- 1Department of Pathogenic Biology, School of Medical Science, Jiangsu University, Zhenjiang, China
- 2National Institute for Communicable Disease Control and Prevention, Chinese Center for Disease Control and Prevention, State Key Laboratory of Infectious Disease Prevention and Control, Collaborative Innovation Center for Diagnosis and Treatment of Infectious Diseases, Beijing, China
In many gram negative bacilli, AmpD plays a key role in both cell well-recycling pathway and β-lactamase regulation, inactivation of the ampD causes the accumulation of 1,6-anhydromuropeptides, and results in the ampC overproduction. In Yersinia enterocolitica, the regulation of ampC expression may also rely on the ampR-ampC system, the role of AmpD in this species is still unknown. In this study, three AmpD homologs (AmpD1, AmpD2, and AmpD3) have been identified in complete sequence of strain Y. enterocolitica subsp. palearctica 105.5R(r). To understand the role of three AmpD homologs, several mutant strains were constructed and analyzed where a rare ampC regulation mechanism was observed: low-effective ampD2 and ampD3 cooperate with the high-effective ampD1 in the three levels regulation of ampC expression. Enterobacteriaceae was used to be supposed to regulate ampC expression by two steps, three steps regulation was only observed in Pseudomonas aeruginosa. In this study, we first reported that Enterobacteriaceae Y. enterocolitica can also possess a three steps stepwise regulation mechanism, regulating the ampC expression precisely.
Introduction
Yersinia enterocolitica is a human enteric pathogen with worldwide distribution. It is a highly heterogeneous species with six biovars (1A, 1B, 2, 3, 4, and 5) that has more than fifty serotypes with different geographical distribution, ecological niches, and pathogenic properties (Wang et al., 2009; Liang et al., 2012). Most Y. enterocolitica strains produce two kinds of chromosomal β-lactamases, BlaA (a non-inducible broad-spectrum carbenicillinase) and BlaB (an AmpC-type inducible group I class C cephalosporinase; Cornelis and Abraham, 1975). A recent study shows in 1B/O:8 strain 8081, the expression of BlaA is constitutive whereas the AmpC (BlaB) was inducible (Bent and Young, 2010). Meanwhile, the two β-lactamases are not expressed simultaneously in all strains because strains with different biotypes tend to display specific resistance phenotypes (Stock et al., 2000). The mechanism of variable expression and activities of these two β-lactamases is unknown (De La Prieta et al., 1995, 2006; Stock et al., 1999; Pham et al., 2000; Sharma et al., 2006).
Chromosomal cephalosporinase AmpC is ubiquitous in Gram-negative organisms (Jacoby, 2009). Most strains express the AmpC β-lactamase constitutively at a low basal level and expression is high in induced conditions. The regulation of ampC expression is controlled by several regulatory genes, e.g., ampR, ampG, and ampD, which belong to the ampR-ampC system as part of the cell-wall recycling pathway (Park and Uehara, 2008). Bacterial peptidoglycan is remodeled in a dynamic balance between synthesis and degradation. During growth, the peptidoglycan in the periplasm is hydrolyzed by murein hydrolases to generate peptidoglycan (PG) degradation products (GlcNAc-1,6-anhydro-MurNAc; Johnson et al., 2013). The products are transported to the cytosol via an inner membrane permease, AmpG (Cheng and Park, 2002; Park and Uehara, 2008; Johnson et al., 2013), playing roles for cell wall precursors (Park and Uehara, 2008) and signaling molecules for the induction of β-lactamase (Jacobs et al., 1994; Hanson and Sanders, 1999). AmpD is a cytoplasmic N-acetyl-anhydromuramyl-L-alanine amidase, which has been well-studied in Enterocobacter cloacae, Citrobacter freundii, and Pseudomonas aeruginosa. GlcNAc-1,6-anhydromuropeptide and 1,6-anhydromuropeptide are hydrolyzed by AmpD to generate 1,6-anhydromuramic acid and peptide where the peptide is reused by enzymes in the cell wall recycling pathway to generate UDP-MurNAc-pentapeptide that binds to the transcriptional regulator AmpR allosterically to repress ampC expression (Lindberg et al., 1987; Holtje et al., 1994; Jacobs et al., 1997).
Inactivation of AmpD is the major cause of constitutive hyper-production of AmpC giving high β-lactam resistant phenotypes (Peter et al., 1988; Jacobs et al., 1995). In a recent study, Juan et al. demonstrated a stepwise upregulation mechanism with three AmpD homologs termed AmpD, AmpDh2, and AmpDh3; a highly sophisticated regulation mechanism for ampC expression in P. aeruginosa PAO1, the first characterized example in a multiple-step sequential regulation of β-lactamase expression in Gram-negative bacteria (Juan et al., 2006). Further, after the discovery of AmpD-like lipoprotein, AmiD, in Escherichia coli (Kerff et al., 2010), Enterobacteriaceae were thought to have ampD homologs (Juan et al., 2006; Yang et al., 2009), regulating ampC expression in two steps, however, there is no structural data are available. Y. enterocolitica was supposed to regulate ampC expression by ampC-ampR system because the presents of ampC-ampR region in chromosome (Seoane et al., 1992), but the feature of ampC regulation in Y. enterocolitica is still a mistiness. In the present study, we found Y. enterocolitica strain subsp. palearctica 105.5R(r) had three putative ampD homologs. This suggested Y. enterocolitica possessed a complex regulation mechanism for β-lactamase expression.
Materials and Methods
Bacterial Strains and Growth Conditions
The bacterial strains used in this study were listed in Table 1. The wild-type Y. enterocolitica strain subsp. palearctica 105.5R(r) (bioserotype 3/O:9) chromosome was completely sequenced as described in our previous work (Wang et al., 2011). Strains were routinely grown in Luria Bertani (LB) broth or on LB plates at 28°C (Y. enterocolitica) and 37°C (E. coli). When appropriate, antibiotics were added to the media as required to a final concentration of 34 μg/ml for chloramphenicol (Cm) and 5 μg/ml for tetracycline (Tc). Yersinia selective supplement was added to the solid LB medium as suggested by the manufacturer (Oxoid, UK).
Construction of ampD1, ampD2, and ampD3 Mutant Strains
After a homology search, we identified three potential Y. enterocolitica ampD homologs termed ampD1, ampD2, and ampD3. To further study them, three ampD single (YEΔD1, YEΔD2, YEΔD3), three double (YEΔD1D2, YEΔD1D3, YEΔD2D3), and one triple (YEΔD1D2D3) mutants were constructed using the following steps. Briefly, to three single ampD mutant strains, two PCR amplicons (Table 2) upstream and downstream of ampD1, ampD2, and ampD3 were cloned into the suicide plasmid, pDS132 (Philippe et al., 2004), using restriction with SphI and SacI with the in-fusion cloning technique to obtain plasmids pDSD1, pDSD2, pDSD3. Recombinant plasmids were then transformed into E. coli DH5α and transformed into the helper strain, S17 λpir (Simon et al., 1983); and selected on 34 μg/ml chloramphenicol LB agar plates. Using conjugation, recombinant plasmids were introduced into Y. enterocolitica 105.5R(r); and transconjugants were selected using LB plates with 34 μg/ml chloramphenicol in Yersinia selective agar medium (Oxoid) and then incubated on LB plates without antibiotic overnight. For mutant selection, bacterial cultures were transferred onto LB plates containing 10% sucrose without NaCl. The mutants were confirmed using antibiotic resistance, PCR amplification, and sequencing. The double mutants and triple mutant strains were then constructed from the single mutants sequentially using the same procedures.
Antibiotic Susceptibility Testing
The minimum inhibitory concentrations (MICs) of 15 antibiotics were determined in triplicate using the standard 2-fold serial broth microdilution method according to the guidelines of the Clinical Laboratory Standards Institute (CLSI, 2015) for ampicillin, ticarcillin, cefazolin, piperacillin, ceftazidime, cefriaxone, cefepime, piperacillin/tazobactam, ampicillin/sulbactam, cefoxitin, cefotetan, aztreonam, imipenem, meropenem, and ciprofloxacin. Escherichia coli ATCC 25922 was used as a control strain in each assay.
Measurement the Expression of blaA and ampC using luxCDABE Reporter System
The expression levels of blaA and ampC were determined using the luxCDABE reporter system. Primers pPampC-F, pPampC-R were used to amplify the ampC promoter region fragment, respectively; and was cloned into the plasmid pBBRLux (Hammer and Bassler, 2007) which carried promoter-less luxCDABE genes to generate the fusion plasmid pLUXampC. The resulting plasmid was then introduced into E. coli S17 λpir and finally transferred into the wild-type strain 105.5R(r) and the seven derivate ampD mutants with or without cefoxitin. The inducibility of BlaA and AmpC β-lactamase was confirmed. Overnight cultures were diluted 1:100 into 15 ml LB broth and incubated with shaking at 28°C until mid-log phase was attained, then the cultures were incubated with the presence of 1/4- to 10-fold of MIC-values of imipenem and cefoxitin for an additional 1 h before harvesting. Luminescence was measured using an Infinite M200 Pro spectrophotometer and calculated with relative light units. Mean luminescence/OD600-values were obtained in three independent experiments (Zhou et al., 2013).
Quantification of β-Lactamase Activity
β-Lactamase specific activity was determined spectrophotometrically in crude sonic extracts from strain 105.5R(r) and the seven above-described ampD mutants. To determine the β-lactamase specific activity after induction, the cultures were incubated in the presence of 40 μg/ml cefoxitin for 1 h before harvesting. The samples were centrifuged for 5 min at 3000 × g and the pellets were washed once in 5 ml 0.01 M phosphate buffered saline, pH 7.0 and re-suspended in 1 ml of the same buffer. The suspensions were sonicated on ice for 3 min, and then centrifuged at 10,000 × g for 30 min at 4°C and the supernatant was retained. After determining the total protein using Bio-Rad protein assay reagents, 10 min reactions were allowed before nitrocefin hydrolysis was measured. The specific activity (U/mg) was calculated as nanomoles of nitrocefin hydrolyzed per minute per milligram of protein, using an extinction coefficient (Δε) of 20,500 M−1 cm−1 for nitrocefin at 486 nm, as suggested by the manufacturer (Oxoid, UK; Kong et al., 2005). The mean β-lactamase activity obtained in three independent experiments was analyzed.
Complementation Assay
In the complementation assay, the ORFs of ampD1, ampD2, and ampD3 were amplified and cloned into the broad-host-range expression vector, pSRKTc, to generate pSRKTcD1, pSRKTcD2, and pSRKTcD3, respectively. The three recombinant plasmids and plasmid pSRKTc (as a control) were transferred into the completely de-repressed strain YEΔD1D2D3. Transformants were selected on 5 μg/ml tetracycline Yersinia selective plates. Finally, β-lactamase activity was determined to evaluate the function of each AmpD homolog.
Results
Yersinia enterocolitica subsp. Palearctica 105.5R(r) Has Three AmpD Homologs
As shown in Figure 1, the predicted amino acid sequences of AmpD were screened using ClustalW software multiple sequence alignment of AmpD1 (accession no. WP_005156822), Y. enteoroclitica AmpD2 (accession no. WP_005164953), AmpD3 (accession no. WP_013649890), and other closely related bacterial strains e.g., the AmpD from the E. coli K-12 (accession no. AAC73221), AmpD of C. freundii OS60 (accession no. Z14002), AmpD of E. cloacae ATCC13047 (accession no. CAA78391) and the AmpD (accession no. NP_253211), AmpDh2 (accession no. NP_254172), and AmpDh3 (accession no. NP_249498) of P. aeruginosa PAO1. The predicted amino acid sequence for the AmpD1 protein exhibited 76.4, 76.1, 76.7, and 70% identity to the AmpD of E. coli K-12, C. freundii OS60, E. cloacae ATCC13047, and P. aeruginosa, respectively. Further, AmpD2 (AmpD3) exhibited 44.9% (38%) and 44.8% (65.2%) identity to P. aeruginosa AmpDh2 and AmpDh3, respectively. All three AmpD homologs in Y. enterocolitica have four common residues at positions 34(H), 69(H), 154(H), and 165(P) shown by Jacobs et al. (1995); and six essential residues 34(H), 116(E), and 154(H), 162(K), 164(D) for C. freundii AmpD activity reported by Genereux et al. were also found (Genereux et al., 2004). The Amino acid phylogenetic trees of AmpD formed two branches: branch A contained Y. enterocolitica AmpD1 and all other “traditional” AmpD from different strains; branch B contained AmpD2, AmpD3 from Y. enterocolitica and AmpDh2 and AmpDh3 from P. aeruginosa PAO1 (Figure 2). Distribution of the three AmpD homologs in Y. enterocolitica was different. Using the DNA BLAST program provided by NCBI, we found the ampD1 and ampD2 were widely distributed in the Y. enterocolitica strains including 8081 (O:8), WA (O:8), Y11 (O:9), W22703 (O:9), Y53/03(O:5), and even in the atypical strain LC20. However, only three Y. enterocolitica strains (2015-87, W22703, Y11) and 105.5R(r) have ampD3 genes among the available uploaded DNA sequences. According to the SignalP4.1 Server prediction (Petersen et al., 2011), the AmpD1 and AmpD3 protein is likely a cytoplasmic protein, AmpD2 is likely a secretory protein with signal peptide sequence.
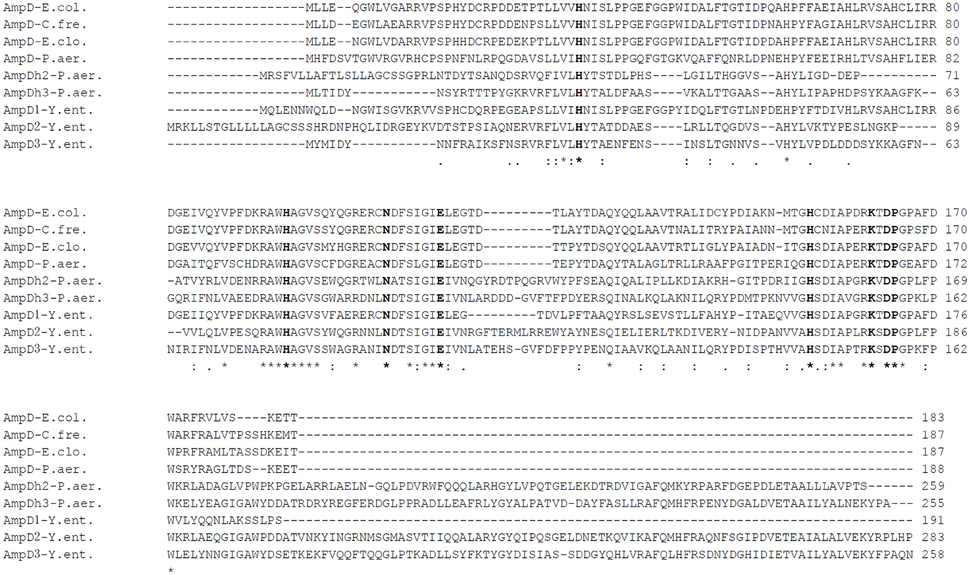
Figure 1. Amino acid sequence alignment of AmpD homologs of different Gram-negative bacteria using ClustalW. AmpD-E.col (E. coli K-12 accession no. AAC73221), AmpD-C.fre (C. freundii OS60 accession no. Z14002), AmpD-E.clo (E. cloacae accession no. CAA78391), AmpD-P.aer (P. aeruginosa PAO1 accession no. NP_253211), AmpDh2-P.aer (P. aeruginosa PAO1 accession no. NP_254172), AmpDh3-P.aer (P. aeruginosa PAO1 accession no. NP_249498), AmpD1-Y.ent (Y. enterocolitica accession no. WP_005156822), AmpD2-Y.ent (Y. enterocolitica 105.5R accession no. WP_005164953) and AmpD3-Y.ent (Y. enterocolitica 105.5R accession no. WP_013649890) are shown. Asterisk, colons, and periods represent identical, conserved, and semi-conserved residues, respectively. The conserved and essential amino acids for AmpD activity are indicated in bold.
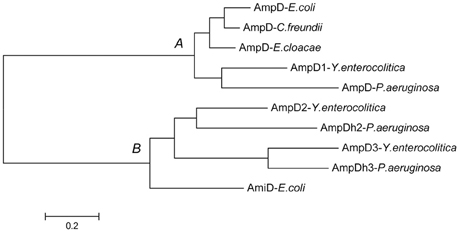
Figure 2. Amino acid sequence phylogenetic analysis of AmpD homologs from different Gram-negative bacteria. Y. enterocolitica AmpD1 belong to the branch A with the other “traditional” AmpD, Y. enterocolitica AmpD2 and AmpD3 belong to the second branch with P. aeruginosa AmpDh2 and AmpDh3 discovered recently.
AmpD1, AmpD2, and AmpD3 Are Negative Regulators of ampC Expression at Different Levels
As shown in Figure 3A, we confirmed the best inducer of the induction assay was cefoxitin (40 mg/L). Figure 3B showed inactivation of the ampD homologs caused three different levels of enhancements in ampC expression. At level one, mutant strains YEΔD2 (ampD2 inactivation), YEΔD3 (ampD3 inactivation), and the double mutant strain YEΔD2D3 (ampD2-ampD3 double inactivation) only slightly increased ampC promoter activity. At level two, luminescence values showed an obvious elevation when the ampD1 inactivated strain, YEΔD1, was examined; and hence, the ampC promoter activity of double mutant strain YEΔD1D2 was further enhanced. Finally, at level three, in ampD1–ampD3 relative double/triple mutant strains, YEΔD1D3 and YEΔD1D2D3, ampC promoter activity was dramatically increased in both basal and induced conditions. But the activity was elevated only at modest level under the inducible conditions. At the same time, not surprisingly, neither any mutation nor inducer change the BlaA expression (data not shown).
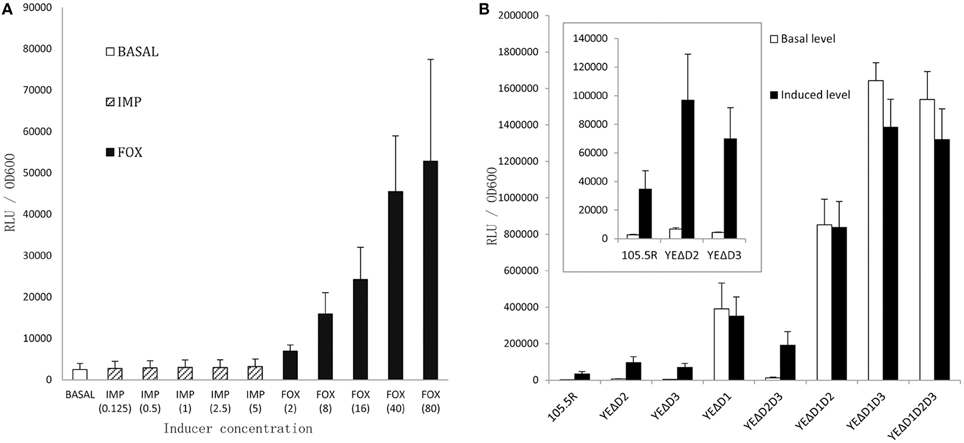
Figure 3. Induction assay by monitor the ampC promoter activity of Y. enterocolitica 105.5R(r) to choose an appropriate inducer (A), each group was added in IMP or FOX by 0.125-, 1-, 2-, 5-, 10-fold MIC-value, respectively. Then the activity of ampC promoter of 105.5R(r) and its seven derived mutants were tested (B), the strains were grown in the 40 mg/L Cefoxitin for 1 h. Error bars indicate standard deviations for triplicate experiments.
Role of AmpD1, AmpD2, and AmpD3 in β-Lactamase Activity and β-Lactam Resistance in Y. enterocolitica
To further understand the role of AmpD homologs in Y. enterocolitica, we tested the β-lactamase activity and the minimal inhibitory concentrations (MICs) of 15 antibiotics in the eight test strains mentioned above. As shown in Figure 4A, the value of β-lactamase activity in wild-type strain with or wothout inducer was consist with the biotype 3 strain reported by Stock et al. (2000). On the ampD mutant aspect, the levels of β-lactamase activity was consistent with luminescence values, three β-lactamase activity levels occur. At level one, β-lactamase activity of mutant strains YEΔD2 (p < 0.05), YEΔD2D3 (p < 0.05), and YEΔD3 (no statistically significant) was only slightly increased compared with the wild-type strain 105.5R(r) (~1.2- to 3-fold), whereas a modest level increase appeared in strain YEΔD1 (p < 0.05) and YEΔD1D2 (p < 0.05; ~10- to 20-fold) compared to at level two. Finally, at level three, complete derepression was reached in YEΔD1D3 (p < 0.05) and YEΔD1D2D3 (p < 0.05) where the β-lactamase activity of these two strains were significantly increased (~38-fold) compared to YE105.5R(r) and could not be further induced by cefoxitin (no statistically significant).
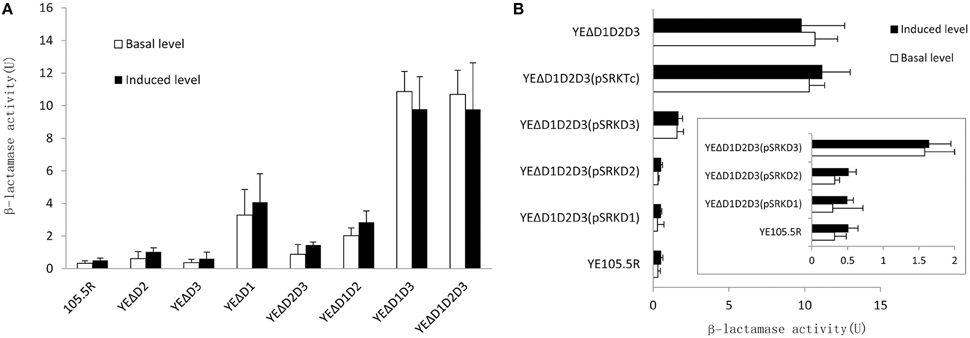
Figure 4. Three AmpD homologs cooperate to regulate the ampC expression in Y. enterocolitica in eight test strains. (A) Inactivation of AmpD homologs causes the elevation on β-lactamase activity in variant degree. (B) In complementation assay, hyperproduction of single AmpD1 (pSRKTcD1) and AmpD2 (pSRKTcD2) in YEΔD1D2D3 will reduce the β-lactamase activity into wild-type strain levels, however, single AmpD3 (pSRKTcD3) do not possess full capacity as AmpD1 and AmpD3. This data are the average of the measurements made in triplicate, the error bars indicate standard deviations.
For β-lactam resistance, as shown in Figure 4A, three levels of AmpC hyper-production elevate the resistance capacity to most penicillins, first-generation cephalosporins, third-generation cephalosporins, monobactam, and cephamycins at the three different levels. At the same time, MICs of imipenem, meropenem, and ciprofloxacin were not changed. It should be noted that no CLSI breakpoint was surpassed in any of the antibiotics tested except for Ampicillin, Ticarcillin, and Cephazolin which were native for resistance in Y. enterocolitica.
The complementation assays were performed introducing pSRKTcD1, pSRKTcD2, and pSRKTcD3 into the triple-mutant strain YEΔD1D2D3. After quantifying the β-lactamase activity of each complementary strain, as shown in Figure 4B, YEΔD1D2D3 (pSRKTcD1) and YEΔD1D2D3 (pSRKTcD2) completely restored the β-lactamase activity to the level of the wild-type strain YE105.5R(r); while in YEΔD1D2D3 (pSRKTcD3), the β-lactamase activity significantly decreased, but not reaching the level of wild-type strain (p < 0.05).
Disscussion
The biological function of AmpD was first characterized in E. cloacae and C. freundii (Lindberg et al., 1987; Peter et al., 1988) where it played an essential role in suppressing the ampC expression through the ampR-ampC system indirectly and later studies on AmpD in other Gram-negative organisms taken Enterbacteraceae as the paradigm (Juan et al., 2006; Yang et al., 2009). However, each Enterobacteriaceae members usually have peculiar features in AmpC regulation (Jacoby, 2009). In E. coli, AmpC production was not inducible because having no AmpR, and it was regulated by a promoter and attenuator mechanism (Jaurin et al., 1981). In addition, expression of ampC in Acinetobacter baumannii was also not inducible lacking AmpR (Bou and Martinez-Beltran, 2000). As a member of Enterobacteriaceae, Y. enterocolitica is not well-understood in the features of ampC regulation. To date, a three-step ampC regulation mechanism driven by three ampD homologs was only observed in P. aeruginosa (Juan et al., 2006), while two AmpD homologs were also identified in Stenotrophomonas maltophilia but only AmpDI was effective in β-lactamase regulation (Yang et al., 2009; Talfan et al., 2013).
In this study, we first reported the role of AmpD in Y. enterocolitica and there has three AmpD homologs with different features termed in AmpD1, AmpD2, and AmpD3 that coordinate to repress the expression of ampC β-lactamase. As shown in Figure 3, inactivation of ampD1 (YEΔD1) results in an obvious increased in basal and inducible levels compared to the wild type (WT) strain, and a higher basal and inducible level was achieved in ampD1–ampD2 double mutant strain (YEΔD1D2). However, although individual AmpD3 was ineffective (elevate the value but no statistical significance), the ampD1–ampD3 double deletion strain YEΔD1D3 caused a significant increase from the basal expression level of ampC and cannot be further induced by imipemem. The full derepression phenotype was achieved in YEΔD1D3, suggesting a synergetic effect between AmpD1 and AmpD3. Finally, at the last level, triple mutants strain YEΔD1D2D3 was as same as YEΔD1D3, achieving the full derepression phenotype.
This three-step regulation mechanism has similarity with the stepwise regulation mechanism in P. aeruginosa with a few differences (Juan et al., 2006). In P. aeruginosa, over-expression of each of the three AmpD homologs can return the high-level expression back to wild-type strain levels, suggesting the three ampD homologs involved are repressing the ampC expression quantitatively rather than qualitatively. However, in Y. enterocolitica, after introducing recombinant expression vector pSRKTcD1 and pSRKTcD2 (high expression AmpD1 and AmpD2, respectively) to YEΔD1D2D3, the high β-lactamase activity was reduced to the low wild-type strain level. Compared with AmpD1 and AmpD2, solely AmpD3 did not possess the full capacity to repress the ampC expression. After introducing pSRKTcD3 (high expression AmpD3) into YEΔD1D2D3, the β-lactamase activity is a limited reduction, not returning to the level of the wild-type strain. Further, in P. aeruginosa, PAΔDDh3 shows a high-level hyper-inducible expression state not observed in Y. enterocolitica.
As shown in Table 3, the ampD mutant strains was maximal quadrupling the MIC-values on TIC, PIP, TZP, CFZ, CAZ, ATM but there is no CLSI breakpoint was surpassed in any of the antibiotics tested in this experiment. This consequence might seem like lack of clinical significance on the surface, but actually, ampC-ampR system may participate in many aspect of bacterial metabolization, as AmpR is a global transcriptional factor in β-lactamases, proteases, quorum sensing, virulence factors, and even iron acquisition (Balasubramanian et al., 2014; Caille et al., 2014). AmpD was also at the crossroads in cell-wall recycling and AmpC regulation simultaneously (Lee et al., 2009). Three AmpD homologs cooperated with each other in cell-wall recycling system results in a stabilized and efficient process to recycle the useless peptidoglycan degradation products into cell wall synthesis, so less energy will be needed in cell growth and division. In survival in eukaryotes, extracellular muropeptides can be recognized by the immune system, a potent cell-wall recycling system ensures less muropeptides release results in higher survival rates (Johnson et al., 2013). Moya et al. (2008) found in competition experiments in the mouse model in vivo, the ampD ampDh2 ampDh3 and ampC quadruple mutant P. aeruginosa strain completely lost its biological competitiveness.
Recent studies demonstrate Y. enterocolitica strains of specific biovars tend to display similar features in expression and the activities of two different β-lactamases (Pham et al., 2000; Stock et al., 2000; Sharma et al., 2006). Further studies are necessary to understand the distribution and function of AmpD homologs in each biovar of the Y. enterocolitica strains. Especially the AmpD3, less effective alone, but has a potent synergetic effect together with AmpD1. Furthermore, recent studies showed that penicillin-binding protein 4 (DacB) affected the AmpC expression in some bacteria (Zamorano et al., 2010), it will be attractive to understand its function in Y. enterocolitica.
In summary, in the present study, we first illustrate the role of AmpD in Y. enterocolitica and a three-step regulation mechanism of ampC expression was found. Furthermore, this was also the first report such complicated ampC regulation mechanism appears in Enterobacteriaceae. It will be very interesting to examine if this minority mechanism appears in other Enterobacteriaceae family strains.
Author Contributions
HJ, SS, and ChaL design the experiment together. ChaL, YC, XL, ChuL, and HH finish the work. JL, RD, and JZ participate in the manuscript translation.
Conflict of Interest Statement
The authors declare that the research was conducted in the absence of any commercial or financial relationships that could be construed as a potential conflict of interest.
Acknowledgments
We thank Liuying Tang and Jim Nelson for critical reading and helpful comments on our manuscript. This work was supported by the National Natural Science Foundation of China (General Project, no. 81470092) and the National Sci-Tech Key Project (2012ZX10004201, 2013ZX10004203-002).
References
Balasubramanian, D., Kumari, H., Jaric, M., Fernandez, M., Turner, K. H., Dove, S. L., et al. (2014). Deep sequencing analyses expands the Pseudomonas aeruginosa AmpR regulon to include small RNA-mediated regulation of iron acquisition, heat shock and oxidative stress response. Nucleic Acids Res. 42, 979–998. doi: 10.1093/nar/gkt942
Bent, Z. W., and Young, G. M. (2010). Contribution of BlaA and BlaB beta-lactamases to antibiotic susceptibility of Yersinia enterocolitica biovar 1B. Antimicrob. Agents Chemother. 54, 4000–4002. doi: 10.1128/AAC.01754-09
Bou, G., and Martínez-Beltrán, J. (2000). Cloning, nucleotide sequencing, and analysis of the gene encoding an AmpC beta-lactamase in Acinetobacter baumannii. Antimicrob. Agents Chemother. 44, 428–432. doi: 10.1128/AAC.44.2.428-432.2000
Caille, O., Zincke, D., Merighi, M., Balasubramanian, D., Kumari, H., Kong, K. F., et al. (2014). Structural and functional characterization of Pseudomonas aeruginosa global regulator AmpR. J. Bacteriol. 196, 3890–3902. doi: 10.1128/JB.01997-14
Cheng, Q., and Park, J. T. (2002). Substrate specificity of the AmpG permease required for recycling of cell wall anhydro-muropeptides. J. Bacteriol. 184, 6434–6436. doi: 10.1128/JB.184.23.6434-6436.2002
Cornelis, G., and Abraham, E. P. (1975). Beta-lactamases from Yersinia enterocolitica. J. Gen. Microbiol. 87, 273–284. doi: 10.1099/00221287-87-2-273
De La Prieta, M. C., Francia, M. V., Seoane, A., and Lobo, J. M. (2006). Characterization of defective beta-lactamase genes in Yersinia enterocolitica. J. Antimicrob. Chemother. 58, 661–664. doi: 10.1093/jac/dkl267
De La Prieta, M. C., Seoame, A., Díaz, J., Navas, J., and Garciá-Lobo, J. M. (1995). Beta-lactamase genes and beta-lactamic susceptibility in Yersinia enterocolitica. Contrib. Microbiol. Immunol. 13, 184–187.
Généreux, C., Dehareng, D., Devreese, B., Van Beeumen, J., Frère, J. M., and Joris, B. (2004). Mutational analysis of the catalytic centre of the Citrobacter freundii AmpD N-acetylmuramyl-L-alanine amidase. Biochem. J. 377, 111–120. doi: 10.1042/bj20030862
Hammer, B. K., and Bassler, B. L. (2007). Regulatory small RNAs circumvent the conventional quorum sensing pathway in pandemic Vibrio cholerae. Proc. Natl. Acad. Sci. U.S.A. 104, 11145–11149. doi: 10.1073/pnas.0703860104
Hanson, N. D., and Sanders, C. C. (1999). Regulation of inducible AmpC beta-lactamase expression among Enterobacteriaceae. Curr. Pharm. Des. 5, 881–894.
Höltje, J. V., Kopp, U., Ursinus, A., and Wiedemann, B. (1994). The negative regulator of beta-lactamase induction AmpD is a N-acetyl-anhydromuramyl-L-alanine amidase. FEMS Microbiol. Lett. 122, 159–164. doi: 10.1111/j.1574-6968.1994.tb07159.x
CLSI (2015). Performance Standards for Antimicrobial Susceptibility Testing. Twenty-fourth Informational Supplement. CLSI Document M100-S25. Wayne, PA.
Jacobs, C., Frère, J. M., and Normark, S. (1997). Cytosolic intermediates for cell wall biosynthesis and degradation control inducible beta-lactam resistance in gram-negative bacteria. Cell 88, 823–832. doi: 10.1016/S0092-8674(00)81928-5
Jacobs, C., Huang, L. J., Bartowsky, E., Normark, S., and Park, J. T. (1994). Bacterial cell wall recycling provides cytosolic muropeptides as effectors for beta-lactamase induction. EMBO J. 13, 4684–4694.
Jacobs, C., Joris, B., Jamin, M., Klarsov, K., Van Beeumen, J., Mengin-Lecreulx, D., et al. (1995). AmpD, essential for both beta-lactamase regulation and cell wall recycling, is a novel cytosolic N-acetylmuramyl-L-alanine amidase. Mol. Microbiol. 15, 553–559. doi: 10.1111/j.1365-2958.1995.tb02268.x
Jacoby, G. A. (2009). AmpC beta-lactamases. Clin. Microbiol. Rev. 22, 161–182, Table of Contents. doi: 10.1128/CMR.00036-08
Jaurin, B., Grundstrom, T., Edlund, T., and Normark, S. (1981). The E. coli beta-lactamase attenuator mediates growth rate-dependent regulation. Nature 290, 221–225. doi: 10.1038/290221a0
Johnson, J. W., Fisher, J. F., and Mobashery, S. (2013). Bacterial cell-wall recycling. Ann. N. Y. Acad. Sci. 1277, 54–75. doi: 10.1111/j.1749-6632.2012.06813.x
Juan, C., Moyá, B., Pérez, J. L., and Oliver, A. (2006). Stepwise upregulation of the Pseudomonas aeruginosa chromosomal cephalosporinase conferring high-level beta-lactam resistance involves three AmpD homologues. Antimicrob. Agents Chemother. 50, 1780–1787. doi: 10.1128/AAC.50.5.1780-1787.2006
Kerff, F., Petrella, S., Mercier, F., Sauvage, E., Herman, R., Pennartz, A., et al. (2010). Specific structural features of the N-acetylmuramoyl-L-alanine amidase AmiD from Escherichia coli and mechanistic implications for enzymes of this family. J. Mol. Biol. 397, 249–259. doi: 10.1016/j.jmb.2009.12.038
Kong, K. F., Jayawardena, S. R., Indulkar, S. D., Del Puerto, A., Koh, C. L., Høiby, N., et al. (2005). Pseudomonas aeruginosa AmpR is a global transcriptional factor that regulates expression of AmpC and PoxB beta-lactamases, proteases, quorum sensing, and other virulence factors. Antimicrob. Agents Chemother. 49, 4567–4575. doi: 10.1128/AAC.49.11.4567-4575.2005
Lee, M., Zhang, W., Hesek, D., Noll, B. C., Boggess, B., and Mobashery, S. (2009). Bacterial AmpD at the crossroads of peptidoglycan recycling and manifestation of antibiotic resistance. J. Am. Chem. Soc. 131, 8742–8743. doi: 10.1021/ja9025566
Liang, J., Wang, X., Xiao, Y., Cui, Z., Xia, S., Hao, Q., et al. (2012). Prevalence of Yersinia enterocolitica in pigs slaughtered in Chinese abattoirs. Appl. Environ. Microbiol. 78, 2949–2956. doi: 10.1128/AEM.07893-11
Lindberg, F., Lindquist, S., and Normark, S. (1987). Inactivation of the ampD gene causes semiconstitutive overproduction of the inducible Citrobacter freundii beta-lactamase. J. Bacteriol. 169, 1923–1928.
Moya, B., Juan, C., Albertí, S., Pérez, J. L., and Oliver, A. (2008). Benefit of having multiple ampD genes for acquiring beta-lactam resistance without losing fitness and virulence in Pseudomonas aeruginosa. Antimicrob. Agents Chemother. 52, 3694–3700. doi: 10.1128/AAC.00172-08
Park, J. T., and Uehara, T. (2008). How bacteria consume their own exoskeletons (turnover and recycling of cell wall peptidoglycan). Microbiol. Mol. Biol. Rev. 72, 211–227, Table of Contents. doi: 10.1128/MMBR.00027-07
Peter, K., Korfmann, G., and Wiedemann, B. (1988). Impact of the ampD gene and its product on beta-lactamase production in Enterobacter cloacae. Rev. Infect. Dis. 10, 800–805. doi: 10.1093/clinids/10.4.800
Petersen, T. N., Brunak, S., Von Heijne, G., and Nielsen, H. (2011). SignalP 4.0: discriminating signal peptides from transmembrane regions. Nat. Methods 8, 785–786. doi: 10.1038/nmeth.1701
Pham, J. N., Bell, S. M., Martin, L., and Carniel, E. (2000). The beta-lactamases and beta-lactam antibiotic susceptibility of Yersinia enterocolitica. J. Antimicrob. Chemother. 46, 951–957. doi: 10.1093/jac/46.6.951
Philippe, N., Alcaraz, J. P., Coursange, E., Geiselmann, J., and Schneider, D. (2004). Improvement of pCVD442, a suicide plasmid for gene allele exchange in bacteria. Plasmid 51, 246–255. doi: 10.1016/j.plasmid.2004.02.003
Seoane, A., Francia, M. V., and García Lobo, J. M. (1992). Nucleotide sequence of the ampC-ampR region from the chromosome of Yersinia enterocolitica. Antimicrob. Agents Chemother. 36, 1049–1052. doi: 10.1128/AAC.36.5.1049
Sharma, S., Mittal, S., Mallik, S., and Virdi, J. S. (2006). Molecular characterization of beta-lactamase genes blaA and blaB of Yersinia enterocolitica biovar 1A. FEMS Microbiol. Lett. 257, 319–327. doi: 10.1111/j.1574-6968.2006.00191.x
Simon, R., Priefer, U., and, A., Puhler (1983). A broad range mobilization system for in vivo genetic engineering: transposon mutagenesis in gramnegative bacteria. Nat. BioTechnol. 1, 784–791. doi: 10.1038/nbt1183-784
Stock, I., Heisig, P., and Wiedemann, B. (1999). Expression of beta-lactamases in Yersinia enterocolitica strains of biovars 2, 4 and 5. J. Med. Microbiol. 48, 1023–1027. doi: 10.1099/00222615-48-11-1023
Stock, I., Heisig, P., and Wiedemann, B. (2000). Beta-lactamase expression in Yersinia enterocolitica biovars 1A, 1B, and 3. J. Med. Microbiol. 49, 403–408. doi: 10.1099/0022-1317-49-5-403
Talfan, A., Mounsey, O., Charman, M., Townsend, E., and Avison, M. B. (2013). Involvement of mutation in ampD I, mrcA, and at least one additional gene in beta-lactamase hyperproduction in Stenotrophomonas maltophilia. Antimicrob. Agents Chemother. 57, 5486–5491. doi: 10.1128/AAC.01446-13
Wang, X., Cui, Z., Jin, D., Tang, L., Xia, S., Wang, H., et al. (2009). Distribution of pathogenic Yersinia enterocolitica in China. Eur. J. Clin. Microbiol. Infect. Dis. 28, 1237–1244. doi: 10.1007/s10096-009-0773-x
Wang, X., Li, Y., Jing, H., Ren, Y., Zhou, Z., Wang, S., et al. (2011). Complete genome sequence of a Yersinia enterocolitica “Old World” (3/O:9) strain and comparison with the “New World” (1B/O:8) strain. J. Clin. Microbiol. 49, 1251–1259. doi: 10.1128/JCM.01921-10
Yang, T. C., Huang, Y. W., Hu, R. M., Huang, S. C., and Lin, Y. T. (2009). AmpDI is involved in expression of the chromosomal L1 and L2 beta-lactamases of Stenotrophomonas maltophilia. Antimicrob. Agents Chemother. 53, 2902–2907. doi: 10.1128/AAC.01513-08
Zamorano, L., Reeve, T. M., Deng, L., Juan, C., Moyá, B., Cabot, G., et al. (2010). NagZ inactivation prevents and reverts beta-lactam resistance, driven by AmpD and PBP 4 mutations, in Pseudomonas aeruginosa. Antimicrob. Agents Chemother. 54, 3557–3563. doi: 10.1128/AAC.00385-10
Keywords: Yersinia enterocolitica, AmpC β-lactamase, AmpD, synergy effect, antimicrobial resistance
Citation: Liu C, Wang X, Chen Y, Hao H, Li X, Liang J, Duan R, Li C, Zhang J, Shao S and Jing H (2016) Three Yersinia enterocolitica AmpD Homologs Participate in the Multi-Step Regulation of Chromosomal Cephalosporinase, AmpC. Front. Microbiol. 7:1282. doi: 10.3389/fmicb.2016.01282
Received: 22 March 2016; Accepted: 03 August 2016;
Published: 18 August 2016.
Edited by:
Miguel Cacho Teixeira, University of Lisbon, PortugalReviewed by:
Xue-jie Yu, University of Texas Medical Branch, USADongsheng Zhou, Beijing Institute of Microbiology and Epidemiology, China
Copyright © 2016 Liu, Wang, Chen, Hao, Li, Liang, Duan, Li, Zhang, Shao and Jing. This is an open-access article distributed under the terms of the Creative Commons Attribution License (CC BY). The use, distribution or reproduction in other forums is permitted, provided the original author(s) or licensor are credited and that the original publication in this journal is cited, in accordance with accepted academic practice. No use, distribution or reproduction is permitted which does not comply with these terms.
*Correspondence: Shihe Shao, shaoshihe2006@163.com
Huaiqi Jing, jinghuaiqi@icdc.cn
†These authors have contributed equally to this work.