- 1Department of Food Sciences and Technology, University of Natural Resources and Life Science Vienna, Vienna, Austria
- 2Department of Food and Cosmetic Science, Tokyo University of Agriculture, Hokkaido, Japan
- 3Instituto de Productos Lácteos de Asturias, Spanish Higher Research Council, Villaviciosa, Spain
- 4Instituto de Lactología Industrial (UNL-CONICET), National University of the Litoral, Santa Fe, Argentina
- 5Laboratory of Microbiology, Wageningen University and Research, Wageningen, Netherlands
- 6Immunobiology Research Program, Research Programs Unit, Faculty of Medicine, University of Helsinki, Helsinki, Finland
- 7Functional Foods Forum, Faculty of Medicine, University of Turku, Turku, Finland
Novel microbes are either newly isolated genera and species from natural sources or bacterial strains derived from existing bacteria. Novel microbes are gaining increasing attention for the general aims to preserve and modify foods and to modulate gut microbiota. The use of novel microbes to improve health outcomes is of particular interest because growing evidence points to the importance of gut microbiota in human health. As well, some recently isolated microorganisms have promise for use as probiotics, although in-depth assessment of their safety is necessary. Recent examples of microorganisms calling for more detailed evaluation include Bacteroides xylanisolvens, Akkermansia muciniphila, fructophilic lactic acid bacteria (FLAB), and Faecalibacterium prausnitzii. This paper discusses each candidate's safety evaluation for novel food or novel food ingredient approval according to European Union (EU) regulations. The factors evaluated include their beneficial properties, antibiotic resistance profiling, history of safe use (if available), publication of the genomic sequence, toxicological studies in agreement with novel food regulations, and the qualified presumptions of safety. Sufficient evidences have made possible to support and authorize the use of heat-inactivated B. xylanisolvens in the European Union. In the case of A. muciniphila, the discussion focuses on earlier safety studies and the strain's suitability. FLAB are also subjected to standard safety assessments, which, along with their proximity to lactic acid bacteria generally considered to be safe, may lead to novel food authorization in the future. Further research with F. prausnitzii will increase knowledge about its safety and probiotic properties and may lead to its future use as novel food. Upcoming changes in EUU Regulation 2015/2283 on novel food will facilitate the authorization of future novel products and might increase the presence of novel microbes in the food market.
Introduction
Since ancient times, microorganisms have constituted an essential part of human nutrition and been consumed through naturally microbially fermented products containing viable bacteria, such as fermented honey, fruits, berries, and their juices and fermented products of animal origin. Without even knowing of the existence of bacteria, humans historically used them to initiate many food production processes (Selhub et al., 2014). Today, an enormous variety of fermented foods and beverages exists and provides approximately one-third of the human diet globally (Borresen et al., 2012). Certain fermented foods containing significant amounts of viable microbes, especially dairy products and fermented vegetables, are part of daily consumption by millions of people around the globe.
Studies involving new analytical technologies have generated new insights into the human and animal guts and yielded new isolates and candidates that perform special functions in this complex intestinal environment (Marchesi et al., 2016). The composition of human gut microbiota plays an important role in homeostasis and gut functionality, which are also strongly influenced by age, diet, environmental factors, disorders, diseases, and therapies. In recent decades, probiotic microorganisms have been identified as efficient modulators of intestinal microbial balance (Gómez-Gallego and Salminen, 2016). Probiotics are defined as live microorganisms that confer a health benefit to the host when administered in adequate amounts (Cammarota et al., 2014; Hill et al., 2014). These health benefits are provided through relatively common mechanisms found in the vast majority of the investigated probiotics, such as regulation of the intestinal transit, competitive exclusion of pathogens, and production of specific short chain fatty acids (SCFA). In addition, frequently observed mechanisms mostly found on a species level include vitamin synthesis, enzymatic activity, gut barrier reinforcement, and neutralization of carcinogens. The existence of a group of basic benefits shared by strains of the same species in a non-strain specific manner supports the concept of core benefits for specific species. Moreover, mechanisms described exclusively on the individual strain level provide immunological, neurological, and endocrinological effects. Such benefits in general support a healthy digestive tract and immune system (Aureli et al., 2011; Gómez-Gallego and Salminen, 2016).
Traditionally fermented foods, especially fermented dairy products, usually contain viable bacteria. The consumption of such foods is widely connected with the reduction of certain disease types (e.g., childhood obesity, type-2 diabetes, and cardiovascular diseases), improved body composition, and weight loss in adults during energy restriction (Thorning et al., 2016). The International Scientific Association for Probiotics and Prebiotics (ISAPP) panel acknowledged convincing results but also emphasized the difficulty of verifying whether the beneficial results originate from the food matrices, the (viable) microorganisms, or a combination of both. Additionally, traditional fermented foods do not qualify for the term probiotic because they may contain varying amounts and changing microbial species over time, and their microbiological composition is not completely known or controlled. A relevant example is traditional Kefir. The ISAPP, therefore, has proposed the term “containing live and active bacterial cultures” for traditional fermented foods (Hill et al., 2014).
Probiotics relevant to the human gut microbiota mainly belong to the genera Lactobacillus and Bifidobacterium, but several other microbial candidates, such as Akkermansia muciniphila and Faecalibacterium prausnitzii, could play roles in future probiotic products. Although, commensal gut microbes can provide beneficial health effects, the ISAPP panel suggested conducting strain-by-strain assessment until sufficient research data are available to grant probiotic status on the species level (Hill et al., 2014).
European Union Regulations of Microorganisms Intentionally Added to Food
The European Union (EU) is a single-market system consisting of 28 member states and the four members of the European Free Trade Association (Iceland, Liechtenstein, Norway, and Switzerland), which are required to follow European legislation as part of the EU single market. The European Food Safety Authority (EFSA) has the mandate to assess and communicate all risks associated with the food chain in the EU. The EFSA Scientific Committees and Panels are responsible for providing scientific opinions in response to requests from the European Commission, European Parliament, and EU member states. Before the EFSA was established, risk assessment was performed by Scientific Committees assisting the Directorate General for Health and Consumers (DG SANCO). DG SANCO, re-named to DG SANTE, continues to perform a crucial regulatory role regarding microorganisms in food and feed. Standing Committees representing member states, and their interests have strong influence on the work of DG SANTE (von Wright, 2012).
Amid globalization and ongoing technical progress, the number of new food products in the EU has increased significantly, and food safety has become a growing concern. A uniform regulation for novel food was needed to ensure the protection of both European consumers and the market. The introduction of novel foods is governed by Regulation 285/97/EC (European Commission, 1997b) and Regulation 2015/2283 (European Commission, 2015), covering all foods not been used in the EU before 15 May 1997. Under Regulation 2015/2283, the EFSA, rather than competent national authorities, will perform the scientific risk assessment for novel foods; the system of individual authorization is replaced by a system of generic authorization; and the Commission will manage all applicants' files and prepares proposals for the authorization of novel foods found to be safe (European Commission, 2015). To speed decision-making, the EFSA is required to issue its opinion within 9 months of the date of receipt of a valid application.
The guidance lists general requirements for every application: (1) a description; (2) compositional data; (3) production process; (4) specifications; (5) proposed uses and use levels; and (6) anticipated intake of the novel food. Applicants also have to provide information about the absorption, distribution, metabolism, excretion, nutrition, toxicology, allergenicity, and history of use of novel foods or their source. In addition, all the novel foods that belong to the group of “foods consisting of, isolated from or produced from micro-organisms, fungi or algae” must provide the following information (EFSA Panel on Dietetic Products, 2016): (1) scientific (Latin) name (family, genus, species, strain) according to the international codes of nomenclature; (2) synonyms used interchangeably with the preferred scientific name; (3) for bacteria and yeasts (unicellular organisms), verification of the species and strain identity according to internationally accepted methods; (4) origin of the organism; and (5) if available, deposition in an officially recognized culture collection with an access number.
Qualified Presumption of Safety
EFSA introduced the concept of qualified presumption of safety (QPS) to establish a generic risk assessment approach for biological agents, with the goal to simplify and harmonize assessment of notified biological agents across the EFSA's various Scientific Panels and Units. The QPS approach also enables more focused application of the available resources to agents with higher risk potential (Leuschner et al., 2010), simplifying the assessment of low-risk microorganisms. The QPS concept is intended to establish a safety assessment for microorganisms used in feed and food production. If a specific strain notified for market authorization can unambiguously be connected to a taxonomic unit on the QPS list, the necessary safety assessment steps, if any, are indicated in the list for that taxonomic unit.
Bacteria with a previous history of safe use are usually included on the QPS list. The EFSA's Panel on Biological Hazards assesses the QPS status of a strain and declares that the assigned microorganism displays either no safety concerns or minor concerns defined and addressed with qualifications as expressed in the QPS list. Microorganisms on the QPS list, therefore, need no exhaustive safety assessment except for those specified in the QPS list.
The assessment for QPS suitability is well structured and relies on the following criteria (EFSA, 2007):
(a) Taxonomic unit: This is the most crucial part of the assessment procedure. The genus and all known species, including type species, are listed and described according to their main characteristics. The evaluated taxonomic unit must be unambiguously defined. If the assessed bacterial agent cannot be connected to any known species, it will not be recommended for the QPS list. QPS status is also denied if the taxonomic identification is insufficient.
(b) Body of knowledge: This should be related to the history of use for the assessed bacterial agent, the field of application, the existence of sufficient scientific literature about the microorganism, and the possibility to avoid potential adverse effects for humans, livestock, and the wider environment.
(c) Possible pathogenicity: The bacterial agent's lack of pathogenic and virulence properties is confirmed, and the findings must be supported by clinical data and scientific literature.
(d) Description of end use: This includes the presence and viability of the microorganism in the final product.
Most bacteria recommended for the QPS list belong to the group of Gram-positive non-sporulating bacteria. This group includes many inhabitants of the digestive tract with long histories in food and feed production. For this group, the EFSA's Scientific Committee applies a generic qualification for all taxonomic units on the list, requiring that all strains not carry any transferable antimicrobial resistance unless the final product contains no viable cells (Leuschner et al., 2010). The number of recommended Gram-negative bacteria is very low, with only one, Gluconobacter oxydans, on the QPS list. Many members have a long history of safe use, but safety concerns cannot be excluded. E. coli strain Nissle 1917, for example, has long been used as a probiotic but can cause a variety of diseases and exhibits versatile virulence mechanisms. Nevertheless, opportunistic bacteria may be placed on the QPS list with additional qualifications, but pathogenic and toxin-producing bacteria are not included.
Microorganisms whose safety properties are not fully understood are subjected to safety assessments. Such assessments need to include a distinct taxonomic classification on the species or the strain level and a comprehensive strain characterization, including whole-genome sequence analysis, to identify potential virulence and antibiotic resistance genes and their horizontal transfer capabilities. However, in the case of new and recently discovered microorganisms from previously unknown microbial groups, the availability of genome sequences might not be enough to identify potential virulence or antibiotic resistance genes. These often require comparison with available data, so functional studies on these microorganisms are needed. For accurate safety evaluation, the food business operator should include the number and the viability of microorganisms in the final product by.
The QPS approach was inspired and influenced by the American Generally Recognized As Safe (GRAS) concept, but differences exist. QPS is defined as an “assumption based on reasonable evidence” (H. C. P. Directorate-General, 2003), providing a helpful assessment tool for the EFSA but, in contrast to GRAS, offers no legal status. The EFSA is responsible for providing the burden of proof, while GRAS lays the responsibility on the food business operator. GRAS, though, requires safety assessment by independent experts to the degree that another panel of independent experts would reach the same conclusions. The QPS assessment's strong focus on the absence of acquired antibiotic resistances and virulence factors is another difference between the two concepts.
Microorganisms Authorized as Novel Food in the EU
Leuconostoc mesenteroides
In January 2001, the European Commission authorized market placement of a dextran preparation produced by L. mesenteroides as a novel food ingredient in bakery products. Puracor NV (Groot-Bijgaarden, Belgium) filed the application, and the Belgian competent authorities performed the initial assessment. The Scientific Committee for Food found that the dextran preparation by L. mesenteroides was safe for human consumption up to 5% in bakery products. Dextran was identified as a highly digestible bakery ingredient with similar nutritional properties as starch (European Commission, 2001).
L. mesenteroides is a Gram-positive, non-sporulating, coccoid-shaped bacterium in the order Lactobacillales. The organism is often found on the surfaces of various plant parts and is responsible for the fermentation of white cabbage into sauerkraut. L. mesenteroides can be also found in artisanal and industrial cheeses, possibly contributing to their safety for long-term consumption (Cibik et al., 2000; D'Angelo et al., 2017). The species can metabolize a broad range of sugars; in particular, sucrose is used to build dextran, and certain strains can produce bacteriocins with anti-listerial activity (de Paula et al., 2015).
Bacillus subtilis Natto
In April 2009, the European Commission allowed placing Vitamin K2 (menaquinone), produced by B. subtilis natto, in the market as a novel food ingredient under Regulation (EC) No 258/97. NattoPharma (Oslo, Norway) requested that the Irish competent authorities place B. subtilis natto derived Vitamin K2 in the market as a novel food ingredient to be used in foods which served particular nutritional uses and which had added vitamins and minerals (European Commission, 2009). In an initial report, the Irish competent authorities required an additional assessment, so the EFSA was requested to conduct an extended assessment. The Scientific Panel on Dietetic Products, Nutrition, and Allergies concluded that B. subtilis natto is a safe source of vitamin K2.
B. subtilis natto is a Gram-positive, aerobic member of the spore-forming genus Bacillus. This species, discovered in Japan in 1906, is responsible for production of natto, a sticky fermented soy beans. Natto, produced by B. subtilis natto, has long been used in the Japanese diet, so it gained Foods for Specified Health Use approval based on its health benefits from the Japanese Ministry of Health, Labor, and Welfare. B. subtilis has a recognized history of safe use, so the EFSA granted it QPS status (EFSA Panel on Biological Hazards, 2010).
Clostridium butyricum
In December 2014, the European Commission authorized placing in the market C. butyricum CBM 588 as a novel food ingredient according to Regulation (EC) No 258/97 (European Commission, 2014). The British competent authorities performed the initial assessment after Miyarisan Pharmaceutical Co. Ltd. (Tokyo, Japan) requested using C. butyricum on the market as a novel food ingredient used in food supplements. Some beneficial effects reported for C. butyricum are related to the production of SCFA and its role in lipid metabolism (Zhao et al., 2014; Shang et al., 2016). No additional assessment was necessary because further explanations provided by the applicant addressed the objections raised by member states. C. butyricum was authorized as novel food ingredient in food supplements at a maximum dose of 1.35 × 108 CFU per day. Intervention studies revealed the health benefits of the strain, including prevention of pouchitis in patients with ulcerative colitis and decreased incidence of side effects in patients receiving therapy for the eradication of Helicobacter pylori (Shimbo et al., 2005; Yasueda et al., 2016). An antibiotic resistance profile, a study for genes encoding toxins, and an animal study were conducted to ensure the safety of the strain (Isa et al., 2016). The authorized strain is a Gram-positive, spore-forming, obligate aerobic, non-pathogenic, non-genetically modified organism. The product is characterized as a white or pale gray tablet with a specific odor and sweet taste (European Commission, 2014).
Bacteroides xylanisolvens
The most recent authorization for bacteria according to Regulation (EC) No 258/97 was granted in 2015. The European Commission approved placing pasteurized milk products fermented with B. xylanisolvens DSM 23964 in the market as a novel food in a heat-treated, non-viable form. Details concerning B. xylanisolvens are discussed in the following section.
Novel Microbes: Details and Background Regarding Safety
Bacteroides xylanisolvens DSM 23964
In 2015, pasteurized milk products fermented with B. xylanisolvens DSM 23964 were approved as a novel food under Novel Food Regulation No 258/97. Usage of this specific strain was restricted as the starter culture in the fermentation of pasteurized milk products. Only heat-treated and therefore inactivated cells of B. xylanisolvens were allowed in the final product (EFSA Panel on Dietetic Products, Nutrition and Allergies, 2015). In addition to the novel food evaluation, the EFSA automatically assessed B. xylanisolvens for admission to the QPS list. Interestingly, the EFSA revealed that the available information was not sufficient to include it in the QPS list.
The genus Bacteroides is a main inhabitant of the human colon, on average accounting for ~30% of intestinal microbiota, although large inter-individual variability exists (Sears, 2005). Bacteroides spp. is a Gram-negative, obligately anaerobic, bile resistant, non-spore forming rod originally isolated from human stool. This genus can have commensal attributes within the human body but can also promote the development of different diseases. Bacteroides xylanisolvens plays a major part in the fermentation and degradation of xylan and other plant fibers (Chassard et al., 2008). Bacteroides start colonization of the gut in the newborn child ~10 days after birth, (Gregory et al., 2015). Polysaccharides in the human diet are an important nutrition source for gut commensals, including Bacteroides spp. They can be metabolized into short chain fatty acids and branched chain fatty acids that are reabsorbed through the large intestine and provide an essential part of the host's daily required energy (Hooper et al., 2002).
The levels of Bacteroidetes and Firmicutes seem to be connected to obesity. Obese individuals show lower levels of Bacteroidetes and higher levels of Firmicutes in the gastro-intestinal tract. Turnbaugh and his colleagues (Turnbaugh et al., 2006) suggested that a higher Bacteroidetes/Firmicutes ratio can extract more energy from a given diet and that increased Bacteroidetes levels in the gut led to weight loss in people with obesity.
While investigating xylan-degrading microorganisms in the human gastro-intestinal tract, Chassard et al. (2008) isolated 6 xylanolytic, Gram-negative anaerobic rods. Subsequent 16S rRNA analysis revealed that the obtained strains belonged to the genus Bacteroides, and after proper analysis, the 6 strains were considered to be novel species, and the researchers proposed the name B. xylanisolvens based on their xylan-degrading properties. The main characteristics of the designated type strain are shown in Table 1.
The German Federal Institute for Occupational Safety in Health (Bundesanstalt für Arbeitsschutz und Arbeitsmedizin, BAuA) assessed the safety of B. xylanisolvens as a working material (BAuA, 2011). Due to the lack of pathogenicity and diseases, the agency classified the microorganism in the lowest risk group. The recently approved strain B. xylanisolvens DSM 23964 was first analyzed by Ulsemer and colleagues in 2011 (Ulsemer et al., 2012a,b). They isolated this strain from human feces and performed several analytical techniques to verify that it was new. Biochemical characteristics (no catalase activity, no indole production, and incapable of degrading starch), phylogenetic analysis, and DNA-DNA hybridization classified the strain as B. xylanisolvens. So far, the performed analyses have established no differences between the new strain and the type strain. Finally, the Random Amplification of Polymorphic DNA profile revealed significant differences, confirming the existence of the new strain B. xylanisolvens DSM 23964.
Scientific Opinion of the EFSA Panel on Bacteroides xylanisolvens DSM 23964
Following a request from the European Commission, the EFSA Panel on Dietetic Products, Nutrition and Allergies carried out additional assessment for pasteurized milk products fermented with B. xylanisolvens DSM 23964 as a novel food under Regulation (EC) No 258/97 (European Commission, 1997b). This strain has no history of use in the food industry, and no strain in the genus Bacteroides has a proven history of use in food production. The novel food status is related to low-fat and skimmed milk products fermented with B. xylanisolvens DSM 23964 as the starter culture. After fermentation, the product is heat treated for 1 h at 75°C, and no viable cells of B. xylanisolvens are found in the final product.
The relevant phenotypic and genotypic data were provided by the applicant, and the EFSA Panel considered this information regarding the characterization of B. xylanisolvens DSM 23964 to be sufficient. The production process was clearly described in the application, and a study to guarantee the effectiveness of the heat treatment was conducted. Consequently, the EFSA Panel deemed the applied techniques to be standardized across the dairy industry, considered that they are sufficiently described, and identified no safety concerns (EFSA Panel on Dietetic Products, Nutrition and Allergies, 2015).
The applicant also provided the anticipated intake of the product. The novel food would be marketed in liquid and semi-liquid forms in fermented, low-fat milk and skimmed milk products (fermented milk, buttermilk, yogurt, and yogurt drinks) or as a spray-dried powder (the fillings and coatings of cereals, cereal bars, fruits, and nuts). Due to a lack of European data, the applicant used consumption data from the United States. A conservative scenario was used to estimate the intake, proposing that the applicant's products would replace all existing products (e.g., yogurt and buttermilk; EFSA Panel on Dietetic Products, Nutrition and Allergies, 2015).
The applicant provided compositional data for spray-dried skimmed milk cultured with B. xylanisolvens DSM 23964, including an overview of relevant macronutrients. The applicant also supplied an overview of the vitamin B2, vitamin B12, free lysine, and furosine (as a marker for Maillard reaction) content to ensure that the heat treatment had no impact on these vitamins. Although, lactose content was missing from the analysis (a comparable amount to traditional products was assumed), the EFSA Panel considered consumption of the novel food to not be nutritionally disadvantageous.
The applicant also provided toxicological information about the novel food, including a study on an intraperitoneal abscess formation model in mice (Ulsemer et al., 2012b). Abscess formation is a relevant pathology because some species in the genus Bacteroides, such as B. fragilis, can induce abscesses on multiple sites in the body. B. xylanisolvens DSM 23964 was administered in varying doses, but none of the mice developed abscesses. Human studies were also supplied to the EFSA (Ulsemer et al., 2012a,c).
Although, the applicant did not provide material regarding the allergenicity of the product, the EFSA panel judged that it would be unlikely that the allergenic potential would differ from that of other fermented dairy products. The EFSA itself had previously considered the effect of heat treatment on the allergenicity of milk (EFSA Panel on Dietetic Products EFSA Panel on Dietetic Products, Nutrition and Allergies, 2014).
QPS Evaluation of B. xylanisolvens
When the EFSA receives an application for a novel bacterial strain or a food product containing a novel strain, it conducts a mandatory, in-depth evaluation based on the QPS scheme. Consequently, after the EFSA Panel on Dietetic Products, Nutrition, and Allergies was asked to perform an additional assessment on B. xylanisolvens DSM 23964, the EFSA Panel on Biological Hazards (BIOHAZ) conducted a QPS assessment for B. xylanisolvens (EFSA Panel on Biological Hazards, 2014). This panel found that the published studies about B. xylanisolvens were not sufficient to include the organism on the QPS list, although no relevant safety concerns could be established.
The cepA gene in the genomic DNA of B. xylanisolvens DSM 23964 provides the strain with resistance to β-lactam antibiotics (Ulsemer et al., 2012b). This resistance is very common in the genus Bacteroides (Wexler, 2007). No mobile elements, such as conjugative transposons and plasmids, were found. The strain was also screened for 8 potential virulence genes common in other Bacteroides species, but none was detected, and B. xylanisolvens DSM 23964 showed no adhesion to Caco-2 cells in the cell culture model (Ulsemer et al., 2012b). The panel considered transfer of genes unlikely to take place due to the heat inactivation and lack of plasmids.
The panel emphasized that the body of knowledge was insufficient. B. xylanisolvens had no history of use in fermentation processes, and the few existing studies were limited to fermented milk products. Although, safety concerns do not seem likely, the panel found the number of published studies to be too low to definitely exclude safety issues. The pilot studies involved only small, healthy cohorts, and the administered bacterial cells were inactivated. Considering all these arguments, the panel did not recommend putting B. xylanisolvens on the QPS list (EFSA Panel on Biological Hazards, 2014).
Bacteroides xylanisolvens and Its Beneficial Properties
Most bacteria found in the human intestine are anaerobic. Among the many requirements for a bacterial strain to be categorized as probiotic, an essential characteristic is survival along the gastro-intestinal tract to finally provide beneficial effects for the host. B. xylanisolvens DSM 23964 had a survival rate of 90% after spending 3 h in simulated gastric juice and a survival rate of 96% after spending 4 h in simulated intestinal juices (Ulsemer et al., 2012b).
Immunomodulatory properties are important attributes of probiotics. The genus Bacteroides achieved a higher induction of mucosal IgA production than Lactobacillus when co-cultured with Peyer's patches lymphocytes (Yanagibashi et al., 2009). The fermentation of dietary polysaccharides with production of SCFA by B. xylanisolvens is connected to health-promoting effects through lower cholesterol levels, satiety stimulation, and even an anti-carcinogenic effect (Hosseini et al., 2011).
Published studies on fermented milk products containing B. xylanisolvens DSM 23964 are limited to inactivated bacterial cells, but by definition, probiotics need to be alive when administered to the consumer. Moreover, despite this microorganism's high survival and ability to ferment carbohydrates, these properties are irrelevant in the context of the approved product because it contains a heat-killed strain; therefore, no survival or metabolic activity is expected.
B. xylanisolvens offers several potential beneficial properties. However, its inability to bind to epithelial cells in vitro (Ulsemer et al., 2012b) is a major disadvantage in the assessment of probiotic properties because the capability to bind to intestinal epithelial cells in the host is a key step in probiotic activity. As well, studies with living bacteria are needed to increase the chances of acceptance as a probiotic.
Admission of B. xylanisolvens as a Novel Food: Impact on Other Candidates
B. xylanisolvens is only the fourth bacterium, after L. mesenteroides, B. subtilis natto, and C. butyricum, to be approved under Novel Food Regulation No 258/97 (European Commission, 1997b).
The positive outcome of the novel food assessment is independent from the QPS evaluation results. B. xylanisolvens and C. butyricum have not yet been included on the QPS list. It should be pointed out that during the QPS assessment, only the species itself is analyzed, and the potential food products containing the bacteria are not of interest.
Thermal inactivated bacterial form falls out of the accepted definitions of probiotics described above, because the viability of bacterial cells is an essential condition. Nevertheless, non-viable and therefore non-culturable, and immunologically active microbial cells have been reported to provide health benefits to consumers (de Almada et al., 2016) The use of killed bacteria, as long as the beneficial effect is totally or partially retained, represent and advantage when the use of live bacteria is not authorized or may be potentially harmful for some individuals such as patients with weak immune system or under inflammatory conditions (Taverniti and Guglielmetti, 2011; Tsilingiri and Rescigno, 2012). To define the use of non-viable microorganisms which, when administered in adequate amounts, confer a benefit on the consumer the term “paraprobiotic” has been proposed (Taverniti and Guglielmetti, 2011; de Almada et al., 2016), but it still is not a wide accepted and employed term by scientific community and institutions.
Several mechanistic studies have demonstrated that specific chemical compounds isolated from bacteria can induce specific immune responses (Taverniti and Guglielmetti, 2011). In these cases, the term “postbiotic” has been proposed to refer any factor resulting from the metabolic activity of a bacteria or any released bacterial molecule capable of conferring beneficial effects to the consumer in a direct or indirect way (Tsilingiri and Rescigno, 2012; de Almada et al., 2016). But, similarly with “parabiotic” concept is not an officially accepted and used term.
The acceptance of the thermal inactivated form of B. xylanisolvens as a novel food may pave the way for other novel microorganisms in non-viable form or novel bacterial molecules to exert positive health impacts.
Akkermansia muciniphila
The relatively newly described species A. muciniphila is the first discovered taxonomic member in the genus Akkermansia. By June 2017, there has been no evaluation according to the QPS status, which would be performed and published by EFSA. Due to the recent description of this microorganism, it has no history of use in the food industry, so it must be treated according to the Novel Food Regulation (European Commission, 1997b).
A. muciniphila was discovered in 2004 during the search for new mucus-degrading bacteria in human fecal samples. The newly isolated organism was named after Antoon Akkermans, a well-respected Dutch microbiologist who has made significant contributions to the field of microbial ecology (Belzer and De Vos, 2012). This oval-shaped, Gram-negative microorganism belongs to the phylum Verrucomicrobia. A. muciniphila further belongs to the class Verrucomicrobiae, the order Verrucomicrobiales, and the family Verrucomicrobiaceae. A. muciniphila was originally classified as a strict anaerobe, but in the same way as other anaerobic gut colonizers such as Bacteroides fragilis and Bifidobacterium adolescentis, A. muciniphila can tolerate small amounts of oxygen and even can use it at low oxygen concentrations (Ouwerkerk et al., 2016b). This contrasts with some of the butyrate producing bacteria that are really strict anaerobes.
Metagenomic analysis have indicated that at least 8 additional A. muciniphila-related species are present in the human intestine (van Passel et al., 2011). Belzer and de Vos suggested that the genus Akkermansia consists of 5 distinct clades (Belzer and De Vos, 2012). Four of these 5 clades are associated with A. muciniphila, with sequence similarity range of 80–100%.
Nearly all investigated mammals showed Akkermansia-related sequences in their intestinal samples. Akkermansia-derived sequences are not limited to mammals but also found in other vertebrates: similar sequences were detected in zebrafish and the Burmese python (Costello et al., 2010; Roeselers et al., 2011). From the latter a new species, Akkermansia glyciniphila was isolated and characterized from reticulated python (Ouwerkerk et al., 2016a, 2017). These ubiquitous findings suggest that Akkermansia played a crucial role in the early stages of vertebrata evolution and that its presence might be related to essential functions in the intestine.
Genomic analyses revealed that the single chromosome of A. muciniphila has 2,176 genes with a GC content of 55.8% (Donohue and Salminen, 1996). The secretome responsible for the degradation of mucin involves 61 different proteins, representing 11% of the total protein content (Belzer and De Vos, 2012).
Akkermansia is a relatively new genus, so it is expected that additional species similar to A. muciniphila will be found in coming years as is illustrated by the discovery of A. glyciniphila. It obviously has no history of use in the food sector, and its potential areas of application should be explored.
Despite the short time since the discovery of Akkermansia, its basic characteristics are well-known. This organism is non-motile and specialized in degrading mucin. A. muciniphila is anaerobe and chemo-organotroph and can use mucin as its sole nitrogen, carbon and energy source (Derrien et al., 2004). The habitat of Akkermansia spp. is also well-documented due to its ubiquitous occurrence in the intestines of many vertebrates (Belzer and De Vos, 2012). The general properties of A. muciniphila are listed in Table 2.
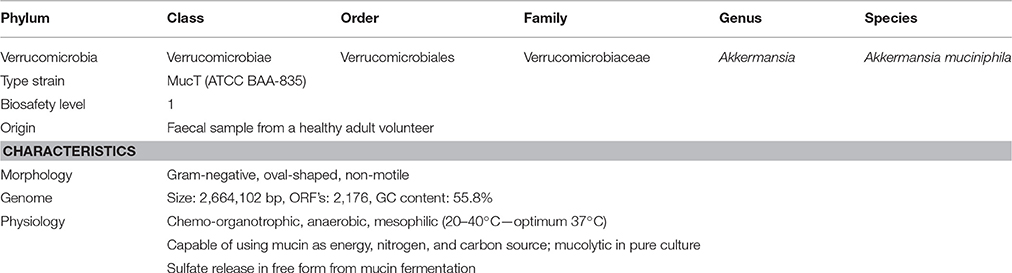
Table 2. General characteristics of Akkermansia muciniphila -adapted from Gomez-Gallego et al. (2016).
Safety Aspects
A. muciniphila is a common inhabitant of the human intestine, accounting for 1–4% of the overall colon microbiota (Derrien et al., 2008). Currently, there are no published clinical human trials in which viable cells were administered to people. Such studies have been conducted only with animal models (Everard et al., 2013). Lagier and colleagues reported two cases in humans where A. muciniphila accounted for up to 80% of the total intestinal microbiota without any noticeable decline in health (Lagier et al., 2015), and there is no evidence that A. muciniphila is connected to any specific disease (Derrien et al., 2010).
There are general concerns because Akkermansia shows pathogen-like behavior. Adhesion to mucus is considered to be a crucial step during infection but is also a behavior found in several probiotics. In contrast to pathogens, A. muciniphila, as a mucin degrader, stays in the outer layer of the mucus and never reaches the inner layer, which is necessary for a successful infection (Gomez-Gallego et al., 2016). In addition, mucin degradation itself resembles pathogen-like behavior (Donohue and Salminen, 1996) but is regarded as a regular process in a balanced, self-renewing intestine (Gomez-Gallego et al., 2016).
Colorectal cancer patients showed a four-fold increase of A. muciniphila in stool samples compared to healthy subjects (Weir et al., 2013). However, patients with colorectal cancer have reduced food intake, and studies have demonstrated that fasting correlates with increased levels of A. muciniphila (Remely et al., 2015). In addition, colorectal cancer is related to increased cell proliferation and mucus production; therefore, it is evident that levels of the main mucus-degrading bacterium could increase in this situation (Gomez-Gallego et al., 2016).
Overall, there seem to be no specific safety concerns regarding the genus Akkermansia and its type strain A. muciniphila MucT. Potential future concerns could be eliminated through individual, case-by-case review. There are no applications so far for A. muciniphila and, therefore, no history of safe use, so the QPS status of this species has not yet been determined.
Akkermansia Muciniphila as Candidate for Novel Food
Currently, there are no pending applications for products containing A. muciniphila under Regulation (EC) 258/97 (European Commission, 1997b). A. muciniphila was only discovered and isolated in 2004, so comprehensive research is still needed to better understand this microorganism.
A. muciniphila was not used for human consumption to any “significant degree” in the EU before 15 May 1997. A food business operator interested in putting a product containing A. muciniphila in the market would have to submit an application according to the Novel Food Regulation. The European Commission published Recommendation (European Commission, 1997a) to give food companies the information needed for a successful application. In the case of A. muciniphila, there are still many unknown aspects that are needed for Novel Food application, as well as some limitations: (1) specification of the possible novel products which will contain A. muciniphila; (2) production processes to keep A. muciniphila alive in the final product to fulfill its potential probiotic properties; (3) anticipated human intake taking into account the lack of known intake patterns; (4) the lack of clinical trials in humans (toxicological and nutritional assessments have been conducted only with animal models); and (5) the lack of knowledge about the allergenic potential.
Based on the knowledge acquired so far, though, this microorganism could be very interesting for food companies and consumers. A. muciniphila is part of the gut microbiota in all humans and may display probiotic properties due to its contribution to the functional gastro-intestinal tract. In addition, current data indicate that a decline in A. muciniphila in the colon correlates with several diseases, such as obesity, type-2 diabetes, and inflammatory bowel disease (Png et al., 2010; Cani and Everard, 2014; Schneeberger et al., 2015). This reduction could be related with a decrease in mucus thickness. But the complex relation between A. muciniphila and the mucus layer remains unclear and the administration of A. muciniphila might stimulate the recovery of the mucus layer (Everard et al., 2013).
Recently, A. muciniphila has been demonstrated to provide beneficial effects even after heat treatment for 30 min at 70°C. The heat-inactivated bacteria could reduce fat mass development, insulin resistance, and dyslipidemia in obese and diabetic mice. Some membrane proteins showed stability and interaction with Toll-like receptor 2 even during the thermal process. This membrane protein also improved the gut barrier and provided ongoing beneficial effects after bacterial inactivation (Plovier et al., 2017). Like B. xylanisolvens, therefore, A. muciniphila may enter the food market in this non-viable form.
The link between obesity and decreased levels of A. muciniphila extends beyond rodents. A Swedish investigation showed that obese pre-school children have significantly lower levels of A. muciniphila than their normal-weight peers (Karlsson et al., 2012). Similarly, pre-school infants that used macrolide antibiotics showed a higher BMI than the non-antibiotic users and this was associated with a depletion of A.muciniphila (Korpela et al., 2016).
Despite the growing attention to A. muciniphila, much research is needed to gain more specific and age-restricted information. No randomized, double-blind, placebo-controlled human clinical trials have studied the effects of A. muciniphila intake. Most research has involved animals, and long-term studies on the human level are the next step to take. Despite the lack of information relevant to the European Commission's recommendation on novel food, the microorganism has quite promising prospects for future novel food applications, especially for its potential probiotic health benefits.
It is important to point out that A. muciniphila has been detected in breast tissue (Urbaniak et al., 2014) and then has been consumed by humans via breast milk (Collado et al., 2012). The question, therefore, arises whether it is necessary to prove the safety of an organism that is already part of nutrition in the early stages of life. However, the levels of viable cells in breast milk might be much lower than those added to food products. Safety concern may arise due not to the nature of A. muciniphila but to the levels used in food and supplements. However, assuming around 1013 bacteria to be present in the human intestine, an average healthy adult may carry over 1011 Akkermansia in its colon. Further discussions are needed, but for such specific cases, European legislation needs to be modified in the future. Placement of A. muciniphila on the QPS list under the given conditions may be complicated because a history of safe use cannot be established.
Fructophilic Lactic Acid Bacteria: New Members of the Family
Characteristics
Long before Orla-Jensen described the group of lactic acid bacteria (LAB) in 1919, humankind benefited from their positive attributes in various food production processes (von Wright, 2012). LAB are involved in various industrial fermentation processes, such as fermented milk products and sausages. Along with fermented food production, the growing sector of probiotics represents the biggest operational area for LAB.
Fructophilic lactic acid bacteria (FLAB) constitute a subgroup in the diverse LAB order. FLAB preferably use fructose as a substrate. They show poor growth on glucose, and external electron acceptors (e.g., pyruvate, oxygen, and fructose) dramatically enhance their growth. FLAB, therefore, inhabit fructose-rich niches: flowers, fruits, fermented foods, such as wine and cocoa beans, and even the gastro-intestinal tracts of insects harbor these microorganisms (Endo, 2012). The FLAB group is divided into the genera Fructobacillus (its type species: F. fructosus) and Lactobacillus (Endo et al., 2009).
Four Fructobacillus species formerly were members of the genus Leuconostoc, but recent analyses suggested that their unique characteristics and phylogenetic positions supported reclassification of this bacterial group (Endo and Okada, 2008). Fructobacillus shows a clear preference for fructose over glucose (Endo and Okada, 2008). Several findings related to metabolism, genome size, and gene losses suggest reductive evolution in Fructobacillus genus compared to Leuconostoc, providing strong support for the reclassification and renaming of Fructobacillus (Endo et al., 2015).
The number of species in FLAB subgroup is growing in both genera, Fructobacillus and Lactobacilllus. Fructobacillus consists of five species: F. fructosus, F. pseudoficulneus, F. ficulneus, F. durionis, and F. tropaeoli (Endo et al., 2015). F. fructosus and F. pseudoficulneus are the most frequently detected species in natural sources. These microorganisms have been isolated from figs, bananas, flowers, the honeybee gut, wine, and even taberna, a traditional beverage in Southern Mexico (Alcantara-Hernandez et al., 2010; Endo, 2012). The FLAB members in the genus Lactobacillus are L. kunkeei, L. apinorum, and L. florum (Neveling et al., 2012). L. kunkeei was originally isolated from wine and characterized as obligately FLAB (Endo et al., 2012). Interestingly, this microorganism is the major component in the gut microbiota of honeybees (Endo and Salminen, 2013). L. apinorum is a recently described species from honeybees, and its fructophilic characteristic were recently reported (Maeno et al., 2017). These two lactobacilli share 98.9% sequence similarity based on 16S rRNA gene sequences. The genomic characteristics of the two species, especially the gene reduction system, are similar to Fructobacillus spp. but not to other members of lactobacilli, suggesting that fructose-richness induced an environment-specific gene reduction in phylogenetically distant microorganisms (Maeno et al., 2016). L. florum was formerly the only facultatively FLAB. It is differentiated from the obligately FLAB based on the growth ratio on glucose. L. florum grows more slowly on glucose than fructose. Like obligately FLAB, electron acceptors enhance growth on glucose.
Practical Impact and Safety
Although, FLAB were only recently discovered, this group of microorganisms might have great potential for the food industry. LAB are generally considered to be safe and have been involved in human food production and nutrition since ancient times (von Wright, 2012). These properties might be extended to FLAB. Recent investigations detected the presence of FLAB in many different food items. For example, several Fructobacillus spp. have been observed during the spontaneous cocoa bean fermentation process (Lefeber et al., 2011; Papalexandratou et al., 2011), and L. florum has been found in grapes and wine, possibly contributing important properties from an oenological perspective (Mtshali et al., 2012).
The presence of FLAB in the gastro-intestinal tracts of bees, giant ants, tropical fruit flies, and bumblebees indicate possible probiotic characteristics (Endo, 2012), although FLAB has not been detected in vertebrates' intestines (Endo and Salminen, 2013). Bee commensal L. kunkeei is considered to be a candidate for honeybee probiotics and paratransgenesis. There is no clinical evidence regarding the pathogenicity of FLAB, which might be an indicator of potential safety. However, this possibility should be treated cautiously because consumers' level of exposure to this bacterial group through food is not well known. Recent studies have suggested that administration of heat-killed L. kunkeei YB38 has potential beneficial properties for human health, including increased bowel movements and enhanced immunoglobulin A production (Asama et al., 2015, 2016). As in the case of B. xylanisolvens, therefore, L. kunkeei might enter the food market in thermal-treated form.
Faecalibacterium prausnitzii
Fusobacterium prausnitzii is the sole member of the genus Faecalibacterium and a commensal bacterium of the human gut microbiota (Miquel et al., 2013). This Gram-positive, obligately anaerobe microorganism belongs to class Clostridia. It accounts for 3–5% of total fecal bacteria and, therefore, is a predominant species in human feces (Breyner et al., 2017). F. prausnitzii is a non-motile, non-spore-forming bacterium and extremely sensitive to oxygen (Foditsch et al., 2014). F. prausnitzii was initially classified as F. prausnitzii, but the sequence of the 16s rRNA gene established that it is only distantly related to Fusobacterium and more closely related to members of Clostridium cluster IV (Miquel et al., 2013). The general properties of F. prausnitzii are listed in Table 3.
F. prausnitzii can digest dietary fibers producing mostly butyrate, along with other SCFA (Miquel et al., 2013), and has been shown to respond to prebiotic supplementation using a mixed chain length fructan supplement and pectin (Scott et al., 2015). This microbe may play a crucial role in human health because changes in levels of F. prausnitzii have been associated with several gastrointestinal diseases, such as Crohn's disease, and depressive disorders (Miquel et al., 2013; Jiang et al., 2015). The microorganism also displays beneficial anti-inflammatory effects on the host, suggesting that it may be used to counterbalance the dysbiosis linked to certain diseases (Sokol et al., 2008; Jiang et al., 2015; Miquel et al., 2015). Butyrate has several beneficial effects on the host, including provision of an energy source for epithelial cells, induction of colonic regulatory T cells, induction of apoptosis in human colonic carcinoma cells, inhibition of inflammatory responses in intestinal biopsy specimens, and improvement of metabolic syndrome. Moreover, recent investigations have revealed possible contributions to protective mechanisms, such as self-defense against inflammatory reactions. F. prausnitzii has been involved in the inhibition of pro-inflammatory cytokines and the secretion of bioactive molecules which lead to blockage of the NF-κB pathway, showing protective effects in chemically induced colitis mouse models (Breyner et al., 2017). Further research with Faecalibacterium could increase knowledge about its probiotic effects and may lead to its future use in the food industry.
The successful use of F. prausnitzii as probiotic most likely will depend on the administration method. Studies related to bacterial resistance to gastric pH in vitro recommend oral administration of F. prausnitzii after feeding to avoid low gastric pH (Breyner et al., 2017). Moreover, cultivating F. prausnitzii is difficult even in anaerobic conditions (Miquel et al., 2013).
The inclusion of Faecalibacterium on the QPS list might be difficult due to its lack of a history of safe use and to the multiple antibiotic resistance genes observed in Faecalibacterium sp. isolates which could contribute to resistance dissemination (Breyner et al., 2017). In addition, full toxicology assays and characterization of the strain are still needed for regulatory approval (Thomas et al., 2015).
Conclusions and Outlook
Remarks on the Candidates
A brief evaluation of the benefits and drawbacks of the new microbial candidates based on existing knowledge is given in Table 4. Novel microbes, including many not yet assessed in humans, are increasingly proposed as potential probiotics and assessed as novel food. The introduction of these new species further challenges the traditional selection criteria because the question of their safety and efficacy arises in an untraditional manner, with no previous experience in exposure to these bacteria or their use in foods or supplements. Most probiotics belong to well-known microbial groups (lactobacilli and bifidobacteria) with long histories of safe use, which facilitates preliminary evaluation of their safety and functionality based on the available body of knowledge about these groups. However, some newly proposed probiotics were first described, cultured, and isolated during the past decade and thus present a challenge to both scientific and regulatory frontiers. Nevertheless, A. muciniphila, FLAB, and F. prausnitzii are regarded as promising candidates. The many proven and potential probiotic properties provide good opportunities for future food authorizations, but their safety in human trials still must be demonstrated.
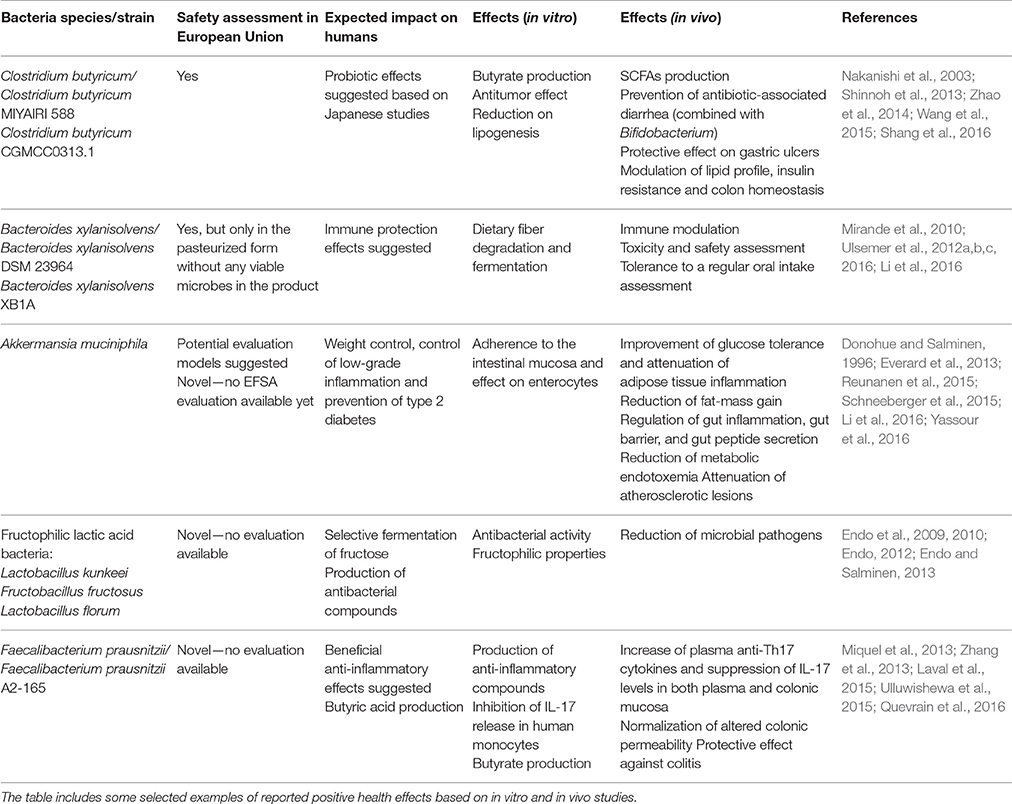
Table 4. Examples of potential future probiotics suggested for human use and/or assessed as novel foods in the European Union.
In the context to the definition of probiotics, Plovier and colleagues made an especially interesting observation: even the pasteurized and non-replicating Akkermansia cells were capable of providing beneficial effects. Decreased fat-mass development, dyslipidaemia, and insulin resistance were demonstrated in obese and diabetic mice (Everard et al., 2013). This new insight might have crucial relevance and allow future authorizations because nonviable cells pose rise fewer concerns than living bacteria. Nonviable bacteria are more easily deemed safe in novel food evaluation, albeit usually in case-by-case decisions. In comparison to B. xylanisolvens, some information about A. muciniphila is still lacking. Due to the absence of practical applications, specification of the novel food and its production process and nutritional information has not yet been performed. However, there is information available about its taxonomy, culturing methods, pathogenic and toxicological nature, and nutritional assessment data in animal models (Donohue and Salminen, 1996). A major key for the approval of novel food is a sufficient number of clinical trials in humans, but A. muciniphila lacks enough appropriate human studies. Randomized, double-blind, placebo-controlled clinical trials, dose-response studies, and toxicological studies are still needed to, for example, establish the appropriate number of bacteria to be administered and the right matrix to provide probiotic properties. If the missing data become available, though, A. muciniphila has similar chances of acceptance as a novel food as the recently officially accepted B. xylanisolvens. Notably the EFSA positively assessed B. xylanisolvens although the applicant supplied only two human studies.
FLAB were also discovered and described recently, so it is challenging to predict how they might be accepted as part of future food products. Further research is needed to understand, for example, what differentiates this bacterium from traditional LAB and its exact relevance to the production of fermented foods. Recent studies revealed that heat-killed L. kunkeei possess beneficial properties, such as increased bowel movement and enhanced immunoglobulin A production (Asama et al., 2015, 2016). Their special relation to LAB, a very widely used group in the food industry, may lead to inclusion on the QPS list and novel food authorization in the future.
The growing amount of evidence supporting that the modulation of F. prausnitzii levels using prebiotics, probiotics, and symbiotics might have prophylactic or therapeutic applications in human health makes this bacterium interesting from the perspective of novel foods. Factors such as sensitivity to oxygen, gastric pH, and bile salts and industrial production for probiotic use need to be optimized, and toxicological assays and antibiotic resistance tests should be carefully conducted before clinical trials in humans.
The potential candidates for novel foods discussed as examples in the present work represent only a small fraction of the potential bacteria that may be included in novel foods in the future. Other potential candidates are Eubacterium hallii and bacteria in the genus Roseburia. E. hallii is a common inhabitant of the human gut microbiota and is known for its versatile utilization of different carbon sources. E. hallii can produce butyrate from lactate, acetate, and glucose and is one of the first butyrate producers in the infant gut (Pham et al., 2016). In addition, a recent study showed the impact of E. hallii in treating insulin resistance in a mouse model (Udayappan et al., 2016). Its early appearance in the human intestine and production of essential SCFA may enable future novel food applications in the food industry.
The genus Roseburia consists of five species which are Gram-positive, obligately anaerobic bacteria and motile due to the presence of subterminal flagella (Tamanai-Shacoori et al., 2017). Roseburia spp. are a dominant intestinal bacterial species, accounting for 2–15% of the total human gut microbiota that produce SCFA (Dostal et al., 2015). Considering the production of essential SCFA and the high levels of Roseburia spp. in the human microbiota, members of this genus may have great potential as future novel probiotics.
Remarks on Regulatory Aspects
Ongoing revisions of the regulations on novel food, along with the continuous updating of the QPS list, will accelerate novel food authorization and biological agent safety assessment. Both processes support the work of the EFSA and access to novel products in the food market and ensure the safety of the European consumer. The lengthy process to revise the novel food regulations has demonstrated the difficulty of changing legislation on the European level. Most decisions need to be unanimous, and it is challenging to please all member states. Although, these developments are remarkable, the EU authorization of novel food still seems more comprehensive than the GRAS concept in the United States. The precautionary principle is a fundamental pillar of environmental, food, and health policies in the EU. Prevention of any possible harm or danger to the consumer is the most important priority, especially for marketing of food containing potentially harmful bacteria. The elimination of any possible health threat, therefore, is essential before a product is allowed to enter the European market. The European consumer is the most relevant player in European legislation although companies understandably complain about such strict conditions.
Regulation (EU) 2015/2283 on novel foods (European Commission, 2015), which will come into force on January 1, 2018, is aimed at facilitating the authorization of novel food. The EFSA is responsible for the scientific risk assessment, ensuring the shift from an individual assessment by the national competent authorities to a generic assessment. The new regulation also directs the EFSA to deliver scientific opinions within 9 months after receiving all valid applications. This mandate represents enormous time savings and a significant decrease in the financial costs for food companies submitting applications. The newly introduced data protection for approved novel food authorization will also help companies successfully compete in the food market.
Author Contributions
WK, SS, and CG designed the study; TB performed the bibliographical review; TB and CG drafting the manuscript; AE, GV, MG, Wd, WK, and SS contributed with the latest achievements in their field of expertise. AE, MG, GV, Wd, WK, SS, and CG interpreted the main points related with EU legislation discussed in the review for each novel bacteria. AE, MG, GV, Wd, WK, SS, and CG discussed the final version and revising them critically. All the authors give the final approval of the version to be published.
Conflict of Interest Statement
The authors declare that the research was conducted in the absence of any commercial or financial relationships that could be construed as a potential conflict of interest.
Acknowledgments
CG is a recipient of the Seneca Postdoctoral Grant from the Seneca Foundation, the Regional Agency of Science and Technology of the Region of Murcia (funded by the Education and Universities Council—Autonomous Community of the Region of Murcia, Spain).
References
Alcantara-Hernandez, R. J., Rodriguez-Alvarez, J. A., Valenzuela-Encinas, C., Gutierrez-Miceli, F. A., Castanon-Gonzalez, H., Marsch, R., et al. (2010). The bacterial community in 'taberna' a traditional beverage of Southern Mexico. Lett. Appl. Microbiol. 51, 558–563. doi: 10.1111/j.1472-765X.2010.02934.x
Asama, T., Arima, T. H., Gomi, T., Keishi, T., Tani, H., Kimura, Y., et al. (2015). Lactobacillus kunkeei YB38 from honeybee products enhances IgA production in healthy adults. J. Appl. Microbiol. 119, 818–826. doi: 10.1111/jam.12889
Asama, T., Kimura, Y., Kono, T., Tatefuji, T., Hashimoto, K., and Benno, Y. (2016). Effects of heat-killed Lactobacillus kunkeei YB38 on human intestinal environment and bowel movement: a pilot study. Benef. Microbes 7, 337–344. doi: 10.3920/BM2015.0132
Aureli, P., Capurso, L., Castellazzi, A. M., Clerici, M., Giovannini, M., Morelli, L., et al. (2011). Probiotics and health: an evidence-based review. Pharmacol. Res. 63, 366–376. doi: 10.1016/j.phrs.2011.02.006
BAuA (2011). Begründungspapier zur Einstufung von Bacteroides xylanisolvens in Risikogruppe 1 nach BioStoffV. Bundesanstalt für Arbeitsschutz und Arbeitsmedizin, BAuA.
Belzer, C., and De Vos, W. M. (2012). Microbes insidefrom diversity to function: the case of Akkermansia. ISME J. 6, 1449–1458. doi: 10.1038/ismej.2012.6
Borresen, E. C., Henderson, A. J., Kumar, A., Weir, T. L., and Ryan, E. P. (2012). Fermented foods: patented approaches and formulations for nutritional supplementation and health promotion. Recent Pat. Food Nutr. Agric. 4, 134–140. doi: 10.2174/2212798411204020134
Breyner, N. M., Michon, C., de Sousa, C. S., Vilas Boas, P. B., Chain, F., Azevedo, V., et al. (2017). Microbial Anti-Inflammatory Molecule (MAM) from Faecalibacterium prausnitzii shows a protective effect on DNBS and DSS-induced colitis model in mice through inhibition of NF-kappaB pathway. Front. Microbiol. 8:114. doi: 10.3389/fmicb.2017.00114
Cammarota, G., Ianiro, G., Bibbo, S., and Gasbarrini, A. (2014). Gut microbiota modulation: probiotics, antibiotics or fecal microbiota transplantation? Intern. Emerg. Med. 9, 365–373. doi: 10.1007/s11739-014-1069-4.
Cani, P. D., and Everard, A. (2014). Akkermansia muciniphila: a novel target controlling obesity, type 2 diabetes and inflammation? Med. Sci. 30, 125–127. doi: 10.1051/medsci/20143002003
Chassard, C., Delmas, E., Lawson, P. A., and Bernalier-Donadille, A. (2008). Bacteroides xylanisolvens sp. nov., a xylan-degrading bacterium isolated from human faeces. Int. J. Syst. Evol. Microbiol. 58(Pt 4), 1008–1013. doi: 10.1099/ijs.0.65504-0
Cibik, R., Lepage, E., and Talliez, P. (2000). Molecular diversity of leuconostoc mesenteroides and leuconostoc citreum isolated from traditional french cheeses as revealed by RAPD fingerprinting, 16S rDNA sequencing and 16S rDNA fragment amplification. Syst. Appl. Microbiol. 23, 267–278. doi: 10.1016/S0723-2020(00)80014-4
Collado, M. C., Laitinen, K., Salminen, S., and Isolauri, E. (2012). Maternal weight and excessive weight gain during pregnancy modify the immunomodulatory potential of breast milk. Pediatr. Res. 72, 77–85. doi: 10.1038/pr.2012.42
Costello, E. K., Gordon, J. I., Secor, S. M., and Knight, R. (2010). Postprandial remodeling of the gut microbiota in Burmese pythons. ISME J. 4, 1375–1385. doi: 10.1038/ismej.2010.71
D'Angelo, L., Cicotello, J., Zago, M., Guglielmotti, D., Quiberoni, A., and Suarez, V. (2017). Leuconostoc strains isolated from dairy products: response against food stress conditions. Food Microbiol. 66, 28–39. doi: 10.1016/j.fm.2017.04.001
de Almada, C. N., Almada, C. N., Martinez, R. C. R., and Sant'Ana, A. S. (2016). Paraprobiotics: evidences on their ability to modify biological responses, inactivation methods and perspectives on their application in foods. Trends Food Sci. Technol. 98, 56–114. doi: 10.1016/j.tifs.2016.09.011
de Paula, A. T., Jeronymo-Ceneviva, A. B., Todorov, S. D., and Penna, A. L. B. (2015). The two faces of Leuconostoc mesenteroides in food systems. Food Rev. Int. 31, 147–171. doi: 10.1080/87559129.2014.981825
Derrien, M., Collado, M. C., Ben-Amor, K., Salminen, S., and De Vos, W. M. (2008). The mucin degrader Akkermansia muciniphila is an abundant resident of the human intestinal tract. Appl. Environ. Microbiol. 74, 1646–1648. doi: 10.1128/AEM.01226-07
Derrien, M., van Passel, M. W. J., van de Bovenkamp, J. H. B., Schipper, R. G., de Vos, W. M., and Dekker, J. (2010). Mucin-bacterial interactions in the human oral cavity and digestive tract. Gut Microbes 1, 254–268. doi: 10.4161/gmic.1.4.12778
Derrien, M., Vaughan, E. E., Plugge, C. M., and de Vos, W. M. (2004). Akkermansia municiphila gen. nov., sp. nov., a human intestinal mucin-degrading bacterium. Int. J. Syst. Evol. Microbiol. 54, 1469–1476. doi: 10.1099/ijs.0.02873-0
Donohue, D., and Salminen, S. (1996). Safety of probiotic bacteria. Asia Pac. J. Clin. Nutr. 5, 25–28.
Dostal, A., Lacroix, C., Bircher, L., Pham, V. T., Follador, R., Zimmermann, M. B., et al. (2015). Iron modulates butyrate production by a child gut microbiota in vitro. MBio 6, e01453–e01415. doi: 10.1128/mBio.01453-15
EFSA (2007). Introduction of a Qualified Presumption of Safety (QPS) Approach for Assessment of Selected Microorganisms Referred to EFSA- Opinion of the Scientific Committee.
EFSA Panel on Biological Hazards (2014). Statement on the update of the list of QPS-recommended biological agents intentionally added to food or feed as notified to EFSA 1: Suitability of taxonomic units notified to EFSA until October 2014. EFSA J. 12, 1–42. doi: 10.2903/j.efsa.2014.3938
EFSA Panel on Biological Hazards (2010). Scientific Opinion on the maintenance of the list of QPS biological agents intentionally added to food and feed (2010 update). EFSA J. 8, 1–56. doi: 10.2903/j.efsa.2010.1944
EFSA Panel on Dietetic Products (2016). Guidance on the preparation and presentation of an application for authorisation of a novel food in the context of Regulation (EU) 2015/2283. EFSA J. 14, 1–24. doi: 10.2903/j.efsa.2016.4594
EFSA Panel on Dietetic Products, Nutrition and Allergies. (2014). Scientific Opinion on the evaluation of allergenic foods and food ingredients for labelling purposes. EFSA J. 12, 1–286. doi: 10.2903/j.efsa.2014.3894
EFSA Panel on Dietetic Products, Nutrition and Allergies. (2015). Scientific Opinion on the safety of ‘heat-treated milk products fermented with Bacteroides xylanisolvens DSM 23964’ as a novel food. EFSA J. 13, 1–18. doi: 10.2903/j.efsa.2015.3956
Endo, A. (2012). Fructophilic lactic acid bacteria inhabit fructose-rich niches in nature. Microb. Ecol. Health Dis. 23, 6–12 doi: 10.3402/mehd.v23i0.18563
Endo, A., Futagawa-Endo, Y., and Dicks, L. M. (2009). Isolation and characterization of fructophilic lactic acid bacteria from fructose-rich niches. Syst. Appl. Microbiol. 32, 593–600. doi: 10.1016/j.syapm.2009.08.002
Endo, A., Futagawa-Endo, Y., Sakamoto, M., Kitahara, M., and Dicks, L. M. T. (2010). Lactobacillus florum sp. nov., a fructophilic species isolated from flowers. Int. J. Syst. Evol. Microbiol. 60, 2478–2482. doi: 10.1099/ijs.0.019067-0
Endo, A., Irisawa, T., Futagawa-Endo, Y., Takano, K., du Toit, M., Okada, S., et al. (2012). Characterization and emended description of Lactobacillus kunkeei as a fructophilic lactic acid bacterium. Int. J. Syst. Evol. Microbiol. 62, 500–504. doi: 10.1099/ijs.0.031054-0
Endo, A., and Okada, S. (2008). Reclassification of the genus Leuconostoc and proposals of Fructobacillus fructosus gen. nov., comb. nov., Fructobacillus durionis comb. nov., Fructobacillus ficulneus comb. nov. and Fructobacillus pseudoficulneus comb. nov. Int. J. Syst. Evol. Microbiol. 58(Pt 9), 2195–2205. doi: 10.1099/ijs.0.65609-0
Endo, A., and Salminen, S. (2013). Honeybees and beehives are rich sources for fructophilic lactic acid bacteria. Syst. Appl. Microbiol. 36, 444–448. doi: 10.1016/j.syapm.2013.06.002
Endo, A., Tanizawa, Y., Tanaka, N., Maeno, S., Kumar, H., Shiwa, Y., et al. (2015). Comparative genomics of Fructobacillus spp. and Leuconostoc spp. reveals niche-specific evolution of Fructobacillus spp. BMC Genomics 16:1117. doi: 10.1186/s12864-015-2339-x
European Commission (1997a). 97/618/EC. Commission Recommendation of 29 July 1997 Concerning the Scientific Aspects and the Presentation of Information Necessary to Support Applications for the Placing on the Market of Novel Foods and Novel Food Ingredients and the Preparation of Initial Assessment Reports Under Regulation (EC) No 258/97 of the European Parliament and of the Council.
European Commission (1997b). R. 285/97/ERegulation, C. (EC) No. 258/97 of the European Parliament and of the Council of 27 January 1997 Concerning Novel Foods and Novel Food Ingredients.
European Commission (2001). E. Commission: 2001/122/EC. Commission Decision of 30 January 2001 on Authorising the Placing on the Market of a Dextran Preparation Produced by Leuconostoc mesenteroides as a Novel Food Ingredient in Bakery Products under Regulation (EC) No 258/97 of the European Parliament and of the Council (Notified Under Document Number C(2001) 174).
European Commission (2009). E. Commission: 2009/345/EC. Commission Decision of 22 April 2009 Authorising the Placing on the Market of Vitamin K2 (Menaquinone) from Bacillus subtilis natto as a Novel Food Ingredient under Regulation (EC) No 258/97 of the European Parliament and of the Council (Notified Under Document Number C(2009) 2935).
European Commission (2014). E. Commission: 2014/907/EU. Commission Implementing Decision of 11 December 2014 Authorising the Placing on the Market of Clostridium butyricum (CBM 588) as a Novel Food Ingredient under Regulation (EC) No 258/97 of the European Parliament and of the Council (Notified Under Document C(2014) 9345).
European Commission (2015). R. E. 2015/2283. Regulation (EU) 2015/2283 of the European Parliament and of the Council of 25 November 2015 on Novel Foods, Amending Regulation (EU) No 1169/2011 of the European Parliament and of the Council and Repealing Regulation (EC) No 258/97 of the European Parliament and of the Council and Commission Regulation (EC) No, 1852/2001.
Everard, A., Belzer, C., Geurts, L., Ouwerkerk, J. P., Druart, C., Bindels, L. B., et al. (2013). Cross-talk between Akkermansia muciniphila and intestinal epithelium controls diet-induced obesity. Proc. Natl. Acad. Sci. U.S.A. 110, 9066–9071. doi: 10.1073/pnas.1219451110
Foditsch, C., Santos, T. M., Teixeira, A. G., Pereira, R. V., Dias, J. M., Gaeta, N., et al. (2014). Isolation and characterization of Faecalibacterium prausnitzii from calves and piglets. PLoS ONE 9:e116465. doi: 10.1371/journal.pone.0116465
Gomez-Gallego, C., Pohl, S., Salminen, S., De Vos, W. M., and Kneifel, W. (2016). Akkermansia muciniphila: a novel functional microbe with probiotic properties. Benef. Microbes 7, 571–584. doi: 10.3920/BM2016.0009
Gómez-Gallego, C., and Salminen, S. (2016). Novel probiotics and prebiotics: how can they help in human gut microbiota dysbiosis? Appl. Food Biotechnol. 3, 72–81. doi: 10.22037/afb.v3i2.11276
Gregory, K. E., LaPlante, R. D., Shan, G., Kumar, D. V., and Gregas, M. (2015). Mode of birth influences preterm infant intestinal colonization with bacteroides over the early neonatal period. Adv. Neonatal Care 15, 386–393. doi: 10.1097/ANC.0000000000000237
H. C. P. Directorate-General (2003). Working Document on a Generic Approach to the Safety Assessment of Micro-Organisms Used in Feed/Food and Feed/Food Production. Available online at: https://ec.europa.eu/food/sites/food/files/safety/docs/sci-com_scf_out178_en.pdf
Hill, C., Guarner, F., Reid, G., Gibson, G. R., Merenstein, D. J., Pot, B., et al. (2014). The International Scientific Association for Probiotics and Prebiotics consensus statement on the scope and appropriate use of the term probiotic. Nat. Rev. Gastroenterol. Hepatol. 11, 506–514. doi: 10.1038/nrgastro.2014.66
Hooper, L. V., Midtvedt, T., and Gordon, J. I. (2002). How host-microbial interactions shape the nutrient environment of the mammalian intestine. Annu. Rev. Nutr. 22, 283–307. doi: 10.1146/annurev.nutr.22.011602.092259
Hosseini, E., Grootaert, C., Verstraete, W., and Van de Wiele, T. (2011). Propionate as a health-promoting microbial metabolite in the human gut. Nutr. Rev. 69, 245–258. doi: 10.1111/j.1753-4887.2011.00388.x
Isa, K., Oka, K., Beauchamp, N., Sato, M., Wada, K., Ohtani, K., et al. (2016). Safety assessment of the Clostridium butyricum MIYAIRI 588(R) probiotic strain including evaluation of antimicrobial sensitivity and presence of Clostridium toxin genes in vitro and teratogenicity in vivo. Hum. Exp. Toxicol. 35, 818–832. doi: 10.1177/0960327115607372
Jiang, H., Ling, Z., Zhang, Y., Mao, H., Ma, Z., Yin, Y., et al. (2015). Altered fecal microbiota composition in patients with major depressive disorder. Brain Behav. Immun. 48, 186–194. doi: 10.1016/j.bbi.2015.03.016
Karlsson, C. L., Önnerfält, J., Xu, J., Molin, G., Ahrné, S., and Thorngren-Jerneck, K. (2012). The microbiota of the gut in preschool children with normal and excessive body weight. Obesity 20, 2257–2261. doi: 10.1038/oby.2012.110
Korpela, K., Salonen, A., Virta, L. J., Kekkonen, R. A., Forslund, K., Bork, P., et al. (2016). Intestinal microbiome is related to lifetime antibiotic use in Finnish pre-school children. Nat. Commun. 7:10410. doi: 10.1038/ncomms10410
Lagier, J. C., Hugon, P., Khelaifia, S., Fournier, P. E., La Scola, B., and Raoult, D. (2015). The rebirth of culture in microbiology through the example of culturomics to study human gut microbiota. Clin. Microbiol. Rev. 28, 237–264. doi: 10.1128/CMR.00014-14
Laval, L., Martin, R., Natividad, J. N., Chain, F., Miquel, S., Desclee de Maredsous, C., et al. (2015). Lactobacillus rhamnosus CNCM I-3690 and the commensal bacterium Faecalibacterium prausnitzii A2-165 exhibit similar protective effects to induced barrier hyper-permeability in mice. Gut Microbes 6, 1–9. doi: 10.4161/19490976.2014.990784
Lefeber, T., Gobert, W., Vrancken, G., Camu, N., and De Vuyst, L. (2011). Dynamics and species diversity of communities of lactic acid bacteria and acetic acid bacteria during spontaneous cocoa bean fermentation in vessels. Food Microbiol. 28, 457–464. doi: 10.1016/j.fm.2010.10.010
Leuschner, R. G. K., Robinson, T. P., Hugas, M., Cocconcelli, P. S., Richard-Forget, F., Klein, G., et al. (2010). Qualified presumption of safety (QPS): a generic risk assessment approach for biological agents notified to the European Food Safety Authority (EFSA). Trends Food Sci. Technol. 21, 425–435. doi: 10.1016/j.tifs.2010.07.003
Li, M., Li, G., Shang, Q., Chen, X., Liu, W., Pi, X. E., et al. (2016). In vitro fermentation of alginate and its derivatives by human gut microbiota. Anaerobe 39, 19–25. doi: 10.1016/j.anaerobe.2016.02.003
Maeno, S., Dicks, L., Nakagawa, J., and Endo, A. (2017). Lactobacillus apinorum belongs to the fructophilic lactic acid bacteria. Biosci. Microb. Food Health. doi: 10.12938/bmfh.17-008
Maeno, S., Tanizawa, Y., Kanesaki, Y., Kubota, E., Kumar, H., Dicks, L., et al. (2016). Genomic characterization of a fructophilic bee symbiont Lactobacillus kunkeei reveals its niche-specific adaptation. Syst. Appl. Microbiol. 39, 516–526. doi: 10.1016/j.syapm.2016.09.006
Marchesi, J. R., Adams, D. H., Fava, F., Hermes, G. D., Hirschfield, G. M., Hold, G., et al. (2016). The gut microbiota and host health: a new clinical frontier. Gut 65, 330–339. doi: 10.1136/gutjnl-2015-309990
Miquel, S., Beaumont, M., Martín, R., Langella, P., Braesco, V., and Thomas, M. (2015). A proposed framework for an appropriate evaluation scheme for microorganisms as novel foods with a health claim in Europe. Microb. Cell Fact. 9, 14–48. doi: 10.1186/s12934-015-0229-1
Miquel, S., Martin, R., Rossi, O., Bermudez-Humaran, L. G., Chatel, J. M., Sokol, H., et al. (2013). Faecalibacterium prausnitzii and human intestinal health. Curr. Opin. Microbiol. 16, 255–261. doi: 10.1016/j.mib.2013.06.003
Mirande, C., Kadlecikova, E., Matulova, M., Capek, P., Bernalier-Donadille, A., Forano, E., et al. (2010). Dietary fibre degradation and fermentation by two xylanolytic bacteria Bacteroides xylanisolvens XB1AT and Roseburia intestinalis XB6B4 from the human intestine. J. Appl. Microbiol. 109, 451–460. doi: 10.1111/j.1365-2672.2010.04671.x
Mtshali, P. S., Divol, B., and du Toit, M. (2012). Identification and characterization of Lactobacillus florum strains isolated from South African grape and wine samples. Int. J. Food Microbiol. 153, 106–113. doi: 10.1016/j.ijfoodmicro.2011.10.023
Nakanishi, S., Kataoka, K., Kuwahara, T., and Ohnishi, Y. (2003). Effects of high amylose maize starch and Clostridium butyricum on microbiota and formation metabolism in colonic of azoxymethane-induced aberrant crypt foci in the rat colon. Microbiol. Immunol. 47, 951–958. doi: 10.1111/j.1348-0421.2003.tb03469.x
Neveling, D. P., Endo, A., and Dicks, L. M. (2012). Fructophilic Lactobacillus kunkeei and Lactobacillus brevis isolated from fresh flowers, bees and bee-hives. Curr. Microbiol. 65, 507–515. doi: 10.1007/s00284-012-0186-4
Ouwerkerk, J. P., Aalvink, S., Belzer, C., and de Vos, W. M. (2016a). Akkermansia glycaniphila sp. nov., an anaerobic mucin-degrading bacterium isolated from reticulated python faeces. Int. J. Syst. Evol. Microbiol. 66, 4614–4620. doi: 10.1099/ijsem.0.001399
Ouwerkerk, J. P., Koehorst, J. J., Schaap, P. J., Ritari, J., Paulin, L., Belzer, C., et al. (2017). Complete genome sequence of Akkermansia glycaniphila strain PytT, a mducin-degrading specialist of the reticulated python gut. Genome Announc. 5:e01098–16. doi: 10.1128/genomeA.01098-16
Ouwerkerk, J. P., van der Ark, K. C., Davids, M., Claassens, N. J., Robert Finestra, T., de Vos, W., et al. (2016b). Adaptation of Akkermansia muciniphila to the oxic-anoxic interface of the mucus layer. Appl. Environ. Microbiol. 82, 6983–6993. doi: 10.1128/AEM.01641-16
Papalexandratou, Z., Vrancken, G., De Bruyne, K., Vandamme, P., and De Vuyst, L. (2011). Spontaneous organic cocoa bean box fermentations in Brazil are characterized by a restricted species diversity of lactic acid bacteria and acetic acid bacteria. Food Microbiol. 28, 1326–1338. doi: 10.1016/j.fm.2011.06.003
Pham, V. T., Lacroix, C., Braegger, C. P., and Chassard, C. (2016). Early colonization of functional groups of microbes in the infant gut. Environ. Microbiol. 18, 2246–2258. doi: 10.1111/1462-2920.13316
Plovier, H., Everard, A., Druart, C., Depommier, C., Van Hul, M., Geurts, L., et al. (2017). A purified membrane protein from Akkermansia muciniphila or the pasteurized bacterium improves metabolism in obese and diabetic mice. Nat. Med. 23, 107–113. doi: 10.1038/nm.4236
Png, C. W., Lindén, S. K., Gilshenan, K. S., Zoetendal, E. G., McSweeney, C. S., Sly, L., et al. (2010). Mucolytic bacteria with increased prevalence in IBD mucosa augment in vitro utilization of mucin by other bacteria. Am. J. Gastroenterol. 105, 2420–2428. doi: 10.1038/ajg.2010.281
Quevrain, E., Maubert, M. A., Sokol, H., Devreese, B., and Seksik, P. (2016). The presence of the anti-inflammatory protein MAM, from Faecalibacterium prausnitzii, in the intestinal ecosystem. Gut. 65:882. doi: 10.1136/gutjnl-2015-311094
Remely, M., Hippe, B., Geretschlaeger, I., Stegmayer, S., Hoefinger, I., and Haslberger, A. (2015). Increased gut microbiota diversity and abundance of Faecalibacterium prausnitzii and Akkermansia after fasting: a pilot study. Wien. Klin. Wochenschr. 127, 394–398. doi: 10.1007/s00508-015-0755-1
Reunanen, J., Kainulainen, V., Huuskonen, L., Ottman, N., Belzer, C., Huhtinen, H., et al. (2015). Akkermansia muciniphila adheres to enterocytes and strengthens the integrity of the epithelial cell layer. Appl. Environ. Microbiol. 81, 3655–3662. doi: 10.1128/AEM.04050-14
Roeselers, G., Mittge, E. K., Stephens, W. Z., Parichy, D. M., Cavanaugh, C. M., Guillemin, K., et al. (2011). Evidence for a core gut microbiota in the zebrafish. ISME J. 5, 1595–1608. doi: 10.1038/ismej.2011.38
Schneeberger, M., Everard, A., Gomez-Valades, A. G., Matamoros, S., Ramirez, S., Delzenne, N. M., et al. (2015). Akkermansia muciniphila inversely correlates with the onset of inflammation, altered adipose tissue metabolism and metabolic disorders during obesity in mice. Sci. Rep. 5:16643. doi: 10.1038/srep16643
Scott, K. P., Antoine, J. M., Midtvedt, T., and van Hemert, S. (2015). Manipulating the gut microbiota to maintain health and treat disease. Microb. Ecol. Health Dis. 26:25877. doi: 10.3402/mehd.v26.25877
Sears, C. L. (2005). A dynamic partnership: celebrating our gut flora. Anaerobe 11, 247–251. doi: 10.1016/j.anaerobe.2005.05.001
Selhub, E. M., Logan, A. C., and Bested, A. C. (2014). Fermented foods, microbiota, and mental health: ancient practice meets nutritional psychiatry. J. Physiol. Anthropol. 33:2 doi: 10.1186/1880-6805-33-2
Shang, H., Sun, J., and Chen, Y. Q. (2016). Clostridium butyricum CGMCC0313.1 modulates lipid profile, insulin resistance and colon homeostasis in obese mice. PLoS ONE 11:e0154373. doi: 10.1371/journal.pone.0154373
Shimbo, I., Yamaguchi, T., Odaka, T., Nakajima, K., Koide, A., Koyama, H., et al. (2005). Effect of Clostridium butyricum on fecal flora in Helicobacter pylori eradication therapy. World J. Gastroenterol. 11, 7520–7524. doi: 10.3748/wjg.v11.i47.7520
Shinnoh, M., Horinaka, M., Yasuda, T., Yoshikawa, S., Morita, M., Yamada, T., et al. (2013). Clostridium butyricum MIYAIRI 588 shows antitumor effects by enhancing the release of TRAIL from neutrophils through MMP-8. Int. J. Oncol. 42, 903–911. doi: 10.3892/ijo.2013.1790
Sokol, H., Pigneur, B., Watterlot, L., Lakhdari, O., Bermudez-Humaran, L. G., Gratadoux, J. J., et al. (2008). Faecalibacterium prausnitzii is an anti-inflammatory commensal bacterium identified by gut microbiota analysis of Crohn disease patients. Proc. Natl. Acad. Sci. U.S.A. 105, 16731–16736. doi: 10.1073/pnas.0804812105
Tamanai-Shacoori, Z., Smida, I., Bousarghin, L., Loreal, O., Meuric, V., Fong, S. B., et al. (2017). Roseburia spp.: a marker of health? Future Microbiol. 12, 157–170. doi: 10.2217/fmb-2016-0130
Taverniti, V., and Guglielmetti, S. (2011). The immunomodulatory properties of probiotic microorganisms beyond their viability (ghost probiotics: proposal of paraprobiotic concept). Genes Nutr. 6, 261–274. doi: 10.1007/s12263-011-0218-x
Thomas, L. V., Suzuki, K., and Zhao, J. (2015). Probiotics: a proactive approach to health. A symposium report. Br. J. Nutr. 114(Suppl. 1), S1–S15. doi: 10.1017/S0007114515004043
Thorning, T. K., Raben, A., Tholstrup, T., Soedamah-Muthu, S. S., Givens, I., and Astrup, A. (2016). Milk and dairy products: good or bad for human health? An assessment of the totality of scientific evidence. Food Nutr. Res. 60:32527. doi: 10.3402/fnr.v60.32527
Tsilingiri, K., and Rescigno, M. (2012). Postbiotics: what else? Benef. Microbes 4, 101–107. doi: 10.3920/BM2012.0046
Turnbaugh, P. J., Ley, R. E., Mahowald, M. A., Magrini, V., Mardis, E. R., and Gordon, J. I. (2006). An obesity-associated gut microbiome with increased capacity for energy harvest. Nature 444, 1027–1031. doi: 10.1038/nature05414
Udayappan, S., Manneras-Holm, L., Chaplin-Scott, A., Belzer, C., Herrema, H., Dallinga-Thie, G. M., et al. (2016). Oral treatment with. Npj Biofilms Microb. 2, 1–10. doi: 10.1038/npjbiofilms.2016.9
Ulluwishewa, D., Anderson, R. C., Young, W., McNabb, W. C., van Baarlen, P., Moughan, P. J., et al. (2015). Live Faecalibacterium prausnitzii in an apical anaerobic model of the intestinal epithelial barrier. Cell. Microbiol. 17, 226–240. doi: 10.1111/cmi.12360
Ulsemer, P., Toutounian, K., Kressel, G., Goletz, C., Schmidt, J., Karsten, U., et al. (2016). Impact of oral consumption of heat-treated Bacteroides xylanisolvens DSM 23964 on the level of natural TFα-specific antibodies in human adults. Benef. Microbes 6:16. doi: 10.3920/BM2015.0143
Ulsemer, P., Toutounian, K., Kressel, G., Schmidt, J., Karsten, U., Hahn, A., et al. (2012a). Safety and tolerance of Bacteroides xylanisolvens DSM 23964 in healthy adults. Benef. Microbes 3, 99–111. doi: 10.3920/BM2011.0051
Ulsemer, P., Toutounian, K., Schmidt, J., Karsten, U., and Goletz, S. (2012b). Preliminary safety evaluation of a new Bacteroides xylanisolvens isolate. Appl. Environ. Microbiol. 78, 528–535. doi: 10.1128/AEM.06641-11
Ulsemer, P., Toutounian, K., Schmidt, J., Leuschner, J., Karsten, U., and Goletz, S. (2012c). Safety assessment of the commensal strain Bacteroides xylanisolvens DSM 23964. Regul. Toxicol. Pharmacol. 62, 336–346. doi: 10.1016/j.yrtph.2011.10.014
Urbaniak, C., Cummins, J., Brackstone, M., MacKlaim, J. M., Gloor, G. B., Baban, C. K., et al. (2014). Microbiota of human breast tissue. Appl. Environ. Microbiol. 80, 3007–3014. doi: 10.1128/AEM.00242-14
van Passel, M. W. J., Kant, R., Zoetendal, E. G., Plugge, C. M., Derrien, M., Malfatti, S., et al. (2011). The genome of Akkermansia muciniphila, a dedicated intestinal mucin degrader, and its use in exploring intestinal metagenomes. PLoS ONE 6:e16876. doi: 10.1371/journal.pone.0016876
von Wright, A. (2012). Microbes for human and animal consumption. Benef. Microorg. Agric. Food Environ. 27–40 doi: 10.1079/9781845938109.0027
Wang, F.-Y., Liu, J.-M., Luo, H.-H., Liu, A.-H., and Jiang, Y. (2015). Potential protective effects of Clostridium butyricum on experimental gastric ulcers in mice. World J. Gastroenterol. 21, 8340–8351. doi: 10.3748/wjg.v21.i27.8340
Weir, T. L., Manter, D. K., Sheflin, A. M., Barnett, B. A., Heuberger, A. L., and Ryan, E. P. (2013). Stool microbiome and metabolome differences between colorectal cancer patients and healthy adults. PLoS ONE 8:e70803. doi: 10.1371/journal.pone.0070803
Wexler, H. M. (2007). Bacteroides: the good, the bad, and the nitty-gritty. Clin. Microbiol. Rev. 20, 593–621. doi: 10.1128/CMR.00008-07
Yanagibashi, T., Hosono, A., Oyama, A., Tsuda, M., Hachimura, S., Takahashi, Y., et al. (2009). Bacteroides induce higher IgA production than Lactobacillus by increasing activation-induced cytidine deaminase expression in B cells in murine Peyer's patches. Biosci. Biotechnol. Biochem. 73, 372–377. doi: 10.1271/bbb.80612
Yassour, M., Lim, M. Y., Yun, H. S., Tickle, T. L., Sung, J., Song, Y. M., et al. (2016). Sub-clinical detection of gut microbial biomarkers of obesity and type 2 diabetes. Genome Med. 8:17. doi: 10.1186/s13073-016-0271-6
Yasueda, A., Mizushima, T., Nezu, R., Sumi, R., Tanaka, M., Nishimura, J., et al. (2016). The effect of Clostridium butyricum MIYAIRI on the prevention of pouchitis and alteration of the microbiota profile in patients with ulcerative colitis. Surg Today 46, 939–949. doi: 10.1007/s00595-015-1261-9
Zhang, X., Shen, D., Fang, Z., Jie, Z., Qiu, X., Zhang, C., et al. (2013). Human gut microbiota changes reveal the progression of glucose intolerance. PLoS ONE 8:e71108. doi: 10.1371/journal.pone.0071108
Keywords: novel microbes, safety, Bacteroides xylanisolvens, Akkermansia muciniphila, fructophilic lactic acid bacteria, Faecalibacterium prausnitzii
Citation: Brodmann T, Endo A, Gueimonde M, Vinderola G, Kneifel W, de Vos WM, Salminen S and Gómez-Gallego C (2017) Safety of Novel Microbes for Human Consumption: Practical Examples of Assessment in the European Union. Front. Microbiol. 8:1725. doi: 10.3389/fmicb.2017.01725
Received: 24 June 2017; Accepted: 24 August 2017;
Published: 12 September 2017.
Edited by:
Rebeca Martín, INRA Centre Jouy-en-Josas, FranceReviewed by:
Giorgio Giraffa, Centro di Ricerca per le Produzioni Foraggere e Lattiero-Casearie (CREA), ItalyVictor Ladero, Consejo Superior de Investigaciones Científicas (CSIC), Spain
Copyright © 2017 Brodmann, Endo, Gueimonde, Vinderola, Kneifel, de Vos, Salminen and Gómez-Gallego. This is an open-access article distributed under the terms of the Creative Commons Attribution License (CC BY). The use, distribution or reproduction in other forums is permitted, provided the original author(s) or licensor are credited and that the original publication in this journal is cited, in accordance with accepted academic practice. No use, distribution or reproduction is permitted which does not comply with these terms.
*Correspondence: Carlos Gómez-Gallego, cargom@utu.fi