314 susceptible strain. The two echinocandin-resistant strains DPL-1008 and DPL-1010 were highly sensitive to VPA as compared to other strains and their MIC was noticed at 1.44 μg/ml of VPA. These results demonstrate that, in addition to its antifungal activity on planktonic cells, VPA is also active on sessile forms of C. albicans at acidic pH.
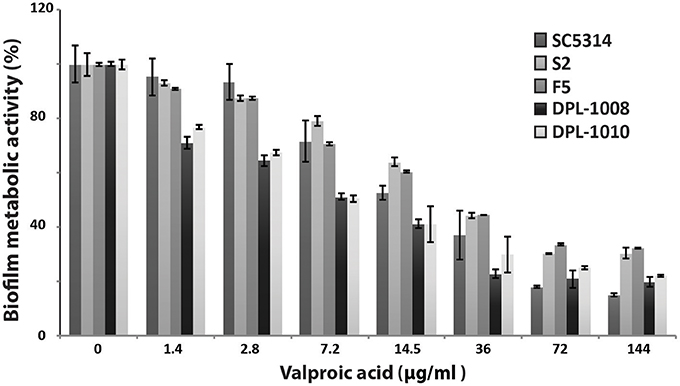
Figure 3. Anti-biofilm activity of valproic acid. The effect of VPA on biofilm formation was evaluated using the metabolic colorimetric assay based on the reduction of XTT at pH4. Sensitive (SC5314) and azole- (S2 and F5), and echinocandin-resistant (DPL-1008 and DPL-1010) C. albicans strains were tested. Results represent growth inhibition (%) and are the mean of at least three independent replicates.
Mutants Defective in Vacuolar Functions Are Hypersensitive to VPA
To gain insight into the mechanism of action of VPA associated with its antifungal property, a comprehensive regulatory and generalist mutant collections of C. albicans were screened for their sensitivity to VPA. Among the 947 unique mutants that were screened, 55 were confirmed to be hypersensitive to VPA (Table S3). To identify the functional categories that are associated with mutations affecting VPA susceptibility, we performed gene ontology (GO) enrichment analysis. Our data demonstrated that VPA sensitive mutants are defective in genes related primarily to vacuole transport (p = 1.72e-08) and organization (p = 8.86e-09) (Table 3, Table S4). This include mutants of vacuolar protein sorting (vps15, vps34, vps64, and ypt72), proteins associated with the retromer complex (pep7 and pep8), and proteins required for vacuole inheritance, and organization (cla4, pep12, vam6, vps41, and pep12). Requirement of vacuolar functions for VPA tolerance was also reported previously in S. pombe (Zhang et al., 2013) and S. cerevisiae (Deranieh et al., 2015) where genome-wide screens demonstrated that retromer complex and vacuolar ATPases, respectively, were associated with VPA sensitivity. Taken together, our chemogenetic screen provides a rational for mechanistic investigation into the effect of VPA on fungal vacuole.
VPA Alters Vacuole Morphology
Our chemogenetic screen demonstrated clearly that C. albicans sensitivity to VPA were exaggerated in mutant of vacuolar transport, organization and inheritance. The requirement of intact vacuolar pathways for VPA tolerance suggests that VPA might alters the function and/or the integrity of the vacuole. To verify this hypothesis, the integrity of C. albicans vacuoles were assessed using the vacuole membrane marker, MDY-64, in cells treated or not with different concentrations of VPA at pH 4.5. A dominant fraction of non-treated cells internalized the MDY-64 dye and exhibited well-structured vacuoles with two to four compartments comprising discernable lumens (Figure 4A). However, cells treated with either 10 or 50 μg/ml of VPA displayed an altered vacuole structure with a foamy fluorescence pattern and indistinguishable lumens (Figure 4B). These findings suggest that VPA affect the morphology and the integrity of vacuoles in C. albicans.
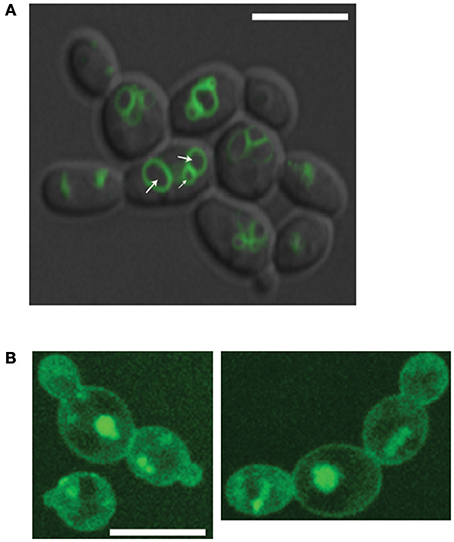
Figure 4. Valproic acid alters vacuolar morphology. C. albicans SC5314 strains was grown in RPMI pH 4.5 in the absence (A) or presence of 50 μg/ml of VPA (B) and stained for 3 min with the vacuole membrane marker, MDY-64. Cells were visualized using confocal microscopy. The white arrows indicate representatively intact vacuole lumens. Fluorescence PMT gain were increased five times for VPA-treated cells due to low incorporation of MDY-64. Bars, 8 μm.
VPA-Induced Vacuolar Phenotypes Are Not a Consequence of Endocytosis, Actin Filaments Perturbations, or Inositol Depletion
Perturbation of vacuoles by VPA might be either a direct consequence where VPA act in situ on vacuole or by impacting indirectly other process required for proper vacuole biogenesis and organization. Indeed, vacuolar integrity and homeostasis depends on the proper functioning of different cellular processes including actin filaments organization (Eitzen et al., 2002), endocytosis (Michaillat and Mayer, 2013), and the phosphatidylinositol phosphate signaling (Michell et al., 2006). Recent work by Deranieh et al. (2015) indicated that VPA led to cellular depletion of inositol, which disrupts the vacuolar phosphoinositide, PI(3,5)P2 (Phosphatidylinositol 3,5-bisphosphate) homeostasis and consequently compromise vacuole morphology. In this regard, we checked whether VPA-mediated vacuole alteration can be bypassed by inositol supplementation or deprivation. Our data demonstrate that the altered vacuole phenotype was not influenced by lack or excess of inositol (data not shown), suggesting that the probable VPA-induced inositol depletion in C. albicans is unlikely to account for the toxicity of this compound under our conditions.
To check whether the vacuole alteration caused by VPA is related to a defect in endocytosis or actin filament organization, we have used a Pan1- and End3-GFP fusions as clathrin-coated vesicles markers, and LIFEACT-GFP (Epp et al., 2013) to monitor actin patches and cables. VPA treatment did not cause any apparent alteration of the organization of actin filaments or endocytic vesicles (Figure S1). Taken together, these results support that the direct alteration of vacuole is the cellular mechanism underlying the antifungal activity of VPA.
Discussion
Candida pathogenic species are adapted to survive in different acidic environments inside their host such as the vagina, inflammatory foci like abscesses (Park et al., 2012) and phagolysosomes of neutrophils and macrophages (Erwig and Gow, 2016). In such acidic condition, several studies demonstrated that the in vitro activity of standard antifungals is compromised as evidenced by the increase of their MICs (Marr et al., 1999; Pai and Jones, 2004; Danby et al., 2012). In the current study, we demonstrated that the antifungal activity of the VPA, a histone deacetylase inhibitor and the widely prescribed as antipsychotic, is potentiated at acidic pH that resemble to that of host niches cited above. We also demonstrated that VPA potentiates the antifungal activity of the widely prescribed terbinafine at acidic pH. In this regard, VPA, alone or with terbinafine, may be useful against fungal vaginosis caused primarily by C. albicans. VPA was also found to be effective against both echinocandin- and azole-resistant strains suggesting that this molecule represents an alternative solution to circumvent VVC or recurrent VVC caused by C. albicans strains that are resistant to standard antifungals. In the current study, VPA were also potent against C. albicans biofilm in a similar fashion as for planktonic cells and for both sensitive and clinical resistant strains. As for vaginal bacterial pathogens, C. albicans is able to form infective biotic biofilms on the vaginal mucosal surfaces (Harriott et al., 2010). Due to the fact that biofilm growth is impervious to all conventional antifungals, and since efficiency of these drugs is compromised at acidic pH, VPA may represent thus a promising alternative for antibiofilm therapy.
Importantly, this work supports a direct clinical repurposing of VPA as an antifungal against VVC or recurrent VVC due to the fact that its safety profile has been extensively characterized in vivo over the past decades of its clinical use in systemic forms as anticonvulsant (Lagace et al., 2004) or anticancer (Gupta et al., 2013). VPA had also a broad therapeutic safety margin when used topically (Choi et al., 2013). It does not cause skin irritations such as erythema and edema and had no toxicity to different human cells including keratinocytes, fibroblasts, and mast cells (Choi et al., 2013). In the current work, we also find that VPA did not impair the growth and the integrity of the vaginal epithelial cells VK2/E6E7 as judged by the LDH cytotoxicity assay (Figure S2). While a whole animal vaginal model is required to confirm that VPA does not cause vaginal irritations, the aforementioned studies are supportive of a safe use of VPA topically against VVC.
It is intriguing that the antifungal activity of VPA was acidic pH-dependant. This could be explained by the chemical nature of VPA, which is an eight-carbon branched-chain acid with proprieties of weak acid (pKa 4.8). Low pH is expected to decrease its ionization state and increase its liposolubility, which in turn may facilitate the passage through the plasma membrane and its accumulation in the cells. Future structure-guided medicinal chemistry approach by introducing structural changes in VPA that can lead to beneficial biological activity in a pH-independent manner will allow expanding the potential use of this molecules form VVC and recurrent VVC to treat oral C. albicans infections and even systemic candidiasis.
In the current study, we undertook a chemogenetic screen to delineated biological process that underlies VPA-sensitivity in C. albicans. This screen enables the identification of different vacuole-related functions as being required to tolerate VPA and provide thus a rational to examine the effect of this molecule on fungal vacuole. Our data demonstrates clearly that VPA antifungal activity is a consequence of the impairment of vacuole integrity and illuminate thus a previously unappreciated mechanism of action of this drug. Recent work in S. cerevisiae indicates that cellular depletion of inositol by VPA disrupts the vacuolar phosphoinositide, PI3,5P2 homeostasis which compromise the function of V-ATPase activity and proton pumping (Deranieh et al., 2015). This V-ATPase phenotype was rescued by supplementing the growth medium by inositol. Despite the requirement of V-ATPases to tolerate VPA in S. cerevisiae, the authors did not report any alteration of the vacuolar morphology by VPA as seen in our investigation. Furthermore, the vacuole defects in C. albicans were not recovered by adding inositol to the growth medium suggesting that VPA may act via a different mechanism in this pathogenic yeast. Similarly, in S. pombe, genetic screens revealed that mutant of genes operating in Golgi-endosome membrane trafficking and vacuole retromer complex were hypersensitive to VPA (Miyatake et al., 2007; Ma et al., 2010; Zhang et al., 2013), however, no apparent alteration of vacuole was seen in this yeast model.
Regardless of the exact vacuolar process that is targeted by VPA, our study reinforces the fact that pharmacological perturbation of vacuole leads to fungal growth inhibition and is protective for host cells. Different C. albicans vacuolar proteins has been previously characterized and linked to the ability to infect the host and to control different virulence traits including biofilm formation, filamentation, and resistance to antifungals. This include for instance vacuolar membrane and cytosolic V-ATPases (Vma2, Vma3, and Vph1) (Patenaude et al., 2013; Rane et al., 2013, 2014), proteins mediating vesicular trafficking to the vacuole (Pep12, Vps11, and Vps21) (Palmer et al., 2005; Johnston et al., 2009; Palanisamy et al., 2010; Wachtler et al., 2011) and the vacuolar calcium channel, Yvc1 (Wang et al., 2011). This makes the vacuole an ideal therapeutic target to manage fungal infections. However, the functional resemblance of fungal vacuoles with their human counterpart organelle, lysosomes, raises uncertainty regarding their druggability. Indeed, while the two V-ATPase inhibitors bafilomycin A1 and concanamycin A from Streptomyces, exhibit a potent activity against C. albicans they also compromise the activity of the mammalian V-ATPases (Olsen, 2014). Meanwhile, the fungal vacuoles had distinctive proteins such as the V0-ATPase subunit with no apparent human homologs that could be specifically targeted for pharmacological interventions in the treatment of fungal infections. In this regard, we demonstrate that VPA had no cytotoxicity on vaginal epithelial cells at concentrations above 10 times the MIC of C. albicans suggesting that VPA-mediated vacuole alteration is fungus-specific (Figure S2).
In conclusion, we have shown that VPA is a potent antifungal at acidic pH and consequently an attractive therapeutic molecule against vulvovaginal candidiasis. We have also described an unreported effect of VPA on the structural integrity of fungal vacuoles which might be the main cause of its cytotoxicity.
Author Contributions
AS designed the experiments; JC, FT, CG performed the experiments; JC, FT, CG, HW, RP, and AS analyzed the data; AS and JC wrote the manuscript with the help of all authors.
Conflict of Interest Statement
A provisional patent application for an antifungal vaginal gel formulation related to this work (Patent number US 62/532,603) has been filled by Université Laval. The authors declare that the research was conducted in the absence of any commercial or financial relationships that could be construed as a potential conflict of interest.
Acknowledgments
We thank Hassan Badrane and Cornelius Clancy (University of Pittsburgh) for providing strain irs4, Jaideep Mallick and Malcolm Whiteway (Concordia University-Montréal) for providing strains LIFEACT, Pan1-GFP, and End3-GFP, Sandra Weber and Martine Raymond (IRIC-Université de Montréal) for providing C. albicans clinical strains (5457, 5833, 5674, and 6692), Philippe Dufresne (Laboratoire de santé publique du Québec) for providing DPL-1007,−1008,−1009, and−1010) and Ingrid Saba and Stéphane Bolduc (LOEX-CHUQ, Université Laval) for the VK2/E6E7 cell line. A provisional patent application for an antifungal vaginal gel formulation related to this work (Patent number US 62/532,603) has been filled by Université Laval. This work was supported by the Fonds de Recherche du Québec-Santé (FRQS) (Établissement de jeunes chercheurs), the Canada Foundation for Innovation (CFI- # 34171) and Fondation de l'Université Laval. AS is a recipient of the Fonds de Recherche du Québec-Santé (FRQS) J1 salary award. JC received a Faculty of Medicine Ph.D. scholarship from Université Laval. HW is a recipient of the Fonds de Recherche du Québec-Santé (FRQS) J2 salary award.
Supplementary Material
The Supplementary Material for this article can be found online at: https://www.frontiersin.org/articles/10.3389/fmicb.2017.01956/full#supplementary-material
References
Askew, C., Sellam, A., Epp, E., Mallick, J., Hogues, H., Mullick, A., et al. (2011). The zinc cluster transcription factor Ahr1p directs Mcm1p regulation of Candida albicans adhesion. Mol. Microbiol. 79, 940–953. doi: 10.1111/j.1365-2958.2010.07504.x
Berman, J., and Sudbery, P. E. (2002). Candida albicans: a molecular revolution built on lessons from budding yeast. Nat. Rev. Genet. 3, 918–930. doi: 10.1038/nrg948
Blankenship, J. R., Fanning, S., Hamaker, J. J., and Mitchell, A. P. (2010). An extensive circuitry for cell wall regulation in Candida albicans. PLoS Pathog. 6:e1000752. doi: 10.1371/journal.ppat.1000752
Choi, S. Y., Seop, S. Y., Hyun, M. Y., Yoo, K. H., Kim, B. J., Kim, M. N., et al. (2013). Safety evaluation of topical valproate application. Toxicol. Res. 29, 87–90. doi: 10.5487/TR.2013.29.2.087
Clinical and Laboratory Standards Institute (2008). Reference Method for Broth Dilution Antifungal Susceptibility Testing of Yeasts, Approved Standard M27-A3, 3rd Edn. Approved Standard M27-A3 (Wayne, PA: CLSI).
Danby, C. S., Boikov, D., Rautemaa-Richardson, R., and Sobel, J. D. (2012). Effect of pH on in vitro susceptibility of Candida glabrata and Candida albicans to 11 antifungal agents and implications for clinical use. Antimicrob. Agents Chemother. 56, 1403–1406. doi: 10.1128/AAC.05025-11
Deranieh, R. M., Shi, Y., Tarsio, M., Chen, Y., McCaffery, J. M., Kane, P. M., et al. (2015). Perturbation of the Vacuolar ATPase: A NOVEL CONSEQUENCE OF INOSITOL DEPLETION. J. Biol. Chem. 290, 27460–27472. doi: 10.1074/jbc.M115.683706
Desfosses-Baron, K., Hammond-Martel, I., Simoneau, A., Sellam, A., Roberts, S., and Wurtele, H. (2016). Valproate inhibits MAP kinase signalling and cell cycle progression in S. cerevisiae. Sci. Rep. 6:36013. doi: 10.1038/srep36013
Eitzen, G., Wang, L., Thorngren, N., and Wickner, W. (2002). Remodeling of organelle-bound actin is required for yeast vacuole fusion. J. Cell Biol. 158, 669–679. doi: 10.1083/jcb.200204089
Epp, E., Nazarova, E., Regan, H., Douglas, L. M., Konopka, J. B., Vogel, J., et al. (2013). Clathrin- and Arp2/3-independent endocytosis in the fungal pathogen Candida albicans. MBio 4:e00476–e00413. doi: 10.1128/mBio.00476-13
Epp, E., Vanier, G., Harcus, D., Lee, A. Y., Jansen, G., Hallett, M., et al. (2010). Reverse genetics in Candida albicans predicts ARF cycling is essential for drug resistance and virulence. PLoS Pathog. 6:e1000753. doi: 10.1371/journal.ppat.1000753
Erwig, L. P., and Gow, N. A. (2016). Interactions of fungal pathogens with phagocytes. Nat. Rev. Microbiol. 14, 163–176. doi: 10.1038/nrmicro.2015.21
Galgoczy, L., Toth, L., Viragh, M., Papp, T., and Vagvolgyi, C. S. (2012). In vitro interactions of amantadine hydrochloride, R-(-)-deprenyl hydrochloride and valproic acid sodium salt with antifungal agents against filamentous fungal species causing central nervous system infection. Acta Biol. Hung. 63, 490–500. doi: 10.1556/ABiol.63.2012.4.8
Gottlicher, M., Minucci, S., Zhu, P., Kramer, O. H., Schimpf, A., Giavara, S., et al. (2001). Valproic acid defines a novel class of HDAC inhibitors inducing differentiation of transformed cells. EMBO J. 20, 6969–6978. doi: 10.1093/emboj/20.24.6969
Gupta, S. C., Sung, B., Prasad, S., Webb, L. J., and Aggarwal, B. B. (2013). Cancer drug discovery by repurposing: teaching new tricks to old dogs. Trends Pharmacol. Sci. 34, 508–517. doi: 10.1016/j.tips.2013.06.005
Harriott, M. M., Lilly, E. A., Rodriguez, T. E., Fidel, P. L. Jr., and Noverr, M. C. (2010). Candida albicans forms biofilms on the vaginal mucosa. Microbiology 156, 3635–3644. doi: 10.1099/mic.0.039354-0
Homa, M., Galgoczy, L., Toth, E., Toth, L., Papp, T., Chandrasekaran, M., et al. (2015). In vitro antifungal activity of antipsychotic drugs and their combinations with conventional antifungals against Scedosporium and Pseudallescheria isolates. Med. Mycol. 53, 890–895. doi: 10.1093/mmy/myv064
Homann, O. R., Dea, J., Noble, S. M., and Johnson, A. D. (2009). A phenotypic profile of the Candida albicans regulatory network. PLoS Genet. 5:e1000783. doi: 10.1371/journal.pgen.1000783
Inglis, D. O., Arnaud, M. B., Binkley, J., Shah, P., Skrzypek, M. S., Wymore, F., et al. (2012). The Candida genome database incorporates multiple Candida species: multispecies search and analysis tools with curated gene and protein information for Candida albicans and Candida glabrata. Nucleic Acids Res. 40, D667–D674. doi: 10.1093/nar/gkr945
Johnston, D. A., Eberle, K. E., Sturtevant, J. E., and Palmer, G. E. (2009). Role for endosomal and vacuolar GTPases in Candida albicans pathogenesis. Infect. Immun. 77, 2343–2355. doi: 10.1128/IAI.01458-08
Ju, S., and Greenberg, M. L. (2003). Valproate disrupts regulation of inositol responsive genes and alters regulation of phospholipid biosynthesis. Mol. Microbiol. 49, 1595–1603. doi: 10.1046/j.1365-2958.2003.03641.x
Lagace, D. C., O'brien, W. T., Gurvich, N., Nachtigal, M. W., and Klein, P. S. (2004). Valproic acid: how it works. Or not. Clin. Neurosci. Res. 4, 215–225. doi: 10.1016/j.cnr.2004.09.013
Ma, Y., Sugiura, R., Zhang, L., Zhou, X., Takeuchi, M., He, Y., et al. (2010). Isolation of a fission yeast mutant that is sensitive to valproic acid and defective in the gene encoding Ric1, a putative component of Ypt/Rab-specific GEF for Ryh1 GTPase. Mol. Genet. Genomics 284, 161–171. doi: 10.1007/s00438-010-0550-7
Marchaim, D., Lemanek, L., Bheemreddy, S., Kaye, K. S., and Sobel, J. D. (2012). Fluconazole-resistant Candida albicans vulvovaginitis. Obstet. Gynecol. 120, 1407–1414. doi: 10.1097/AOG.0b013e31827307b2
Marr, K. A., Rustad, T. R., Rex, J. H., and White, T. C. (1999). The trailing end point phenotype in antifungal susceptibility testing is pH dependent. Antimicrob. Agents Chemother. 43, 1383–1386.
Michaillat, L., and Mayer, A. (2013). Identification of genes affecting vacuole membrane fragmentation in Saccharomyces cerevisiae. PLoS ONE 8:e54160. doi: 10.1371/journal.pone.0054160
Michell, R. H., Heath, V. L., Lemmon, M. A., and Dove, S. K. (2006). Phosphatidylinositol 3,5-bisphosphate: metabolism and cellular functions. Trends Biochem. Sci. 31, 52–63. doi: 10.1016/j.tibs.2005.11.013
Mitsui, K., Nakagawa, D., Nakamura, M., Okamoto, T., and Tsurugi, K. (2005). Valproic acid induces apoptosis dependent of Yca1p at concentrations that mildly affect the proliferation of yeast. FEBS Lett. 579, 723–727. doi: 10.1016/j.febslet.2004.12.051
Miyatake, M., Kuno, T., Kita, A., Katsura, K., Takegawa, K., Uno, S., et al. (2007). Valproic acid affects membrane trafficking and cell-wall integrity in fission yeast. Genetics 175, 1695–1705. doi: 10.1534/genetics.107.070946
Noble, S. M., French, S., Kohn, L. A., Chen, V., and Johnson, A. D. (2010). Systematic screens of a Candida albicans homozygous deletion library decouple morphogenetic switching and pathogenicity. Nat. Genet. 42, 590–598. doi: 10.1038/ng.605
Odds, F. C. (1987). Candida infections: an overview. Crit. Rev. Microbiol. 15, 1–5. doi: 10.3109/10408418709104444
Olsen, I. (2014). Attenuation of Candida albicans virulence with focus on disruption of its vacuole functions. J. Oral Microbiol. 6:10.3402/jom.v6.23898. doi: 10.3402/jom.v6.23898
Pai, M. P., and Jones, A. L. (2004). Altered susceptibility of Candida glabrata bloodstream isolates to triazoles at clinically relevant pH values: comparison of the NCCLS M27-A2, sensititre yeastone, and etest methods. Antimicrob. Agents Chemother. 48, 4441–4443. doi: 10.1128/AAC.48.11.4441-4443.2004
Palanisamy, S. K., Ramirez, M. A., Lorenz, M., and Lee, S. A. (2010). Candida albicans PEP12 is required for biofilm integrity and in vivo virulence. Eukaryot. Cell 9, 266–277. doi: 10.1128/EC.00295-09
Palmer, G. E., Kelly, M. N., and Sturtevant, J. E. (2005). The Candida albicans vacuole is required for differentiation and efficient macrophage killing. Eukaryot. Cell 4, 1677–1686. doi: 10.1128/EC.4.10.1677-1686.2005
Park, S. Y., Bae, D. J., Kim, M. J., Piao, M. L., and Kim, I. S. (2012). Extracellular low pH modulates phosphatidylserine-dependent phagocytosis in macrophages by increasing stabilin-1 expression. J. Biol. Chem. 287, 11261–11271. doi: 10.1074/jbc.M111.310953
Patenaude, C., Zhang, Y., Cormack, B., Kohler, J., and Rao, R. (2013). Essential role for vacuolar acidification in Candida albicans virulence. J. Biol. Chem. 288, 26256–26264. doi: 10.1074/jbc.M113.494815
Phiel, C. J., Zhang, F., Huang, E. Y., Guenther, M. G., Lazar, M. A., and Klein, P. S. (2001). Histone deacetylase is a direct target of valproic acid, a potent anticonvulsant, mood stabilizer, and teratogen. J. Biol. Chem. 276, 36734–36741. doi: 10.1074/jbc.M101287200
Privitera, M. D., Welty, T. E., Ficker, D. M., Kaplan, M. J., Szaflarski, J. P., and Cavitt, J. (2006). Clinician's Guide to Antiepileptic Drug Use. Philadelphia, PA: Lippincott Williams & Wilkins.
Rane, H. S., Bernardo, S. M., Hayek, S. R., Binder, J. L., Parra, K. J., and Lee, S. A. (2014). The contribution of Candida albicans vacuolar ATPase subunit V(1)B, encoded by VMA2, to stress response, autophagy, and virulence is independent of environmental pH. Eukaryot. Cell 13, 1207–1221. doi: 10.1128/EC.00135-14
Rane, H. S., Bernardo, S. M., Raines, S. M., Binder, J. L., Parra, K. J., and Lee, S. A. (2013). Candida albicans VMA3 is necessary for V-ATPase assembly and function and contributes to secretion and filamentation. Eukaryot. Cell 12, 1369–1382. doi: 10.1128/EC.00118-13
Sanglard, D., Ischer, F., Marchetti, O., Entenza, J., and Bille, J. (2003). Calcineurin A of Candida albicans: involvement in antifungal tolerance, cell morphogenesis and virulence. Mol. Microbiol. 48, 959–976. doi: 10.1046/j.1365-2958.2003.03495.x
Sobel, J. D. (2007). Vulvovaginal candidosis. Lancet 369, 1961–1971. doi: 10.1016/S0140-6736(07)60917-9
Vandeputte, P., Pradervand, S., Ischer, F., Coste, A. T., Ferrari, S., Harshman, K., et al. (2012). Identification and functional characterization of Rca1, a transcription factor involved in both antifungal susceptibility and host response in Candida albicans. Eukaryot. Cell 11, 916–931. doi: 10.1128/EC.00134-12
Wachtler, B., Wilson, D., Haedicke, K., Dalle, F., and Hube, B. (2011). From attachment to damage: defined genes of Candida albicans mediate adhesion, invasion and damage during interaction with oral epithelial cells. PLoS ONE 6:e17046. doi: 10.1371/journal.pone.0017046
Wang, H., Liang, Y., Zhang, B., Zheng, W., Xing, L., and Li, M. (2011). Alkaline stress triggers an immediate calcium fluctuation in Candida albicans mediated by Rim101p and Crz1p transcription factors. FEMS Yeast Res. 11, 430–439. doi: 10.1111/j.1567-1364.2011.00730.x
Keywords: Candida albicans, valproic acid, antifungal, vacuole, vulvovaginal candidiasis
Citation: Chaillot J, Tebbji F, García C, Wurtele H, Pelletier R and Sellam A (2017) pH-Dependant Antifungal Activity of Valproic Acid against the Human Fungal Pathogen Candida albicans. Front. Microbiol. 8:1956. doi: 10.3389/fmicb.2017.01956
Received: 06 August 2017; Accepted: 22 September 2017;
Published: 09 October 2017.
Edited by:
Santi M. Mandal, Indian Institute of Technology Kharagpur, IndiaReviewed by:
Divakar Sharma, Aligarh Muslim University, IndiaJason Sahl, Northern Arizona University, United States
Copyright © 2017 Chaillot, Tebbji, García, Wurtele, Pelletier and Sellam. This is an open-access article distributed under the terms of the Creative Commons Attribution License (CC BY). The use, distribution or reproduction in other forums is permitted, provided the original author(s) or licensor are credited and that the original publication in this journal is cited, in accordance with accepted academic practice. No use, distribution or reproduction is permitted which does not comply with these terms.
*Correspondence: Adnane Sellam, YWRuYW5lLnNlbGxhbUBnbWFpbC5jb20=