- 1Centro de Ciências Biológicas e da Saúde, Universidade Federal do Maranhão, São Luís, Brazil
- 2Centro de Ciências da Saúde, Universidade CEUMA, São Luís, Brazil
- 3Vascular Biology and Inflammation Section, Cardiovascular Division, King’s College London, London, UK
- 4Centro de Detección Biomolecular, Benemérita Universidad Autónoma de Puebla, Puebla, Mexico
Lactobacilli are involved in the microbial homeostasis in the female genital tract. Due to the high prevalence of many bacterial diseases of the female genital tract and the resistance of microorganisms to various antimicrobial agents, alternative means to control these infections are necessary. Thus, this study aimed to evaluate the probiotic properties of well-characterized Lactobacillus species, including L. acidophilus (ATCC 4356), L. brevis (ATCC 367), L. delbrueckii ssp. delbrueckii (ATCC 9645), L. fermentum (ATCC 23271), L. paracasei (ATCC 335), L. plantarum (ATCC 8014), and L. rhamnosus (ATCC 9595), against Candida albicans (ATCC 18804), Neisseria gonorrhoeae (ATCC 9826), and Streptococcus agalactiae (ATCC 13813). The probiotic potential was investigated by using the following criteria: (i) adhesion to host epithelial cells and mucus, (ii) biofilm formation, (iii) co-aggregation with bacterial pathogens, (iv) inhibition of pathogen adhesion to mucus and HeLa cells, and (v) antimicrobial activity. Tested lactobacilli adhered to mucin, co-aggregated with all genital microorganisms, and displayed antimicrobial activity. With the exception of L. acidophilus and L. paracasei, they adhered to HeLa cells. However, only L. fermentum produced a moderate biofilm and a higher level of co-aggregation and mucin binding. The displacement assay demonstrated that all Lactobacillus strains inhibit C. albicans binding to mucin (p < 0.001), likely due to the production of substances with antimicrobial activity. Clinical isolates belonging to the most common Candida species associated to vaginal candidiasis were inhibited by L. fermentum. Collectively, our data suggest that L. fermentum ATCC 23271 is a potential probiotic candidate, particularly to complement candidiasis treatment, since presented with the best probiotic profile in comparison with the other tested lactobacilli strains.
Introduction
The microbiota of the female genital tract is composed of diverse aerobic, anaerobic, and microaerophilic microorganisms (Ravel et al., 2011). The genus Lactobacillus is considered a biological marker of the normal vaginal microbiota (Lidbeck and Nord, 1993; Witkin et al., 2007). The majority of reproductive-aged women carry 107–109 Lactobacillus/g of vaginal secretion (Ferris et al., 2007; Zhou et al., 2007). Lactobacilli display several defense mechanisms against pathogens, such as the production of antimicrobial components and adhesion to the mucosa to create a barrier that inhibits pathogen colonization by competing for binding sites on epithelial cells (Walencka et al., 2008; Medellin-Pena and Griffiths, 2009; Nami et al., 2014; Gorska et al., 2016).
Several factors or conditions can alter the balance of the genital tract microbiota, including inadequate or prolonged antibiotic therapy, tight or synthetic-fiber clothing and an acidic diet. Such conditions facilitate the excessive growth of aerobic and anaerobic microorganisms in the genital tract, leading to the development of two clinical syndromes, vaginitis and vaginosis, which are very common among females (Ravel et al., 2011). Vaginitis is characterized by the presence of inflammation and discharge, which are triggered by the proliferation of some pathogens, especially Candida albicans, amongst others (Donders et al., 2002; Lobos et al., 2013; Wang et al., 2016).
In addition to these microorganisms, several agents of sexually transmitted diseases (STDs) represent a serious public health burden due to their high morbidity rates. For example, infections due to Neisseria gonorrhoeae are among the most prevalent STDs. The World Health Organization reports 106 million gonorrhea new cases annually. Gonococcal infections that are not adequately treated may cause permanent damage to the female reproductive tract, resulting in ectopic pregnancy or even infertility (WHO, 2012).
Other microorganisms, such as Streptococcus agalactiae, colonize the female genital tract as commensals of the vagina, but can be transmitted to newborns during delivery (Hansen et al., 2004), leading to neonatal infection and meningitis (Puopolo et al., 2005). Although antibiotic prophylaxis has decreased the incidence of these infections, case reports of disease caused by S. agalactiae continue to occur in infants with significant morbidity and mortality (Puopolo et al., 2005). Therefore, new alternative approaches are needed, since the current methods to prevent genital infections are not always effective against those caused by primary pathogens or commensal microorganisms transmitted during birth.
Bacterial adhesion to the mucosa and epithelial cell surface is a key event in the colonization of the host and disease progression. Understanding the adhesion strategies used by pathogens in order to colonize the host is crucial for the development of preventive or therapeutic interventions against infectious diseases. One approach is the use of non-pathogenic bacteria that are natural components of the microbiota and that can potentially inhibit the adhesion and establishment of pathogenic bacteria (Spurbeck and Arvidson, 2010).
Members of the Lactobacillus genus have been proposed as probiotics as they can protect against infectious disease agents (Li et al., 2012; Ren et al., 2012; Zabihollahi et al., 2012; Alexandre et al., 2014). From those, some (L. crispatus and L. acidophilus) commonly colonize the female genital tract and have been studied as probiotics (Mastromarino et al., 2002; Antonio et al., 2009; Zhang et al., 2012; Kaewnopparat et al., 2013; Nami et al., 2014; Verdenelli et al., 2014). However, it is not known whether Lactobacillus species that are less frequent in the vagina also display probiotic activities. Here, we evaluated the probiotic potential of several reference species of the Lactobacillus genus not usually found in the vaginal microbiome against three important microorganisms associated with genital infections, neonatal sepsis, and meningitis.
Materials and Methods
Bacterial Strains and Growth Conditions
The following Lactobacillus reference strains were used: L. brevis (ATCC 367), L. delbrueckii ssp. delbrueckii (ATCC 9645), L. fermentum (ATCC 23271), L. paracasei (ATCC 335), L. plantarum (ATCC 8014), and L. rhamnosus (ATCC 9595). For comparison, a reference strain of Lactobacillus acidophilus (ATCC 4356) was included in the study as a representative species of the female genital tract. All strains were routinely grown in Man, Rogosa, and Sharpe (MRS) broth or agar (Difco, USA) and incubated in an anaerobic atmosphere (Probac, Brazil) at 37°C for 24 h. The pathogens evaluated were: (i) C. albicans (ATCC 18804), which was grown on Sabouraud dextrose agar (Difco, USA) at 37°C for 24 h; (ii) N. gonorrhoeae (ATCC 9826), which was cultured on gonococci (GC) agar (Difco, USA) supplemented with VX supplement (LaborClin, Brazil) and hemoglobin (Difco, USA) at 37°C for 48 h under a 5% CO2 atmosphere; and (iii) S. agalactiae (ATCC 13813), which was cultivated on Columbia agar (Difco, USA) supplemented with 5% sheep blood, under 5% CO2 at 37°C for 24 h. All strains were obtained from the Culture Collection Laboratory of the Fundação Instituto Oswaldo Cruz (Rio de Janeiro, Brazil) and stored in Brain Heart Infusion (BHI) broth (Difco, USA) with 20% glycerol (Amresco, Australia) at -86°C. Clinical isolates of Candida spp. were kindly donated by Dr. Cristina A. Monteiro from the Universidade CEUMA.
HeLa Cell Culture
Human cervical epithelial (HeLa) cells were cultured in Dulbecco’s Modified Eagle’s Medium (DMEM) containing GlutaMAX (GibcoTM, USA) and supplemented with 1× antibiotic-antimycotic solution (Gibco, USA) and 10% fetal bovine serum (Gibco, USA) at 37°C under a 5% CO2 atmosphere.
Adhesion Assays to Eukaryotic Cells
Prior to the adhesion assays, Lactobacillus strains were cultured in MRS broth under the conditions described above. After 24 h, the bacterial cultures were centrifuged at 6,000 × g and washed three times with phosphate-buffered saline (PBS, pH 7.4, Sigma, USA) in order to remove organic acids produced during growth. The pellets were resuspended in 300 μl of DMEM. The adhesion assay was performed as previously described (Kaewnopparat et al., 2013), with minor modifications. Briefly, HeLa cell monolayers were seeded into 24-well plates with or without glass coverslips and allowed to grow to 80–90% confluence. Fifty microliters (~2.3 × 107) of the bacterial suspensions were added to each well, and the plates were incubated at 37°C under 5% CO2 for 3 h. Then, each well was washed three times with PBS for removal of non-adherent bacteria. Immediately after, the cells were incubated with 0.1% Triton X-100 (Sigma, USA) for 5 min, and 10-fold serial dilutions were spread onto agar plates for quantification of the total number of bacteria adhered to the cells (in colony forming unit; CFU). To visualize the bacterial adherence to HeLa cells, each glass coverslip was fixed with methanol (P.A., Amresco, Australia) and stained with 0.2% May-Grunwald and 0.6% Giemsa (Amresco, Australia). The coverslips were then mounted onto glass slides and visualized by light microscopy under oil-immersion objective 100× (Leica ICC50 HD microscope). HeLa cells incubated in the absence of bacteria were used as negative controls.
Biofilm Formation by Lactobacillus spp.
Biofilm formation on a polystyrene surface was quantified in 96-well plates as described by Stepanovic et al. (2000). Briefly, Lactobacillus spp. were cultured for 24 h and then adjusted to an optical density of 0.7 at 540 nm. Then, 200 μl of the bacterial suspensions were added into the wells. Wells containing MRS broth only were used as negative controls. The plates were incubated at 37°C for 24 h, washed three times with 300 μl of PBS (pH 7.4) and air-dried. Each well was then fixed with 200 μl of methanol (P.A., Amresco, Australia) and the plate was incubated at room temperature for 15 min. Following, the methanol was discarded, and the microplate was air-dried. Two hundred microliters of 2% crystal violet (Amresco, Australia) was added to each well and incubated for 15 min. The plate was then washed three times with distilled water and air-dried. To extract the dye impregnated in the biofilm, 160 μl of 33% glacial acetic acid (Amresco, Australia) were added per well. The absorbance was immediately measured at 540 nm using an ELISA plate reader (Bio-Tek Instrument Co., WA, USA) and taken as indicative of biofilm formation. Based on the optical densities of the isolates (OD) and the negative controls (ODC), the biofilm formation by Lactobacillus was classified as follows: 1, non-adherent: OD ≤ ODC; 2, weakly adherent: ODC < OD ≤ (2 × ODC); 3, moderately adherent: (2 × ODC) < OD ≤ (4 × ODC); 4, strongly adherent: (4 × ODC) < OD.
Hydrophobicity of Lactobacillus spp. Cell Surface
The hydrophobicity of Lactobacillus cell surface was evaluated using the salt aggregation assay (Andreu et al., 1995). For this, bacteria were resuspended in 0.02 M sodium phosphate (pH 6.8). Bacterial suspensions (~1.2 × 109 CFU/ml) were mixed (1:1, v/v) with different concentrations of ammonium sulfate (0.5–4.0 M; Amresco, Australia) on a glass slide. The lowest final concentration of ammonium sulfate able to promote aggregation of Lactobacillus was defined as the salt aggregation value. Hydrophobicity was classified as high, intermediate, or hydrophilic at salt aggregation values of <0.9, 0.9–1.5, and >1.5 M, respectively. For comparison, a solution of 0.02 M sodium phosphate (pH 6.8) without bacteria was used as negative control.
Binding of Lactobacillus spp. and Genital Pathogens to Mucin
The ability of the pathogen and Lactobacillus strains to adhere to mucin was evaluated as previously described (Tallon et al., 2007), with minor modifications. Initially, porcine gastric mucin-type III (Sigma, Saint-Quentin-Fallavier, France) was solubilized in PBS (Sigma, USA; pH 7.4) at a final concentration of 10 mg/ml. Five hundred microliters of mucin solution were added into a 24-well plate and allowed to bind to the wells for 16 h at 4°C. The mucin solution was removed; the wells were washed three times with 1 ml of PBS, saturated with 2% bovine serum albumin for 4 h at 4°C, and washed four times with PBS. Bacterial cultures were centrifuged at 6,000 × g for 5 min, washed three times with PBS, and resuspended in the same buffer to an optical density of 0.1 at 600 nm (~4.5 × 107 CFU/ml for lactobacilli and ~1.5 × 108 CFU/ml for pathogen strains). Next, 500 μl of each bacterial suspension were added to the wells, and the plate was incubated at 37°C for 1 h. After incubation, the wells were washed three times with 1 ml of PBS to remove unbound bacteria. In order to quantify the number of bound bacteria, each well was treated with 1 ml of 0.5% Triton X-100, and the plates were incubated for 30 min at room temperature under agitation. Aliquots (100 μl) were taken from each well, and 10-fold serial dilutions were prepared and spread onto agar plates for quantification of the total number of bacteria adhered to mucin (in CFU). For all experiments, the purity of the bacterial colonies was checked both macroscopically (by the growth aspects) and microscopically (by the Gram stain). Mucin-containing wells without bacteria were used as negative controls.
Exclusion and Displacement Inhibition Assays
After determining which organisms are able to adhere to mucin, we performed both exclusion and displacement inhibition assays. For this, Lactobacillus spp. (~4.5 × 107 CFU/ml) were inoculated either 1 h prior (exclusion assay) or after (displacement assay) the tested pathogens (~1.5 × 108 CFU/ml) (Tallon et al., 2007). Quantification of Lactobacillus and genital pathogens was carried out as described above (see Binding of Lactobacillus spp. and Genital Pathogens to Mucin). Mucin-containing wells without bacteria were used as negative controls.
Co-aggregation of Lactobacillus spp. with Genital Pathogens
This assay was performed to determine whether the lactobacilli are able to co-aggregate with the tested genital pathogens. The lactobacilli and pathogen suspensions (in PBS) were adjusted to achieve turbidity equivalent to that of McFarland’s No. 4 standard (~1.2 × 109 CFU/ml). Five hundred microliters of each Lactobacillus were added per well and mixed with 500 μl of each pathogen suspension into 24-well plates. The plates were then incubated at 37°C for 4 h under constant agitation (100 rpm) in an orbital shaker. The microbial suspension of each well was evaluated under an inverted light microscope and a score was attributed as described by Reid et al. (1990): 0 = no aggregation, 1 = small aggregates comprising small visible clusters of bacteria, 2 = aggregates comprising larger numbers of bacteria, settling to the center of the well, 3 = macroscopically visible clumps comprising larger groups of bacteria which settle to the center of the well, 4 = maximum score allocated to describe a large, macroscopically visible clump in the center of the well. The aggregates were visualized using an inverted light microscope with a 16× magnification lens. Accordingly, results were expressed as aggregation score.
Antimicrobial Activity of Lactobacillus spp. against Genital Pathogens
The antimicrobial actions of Lactobacillus spp. against genital pathogens were assessed as previously described by Mastromarino et al. (2002) with minor modifications. For this, 10 μl (~107 CFU/ml) of Lactobacillus spp. suspensions were spotted onto MRS agar, and bacteria were allowed to grow for 24 h at 37°C, under anaerobiosis. In a typical assay, three to four distinct suspensions of Lactobacillus strains were spotted onto a 90 mm-diameter plate. After incubation, 10 ml of the specific culture medium for each pathogen was cooled to 50°C and poured onto the plate. Pathogen suspensions were adjusted to achieve turbidity equivalent to that of McFarland’s No. 0.5 standard (~1.5 × 108 CFU/ml, for bacterial pathogens and 1–5 × 106 CFU/ml, for C. albicans). Pathogens were then spread separately onto agar plates and incubated for 24 or 48 h at 37°C under aerobic or 5% CO2 culture conditions, depending on the microorganism. Antimicrobial activities were characterized by the diameter (in millimeter) of the zone of growth inhibition directly above the Lactobacillus growth area.
Statistical Analysis
GraphPad Prism (version 5.0; GraphPad Software, Inc. San Diego, CA, USA) software was employed for statistical analysis. For the mucin adhesion and inhibition assays, the Shapiro–Wilk normal test and analysis of variance (ANOVA) test were employed. The Dunnett’s test was employed to compare different groups of microorganisms with respect to the control. Statistical significance was established at p < 0.05. All assays were performed in triplicate on three independent days.
Results
Adhesion Ability of Lactobacillus spp. to HeLa Cells
We investigated the ability of Lactobacillus spp. to adhere to human epithelial (HeLa) cells. Amongst the tested Lactobacillus, L. brevis ATCC 367, L. delbrueckii ssp. delbrueckii ATCC 9645, L. fermentum ATCC 23271, L. plantarum ATCC 8014, and L. rhamnosus ATCC 9595 displayed the highest levels of adhesion to epithelial cells, as denoted by the number of bacteria adhered to the cells, which ranged from 107 to 108 CFU/ml (Figure 1). On the other hand, L. acidophilus ATCC 4356 and L. paracasei ATCC 335 presented the lowest capacity to adhere to HeLa cells, with a recovery of less than 103 CFU/ml from the cell surface.
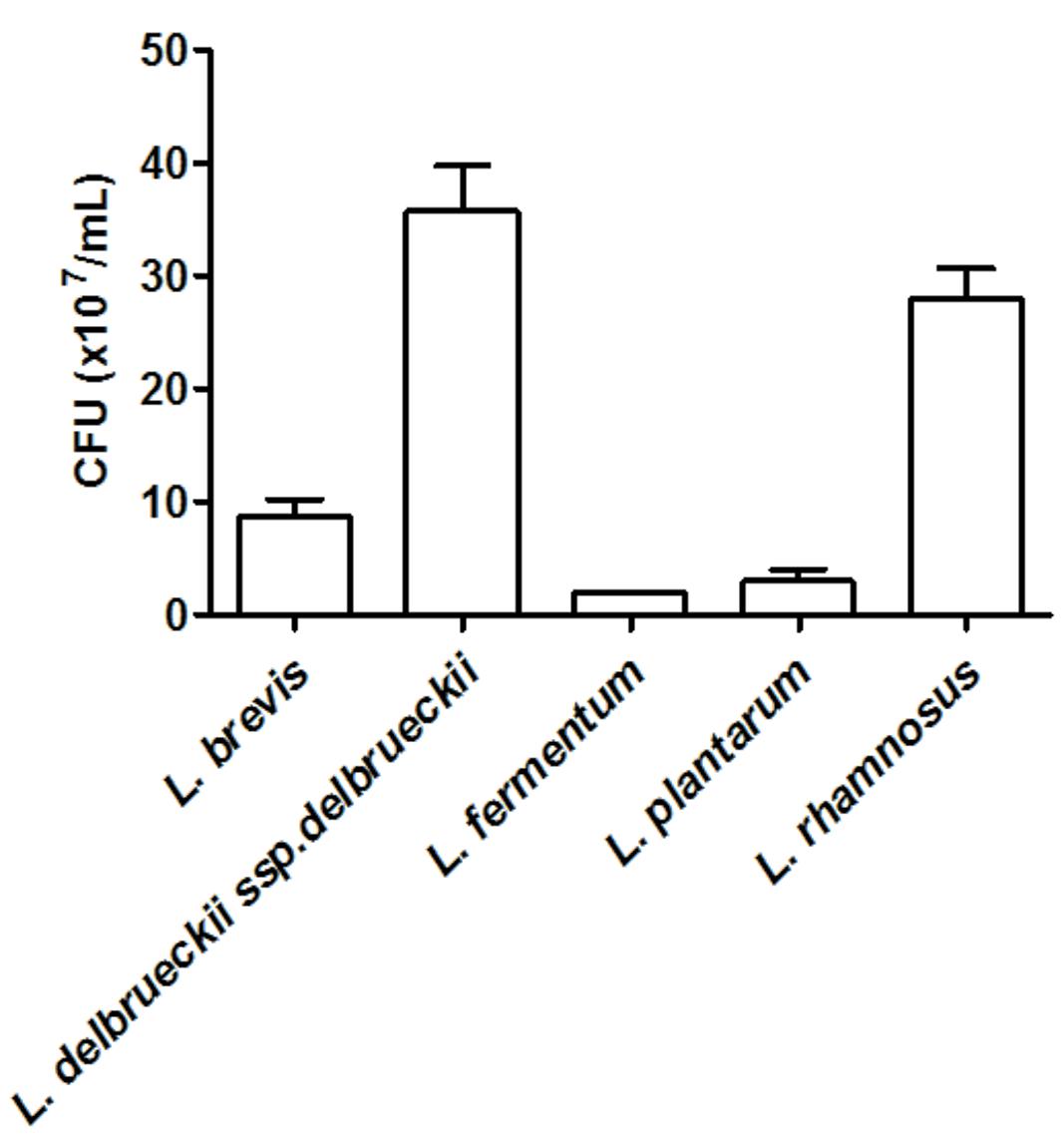
FIGURE 1. Quantification of the adhesion of Lactobacillus (~2.3 × 106 CFU) strains to HeLa cells. The values are expressed in CFU/ml and represent the means of the values obtained. The assay was performed in triplicate.
Hydrophobicity of Lactobacillus spp. Cell Surface and Biofilm Formation
Overall, none of the tested lactobacilli were great biofilm producers with only L. fermentum presenting a moderate ability to form biofilm on a plastic surface (Table 1). L. acidophilus was unable to form biofilm under the used experimental settings. Also, with the exception of L. acidophilus, all strains exhibited high cell surface hydrophobicity (Table 1).
Binding of Lactobacillus spp. and Genital Pathogens to Mucin
All tested microorganisms presented similar ability to bind to mucin, as the number of cells recovered at the end of the assay was greater than 104 CFU/ml for both the lactobacilli and the genital pathogens (Figure 2). Interestingly, L. fermentum binding to mucin was twofold higher than that observed for the remaining Lactobacillus strains. When evaluated in an exclusion assay in the presence of genital pathogens, both L. brevis and L. delbrueckii ssp. delbrueckii significantly increased (twofold) C. albicans binding to mucin in comparison to the pathogen binding in the absence of lactobacilli (p < 0.05; Figure 3A). Similarly, all lactobacilli (excepting L. plantarum) enhanced S. agalactiae binding to mucin (three- to sixfold increase) (Figure 3B). No effects were observed for any of the tested lactobacilli on N. gonorrhoeae binding (p = 0.42; Figure 3C). Assessment of lactobacilli effects on pathogen binding in a displacement assay demonstrated that C. albicans binding to mucin is markedly inhibited (~80% inhibition; p < 0.001) by all tested Lactobacillus spp. (Figure 4A). None of the evaluated lactobacilli affected S. agalactiae and N. gonorrhoeae ability to bind to mucin (p = 0.51 and p = 0.36, respectively; Figures 4B,C).
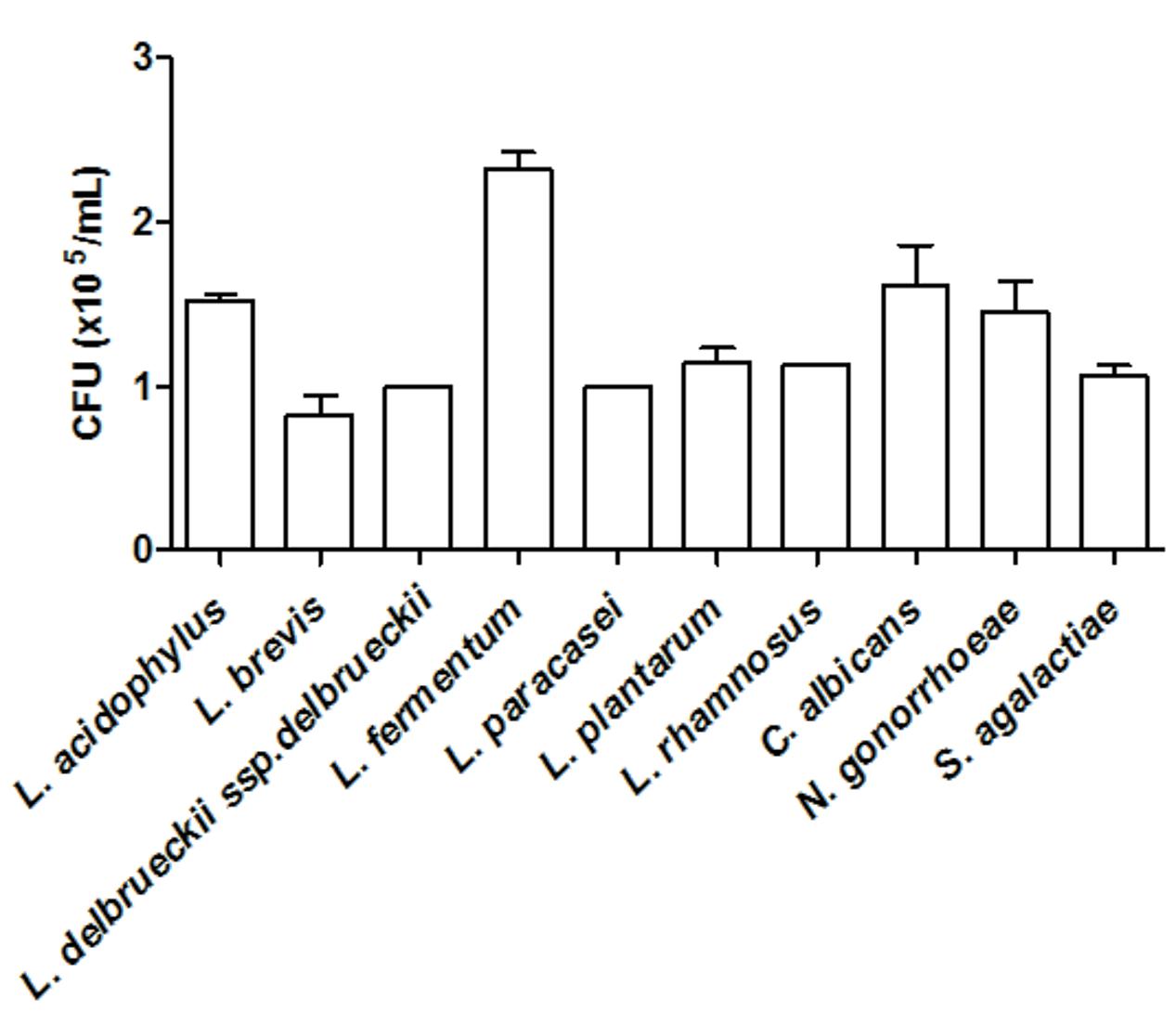
FIGURE 2. Quantification of mucin adhesion by Lactobacillus spp. (~4.5 × 107 CFU/ml) and genital pathogens (~1.5 × 108 CFU/ml). The values are expressed in CFU/ml and represent the means of the values obtained. The assay was performed in triplicate.
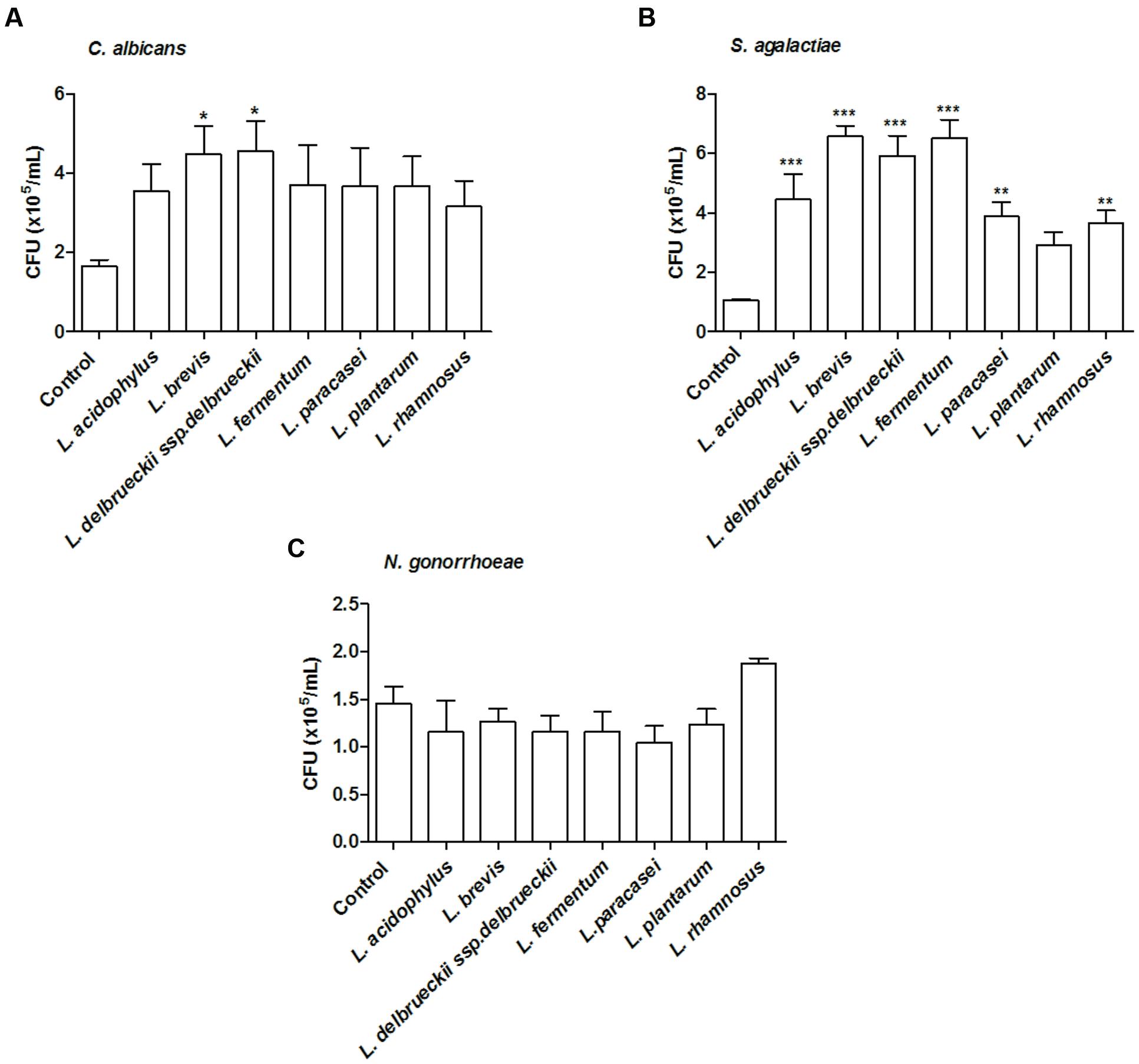
FIGURE 3. Inhibition of mucin adhesion by exclusion. Effect of different lactobacilli on (A) C. albicans, (B) S. agalactiae, and (C) N. gonorrhoeae binding to mucin. The values are expressed in CFU/ml and represent the means of the values obtained. For all experiments, the purity of the colonies was checked both macroscopically (by the growth aspects) and microscopically (by the Gram stain). The assay was performed in triplicate. ∗p < 0.05; ∗∗p < 0.01; ∗∗∗p < 0.001.
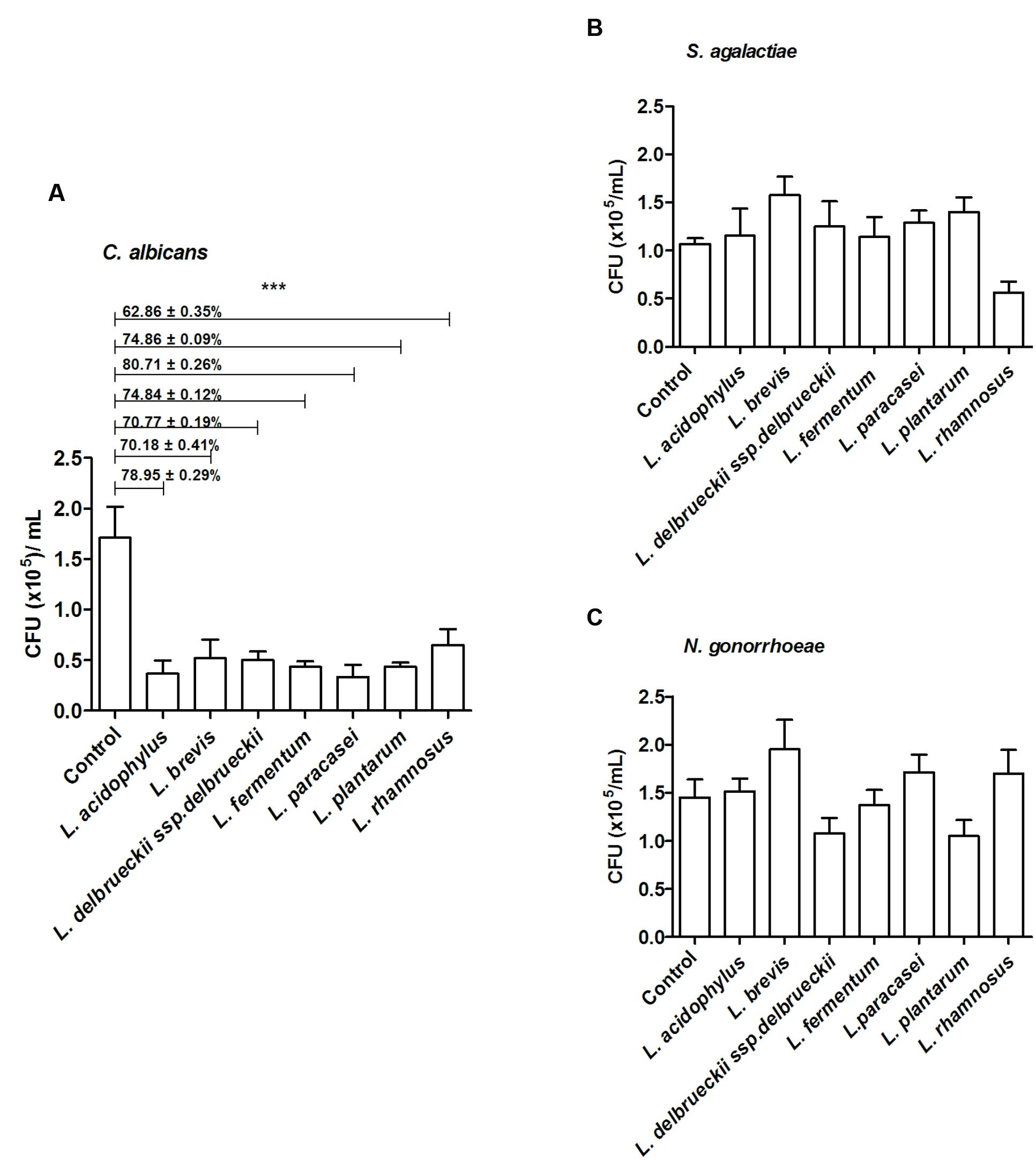
FIGURE 4. Inhibition of mucin adhesion by displacement. Effect of different lactobacilli on (A) C. albicans, (B) S. agalactiae, and (C) N. gonorrhoeae binding to mucin. The values are expressed in CFU/ml and represent the means of the values obtained. For all experiments, the purity of the colonies was checked both macroscopically (by the growth aspects) and microscopically (by the Gram stain). The assay was performed in triplicate. ∗∗∗p < 0.001.
Co-aggregation of Lactobacillus spp. with Genital Pathogens
A co-aggregation assay was performed to evaluate whether Lactobacillus strains interact directly with genital pathogens. All lactobacilli co-aggregated with the tested genital pathogens, with distinct levels of interaction (Table 2). The highest co-aggregation score was observed for L. fermentum, which was able to interact with all pathogens, in especial C. albicans (score = 4). L. paracasei exhibited a high ability to co-aggregate with both S. agalactiae (score = 4) and N. gonorrhoeae (score = 3), but a low degree of co-aggregation with C. albicans (score = 1). Overall, Lactobacillus strains presented a low ability to interact with S. agalactiae (score = 1); excepting L. fermentum (score = 3) and L. paracasei (score = 4) to which it was observed a greater capacity to co-aggregate with this pathogen in comparison with the other tested lactobacilli.
Antimicrobial Activity of Lactobacillus Strains against Genital Pathogens
We next assessed whether Lactobacillus spp. possess antimicrobial activity against genital pathogens. All lactobacilli inhibited pathogen growth, presenting inhibition zones ranging from 9.0 ± 0.8 to 19.6 ± 0.4 mm of diameter (Table 3; Supplementary Figures S1A–F). L. rhamnosus only reduced the growth of C. albicans in the area immediately above Lactobacillus growth, without producing a large inhibition zone (Supplementary Figure S1B). A similar activity was observed for L. delbrueckii ssp. delbrueckii when tested against S. agalactiae and N. gonorrhoeae (Supplementary Figures S1C,E).
Antimicrobial Activity of L. fermentum against Clinical Isolates of Candida spp.
As L. fermentum ATCC 23271 was the one that presented the greatest ability to form biofilm on surfaces, and the one that presented the greatest ability to co-aggregate with all tested pathogens, especially C. albicans, we carried out additional experiments in order to evaluate the antimicrobial effects of L. fermentum on clinical strains of Candida spp. Seven isolates of five species were tested in the same overlay inhibition assay and included C. albicans (two strains), C. glabrata (two strains), C. parapsilosis (one strain), C. tropicalis (one strain), and C. krusei (one strain). With the exception of C. krusei, all the remaining clinical strains had their growth inhibited by L. fermentum. A large inhibition zone was observed for one of the C. albicans isolates (18 mm), whereas the growth of the other Candida isolates were inhibited only in the area immediately above the Lactobacillus growth zone (Table 4).
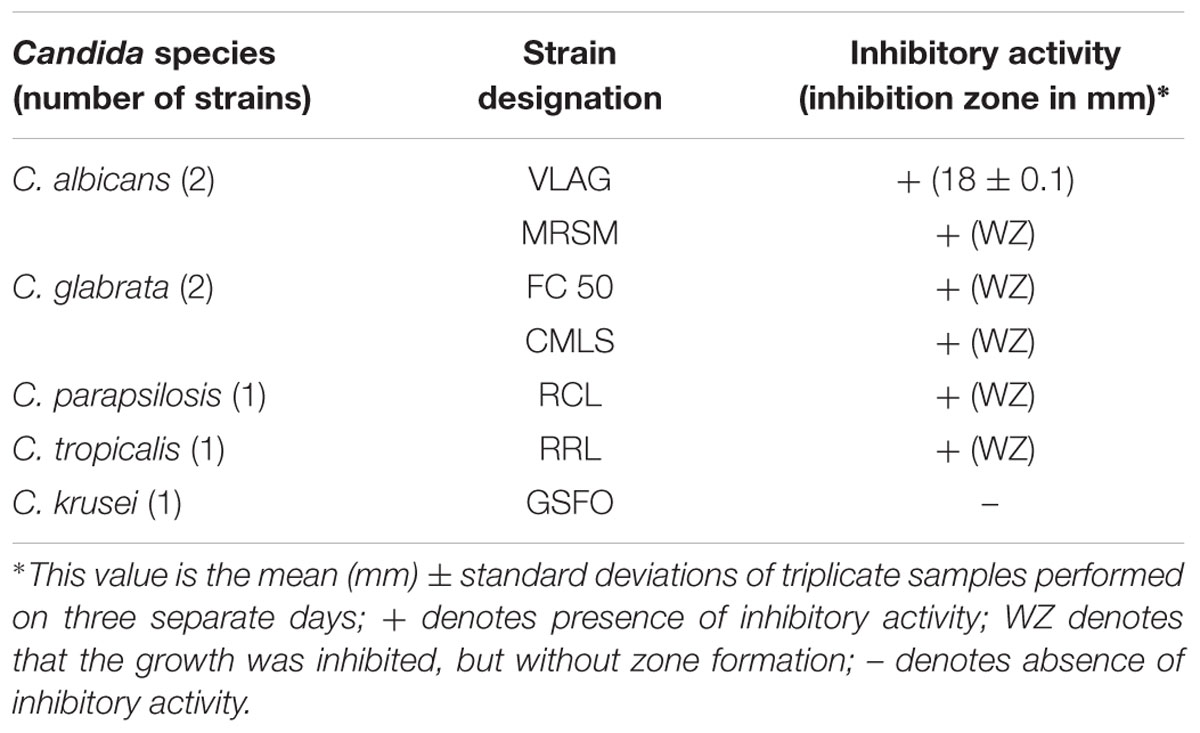
TABLE 4. Antimicrobial activity of L. fermentum ATCC 23270 against clinical isolates of Candida spp.
Discussion
Herein, we presented novel evidence on that Lactobacillus species that are less frequent in the female genital tract are potential probiotics. Although L. fermentum was not the best in terms of adhesion to HeLa cells and presented with similar effects to those of the other tested lactobacilli in regards of the exclusion and displacement assays, this bacteria was the one that presented the greatest ability to form biofilm and co-aggregate with all tested pathogens, especially C. albicans. We highlight that all lactobacilli tested only inhibited C. albicans in the displacement assay, thus suggesting a potential for treating vaginal infections caused by C. albicans.
Several parameters are considered in order to attribute probiotic properties to a microorganism. Criteria include (i) ability to adhere to host epithelial cells and mucus, (ii) biofilm production, (iii) capability to co-aggregate with bacterial pathogens, (iv) inhibition of pathogen binding to mucus and epithelial cells, and (v) antimicrobial activity (Andreu et al., 1995; Mastromarino et al., 2002; Martin et al., 2008; Van Tassell and Miller, 2011; Castro et al., 2013; Dhanani and Bagchi, 2013; Nishiyama et al., 2013).
We observed distinct adhesion profiles for the lactobacilli when tested with HeLa cells. This was expected as bacterial adhesion to cellular surfaces is multifactorial and involves different specific and non-specific interactions between bacterial and cell surface components. Non-specific mechanisms involve electrostatic and hydrophobic interactions, whereas the specific mechanisms are related to surface molecules, such as outer membrane proteins (Frece et al., 2005; Chen et al., 2007; Johnson-Henry et al., 2007; Zhang et al., 2013; Meng et al., 2014), exopolysaccharides (EPSs, Lebeer et al., 2011), lipoteichoic acids (Granato et al., 1999), peptidoglycans (Van Tassell and Miller, 2011), glycosaminoglycans (Martin et al., 2013), flagella, and fimbriae (Singh et al., 2013). This complex scenario results in species-specific adhesion (Deepika and Charalampopoulos, 2010; Messaoudi et al., 2012; Verdenelli et al., 2014) and may be related to the differences observed in the present study.
All the analyzed Lactobacillus species exhibited strong mucin binding at levels even higher (1 log10 difference) than those previously reported for other strains (Tallon et al., 2007). The main component of cervical mucus is mucin, which functions as a protective barrier against invasive pathogens in the uterus and vagina. An ideal probiotic may exhibit increased binding to mucus to facilitate its permanence and growth in the female genital tract, similar to their binding in the gastrointestinal tract (Perea Velez et al., 2007). Porcine intestinal mucin has been widely used to identifying the bind capacity of a certain bacteria to mucus (Tuomola et al., 1999; Izquierdo et al., 2008; Laparra and Sanz, 2009). We found that L. acidophilus ATCC 4356 and L. paracasei ATCC 335 poorly adhere to HeLa cells, although they are able to bind to mucin, suggesting these lactobacilli present mucin-specific surface lectins. On the other hand, L. brevis ATCC 367, L. fermentum ATCC 23271, L. delbrueckii ssp. delbrueckii ATCC 9645, L. plantarum ATCC 8014, and L. rhamnosus ATCC 9595 were able to adhere to HeLa cells and to bind to immobilized mucin, possibly due to their hydrophobic surfaces and/or expression of some adhesins. Mucin binding by probiotics is considered as a pre-requisite for creating a protective barrier against pathogen adhesion to epithelial cells by either occupying mucin-binding sites or producing antimicrobial compounds (Messaoudi et al., 2012; Zhang et al., 2013). It is possible that the greater the ability of Lactobacillus spp. to bind to mucin, the greater their ability to impair pathogen binding to the genital tract. It was previously described that microorganisms with a hydrophobic cell wall harbor a large variety of surface glycoproteins, increasing the probability of adhesion to cellular receptors (Kos et al., 2003) or to cell wall-anchored proteins (Polak-Berecka et al., 2014; Zhang et al., 2015). Indeed, adhesion-promoting proteins that are involved in the binding of distinct Lactobacillus species to mucin have been previously identified, including a mucin adhesion-promoting protein (MAPP) in L. fermentum strain 104R (Rojas et al., 2002), the elongation factor Tu in L. johnsonii strain La1 (Granato et al., 2004), and a fibronectin-binding protein (FbpB) in L. acidophilus strain NCFM (Hymes et al., 2016).
Interaction with mucin allows the lactobacilli to remain within the mucus layers, thereby contributing to the formation of multi-species biofilms (Wickstrom et al., 2013), which are essential for protection against intruding pathogens (Pi et al., 2011). Amongst the tested Lactobacillus species, L. fermentum ATCC 23271 was the only to produce moderate biofilm on plastic surface in comparison with other lactobacilli. Biofilm formation by Lactobacillus species is influenced by many variables, including the culture medium, the concentration of the inoculum, the tested strain, and even the chemical nature of the support used for the assay (Terraf et al., 2012). All tested genital pathogens adhered to mucin at the same level as the lactobacilli (>105 CFU/ml). It is possible that in order to establish a genital infection, a successful pathogen has to overcome Lactobacillus colonization thus promoting host cell adherence and/or invasion. To test this hypothesis, we performed two competitive binding assays to mucin, exclusion (Lactobacillus added and then, pathogen) and displacement (pathogen added and then, Lactobacillus). Interestingly, the exclusion assay indicated lactobacilli enhance pathogen adherence to mucin. A previous study reported that some lactobacilli isolated from the vaginal tract enhance the adherence of C. albicans and Actinomyces neuii to human epithelial cells in vitro (Martin et al., 2012). The same study suggested that secretion of aggregation proteins by lactobacilli might favor a positive interaction of pathogens within host cells. Additional studies have suggested that expression of EPSs may enhance the interaction of pathogens with host cells (Marcotte et al., 2004; Ruas-Madiedo et al., 2006; Macias-Rodriguez et al., 2009). Apparently, this increased adhesion of pathogens might be explained by the increased binding of pathogens to specific EPSs, which in turn, enhanced adherence to mucus (Ruas-Madiedo et al., 2006). Although these events have been observed for enteropathogens, they may also occur for genital pathogens.
On the other hand, all lactobacilli inhibited the adhesion of C. albicans in the displacement assay, suggesting they could potentially be used to complement the treatment of infections caused by this pathogen, at least as adjuvants. C. albicans displacement may be attributed to the production of antimicrobial substances by the lactobacilli, as supported by the antimicrobial activity assay. Furthermore, Lactobacillus species exhibited inhibitory effects against yeasts at low concentrations (105 CFU/ml). This finding may be of clinical relevance for probiotic therapy in immunocompromised patients in whom high concentrations of these microorganisms can trigger clinical complications (Kirjavainen et al., 1999; Apostolou et al., 2001; Ren et al., 2012). None of the tested lactobacilli affected either S. agalactiae or N. gonorrhoeae in the displacement assay. It is possible that these bacteria express proteins (or other components) that bind with high affinity to mucin, and consequently, this has hindered the displacement effect for the lactobacilli analyzed. In fact, adhesins involved in host cell colonization and in the interaction to host extracellular components have been described for both pathogens (Edwards and Apicella, 2004; Lalioui et al., 2005).
In the present study, lactobacilli presented with different co-aggregation activities. L. fermentum co-aggregated most strongly with the genital pathogens C. albicans, N. gonorrhoeae, and S. agalactiae. In agreement with previous studies, Lactobacillus species, including L. acidophilus, L. gasseri, amongst others, exhibit different co-aggregation activities with the same pathogens, suggesting a species-specific process (Boris et al., 1998; Osset et al., 2001). The formation of co-aggregates has been suggested as a key event for the elimination of pathogens as this close interaction allows the lactobacilli to create an adverse microenvironment for pathogens by delivering antimicrobial substances in a localized manner, and thus, impairing epithelial colonization (Mastromarino et al., 2002; Younes et al., 2012). Herein, all tested Lactobacillus strains were inhibitory against the tested genital pathogens. This is in agreement with previous reports showing that Lactobacillus species, including those assessed in our study, are able to produce antibacterial metabolites, such as bacteriocins (Todorov et al., 2011), biosurfactants, organic acids, and H2O2 (Verdenelli et al., 2014); which can act in synergy, reducing pathogen survival.
The probiotic properties of L. fermentum ATCC 23271 observed for the reference strain of C. albicans as well as the growth inhibition of Candida clinical isolates suggest that it is possible this Lactobacillus may be useful for the treatment or prophylaxis of vaginal candidiasis. Interestingly, L. fermentum inhibited the growth of two of the most relevant Candida species involved in vaginal candidiasis: C. albicans, which can be found in 80–90% of cases and C. glabrata, which is the second most common species, being responsible for 5–15% of the vaginal candidiasis cases (Ferrer, 2000; Sobel, 2007). Indeed, L. fermentum has been previously shown to inhibit pathogen growth. In a recent study, the growth and virulence of Escherichia coli and Gardnerella vaginalis were inhibited by the L. fermentum strain SK5 (Kaewnopparat et al., 2013). Similar effects were observed for the L. fermentum strain Ess-1, which was shown to inhibit C. albicans and C. glabrata growth (Rönnqvist et al., 2007) and for L. fermentum HV6b MTCC 10770, which was able to reduce the growth of Bacteroides, G. vaginalis, Mobiluncus, Staphylococcus spp., and Streptococcus spp L. fermentum antimicrobial actions have been attributed to its ability to produce a bacteriocin-like compound (Sabia et al., 2014) and to secrete the antimicrobial peptide fermenticin HV6b (Kaur et al., 2013). Additionally, Pascual et al. (2010) demonstrated that L. fermentum L23 presents in vivo probiotic actions against vaginitis by treating and preventing E. coli-induced infections.
Collectively, our data show for the first time that L. fermentum ATCC 23271 is a prominent probiotic, exhibiting the highest levels of adhesion to HeLa cells and binding to mucin, in addition to antimicrobial properties against pathogens of importance to female genital tract infections, particularly against Candida spp. Herein, L. fermentum presented with the best probiotic profile in comparison with the other tested lactobacilli species. Finally, we suggest that L. fermentum could be used alone or in combination with other species in order to treat genital infections.
Author Contributions
MC designed and performed the experiments, contributed to data interpretation, manuscript writing, and approval of the final version for publication; FN, MA, EdS, and MB performed the experiments, contributed to data interpretation, and approval of the final version for publications; AM and TF contributed to data interpretation and revised the manuscript; EF and JG contributed to data interpretation, revised the manuscript, and approved the final version for publication; VM-N conceived the study, participated in its design and coordination, and critically revised the manuscript.
Funding
This study was supported by the Foundation for Research Support and to Scientific and Technological Development of Maranhão (FAPEMA, grant award 01041/13) and by the National Council for Scientific and Technological Development (CNPq, grant award 482037).
Conflict of Interest Statement
The authors declare that the research was conducted in the absence of any commercial or financial relationships that could be construed as a potential conflict of interest.
Supplementary Material
The Supplementary Material for this article can be found online at: http://journal.frontiersin.org/article/10.3389/fmicb.2016.01722/full#supplementary-material
References
Alexandre, Y., Le Berre, R., Barbier, G., and Le Blay, G. (2014). Screening of Lactobacillus spp. for the prevention of Pseudomonas aeruginosa pulmonary infections. BMC Microbiol. 14:107. doi: 10.1186/1471-2180-14-107
Andreu, A., Stapleton, A. E., Fennell, C. L., Hillier, S. L., and Stamm, W. E. (1995). Hemagglutination, adherence, and surface properties of vaginal Lactobacillus species. J. Infect. Dis. 171, 1237–1243. doi: 10.1093/infdis/171.5.1237
Antonio, M. A., Meyn, L. A., Murray, P. J., Busse, B., and Hillier, S. L. (2009). Vaginal colonization by probiotic Lactobacillus crispatus CTV-05 is decreased by sexual activity and endogenous Lactobacilli. J. Infect. Dis. 199, 1506–1513. doi: 10.1086/598686
Apostolou, E., Kirjavainen, P. V., Saxelin, M., Rautelin, H., Valtonen, V., Salminen, S. J., et al. (2001). Good adhesion properties of probiotics: a potential risk for bacteremia? FEMS Immunol. Med. Microbiol. 31, 35–39. doi: 10.1111/j.1574-695X.2001.tb01583.x
Boris, S., Suarez, J. E., Vazquez, F., and Barbes, C. (1998). Adherence of human vaginal lactobacilli to vaginal epithelial cells and interaction with uropathogens. Infect. Immun. 66, 1985–1989.
Castro, J., Henriques, A., Machado, A., Henriques, M., Jefferson, K. K., and Cerca, N. (2013). Reciprocal interference between Lactobacillus spp. and Gardnerella vaginalis on initial adherence to epithelial cells. Int. J. Med. Sci. 10, 1193–1198. doi: 10.7150/ijms.6304
Chen, X., Xu, J., Shuai, J., Chen, J., Zhang, Z., and Fang, W. (2007). The S-layer proteins of Lactobacillus crispatus strain ZJ001 is responsible for competitive exclusion against Escherichia coli O157:H7 and Salmonella typhimurium. Int. J. Food Microbiol. 115, 307–312. doi: 10.1016/j.ijfoodmicro.2006.11.007
Deepika, G., and Charalampopoulos, D. (2010). Surface and adhesion properties of lactobacilli. Adv. Appl. Microbiol. 70, 127–152. doi: 10.1016/S0065-2164(10)70004-6
Dhanani, A. S., and Bagchi, T. (2013). The expression of adhesin EF-Tu in response to mucin and its role in Lactobacillus adhesion and competitive inhibition of enteropathogens to mucin. J. Appl. Microbiol. 115, 546–554. doi: 10.1111/jam.12249
Donders, G. G., Vereecken, A., Bosmans, E., Dekeersmaecker, A., Salembier, G., and Spitz, B. (2002). Definition of a type of abnormal vaginal flora that is distinct from bacterial vaginosis: aerobic vaginitis. BJOG 109, 34–43. doi: 10.1111/j.1471-0528.2002.00432.x
Edwards, J. L., and Apicella, M. A. (2004). The molecular mechanisms used by Neisseria gonorrhoeae to initiate infection differ between men and women. Clin. Microbiol. Rev. 17, 965–981. doi: 10.1128/CMR.17.4.965-981.2004
Ferrer, J. (2000). Vaginal candidosis: epidemiological and etiological factors. Int. J. Gynaecol. Obstet. 71(Suppl. 1), S21–S27. doi: 10.1016/S0020-7292(00)00350-7
Ferris, M. J., Norori, J., Zozaya-Hinchliffe, M., and Martin, D. H. (2007). Cultivation-independent analysis of changes in bacterial vaginosis flora following metronidazole treatment. J. Clin. Microbiol. 45, 1016–1018. doi: 10.1128/JCM.02085-06
Frece, J., Kos, B., Svetec, I. K., Zgaga, Z., Mrsa, V., and Suskovic, J. (2005). Importance of S-layer proteins in probiotic activity of Lactobacillus acidophilus M92. J. Appl. Microbiol. 98, 285–292. doi: 10.1111/j.1365-2672.2004.02473.x
Gorska, S., Buda, B., Brzozowska, E., Schwarzer, M., Srutkova, D., Kozakova, H., et al. (2016). Identification of Lactobacillus proteins with different recognition patterns between immune rabbit sera and nonimmune mice or human sera. BMC Microbiol. 16:17. doi: 10.1186/s12866-016-0631-9
Granato, D., Bergonzelli, G. E., Pridmore, R. D., Marvin, L., Rouvet, M., and Corthesy-Theulaz, I. E. (2004). Cell surface-associated elongation factor Tu mediates the attachment of Lactobacillus johnsonii NCC533 (La1) to human intestinal cells and mucins. Infect. Immun. 72, 2160–2169. doi: 10.1128/IAI.72.4.2160-2169.2004
Granato, D., Perotti, F., Masserey, I., Rouvet, M., Golliard, M., Servin, A., et al. (1999). Cell surface-associated lipoteichoic acid acts as an adhesion factor for attachment of Lactobacillus johnsonii La1 to human enterocyte-like Caco-2 cells. Appl. Environ. Microbiol. 65, 1071–1077.
Hansen, S. M., Uldbjerg, N., Kilian, M., and Sorensen, U. B. (2004). Dynamics of Streptococcus agalactiae colonization in women during and after pregnancy and in their infants. J. Clin. Microbiol. 42, 83–89. doi: 10.1128/JCM.42.1.83-89.2004
Hymes, J. P., Johnson, B. R., Barrangou, R., and Klaenhammer, T. R. (2016). Functional analysis of an S-layer associated fibronectin-binding protein in Lactobacillus acidophilus NCFM. Appl. Environ. Microbiol. 82, 2676–2685. doi: 10.1128/AEM.00024-16
Izquierdo, E., Medina, M., Ennahar, S., Marchioni, E., and Sanz, Y. (2008). Resistance to simulated gastrointestinal conditions and adhesion to mucus as probiotic criteria for Bifidobacterium longum strains. Curr. Microbiol. 56, 613–618. doi: 10.1007/s00284-008-9135-7
Johnson-Henry, K. C., Hagen, K. E., Gordonpour, M., Tompkins, T. A., and Sherman, P. M. (2007). Surface-layer protein extracts from Lactobacillus helveticus inhibit enterohaemorrhagic Escherichia coli O157:H7 adhesion to epithelial cells. Cell Microbiol. 9, 356–367. doi: 10.1111/j.1462-5822.2006.00791.x
Kaewnopparat, S., Dangmanee, N., Kaewnopparat, N., Srichana, T., Chulasiri, M., and Settharaksa, S. (2013). In vitro probiotic properties of Lactobacillus fermentum SK5 isolated from vagina of a healthy woman. Anaerobe 22, 6–13. doi: 10.1016/j.anaerobe.2013.04.009
Kaur, B., Balgir, P. P., Mittu, B., Kumar, B., and Garg, N. (2013). Biomedical applications of fermenticin HV6b isolated from Lactobacillus fermentum HV6b MTCC10770. Biomed. Res. Int. 2013:168438. doi: 10.1155/2013/168438
Kirjavainen, P. V., Tuomola, E. M., Crittenden, R. G., Ouwehand, A. C., Harty, D. W., Morris, L. F., et al. (1999). In vitro adhesion and platelet aggregation properties of bacteremia-associated lactobacilli. Infect. Immun. 67, 2653–2655.
Kos, B., Suskovic, J., Vukovic, S., Simpraga, M., Frece, J., and Matosic, S. (2003). Adhesion and aggregation ability of probiotic strain Lactobacillus acidophilus M92. J. Appl. Microbiol. 94, 981–987. doi: 10.1046/j.1365-2672.2003.01915.x
Lalioui, L., Pellegrini, E., Dramsi, S., Baptista, M., Bourgeois, N., Doucet-Populaire, F., et al. (2005). The SrtA Sortase of Streptococcus agalactiae is required for cell wall anchoring of proteins containing the LPXTG motif, for adhesion to epithelial cells, and for colonization of the mouse intestine. Infect. Immun. 73, 3342–3350. doi: 10.1128/IAI.73.6.3342-3350.2005
Laparra, J. M., and Sanz, Y. (2009). Comparison of in vitro models to study bacterial adhesion to the intestinal epithelium. Lett. Appl. Microbiol. 49, 695–701. doi: 10.1111/j.1472-765X.2009.02729.x
Lebeer, S., Claes, I. J., Verhoeven, T. L., Vanderleyden, J., and De Keersmaecker, S. C. (2011). Exopolysaccharides of Lactobacillus rhamnosus GG form a protective shield against innate immune factors in the intestine. Microb. Biotechnol. 4, 368–374. doi: 10.1111/j.1751-7915.2010.00199.x
Li, J., McCormick, J., Bocking, A., and Reid, G. (2012). Importance of vaginal microbes in reproductive health. Reprod. Sci. 19, 235–242. doi: 10.1177/1933719111418379
Lidbeck, A., and Nord, C. E. (1993). Lactobacilli and the normal human anaerobic microflora. Clin. Infect. Dis. 16(Suppl. 4), S181–S187. doi: 10.1093/clinids/16.Supplement_4.S181
Lobos, O., Padilla, A., and Padilla, C. (2013). [Genetic analysis and virulent properties of Escherichia coli strains isolated from vaginal infection]. Rev. Chilena Infectol. 30, 381–387. doi: 10.4067/S0716-10182013000400005
Macias-Rodriguez, M. E., Zagorec, M., Ascencio, F., Vazquez-Juarez, R., and Rojas, M. (2009). Lactobacillus fermentum BCS87 expresses mucus- and mucin-binding proteins on the cell surface. J. Appl. Microbiol. 107, 1866–1874. doi: 10.1111/j.1365-2672.2009.04368.x
Marcotte, H., Ferrari, S., Cesena, C., Hammarstrom, L., Morelli, L., Pozzi, G., et al. (2004). The aggregation-promoting factor of Lactobacillus crispatus M247 and its genetic locus. J. Appl. Microbiol. 97, 749–756. doi: 10.1111/j.1365-2672.2004.02364.x
Martin, R., Martin, C., Escobedo, S., Suarez, J. E., and Quiros, L. M. (2013). Surface glycosaminoglycans mediate adherence between HeLa cells and Lactobacillus salivarius Lv72. BMC Microbiol. 13:210. doi: 10.1186/1471-2180-13-210
Martin, R., Sanchez, B., Suarez, J. E., and Urdaci, M. C. (2012). Characterization of the adherence properties of human Lactobacilli strains to be used as vaginal probiotics. FEMS Microbiol. Lett. 328, 166–173. doi: 10.1111/j.1574-6968.2011.02495.x
Martin, R., Soberon, N., Vazquez, F., and Suarez, J. E. (2008). [Vaginal microbiota: composition, protective role, associated pathologies, and therapeutic perspectives]. Enferm. Infecc. Microbiol. Clin. 26, 160–167.
Mastromarino, P., Brigidi, P., Macchia, S., Maggi, L., Pirovano, F., Trinchieri, V., et al. (2002). Characterization and selection of vaginal Lactobacillus strains for the preparation of vaginal tablets. J. Appl. Microbiol. 93, 884–893. doi: 10.1046/j.1365-2672.2002.01759.x
Medellin-Pena, M. J., and Griffiths, M. W. (2009). Effect of molecules secreted by Lactobacillus acidophilus strain La-5 on Escherichia coli O157:H7 colonization. Appl. Environ. Microbiol. 75, 1165–1172. doi: 10.1128/AEM.01651-08
Meng, J., Zhu, X., Gao, S. M., Zhang, Q. X., Sun, Z., and Lu, R. R. (2014). Characterization of surface layer proteins and its role in probiotic properties of three Lactobacillus strains. Int. J. Biol. Macromol. 65, 110–114. doi: 10.1016/j.ijbiomac.2014.01.024
Messaoudi, S., Madi, A., Prevost, H., Feuilloley, M., Manai, M., Dousset, X., et al. (2012). In vitro evaluation of the probiotic potential of Lactobacillus salivarius SMXD51. Anaerobe 18, 584–589. doi: 10.1016/j.anaerobe.2012.10.004
Nami, Y., Abdullah, N., Haghshenas, B., Radiah, D., Rosli, R., and Khosroushahi, A. Y. (2014). Probiotic potential and biotherapeutic effects of newly isolated vaginal Lactobacillus acidophilus 36YL strain on cancer cells. Anaerobe 28, 29–36. doi: 10.1016/j.anaerobe.2014.04.012
Nishiyama, K., Ochiai, A., Tsubokawa, D., Ishihara, K., Yamamoto, Y., and Mukai, T. (2013). Identification and characterization of sulfated carbohydrate-binding protein from Lactobacillus reuteri. PLoS ONE 8:e83703. doi: 10.1371/journal.pone.0083703
Osset, J., Garcia, E., Bartolome, R. M., and Andreu, A. (2001). [Role of Lactobacillus as protector against vaginal candidiasis]. Med. Clin. (Barc) 117, 285–288. doi: 10.1016/S0025-7753(01)72089-1
Pascual, L., Ruiz, F., Giordano, W., and Barberis, I. L. (2010). Vaginal colonization and activity of the probiotic bacterium Lactobacillus fermentum L23 in a murine model of vaginal tract infection. J. Med. Microbiol. 59, 360–364. doi: 10.1099/jmm.0.012583-0
Perea Velez, M., Hermans, K., Verhoeven, T. L., Lebeer, S. E., Vanderleyden, J., and De Keersmaecker, S. C. (2007). Identification and characterization of starter lactic acid bacteria and probiotics from Columbian dairy products. J. Appl. Microbiol. 103, 666–674. doi: 10.1111/j.1365-2672.2007.03294.x
Pi, W., Ryu, J. S., and Roh, J. (2011). Lactobacillus acidophilus contributes to a healthy environment for vaginal epithelial cells. Korean J. Parasitol. 49, 295–298. doi: 10.3347/kjp.2011.49.3.295
Polak-Berecka, M., Wasko, A., Paduch, R., Skrzypek, T., and Sroka-Bartnicka, A. (2014). The effect of cell surface components on adhesion ability of Lactobacillus rhamnosus. Antonie Van Leeuwenhoek 106, 751–762. doi: 10.1007/s10482-014-0245-x
Puopolo, K. M., Madoff, L. C., and Eichenwald, E. C. (2005). Early-onset group B streptococcal disease in the era of maternal screening. Pediatrics 115, 1240–1246. doi: 10.1542/peds.2004-2275
Ravel, J., Gajer, P., Abdo, Z., Schneider, G. M., Koenig, S. S., McCulle, S. L., et al. (2011). Vaginal microbiome of reproductive-age women. Proc. Natl. Acad. Sci. U.S.A. 108(Suppl. 1), 4680–4687. doi: 10.1073/pnas.1002611107
Reid, G., McGroarty, J. A., Domingue, P. G., Chow, A. W., Bruce, A. W., Eisen, A., et al. (1990). Coaggregation of urogenital bacteria in vitro and in vivo. Curr. Microbiol. 20, 47–52. doi: 10.1007/BF02094024
Ren, D., Li, C., Qin, Y., Yin, R., Li, X., Tian, M., et al. (2012). Inhibition of Staphylococcus aureus adherence to Caco-2 cells by lactobacilli and cell surface properties that influence attachment. Anaerobe 18, 508–515. doi: 10.1016/j.anaerobe.2012.08.001
Rojas, M., Ascencio, F., and Conway, P. L. (2002). Purification and characterization of a surface protein from Lactobacillus fermentum 104R that binds to porcine small intestinal mucus and gastric mucin. Appl. Environ. Microbiol. 68, 2330–2336. doi: 10.1128/AEM.68.5.2330-2336.2002
Rönnqvist, D., Forsgren-Brusk, U., Husmark, U., and Grahn-Håkansson, E. (2007). Lactobacillus fermentum Ess-1 with unique growth inhibition of vulvo-vaginal candidiasis pathogens. J. Med. Microbiol. 56, 1500–1504. doi: 10.1099/jmm.0.47226-0
Ruas-Madiedo, P., Gueimonde, M., Margolles, A., de los Reyes-Gavilan, C. G., and Salminen, S. (2006). Exopolysaccharides produced by probiotic strains modify the adhesion of probiotics and enteropathogens to human intestinal mucus. J. Food Prot. 69, 2011–2015.
Sabia, C., Anacarso, I., Bergonzini, A., Gargiulo, R., Sarti, M., Condò, C., et al. (2014). Detection and partial characterization of a bacteriocin-like substance produced by Lactobacillus fermentum CS57 isolated from human vaginal secretions. Anaerobe 26, 41–45. doi: 10.1016/j.anaerobe.2014.01.004
Singh, D., von Ossowski, I., Palva, A., and Krishnan, V. (2013). Purification, crystallization and preliminary crystallographic analysis of the SpaA backbone-pilin subunit from probiotic Lactobacillus rhamnosus GG. Acta Crystallogr. Sect. F Struct. Biol. Cryst. Commun. 69(Pt 10), 1182–1185. doi: 10.1107/S1744309113024676
Sobel, J. D. (2007). Vulvovaginal candidosis. Lancet 369, 1961–1971. doi: 10.1016/S0140-6736(07)60917-9
Spurbeck, R. R., and Arvidson, C. G. (2010). Lactobacillus jensenii surface-associated proteins inhibit Neisseria gonorrhoeae adherence to epithelial cells. Infect. Immun. 78, 3103–3111. doi: 10.1128/IAI.01200-09
Stepanovic, S., Vukovic, D., Dakic, I., Savic, B., and Svabic-Vlahovic, M. (2000). A modified microtiter-plate test for quantification of staphylococcal biofilm formation. J. Microbiol. Methods 40, 175–179. doi: 10.1016/S0167-7012(00)00122-6
Tallon, R., Arias, S., Bressollier, P., and Urdaci, M. (2007). Strain-and matrix-dependent adhesion of Lactobacillus plantarum is mediated by proteinaceous bacterial compounds. J. Appl. Microbiol. 102, 442–451. doi: 10.1111/j.1365-2672.2006.03086.x
Terraf, M. C., Juarez Tomas, M. S., Nader-Macias, M. E., and Silva, C. (2012). Screening of biofilm formation by beneficial vaginal lactobacilli and influence of culture media components. J. Appl. Microbiol. 113, 1517–1529. doi: 10.1111/j.1365-2672.2012.05429.x
Todorov, S. D., Furtado, D. N., Saad, S. M., and Gombossy de Melo Franco, B. D. (2011). Bacteriocin production and resistance to drugs are advantageous features for Lactobacillus acidophilus La-14, a potential probiotic strain. New Microbiol. 34, 357–370.
Tuomola, E., Ouwehand, A., and Salminen, S. (1999). Human ileostomy glycoproteins as a model for small intestinal mucus to investigate adhesion of probiotics. Lett. Appl. Microbiol. 28, 159–163. doi: 10.1046/j.1365-2672.1999.00518.x
Van Tassell, M. L., and Miller, M. J. (2011). Lactobacillus adhesion to mucus. Nutrients 3, 613–636. doi: 10.3390/nu3050613
Verdenelli, M. C., Coman, M. M., Cecchini, C., Silvi, S., Orpianesi, C., and Cresci, A. (2014). Evaluation of antipathogenic activity and adherence properties of human Lactobacillus strains for vaginal formulations. J. Appl. Microbiol. 116, 1297–1307. doi: 10.1111/jam.12459
Walencka, E., Rozalska, S., Sadowska, B., and Rozalska, B. (2008). The influence of Lactobacillus acidophilus-derived surfactants on staphylococcal adhesion and biofilm formation. Folia Microbiol. (Praha) 53, 61–66. doi: 10.1007/s12223-008-0009-y
Wang, Z. L., Fu, L. Y., Xiong, Z. A., Qin, Q., Yu, T. H., Wu, Y. T., et al. (2016). Diagnosis and microecological characteristics of aerobic vaginitis in outpatients based on preformed enzymes. Taiwan J Obstet Gynecol 55, 40–44. doi: 10.1016/j.tjog.2015.06.012
WHO (2012). Global incidence and prevalence of selected curable sexually transmitted infections – 2008. Geneva: World Health Organization.
Wickstrom, C., Chavez de Paz, L., Davies, J. R., and Svensater, G. (2013). Surface-associated MUC5B mucins promote protease activity in Lactobacillus fermentum biofilms. BMC Oral Health 13:43. doi: 10.1186/1472-6831-13-43
Witkin, S. S., Linhares, I. M., and Giraldo, P. (2007). Bacterial flora of the female genital tract: function and immune regulation. Best Pract. Res. Clin. Obstet. Gynaecol. 21, 347–354. doi: 10.1016/j.bpobgyn.2006.12.004
Younes, J. A., van der Mei, H. C., van den Heuvel, E., Busscher, H. J., and Reid, G. (2012). Adhesion forces and coaggregation between vaginal staphylococci and lactobacilli. PLoS ONE 7:e36917. doi: 10.1371/journal.pone.0036917
Zabihollahi, R., Motevaseli, E., Sadat, S. M., Azizi-Saraji, A. R., Asaadi-Dalaie, S., and Modarressi, M. H. (2012). Inhibition of HIV and HSV infection by vaginal lactobacilli in vitro and in vivo. Daru 20:53. doi: 10.1186/2008-2231-20-53
Zhang, B., Zuo, F., Yu, R., Zeng, Z., Ma, H., and Chen, S. (2015). Comparative genome-based identification of a cell wall-anchored protein from Lactobacillus plantarum increases adhesion of Lactococcus lactis to human epithelial cells. Sci. Rep. 5:14109. doi: 10.1038/srep14109
Zhang, R., Daroczy, K., Xiao, B., Yu, L., Chen, R., and Liao, Q. (2012). Qualitative and semiquantitative analysis of Lactobacillus species in the vaginas of healthy fertile and postmenopausal Chinese women. J. Med. Microbiol. 61(Pt 5), 729–739. doi: 10.1099/jmm.0.038687-0
Zhang, W., Wang, H., Liu, J., Zhao, Y., Gao, K., and Zhang, J. (2013). Adhesive ability means inhibition activities for Lactobacillus against pathogens and S-layer protein plays an important role in adhesion. Anaerobe 22, 97–103. doi: 10.1016/j.anaerobe.2013.06.005
Keywords: Lactobacillus fermentum, probiotic, genital infections, STD, Candida
Citation: Carmo MS, Noronha FMF, Arruda MO, Costa ÊPS, Bomfim MRQ, Monteiro AS, Ferro TAF, Fernandes ES, Girón JA and Monteiro-Neto V (2016) Lactobacillus fermentum ATCC 23271 Displays In vitro Inhibitory Activities against Candida spp. Front. Microbiol. 7:1722. doi: 10.3389/fmicb.2016.01722
Received: 29 June 2016; Accepted: 13 October 2016;
Published: 27 October 2016.
Edited by:
Maria Tereza Dos Santos Correia, Federal University of Pernambuco, BrazilReviewed by:
Kimberly B. Ritchie, Mote Marine Laboratory, USARaffaele Coppola, University of Molise, Italy
Copyright © 2016 Carmo, Noronha, Arruda, Costa, Bomfim, Monteiro, Ferro, Fernandes, Girón and Monteiro-Neto. This is an open-access article distributed under the terms of the Creative Commons Attribution License (CC BY). The use, distribution or reproduction in other forums is permitted, provided the original author(s) or licensor are credited and that the original publication in this journal is cited, in accordance with accepted academic practice. No use, distribution or reproduction is permitted which does not comply with these terms.
*Correspondence: Valério Monteiro-Neto, valerio.monteiro@ceuma.br