- Department of Microbiology and Molecular Genetics, University of the Punjab, Lahore, Pakistan
In this study 98 rhizospheric actinomycetes were isolated from different wheat and tomato fields, Punjab, Pakistan. The isolates were characterized morphologically, biochemically, and genetically and were subjected to a comprehensive in vitro screening for various plant growth promoting (PGP) traits. About 30% of the isolates screened were found to be the promising PGP rhizobacteria (PGPRs), which exhibited maximum genetic similarity (up to 98–99%) with different species of the genus Streptomyces by using16S rRNA gene sequencing. The most active indole acetic acid (IAA) producer Streptomyces nobilis WA-3, Streptomyces Kunmingenesis WC-3, and Streptomyces enissocaesilis TA-3 produce 79.5, 79.23, and 69.26 μg/ml IAA respectively at 500 μg/ml L-tryptophan. The highest concentration of soluble phosphate was produced by Streptomyces sp. WA-1 (72.13 mg/100 ml) and S. djakartensis TB-4 (70.36 mg/100 ml). All rhizobacterial isolates were positive for siderophore, ammonia, and hydrogen cyanide production. Strain S. mutabilis WD-3 showed highest concentration of ACC-deaminase (1.9 mmol /l). For in-vivo screening, seed germination, and plant growth experiment were conducted by inoculating wheat (Triticum aestivum) seeds with the six selected isolates. Significant increases in shoot length was observed with S. nobilis WA-3 (65%), increased root length was recorded in case of S. nobilis WA-3 (81%) as compared to water treated control plants. Maximum increases in plant fresh weight were recorded with S. nobilis WA-3 (84%), increased plant dry weight was recorded in case of S. nobilis WA-3 (85%) as compared to water treated control plants. In case of number of leaves, significant increase was recorded with S. nobilis WA-3 (27%) and significant increase in case of number of roots were recorded in case of strain S. nobilis WA-3 (30%) as compared to control plants. Over all the study revealed that these rhizospheric PGP Streptomyces are good candidates to be developed as bioferlizers for growth promotion and yield enhancement in wheat crop and can be exploited for the commercial production of different agro-active compounds.
Introduction
Effective farming practices, now-a-days, rely on extensive use of chemical fertilizers in order to enhance plant growth and yield. However, the cost, environmental concerns and the resulting human health hazards due to the inclusion of these chemical fertilizers in food chain are the major limiting factors. Microorganisms have been considered an important source of natural compounds of agro active importance. Use of microbial consortia in the form of bio fertilizers for reduction in the application of chemical fertilizers, pesticides and related agrochemicals, without compromising the plant yield is currently a significant research area in the field of agriculture, microbiology, and biotechnology (Ahmad et al., 2008).
Plant growth promoting rhizobacteria (PGPR) is a group of naturally occurring, free living rhizosphere colonizing bacteria that improve plant growth, increase yield, enhance soil fertility, and reduce pathogens as well as biotic or abiotic stresses (Vessey, 2003; Kumar et al., 2014). PGPR help the plants by producing plant growth phytohormones such as indole acetic acid (IAA), cytokinins, and gibberellins (Marques et al., 2010), solubilization of inorganic phosphate (Jeon et al., 2003), asymbiotic nitrogen fixation (Khan, 2005), antagonistic effect against phytopathogenic microorganisms by producing siderophore, antibiotics, and fungicidal compounds (Lucy et al., 2004; Barriuso et al., 2008; Majeed et al., 2015).
Actinomycetes are present extensively in the plant rhizosphere and produce various agroactive compounds. In the last few years, this group of bacteria, due to its strong antimicrobial potential, and soil dominant saprophytic nature, gained much attention as plant growth promoters (PGP; Franco-Correa et al., 2010). Actinobacteria can actively colonize plant root systems, can degrade a wide range of biopolymers by secreting several hydrolytic enzymes and tolerate hostile conditions by forming spores (Alexander, 1977). Actinobacteria, especially Streptomyces, also exhibit immense biocontrol action against a range of phytopathogens (Wang et al., 2013). Actinobacteria can produce phytohormones (IAA) and siderophore as well as solubilize phosphate and promote plant growth (Jeon et al., 2003). Actinomycetes have been mainly exploited in pharmaceutical industry since 1940s, Whereas, only a few have been developed as commercial products for plant application in agriculture (Minuto et al., 2006). Streptomycetes have been long considered simply as free-living soil inhabitants, but recently the importance of their complex interactions with plants, and other organisms is being uncovered (Seipke et al., 2011).
Interest in the beneficial rhizobacteria associated with cereals has increased recently and several studies clearly demonstrated the positive and beneficial effects of PGPR on growth and yield of different crops especially wheat at different environments under variable ecological conditions (Marques et al., 2010; Mehnaz et al., 2010; Zhang et al., 2012). Wheat (Triticum aestivum) ranked third most abundant cereal crop after maize and rice and covers approximately 30% of the total cereal products worldwide (Fageria and Baligar, 1997). Pakistan is the 18 largest wheat producing country in the world (Tunio et al., 2006) with an average of 20–24 million tons of wheat grown a year. Wheat is grown on major areas in Pakistan but its average yield per hectare is far less than the actual potential (Akhtar et al., 2009). Understanding the diversity and distribution of indigenous actinobacteria in the rhizosphere of particular crops is depended on the knowledge of native actinobacterial populations, their isolation, identification, and characterization. It is therefore mandatory to explore region specific actinobacterial strains that can be used as growth promoters to achieve desired crop production (Deepa et al., 2010). However, in spite of actinobacterial high soil population, secondary metabolite production and capability to endure hostile environments, Streptomyces, and other actinobacteria are unexpectedly under explored for plant-growth promotion, as compared to Pseudomonas or Bacillus spp. (Doumbou et al., 2002).
The main objectives of the present study included: to isolate indigenous actinobacteria from the wheat (Triticum aestivum) and tomato (Solanum lycopersicum) rhizosphere, characterize these isolates on the basis of morphological, and physiological characteristics as well as by 16S rRNA gene sequence analysis, to screen actinobacteria for various plant growth promoting activities (PGPAs), such as IAA production, phosphate solubilization, siderophore production, and in-vitro 1-aminocyclopropane-1-carboxylate (ACC) deaminase activity. The PGP potential of selected isolates was also studied in-vivo under axenic conditions and their effect on wheat growth was investigated. Keeping in mind, there is a lack of data on the Plant growth promotion (PGP) potential of actinomycetes isolated from Pakistan, Therefore, this study provides new and novel information.
Materials and Methods
Collection and Enrichment of Soil Samples
The soil samples for the isolation of actinobacteria were collected from the rhizosphere of wheat (Triticum aestivum) and tomato (Solanum lycopersicum) plants cultivated in the Punjab province (District: Lahore, Gujranwala, Sheikhupura) Pakistan, during the months of December, 2013–March, 2014. Samples were collected in properly labeled sterile polythene sampling bags. The samples were subjected to physical treatment (heating, 55°C/60 min) according to the method of Seong et al. (2001) and chemical treatment; CaCO3: soil (1:10 w/w; Oskay, 2009), for the enrichment of actinobateria.
Isolation and Preservation of Actinobacteria
One Gram of soil sample was mixed in 9 ml of autoclaved water and serially diluted to a final dilution of 10−3, 10−4, and 10−5. The 0.1 ml of each dilution was spreaded on ISP-4 medium (Soluble starch 10 g, CaCO3 2 g, (NH4)2SO4 2 g, K2HPO4 1.0 g, MgSO4.7H2O 1.0 g, NaCl 1.0 g, FeSO4.7H2O 1.0 mg, MnCl2.7H2O 1.0 mg, ZnSO4.7H2O 1.0 mg, Agar 15 g, distilled water 1 L, pH = 7.5 ± 0.2, 28°C) supplemented with 25 μg/ml nalidixic acid and 50 g/ml nystatin as antibacterial and antifungal agents (Taechowisan et al., 2003). Isolated actinobacteria were sub-cultured on ISP-2 medium (Yeast extract 4 g, Glucose 4 g, Malt extract 10 g, Agar15 g, distilled water 1 L, pH = 7.5) and the plates were incubated at 28°C for 7–12 days. All the isolated strains were preserved in 25% glycerol and kept at −80°C. After incubation, 98 colonies of actinobacteria were selected on the basis of morphological characters such as distinct color and colony shape. Among them, 30 strains were detected to be exhibiting Plant growth promoting activities in initial in-vitro screening. Finally, six isolates that included WA-1, WA-3, WC-3, WD-3, TA-3, and TB-4 which exhibited most significant PGP traits were selected.
Taxonomic Studies
Biochemical and Physiological Characterization
A comprehensive morphological, biochemical, and physiological characterization scheme was adopted to determine the taxonomic status of the selected actinobacterial strains. Among the different biochemical characteristics studied, melanin pigment production was determined by following the methods described by Pridham and Lyons (1969) and positive results were indicated by blackening of the media. Carbohydrate utilization was performed by using ISP-9 medium supplemented with different sugars. Decomposition of oxalic acid and other organic acids was performed by using the method of Nitsch and Kutzner (1969). Esculin degradation was detected by blackening of the media after 12 days of incubation was recorded as positive result. Trysoine agar was used for determining tyrosine hydrolysis by actinomycetes (Gordon and Smith, 1953). Xanthine and hypoxanthine utilization is determined by clear zone formation around the colony after 12 days of incubation (Gordon and Smith, 1953). Starch hydrolysis was performed as described by Cowan (1974). After 12 days of incubation, plates were flooded with lugol's iodine and clear zone around colonies were recorded as positive. Urease releases ammonia from urea and the increase in the pH was detected by the indicator phenol red changing from yellow to pink (Gordon et al., 1974), Cell wall type was determined based on the isomers of diaminopimelic acid (DAP) by using the method of Becker et al. (1964). Cultural characteristics such as color of aerial and substrate mycelium and pigmentation of the selected actinobacteria was recorded on ISP-2 medium according to the method of Shirling and Gottlieb (1966). Growth at different pH 5, 6, 7, 8 was noted after 14 days of incubation on the agar plates. Tolerance to temperature was tested at 10, 28, 37, 45°C and visible growth was recorded as positive result. Agar supplemented with different NaCl Concentration 0, 4, 7, 10, 13% was used for determining NaCl tolerance of actinomycetes.
In-vitro Screening of Actinobacteria for Their Plant Growth Promoting Activities
Colorimetric Analysis of Indole Acetic Acid (IAA) Production
Auxin form different strains of actinomycetes was quantified using the method of Tang and Borner (1979) as described by Ali et al. (2009). All Actinobacteria were grown at 28°C in ISP-2 liquid medium in triplicates for 7–12 days at 120 rpm on an orbital shaker. Media treatments were supplemented with different concentrations of L-tryptophan (0, 100, 200, 300, 400, and 500 μg/ml). Cells were removed from culture medium by centrifugation at 14,000 rpm for 15 min (Sigma 2–5, sigma Laborzentrifugen, Osterode, Germany). The supernatant (1 ml) was mixed with 2 ml of Salkowski's reagent (50 ml, 35% perchloric acid, 1 ml of 0.5 M FeCl3 solution) and was incubated at room temperature for 30 min in dark. Development of pink or red color indicates IAA production. Optical density was taken at 535 nm by using spectrophotometer (S-300D; R and M Marketing, Hounslow, UK). Standard curve of IAA was used to measure the concentration of IAA produced by the actinobacteria.
Siderophore Production
The strains were assayed for the siderophore production on the Chrome Azurol S agar according to the method of Alexander and Zuberer (1991). CAS agar plates were prepared and spot inoculated with actinobacterial strains and incubated at 28°C for 7 days. The colonies producing yellow to orange halos were considered positive for siderophore production.
Solubilization of Phosphate
Pikovskaya's agar plates were used for qualitative screening of all actinobacterial isolates (Gaur, 1990). Solubilization index (SI) was calculated by using the formula of Edi Premono et al. (1996). King (1932) method was used for quantitative analysis of solubilization of tri-calcium phosphate in liquid medium.
Ammonia Production
Freshly grown actinobacterial cultures were inoculated into 1 ml of peptone water and incubated at 28°C for 7–12 days with shaking at 120 rpm. After incubation, 0.5 ml of Nessler's reagent was added in each culture tube. Development of yellow to brown color indicates positive result for ammonia production (Cappuccino and Sherman, 2002).
Hydrogen Cyanide Production
Producton of hydrogen cyanide by actinobacterial culture was evaluated by adapting the method of Lorck (1948). Actionobacteria were streaked on ISP-2 medium amended with 4.4 glycine/l and whatman filter paper No. 1 dipped in 2% sodium carbonate in 0.5% picric acid for a minute was placed underneath the petri plates lids. Plates were sealed with parafilm and incubated at 28°C for 7–12 days. Orange to red color of filter was indicative of HCN production.
Colorimetric Ninhydrin Assay for Screening ACC Utilizing Actinobacteria
Li et al. (2011) method was used. ISP-2 medium was inoculated with actinobacteria, incubated at 28°C with shaking 200 rpm for 7–12 days. After centrifugation of 2 ml of culture at 14,000 rpm for 5 min, supernatant was discarded, pellet washed with 1 ml of liquid DF- medium, later mixed with 2 ml of ACC substrate containing DF mineral medium. 2 ml of DF-ACC medium without inoculation served as control. All the samples were incubated for 4 days at 200 rpm. After incubation, 1 ml of each bacterial culture was centrifuged at 14,000 rpm for 5 min. 100 μl of each supernatant was shifted to another tube and was diluted with 1 ml of liquid DF medium. In 96-well PCR plate, 60 μl of each diluted supernatant was mixed 120 μl of ninhydrin reagent, covered with parafilm, and placed in boiling water bath for 30 min. DF medium was used as a blank.
The resulted purple color depth was record for visual comparison of actinobacterial strains. 100 μl of the reaction mixture was transfer to the microtitre plate in triplicates and absorbance was taken at 570 nm with spectrophotometer. The actinobacterial isolate with visibly reduced color depth and less supernatant absorbance compared to DF-ACC medium without inoculation were considered as ACC utilizing actinobacterial strains.
In-vivo Screening for Plant Growth Promotion Activities
Actinobacterial isolates were grown on ISP-2 broth at 28°C for 7–12 days with continuous shaking 200 rpm. Actinobacterial suspensions were prepared according to the method of Errakhi et al. (2007). Wheat seeds were surface sterilized by using the method of Khalid et al. (2004). Sterilized seeds were soaked for 1 h in the suspension and dried under laminar flow hood overnight. For control, seeds were dipped in distilled water only.
Germination Bioassay
Germination assay was performed by using actinobacterial treated wheat (Triticum aestivum) seeds (three replicates, 5 seeds/plate) were placed in sterilized petri dishes covered with two sheets of filter papers, moistened with 10 ml of sterile distilled water. Water treated control seeds were used. All the petri dishes were incubated in Versatile Environmental Test Chamber (TEMI 850 WISE CUBE) with light intensity of 2000 lux for 16 h daily at 28°C. After 2 weeks, effect of actinobacterial cultures on root length and number of roots was observed.
Pot Experiment
Actinobacterial treated eight wheat seeds (as described above) were sown to a depth of 1 cm in plastic pots (12 cm high × 10 cm diameter) filled with sterilized soil. Three replicates were used for actino-bacterial as well as for control treatments. For control, seeds were dipped in distilled water only. Pots were arranged in fully randomized complete block, under standard conditions, in plant growth room (22–26°C, 16 h light/8 h dark). All pots were watered daily with 10 ml of distilled autoclaved water to achieve moisture level sufficient for seed germination. After 25 days, five plants were removed carefully from the soil, washed with tap water to remove soil particles. Total 15 plants per treatment were considered for statistical analysis. Data was recorded for number of seeds germinated, root and shoot length, root and shoot fresh weight, root, and shoot dry weight (80°C, 24 h electric oven) and number of roots.
Root colonization potential of inoculated Streptomyces was determined at every 8 days by using serial dilution plating technique on GYM agar and number of viable cells was recorded as colony forming units (CFU) as described in Somasegaran and Hoben (1994).
Genomic DNA Isolation, PCR Amplification and Sequencing Of the 16s rRNA Gene
Total genomic DNA was isolated according to the CTAB method described by the Liu et al. (2000). Universal Primers 27F 5′ AGAGTTTGATCMTGGCTCAG 3 and 1492 R′ TACGGYTACCTTGTTACGACTT 3′ were used for the PCR amplification of 16S rRNA gene of the selected strains. Agarose gel electrophoresis (1%) was used for analyzing PCR product and remaining mixture was purified by using PCR Purification kit (FavorPrep™). Purified PCR products were sequenced commercially by GATC Biotech (Germany). The obtained gene sequences were compared with others in the Gen Bank databases using the NCBI Nucleotide BLAST at http://blast.ncbi.nlm.nih.gov/Blast.cgi. Sequences were submitted to NCBI GenBank data base and accession numbers were obtained.
Statistical Analysis
For all experiments, the data were subjected to statistical analysis using software IBM SPSS Statistics version 21. Data were subjected to analysis of variance (ANOVA) and means separated using Duncan's multiple range test (P = 0.05). The correlation coefficients between bacterial auxin production and L-tryptophan concentrations as well as between bacterial growth traits and plant growth parameters were also calculated (P = 0.01 or P = 0.05).
Results
Taxonomic Characteristics of the Selected Rhizopsheric Actinomycetes
Ninty eight actinomycetes were isolated from six different wheat and four different tomato rhizospheric soil samples. All of them were found to be gram positive filamentous rods. On the basis of color of aerial mycelium they were grouped into gray (TA-3, TB-4), yellow (WC-3, WD-3), green (WA-1), and orange (WA-3) color series. Diffusible pigment was produced by the isolate WC-3. All strains contained LL-diaminopimelic acid isomer in their cell wall. Physiologically, most of the actinomycetes isolates were able to utilize different sugars as the carbon source. All strains were able to utilize glucose, xylose, galactose, arabinose, mannose, and mannitol. Strain WA-1 and TA-3 were unable to utilize sucrose, while strain WC-3, and WD-3 were unable to utilize raffinose as their carbon source (Table 1). Most of them were able to grow at temperature 28 and 37°C. None of them were able to grow at temperature 4°C. Optimum temperature and pH was found to be 28°C and eight respectively (Table 2; Tables S1, S2, Figures S1, S2).
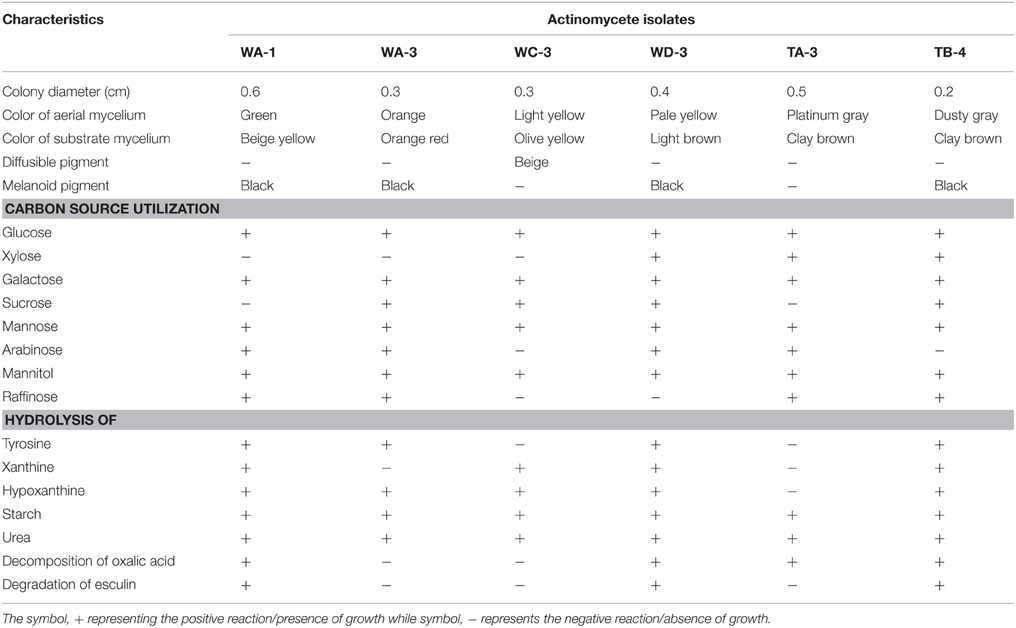
Table 1. Morphological, biochemical, and physiological characteristics of the selected rhizospheric actinomycete strains.
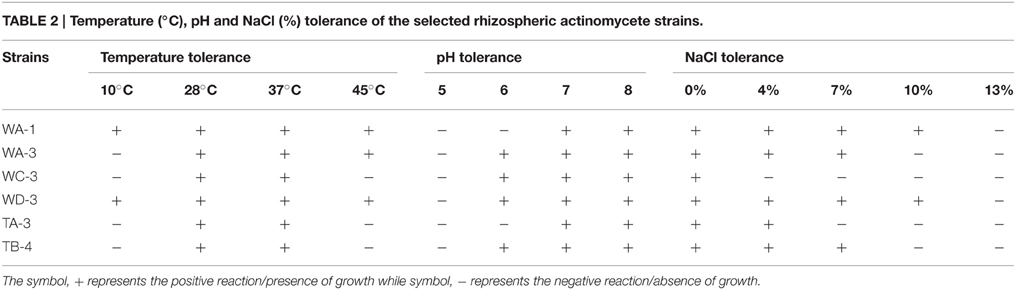
Table 2. Temperature (°C), pH and NaCl (%) tolerance of the selected rhizospheric actinomycete strains.
IAA Production by Selected Actinomycetes
Qualitative analysis of culture supernatant of selected actinomycete isolates revealed production of variable amounts of IAA in the absence and presence of different concentrations of tryptophan (100–500 μg/ml). In the absence of L-tryptophan, IAA production was not observed. With increasing concentration of L-tryptophan, the IAA production was increased. For instance, isolate Streptomyces sp. WA-1(r = 0.979, P = 0.01), S. nobilis WA-3 (r = 0.942, P = 0.01), S. kunmingensis WC-3 (r = 0.995, P = 0.01), S. mutabilis WD-3 (r = 0.932, P = 0.01), S. enissocaesilis TA-3 (r = 0.971, P = 0.01), and S. djakartensis TB-4 (r = 0.919, P = 0.05) showed significant positive correlation with increasing L-tryptophan concentrations. Isolate WA-3 produced highest amount of IAA followed by WC-3, TA-3, WD-3, WA-1, and TB-4. The most active IAA producer S. nobilis WA-3, S. Kunmingenesis WC-3 and S. enissocaesilis TA-3 produce 79.5, 79.23, and 69.26 μg/ml IAA respectively at 500 μg/ml L-tryptophan (Figure 1).
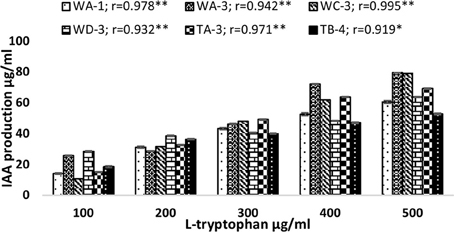
Figure 1. Effect of different L-tryptophan concentrations (100, 200, 300, 400, and 500 μg/ml) on IAA production by actinomycetes. The results shown are representative of three repetitions of the experiment. Value “r” indicates highly significant positive correlation between L-tryptophan and bacterial IAA production. **P = 0.01, *P = 0.05.
Phosphate Solubilization
Among the selected isolates, six Streptomyces were able to solubilize phosphate by producing clear zones around the colonies after 7 days of incubation. Streptomyces sp. WA-1 showed highest phosphate solubilization index of 2.33 followed by S. djakartensis TB-4 (2.27) > S. enissocaesilis TA-3 (2.25) > S. nobilis WA-3 (2.22) > S. mutabilis WD-3 (2.21) > S. kunmingensis WC-3 (2.20). Quantitatively, the highest concentration of soluble phosphate was produced by Streptomyces sp. WA-1 (72.13 mg/100 ml) and S. djakartensis TB-4 (70.36 mg/100 ml; Table 3).
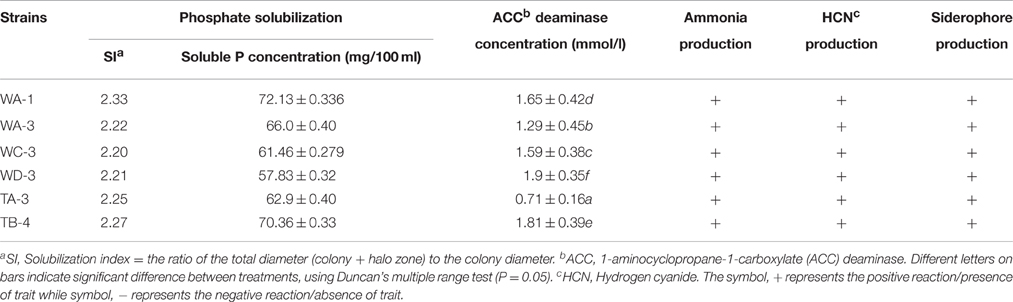
Table 3. Plant growth promoting traits of the selected rhizospheric actinomycetes under in-vitro conditions.
Siderophore, Ammonia, and HCN Production
Among the selected isolates, six rhizospheric actinomycetes were positive for the ammonia production. Siderophore production was detected in all six isolates on CAS agar media, forming clear orange halo zone around the colonies. (Figure 2). All the six isolates were positive for HCN production (Table 3). Streptomyces sp. WA-1 and S. djakartensis TB-4 displayed the highest amount of HCN production as depicted by a very deep red color on the filter paper (Table 3).
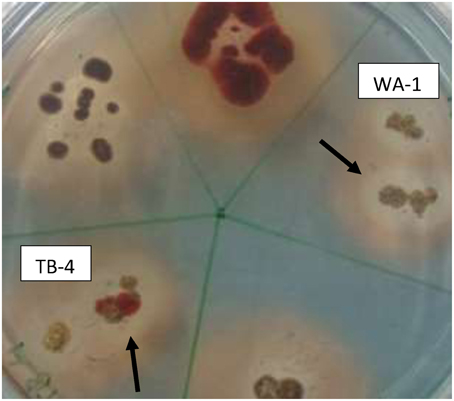
Figure 2. Screening of siderophore producing actinomycete isolates using CAS agar plates after 7 days of growth at 28 ± 2°C. The arrows indicate the halo zone around the colonies of isolate WA-1 (Streptomyces sp.) and TB-4 (Streptomyces djakartensis).
ACC Deaminase Activity by Actinomycetes
All strains showed variable concentration of ACC-deaminase in the culture media. Strain S. mutabilis WD-3 showed highest concentration of ACC-deaminase 1.9 mmol /l). On the other hand, strain S. djakartensis TB-4, Streptomyces sp. WA-1, S. kunmingensis WC-3, S. nobilis WA-3 produce 1.81, 1.65, 1.59, 1.29 mmol/l concentration of ACC-deaminase, respectively. Strain S. djarkartensis TA-3 produce the lowest concentration (0.71) of ACC deaminase (Table 3).
Seed Germination Assay
Inoculation of wheat seeds with selected actinomycetes stimulated root growth in majority of the strains (Figure 3). In case of root length, significant increases were recorded for S. djakartensis TB-4 (61%), S. Kunmingenesis WC-3 (47%) and S. mutabilis (42%). Increases in number of roots were also observed with S. mutabilis WD-3 (55%), Streptomyces sp. WA-1 (50%), and S. djakartensis TB-4 (41%).
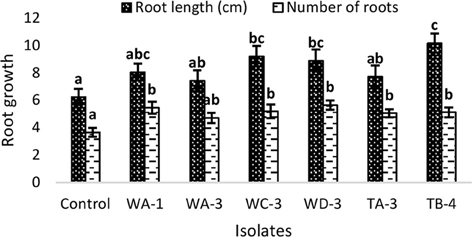
Figure 3. Effect of actinomycetes spore suspension on seed germination of Triticum aestivum. Bars represents mean ± SE of three replicates (15 plants). Different letters on bars indicate significant difference between treatments, using Duncan's multiple range test (P = 0.05).
Root Colonization Potential
Actinobacterial population size was estimated by plate count method on GYM agar at three (8, 16, 24 days) different time intervals. It was observed that all rhizospheric actinobacteria were able to colonize wheat plant roots and displayed persistence in the rhizosphere up to 25 days after inoculation (Figure 4). Maximum colonization was recorded between 8 and 16 days post inoculation. Actinobacterial isolate WA-3 showed maximum number of root colonizing colonies at all times as compared to other actinobacterial isolates.
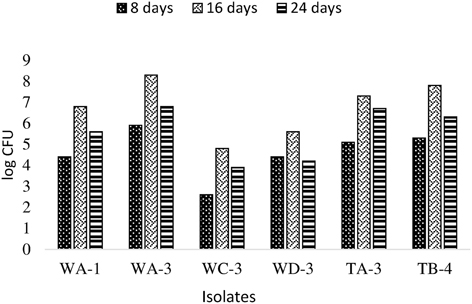
Figure 4. Population density of different actinobacterial isolates inoculated to wheat at different time intervals under axenic conditions.
Plant Growth Promotion Experiment with Selected Actinomycetes
Wheat seeds inoculated with six selected actinomycetes showed variable growth and yield parameters after 3 weeks growth (Table 4). Significant increases in shoot length were observed with S. nobilis WA-3 (65%), S. djakartensis TB-4 (54%), and S. enissocaesilis TA-3 (53%) as compared to water treated control. Significant increase in root length was recorded with S. nobilis WA-3 (81%), S. djakartensis TB-4 (69%), and S. enissocaesilis TA-3 (51%) as compared to water treated control. Maximum increases in plant fresh weight were recorded with S.nobilis WA-3 (84%) and S. djakartensis TB-4 (75%), increased plant dry weight was recorded in case of S. nobilis WA-3(85%), S. djakartensis TB-4 (66%), and S enissocaesilis TA-3 (60%). In case of number of leaves, significant increases were recorded with S. nobilis WA-3 (27%), S. djakartensis TB-4 (27%), and S. enissocaesilis TA-3 (23%). Significant increase in case of number of roots were recorded in case of S. nobilis WA-3 (30%), S. djakartensis TB-4 (26%), and S. enissocaesilis TA-3 (22%) as compared to water treated control (Figure 5).
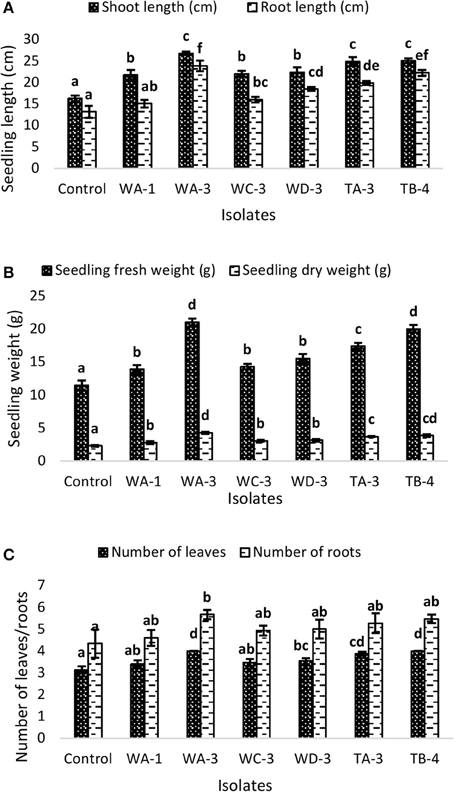
Figure 5. Effect of seed treatment with actinomycetes spore suspensions on plant growth promotion of Triticum aestivum. (A) Seedling root and shoot length (cm) (B), seedling fresh and dry weight (g) (C) number of leaves and roots per plant. Evaluation was made 30 days after planting. Bars represents mean ± SE of three replicates (15 plants). Different letters on bars indicate significant difference between treatments, using Duncan's multiple range test (P = 0.05).
Identification of Selected Actinomycetes Strains by 16s rRNA Gene Sequencing
Six of them including WA-1, WA-3, WC-3, WD-3, TA-3, and TB-4 were selected based on their ability to produce phytohormone IAA, solubilization of inorganic phosphates, ACC deaminase activity, siderophore, ammonia, and hydrogen cyanide production. For all the selected actinomycetes, single band PCR product of 1.5 kb length were achieved with the universal primers (Figure 6). The sequences of 16S rRNA gene were analyzed by comparison with sequences in GenBank through Nucleotide BLAST (http://www.ncbi.nlm.nih.gov/BLAST). After comparison, strains WA-1 showed 98% similarity with Streptomyces sp. while the strains WA-3, WC-3, WD-3, TA-3, and TB-4 showed 99% similarity with Streptomyces nobilis, Streptomyces kunmingenesis, Streptomyces mutabilis, Streptomyces enissocaesilis, Streptomyces djakartensis, respectively. The sequences from strains WA-1, WA-3, WC-3, WD-3, TA-3, and TB-4 have been deposited in the GenBank and accession numbers were obtained (Table 5).
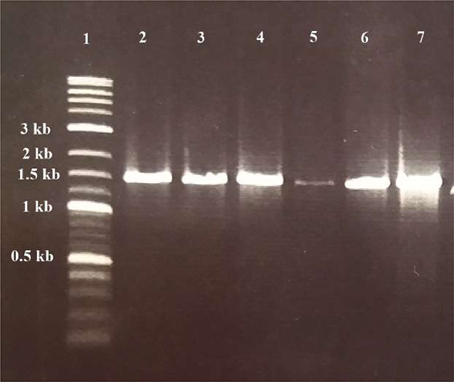
Figure 6. Gel electrophoresis of PCR products for detection of Actinomycetes isolates. Lane 1: DNA ladder (Fermentas, Germany); lane 2, 3, 4, 5, 6, and 7: positive samples of Streptomyces sp. (WA-1), S. nobilis (WA-3), S. kunmingenesis (WC-3), S. mutabilis (WD-3), Streptomyces enissocaesilis (TA-3), and S. djakartensis (TB-4) respectively (1.5 kb).

Table 5. Genetic similarity of the selected strains with different species of the genus Streptomyces determined by 16S rRNA gene sequencing.
Discussion
In this study, a total of 98 actinomycetes strains were isolated from wheat and tomato rhizospheric soil. All of the isolates were screened for different PGP traits and 30 of them were found to be showing excellent PGP activities in initial screening. Out of these, six most promising actinobacterial strains belonging to the genus Streptomyces were investigated by in-vivo studies. The 16S rRNA gene sequence of these six selected actinomycetes strains including WA-1, WA-3, WC-3, WD-3, TA-3, and TB-4 showed maximum sequence similarity with members of the genus Streptomyces.
Majority of the PGPR actinomycetes synthesize IAA which is responsible for increased number of adventitious roots which help plant to uptake a large volume of nutrients and absorb water, while increased root exudates in turn benefits the bacteria (El-Tarabily, 2008). In our study, the IAA production ranges between 10 and 79.5 μg/ml and Streptomyces nobilis strain WA-3 was detected as the best strain for the IAA production (79.5 ug/ml) that exceeds the level of previously reported work by Khamna et al. (2009). Abd-Alla et al. (2013) reported Streptomyces sp. CMU-MH021 that could produce 28.5 μg/ml of IAA. Several Streptomyces species, such as S. olivaceoviridis, S. rimosus, S. Rochei, S. griseoviridis, and S. lydicus have the ability to produce IAA and improve plant growth by increasing seed germination, root elongation and root dry weight (Mahadevan and Crawford, 1997).
Soil acidification often resulted due to the growth of phosphate-solubilizing bacteria (PSB), which in turn resulted in phosphorus solubilization. Therefore, PSB are well known as solubilizers of inorganic phosphate (Verma et al., 2001). The maximum phosphate solubilization activity was detected in the strain Streptomyces sp. WA-1 (72.13 mg/100 ml). Hamdali et al. (2008) reported 83.3, 58.9, and 39 mg/100 ml phosphate solubilization by Streptomyces cavourensis, Streptomyces griseus, and Micromonospora aurantiaca, respectively.
Almost all of the rhizospheric actinomycetes were also able to produce ammonia and hydrogen cyanide. Marques et al. (2010) recommended that bacteria can synthesize ammonia and supply nitrogen to the host plant. Additionally, over production of ammonia serve as a prompting factor for the virulence of opportunistic plant pathogens. Ammonia production also plays an important role HCN production play an essential role in suppression of plant disease. In this study, all the ammonia and HCN producing isolates belong to the genus Streptmyces. Similarly, Husen et al. (2011) reported Streptomyces sp. LSW05 strain as a potent HCN producer.
Siderophore production is one more feature that stimulates plant growth by forming complex with iron form (Fe 3+) in the rhizosphere making iron unavailable to the phytopathogens. It is suggested by Tan et al. (2009) siderophore production that the production of siderophore is an important factor for phytopathogen antagonism and developing growth of the plant. In our study, we detected the 85.7% isolates positive for siderophore production. Similarly, Khamna et al. (2010) has revealed that Streptomyces CMU-SK 126 isolated from Curcuma mangga rhizospheric soil exhibited high amount of siderophore.
ACC deaminase-producing bacteria have been known to promote plant growth by decreasing ethylene inhibition of various plant processes (Husen et al., 2011). They can increase root growth by lowering endogenous ACC levels (Glick, 2005). Plant roots must be able to perceive and recognize such elicitors in ways similar to the recognition of elicitors from plant pathogens. In fact, plant pathogens might interfere with the action of PGPR by being perceived by similar receptors (Husen, 2003).
The effect of soil microbes on PGP including root development has been reported by Uphoff et al. (2009). In the present study, root elongation assay and pot experiment performed by using wheat seeds inoculated with PGP Streptomyces strains, significantly enhanced the plant growth by increasing plant root length, plant shoot length, dry weight, fresh weight, number of leaves, and number of roots over the un-inoculated control. The Streptomyces strains are extensively reported in the literature for its PGP potential (Nassar et al., 2003; El-Tarabily, 2008; Gopalakrishnan et al., 2011b). As hypothesized earlier, the mechanism by which the Streptomyces enhanced the morphological and yield parameters on both sorghum and rice could be their PGP traits including IAA and siderophore production (direct stimulation of PGP; Gopalakrishnan et al., 2011b).
Conclusion
The study revealed that these rhizospheric actinomycetes are potential microbial inoculants because of their intensified PGP activities such as IAA production, phosphate solubilization, siderophore, and HCN production and ACC deaminase production. The strains reported in this study are promising candidates to be developed as commercial biofertilizer formulation and can also be exploited for the production of various agroactive compounds like auxins etc. As such this is the first comprehensive report from Pakistan about the PGP traits and potential agricultural applications of actinomycetes.
Author Contributions
SA: Isolation, biochemical characterization, identification and in-vitro and in-vivo screening of actinomycetes for multiple PGP traits/hormones. Did all the experimental work. IS: Supervise in the sampling of the rhizospheric soils. Teach how to isolate actinomycetes by using enrichment, help in DNA isolation and PCR purification technique. BA: Co-supervise in performing PGP experiments. Both in-vitro and in-vivo screening of actinomycetes.
Conflict of Interest Statement
The authors declare that the research was conducted in the absence of any commercial or financial relationships that could be construed as a potential conflict of interest.
Acknowledgments
The financial support for this study by Higher Education Commission (HEC) of Pakistan, under indigenous Ph.D. fellowships program is gratefully acknowledged.
Supplementary Material
The Supplementary Material for this article can be found online at: http://journal.frontiersin.org/article/10.3389/fmicb.2016.01334
References
Abd-Alla, M. H., El-Sayed, E. S. A., and Rasmey, A. H. M. (2013). Indole-3-acetic acid (IAA) production by Streptomyces atrovirens. J. Biol. Earth Sci. 3, 82–93.
Ahmad, F., Ahmad, A. I., and Khan, M. S. (2008). Screening of free-living rhizospheric bacteria for their multiple plant growth promoting activities. Microbiol. Res. 163, 173–181. doi: 10.1016/j.micres.2006.04.001
Akhtar, M. J., Asghar, H. N., Shahzad, K., and Arshad, M. (2009). Role of plant growth promoting rhizobacteria applied in combination with compost and mineral fertilizers to improve growth and yield of wheat (Triticum Aestivum L.). Pak. J. Bot. 41, 381–390.
Alexander, D. B., and Zuberer, D. A. (1991). Use of Chrome Azurol S reagents to evaluate siderophore production by rhizosphere bacteria Biol. Fertility Soils. 12, 39–45. doi: 10.1007/BF00369386
Alexander, M. (1977). Introduction to Soil Microbiology. 2nd Edn., Malabar, FL: Krieger Publishing Company.
Ali, B., Sabri, A. N., Ljung, K., and Hasnain, S. (2009). Quantification of indole-3-acetic acid from plant associated Bacillus spp. and their phytostimulatory effect on Vigna radiata (L.). World J. Microbiol. Biotechnol. 25, 519–526. doi: 10.1007/s11274-008-9918-9
Barriuso, J., Ramos Solano, B., and Gutiérrez Mañero, F. J. (2008). Protection against pathogen and salt stress by four plant growth-promoting rhizobacteria isolated from Pinus sp. on Arabidopsis thaliana. Biol. Control. 98, 666–672. doi: 10.1094/PHYTO-98-6-0666
Becker, B., Lechevalier, M. P., Gordon, R. E., and Lechevalier, H. A. (1964). Rapid differentiation between Nocardia and Streptomyces by paper chromatography of whole-cell hydrolysates. Appl. Microbiol. 12, 421–423.
Cappuccino, J. G., and Sherman, N. (2002). Microbiology: A Laboratory Manual, 6th Edn. California City, CA: Pearson Education.
Cowan, S. T. (1974). Cowan and Steel's Manual for the Identification of Medical Bacteria, 2nd Edn. London: Cambridge University Press.
Deepa, C. K., Dastager, S. G., and Pandey, A. (2010). Isolation and characterization of plant growth promoting bacteria from non-rhizospheric soil and their effect on cowpea (Vigna unguiculata (L.) Walp.) seedling growth. World J. Microbiol. Biotechnol. 26, 1233–1240. doi: 10.1007/s11274-009-0293-y
Doumbou, C. L., Hamby-Salove, M. K., Crawford, D. L., and Beaulieu, C. (2002). Actinomycetes, promising tools to control plant diseases and to promote plant growth. Phytoprotection 82, 85–102. doi: 10.7202/706219ar
Edi Premono, M., Moawad, A. M., and Vlek, P. L. G. (1996). Effect of phosphate-solubilizing Pseudomonas putida on the growth of maize and its survival in the rhizosphere. Indones. J. Crop Sci. 11, 13–23.
El-Tarabily, K. A. (2008). Promotion of tomato (Lycopersicon esculentun Mill.) plant growth by rhizosphere competent 1-aminocyclopropane-1-carboxylic acid deaminase–producing Streptomycete actinomycetes. Plant Soil. 308, 161–174. doi: 10.1007/s11104-008-9616-2
Errakhi, R., Bouteau, F., Lebrihi, A., and Barakate, M. (2007). Evidences of biological control capacities of Streptomyces spp. against Sclerotium rolfsii responsible for damping-off disease in sugar beet (Beta vulgaris L.). World J. Microbiol. Biotechnol. 23, 1503–1509. doi: 10.1007/s11274-007-9394-7
Fageria, N. K., and Baligar, V. C. (1997). Integrated plant nutrient management for sustainable crop production—An over. Inter. J. Trop. Agri. 15, 7–18.
Franco-Correa, M., Quintana, A., Duque, C., Suarez, C., Rodrıguez, M. X., and Barea, J. M. (2010). Evaluation of actinomycete strains for key traits related with plant growth promotion and mycorrhiza helping activities. Appl. Soil Ecol. 45, 209–217. doi: 10.1016/j.apsoil.2010.04.007
Gaur, A. C. (1990). Phosphate Solubilizing Microorganisms as Biofertilizer. New Delhi: Omega Scientific Publishers.
Glick, B. R. (2005). Modulation of plant ethylene levels by bacterial enzyme ACC deaminase. FEMS Microbiol. Lett. 251, 1–7. doi: 10.1016/j.femsle.2005.07.030
Gopalakrishnan, S., Pande, S., Sharma, M., Humayun, P., Kiran, B. K., Sandeep, D., et al. (2011b). Evaluation of actinomycete isolates obtained from herbal vermicompost for biological control of Fusarium wilt of chickpea. Crop Protect. 30, 1070–1078. doi: 10.1016/j.cropro.2011.03.006
Gordon, R. E., Barnett, D. A., Handerhan, J. E., and Pang, C. H. (1974). Nocardia coeliaca, Nocardia autotrophica, and the nocardin strain. Int. J. Syst. Bacteriol. 24, 54–63. doi: 10.1099/00207713-24-1-54
Gordon, R. E., and Smith, M. M. (1953). Rapidly growing, acid fast bacteria. J. Bacteriol. 66, 41–48.
Hamdali, H., Hafidi, M., Virolle, M. J., and Ouhdouch, Y. (2008). Growth promotion and protection against damping-off of wheat by two rock phosphate solubilizing actinomycetes in a P-deficient soil under greenhouse conditions. Appl. Soil Ecol. 40, 510–517. doi: 10.1016/j.apsoil.2008.08.001
Husen, E. (2003). Screening of soil bacteria for plant growth promotion activities in vitro. Indo. J. Agric. Sci. 4, 27–31.
Husen, E., Wahyudi, A. T., Suwanto, A., and Giyanto. (2011). Growth enhancement and disease reduction of soybean by 1-aminocyclopropane-1-carboxylate deaminase-producing Pseudomonas. Am. J. Appl. Sci. 8, 1073–1080. doi: 10.3844/ajassp.2011.1073.1080
Jeon, J. S., Lee, S. S., Kim, H. Y., Ahn, T. S., and Song, H. G. (2003). Plant growth promotion in soil by some inoculated microorganisms. J. Microbiol. 41, 271–276.
Khalid, A., Arshad, M., and Zahir, Z. A. (2004). Screening plant growth-promoting rhizobacteria for improving growth and yield of wheat. J. Appl. Microbiol. 96, 473–480. doi: 10.1046/j.1365-2672.2003.02161.x
Khamna, S., Yokota, A., and Lumyong, S. (2009). Actinomycetes isolated from medicinal plant rhizosphere soils: diversity and screening of antifungal compounds, indole-3-acetic acid and siderophore production. World J. Microbiol. Biotechnol. 25, 649–655. doi: 10.1007/s11274-008-9933-x
Khamna, S., Yokota, A., Peberdy, J. F., and Lumyong, S. (2010). Indole-3-acetic acid production by Streptomyces sp. isolated from some Thai medicinal plant rhizosphere soils. Eurasia J. Biosci. 4, 23–32. doi: 10.5053/ejobios.2010.4.0.4
Khan, A. G. (2005). Role of soil microbes in the rhizospheres of plants growing on trace metal contaminated soils in phytoremediation. J. Trace Elem. Med. Biol. 18, 355–364. doi: 10.1016/j.jtemb.2005.02.006
Kumar, A., Maurya, B. R., and Raghuwanshi, R. (2014). Isolation and characterization of PGPR and their effect on growth, yield and nutrient content in wheat (Triticum aestivum L.). Biocatal. Agric. Biotechnol. 3, 121–128. doi: 10.3389/fmicb.2015.00198
Li, J., Zhao, G. Z., Huang, H. Y., Zhu, W. Y., Lee, J. C., Xu, L. H., et al. (2011). Nonomuraea endophytica sp. nov., an endophytic actinomycete isolated from Artemisia annua L. Int. J. Syst. Evol. Microbiol. 61, 757–761. doi: 10.1099/ijs.0.022558-0
Liu, D., Coloe, S., Baird, R., and Pedersen, J. (2000). Rapid mini-preparation of fungal DNA for PCR. J. Clin. Microbiol. 38:471.
Lorck, H. (1948). Production of hydrocyanic acid by bacteria. Physiol. Plant. 1, 142–146. doi: 10.1111/j.1399-3054.1948.tb07118.x
Lucy, M., Reed, E., and Glick, B. R. (2004). Application of free living plant-promoting rhizobacteria. Antonie van Leeuwenhoek. 86, 1–25. doi: 10.1023/B:ANTO.0000024903.10757.6e
Mahadevan, B., and Crawford, D. L. (1997). Properties of the chitinase of the antifungal biocontrol agent Streptomyces lydicusWYEC108. Enzyme Microb. Technol. 20, 489–493.
Majeed, A., Abbasi, M. K., Hameed, S., Imran, A., and Rahim, N. (2015). Isolation and characterization of plant growth-promoting rhizobacteria from wheat rhizosphere and their effect on plant growth promotion. Front. Microbiol. 6:198. doi: 10.3389/fmicb.2015.00198
Marques, A. P. G. C., Pires, C., Moreira, H., Rangel, A. O. S. S., and Castro, P. M. L. (2010). Assessment of the plant growth promotion abilities of six bacterial isolates using Zea mays as indicator plant. Soil Biol. Biochem. 42, 1229–1235. doi: 10.1016/j.soilbio.2010.04.014
Mehnaz, S., Baig, D. N., and Lazarovits, G. (2010). Genetic and phenotypic diversity of plant growth promoting rhizobacteria isolated from sugarcane plants growing in Pakistan. J. Microbiol. Biotechnol. 20, 1614–1623.
Minuto, A., Spadaro, D., Garibaldi, A., and Gullino, M. L. (2006). Control of soilborne pathogens of tomato using a commercial formulation of Streptomyces griseoviridis and solarization. Crop. Prot. 25, 468–475. doi: 10.1016/j.cropro.2005.08.001
Nassar, A. H., El-Tarabily, K. A., and Sivasithamparam, K. (2003). Growth promotion of bean (Phaseolus vulgaris L.) by a polyamine producing isolate of Streptomyces griseoluteus. Plant Growth Reg. 40, 97–106. doi: 10.1023/A:1024233303526
Nitsch, B., and Kutzner, H. J. (1969). Egg-yolk agar as a diagnostic medium for Streptomyces. Cell Mol. Life Sci. 25, 220–221. doi: 10.1007/BF01899136
Oskay, M. (2009). Comparison of Streptomyces diversity between agricultural and non- agricultural soils by using various culture media. Sci. Res. Essay. 10, 997–1005.
Pridham, T. G., and Lyons, A. J. (1969). Progress in clarification of the taxonomic and nomenclatural status of some problem actinomycetes. Dev. Ind. Microbiol. 10, 183–221.
Seipke, R. F., Barke, J., and Brearley, C. (2011). A single Streptomyces symbiont makes multiple antifungals to support the fungus farming ant Acromyrmex octospinosus, PLoS ONE 6:e22028. doi: 10.1371/journal.pone.0022028
Seong, C. H., Choi, J. H., and Baik, K. S. (2001). An improved selective isolation of rare actinomycetes from forest soil. J. Microbiol. 17, 23–39.
Shirling, E. B., and Gottlieb, D. (1966). Methods for characterization of Streptomyces species. Int. J. Syst. Bacteriol. 16, 313–340. doi: 10.1099/00207713-16-3-313
Somasegaran, P., and Hoben, H. J. (1994). Handbook for Rhizobia. Methods in Legume–Rhizobium Technology. Heidelberg, NY: Springer.
Taechowisan, T., Peberdy, J. F., and Lumyong, S. (2003). Chitinase production by endophytic Streptomyces aureofaciens CMUAc 130 and its antagonism against phytopathogenic fungi. Ann. Microbiol. 53, 447–461.
Tan, H., Deng, Z., and Cao, L. (2009). Isolation and characterization of actinomycetes from healthy goat faeces, Lett. Appl. Microbiol. 49, 248–253. doi: 10.1111/j.1472-765X.2009.02649.x
Tang, W. Y., and Borner, J. (1979). “Enzymes involved in synthesis and breakdown of indoleacetic acid,” in Modern Methods of Plant Analysis, Vol. 7, eds K. Paech and M. V. Tracey, (Gohingen; Heidelberg: Springer Verlag), 238–241.
Tunio, S. D., Korejo, M. N., Jarwar, A. D., and Waggan, M. R. (2006). Studies on indegenious and exotic weed competition in wheat. Pak. J. Agri. Biol. 5, 1–8.
Uphoff, N., Anas, I., Rupela, O. P., Thakur, A. K., and Thyagarajan, T. M. (2009). Learning about positive plant-microbial interactions from the system of rice intensification (SRI). Aspect. Appl. Biol. 98, 29–54.
Verma, K., Kukreja, D. V., Pathak, S., Suneja, N., and Narula. (2001). In vitro production of plant growth regulators (PGRs) by Azorobacter chroococcum. Indian J. Microbiol. 41, 305–307.
Vessey, J. K. (2003). Plant growth promoting rhizobacteria as biofertilizers. Plant Soil. 255, 571–586. doi: 10.1023/A:1026037216893
Wang, X. J., Zhang, J., Wang, J. D., Qian, P. T., Liu, C. X., and Xiang, W. S. (2013). Novel Novel cyclopentenone derivatives produced by a rare actinobacterial strain Actinoalloteichus nanshanensis sp. nov. NEAU 119. Nat. Prod. Res. 27, 1863–1869. doi: 10.1080/14786419.2013.771349
Keywords: plant growth promoting Streptomyces, indole acetic acid (IAA), wheat, 16S rRNA gene sequencing, biofertilizers, agro-active compounds
Citation: Anwar S, Ali B and Sajid I (2016) Screening of Rhizospheric Actinomycetes for Various In-vitro and In-vivo Plant Growth Promoting (PGP) Traits and for Agroactive Compounds. Front. Microbiol. 7:1334. doi: 10.3389/fmicb.2016.01334
Received: 14 April 2016; Accepted: 12 August 2016;
Published: 29 August 2016.
Edited by:
Anton Hartmann, Helmholtz Zentrum München, GermanyCopyright © 2016 Anwar, Ali and Sajid. This is an open-access article distributed under the terms of the Creative Commons Attribution License (CC BY). The use, distribution or reproduction in other forums is permitted, provided the original author(s) or licensor are credited and that the original publication in this journal is cited, in accordance with accepted academic practice. No use, distribution or reproduction is permitted which does not comply with these terms.
*Correspondence: Imran Sajid, imran.mmg@pu.edu.pk