- 1Department of Biotechnology, Institute of Life Sciences, Bhubaneswar, India
- 2Computational and Systems Biology Group, Genome Institute of Singapore, Singapore, Singapore
This study describes the community composition and functions of the microbiome associated with the mucus of the coral Fungia echinata based on metagenomic approach. Metagenome sequence data showed a dominance of the class Gammaproteobacteria followed by Alphaproteobacteria, Betaproteobacteria, Deltaproteobacteria, Flavobacteriia, Bacilli, and Clostridia. At the order level, the most abundant groups were Pseudomonadales, Oceanospirillales, Alteromonadales, and Rhodobacterales. The genus Psychrobacter was the most predominant followed by Thalassolituus and Cobetia, although other genera were also present, such as Sulfitobacter, Pseudoalteromonas, Oleispira, Halomonas, Oceanobacter, Acinetobacter, Pseudomonas, Vibrio, and Marinobacter. The metabolic profile of the bacterial community displayed high prevalence of genes associated with core-housekeeping processes, such as carbohydrates, amino acids, proteins, and nucleic acid metabolism. Further, high abundance of genes coding for DNA replication and repair, stress response, and virulence factors in the metagenome suggested acquisition of specific environmental adaptation by the microbiota. Comparative analysis with other coral metagenome exhibits marked differences at the taxonomical and functional level. This study suggests the bacterial community compositions are influenced by the specific coral micro-niche and the oligotrophic marine environment.
Introduction
India has a vast reserve of coral reef ecosystem in the east coast of Andaman and Nicobar Islands. Microbial communities are vital in the functioning of all ecosystems; however, most microorganisms are uncultivated, and their roles in natural systems are unclear. In this regard, microbial diversity in the Andaman Sea is one of the least studied among marine environments. In the recent past, metagenomic study has been employed in the field of marine microbial ecology to explore the diversity of unknown microbes in different marine habitats. It has been demonstrated by comparative metagenomic study that functional attributes in the microbial communities are evolved by typical environmental adaptation (Tyson et al., 2004; Daniel, 2005; Simon and Daniel, 2011). Bacteria are known to be abundant in seawater around coral zones, in coral skeleton, tissues, and surface mucus layer (Rohwer et al., 2002; Lampert et al., 2006, 2008; Kooperman et al., 2007; Rosenberg et al., 2007; Bayer et al., 2013), and each of these habitat supports different bacterial species (Koren and Rosenberg, 2006; Littman et al., 2009). However, functional role of microbiome associated with coral is poorly understood, although few studies have suggested their involvement in the supply of nutrients (Lesser et al., 2007; Olson et al., 2009) and inhibition of coral diseases by the production of antibiotics (Rohwer et al., 2002; Ritchie, 2006; Krediet et al., 2013). Metagenomic approach gives a description of the taxonomic information (Tyson et al., 2004), the relative abundance of phylotypes and genes (Wegley et al., 2007; Littman et al., 2011), antibiotic resistance reservoir (Sommer et al., 2009), and identification of genes involved in various biosynthetic and metabolic pathways (Schirmer et al., 2005; Tringe et al., 2005; Rodriguez-Brito et al., 2006). This approach enables assessment of taxonomic and functional characteristics of complex microbial communities.
Coral mucus is a rich source of nutrients for microorganisms (Wild et al., 2004; Brown and Bythell, 2005; Tremblay et al., 2011) and as such the mucus layer constitutes an important ecological niche for microbiome (Lampert et al., 2006, 2008; Nithyanand and Pandian, 2009). Following culture-dependent approach, we have identified several new species of bacteria with unique functions from the corals collected from Andaman Sea (Badhai et al., 2013; Kumari et al., 2014; Lepcha et al., 2015). However, taxonomic and functional profiling of the microbiome of the coral Fungia echinata from Andaman Sea has not been performed so far. As the microbial diversity and functions are directly linked with local environment, it is justifying examination of new niches for novel genes and species. In this regard, our metagenomic study with the coral mucus of F. echinata provides distinct taxonomical and functional attributes that has not been reported earlier in other marine environment.
Materials and Methods
Collection of the Metagenome and Sequencing
Metagenomic DNA was prepared from the mucus of multiple specimens (five numbers) of coral F. echinata collected from five different locations within the reefs around the Havelock Island in Andaman Sea (Figure 1). Coral specimens were collected during March, 2012 from a depth of about 3.5–5 m. Metagenomic DNA was prepared from pooled mucus samples following an earlier protocol (Goldenberger et al., 1995) with slight modifications. Typically, the metagenome extraction process starts by filtering samples onto 0.22-μm Millex filters. In this regard, corals were briefly expose to air (Wild et al., 2005), placed in a sterile tray and then repeatedly flushed with sterile marine water using a 50 ml syringe. About 500 ml of the pooled coral mucus suspension in marine water was filtered and the membrane was collected and washed with 10 ml Milli-Q water by vortexing. The suspension was centrifuged at 14,000 rpm for 10 min to collect the cell pellet. Cell pellet was transferred to a Lysing Matrix E tube. Reaming procedures was followed as per manufacturer instruction (FastDNA® SPIN Kit for Soil, MP Biomedicals, CA, USA). The concentration of DNA was approximately 150 ng μl-1. Sequencing of the metagenomic DNA samples were done by a commercial source (NxGenBio Life Sciences, New Delhi, India) applying shotgun pyrosequencing approach on a Roche 454 GS-FLX platform (Roche Applied Sciences, Manheim, Germany) according to the manufacturer’s protocol. A total of 40,938 reads representing cumulative 20.61 Mb of sequence data were obtained.
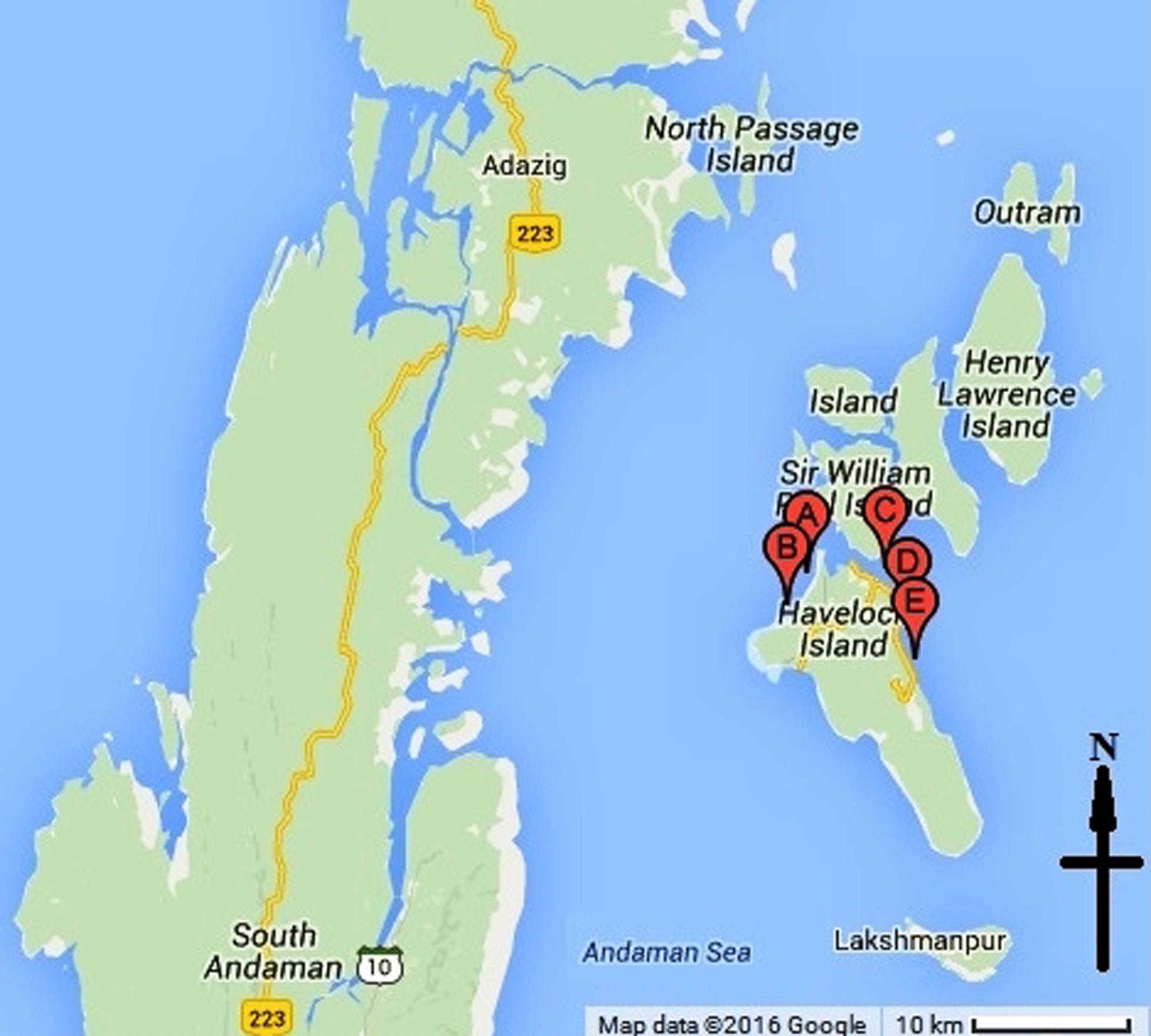
FIGURE 1. Location of the sampling sites around the Havelock Island in Andaman Sea. Points A, B, C, D, and E on the map represent the five sampling sites.
Metagenomic DNA Sequence Data Generation
Raw sequence reads were processed and calculation of DNA sequence statistics such as length and GC content of the processed reads were carried out using the freely available online WebMGA server1 (Wu et al., 2011). The total shotgun metagenomic sequences from each site were preprocessed using the following parameters: (i) quality filtration (at min length = 200 bases and min average quality score = 21) and (ii) CD-HIT-454 clustering at a sequence identity threshold of 0.98 to remove artificial duplicates generated during sequencing (Niu et al., 2010). Subsequently, after the preprocessing approx. 26,534 sequence reads (av. sequence length = 516 bases, av. % GC content = 48.73) were retained for further analysis.
Taxonomic and Functional Analysis of Metagenome
Taxonomic and functional assignments for the protein-coding sequences in each metagenomic reads dataset were obtained using BLASTX (Altschul et al., 1997; applying an e-value cut-off of 1e–10) against NCBI-NR database (local BLAST-2.2.29+ package; BLAST® Help, 2008; Camacho et al., 2009) and the standalone MEtaGenome analyzer software (MEGAN v5.5.3; Huson et al., 2011) according to suggested parameters for the lowest common ancestor (LCA) assignment algorithm (min score: 50.0; max expected: 0.01; top percent: 10.0; and min support percent: 0.01). To perform taxonomic classification, the MEGAN5 program placed the reads/genes onto the NCBI taxonomy tree, whereas for the functional classifications, genes were mapped onto the SEED and KEGG classification using SEED and KEGG identifiers, respectively. Additionally, taxonomic and functional annotation, and comparisons with other coral metagenomes (Dinsdale et al., 2008; Vega Thurber et al., 2009; Littman et al., 2011) were carried out on the MG-RAST v3.6 metagenome analysis server2 (Meyer et al., 2008) using the default set parameters (e-value cutoff: < 1e–5, min. % identity cutoff: 60%, and min. alignment length cutoff: 15).
Statistical Analysis of Data
Comparative taxonomic and functional profiling were performed with the reference coral metagenomes available in MG-RAST database using the STAMP v2.0.8 software (Parks and Beiko, 2010; Parks et al., 2014) for statistical analyses. The gene counts were normalized by dividing the number of gene hits to individual taxa/function by total number of gene hits in each metagenome dataset to remove bias due to difference in sequencing efforts. To identify differentially abundant SEED or KEGG functions in the metagenome, statistical tests of the relative gene abundances compared to the other coral metagenomes were carried out by applying two-sided Welch’s exact test with Benjamini–Hochberg False Discovery Rate (FDR) multiple test correction method and a p-value < 0.05.
Sequence Data Availability
Metagenomic sequence dataset obtained from the mucus of coral F. echinata is available on the MG-RAST server under accession ID 4653307.3. The other 12 metagenomes used in the comparative analysis can be accessed through the MG-RAST website under the accession IDs: 4440037.3, 4440039.3, 4440041.3, 4440279.3, 4445755.3, 4445756.3, 4440372.3, 4440373.3, 4440378.3, 4440379.3, 4440380.3, and 4440381.3.
Results and Discussions
Taxonomic Composition of the Microbial Community
Bacteria constituted the single largest kingdom within the mucus-associated microbial assemblage of the coral F. echinata. Approximately 75.96% of the metagenomic sequences were classified as Bacteria and only 0.21% as viruses; the remaining 23.83% of the sequences could not be classified due to lack of reference sequences from close taxonomic relatives. The bacterial community in the coral mucus was dominated by sequences affiliated to the class Gammaproteobacteria (64.7%), followed by the class Alphaproteobacteria (3.5%); the other bacterial sequences (abundances <0.1–0.2%) were affiliated to the Betaproteobacteria, Flavobacteriia, Clostridia, Deltaproteobacteria, and Bacilli (Figure 2). The virus-like sequences were classified as dsDNA viruses of the order Caudovirales. Gammaproteobacteria was the sole dominant phylum. This phylum displayed a large phylogenetic diversity that might explain the colonization of a large range of ecological environment (Williams et al., 2010). Our analysis of the microbiome of F. echinata supported the fact that microbial diversity is directly linked with local environment. Further, this community was different from those reported in earlier studies, which suggested that microbial association with coral species reveal species-specific distribution and geographic variability (Rohwer et al., 2002; Lampert et al., 2006, 2008; Wegley et al., 2007; Littman et al., 2009; Morrow et al., 2012).
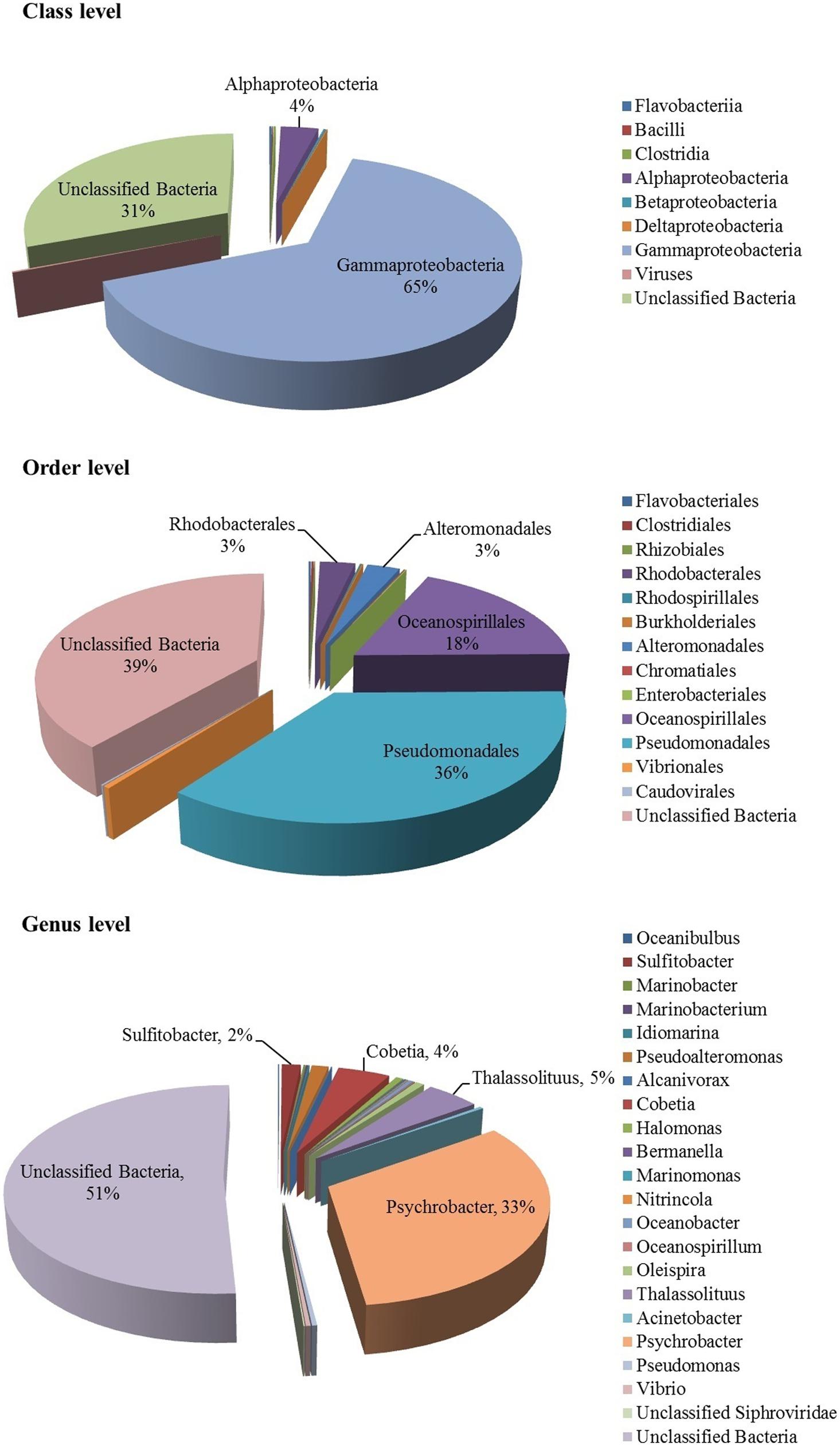
FIGURE 2. Taxonomic composition of the mucus-associated microbiome of coral F. echinata from Andaman Sea based on metagenomic sequencing.
At the order level (Figure 2), the major bacterial groups identified were Pseudomonadales (35.6%), Oceanospirillales (17.9%), Rhodobacterales (3.0%), and Alteromonadales (2.8%), whereas the abundance of taxa (< 1%) were Vibrionales, Clostridales, Flavobacteriales, Rhodospirillales, Burkholderiales, Enterobacteriales, Chromatiales, and Rhizobiales. Further classification at the genus level suggested that the Psychrobacter (32.7%) was the most predominant bacterial group in the metagenome and the other genera that were identified include Thalassolituus (4.6%), Cobetia (4.5%), Sulfitobacter (1.6%), and Pseudoalteromonas (1.4%). In addition, less abundant (<1%) genera were also observed (Figure 2). The previous study with the metagenome of the coral Ctenactis echinata from Red Sea (Roder et al., 2015) demonstrated the predominance of the genus Endozoicomonas. In contrast, a significant change was observed in the microbial community of F. echinata from Andaman Sea, which become dominated by Psychrobacter. As we found that mucus microbiome become more dissimilar from other studies with geographical distance, indicating that different regions harbor distinct communities (Sunagawa et al., 2009; Littman et al., 2010). One possible explanation could be water temperature in the Andaman Sea (25–28°C), leading to the development of Psychrobacter community in the mucus layer, which has been frequently identified in the Antarctic deep waters (López-García et al., 2001) and it is more dissimilar with the bacterial communities of fungid coral C. echinata in Red Sea (Roder et al., 2015). These differences might be in part due to the physicochemical condition of the Red Sea which has been characterized as an oligotrophic environment with year round UV irradiation and high surface temperature up to 34°C (Ngugi et al., 2012). Further, bacteria particularly Vibrio species are ubiquitous have been implicated as the causative agent in some cases of coral and influencing the coral health (Rosenberg et al., 2007; Bourne et al., 2008), but very low abundance of sequences affiliated to the Vibrio group probably due to specific micro-niche (i.e., surface mucus layer) or the general structure of the bacteria–coral association. The unclassified group representing 23.83% of the sequences may serve important functional roles in biogeochemical cycle and degradation of xenobiotic compounds and warrant further investigation, especially in highly dynamic ecosystem.
Functional Analysis of the Metagenome
Functional analysis of the metagenomic showed approximately 60 and 36% of the predicted protein coding sequences or genes were matched to 25 SEED subsystems and 23 KEGG categories, respectively (Figure 3). Analysis of metagenome suggested that genes associated with the core-housekeeping functions, such as carbohydrates, amino acids, proteins, nucleotides, cofactors, and vitamin metabolism were the most abundant metabolic categories (Supplementary Figure S1). Our result corroborated the findings on coral-associated microbiomes in tropical environments (Wegley et al., 2007; Tout et al., 2014). In addition, genes affiliated to virulence, stress response, signal transduction, membrane transport, and DNA replication and repair functions were also highly abundant in our dataset (Supplementary Figure S1).
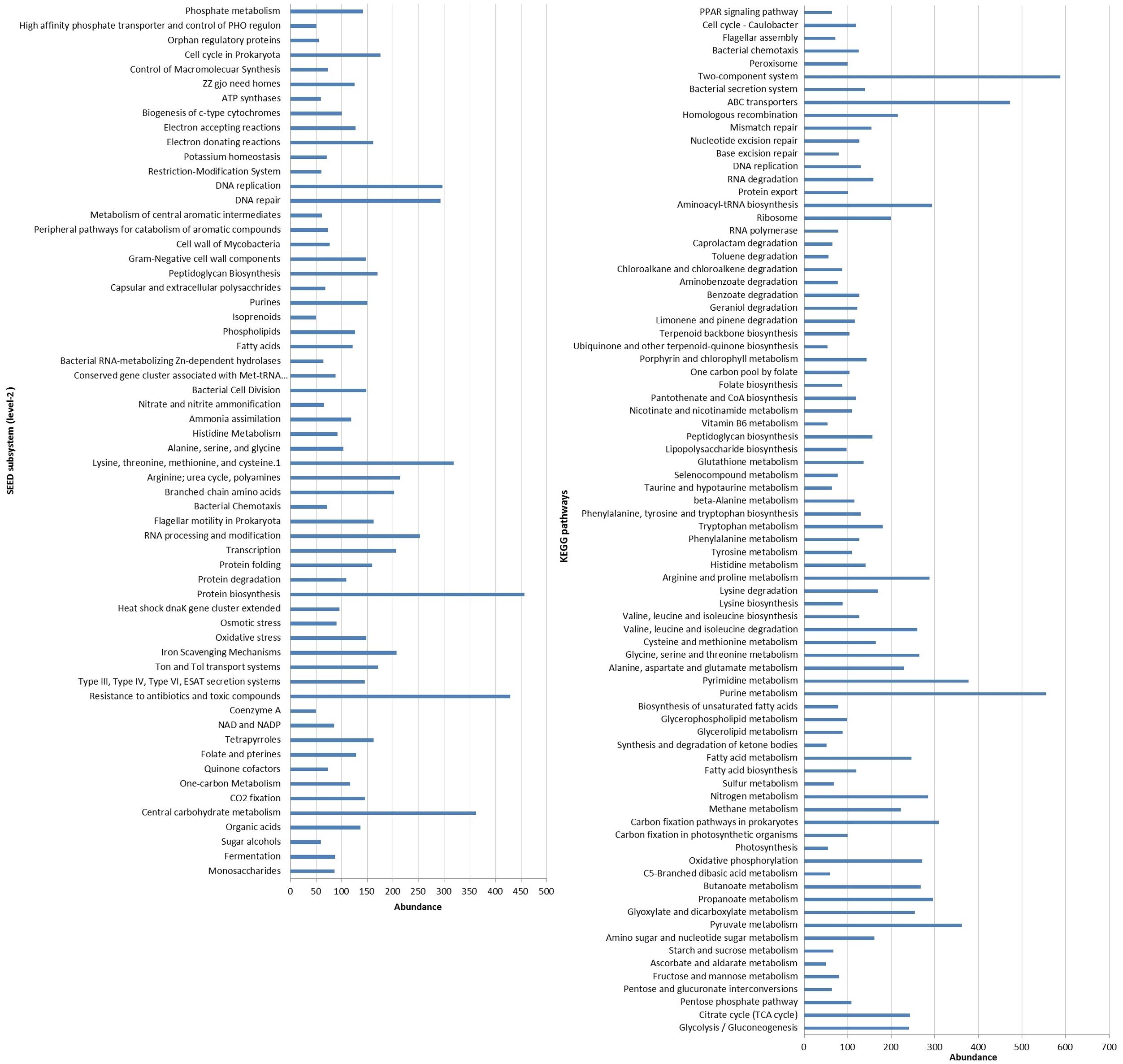
FIGURE 3. Distribution of functional genes related to various SEED subsystems (level 2) and KEGG pathways present in the metagenome of the coral F. echinata. Only genes with an abundance of at least 50 are shown.
The predicted genes encoding virulence functions (SEED level 2), such as iron-scavenging mechanisms, Ton and Tol transport systems, and protein secretion systems, were highly abundant (Supplementary Figure S2). As iron is an important micronutrient and poorly available in the seawater (Wells et al., 1994), the metabolic ability to take up and store iron is advantageous and these functional subsystems may have a modulatory role in the structuring of the coral-associated bacterial community. Further, genes encoding resistance to antibiotics and toxic compounds along with the oxidative stress, and DNA replication and repair functions were also highly represented (Figure 3, Supplementary Figure S2) and predicted that these coral-associated bacteria are more suited to deal with intense selection pressure and environmental stressors, such as heavy metal ions, reactive oxygen, and ultraviolet radiations, etc. The abundance of genes associated with the metabolic functions such as bacterial chemotaxis, two-component systems and ABC transporters (Figure 3) predicted higher level of cellular interactions and metabolic exchanges between the bacterial community and coral host (Tout et al., 2014; Ainsworth et al., 2015).
Earlier, McKew et al. (2012) reported the abundance of Psychrobacter in the mucus of Acropora spp. and Porites spp., but they have not analyzed the functions of these bacteria in the coral holobiont. In this regard, our analysis of genes affiliated to the genus Psychrobacter identified their functional role in the carbon and nitrogen metabolism within the coral niche. Accordingly, the abundance of genes related to carbohydrates, lipids and amino acids metabolisms (Figure 4) indicated the high metabolic potential of these bacteria to efficiently utilize the complex organic compounds in the coral mucus. Further, presence of the qseC gene predicted these bacteria respond to quorum sensing and form biofilm-like structure to successfully colonize the coral surface (Yang et al., 2014). In addition, the presence of genes corresponding to the two-component systems for sensing phosphate limitation (phoR, phoB, and phoD), stress (rstA and rstB), copper tolerance (cusS and cusR), nitrate respiration (narX and narL), and low nitrogen availability (glnL and glnG) revealed that these bacteria are better adapted to the continuous chemical fluctuations in the seawater surrounding the coral niche. Of the other metabolic categories that increased significantly within the coral metagenome, it is notable that genes associated with ABC transporters for ions of sulfate (cysPUWA), phosphate (pstS, pstC, and pstB), phosphonate (phnD), zinc (znuABC), nickel (oppABCDF), and iron (afuABC and fhuBCD). Furthermore, genes homologous to virulence, stress response, and DNA replication and repair functions were examined (Figure 4). Taken together, it appears that microorganisms associated with the mucus of the coral F. echinata have mechanisms to deal with biotic or abiotic stressors within the coral niche and help in their colonization.
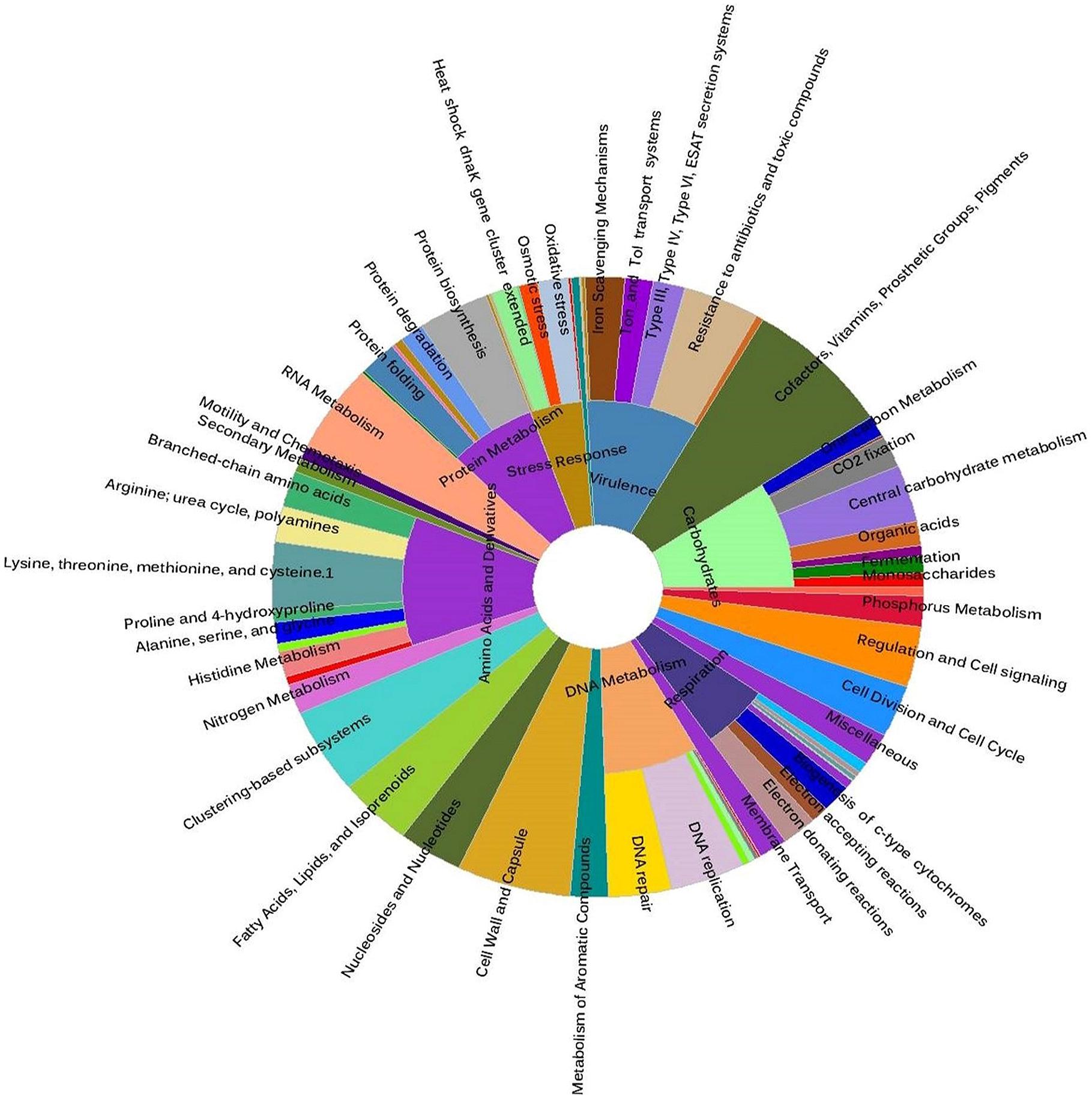
FIGURE 4. Metabolic profile of the mucus-associated bacterial genus Psychrobacter, based on SEED subsystem classification of the metagenomic sequences obtained in this study.
Comparative Analysis of Metagenomic Sequences with MG-RAST DataBase
The taxonomic and functional profiles of mucus-associated microbiome of F. echinata was compared with the microbial communities associated with (i) colonies of coral Acropora millepora from the Great Barrier Reef (Littman et al., 2011), (ii) coral Porites compressa from Hawaii (Vega Thurber et al., 2009), and (iii) four coral atolls (Kingman, Kiritimati, Palmyra, and Tabuaeran) in the Northern Line Islands, central Pacific (Dinsdale et al., 2008).
The taxonomic profile of microbial community associated with the mucus of F. echinata showed an exclusive dominance of Bacteria, belonging to the phylum Proteobacteria (Figure 5), whereas the functional profile showed higher abundance of genes involved in core-housekeeping functions, as well as genes encoding specialized and ecologically important metabolic functions. These genes are related to the metabolisms of glutathione, biotin, riboflavin, butanoate, tyrosine, tryptophan, glycerophospholipid, glyoxylate and dicarboxylate, lysine degradation, biosynthesis of folate, zeatin and lipopolysaccharide, degradation of nitrotoluene, benzoate, chlorocyclohexane and chlorobenzene, drug metabolism, catabolism of aromatic compounds, etc. (Supplementary Figures S3 and S4). Our analysis demonstrates the microbial diversity and distribution of various metabolic pathways over a previously unexplored range of bacterial phyla.
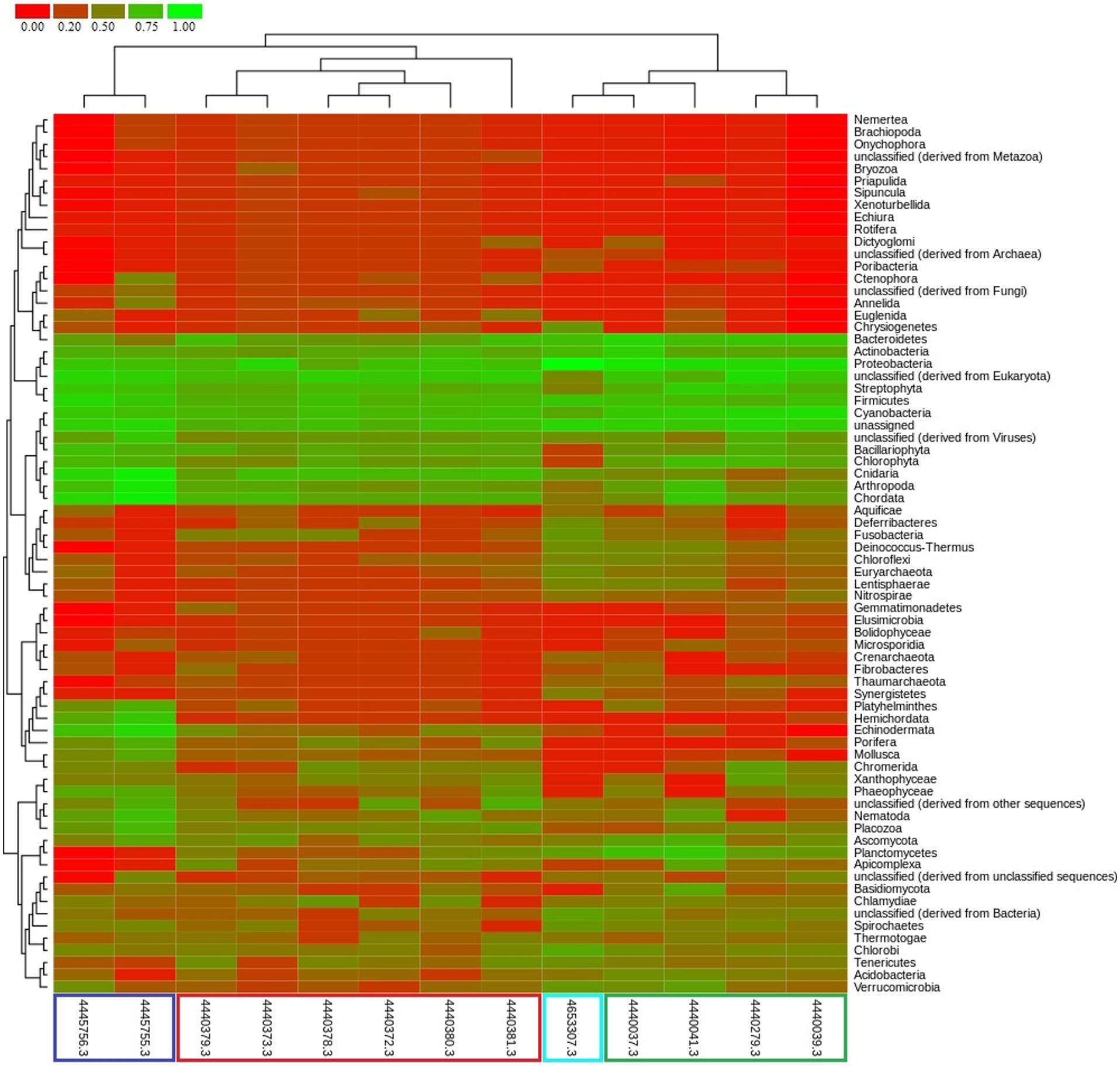
FIGURE 5. Comparative taxonomic profile of metagenomes generated using MG-RAST based on best hits to M5NR database. Metagenome data source: this study (cyan color), Dinsdale et al., 2008 (green color), Vega Thurber et al., 2009 (red color), and Littman et al., 2011 (blue color).
Clustering of the metagenome of F. echinata based on relative abundance of functional genes with those of healthy corals from the Great Barrier Reef and the Northern Line Islands’ reefs (Figure 6, Supplementary Figure S4) suggested that the association between the coral host and bacterial community very likely mutualistic in nature. Further, statistical tests of the relative abundance of genes encoding functions related to various SEED (level 2) and KEGG (level 2) categories showed significantly (p-value <0.05) higher proportions of genes encoding functions affiliated to resistance to antibiotics and toxic compounds, iron acquisition and metabolism, potassium, phosphorous and nitrogen metabolism, RNA processing and modification, oxidative stress, DNA replication and repair, signal transduction, membrane transport, cell motility, cofactors and vitamins metabolism, carbohydrates, lipids and amino acids metabolism, glycan biosynthesis and metabolism. In contrast, the genes related to the functions of energy metabolism and respiration, such as ATP synthases, electron donating and accepting reactions, and metabolism of di- and oligosaccharides were detected at significantly (p-value <0.05) lower proportions compared to the other coral metagenomes (Figure 7).
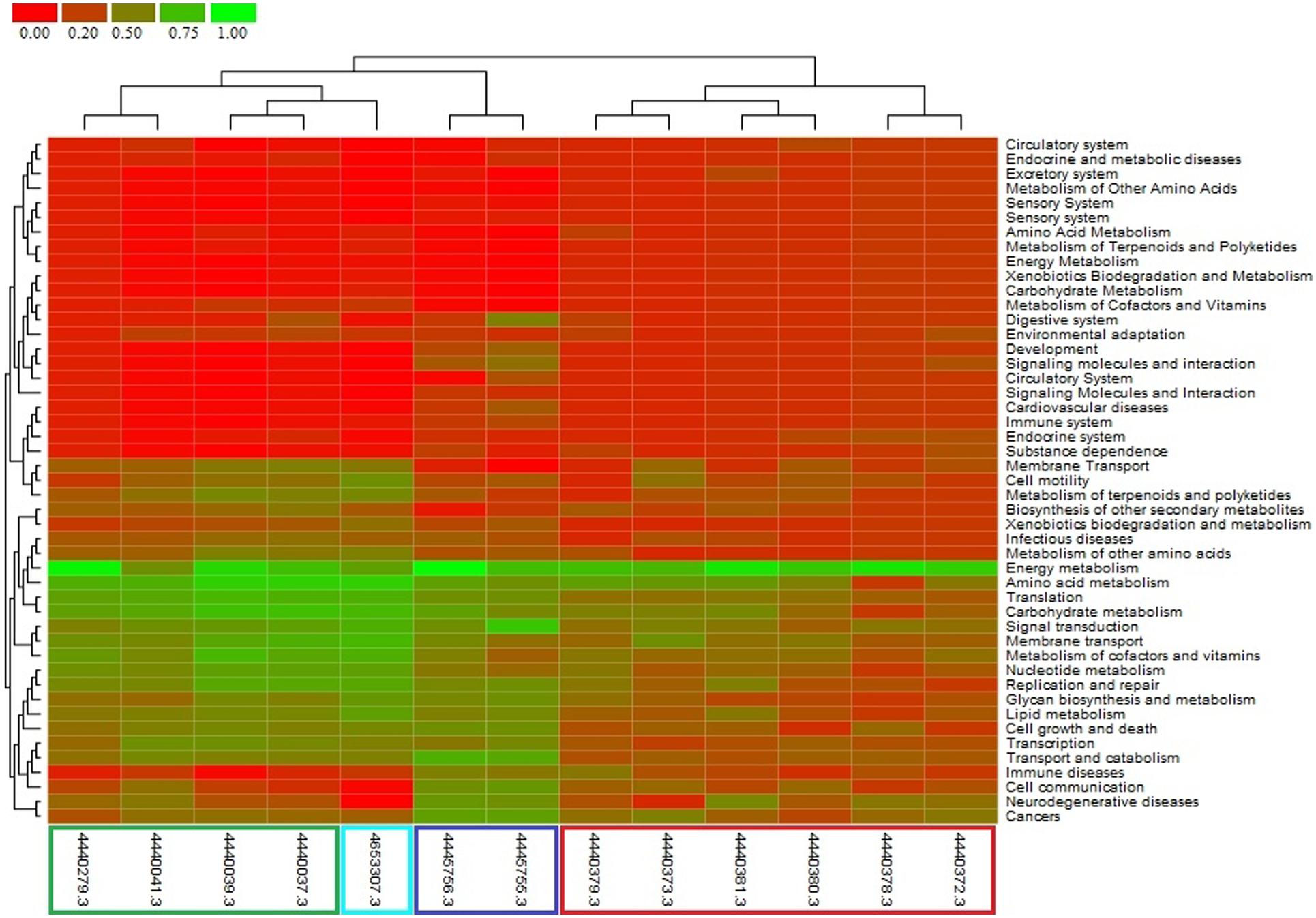
FIGURE 6. Comparative functional profile of metagenomes generated using the KEGG (level 2) classification of reads on MG-RAST server. Metagenome data source: this study (cyan color), Dinsdale et al., 2008 (green color), Vega Thurber et al., 2009 (red color), and Littman et al., 2011 (blue color).
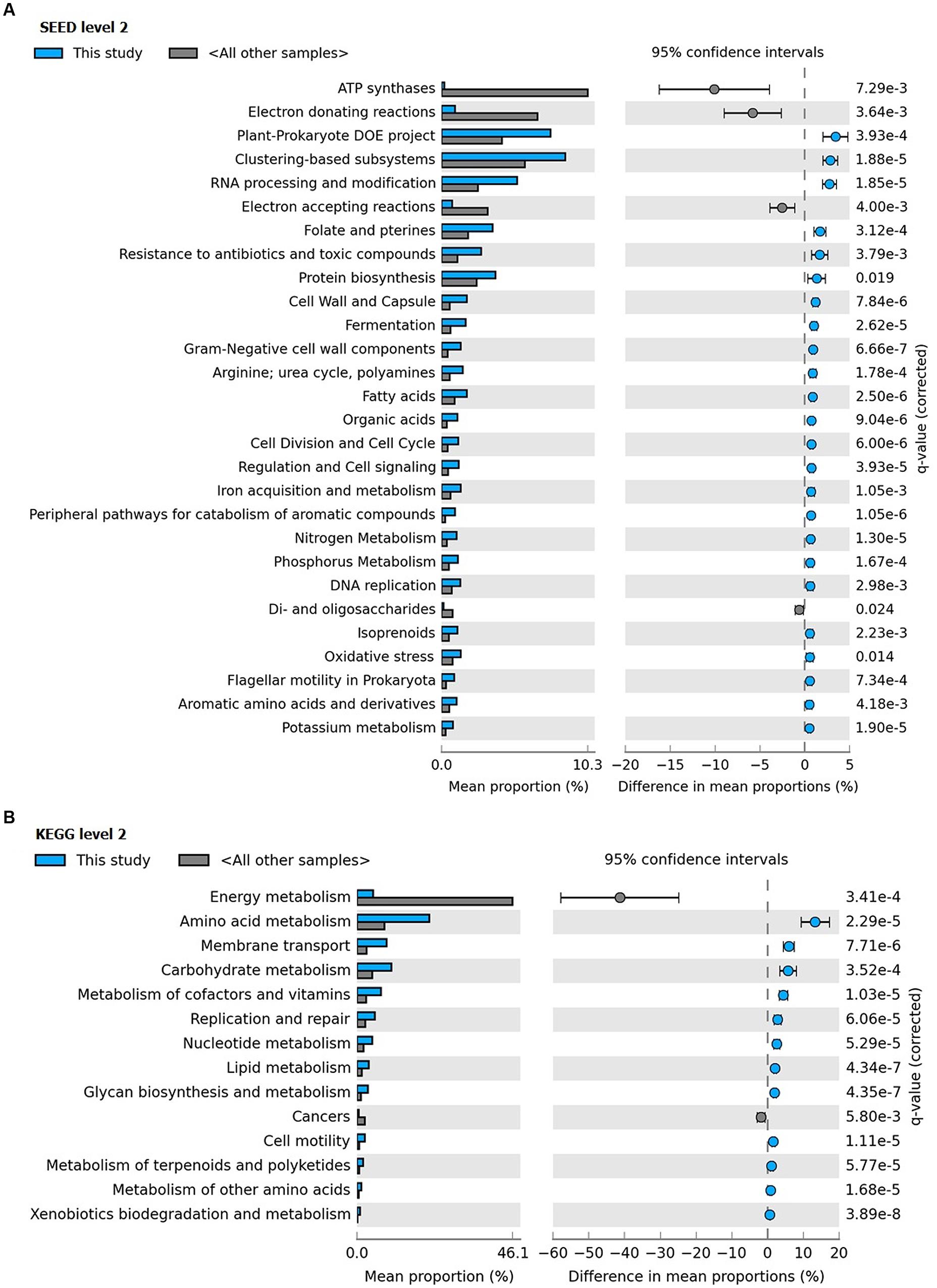
FIGURE 7. Distribution of the (A) SEED (level 2) and (B) KEGG (level 2) categories with differences between the coral F. echinata metagenome (this study; cyan color) and other coral metagenomes (dark gray color).
These findings indicated that specific environmental stressors and niches promote enrichment of specific metabolic pathways and functions which provides adaptive advantages to the bacterial community associated with corals in oligotrophic seawater. The metabolic processes related to signal transduction, membrane transport, and cell motility play an important role in structuring of the microbial communities. The high proportion of genes associated with these functions in the F. echinata metagenome as compared to the other coral metagenomes indicated that the mucus-associated bacteria have the metabolic flexibility to adapt in the changing nutrient concentrations and chemical gradients in the coral micro-niche.
Conclusions
The present study provides a metagenomic snapshot of the microbial community composition and functions in the mucus layer of the coral F. echinata from Andaman Sea. Our results showed an exclusive dominance of Bacteria affiliated to the class Gammaproteobacteria (specially, genus Psychrobacter, Thalassolituus, and Cobetia), while the virus-like sequences affiliated to the order Caudovirales constituted only a small fraction. Overall, the coral mucus-associated bacterial community was heterotrophic and carried genes required for the metabolism of complex organic compounds, such as proteins, lipids, and polysaccharides found in the coral mucus. Comparison with other coral metagenomes (both healthy and diseased corals) identified metabolic functions that are either required or advantageous for the coral colonization, such as resistance to antibiotics and toxic compounds, signal transduction, cell motility and membrane transport. Finally, mucus-associated microbiome of F. echinata is directly linked with local chemical gradients in the environment and different from those reported in other coral species from different geographic regions.
Author Contributions
SD conceived the idea of the work. JB designed the experiments and performed the experiments. JB, TG, and SD analyzed the data and wrote the manuscript.
Funding
This work was supported in part by the funding received from Ministry of Earth Sciences, Government of India (MoES/11-MRDF/1/59/P/08), and Department of Biotechnology, Government of India (D.O. No. BT/PR7661/AAQ/3/629/2013) to SD.
Conflict of Interest Statement
The authors declare that the research was conducted in the absence of any commercial or financial relationships that could be construed as a potential conflict of interest.
Acknowledgments
We are grateful to Ministry of Environment and Forest, Wild life Division, Government of India and The Chief Conservator of Forests (Wild life), Andaman and Nicobar Islands, Port Blair officially allowing us to collect coral samples from the Andaman Sea. The author JB acknowledges the Department of Biotechnology, Government of India for providing the research fellowship. We acknowledge the Distributed Information Sub-Center (DISC) at Institute of Life Sciences, Bhubaneswar for the computational facility. The geographical map was created from Google Maps using the Geoplaner software available at www.geoplaner.com.
Supplementary Material
The Supplementary Material for this article can be found online at: http://journal.frontiersin.org/article/10.3389/fmicb.2016.00936
Footnotes
References
Ainsworth, T. D., Krause, L., Bridge, T., Torda, G., Raina, J. B., Zakrzewski, M., et al. (2015). The coral core microbiome identifies rare bacterial taxa as ubiquitous endosymbionts. ISME J. 9, 2261–2274. doi: 10.1038/ismej.2015.39
Altschul, A. F., Madden, T. L., Schaffer, A. A., Zhang, J., Zhang, Z., Miller, W., et al. (1997). Gapped BLAST and PSI-BLAST: a new generation of protein database search programs. Nucleic Acids Res. 25, 3389–3402. doi: 10.1093/nar/25.17.3389
Badhai, J., Kumari, P., Krishnan, P., Ramamurthy, T., and Das, S. K. (2013). Presence of SXT integrating conjugative element in marine bacteria isolated from the mucus of the coral Fungia echinata from Andaman Sea. FEMS Microbiol. Lett. 338, 118–123. doi: 10.1111/1574-6968.12033
Bayer, T., Neave, M. J., Alsheikh-Hussain, A., Aranda, M., Yum, L. K., Mincer, T., et al. (2013). The microbiome of the Red Sea coral Stylophora pistillata is dominated by tissue-associated endozoicomonas Bacteria. Appl. Environ. Microbiol. 79, 4759–4762. doi: 10.1128/AEM.00695-13
BLAST® Help (2008). National Center for Biotechnology Information (US). Bethesda, MD. Available at : http://www.ncbi.nlm.nih.gov/books/NBK1762/
Bourne, D., Iida, Y., Uthicke, S., and Smith-Keune, C. (2008). Changes in coral-associated microbial communities during a bleaching event. ISME J. 2, 350–363. doi: 10.1038/ismej.2007.112
Brown, B. E., and Bythell, J. C. (2005). Perspectives on mucus secretion in reef corals. Mar. Ecol. Prog. Ser. 296, 291–309. doi: 10.3354/meps296291
Camacho, C., Coulouris, G., Avagyan, V., Ma, N., Papadopoulos, J., Bealer, K., et al. (2009). BLAST+: architecture and applications. BMC Bioinformatics 10:421. doi: 10.1186/1471-2105-10-421
Daniel, R. (2005). The metagenomics of soil. Nat. Rev. Microbiol. 3, 470–478. doi: 10.1038/nrmicro1160
Dinsdale, E. A., Pantos, O., Smriga, S., Edwards, R. A., Angly, F., Wegley, L., et al. (2008). Microbial ecology of four coral atolls in the northern line islands. PLoS ONE 3:e1584. doi: 10.1371/journal.pone.0001584
Goldenberger, D., Perschil, I., Ritzler, M., and Altwegg, M. (1995). A simple “universal” DNA extraction procedure using SDS and proteinase K is compatible with direct PCR amplification. PCR Methods Appl. 4, 368–370. doi: 10.1101/gr.4.6.368
Huson, D. H., Mitra, S., Ruscheweyh, H. J., Weber, N., and Schuster, S. C. (2011). Integrative analysis of environmental sequences using MEGAN 4. Genome Res. 21, 1552–1560. doi: 10.1101/gr.120618.111
Kooperman, N., Ben-Dov, E., Karmarsky-Winter, E., Barak, Z., and Kushmaro, A. (2007). Coral mucus-associated bacterial communities from natural and aquarium environments. FEMS Microbiol. Lett. 276, 106–113. doi: 10.1111/j.1574-6968.2007.00921.x
Koren, O., and Rosenberg, E. (2006). Bacteria associated with mucus and tissues of the coral Oculina patagonica in summer and winter. Appl. Environ. Microbiol. 72, 5254–5259. doi: 10.1128/AEM.00554-06
Krediet, C. J., Ritchie, K. B., Paul, V. J., and Teplitski, M. (2013). Coral-associated microorganisms and their roles in promoting coral health and thwarting diseases. Proc. R. Soc. B. 280, 2012–2328. doi: 10.1098/rspb.2012.2328
Kumari, P., Poddar, A., and Das, S. K. (2014). Marinomonas fungiae sp. nov., isolated from the coral Fungia echinata from the Andaman Sea. Int. J. Syst. Evol. Microbiol. 64, 487–494. doi: 10.1099/ijs.0.054809-0
Lampert, Y., Kelman, D., Dubinsky, Z., Nitzan, Y., and Hill, R. T. (2006). Diversity of culturable bacteria in the mucus of the Red Sea coral Fungia scutaria. FEMS Microbiol. Ecol. 58, 99–108. doi: 10.1111/j.1574-6941.2006.00136.x
Lampert, Y., Kelman, D., Nitzan, Y., Dubinsky, Z., Behar, A., and Hill, R. T. (2008). Phylogenetic diversity of bacteria associated with the mucus of Red Sea corals. FEMS Microbiol. Ecol. 64, 187–198. doi: 10.1111/j.1574-6941.2008.00458.x
Lepcha, R. T., Poddar, A., Schumann, P., and Das, S. K. (2015). Comparative 16S rRNA signatures and multilocus sequence analysis for the genus Salinicola and description of Salinicola acroporae sp. nov., isolated from coral Acropora digitifera. Antonie Van Leeuwenhoek 108, 59–73. doi: 10.1007/s10482-015-0464-9
Lesser, M., Falcón, L. I., Rodríguez-Román, A., Enríquez, S., Hoegh-Guldberg, O., and Iglesias-Prieto, R. (2007). Nitrogen fixation by symbiotic cyanobacteria provides a source of nitrogen for the scleractinian coral Montastraea cavernosa. Mar. Ecol. Prog. Ser. 346, 143–152. doi: 10.3354/meps07008
Littman, R. A., Bourne, D. G., and Willis, B. L. (2010). Responses of coral-associated bacterial communities to heat stress differ with Symbiodinium type on the same coral host. Mol. Ecol. 19, 1978–1990. doi: 10.1111/j.1365-294X.2010.04620.x
Littman, R. A., Willis, B., Pfeffer, C., and Bourne, D. (2009). Diversity of coral-associated bacteria differs with location but not species for three acroporids on the Great Barrier Reef. FEMS Microbiol. Ecol. 68, 152–163. doi: 10.1111/j.1574-6941.2009.00666.x
Littman, R. A., Willis, B. L., and Bourne, D. G. (2011). Metagenomic analysis of the coral holobiont during a natural bleaching event on the Great Barrier Reef. Environ. Microbiol. Rep. 3, 651–660. doi: 10.1111/j.1758-2229.2010.00234.x
López-García, P., López-López, A., Moreira, D., and Rodríguez-Valera, F. (2001). Diversity of free-living prokaryotes from a deep-sea site at the Antarctic Polar Front. FEMS Microbiol. Ecol. 36, 193–202. doi: 10.1016/S0168-6496(01)00133-7
McKew, B. A., Dumbrell, A. J., Daud, S. D., Hepburn, L., Thorpe, E., Mogensen, L., et al. (2012). Characterization of geographically distinct bacterial communities associated with coral mucus produced by Acropora spp. and Porites spp. Appl. Environ. Microbiol. 78, 5229–5237. doi: 10.1128/AEM.07764-11
Meyer, F., Paarmann, D., D’Souza, M., Olson, R., Glass, E. M., Kubal, M., et al. (2008). The Metagenomics RAST server - A public resource for the automatic phylogenetic and functional analysis of metagenomes. BMC Bioinformatics 9:386. doi: 10.1186/1471-2105-9-386
Morrow, K. M., Moss, A. G., Chadwick, N. E., and Liles, M. R. (2012). Bacterial associates of two Caribbean coral species reveal species-specific distribution and geographic variability. Appl. Environ. Microbiol. 78, 6438–6449. doi: 10.1128/AEM.01162-12
Ngugi, D. K., Antunes, A., Brune, A., and Stingl, U. (2012). Biogeography of pelagic bacterioplankton across an antagonistic temperature–salinity gradient in the Red Sea. Mol. Ecol. 21, 388–405. doi: 10.1111/j.1365-294X.2011.05378.x
Nithyanand, P., and Pandian, S. K. (2009). Phylogenetic characterization of culturable bacterial diversity associated with the mucus and tissue of the coral Acropora digitifera from the Gulf of Mannar. FEMS Microbiol. Ecol. 69, 384–394. doi: 10.1111/j.1574-6941.2009.00723.x
Niu, B., Fu, L., Sun, S., and Li, W. (2010). Artificial and natural duplicates in pyrosequencing reads of metagenomic data. BMC Bioinformatics 11:187. doi: 10.1186/1471-2105-11-187
Olson, N. D., Ainsworth, T. D., Gates, R. D., and Takabayashi, M. (2009). Diazotrophic bacteria associated with Hawaiian Montipora corals: diversity and abundance in correlation with symbiotic dinoflagellates. J. Exp. Mar. Bio. Ecol. 371, 140–146. doi: 10.1016/j.jembe.2009.01.012
Parks, D. H., and Beiko, R. G. (2010). Identifying biologically relevant differences between metagenomic communities. Bioinformatics 26, 715–721. doi: 10.1093/bioinformatics/btq041
Parks, D. H., Tyson, G. W., Hugenholtz, P., and Beiko, R. G. (2014). STAMP: Statistical analysis of taxonomic and functional profiles. Bioinformatics 30, 3123–3124. doi: 10.1093/bioinformatics/btu494
Ritchie, K. B. (2006). Regulation of marine microbes by coral mucus and mucus associated bacteria. Mar. Ecol. Prog. Ser. 322, 1–14. doi: 10.3354/meps322001
Roder, C., Bayer, T., Aranda, M., Kruse, M., and Voolstra, C. R. (2015). Microbiome structure of the fungid coral Ctenactis echinata aligns with environmental differences. Mol. Ecol. 24, 3501–3511. doi: 10.1111/mec.13251
Rodriguez-Brito, B., Rohwer, F., and Edwards, R. A. (2006). An application of statistics to comparative metagenomics. BMC Bioinformatics 7:162. doi: 10.1186/1471-2105-7-162
Rohwer, F., Seguritan, V., Azam, F., and Knowlton, N. (2002). Diversity and distribution of coral-associated bacteria. Mar. Ecol. Prog. Ser. 243, 1–10. doi: 10.3354/meps243001
Rosenberg, E., Koren, O., Reshef, L., Efrony, R., and Rosenberg, I. Z. (2007). The role of microorganisms in coral health, disease and evolution. Nat. Rev. Microbiol. 355, 349–360.
Schirmer, A., Gadkari, R., Reeves, C. D., Ibrahim, F., DeLong, E. F., and Hutchinson, C. R. (2005). Metagenomic analysis reveals diverse polyketide synthese gene clusters in microorganisms associated with the marine sponge Discodermia dissoluta. Appl. Environ. Microbiol. 71, 4840–4849. doi: 10.1128/AEM.71.8.4840-4849.2005
Simon, C., and Daniel, R. (2011). Metagenomic analyses: past and future trends. Appl. Environ. Microbiol. 77, 1153–1161. doi: 10.1128/AEM.02345-10
Sommer, M. O., Dantas, G., and Church, G. M. (2009). Functional characterization of the antibiotic resistance reservoir in the human microflora. Science 325, 1128–1131. doi: 10.1126/science.1176950
Sunagawa, S., DeSantis, T. Z., Piceno, Y. M., Brodie, E. L., DeSalvo, M. K., Voolstra, C. R., et al. (2009). Bacterial diversity and white plague disease associated community changes in the Caribbean coral Montastraea faveolata. ISME J. 3, 512–521. doi: 10.1038/ismej.2008.131
Tout, J., Jeffries, T. C., Webster, N. S., Stocker, R., Ralph, P. J., and Seymour, J. R. (2014). Variability in microbial community composition and function between different niches within a coral reef. Microb. Ecol. 67, 540–552. doi: 10.1007/s00248-013-0362-5
Tremblay, P., Weinbauer, M. G., Rottier, C., Guerardel, Y., Nozais, C., and Ferrier-Pages, C. (2011). Mucus composition and bacterial communities associated with the tissue and skeleton of three scleractinian corals maintained under culture conditions. J. Mar. Biolog. Assoc. 91, 649–657. doi: 10.1017/S002531541000130X
Tringe, S. G., von Mering, C., Kobayashi, A., Salamov, A. A., Chen, K., Chang, H. W., et al. (2005). Comparative metagenomics of microbial communities. Science 308, 554–557. doi: 10.1126/science.1107851
Tyson, G. W., Chapman, J., Hugenholtz, P., Allen, E. E., Ram, R. J., Richardson, P. M., et al. (2004). Community structure and metabolism through reconstruction of microbial genomes from the environment. Nature 428, 37–43. doi: 10.1038/nature02340
Vega Thurber, R., Willner-Hall, D., Rodriguez-Mueller, B., Desnues, C., Edwards, R. A., Angly, F., et al. (2009). Metagenomic analysis of stressed coral holobionts. Environ. Microbiol. 11, 2148–2163. doi: 10.1111/j.1462-2920.2009.01935.x
Wegley, L., Edwards, R., Rodriguez-Brito, B., Liu, H., and Rohwer, F. (2007). Metagenomic analysis of the microbial community associated with the coral Porites astreoides. Environ. Microbiol. 9, 2707–2719. doi: 10.1111/j.1462-2920.2007.01383.x
Wells, M. L., Price, N. M., and Bruland, K. W. (1994). Iron chemistry in seawater nd its relationship to phytoplankton: a workshop report. Mar. Chem. 14, 157–182.
Wild, C., Huettel, M., Klueter, A., Kremb, S. G., Rasheed, M. Y. M., and Jørgensen, B. B. (2004). Coral mucus functions as an energy carrier and particle trap in the reef ecosystem. Nature 428, 66–70. doi: 10.1038/nature02344
Wild, C., Rasheed, M., Jantzen, C., Cook, P., Struck, U., Huettel, M., et al. (2005). Benthic metabolism and degradation of natural particulate organic matter in carbonate and silicate reef sands of the northern Red Sea. Mar. Ecol. Prog. Ser. 298, 69–78. doi: 10.3354/meps298069
Williams, K. P., Gillespie, J. J., Sobral, B. W., Nordberg, E. K., Snyder, E. E., Shallom, J. M., et al. (2010). Phylogeny of gammaproteobacteria. J. Bacteriol. 192, 2305–2314. doi: 10.1128/JB.01480-09
Wu, S., Zhu, Z., Fu, L., Niu, B., and Li, W. (2011). WebMGA: a customizable web server for fast metagenomic sequence analysis. BMC Genomics 12:444. doi: 10.1186/1471-2164-12-444
Keywords: coral, Andaman Sea, metagenome, microbial composition, functional analysis
Citation: Badhai J, Ghosh TS and Das SK (2016) Composition and Functional Characterization of Microbiome Associated with Mucus of the Coral Fungia echinata Collected from Andaman Sea. Front. Microbiol. 7:936. doi: 10.3389/fmicb.2016.00936
Received: 15 February 2016; Accepted: 31 May 2016;
Published: 16 June 2016.
Edited by:
Senjie Lin, University of Connecticut, USAReviewed by:
Ariel-Kushmaro, Ben-Gurion University of the Negev, IsraelWei Xie, Tongji University, China
Copyright © 2016 Badhai, Ghosh and Das. This is an open-access article distributed under the terms of the Creative Commons Attribution License (CC BY). The use, distribution or reproduction in other forums is permitted, provided the original author(s) or licensor are credited and that the original publication in this journal is cited, in accordance with accepted academic practice. No use, distribution or reproduction is permitted which does not comply with these terms.
*Correspondence: Subrata K. Das, subratkdas@hotmail.com; subrata@ils.res.in