- Laboratory of Biochemistry, Proteomics and Metabolic Engineering of Prokaryotes, Department of Life Sciences and Systems Biology, University of Torino, Torino, Italy
Lactic acid bacteria (LAB) can produce a huge amount of bioactive compounds. Since their elective habitat is food, especially dairy but also vegetal food, it is frequent to find bioactive molecules in fermented products. Sometimes these compounds can have adverse effects on human health such as biogenic amines (tyramine and histamine), causing allergies, hypertensive crises, and headache. However, some LAB products also display benefits for the consumers. In the present review article, the main nitrogen compounds produced by LAB are considered. Besides biogenic amines derived from the amino acids tyrosine, histidine, phenylalanine, lysine, ornithine, and glutamate by decarboxylation, interesting peptides can be decrypted by the proteolytic activity of LAB. LAB proteolytic system is very efficient in releasing encrypted molecules from several proteins present in different food matrices. Alpha and beta-caseins, albumin and globulin from milk and dairy products, rubisco from spinach, beta-conglycinin from soy and gluten from cereals constitute a good source of important bioactive compounds. These encrypted peptides are able to control nutrition (mineral absorption and oxidative stress protection), metabolism (blood glucose and cholesterol lowering) cardiovascular function (antithrombotic and hypotensive action), infection (microbial inhibition and immunomodulation) and gut-brain axis (opioids and anti-opioids controlling mood and food intake). Very recent results underline the role of food-encrypted peptides in protein folding (chaperone-like molecules) as well as in cell cycle and apoptosis control, suggesting new and positive aspects of fermented food, still unexplored. In this context, the detailed (transcriptomic, proteomic, and metabolomic) characterization of LAB of food interest (as starters, biocontrol agents, nutraceuticals, and probiotics) can supply a solid evidence-based science to support beneficial effects and it is a promising approach as well to obtain functional food. The detailed knowledge of the modulation of human physiology, exploiting the health-promoting properties of fermented food, is an open field of investigation that will constitute the next challenge.
Introduction
Human health is the result of a correct physiological status often resulting from the reciprocal interaction of gene-derived signals (genetics) and environment–generated information (epigenetics). Recognizing gene signals is relatively easy whereas environmental stimuli are often multiple, complex, and reciprocally interacting. Temperature, pH, redox balance, sleep, diet, drugs, and psychological status can deeply affect gene expression, metabolic pathways, and homeostasis (Carey, 2012). However, human genes and environment are not the only players: microorganisms can take part to the complex molecular cross-talk existing between external world and “self.”
Firstly, endogenous symbiont microorganisms, the so-called microbiota, can modulate gene expression, induce preferential food intake (Aydin, 2007), influence pH, redox balance and the ratio between pro-inflammatory and anti-inflammatory cytokines (Belkaid and Hand, 2014). Briefly, control brain, metabolism, immune system, and several homeostatic routes.
Secondarily, food-derived bacteria and yeasts can exogenously affect nutritional parameters, metabolism, oxidative status, immunity, blood pressure, appetite, behavior, also controlling the endogenous microbiota (Arora et al., 2013) by altering the ratio among saccharolytic and proteolytic species, by modulating symbionts gene expression and several other functions (O’Flaerty, 2014). Hence, all fermented food, containing living organisms, can contribute to modulation of the host physiological balance and it constitutes an opportunity to enrich the diet with new bioactive molecules finally resulting in phenotypic effects (appetite, cholesterol and blood pressure lowering, improvement of mood, antioxidant, and immune defenses) on humans (Pessione, 2010). Actually, all types of modulations occur via a complex network of signals, among which proteinaceous compounds play a crucial role.
Microorganisms are able to synthesize a large number of metabolites with assessed beneficial or detrimental properties for human health. Among these, nitrogen-bearing molecules such as amino acids, amino acid derivatives and oligopeptides have received great attention since they can affect human physiology in multiple ways.
As an example, amino acid derivatives such as selenocysteines and selenomethionines, have recently been reported to be biosynthesized in both Lactobacilli (Lamberti et al., 2011) and yeasts (Porto et al., 2015). Although selenoaminoacids are not true “bioactive compounds,” directly stimulating receptors on human cells, they can trigger effects deeply affecting human health. The bioactive role of seleno-fixing microorganisms lies in the fact that diet-derived inorganic selenium is toxic (selenate and selenite) or poorly active (elemental selenium) whereas fixed selenium forms (selenomethionines and selenocysteines) are the only bioavailable for humans. On the other hand, only bacteria and yeasts can produce seleno-amino acids from inorganic selenium. Once properly inserted into selenoproteins (i.e., glutathione peroxidase), they can counteract oxidative stress. Besides this well-known antioxidant function, there are data indicating that selenoproteins can modulate immune system (Huang et al., 2012) and activating anabolic circuits such as thyroid hormone biosynthesis (Mullur et al., 2014). Furthermore, epidemiological studies show an inverse correlation between selenium level in blood and cancer mortality, and laboratory experiments have shown a selenium protective effect against cancer initiation and development (Gromadzińska et al., 2008). In Lactobacillus reuteri exoproteome studies have demonstrated that two secreted proteins (GAPDH and Phosphoketolase) contain selenocysteines opening the way to employ this strain to supply organic bioavailable forms of selenium (Galano et al., 2013; Mangiapane et al., 2014a,b).
Among amino acid derivatives found in food, biogenic amines are worth of a special mention. Such compounds, although sometimes naturally present (especially in vegetal food) are often the result of the bacterial decarboxylative activity on free amino acids in food. Biogenic amines can be present in non-fermented food, like fish, due to spoilage bacteria that during protein putrefaction release free amino acids undergoing decarboxylation. E. coli can produce cadaverine from lysine and putrescine from ornithine (Applebaum et al., 1975). Proteus can produce putrescine from ornithine as a communication signal (Visick and Fuqua, 2005). However, also not-spoiled food, such as fermented food, can present the risk of biogenic amine accumulation. Although starters, exogenously added to perform controlled fermentations, are accurately typed to avoid any risk, autochthonous or contaminant lactic acid bacteria (LAB) can contribute to amine release. LAB are strong amine producers since they use this metabolic pathway (at the place of respiration) to both create a proton gradient and hence energy (for exhaustive review, see Pessione et al., 2010) and to alkalinize the environment, very acidic since their main fermentation products are acids (lactic acid for homofermenter LAB and lactic + formic + acetic acid in heterofermenters).
Many experimental evidences demonstrate that some LAB strains also produce anti-hypertensive, anti-thrombotic, cholesterol-lowering, metal-chelating, antimicrobial, anti-oxidant, immune-modulating, chaperone-like and opioid/opioid antagonist peptides from food proteins (Pessione, 2012), and they can modulate the concentration of opioid and cannabinoid receptors in the gut epithelium (Hayes et al., 2007b). The next sections will focus on some of the referred compounds and will illustrate the main effects exerted on human health.
Encrypted Bioactive Peptides
Several bioactive peptides, lacking activity when protein-encrypted but acquiring their biological effects when proteolytically released, have been shown to have health-promoting properties as anti-microbials, hypocholesterolemics, opioid and opioid antagonists, angiotensin-converting enzyme inhibitors, anti-thrombotics, immunomodulators, cytomodulators, and anti-oxidants (Hayes et al., 2007b).
The human pool of digestive proteases and peptidases can liberate food-encrypted bioactive peptides that can be absorbed by the gut and then reach peripheral organs. However, the enzymatic activity of LAB largely contribute to their release, either into the food matrix (starter or autochthonous LAB) or in the gut (endogenous microbiota or probiotics). LAB are ancient organisms adapted to an anoxic environment that never evolved the capability to biosynthesize heme and hence to have functional cytochromes, catalases, and peroxidases. They are very sensitive to oxygen and devote most of their genes to oxygen stress counteracting. Due to the limited length of the overall genome, the biosynthetic abilities of LAB are very limited especially in amino acid synthesis (Pessione, 2012). Therefore, LAB evolved a complex and sophisticated proteolytic system allowing them to get amino acids from the proteins present in the external environment. A schematic representation of this system, which includes proteases, peptidases and membrane transporters, is referred in Figure 1.
LAB Proteolytic System
Extracellular protein hydrolysis into various long oligopeptides is initiated by a cell-envelope proteinase (CEP). Oligopeptides generated by this first cleavage are subsequently taken up by cells via specific transport systems and they undergo further degradation into shorter peptides (bioactive or possible precursors of bioactive compounds) and amino acids in the cytoplasm (Savijoki et al., 2006). In LAB the oligopeptide transporter system (Opp) is the main transporter, belonging to a superfamily of highly conserved ATP-binding cassette transporters (Doeven et al., 2005). The Opp system of L. lactis transports peptides up to at least 18 residues (Savijoki et al., 2006). It should be underlined that most bioactive peptides are released into the external environment only when cells undergo autolysis, because only occasionally the longer peptides (originated by the first hydrolytic step) possess biological activity (Meisel and Bockelmann, 1999). Nevertheless, some authors reported that CEP from Lactobacillus delbrueckii subsp. lactis are suitable to liberate both metal-chelating and anti-hypertensive peptides directly from caseins and without necessity of prior cell autolysis. Maximum CEP activity was a little bit decreased by the addition of NaCl (1%) and glycerol (5%; Hébert et al., 2008). However, proteolytic enzymes released by LAB proved to be very different in the different LAB species and strains, giving rise to a different pool of bioactive peptides (Hébert et al., 1999).
Food Matrices Containing Encrypted Peptides
Lactic acid bacteria proteolytic system is suitable to produce bioactive peptides from several food proteins, especially caseins that constitute the main nitrogen substrate present in their habitat (milk and milk derivatives; Clare and Swaisgood, 2000). Casein consists of four main proteins (whose ratio is about 38:11:38:13): alpha s1 casein, alpha s2 casein, beta-casein and k-casein, differing in amino acid sequence, hydropathicity index, glycosylation and phosphorylation degree (Pessione, 2012). Usually, CEPs have a strong preference for hydrophobic caseins and Lactococcus CEPs have been classified into several types and subtypes depending on their substrate specificity for αS1-, β-, and κ-caseins (Kunji et al., 1996). Casein hydrolysis can give rise mainly to opioid/anti-opioid peptides, antithrombotic and antihypertensive, immunomodulatory, mineral-binding and antimicrobial peptides (Thakur et al., 2012) easily detectable by means of chromatographic techniques after microbial food digestion (Saraswat et al., 2012).
Hydrolytic cleavage of milk whey proteins (alpha-lacto-albumine, beta-lacto-globulin, lactoferrin, and immunoglobulins) can also generate bioactive molecules, such as hypocholesterolemic peptides, which can decrease absorption and enhance fecal extrusion of cholesterol. Actually, milk whey proteins display a greater cholesterol-lowering activity than casein, in particular beta-lactoglobulin (Nagaoka et al., 2001). Beta-lactoglobulin-released peptides also exhibit antioxidant (Hernández-Ledesma et al., 2005) and immune-modulating (Prioult et al., 2004) activity.
Bio-active molecules, especially ACE-inhibiting peptides, can also be found encrypted in bovine meat proteins, such as hemoglobin (i.e., hemorphins) and serum albumin (i.e., serorphin), collagen, elastine, fibrinogen (Minkiewicz et al., 2011; Lafarga et al., 2015).
However, also food of vegetal origin can be a source of bioactive peptides. In soy β-conglycinin β-subunit (Kaneko et al., 2010) and in the large subunit of spinach rubisco, both antihypertensive (Yang Y. et al., 2003) and opioid peptides (i.e., soymorphins-5,-6, and -7, and rubiscolin-5 and -6, respectively), displaying anxiolytic effects, food intake controlling action and enhancement of memory, have been described (Yang et al., 2001; Yang S. et al., 2003; Hirata et al., 2007).
A special mention needs gluten: opioid peptides (i.e., gluten exorphins A4, A5, B4, B5, and C), have been detected and characterized in wheat gluten since 1992 (Fukudome and Yoshikawa, 1992). Some of these peptides have analgesic action on the CNS (Takahashi et al., 2000) but some can also act on opioid receptors located outside the blood–brain barrier, inducing prolactin secretion (Fanciulli et al., 2005). Both antihypertensive (Rizzello et al., 2008) and antioxidant (Coda et al., 2012) peptides are the result of LAB activity on wheat and other cereals. Generally, the high proline content of gluten alpha gliadins prevent hydrolysis by enzymes of the gastrointestinal tract, whereas LAB possess proline-specific peptidase systems. Alternatively, peptide degradation is achieved by the combined action of peptidase from different LAB strains simultaneously present in fermented food (Gerez et al., 2008).
To date, LAB strains able to release bioactive peptides from food proteins, with particular reference to milk caseins, include L. helveticus CP790, L. rhamnosus GG, L. bulgaricus SS1, and L. lactis subsp. cremoris FT4 (Gobbetti et al., 2002). The hydrolytic ability is related to both the protein substrate, i.e., its amino acid sequence and the proteolytic enzyme panel of each microbial strain (Griffiths and Tellez, 2013). It is possible to enhance (100-fold) the proteolytic potential of LAB by growing them in milk (Wakai and Yamamoto, 2012). Actually it has been demonstrated that peptide-rich media like MRS supplying a ready-to-eat nitrogen source are unfavorable to the induction of most proteolytic enzymes for instance those encoded by Pep N and PepX (Smeianov et al., 2007). On the other hand, CEP are able to hydrolyze caseins only after growth on skim milk but not after MRS pre-culture (Jensen et al., 2009). Generally, lactobacilli genome encodes a larger number of proteases, peptidases, amino acid permeases, and Opp transport systems than lactococci (Klaenhammer et al., 2005). However, more studies on a larger number of species and strains are necessary to establish the true potential of each strain.
Finally, it is worth considering that most information on LAB proteolytic system has been referred to food matrix isolated microflora and to its action on bovine milk casein (Korhonen and Pihlanto, 2003). However, endogenous or probiotic or food –derived LAB can also produce bioactive peptides from the different protein pool present in the host gut, opening interesting perspectives for improving human health (Sharon et al., 2014). In the following paragraphs, the best known bioactive molecules generated from LAB proteases and peptidases are described.
Antimicrobial Peptides
Lactic acid bacteria proteolytic activity on caseins gives rise to small peptides displaying antimicrobial (both bactericidal and bacteriostatic) activity. Alpha s1 casein and K-casein hydrolysis gives rise to isracidin and k-casecidin, respectively (McCann et al., 2006). Both peptides display inhibitory action on S. aureus growth (Meisel and Bockelmann, 1999; Atanasova et al., 2014). K-casein can also originate kappacin, a long bioactive peptide active against Streptococcus mutans. This peptide, although deriving from K-casein, is phosphorylated but not glycosylated, and displays the ability to prevent bacterial adhesion to gingival mucosa and to bind enterotoxins (Malkoski et al., 2001). The alpha-s-2 casein fragment 165-203, called casocidin-I can inhibit both S. carnosus and E. coli (Zucht et al., 1995). Lactobacillus helveticus can hydrolyze beta casein, by means of a PR4 proteinase, decrypting an antimicrobial peptide active toward several strains of Gram-positive bacteria (including S. aureus and Listeria innocua) but also against Gram-negative pathogens such as E. coli, Salmonella, Yersinia, (Minervini et al., 2003). Homology studies revealed that this peptide has a similar length to isracidin, but a stronger hydrophobic nature (lacking positive charges) allowing a better activity on Gram-negative bacteria. Furthermore, it bears some proline residues near the C-terminal, which can prolong its half-life rendering this molecule more resistant to the peptidolytic action (Gobbetti et al., 2004). The critical question concerning this peptide is that it has been decrypted from human milk but not from other caseins present in food (cow, buffalo, goat, ship).
Different peptides produced from caseins by different Lactobacillus species (L. acidophilus, L. helveticus, L. plantarum, and L. rhamnosus) and by Lactococcus lactis are active against Gram-negative rods such as Enterobacter sakazakii (Hayes et al., 2006). An interesting antimicrobial peptide, named Lactoferricin B, having broad spectrum of action including Gram-negative and Gram-positive bacteria, yeasts and filamentous fungi, has been described as the result of the hydrolytic action on bovine lactoferrin (Dionysius and Milne, 1997; Kitts and Weiler, 2003). This cationic peptide alters membrane permeability with consequent dissipation of the proton gradient and has therefore bactericidal action (FitzGerald and Murray, 2006). It was cloned and expressed in E. coli, showing activity against Klebsiella pneumoniae, Streptococcus Mutans, and S. aureus (Luo et al., 2007). Lactoferricin B is especially active toward the enterohemorrhagic strain of E. coli 0157h:7 (Muro-Urista et al., 2011) and proved to be active also against wine-spoilage LAB such as Oenococcus oeni, Pediococcus damnosus, and Lactobacillus brevis (Enrique et al., 2009). It is difficult to establish the real LAB potential in hydrolyzing lactoferrin. Some experiments were performed by using both microbial-derived enzymes and fermentation with proteolytic starter cultures (Sharma et al., 2011), however, the true potential of each strain has to be experimentally proved since a great variability was observed among strains (Korhonen, 2009). More recently, the proteolytic activity of different LAB strains on goat milk beta casein and beta-lactoglobulin were tested. Lactococcus lactis l598, Lactobacillus lactis 1043, Streptococcus thermophilus t3D1, Dt1 and Lb. delbrueckii subsp. bulgaricus b38, b122 and b24 revealed a significant potential in producing antimicrobial peptides inhibiting S. aureus, L. monocytogenes, Listeria innocua, Enterobacter aerogenes, and Salmonella enteritidis (Atanasova et al., 2014).
Concerning the mechanism of action of such antibacterial compounds, several models have been proposed. Being amphipathic molecules, bearing a hydrophobic moiety and a strongly positively charged domain, this peptides can firstly bind to teichoic acids in Gram-positive-, or to LPS in Gram-negative bacteria (Vorland, 1999) and then interact with the negatively charged bacterial membranes. Most of them act as proton gradient perturbing molecules, (causing membrane depolarization like polymyxin B and colistin; Lohner and Blondelle, 2005), however, some authors suggest that also removal/chelating of membrane-bound iron can affect bacterial viability (Sharma et al., 2011). For what concern lactoferrin-derived peptides, however, it has long been established (Bellamy et al., 1992) that the domains of lactoferrin involved in the antibacterial activity are different from those involved in iron binding. Complex mechanism, including inhibition of the synthesis of macromolecules (Ulvatne et al., 2004) as well as synergic action with host innate immunity compounds were also reported (Brogden, 2005).
These antimicrobial compounds are appreciated in the food industry (especially dairy industry) as natural preservatives counteracting undesired contamination. This allows to reduce the amount of sugar and salt with excellent benefits for diabetics, obese, and hypertensive subjects. Furthermore, their stability in blood and serum render them promising infection control agents. Some of them are also good candidates to be tested for their anti-viral potential. Bojsen et al. (2007) reported the antiviral effect of bovine whey proteins, whereas Civra et al. (2015) very recently identified from donkey milk lactadherin a peptide displaying anti-Rotavirus activity. The actual role of LAB proteolysis in the decrypting of the antiviral potential is far to be fully elucidated. An overview of antibacterial peptides is proposed in Table 1.
Metal-Binding Peptides
Some LAB strains digest casein, releasing casein phosphopeptides (CPP) phosphorylated on Ser residues. CPP have a strong anionic character and hence are very resistant to further proteolytic degradation (Sharma et al., 2011). They have been identified during cheese ripening due to microbial protease activity (Singh et al., 1997). The typical cluster is Ser(P)-Ser(P)Ser(P)-Glu-Glu, but they may contain also phosphorylated cysteines. Even if this motif is crucial for metal-binding, various phosphopeptide fractions revealed significant differences in this capability, which may be due to variant amino acid composition around the phosphorylated region. Hence, other factors can affect CPP-metal interaction, namely the total number of amino acids and the total negative charge (Cross et al., 2005).
It has long been established (Gagnaire et al., 1996) that beta and k-caseins (being poor in hydroxylated amino acids and therefore less suitable for phosphorylation) generate peptides less active in metal binding, whereas the Ser/Thr rich alpha s1 and alpha s2 caseins are the most suitable for chelating cations by means of CPP. However, alpha s1 casein-derived peptide (59–79), although bearing five phosphate groups, is less active in allowing mineral uptake by the human tumor cells HT29 than beta casein-derived peptides (1–25). Actually, besides the typical cluster sequence, specific secondary structure motifs in the bioactive peptide (such as beta-turn and loop), as well as the degree of aggregation of CPP in presence of divalent cations, are required for a correct mineral absorption (Ferraretto et al., 2003).
Casein phosphopeptides can chelate up to 250 mgCa/g with a dissociation constant in the mM range, with an affinity even higher for trace elements like zinc, iron, and copper (FitzGerald, 1998). Their mechanism of action rely on the fact that they can form soluble complexes with calcium even at alkaline pH (Berrocal et al., 1989). This results in increased absorption of calcium in the intestine, useful in the treatment of osteoporosis. Re-calcification of dental enamel and prevention of dental caries were also reported (Reynolds et al., 2008). Enhanced gut bioavailability and potential higher absorption of enzyme co-factors such as iron, zinc, copper, selenium, magnesium, and manganese, due to CPP was also described (Meisel and Olieman, 1998). On the other hand, controversial clinical results concerning both calcium (Korhonen and Pihlanto, 2006) zinc (Miquel and Farré, 2007) and calcium and iron absorption obtained on patients receiving CPP supplementation and controls, underline the difficulty to standardize the different supplements (Teucher et al., 2006). Finally, calcium availability is strongly dependent on meal composition (i.e., phytates) and complex interactions between different foods ingested.
In spite of all these considerations, all described peptides may constitute interesting tools for microelements nutritional improvement. In our aging western society bone disease is the third cause of nursery care and osteoporosis is not fully controlled by a healthy lifestyle (active movement and diet; Wei et al., 2003). Therefore, calcium-enriching supplements such as CPP open new nutraceutical perspectives. However, the need to use purified and well-characterized CPP (for having a reproducible nutritional impact) highly affects the costs. Hence, the use of LAB as probiotics or food supplements to obtain functional food, especially milk based drinks and yogurts will be a promising strategy. Lactobacillus helveticus LA, is a LAB adapted to the cheese environment displaying good proteolytic activity. It can decrypt from alpha casein a peptide showing calcium-binding activity (Dimitrov, 2009). Lactobacillus helveticus LBK16H can decrypt the tripeptides Ile-Pro-Pro and Val-Pro-Pro, both exerting anabolic effect on bones (Griffiths and Tellez, 2013).
Antioxidant Peptides
The existence of antioxidant peptides in vegetal food (especially cereals and legumes) is a well-established concept, recently reviewed by Malaguti et al. (2014). Marine food, such as algae (Sheih et al., 2009) and squid (Rajapakse et al., 2005b), as well as unusual proteins like royal jelly-derived (Guo et al., 2009), yak milk casein (Mao et al., 2011), goat milk casein (Li et al., 2013), donkey milk (Piovesana et al., 2015), but also egg white lysozyme (Rao et al., 2012), can be a source of antioxidant peptides. Bacteria have the capability to decrypt antioxidant peptides from several food proteins. Antioxidant activities were described in fermented mussel sauce (Rajapakse et al., 2005a), in LAB-fermented milk whey (Virtanen et al., 2007), in fermented shrimps (Faithong et al., 2010), during sourdough fermentation of wheat, rye, and kamut flours (Coda et al., 2012) and in peptides derived from bovine casein after 24 h hydrolysis with Bifidobacterium longum (Chang et al., 2013). Antioxidant efficacy was also found in cell-free extracts of Lactobacillus plantarum isolated from traditional Chinese food (tofu and kefir) but it is rather related to pseudo catalase enzymes, exopolysaccharides and lipoteichoic acids than to bioactive peptides (Li et al., 2012). Recently, mixed cultures of LAB and yeast proved to be able to produce antioxidant peptides from cow milk, however, the peptide sequences having activity were not described (Li et al., 2015).
Antioxidant peptides can control both enzymatic and non-enzymatic peroxidation of fatty acids thus preventing membrane lipid peroxidation (Hartmann and Meisel, 2007). They can act as direct radical scavengers, non-radical oxygen quenchers or metal chelators, but they also can eliminate radical precursors, hence controlling ROS damage to cells and tissues, resulting in a beneficial action on the whole organism. The redox balance between ROS and antioxidant endogenous defenses is highly impaired during chronic inflammation (Zhang et al., 2015) thus, antioxidants are essential in controlling age-related chronic degenerative diseases concerning both the cardiovascular and the central nervous system.
Natural antioxidant peptides such as GSH (Cys-Glu-Gly) or carnosine (beta-Ala-His) play important roles in radical scavenging (Power et al., 2013). Antioxidant properties have been demonstrated for Tyr, Trp, His, and Lys, in particular when Tyr and Trp are in the C-terminus and the hydrophobic residues Val and Leu at the N-terminus of a peptide. Trp and Tyr behave as antioxidant molecules since their indolic and phenolic groups can act as hydrogen donors (Hernández-Ledesma et al., 2008). Basic amino acids can chelate metallic ions and cysteine acts as a proton donor, thanks to its thiolic group but the highest antioxidant activity was demonstrated for the tripeptide Pro, His, His (Malaguti et al., 2014). Furthermore it was demonstrated that Leu-His-His and Pro-His-His can both act synergically with non-peptide antioxidants such as butylated hydroxyanisole (Kitts and Weiler, 2003).
Immunomodulatory Peptides
It is well established that some milk proteins (caseins, whey proteins, lactoferrin, lactoperoxidase) are able to control lymphocyte proliferation (Möller et al., 2008). Milk-derived peptides such as the C-terminus sequence of beta casein (193–209) can increase proliferation of rat lymphocytes (Meisel and Bockelmann, 1999), whereas alpha casein C-terminal hexapeptide (194–199) and beta casein fragments (63–68 and 91–93) induce macrophage maturation and phagocytosis enhancement in vitro (Fiat and Jollès, 1989). In agreement with these data, studies in vivo show that alpha casein-derived peptides can stimulate macrophages phagocytosis exerting a protective effect against Klebsiella pneumoniae infection in mice (Migliore-Samour et al., 1989). Short-chain peptides from milk whey proteins also stimulate the proliferation of murine spleen lymphocytes in vitro (Mercier et al., 2004). More detailed studies report that proliferation of human peripheral blood lymphocytes is induced by the k-casein and alpha lactalbumin fragment Tyr-Gly-Gly (Kayser and Meisel, 1996; Sharma et al., 2011). Natural killer (NK) cell activity and antibody synthesis can also be enhanced by food–derived bioactive peptides (Hartmann and Meisel, 2007). However, milk-derived peptides do not act only as simply stimulators of the immune system: immune-modulating activities including cytokines regulation and attenuation of allergic reactions has also been described (Korhonen and Pihlanto, 2003; FitzGerald and Murray, 2006).
Transferring clinical data to food is not so simple. Actually, in this scenario, LAB (especially probiotic LAB) play a very central role: beta-casein medium fermented with LAB gives rise to bioactive peptides acting on monocytes, macrophages and T helper cells, particularly with Th1-like cells (Laffineur et al., 1996). Lactobacillus paracasei hydrolyzes beta-lactoglobulin originating peptides stimulating Interleukin 10 (IL-10) production and downregulation of IL-4 and gamma interferon secretion (Prioult et al., 2004). Casein hydrolysated by Lactobacillus rhamnosus GG displays modulating effects (both stimulation and suppression) on lymphocyte proliferation (Möller et al., 2008). Furthermore, casein hydrolysates from Lactobacillus rhamnosus GG can induce enhancement of anti-inflammatory cytokines from Th1 lymphocytes and a parallel decrease of pro-inflammatory cytokines and immunoglobulins produced by Th2 lymphocytes, thus controlling allergic reactions (Delcenserie et al., 2008). Lactobacillus helveticus proteolysis products down-regulate cytokines production and stimulate macrophage phagocytosis (Matar et al., 2001) and also show protective effects against E. coli O157 (LeBlanc et al., 2004) and Salmonella typhimurium (Tellez et al., 2011) infection. All the non-proteolytic strains of Lactobacillus helveticus failed to trigger immune system modulation. Three immune-active peptides derived both from beta casein and from alpha lactalbumin after milk fermentation with proteolytic strains of Lactobacillus helveticus were analyzed: they contain high percentage of proline histidine and lysine (Tellez et al., 2010). Establishing how, when and in what extent proteolysis occurs in food (and in the gut) is still an open question.
Cell Cycle and Apoptosis Modulating Peptides
Milk-derived peptides, sometimes resulting from microbial proteolytic activity (Roy et al., 1999), can regulate cell growth, differentiation, and apoptosis (Roy et al., 2002). De Simone et al. (2008) demonstrated that waste milk whey is able to induce apoptosis in CaCo2 cancer cells, but also interfere with the cell cycle (reducing the number of cells in S-phase and enhancing the number of cells in G1 phase), thus inhibiting proliferation. Cooked milk whey loose this property probably due to irreversible denaturation of proteases decrypting the peptides of interest. The endogenous microflora of milk (both starters and contaminating bacteria) seems to be the main responsible of the phenomenon observed, since fresh milk does not exhibit this behavior (De Simone et al., 2008). Although the involved peptides were not characterized, it is very well known that some sequences such as Arg-Gly-Asp-Asp-Asp-Asp-Asp-Asp-Asp-Asp-Asp can have anti-proliferative effects on cancer cells models: the triplet Arg-Gly-Asp can account for adhesion to the extracellular matrix whereas the eight Asp residues can act intracellularly by binding chromatin (Galvez and de Lumen, 1999). Meisel and FitzGerald (2003) also demonstrated that normal cells are less sensitive to the apoptotic induction than malignant cells. Tumor cells apoptosis was also detected in an in vivo model in which mice were treated with milk that underwent fermentation by a highly proteolytic strain of Lactobacillus helveticus. On the contrary, the use of low-proteolytic strains did not gave satisfactory results in terms of breast cancer control in the same mice (De LeBlanc et al., 2005). On the other hand, not all anticancer effects of milk are related to proteolysis. It has been demonstrated that alpha lactalbumin can form a complex with oleic acid in acid conditions. This modified protein can induce apoptosis in cancer cells in vitro (Svensson et al., 2000). Therefore, is it tempting to hypothesize that acidification caused by LAB metabolism can also contribute to these effects, and further experiments proving this will be crucial to confirm such hypothesis and opening new perspectives for cancer control. Taken together all these results suggest that it is possible to increase host defenses against tumor degeneration by using food fermented with LAB.
Opioid and Anti-opioid Peptides
These molecules constitute a promising frontier for treating both stress-related behaviors such as anxiety and depression and season-related mood disorders. They act on the appetite/satiety as well, as demonstrated by Pfluger et al. (2012) who named these molecules “nutropioids.” In general, these peptides are able to control the gut–brain axis at several levels, including gut–brain communication, brain cognitive function, and behavior (Cryan and Dinan, 2012). Their mechanism of action includes stimulation of receptors (K, delta, and mu) present in both the central and the peripheral nervous system and subsequent inhibition on adenylate cyclase activity. Some of these compounds are summarized in Table 2.
The best studied myorelaxant peptides are beta-casomorphins (which act on the mu receptors) and alpha s1 casein-derived peptide (which acts on the delta receptor). Their primary sequences consist of 4–10 amino acids whose N-terminal residue, (essential to trigger biological activity), is Arg in alpha s1 casein-derived peptide and Tyr in beta-casomorphins (Loukas et al., 1983). This difference, together with the presence of six proline residues in beta-casomorphins, can account for the higher resistance to enzymatic digestion of the latter, resulting in enhanced half-life and absorption in human digestive tract. Once in blood, beta-casomorphins can reach receptors in the brain and in the peripheral tissues thus exerting a relaxing action inducing calmness and sleeping (Chabance et al., 1998). Another interesting peptide derived from alpha s1casein is alpha-casozepine, displaying anxiolytic action but not directly interacting with the mu and delta receptors. It has been demonstrated that the biological effect is mediated by activation of serotonin and GABAA receptors, causing release of endogenous serotonin, dopamine, and GABA (Mizushige et al., 2013). Conversely, casoxins peptides (originated from k-casein hydrolysis) behave as opioid-antagonists over both the mu and k-type receptors. Due to this different physiological role, casoxins can be employed to counteract depression (Chiba and Yoshikawa, 1986).
Peptides produced with the contribution of LAB protease system on dairy proteins are named exorphins and casoxins, the former having opioid–like and the latter opioid-antagonist function (Chabance et al., 1998). However, other foods may contain opioid/anti-opioid peptides. It has long been established that wheat gluten is a good source of opioid peptides (Fukudome and Yoshikawa, 1992), and more recently, it has been shown that gluten exorphin B5 can enhance prolactine secretion by acting on receptors present outside the blood brain barrier. In this case, the biological effect is linked to a reduced dopaminergic tone (Fanciulli et al., 2005). Soymorphins are very interesting opioid peptides acting on mu-receptors present in the gut and connected with the serotonin, dopamine, and GABA receptors systems. It was shown that soy-derived opioid peptides act as anorexigenics and also reduce gastrointestinal motility (Kaneko et al., 2010). A delta-opioid peptide called rubiscolin was decrypted from the large subunit of spinach rubisco (Yang et al., 2001). The rubiscolin amino acid sequence is Tyr-Pro-Leu-Asp-Leu-Phe and it displays an analgesic effect besides being involved in memory consolidation (Yang S. et al., 2003). Hirata et al. (2007) also report anxiolytic action of this natural peptide by activation of the sigma1 and D1 dopamine receptors, when delivered orally (100 mg/Kg). The replacement of Leu3 with Ile or Met enhances by a factor four the opioid activity, whereas the replacement of Phe with Val potentiates the opioid activity more than 10-fold. The overall activity of the sequence Tyr-Pro-Met-Asp-Leu-Val is 20 times higher than the natural rubisco derivative (Yang S. et al., 2003).
Antithrombotic Peptides
Blood coagulation occurs through a complex system of proteins undergoing a sequential proteolytic cascade. These proteins include pro-enzymes such as fibrinogen that is proteolytically activated to fibrin by means of thrombin. Bovine k-casein derived oligopeptides (fragment 106–116, 11 amino acids long) named casoplatelins (or thrombin inhibitory peptides), can inhibit the binding of human fibrinogen (gamma chain) on the platelet surface receptor, due to sequence homology between fibrinogen gamma chain and K-casein. Actually, the mechanism involved in milk clotting, defined by interaction of k-casein with chymosin bear a remarkable similarity to the process involved in blood clotting, defined by interaction of fibrinogen with thrombin (Qian et al., 1995). As a result, they prevent aggregation of ADP-activated platelets. Also the glycopeptide present on the N-terminus of k-casein, a smaller molecule called casopiastrin, displays inhibiting activity on fibrinogen binding on the platelet membrane (Ricci-Cabello et al., 2012). Although these molecules are mainly the result of human proteolysis on milk, LAB proteolytic activity on casein can contribute to their release both in food and in vivo. Casoplatelins are present in high amounts in the gut but, after absorption, significant concentrations are still bio-available in blood thus contributing to thrombosis control in humans.
Antihypertensive Peptides
Lactobacillus helveticus, Lactobacillus delbrueckii subsp. Bulgaricus SS1, Lactobacillus delbrueckii subsp. lactis, and Lactococcus lactis subsp. cremoris FT4 can modulate blood pressure by producing angiotensin 1-converting enzyme inhibitory peptides (ACE inhibitors) from milk proteins. ACE is a carboxypeptidase converting angiotensin I (a decapeptide) into angiotensin II (an octapeptide) having a strong vasoconstrictor action (Hartmann and Meisel, 2007). Lactobacillus delbrueckii subsp. lactis hydrolyzes both alpha s1 and beta casein (but not k-casein) by means of a cell envelope proteinase releasing antihypertensive peptides: the proteolytic activity was maximal during the logarithmic growth and addition of NaCl and glycerol prevents correct proteolysis (Hébert et al., 2008). Casein-derived anti-hypertensive peptides are 2–6 amino acids long oligopeptides. Generally, LAB peptidases, by shortening the poly/oligopeptide chain, contribute to enhance the anti ACE potential. Actually the 6 (alpha s1 casokinin-6 = Thre-Thre-Met-Pro-Leu-Trp) and the 5 (alpha s1 casokinin-5 = Phe-Phe-Val-Ala-Pro and betacasokinin = Lys-Val-Leu-Pro-Val) amino acids long oligopeptides are less active than the tripeptides made up of Val-Pro-Pro and Ile-Pro-Pro. A very small dipeptide Tyr-Pro, proved to be effective in blood pressure control as well (Yamamoto et al., 1999). The final active short peptides are resistant to both pH variations and human digestive enzymes thus opening interesting applicative perspectives (Gobbetti et al., 2004).
Cholesterol-Lowering Peptides
Hypocholesterolemic peptides can be originated by beta-lactoglobulin, casein and soy proteins proteolysis (Hori et al., 2001). They can lower the total cholesterol of rats in vivo. Their mechanism of action probably consists in the reduction of the micellar cholesterol solubility or also to an enhanced ability to bind taurocholate (Nagaoka et al., 2002). They overall action prevents cholesterol absorption by CaCo2 cells in vitro and enhances fecal steroid excretion in vivo. The sequences displaying such activities are Ile-Ile-Ala-Glu-Lys, Ala-Leu-Pro-Met-His and Gly-Leu-Asp-Ile-Gln-Lys (Nagaoka et al., 2001).
Glucose-Uptake Stimulating Peptides
It has been demonstrated that dipeptides containing branched chain amino acids (Ile-Ile, Leu Leu, Ile-Leu, Leu-Ile, Ile-Val, Leu-Val, Val-Leu) can enhance glucose uptake in skeletal muscle cells, favoring glycogen synthesis and thus controlling hyperglycemia (Morifuji et al., 2010). Generally, glucose uptake by skeletal muscle cells is induced by exercize-training by means of increased expression or activity of key-signaling proteins (Goodyear and Kahn, 1998). Branched-chain amino acids are the main nitrogen source for skeletal muscles and milk whey proteins are particularly rich in branched chain amino acids (22.3%) as compared to caseins (20.3%), soy proteins (17.5%), or wheat gluten (14.1%; Morifuji et al., 2010). The dipeptide Ile-Leu is the most abundant after milk whey hydrolysis. Therefore, assessing the proteolytic activity of LAB toward milk whey proteins (that constitute natural nitrogen substrates for LAB), is a promising strategy to get nutritional supplements improving health. Proteomic approaches using LC-MS-MS can be a valuable help for identifying small nutritionally relevant peptides and a new discipline, called food peptidomics, is now expanding (Lahrichi et al., 2013).
Chaperone-Like Peptides
A very interesting class of protein-encrypted peptides are those displaying chaperone-mimetic action (Bumagina et al., 2009; Artemova et al., 2010). Synthetic peptides were constructed on the model of bovine hemorphin-6, wheat gluten exorphin C, and spinach rubiscolin-5 (all opioid peptides), and their ability in refolding model proteins was investigated. The target proteins to be refolded were heat-treated or DTT-aggregated carbonic anhydrase, ADH and bovine milk alpha lactoglobulin. The results clearly indicate that the peptides are able to refold damaged proteins thus opening the way to the interesting hypothesis that food proteins, besides their nutritional role, can act as systems involved in quality control of proteins especially during stress (Artemova et al., 2010).
Mixed Function Peptides
Some food encrypted peptides, in case decrypted by LAB proteolytic action, possess mixed function. In Table 3 are reported the peptides displaying two or more biological activities.
As an example the tripeptides Val-Pro-Pro and Ile-Pro-Pro, released from beta and K-casein by Lactobacillus helveticus are immunomodulatory and hypotensive (Hartmann and Meisel, 2007). This effect can be explained by the fact that ACE inhibition favors bradykinin production, and bradykinin plays a crucial role in the inflammation process by stimulating macrophages and increasing lymphokines secretion by lymphocytes (Sharma et al., 2011).
Some immunostimulatory peptides also exhibit antimicrobial features: beta-lactoglobulin-derived peptides can improve phagocytosis but also stimulate the microbial autolytic system. This is not only restricted to bacteria but also to fungi and both naturally autolyzing and non-autolyzing strains are sensitive to this effect (Hernández-Ledesma et al., 2008).
It has long been established that opioid peptides such as casomorphin agonists also decrease cell proliferation by acting on somatostatin receptors (Hatzoglou et al., 1996a,b). Since opioid and somatostatin receptors are present on different cells including central nervous, endocrine and immune system (De Simone et al., 2008) the action of such peptides is more complex than expected.
Several peptides are opioid and hypotensive, especially those derived from bovine milk proteins (casomorphins, lactorphins) and beta-casomorphin 7 is opioid, hypotensive, and immunomodulatory: this last function is targeted on lymphocyte proliferation that is sometimes stimulated and sometimes inhibited depending on the peptide concentration (Meisel and Bockelmann, 1999).
Lactoferricin, the lactoferrin-derived antimicrobial peptide, besides being an antibacterial, antifungal and antiviral molecule, can also control carcinogenesis by means of its anti-inflammatory and immune-modulating properties. This effect seems to be due to a positively charged region of the peptide that is very rich in tryptophan and arginine residues (Sharma et al., 2011)
The reason of these mixed functions lies in the fact that some regions in the primary structure of caseins contain overlapping peptide sequences, exerting different physiological functions (Meisel and Bockelmann, 1999). These domains, highly hydrophobic and rich in proline residues, have been defined as “strategic zones” and are resistant to proteolytic attack (Fiat and Jollès, 1989).
In conclusion, of this paragraph it is important to underline that not all bioactive peptides released by the combined action of LAB proteolytic system and digestive proteases in food and in the gut can be available for humans. Actually, bioactive peptides may be degraded during digestion, may be poorly absorbed and hence reach the target tissues at a concentration lower than the one necessary to exert their biological function. As far as degradation is concerned, supplying bioactive peptides by means of GRAS bacteria (like LAB are) plus protein complements is simpler than encapsulate them and this constitutes therefore a promising strategy to transport the molecules to the intestine, preventing degradation in the upper digestive tract. Efforts have been made also to assess the real in vivo absorption of bioactive peptides: blood levels of antihypertensive peptides have been quantified (by LC ESI triple) after ingestion by human volunteers (van Platerink et al., 2006) but also in vitro studies measuring epithelial translocation across CaCo2 monolayers by means of ESI-LC-MS-MS can supply reasonable information about absorption. One challenging aspect of these approaches is the need of improved “omics” methods aimed to identify low- molecular weight bioactive peptides. MALDI (matrix-assisted laser desorption ionization) TOF-MS has proved to be inadequate because of matrix interference (Saavedra et al., 2013) and matrix-free methods such as NALDI (nanostructure-assisted-laser desorption ionization; Kütt et al., 2011) have been re-proposed.
Bioactive Amines
A number of microorganisms synthesize, as decarboxylation products of precursor amino acids, biogenic amines. In LAB, expression (by transcriptional induction) and/or activation (by catalytic modulation) of amino acid decarboxylation systems are non-essential adaptive responses to energy depletion but also strategies to counteract acid stress (Pessione, 2012).
However, not all biogenic amines are bioactive molecules since most of them only act as spoilage compounds (putrescine, cadaverine) sometimes enhancing the toxicity of true bioactive molecules (Medina et al., 2003). On the contrary, the decarboxylation of certain amino acids, as tyrosine and histidine, can give rise to bioactive molecules (tyramine and histamine) involved in several pathogenic syndromes, more extensively described in the following paragraphs. On the other hand, not all bioactive compounds negatively affect human health: as an example, beta-phenylethylamine derived from phenylalanine or tryptamine originated from tryptophan can exert beneficial actions (such as mood control and appetite/satiety balance regulation) when administered to humans (Shimazu and Miklya, 2004). Similarly, it has been reported that several LAB (L. bulgaricus, L. acidophilus, L. casei, L. plantarum) can synthesize melatonin, a bioactive molecule, deriving from tryptophan decarboxylation and serotonin metabolism, acting on sleep and reproductive behavior but also controlling immunity, inflammation and carcinogenesis (Tan et al., 2014). A particular case is the glutamate product gamma amino butyrate (GABA): this molecule can be naturally present in food but also artificially added to enhance the nutraceutical value of a certain food (GABA-tea, GABA-rice; Abe et al., 1995; Oh, 2003) due to its relaxing action on muscles and to its overall beneficial effects on the nervous system.
Bioamines in Food
Cheese is the food most frequently associated with a too high biogenic amine content. It is very rich in free amino acids due to the desired proteolytic action performed by both bacteria and fungi during “maturation,” when colonized by decarboxylase-positive LAB (either autochthonous or contaminant bacteria) it can be a source of tyramine, histamine, putrescine, and beta-phenylethylamine (Pessione et al., 2009). Tyramine and beta phenylethylamine are particularly abundant in food from animal origin (including fermented sausages) since these matrices are very rich in the precursor amino acids, tyrosine, and phenylalanine, used by animals to synthesize catecholamines. Both tyramine and beta-phenylethylamine are bioactive compounds whose action will be better described in the following paragraphs.
As far as fermented meat products are concerned, tyramine, cadaverine, putrescine, and histamine can be found (Singh et al., 2012). Poor quality processing favoring contamination is the main cause of a too high bio-amine content of meat, however starter strains possessing the capability to form biogenic amines, like Lactobacillus curvatus, have been described as well (Singh et al., 2012). To prevent such a risk, selection of particular starter cultures possessing either amino oxidase activity (Alvarez and Moreno-Arribas, 2014) or bacteriocin synthesis capability (Somda et al., 2011) should be taken into concern to contain the undesired consequences of spontaneous fermentations.
Other fermented foods are alcoholic beverages such as beer, ciders, and wine, that being of vegetal origin, are generally poor of aromatic amino acid precursors (Lehtonen, 1996). At the end of alcoholic fermentation the only amino acid present in wine in significant amount is arginine (2,4 g/L): this can be converted by the arginine decarboxylase into agmatine and then putrescine, or rather be directed to the arginine deiminase pathway (ADI) generating ammonia, ornithine (generating putrescine) and carbamoyl phosphate (Figure 2) (Costantini et al., 2013). Both putrescine and ornithine are not true bioactive compounds even if they can alter the organoleptic properties of wine and enhance the toxicity of other biogenic amines (Costantini et al., 2013). Carbamoyl phosphate can combine with ethanol in wine generating ethyl carbamate a carcinogenic molecule (Jiao et al., 2014).
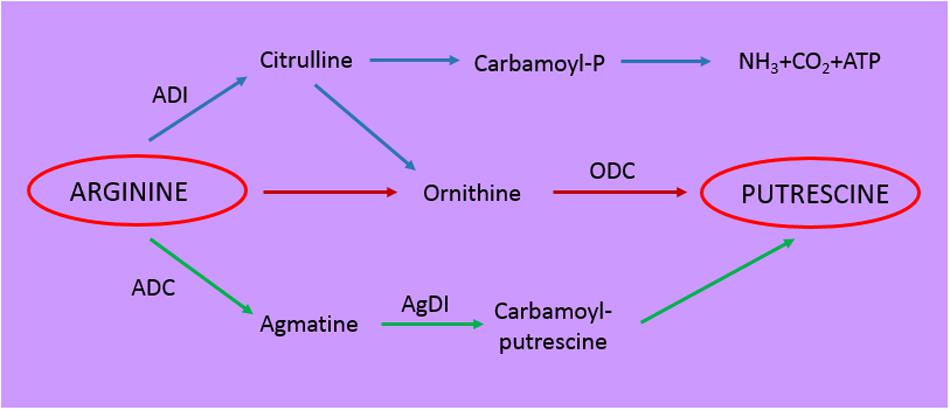
FIGURE 2. Schematic representation of the metabolic pathways involved in putrescine formation. ADI, arginine deiminase; ADC, arginine decarboxylase; AgDI, agmatine deiminase; ODC, ornithine decarboxylase.
However, if the oenological procedures are not correctly performed, other bioactive amines can be generated. Actually, at the end of the alcoholic fermentation when all the grape sugars are exhausted, yeast cells die and undergo autolysis, releasing their own proteins and proteases (from lysosomes). Hence, if dead yeasts cells are not immediately separated from wine, free amino acids (from yeast proteins) become available for autochthonous LAB able to perform malolactic fermentation (MLF; Coton et al., 1999). This secondary fermentation is widely appreciated for its improvement of wine flavor, and it is usually performed by the predominant species Oenococcus oeni or, more occasionally, by other LAB species belonging to the genera Lactobacillus and Pediococcus, which may develop during the winemaking process (Lonvaud-Funel, 1999). Unfortunately, some LAB, besides TPP-dependent-acid decarboxylases, also bear PLP-or pyruvoil-dependent amino acid decarboxylases that can generate undesired amines, depending on the precursor amino acid present at this fermentation stage (Recsei and Snell, 1985). Therefore, histamine, cadaverine, tyramine, and beta-phenylethylamine (whose action will be better described in the following paragraphs) can be produced.
The total content of biogenic amines in food varies from 1 to 50 mg/Kg (alcoholic and no-alcoholic beverages, soy products) to 20–1,500 mg/Kg [sausages, cheeses; EFSA Panel on Biological Hazards (BIOHAZ), 2011] At high concentrations, BA are risk factors for food intoxication, while moderate levels may lead to food intolerance (Ladero et al., 2010) It is very difficult to establish a uniform maximum limit for ingested BA since their toxic effect depends on the type of BA in question. The unique BA for which the European Food Safety Authority (EFSA) has legally set maximum limits is histamine and only related to fish and fish products (Linares et al., 2016). Furthermore, the toxic effects exerted by amines possessing biological activity in humans, strongly depend on individual sensitivity to them (Santos, 1996). As an example, tyramine tolerance is lower in patients treated with antidepressant mono-amino-oxidase inhibitory drugs (IMAO), which interact with amino-oxidase enzymatic systems (MAO, DAO), since these patients have an impaired ability to oxidize amines. For the same reason, the toxic effects of bioactive amines in wine consumers is enhanced by the presence of ethanol, which is one of the most effective inhibitors of amine oxidases (Sessa et al., 1984).
Factors Affecting Amines Production in LAB
When considering the risk of bioactive amine release into food, it is important to underline that maximum energy depletion and acidification occurs during the stationary growth phase. If (theoretically) LAB can be removed from food before resting, no amine accumulation takes place. This statement is supported by proteomic investigations that revealed that, in L. hilgardii, histamine production is induced only during stationary phase (Pessione et al., 2005). On the contrary, northern blotting experiments showed activation of the HDC (histidine decarboxylase) gene already during exponential growth phase in a different L. hilgardii strain (Landete et al., 2008). However, the same authors clearly demonstrated an inhibitory effect of both glucose and fructose (still high during exponential growth phase) over the genes coding for HDC in L. hilgardii, O. oeni, and P. parvulus (Landete et al., 2008). Furthermore, in both L. hilgardii and L. brevis, it has been demonstrated that tyramine accumulation is maximal in presence of either fructose or fructose plus L-malic acid (Moreno-Arribas et al., 2003) thus supporting the idea that it is not necessary to reach the stationary phase to trigger amine accumulation. Actually, also in a different bacterial model (E. faecalis) studies involving sub-proteomes profiling demonstrated a high tyramine content already in the exponential phase when sugars are not yet consumed (experiments performed in chemically defined medium having glucose as sugar carbon source; Pessione et al., 2009). Therefore, the evidences are still controversial and in part related to specific amino acid/amine reaction, in part linked to a strain difference.
A further strong evidence is the fact that amine production is related to the availability of the precursor amino acid. Supplements of tyrosine in the medium can increase about 10-fold tyramine accumulation and about twofold tyrosine decarboxylase (TDC) activity in L. brevis (Moreno-Arribas et al., 2000). Similarly, in Enterococcus faecalis, TDC was found to be more abundant in the membrane extracts when the strain was exposed to free tyrosine (Pessione et al., 2009). Histamine is biosynthesized only in presence of histidine: the cytosolic enzyme HDC was found more abundant in cells grown in histidine medium (stimulated condition) in Lactobacillus hilgardii, and Lactobacillus 30a (Pessione et al., 2005; Mazzoli et al., 2009) as compared to control conditions.
The only exception to this rule seems to be the GAD enzyme that can allow GABA accumulation even in the absence of glutamate. However, the chemically defined-medium medium (CDM) used for growing L. lactis contains glutamine, so it is possible that part of it is converted to glutamate that can undergo decarboxylation to GABA. On the other hand, a transcriptional control exists since addition of glutamate can trigger a light enhance of GABA biosynthesis (Mazzoli et al., 2010).
A critical concern connected with the necessity of free amino acid availability is how bacteria can get them. As far as LAB are concerned, often in the same ecological niche where they live proteins are available (cheese) or microbial autolysis (wine) generates free proteins that can undergo proteolytic breakdown by LAB (Lamberti et al., 2011).
Interestingly, other factors can affect bioactive amine production and, among these, acids (that can undergo or not a similar decarboxylative process). It has been demonstrated that L-lactic acid does not influence both histamine accumulation and HDC biosynthesis (Landete et al., 2008). Similarly, malate has no effect on histamine accumulation (Mangani et al., 2005) or histidine consumption (Mazzoli et al., 2009). In agreement with these data, Moreno-Arribas et al. (2000) showed that tyramine production was not affected by either malate or citrate, whereas Lonvaud-Funel (2001) observed that both tyramine and histamine accumulation was lower during MLF and higher after it. The utilization of the amino acids tyrosine and histidine only after malate does not necessarily indicate malate inhibition, but simply that bacteria prefer utilize carbon sources before consuming nitrogen compounds.
Other factors affecting amine accumulation in food include temperature, spices and salt content, generally controlling microbial growth. The optimum growth for most amine-producing bacteria is 20–37°C. Below this temperature, the risk to find biogenic amines in food decreases (Karovičová and Kohajdová, 2005). The sausage diameter as well as the addition of spices can control a too high amine content in meat: the larger the size, the higher the risk of amines due to higher water activity and lower salt content. However, NaCl not only negatively affects bacterial growth, proteolytic activity and histidine decarboxylase activity but also stimulates both tyrosine and glutamate decarboxylase (GAD) synthesis acting as operon inducer (Singh et al., 2012).
Histamine
The ingestion of histamine-rich food may cause heterogeneous symptoms such as diarrhea, arrhythmia, headache, and allergic syndromes such as respiratory distress (asthma), rhino-conjunctivitis, urticaria, pruritus, and flushing (Manzotti et al., 2016). Histamine is a strong hypotensive compound also acting on many receptors present in the gastro intestinal tract (Santos, 1996). Hence, high content of histamine is responsible of the toxicity of spoiled or not properly stored fish. In black carp fillets, for example, the contents of BAs remained invariable during the early stages of storage and began to increase at the later stages of storage occurs during food storage. In particular, a significantly higher concentration of histamine (132.05 mg/kg on the third day) was detected in the black carp filets stored at 20°C, indicating that this temperature favors the formation of histamine (Hongbing et al., 2016). In wines, especially red wines undergoing spontaneous MLF by LAB, are frequently responsible of these syndromes. Amino acids generating bioactive amines (histidine, tryptophan, glutamate) can be released during the, post-alcoholic fermentation, by yeasts autolysis as referred above (Alexandre et al., 2001). The concentration of free amino acids in wine is also dependent on the proteolytic activity of the strain concerned with MLF (Alexandre et al., 2001).
Tyramine
Tyramine is a bioactive molecule acting both at vascular level (hypertension, vasal-constriction) and on the central nervous system (headache). Despite it is generally assumed that histamine is the most toxic BA, a recent work reveals that unexpectedly, tyramine is more cytotoxic than histamine on an in vitro model of the human intestinal epithelium. Furthermore, the concentrations found to be toxic are commonly reached in BA-rich foods (Linares et al., 2016). Severe syndromes have been described in patients lacking a correct amino-oxidase activity because in treatment with mono-amino-oxidase inhibitory drugs (MAOI). MAOI are used as antidepressant because they prevent the oxidative catabolism of brain-active amines, such as dopamine, serotonin, tryptamine, and β-phenylethylamine. Unfortunately, MAO-inhibitors cannot discriminate among the different MAOs, and their overall effect results in high concentrations of tyramine that can cause hypertensive crises, brain hemorrhage and sometimes death (Pessione et al., 2009). Since tyramine is the biogenic amine most frequently found in cheese (due to the referred high tyrosine content of dairy products) this syndrome is also called “cheese reaction.” For the reasons explained above, MAOI development has led to compounds with improved tolerability profiles compared to the initial irreversible MAOIs, particularly in regard to tyramine-induced hypertension and dietary restrictions (Yanez et al., 2012). The huge number of non-controlled autochthonous bacteria involved in cheese manufacturing and the accidental contamination by unwanted strains during storage render cheese a risky food for tyramine ingestion, although starters are accurately typed. TDC activity has been described in several LAB strains of the genera Lactobacillus, Carnobacterium, and Leuconostoc. The enzyme from Lactobacillus brevis and Enterococcus faecalis has been fully characterized. Proteomic studies reveal the membrane location of such a protein in Enterococcus faecalis (Pessione et al., 2009), thus confirming the high hydrophobic nature of this enzyme (previously predicted on the basis of gene analyses suggesting large hydrophobic domains but also because of the harsh procedures needed to purify it). Interestingly, Enterococcus faecalis TDC proved to be able to produce not only tyramine from tyrosine but also beta-phenylethylamine from phenylalanine (Pessione et al., 2009).
Beta-Phenylethylamine
β-Phenylethylamine, the decarboxylation product of phenylalanine, behaves like an endogenous amphetamine. It can control appetite/satiety thus being useful to treat obesity and related syndromes as well as in weight-control diets. Like amphetamine it can also positively affect mood. However, controversial effects including insomnia, anxiety, and a MAO-blocking activity that may extend the half-life of tyramine thus potentiating its toxicity (Millichap and Yee, 2003) have been described. Evidence of a specific phenylalanine decarboxylase enzyme in bacteria, has been reported only for Bacillus cohnii, a species taxonomically distant from LAB. Nevertheless, both Lactobacilli and Enterococci, frequently found in fermented food, display a clear β-phenylethylamine accumulation. Originally, some authors (Millichap and Yee, 2003) suggested that the β-phenylethylamine found in fermented food is the result of the activity of tyrosine decarboxylating bacteria. It is interesting to underline that in mammals and in several eukaryotic cells models a low-selective aromatic L-amino acid decarboxylase (EC 4.1.1.28) exists. In bacteria, enzymatic studies proved that TDC from E. faecalis is active also toward DOPA, but not toward other amino acids like phenylalanine while Lactobacillus bears a highly tyrosine selective TDC. More recently, the presence in E. faecalis of phenylalanine-decarboxylating TDC (EC 4.1.1.25) has been clearly demonstrated by a bi-phasic kinetic of tyramine and β-phenylethylamine production (Pessione et al., 2009). Tyrosine is consumed first and it is fully converted into tyramine (during logarithmic growth). Then phenylalanine is decarboxylated to β-phenylethylamine, although with lower conversion rate and yield. These results are consistent with a double catalytic activity of the same protein (TDC) having higher specificity for tyrosine and only low affinity for phenylalanine. On the other hand, Marcobal et al. (2006) observed β-phenylethylamine accumulation in a recombinant E. coli expressing the TDC gene of E. faecium.
GABA
γ-Aminobutyric acid is one of the bioactive amines having true positive effects on human health. It acts as a neurotransmitter in the central nervous system of most vertebrates and plays additional roles on the overall human physiology such as lowering the blood pressure in mild hypertensive patients and acting as smooth muscles relaxation system (Mazzoli et al., 2010). Bacteria can produce GABA by the decarboxylation of glutamate through GAD. Glutamate and GABA act as opposite molecules having excitatory and inhibitory roles, respectively (Hyland and Cryan, 2010). Lactococcus lactis NCDO 2118 can obtain GABA from glutamine opportunely converted into glutamate (Mazzoli et al., 2010). It has long been established that L. lactis possess only one gene encoding GAD and that this enzymes is highly specific for glutamate (apparent Km 0.51 mM). Its molecular weight is about 54 kD and it has maximal activity at pH 4.7, whereas no activity is present at neutral pH (Nomura et al., 1999). Differences have been reported between Lactococcus lactis subsp. lactis and L. lactis subsp. cremoris: both possess gadCB genes encoding for GAD, however, one-base deletion of adenine and one-base insertion of thymine were detected in the coding region of the latter rendering it unable to synthesize a functional GAD enzyme (Nomura et al., 2000). Lactobacilli are the best GABA-producers, however, Lactococci, Streptococci, and Bifidobacteria can synthesize GABA as well (Lyte, 2011). Several strategies have been proposed to obtain high GABA amounts from cheap substrates through LAB sourdough fermentation (Coda et al., 2012), batch fermentation using immobilized (Huang et al., 2007) or entrapped (Choi et al., 2006) Lactobacillus brevis cells for the construction of functional food. To achieve maximum GABA production, temperature and pH are crucial (Dhakal et al., 2012). Nowadays, several nutraceutical preparations, such as GABA tea (Abe et al., 1995), GABA rice (Oh, 2003), GABA soymilk (Tsai et al., 2006), and GABA chocolate (Nakamura et al., 2009) are available, especially in Asia. GABA natural content of cacao is about 0.009%, however, this value is lower when considering the market-available chocolate also containing milk, sugar, and other ingredients. Functionalized chocolate, containing 0.28% GABA, proved to be able to lower the level of salivary chromogranin A and cortisol, thus reducing psychological stress (Nakamura et al., 2009).
Conclusion
The knowledge on how, when and where bioactive amines are produced by LAB present in fermented food will be helpful by one side to prevent food borne diseases but on the other side also to use food as a nutraceutical delivery system to control mood, appetite and smooth muscle relaxation.
The knowledge about food-encrypted peptides and their potential can offer, in the coming decades, new tools for valuable therapies for the treatment of infectious diseases as well as metabolic, nutritional and psychiatric disorders, cardiovascular diseases, allergies and cancer, as suggested by Lien and Lowman (2003). The increasing availability of mass spectrometry facilities together with homology-based identification of potential bioactive peptide domains on protein sequences allows in silico prediction of encrypted peptides and will in the future be essential for targeting the right proteins. Several data banks are available for the bio-informatics analysis of potential bioactive peptides (Iwaniak et al., 2005; Lahrichi et al., 2013), especially antibacterial peptides, due to the urgent issue to face antibiotic resistance (Khaldi, 2012; Mooney et al., 2013). APD2 and CAMP are databases set up to classify not only antibacterial but also antifungal and antiviral peptides (Wang et al., 2009; Thomas et al., 2010). An even larger target is supplied by BIOPEP, Pep Bank, and Peptide DB, also classifying peptide hormones, growth factors and cytokines (Shtatland et al., 2007).
A new term, “nutritional peptidomics” has been proposed by Panchaud et al. (2012) to underline the importance of characterizing (mainly by mass spectrometry) food-encrypted peptides displaying health effects. These approaches allow to set up procedures to liberate the hidden bioactive molecules by means of different proteolytic enzymes (pepsin, trypsin, chymotrypsin) but also to synthesize artificial peptides displaying bioactivity (Zambrowicz et al., 2013). Microbial fermentation is one of the most promising strategies to generate bioactive peptides, hence genomic and proteomic characterization of new strains to predict their proteolytic profile is a challenging approach in view of obtaining functional food. As far as LAB are concerned, combining the substrate specificity and the cleavage pattern of LAB proteases can highlight the strain potential to release health-promoting molecules (or possible dangerous compounds) in food (Hayes et al., 2007a,b). This is a valuable strategy for selecting the right starters and to enhance the number of LAB strains to be used as food additives or probiotics. Moreover, the use of industrial by-products, such as milk whey, as growth substrates can lower the cost of functional food production and furtherly improve nutraceutical food availability.
Author Contributions
EP: Overall organization of the paper and content. SC: Figure and table management and critical reading.
Conflict of Interest Statement
The authors declare that the research was conducted in the absence of any commercial or financial relationships that could be construed as a potential conflict of interest.
Acknowledgments
SC benefits from a grant from CRT Foundation; Turin, Italy. The research on bioactive peptides has been supported by Ricerca Locale (ex 60%).
References
Abe, Y., Umemura, S., Sugimoto, K., Hirawa, N., Kato, Y., Yokoyama, N., et al. (1995). Effect of green tea rich in γ-aminobutyric acid on blood pressure of Dahl salt-sensitive rats. Am. J. Hypertens. 8, 74–79. doi: 10.1016/0895-7061(94)00141-W
Alexandre, H., Heintz, D., Chassagne, D., Guilloux-Benatier, M., Charpentier, C., and Feuillat, M. (2001). Protease A activity and nitrogen fractions released during alcoholic fermentation and autolysis in enological conditions. J. Ind. Microbiol. Biotechnol. 26, 235–240. doi: 10.1038/sj.jim.7000119
Alvarez, M. A., and Moreno-Arribas, M. V. (2014). The problem of biogenic amines in fermented foods and the use of potential biogenic amine-degrading microorganisms as a solution. Trends Food Sci. Technol. 39, 146–155. doi: 10.1016/j.tifs.2014.07.007
Applebaum, D., Sabo, D. L., Fischer, E. H., and Morris, D. R. (1975). Biodegradative ornithine decarboxylase of Escherichia coli. Purification, properties, and pyridoxal 5’-phosphate binding site. Biochem 14, 3675–3681. doi: 10.1021/bi00687a025
Arora, T., Singh, S., and Sharma, R. K. (2013). Probiotics: interaction with gut microbiome and antiobesity potential. Nutrition 29, 591–596. doi: 10.1016/j.nut.2012.07.017
Artemova, N. V., Bumagina, Z. M., Kasakov, A. S., Shubin, V. V., and Gurvits, B. Y. (2010). Opioid peptides derived from food proteins suppress aggregation and promote reactivation of partly unfolded stressed proteins. Peptides 31, 332–338. doi: 10.1016/j.peptides.2009.11.025
Bumagina, Z. M., Kasakov, A. S., Shubin, V. V., and Gurvits, B. Y. (2009). Opioid peptides derived from food proteins suppress aggregation and promote reactivation of partly unfolded stressed proteins. Peptides 31, 332–338. doi: 10.1016/j.peptides.2009.11.025
Atanasova, J., Moncheva, P., and Ivanova, I. (2014). Proteolytic and antimicrobial activity of lactic acid bacteria grown in goat milk. Biotechnol. Biotechnol. Equip. 28, 1073–1078. doi: 10.1080/13102818.2014.971487
Aydin, S. (2007). Ghrelin may be a universal peptide in all living organisms. Turk. J. Med. Sci. 37, 123–124.
Bellamy, W., Takase, M., Yamauchi, K., Wakabayashi, H., Kawase, K., and Tomita, M. (1992). Identification of the bactericidal domain of lactoferrin. Biochim. Biophys. Acta 1121, 130–136. doi: 10.1016/0167-4838(92)90346-F
Belkaid, Y., and Hand, T. W. (2014). Role of the microbiota in immunity and inflammation. Cell 157, 121–139. doi: 10.1016/j.cell.2014.03.011
Berrocal, R., Chanton, S., Juillerat, M. A., Favillare, B., Scherz, J. C., and Jost, R. (1989). Tryptic phosphopeptides from whole casein. II. Physicochemical properties related to the solubilization of calcium. J. Dairy Res. 56, 335–341. doi: 10.1017/S0022029900028776
Bojsen, A., Buesa, J., Montava, R., Kvistgaard, A. S., Kongsbak, M. B., Petersen, T. E., et al. (2007). Inhibitory activities of bovine macromolecular whey proteins on rotavirus infections in vitro and in vivo. J. Dairy Sci. 90, 66–74. doi: 10.3168/jds.S0022-0302(07)72609-7
Brogden, K. A. (2005). Antimicrobial peptides: pore formers or metabolic inhibitors in bacteria? Nat. Rev. Microbiol. 3, 238–250. doi: 10.1038/nrmicro1098
Carey, N. (2012). The Epigenetics Revolution: How Modern Biology is rewriting Our Understanding of Genetics, Disease, and Inheritance. New York City, NY: Columbia University Press.
Chabance, B., Marteau, P., Rambaud, J. C., Migliore-Samour, D., Boynard, M., Perrotin, P., et al. (1998). Casein peptide release and passage to the blood in humans during digestion of milk or yogurt. Biochimie 80, 155–165. doi: 10.1016/S0300-9084(98)80022-9
Chang, O. K., Seol, K.-H., Jeong, S.-G., Oh, M.-H., Park, B.-Y., Perrin, C., et al. (2013). Casein hydrolysis by Bifidobacterium longum KACC91563 and antioxidant activities of peptides derived therefrom. J. Dairy Sci. 96, 5544–5555. doi: 10.3168/jds.2013-6687
Chiba H., and Yoshikawa M. (1986). “Biologically functional peptides from food proteins: new opioid peptides from milk proteins,” in Protein Tailoring for Food and Medical Uses, eds R. E. Feeney and J. R. Whitaker (New York: Marcel Dekker Inc.), 123–153.
Choi, S. I., Lee, J. W., Park, S. M., Lee, M. Y., Ge, J. Y., Park, M. S., et al. (2006). Improvement of gamma-aminobutyric acid (GABA) production using cell entrapment of Lactobacillus brevis GABA 057. J. Microbiol. Biotechnol. 16, 562–568.
Civra, A., Giuffrida, M. G., Donalisio, M., Napolitano, L., Takada, Y., Coulson, B. S., et al. (2015). Identification of Equine Lactadherin-derived peptides that inhibit rotavirus infection via integrin receptor competition. J. Biol. Chem. 290, 12403–12414. doi: 10.1074/jbc.M114.620500
Clare, D. A., and Swaisgood, H. E. (2000). Bioactive milk peptides: a prospectus. J. Dairy Sci. 83, 1187–1195. doi: 10.3168/jds.S0022-0302(00)74983-6
Coda, R., Rizzello, C. G., Pinto, D., and Gobbetti, M. (2012). Selected lactic acid bacteria synthesize antioxidant peptides during sourdough fermentation of cereal flours. Appl. Environ. Microbiol. 78, 1087–1096. doi: 10.1128/AEM.06837-11
Costantini, A., Pietroniro, R., Doria, F., Pessione, E., and Garcia-Moruno, E. (2013). Putrescine production from different amino acid precursors by lactic acid bacteria from wine and cider. Int. J. Food Microbiol. 165, 11–17. doi: 10.1016/j.ijfoodmicro.2013.04.011
Coton, E., Torlois, S., Bertrand, A., and Lonvaud-Funel, A. (1999). Biogenic amines and wine lactic acid bacteria. Bullettin 816, 22–34.
Cross, K. J., Huq, N. L., Palamara, J. E., Perich, J. W., and Reynolds, E. C. (2005). Physicochemical characterization of casein phosphopeptide-amorphous calcium phosphate nanocomplexes. J. Biol. Chem. 280, 15362–15369. doi: 10.1074/jbc.M413504200
Cryan, J. F., and Dinan, T. G. (2012). Mind-altering microorganisms: the impact of the gut microbiota on brain and behavior. Nat. Rev. Neurosci. 13, 701–712. doi: 10.1038/nrn3346
De LeBlanc, A. M., Matar, C., Thériault, C., and Perdigón, G. (2005). Effects of milk fermented by Lactobacillus helveticus R389 on immune cells associated to mammary glands in normal and a breast cancer model. Immunobiology 210, 349–358. doi: 10.1016/j.imbio.2005.05.024
De Simone, C., Picariello, G., Mamone, G., Stiuso, P., Dicitore, A., Vanacore, D., et al. (2008). Characterisation and cytomodulatory properties of peptides from Mozzarella di Bufala Campana cheese whey. J. Pept. Sci. 15, 251–258. doi: 10.1002/psc.1093
Delcenserie, V., Martel, D., Lamoureux, M., Amiot, J., Boutin, Y., and Roy, D. (2008). Immunomodulatory effects of probiotics in the intestinal tract. Curr. Issues Mol. Biol. 10, 37–54.
Dhakal, R., Bajpai, V. K., and Baek, K.-H. (2012). Production of gaba (γ - aminobutyric acid) by microorganisms: a review. Braz. J. Microbiol. 43, 1230–1241. doi: 10.1590/S1517-83822012000400001
Dimitrov, Z. (2009). Characterization of bioactive peptides with calcium-binding activity released by specially designed cheese starter. Biotechnol. Biotechnol. Equip. 23, 927–930. doi: 10.1080/13102818.2009.10818574
Dionysius, D. A., and Milne, J. M. (1997). Antibacterial peptides of bovine lactoferrin: purification and characterization. J. Dairy Sci. 80, 667–674. doi: 10.3168/jds.S0022-0302(97)75985-X
Doeven, M. K., Kok, J., and Poolman, B. (2005). Specificity and selectivity determinants of peptide transport in Lactococcus lactis and other microorganisms. Mol. Microbiol. 57, 640–649. doi: 10.1111/j.1365-2958.2005.04698.x
EFSA Panel on Biological Hazards (BIOHAZ) (2011). Scientific Opinion on risk based control of biogenic amine formation in fermented foods. EFSA J. 9:2393. doi: 10.2903/j.efsa.2011.2393
Enrique, M., Manzanares, P., Yuste, M., Martínez, M., Vallés, S., and Marcos, J. F. (2009). Selectivity and antimicrobial action of bovine lactoferrin derived peptides against wine lactic acid bacteria. Food Microbiol. 26, 340–346. doi: 10.1016/j.fm.2008.11.003
Faithong, N., Benjakul, S., Phatcharat, S., and Binsan, W. (2010). Chemical composition and antioxidative activity of Thai traditional fermented shrimp and krill products. Food Chem. 119, 133–140. doi: 10.1016/j.foodchem.2009.06.056
Fanciulli, G., Dettori, A., Demontis, M. P., Tomasi, P. A., Anania, V., and Delitala, G. (2005). Gluten exorphin B5 stimulates prolactin secretion through opioid receptors located outside the blood-brain barrier. Life Sci. 76, 1713–1719. doi: 10.1016/j.lfs.2004.09.023
Ferraretto, A., Gravaghi, C., Fiorilli, A., and Tettamanti, G. (2003). Casein-derived bioactive phosphopeptides: role of phosphorylation and primary structure in promoting calcium uptake by HT-29 tumor cells. FEBS Lett. 551, 92–98. doi: 10.1016/S0014-5793(03)00741-5
Fiat, A.-M., and Jollès, P. (1989). Caseins of various origins and biologically active casein peptides and oligosaccharides: structural and physiological aspects. Mol. Cell. Biochem. 87, 5–30. doi: 10.1007/BF00421079
FitzGerald, R. J. (1998). Potential uses of caseinophosphopeptides. Int. Dairy J. 8, 451–457. doi: 10.1016/s0958-6946(98)00068-5
FitzGerald, R. J., and Murray, B. A. (2006). Bioactive peptides and lactic fermentations. Int. J. Dairy Technol 59, 118–125. doi: 10.1111/j.1471-0307.2006.00250.x
Fukudome, S., and Yoshikawa, M. (1992). Opioid peptides derived from wheat gluten: their isolation and characterization. FEBS Lett. 296, 107–111. doi: 10.1016/0014-5793(92)80414-C
Gagnaire, V., Pierre, A., Molle, D., and Leonil, J. (1996). Phosphopeptides interacting with colloidal calcium phosphate isolated by tryptic hydrolysis of bovine casein micelles. J. Dairy Res. 63, 405–422. doi: 10.1017/S0022029900031927
Galano, E., Mangiapane, E., Bianga, J., Palmese, A., Pessione, E., Szpunar, J., et al. (2013). Privileged incorporation of selenium as selenocysteine in Lactobacillus reuteri proteins demonstrated by selenium-specific imaging and proteomics. Mol. Cell. Proteomics 12, 2196–2204. doi: 10.1074/mcp.M113.027607
Galvez, A. F., and de Lumen, B. O. (1999). A soybean cDNA encoding a chromatin-binding peptide inhibits mitosis of mammalian cells. Nat. Biotechnol. 17, 495–500. doi: 10.1038/8676
Gerez, C. L., Cuezzo, S., Rollán, G., and Font de Valdez, G. (2008). Lactobacillus reuteri CRL 1100 as starter culture for wheat dough fermentation. Food Microbiol. 25, 253–259. doi: 10.1016/j.fm.2007.10.011
Gobbetti, M., Minervini, F., and Rizzello, C. G. (2004). Angiotensin I-converting-enzyme-inhibitory and antimicrobial bioactive peptides. Int. J. Dairy Technol. 69, 5297–5305. doi: 10.1111/j.1471-0307.2004.00139.x
Gobbetti, M., Stepaniak, L., De Angelis, M., Corsetti, A., and Di Cagno, R. (2002). Latent Bioactive peptides in milk proteins: proteolytic activation and significance in dairy processing. Food Sci. Nutr. 42, 223–239. doi: 10.1080/10408690290825538
Goodyear, L. J., and Kahn, B. B. (1998). Exercise, glucose transport, and insulin sensitivity. Annu. Rev. Med. 49, 235–261. doi: 10.1146/annurev.med.49.1.235
Griffiths, M. W., and Tellez, A. M. (2013). Lactobacillus helvictus: the proteolytic system. Front. Microbiol. 4:30. doi: 10.3389/fmicb.2013.00030
Gromadzińska, J., Reszka, E., Bruzelius, K., Wasowicz, W., and Akesson, B. (2008). Selenium and cancer: biomarkers of selenium status and molecular action of selenium supplements. Eur. J. Nutr. 47, 29–50. doi: 10.1007/s00394-008-2005-z
Guo, H., Kouzuma, Y., and Yonekura, M. (2009). Structures and properties of antioxidative peptides derived from royal jelly protein. Food Chem. 113, 238–245. doi: 10.1016/j.foodchem.2008.06.081
Hartmann, R., and Meisel, H. (2007). Food-derived peptides with biological activity: from research to food applications. Curr. Opin. Biotechnol. 18, 163–169. doi: 10.1016/j.copbio.2007.01.013
Hatzoglou, A., Bakogeorgou, E., and Castanas, E. (1996a). The antiproliferative effect of opioid receptor agonists on the T47D human breast cancer cell line, is partially mediated through opioid receptors. Eur. J. Pharmacol. 296, 199–207. doi: 10.1016/0014-2999(95)00703-2
Hatzoglou, A., Bakogeorgou, E., Hatzoglou, C., Martin, P. M., and Castanas, E. (1996b). Antiproliferative and receptor binding properties of α- and β-casomorphins in the T47D human breast cancer cell line. Eur. J. Pharmacol. 310, 217–223. doi: 10.1016/0014-2999(96)00339-1
Hayes, M., Ross, R. P., Fitzgerald, G. F., Hill, C., and Stanton, C. (2006). Casein-derived antimicrobial peptides generated by Lactobacillus acidophilus DPC6026. Appl. Environ. Microbiol. 72, 2260–2264. doi: 10.1128/AEM.72.3.2260-2264.2006
Hayes, M., Stanton, C., Fitzgerald, G. F., and Ross, R. P. (2007a). Putting microbes to work: dairy fermentation, cell factories and bioactive peptides. Part I: overview. Biotechnol. J. 2, 426–434.
Hayes, M., Stanton, C., Fitzgerald, G. F., and Ross, R. P. (2007b). Putting microbes to work: dairy fermentation, cell factories and bioactive peptides. Part II: bioactive peptide functions. Biotechnol. J. 2, 435–449. doi: 10.1002/biot.200600246
Hébert, E. M., Mamone, G., Picariello, G., Raya, R. R., Savoy, G., Ferranti, P., et al. (2008). Characterization of the pattern of αs1- and β-casein breakdown and release of a bioactive peptide by a cell envelope proteinase from Lactobacillus delbrueckii subsp. lactis CRL 581. Appl. Environ. Microbiol. 74, 3682–3689. doi: 10.1128/AEM.00247-08
Hébert, E. M., Raya, R. R., and de Giori, G. S. (1999). Characterisation of a cell-envelope proteinase from Lactobacillus helveticus. Biotechnol. Lett. 21, 831–834.
Hernández-Ledesma, B., Dávalos, A., Bartolomé, B., and Amigo, L. (2005). Preparation of antioxidant enzymatic hydrolysates from α-Lactalbumin and β-Lactoglobulin. Identification of Active Peptides by HPLC-MS/MS. J. Agric. Food Chem. 53, 588–593. doi: 10.1021/jf048626m
Hernández-Ledesma, B., Recio, I., and Amigo, L. (2008). β-Lactoglobulin as source of bioactive peptides. Amino Acids 35, 257–265. doi: 10.1007/s00726-007-0585-1
Hirata, H., Sonoda, S., Agui, S., Yoshida, M., Ohinata, K., and Yoshikawa, M. (2007). Rubiscolin-6, a δ opioid peptide derived from spinach Rubisco, has anxiolytic effect via activating σ1 and dopamine D1 receptors. Peptides 28, 1998–2003. doi: 10.1016/j.peptides.2007.07.024
Hongbing, F., Xiaochang, L., Hui, H., Song, S., Qian, X., Ligeng, F., et al. (2016). Quality changes and biogenic amines accumulation of black carp (Mylopharyngodon piceus) fillets stored at different temperatures. J. Food Prot. 79, 524–586. doi: 10.4315/0362-028X.JFP-15-373
Hori, G., Wang, M.-F., Chan, Y.-C., Komtatsu, T., Wong, Y., Chenc, T.-H., et al. (2001). Soy Protein hydrolyzate with bound phospholipids reduces serum cholesterol levels in hypercholesterolemic adult male volunteers. Biosci. Biotechnol. Biochem. 65, 72–78. doi: 10.1271/bbb.65.72
Huang, J., Mei, L., Wu, H., and Lin, D. (2007). Biosynthesis of γ-aminobutyric acid (GABA) using immobilized whole cells of Lactobacillus brevis. J. Microbiol. Biotechnol. 23, 865–871. doi: 10.1007/s11274-006-9311-5
Huang, Z., Rose, A. H., and Hoffmann, P. R. (2012). The role of selenium in inflammation and immunity: from molecular mechanisms to therapeutic opportunities. Antioxid. Redox. Signal. 1 16, 705–743. doi: 10.1089/ars.2011.4145
Hyland, N. P., and Cryan, J. F. (2010). A gut feeling about GABA: focus on GABAB receptors. Front. Pharmacol. 1:124. doi: 10.3389/fphar.2010.00124
Iwaniak, A., Dziuba, J., and Niklewicz, M. (2005). The BIOPEP database - a tool for the in silico method of classification of food proteins as the source of peptides with antihypertensive activity. Acta Aliment. 34, 417–425. doi: 10.1556/AAlim.34.2005.4.9
Jensen, M. P., Vogensen, F. K., and Ardö, Y. (2009). Variation in caseinolytic properties of six cheese related Lactobacillus helveticus strains. Int. Dairy J. 19, 661–668. doi: 10.1016/j.idairyj.2009.04.001
Jiao, Z., Dong, Y., and Chen, Q. (2014). Ethyl carbamate in fermented beverages: presence, analytical chemistry, formation mechanism, and mitigation proposals. Compr. Rev. Food Sci. Food Saf. 13, 611–626. doi: 10.1111/1541-4337.12084
Kaneko, K., Iwasaki, M., Yoshikawa, M., and Ohinata, K. (2010). Orally administered soymorphins, soy-derived opioid peptides, suppress feeding and intestinal transit via gut μ1-receptor coupled to 5-HT1A, D2, and GABAB systems. Am. J. Physiol. 299, G799–G805. doi: 10.1152/ajpgi.00081.2010
Kayser, H., and Meisel, H. (1996). Stimulation of human peripheral blood lymphocytes by bioactive peptides derived from bovine milk proteins. FEBS Lett. 383, 18–20. doi: 10.1016/0014-5793(96)00207-4
Khaldi, N. (2012). Bioinformatics approaches for identifying new therapeutic bioactive peptides in food. Funct. Foods Health Dis. 2:10.
Kitts, D. D., and Weiler, K. (2003). Bioactive proteins and peptides from food sources. applications of bioprocesses used in isolation and recovery. Curr. Pharm. Des. 9, 1309–1323. doi: 10.2174/1381612033454883
Klaenhammer, T. R., Barrangou, R., Logan Buck, B., Azcarate-Peril, M. A., and Altermann, E. (2005). Genomic features of lactic acid bacteria effecting bioprocessing and health. FEMS Microbiol. Rev. 29, 393–409. doi: 10.1016/j.fmrre.2005.04.007
Korhonen, H. (2009). Milk-derived bioactive peptides: from science to applications. J. Funct. Foods 1, 177–187. doi: 10.1016/j.jff.2009.01.007
Korhonen, H., and Pihlanto, A. (2003). A. food-derived bioactive peptides - opportunities for designing future foods. Curr. Pharm. Des. 9, 1297–1308. doi: 10.2174/1381612033454892
Korhonen, H., and Pihlanto, A. (2006). A. Bioactive peptides: production and functionality. Int. Dairy J. 16, 945–960. doi: 10.1016/j.idairyj.2005.10.012
Kunji, E. R. S., Mierau, I., Hagting, A., Poolman, B., and Konings, W. N. (1996). The proteotytic systems of lactic acid bacteria. Antonie Van Leeuwenhoek 70, 187–221. doi: 10.1007/BF00395933
Kütt, M.-L., Malbe, M., and Stagsted, J. (2011). Nanostructure-assisted laser desorption/ionization (NALDI) for analysis of peptides in milk and colostrum. Agron. Res. 9, 415–420.
Ladero, V., Calles-Enriquez, M., Fernandez, M., and Alvarez, M. A. (2010). Toxicological effects of dietary biogenic amines. Curr. Nutr. Food Sci. 6, 145–156. doi: 10.2174/157340110791233256
Lafarga, T., Rai, D. K., O’Connor, P., and Hayes, M. (2015). A bovine fibrinogen-enriched fraction as a source of peptides with in vitro renin and angiotensin-i-converting enzyme inhibitory activities. J. Agric. Food Chem. 63, 8676–8684. doi: 10.1021/acs.jafc.5b03167
Laffineur, E., Genetet, N., and Leonil, J. (1996). Immunomodulatory activity of β-Casein permeate medium fermented by lactic acid bacteria. J. Dairy Sci. 79, 2112–2120. doi: 10.3168/jds.S0022-0302(96)76585-2
Lahrichi, S. L., Affolter, M., Zolezzi, I. S., and Panchaud, A. (2013). Food peptidomics: large scale analysis of small bioactive peptides — A pilot study. J. Proteomics 88, 83–91. doi: 10.1016/j.jprot.2013.02.018
Lamberti, C., Purrotti, M., Mazzoli, R., Fattori, P., Barello, C., Coïsson, J. D., et al. (2011). ADI pathway and histidine decarboxylation are reciprocally regulated in Lactobacillus hilgardii ISE 5211: proteomic evidence. Amino Acids 41, 517–527. doi: 10.1007/s00726-010-0781-2
Landete, J. M., Pardo, I., and Ferrer, S. (2008). Regulation of hdc expression and HDC activity by enological factors in lactic acid bacteria. J. Appl. Microbial. 105, 1544–1551. doi: 10.1111/j.1365-2672.2008.03865.x
LeBlanc, J., Fliss, I., and Matar, C. (2004). Induction of a humoral immune response following an Escherichia coli O157:H7 Infection with an immunomodulatory peptidic fraction derived from lactobacillus helveticus-fermented milk. Clin. Vaccine Immunol. 11, 1171–1181. doi: 10.1128/CDLI.11.6.1171-1181.2004
Lehtonen, P. (1996). Determination of amines and amino acids in wine - A review. Am. J. Enol. Vitic. 47, 127–133.
Li, S., Zhao, Y., Zhang, L., Zhang, X., Huang, L., Li, D., et al. (2012). Antioxidant activity of Lactobacillus plantarum strains isolated from traditional Chinese fermented foods. Food Chem. 135, 1914–1919. doi: 10.1016/j.foodchem.2012.06.048
Li, Y., Liu, T.-J., and He, G.-Q. (2015). Antioxidant activity of peptides from fermented milk with mix culture of lactic acid bacteria and yeast. Adv. J. Food Sci. Technol. 7, 422–427.
Li, Z., Jiang, A., Yue, T., Wang, J., Wang, Y., and Su, J. (2013). Purification and identification of five novel antioxidant peptides from goat milk casein hydrolysates. J. Dairy Sci. 96, 4242–4251. doi: 10.3168/jds.2012-6511
Lien, S., and Lowman, H. B. (2003). Therapeutic peptides. Trends Biotechnol. 21, 556–562. doi: 10.1016/j.tibtech.2003.10.005
Linares, D. M., del Rio, B., Redruello, B., Ladero, V., Martin, M. C., Fernandez, M., et al. (2016). Comparative analysis of the in vitro cytotoxicity of the dietary biogenic amines tyramine and histamine. Food Chem. 197, 658–663. doi: 10.1016/j.foodchem.2015.11.013
Lohner, K., and Blondelle, S. E. (2005). Molecular mechanisms of membrane perturbation by antimicrobial peptides and the use of biophysical studies in the design of novel peptide antibiotics. Comb. Chem. High Throughput Screen. 8, 241–256. doi: 10.2174/1386207053764576
Lonvaud-Funel, A. (1999). Lactic acid bacteria in the quality improvement and depreciation of wine. Antonie Van Leeuwenhoek 76, 317–331.
Lonvaud-Funel, A. (2001). Biogenic amines in wines: role of lactic acid bacteria. FEMS Microbiol. Lett. 199, 9–13. doi: 10.1111/j.1574-6968.2001.tb10643.x
Loukas, S., Varoucha, D., Zioudrou, C., Streaty, R. A., and Klee, W. A. (1983). Opioid activities and structures of alpha-casein-derived exorphins. Biochemistry 22, 4567–4573. doi: 10.1021/bi00288a034
Luo, H., Chen, S., Ren, F., Guo, H., Lin, S., and Xu, W. (2007). In vitro reconstitution of antimicrobial pathogen activity by expressed recombinant bovine lactoferrin N-terminal peptide in Escherichia coli. J. Dairy Res. 74, 233–238. doi: 10.1017/S0022029907002531
Lyte, M. (2011). Probiotics function mechanistically as delivery vehicles for neuroactive compounds: microbial endocrinology in the design, and use of probiotics. Bioessays 33, 574–581. doi: 10.1002/bies.201100024
Malaguti, M., Dinelli, G., Leoncini, E., Bregola, V., Bosi, S., Cicero, A. F. G., et al. (2014). Bioactive peptides in cereals and legumes: agronomical, biochemical and clinical aspects. Int. J. Mol. Sci. 15, 21120–21135. doi: 10.3390/ijms151121120
Malkoski, M., Dashper, S. G., O’Brien-Simpson, N. M., Talbo, G. H., Macris, M., Cross, K. J., et al. (2001). Kappacin, a novel antibacterial peptide from bovine milk. Antimicrob. Agents Chemother. 45, 2309–2315. doi: 10.1128/AAC.45.8.2309-2315.2001
Mangani, S., Guerrini, S., Granchi, L., and Vincenzini, M. (2005). Putrescine accumulation in wine: role of Oenococcus oeni. Curr. Microbiol. 51, 6–10. doi: 10.1007/s00284-004-4425-1
Mangiapane, E., Lamberti, C., Pessione, A., Galano, E., Amoresano, A., and Pessione, E. (2014a). Selenium effects on the metabolism of a Se-metabolizing Lactobacillus reuteri: analysis of envelope-enriched and extracellular proteomes. Mol. Biosyst. 10, 1272–1280. doi: 10.1039/c3mb70557a
Mangiapane, E., Pessione, A., and Pessione, E. (2014b). Selenium and selenoproteins: an overview on different biological systems. Curr. Protein Pept. Sci. 15, 598–607. doi: 10.2174/1389203715666140608151134
Manzotti, G., Breda, D., Di Gioacchino, M., and Burastero, S. E. (2016). Serum diamine oxidase activity in patients with histamine intolerance. Int. J. Immunopathol. Pharmacol. 29, 105–111. doi: 10.1177/0394632015617170
Mao, X.-Y., Cheng, X., Wang, X., and Wu, S.-J. (2011). Free-radical-scavenging and anti-inflammatory effect of yak milk casein before and after enzymatic hydrolysis. Food Chem. 126, 484–490. doi: 10.1016/j.foodchem.2010.11.025
Marcobal, A., De las Rivas, B., and Muñoz, R. (2006). First genetic characterization of a bacterial β-phenylethylamine biosynthetic enzyme in Enterococcus faecium RM58. FEMS Microbiol. Lett. 258, 144–149. doi: 10.1111/j.1574-6968.2006.00206.x
Matar, C., Carlos Valdez, J., Medina, M., Rachid, M., and Perdigon, G. (2001). Immunomodulating effects of milks fermented by Lactobacillus helveticus and its non-proteolytic variant. J. Dairy Res. 68, 601–609. doi: 10.1017/S0022029901005143
Mazzoli, R., Lamberti, C., Coisson, J. D., Purrotti, M., Arlorio, M., Giuffrida, M. G., et al. (2009). Influence of ethanol, malate and arginine on histamine production of Lactobacillus hilgardii isolated from an Italian red wine. Amino Acids 36, 81–89. doi: 10.1007/s00726-008-0035-8
Mazzoli, R., Pessione, E., Dufour, M., Laroute, V., Giuffrida, M. G., Giunta, C., et al. (2010). Glutamate-induced metabolic changes in Lactococcus lactis NCDO 2118 during GABA production: combined transcriptomic and proteomic analysis. Amino Acids 39, 727–737. doi: 10.1007/s00726-010-0507-5
McCann, K. B., Shiellb, B. J., Michalskib, W. P., Leec, A., Wanc, J., Roginskia, H., et al. (2006). Isolation and characterization of a novel antibacterial peptide from bovine αS1-casein. Int. Dairy J. 16, 316–323. doi: 10.1016/j.idairyj.2005.05.005
Medina, M. A., Urdiales, J. L., Rodríguez-Caso, C., Ramírez, F. J., and Sánchez-Jiménez, F. (2003). Biogenic amines and polyamines: similar biochemistry for different physiological missions and biomedical applications. Crit. Rev. Biochem. Mol. Biol. 38, 23–59. doi: 10.1080/713609209
Meisel, H., and Bockelmann, W. (1999). Bioactive peptides encrypted in milk proteins: proteolytic activation and thropho-functional properties. Antonie Van Leeuwenhoek 76, 207–215. doi: 10.1023/A:1002063805780
Meisel, H., and FitzGerald, R. J. (2003). Biofunctional peptides from milk proteins: mineral binding and cytomodulatory effects. Curr. Pharm. Des. 9, 1289–1295. doi: 10.2174/1381612033454847
Meisel, H., and Olieman, C. (1998). Estimation of calcium-binding constants of casein phosphopeptides by capillary zone electrophoresis. Anal. Chim. Acta 372, 291–297. doi: 10.1016/S0003-2670(98)00335-3
Mercier, A., Gauthier, S. F., and Fliss, I. (2004). Immunomodulating effects of whey proteins and their enzymatic digests. Int. Dairy J. 14, 175–183. doi: 10.1016/j.idairyj.2003.08.003
Migliore-Samour, D., Floc’ha, F., and Jollès, P. (1989). Biologically active casein peptides implicated in immunomodulation. J. Dairy Res. 56, 357–362. doi: 10.1017/S0022029900028806
Millichap, J. G., and Yee, M. M. (2003). The diet factor in pediatric and adolescent migraine. Pediatr. Neurol. 28, 9–15. doi: 10.1016/S0887-8994(02)00466-6
Minervini, F., Algaron, F., Rizzello, C. G., Fox, P. F., Monnet, V., and Gobbetti, M. (2003). Angiotensin I-converting-enzyme-inhibitory and antibacterial peptides from Lactobacillus helveticus PR4 proteinase-hydrolyzed caseins of milk from six species. Appl. Environ. Microbiol. 69, 5297–5305. doi: 10.1128/AEM.69.9.5297-5305.2003
Minkiewicz, P., Dziuba, J., and Michalska, J. (2011). Bovine meat proteins as potential precursors of biologically active peptides - a computational study based on the BIOPEP database. Food Sci. Technol. Res. 1, 39–45. doi: 10.1177/1082013210368461
Miquel, E., and Farré, R. (2007). Effects and future trends of casein phosphopeptides on zinc bioavailability. Trends Food Sci. Technol. 18, 139–143. doi: 10.1016/j.tifs.2006.11.004
Mizushige, T., Sawashi, Y., Yamada, A., Kanamoto, R., and Ohinata, K. (2013). Characterization of Tyr-Leu-Gly, a novel anxiolytic-like peptide released from bovine αS-casein. FASEB J. 27, 2911–2917. doi: 10.1096/fj.12-225474
Möller, N. P., Scholz-Ahrens, K. E., Roos, N., and Schrezenmeir, J. (2008). Bioactive peptides and proteins from foods: indication for health effects. Eur. J. Nutr. 47, 171–182. doi: 10.1007/s00394-008-0710-2
Mooney, C., Haslam, N. J., Holton, T. A., Pollastri, G., and Shields, D. C. (2013). Peptide Locator: prediction of bioactive peptides in protein sequences. Bioinformatics 29, 1120–1126. doi: 10.1093/bioinformatics/btt103
Moreno-Arribas, M. V., Polo, M. C., Jourganes, F., and Munoz, R. (2003). Screening of biogenic amine production by lactic acid bacteria isolated from grape must and wine. Int. J. Food Microbiol. 84, 117–123. doi: 10.1016/S0168-1605(02)00391-4
Moreno-Arribas, V., Torlois, S., Joyeux, A., Bertrand, A., and Lonvaud Funel, A. (2000). Isolation, properties and behaviour of tyramine-producing lactic acid bacteria from wine. J. Appl. Microbiol. 88, 584–593. doi: 10.1046/j.1365-2672.2000.00997.x
Morifuji, M., Kanda, A., Koga, J., Kawanaka, K., and Higuchi, M. (2010). Post-exercise carbohydrate plus whey protein hydrolysates supplementation increases skeletal muscle glycogen level in rats. Amino Acids 38, 1109–1115. doi: 10.1007/s00726-009-0321-0
Mullur, R., Liu, Y.-Y., and Brent, G. A. (2014). Thyroid hormone regulation of metabolism. Physiol. Rev. 94, 355–382. doi: 10.1152/physrev.00030.2013
Muro-Urista, C., Alvarez-Fernandez, R., Riera-Rodriguez, F., Arana Cuenca, A., and Tellez Jurado, A. (2011). Production and functionality of active peptides from milk. Food Sci. Technol. Int. 17, 293–317. doi: 10.1177/1082013211398801
Nagaoka, S., Futamura, Y., Miwa, K., Awano, T., Yamauchi, K., Kanamaru, Y., et al. (2001). Identification of novel hypocholesterolemic peptides derived from bovine milk β-lactoglobulin. Biochem. Biophys. Res. Commun. 281, 11–17. doi: 10.1006/bbrc.2001.4298
Nagaoka, S., Masaoka, M., Zhang, Q., Hasegawa, M., and Watanabe, K. (2002). Egg ovomucin attenuates hypercholesterolemia in rats and inhibits cholesterol absorption in Caco-2 cells. Lipids 37, 267–272. doi: 10.1007/s11745-002-0890-6
Nakamura, H., Takishima, T., Kometani, T., and Yokogoshi, H. (2009). Psychological stress-reducing effect of chocolate enriched with γ-aminobutyric acid (GABA) in humans: assessment of stress using heart rate variability and salivary chromogranin A. Int. J. Food Sci. Nutr. 60, 106–113. doi: 10.1080/09637480802558508
Nomura, M., Kobayashi, M., and Ohmomo, T. (2000). Inactivation of the glutamate decarboxylase gene in Lactococcus lactis subsp. Cremoris. Appl. Environ. Microbiol. 66, 2235–2237. doi: 10.1128/AEM.66.5.2235-2237.2000
Nomura, M., Nakajima, I., Fujita, Y., Kobayashi, M., Kimoto, H., Suzuki, I., et al. (1999). Lactococcus lactis contains only one glutamate decarboxylase gene. Microbiology 145, 1375–1380. doi: 10.1099/13500872-145-6-1375
O’Flaerty, S. (2014). “Probiotic-mediated modulation of host gene expression,” in Interactive Probiotics, ed. E. Pessione (Boca Raton, FL: CRC Press).
Oh, S. H. (2003). Stimulation of γ-aminobutyric acid synthesis activity in brown rice by a chitosan/glutamic acid germination solution and calcium/calmodulin. BMB Rep. 36, 319–325. doi: 10.5483/BMBRep.2003.36.3.319
Panchaud, A., Affolter, M., and Kussman, M. (2012). Mass spectrometry for nutritional peptidomics: how to analyze food bioactives and their health effects. J. Proteomics 75, 3546–3559. doi: 10.1016/j.jprot.2011.12.022
Pessione, A., Lamberti, C., and Pessione, E. (2010). Proteomics as a tool for studying energy metabolism in lactic acid bacteria. Mol. Biosyst. 8, 1419–1430. doi: 10.1039/C001948H
Pessione, E. (2010). “Biochemical aspects of nutrition: probiotics,” in Biochemical Aspects in Human Nutrition, eds L. Avigliano and L. Rossi (Kerala: Transworld research network).
Pessione, E. (2012). Lactic acid bacteria contribution to gut microbiota complexity: lights and shadows. Front. Cell Infect. Microbiol. 2:86. doi: 10.3389/fcimb.2012.00086
Pessione, E., Mazzoli, R., Giuffrida, M. G., Lamberti, C., Garcia-Moruno, E., Barello, C., et al. (2005). A proteomic approach to studying biogenic amine producing lactic acid bacteria. Proteomics 5, 687–689. doi: 10.1002/pmic.200401116
Pessione, E., Pessione, A., Lamberti, C., Coïsson, J. D., Riedel, K., Mazzoli, R., et al. (2009). First evidence of a membrane-bound, tyramine and beta-phenylethylamine producing, tyrosine decarboxylase in Enterococcus faecalis: a two-dimensional electrophoresis proteomic study. Proteomics 9, 2695–2710. doi: 10.1002/pmic.200800780
Pfluger, P. T., Schriever, S. C., and Tschöp, M. H. (2012). Nutropioids, hedonism in the gut? Cell Metab. 16, 137–139. doi: 10.1016/j.cmet.2012.07.011
Piovesana, S., Capriotti, A. L., Cavaliere, C., La Barbera, G., Samperi, R., Zenezini Chiozzi, R., et al. (2015). Peptidome characterization and bioactivity analysis of donkey milk. J. Proteomics 119, 21–29. doi: 10.1016/j.jprot.2015.01.020
Porto, B., Mangiapane, E., Pessione, A., Neves, M. J., and Pessione, E. (2015). Martins FS The effects of sodium selenite on the probiotic Saccharomyces cerevisiae UFMG A-905: a physiological and proteomic analysis. J. Funct. Food 17, 828–836. doi: 10.1016/j.jff.2015.06.048
Power, O., Jakeman, P., and FitzGerald, R. J. (2013). Antioxidative peptides: enzymatic production, in vitro and in vivo antioxidant activity and potential applications of milk-derived antioxidative peptides. Amino Acids 44, 797–820. doi: 10.1007/s00726-012-1393-9
Prioult, G., Pecquet, S., and Fliss, I. (2004). Allergenicity of acidic peptides from bovine β-lactoglobulin is reduced by hydrolysis with Bifidobacterium lactis NCC362 enzymes. Int. Dairy J. 15, 439–448. doi: 10.1016/j.idairyj.2004.09.001
Qian, Z.-Y., Jollès, P., Migliore-Samour, D., Schoentgen, F., and Fiat, A.-M. (1995). Sheep κ-casein peptides inhibit platelet aggregation. Biochim. Biophys. Acta 1244, 411–417. doi: 10.1016/0304-4165(95)00047-F
Rajapakse, N., Mendis, E., Jung, W.-K., Je, J.-Y., and Kim, S.-K. (2005a). Purification of a radical scavenging peptide from fermented mussel sauce and its antioxidant properties. Food Res. Int. 38, 175–182. doi: 10.1016/j.foodres.2004.10.002
Rajapakse, N., Mendisa, E., Byunb, H.-G., and Kima, S.-K. (2005b). Purification and in vitro antioxidative effects of giant squid muscle peptides on free radical-mediated oxidative systems. J. Nutri. Biochem. 16, 562–569. doi: 10.1016/j.jnutbio.2005.02.005
Rao, S., Sun, J., Liu, Y., Zeng, H., Su, Y., and Yang, Y. (2012). ACE inhibitory peptides and antioxidant peptides derived from in vitro digestion hydrolysate of hen egg white lysozyme. Food Chem. 135, 1245–1252. doi: 10.1016/j.foodchem.2012.05.059
Recsei, P. A., and Snell, E. E. (1985). Pyruvoyl-dependent histidine decarboxylases. Mechanism of cleavage of the proenzyme from Lactobacillus buchneri. J. Biol. Chem. 260, 2804–2806.
Reynolds, E. C., Cai, N. J. F., Cochrane, P., Shen, G. D., Walker, M. V., and Morgan, C. (2008). Fluoride and casein phosphopeptide-amorphous calcium phosphate. J. Dent. Res. 87, 344–348. doi: 10.1177/154405910808700420
Ricci-Cabello, I., Herrera, M. O., and Artacho, R. (2012). Possible role of milk-derived bioactive peptides in the treatment and prevention of metabolic syndrome. Nutr. Rev. 70, 241–255. doi: 10.1111/j.1753-4887.2011.00448.x
Rizzello, C. G., Cassone, A., Di Cagno, R., and Gobbetti, M. (2008). Synthesis of angiotensin i-converting enzyme (ACE)-inhibitory peptides and γ-aminobutyric acid (GABA) during sourdough fermentation by selected lactic acid bacteria. J. Agric. Food Chem. 56, 6936–6943. doi: 10.1021/jf800512u
Roy, M. K., Kuwabara, Y., Hara, K., Watanabe, Y., and Tamai, Y. (2002). Peptides From the N-terminal End of Bovine Lactoferrin Induce Apoptosis in Human Leukemic (HL-60) Cells. J. Dairy Sci. 85, 852065–852074. doi: 10.3168/jds.S0022-0302(02)74284-7
Roy, M. K., Watanabe, Y., and Tamai, Y. (1999). Induction of apoptosis in HL-60 cells by skimmed milk digested with a proteolytic enzyme from the yeast Saccharomyces cerevisiae. J. Biosci. Bioeng. 88, 426–432. doi: 10.1016/S1389-1723(99)80221-7
Saavedra, L., Hébert, E. M., Minahk, C., and Ferranti, P. (2013). An overview of “omic” analytical methods applied in bioactive peptide studies. Food Res. Int. 54, 925–934. doi: 10.1016/j.foodres.2013.02.034
Santos, S. M. H. (1996). Biogenic amines: their importance in foods. Int. J. Food Microbiol. 29, 213–231. doi: 10.1016/0168-1605(95)00032-1
Saraswat, S., Snyder, B., and Isailovic, D. (2012). Quantification of HPLC-separated peptides and proteins by spectrofluorimetric detection of native fluorescence and mass spectrometry. J. Chromatogr. B 902, 70–77. doi: 10.1016/j.jchromb.2012.06.018
Savijoki, K., Ingmer, H., and Varmanen, P. (2006). Proteolytic systems of lactic acid bacteria. Appl. Microbiol. Biotechnol. 71, 394–406. doi: 10.1007/s00253-006-0427-1
Sessa, A., Desiderio, M. A., and Perin, A. (1984). Effect of acute ethanol administration on diamine oxidase activity on the upper gastrointestinal tract of rat. Alcohol. Clin. Exp. Res. 8, 185–190. doi: 10.1111/j.1530-0277.1984.tb05835.x
Sharma, S., Singh, R., and Rana, S. (2011). Bioactive peptides: a review. Intc J. BIOautomation 15, 223–250.
Sharon, G., Garg, N., Debelius, J., Knight, R., Dorrestein, P. C., and Mazmanian, S. K. (2014). Specialized metabolites from the microbiome in health and disease. Cell Metab. 20, 719–730. doi: 10.1016/j.cmet.2014.10.016
Sheih, I.-C., Wub, T.-K., and Fang, T. J. (2009). Antioxidant properties of a new antioxidative peptide from algae protein waste hydrolysate in different oxidation systems. Bioresour. Technol. 100, 3419–3425. doi: 10.1016/j.biortech.2009.02.014
Shimazu, S., and Miklya, I. (2004). Pharmacological studies with endogenous enhancer substances: beta phenylethylamine, tryptamine and their synthetic derivatives. Prog. Neuropsychopharmacol. Biol. Psichiatry 28, 421–427. doi: 10.1016/j.pnpbp.2003.11.016
Shtatland, T., Guettler, D., Kossodo, M., Pivovarov, M., and Weissleder, R. (2007). PepBank - a database of peptides based on sequence text mining and public peptide data sources. BMC Bioinf. 8:280. doi: 10.1186/1471-2105-8-280
Singh, T. K., Fox, P. F., and Healy, A. (1997). Isolation and identification of further peptides in the diafiltration retentate of the water-soluble fraction of Cheddar cheese. J. Dairy Res. 64, 433–443. doi: 10.1017/S0022029997002227
Singh, V. P., Pathak, V., and Verma, A. K. (2012). Fermented meat products: organoleptic qualities and biogenic amines–a review. Am. J. Food Technol. 7, 278–288. doi: 10.3923/ajft.2012.278.288
Smeianov, V. V., Wechter, P., Broadbent, J. R., Hughes, J. E., Rodríguez, B. T., Christensen, T. K., et al. (2007). Comparative high-density microarray analysis of gene expression during growth of Lactobacillus helveticus in milk versus rich culture medium. Appl. Environ. Microbiol. 73, 2661–2672. doi: 10.1128/AEM.00005-07
Somda, M. K., Savadogo, A., Barro, N., Thonart, P., and Traore, A. S. (2011). Effect of mineral salts in fermentation process using mango residues as carbon source for bioethanol production. Asian J. Ind. Eng. 3, 29–38. doi: 10.3923/ajie.2011.29.38
Svensson, M., Håkansson, A., Mossberg, A.-K., Linse, S., and Svanborg, C. (2000). Conversion of α-lactalbumin to a protein inducing apoptosis. Proc. Natl. Acad. Sci. U.S.A. 97, 4221–4226. doi: 10.1073/pnas.97.8.4221
Takahashi, M., Fukunaga, H., Kaneto, H., Fukudome, S., and Yoshikawa, M. (2000). Behavioral and pharmacological studies on gluten exorphin a5, a newly isolated bioactive food protein fragment, in mice. Jpn. J. Pharmacol. 84, 259–265. doi: 10.1254/jjp.84.259
Tan, D. X., Zheng, X., Kong, J., Manchester, L. C., Hardeland, R., Kim, S. J., et al. (2014). Fundamental issues related to the origin of melatonin and melatonin isomers during evolution: relation to their biological functions. Int. J. Mol. Sci. 15, 15858–15890. doi: 10.3390/ijms150915858
Tellez, A., Corrediga, M., Brovkoa, L. Y., and Griffith, M. W. (2010). Characterization of immune-active peptides obtained from milk fermented by Lactobacillus helveticus. J. Dairy Res. 77, 129–136. doi: 10.1017/S002202990999046X
Tellez, A., Corredig, M., Turner, P. V., Morales, R., and Griffiths, M. (2011). A peptidic fraction from milk fermented with Lactobacillus helveticus protects mice against Salmonella infection. Int. Dairy J. 21, 607–614. doi: 10.1016/j.idairyj.2011.03.011
Teucher, B., Majsak-Newman, G., Dainty, J. R., McDonagh, D., FitzGerald, R., and Fairweather-Tait, S. J. (2006). Calcium absorption is not increased by caseinophosphopeptides1,2,3. Am. J. Clin. Nutr. 84, 162–166.
Thakur, N., Qureshi, A., and Kumar, M. (2012). AVPpred: collection and prediction of highly effective antiviral peptides. Nucleic Acids Res. 40, W199–W204. doi: 10.1093/nar/gks450
Thomas, S., Karnik, S., Barai, R. S., Jayaraman, V. K., and Idicula-Thomas, S. (2010). CAMP: a useful resource for research on antimicrobial peptides. Nucleic Acids Res. 38, D774–D780. doi: 10.1093/nar/gkp1021
Tsai, J. S., Lin, Y. S., Pan, B. S., and Chen, T. J. (2006). Antihypertensive peptides and γ-aminobutyric acid from prozyme 6 facilitated lactic acid bacteria fermentation of soymilk. Process Biochem. 41, 1282–1288. doi: 10.1016/j.procbio.2005.12.026
Ulvatne, H., Samuelsen,Ø., Haukland, H. H., Krämer, M., and Vorland, L. H. (2004). Lactoferricin B inhibits bacterial macromolecular synthesis in Escherichia coli and Bacillus subtilis. FEMS Microbiol. 237, 377–384. doi: 10.1111/j.1574-6968.2004.tb09720.x
van Platerink, C. J., Janssen, H.-G. M., Horsten, R., and Haverkamp, J. (2006). Quantification of ACE inhibiting peptides in human plasma using high performance liquid chromatography–mass spectrometry. J. Chromatogr. B 830, 151–157. doi: 10.1016/j.jchromb.2005.10.036
Virtanen, T., Pihlanto, A., Akkanen, S., and Korhonen, H. (2007). Development of antioxidant activity in milk whey during fermentation with lactic acid bacteria. J. Appl. Microbiol. 102, 106–115. doi: 10.1111/j.1365-2672.2006.03072.x
Visick, K. L., and Fuqua, C. (2005). Decoding microbial chatter: cell-cell communication in bacteria. J. Bacteriol. 187, 5507–5519. doi: 10.1128/JB.187.16.5507-5519.2005
Vorland, L. H. (1999). Lactoferrin: a multifunctional glycoprotein. APMIS 107, 971–981. doi: 10.1111/j.1699-0463.1999.tb01499.x
Wakai, T., and Yamamoto, N. (2012). “Antihypertensive peptides specific to Lactobacillus helveticus fermented milk,” in Biotechnology – Molecular Studies and Novel Applications for Improved Quality of Human Life, Biochemistry, Genetics and Molecular Biology, ed. R. H. Sammour (Rijeka: InTech), 159–172.
Wang, G., Li, X., and Wang, Z. (2009). APD2: the updated antimicrobial peptide database and its application in peptide design. Nucleic. Acids Res. 37(Suppl. 1), D933–D937. doi: 10.1093/nar/gkn823
Wei, G. S., Jackson, J. L., Hatzigeorgiou, C., and Tofferi, J. K. (2003). Osteoporosis management in the new millennium. Prim. Care 30, 711–741. doi: 10.1016/S0095-4543(03)00090-3
Yamamoto, N., Maeno, M., and Takano, T. (1999). Purification and characterization of an antihypertensive peptide from a yogurt-like product fermented by Lactobacillus helveticus CPN4. J. Dairy Sci. 82, 1388–1393. doi: 10.3168/jds.S0022-0302(99)75364-6
Yanez, M., Padin, J. F., Arranz-Tagarro, J. A., Camina, M., and Laguna, R. (2012). History and therapeutic use of MAO-A inhibitors: a historical perspective of mao-a inhibitors as antidepressant drug. Curr. Top. Med. Chem. 12, 2275–2282. doi: 10.2174/156802612805220011
Yang, S., Yunden, J., Sonoda, S., Doyama, N., Lipkowski, A. W., Kawamura, Y., et al. (2001). Rubiscolin, a δ selective opioid peptide derived from plant Rubisco. FEBS Lett. 509, 213–217. doi: 10.1016/S0014-5793(01)03042-3
Yang, S., Kawamura, Y., and Yoshikawa, M. (2003). Effect of rubiscolin, a δ opioid peptide derived from Rubisco, on memory consolidation. Peptides 24, 325–328. doi: 10.1016/S0196-9781(03)00044-5
Yang, Y., Marczak, E. D., Yokoo, M., Usui, H., and Yoshikawa, M. (2003). Isolation and antihypertensive effect of angiotensin i-converting enzyme (ace) inhibitory peptides from spinach rubisco. J. Agric. Food Chem. 51, 4897–4902. doi: 10.1021/jf026186y
Zambrowicz, A., Timmer, M., Polanowski, A., Lubec, G., and Trziszka, T. (2013). Manufacturing of peptides exhibiting biological activity. Amino Acids 44, 315–320. doi: 10.1007/s00726-012-1379-7
Zhang, H., Hu, C. A., Kovacs-Nolan, J., and Mine, Y. (2015). Bioactive dietary peptides and amino acids in inflammatory bowel disease. Amino Acids 47, 2127–2141. doi: 10.1007/s00726-014-1886-9
Keywords: opioid, antioxidant, chaperon-like, cell-cycle control, antimicrobial peptides, beta-phenylethylamine, tyramine
Citation: Pessione E and Cirrincione S (2016) Bioactive Molecules Released in Food by Lactic Acid Bacteria: Encrypted Peptides and Biogenic Amines. Front. Microbiol. 7:876. doi: 10.3389/fmicb.2016.00876
Received: 09 February 2016; Accepted: 24 May 2016;
Published: 09 June 2016.
Edited by:
Steve Lindemann, Pacific Northwest National Laboratory, USAReviewed by:
Giulia Tabanelli, CIRI Agroalimentare, ItalyGiovanna Suzzi, Università degli Studi di Teramo, Italy
Copyright © 2016 Pessione and Cirrincione. This is an open-access article distributed under the terms of the Creative Commons Attribution License (CC BY). The use, distribution or reproduction in other forums is permitted, provided the original author(s) or licensor are credited and that the original publication in this journal is cited, in accordance with accepted academic practice. No use, distribution or reproduction is permitted which does not comply with these terms.
*Correspondence: Enrica Pessione, enrica.pessione@unito.it