- 1Laboratory of Veterinary Pharmacology, College of Veterinary Medicine, South China Agricultural University, Guangzhou, China
- 2Centre for Veterinary Drug Residues, College of Veterinary Medicine, South China Agricultural University, Guangzhou, China
- 3China Institute of Veterinary Drug Control, Beijing, China
Cefquinome is a fourth-generation Cephalosporin approved for use in animals exclusively. The objective of this study was to explore the relationship of cefquinome pharmacokinetic/pharmacodynamic (PK/PD) indices with resistance selection of Staphylococcus aureus ATCC25923 in an in vitro model. Six dosing regiments of cefquinome at an interval of 24 h for three consecutive times were simulated, resulting in maximum concentrations (Cmax) from 1/2 to 16MIC and terminal half-lives (t1/2β) of 3 and 6 h, respectively. The in vitro sensitivity of S. aureus was monitored by bacterial susceptibility and dynamic time-kill curve experiments over the six cefquinome concentrations. The correlation between changes in bacterial susceptibility (MIC72/MIC0) and the percentage of time within mutant selection window versus dosing interval (TMSW %) was subjected to the Gaussian function and regression analysis. Our results favored the consensus that time above MIC (T > MIC) was recognized as an important PK/PD parameter of cephalosporins for antibacterial efficiency. Cefquinome reached the maximum killing effect when T > MIC% attained approximately 40~60%. The subsequent correlation analysis demonstrated that resistant S. aureus ATCC25923 was easy to occur when TMSW% attained an index of about 20% with t1/2β of 3 h after multiple dosing, and 40% with t1/2β of 6 h after multiple dosing, respectively.
Introduction
Staphylococcus aureus is a major pathogen for animals and humans, which contributes to a variety of severe infections, including bacteremia, meningitis, endocarditis, skin and wood infection or other diseases for animals and humans (Archer, 1998; Lowy, 1998; Azizoglu et al., 2013). More importantly, S. aureus plays an important role in the food contamination by foodborne pathogens. Food poisoning caused by staphylococcal enterotoxin (SE) is a pressing worldwide health problem (Tauxe, 2002; Le Loir et al., 2003). In spite of the progress in antimicrobial therapy, treatment of S. aureus infection has become more and more challenging because drug-resistance of S. aureus has increased globally over the past decade. The methicillin-resistant S. aureus (MRSA) and vancomycin-resistant S. aureus (VRSA) were particularly reported and well documented (Harris et al., 2010; Mohammed Fayaz et al., 2011).
In veterinary medicine, cefquinome (CEQ) has been licensed in European countries by virtue of its broad antibacterial spectrums and remarkable antibacterial activities. Despite of this, the cephalosporins should be in prudent use considering the escalating antimicrobial resistance. Evidence has showed that different animal species may harbor the same resistance determinant and are recognized as possible reservoirs of antimicrobial-resistant bacteria (Guardabassi et al., 2004). It has been also observed that cefquinome exerted a selective effect on blaCTX-M producing Escherichia coli strains (Cavaco et al., 2008). Therefore, further information concerning the ability of cefquinome to prevent occurring of resistant strains seems to be investigated.
Since mutant prevention concentration (MPC) of antibiotics was first described by Dong et al. (1999), it has been successfully applied to evaluate the ability of antibiotics that restrict the selection of resistant strains (Allen et al., 2004; Allen and Hankins, 2009; Wang et al., 2010; Blondeau et al., 2012) and optimize the current dosing regimens of antibiotics to slow the emergence of resistant strains. MPC was taken as the lowest doubling dilution drug concentration that prevented a population of 1010 colony forming unit (CFU)/mL or even more microorganisms from first-step mutation. Zone of the drug concentrations between minimum inhibitory concentration (MIC) and MPC was defined as the mutant selection window (MSW). MPC and MSW concepts, representing the ability of antibiotics to select resistant strains, have been tested in various in vitro studies (Allen et al., 2004; Croisier et al., 2004b; Ferran et al., 2007), in ex vivo pharmacodynamic studies (Bronner et al., 2002) and in in vivo studies (Croisier et al., 2004a; Cui et al., 2006). Here, we developed an in vitro kinetic model to investigate the relationship between pharmacokinetic/pharmacodynamic (PK/PD) indices of cefquinome with terminal half-lives (t1/2β) of 3 or 6 h and resistance development of S. aureus ATCC25923.
Materials and Methods
Antimicrobial Agents, Medium, and Bacterial Strains
Raw material of cefquinome (purity of 84.1%) was obtained from Hebei yuanzheng Pharmaceutical Enterprise, Co., Ltd. Mueller-Hinton broth (MHB) and agar were purchased from Guangzhou huankai Comapany. S. aureus ATCC 25923 was purchased from the China Institute of Veterinary Drug Control (Beijing, China).
In Vitro Susceptibility Testing
The MIC of CEQ against S. aureus with an inoculum of 5 × 105 CFU/mL was determined by standard agar dilution method established by the Clinical and Laboratory Standards Institute (CLSI).
Measurement of MIC99, Mutant Prevention Concentration (MPC) and Selection Index (SI)
The MIC99 was defined as the drug concentration that inhibited 99% of bacteria colony formation. MPC was reckoned as the lowest cefquinome concentration blocking ≥1010 CFU/mL inoculants growth. The measurements of MIC99 and MPC were mainly based on the method reported by Zhao and Drlica (2002) with slight modification. For MIC99, agar plates containing a series of cefquinome (concentrations ranging from 0.5 to 0.164 μg/mL) at 20% per sequential decrease were prepared. 3 × 107 CFU/mL S. aureus suspension in logarithmic phase of growth was then subjected to serial 10-fold dilutions with MHB to 3 × 102 CFU/mL bacteria. Again, 100 μL of each dilution was plated onto the agar plates containing the series of CEQ concentrations mentioned above and incubated at 37°C overnight. Bacterial colonies that recovered growth in each dilution were counted. Drug concentrations versus the percentages of colony recovery were plotted and the interpolation method was adopted to calculate the cefquinome concentration blocking 99% bacteria growth.
The determination of MPC was similar to that of MIC99. Instead, 100 μL of 1010 CFU/mL inoculants was spread on agar plates containing a series of antimicrobial concentrations. The lowest antimicrobial concentration preventing bacterial colony formation at 72 h after incubation was measured as provisional mutant prevention concentration (MPCpr). A second determination that utilized linear MPCpr decreases (about 20% per sequential decrease) was performed. MPC was the lowest CEQ concentration preventing 100% growth of S. aureus colony.
The calculated ratio of MPC/MIC99 for S. aureus was defined as selection index (SI) of cefquinome.
In Vitro Dynamic PK/PD Model Simulation
A previous described dynamic model (Grasso et al., 1978) with modification was developed in this study. One-compartment open model with first-order absorption of pharmacokinetics for cefquinome was simulated. The schematic representation of in vitro PK/PD model used is depicted in Figure 1. Briefly, the system was mainly composed of three sealed containers (compartments) and they were connected with peristaltic pumps in line, each containing sterile MHB and a magnetic stirrer to ensure adequate mixing. One 500 mL sealed compartment containing 300 mL MHB, provided with either a bacterial culture alone (control growth experiments) or a bacterial culture plus antibiotic (killing/re-growth experiments), acted as the central chamber. Another 100 mL sealed container charged with 60 mL of sterile MHB, acted as the absorption chamber, with desired calculated drug concentrations. The third one was used to provide fresh MHB. Waste was also collected. Peristaltic pumps circulated fresh broth to central and absorption compartments at the desired flow rate. The flow rate of the pump was set on the basis of the terminal half-life being simulated. A series of dosage regimens were designed to generate different CEQ concentration profiles with terminal half-lives of 3 and 6 h for three consecutive administrations in this apparatus.
In Vitro PK/PD Model and CEQ Dosing Regimens
The apparatus ran under 37°C. The elimination terminal half-lives of cefquinome reported in the literature varied from 0.5 to 10 h (Limbert et al., 1991; Li et al., 2008; Al-Taher, 2010; Tohamy, 2011; Yuan et al., 2011; Zonca et al., 2011; Dumka et al., 2013), therefore, 3 and 6 h were selected in our study. Peristaltic pumps circulated fresh MHB medium to and from the central compartment at a flow rate of 15.75 r/min for t1/2β of 3 h or 7.86 r/min for t1/2β of 6 h, respectively. 3 mL bacterial suspensions (108 CFU/mL) at the logarithmic phase were injected into the central compartment container. The bacteria were incubated in the model for 2 h to result in exponentially growing cultures before addition of cefquinome. Antibiotic doses were calculated to generate CEQ initial concentrations of 1/2MIC, MIC, 2MIC, 4MIC, 8MIC, and 16MIC levels, respectively. The in vitro pharmacokinetics of cefquinome for all doses after three consecutive administrations with 24 h interval for terminal half-lives of 3 and 6 h were simulated.
Quantification of Cefquinome in MHB
Samples were collected from the central compartment immediately before and at 0.5, 1.0, 2.0, 3.0, 4.0, 6.0, 8.0, 10.0, 12.0, and 24.0 h after the first and second administration, and 0.5, 1.0, 2.0, 3.0, 4.0, 6.0, 8.0, 10.0, 12.0, 24.0, 30.0, 36.0, and 48.0 h after the third administration. These samples were stored at -20°C until analysis.
1.5 mL of MHB was extracted for the determination of cefquinome. The concentrations of cefquinome in MHB were analyzed by high-performance liquid chromatography-MS/MS (HPLC-MS/MS) with an assay range of 0.005–0.5 μg/mL. The protein in MHB sample (1 mL) was precipitated by 2 mL acetonitrile, and the supernatant was directly injected into HPLC-MS/MS after high speed centrifugation. Analysis of quality control (QC) samples at three levels (0.01, 0.05, 0.2 μg/mL) showed that the recoveries of the method were above 70%; the intra-day and inter-day coefficients of variation were within 15%. The pharmacokinetic parameters were calculated by a WinNonlin software (version 5.2.1; Pharsight Corporation, USA).
In Vitro Time-Kill Experiments and Susceptibility Testing of S. aureus
To measure the antimicrobial effect of cefquinome with different terminal half-lives against S. aureus, the colony count and susceptibility of bacteria in each time point were performed after treatment. Half of each sample was subjected to time-kill kinetic assays. Samples (100 μL) in series of 10-fold dilution with sterile saline (0.9% NaCl) were spread onto Mueller-Hinton agar to determine the number of total or resistant cells. The log10 of surviving S. aureus cells (CFU/mL) was plotted against each time point. The other half of each sample was used for the susceptibility test. MIC values of bacteria post-exposed in each time point was conducted using tube dilution method according to the criteria established by the Clinical Laboratory Standards Institute [CLSI] (2013).
Pharmacokinetic/Pharmacodynamic (PK/PD) Integration Analysis
By using the individual pharmacokinetic result from each in vitro dosage regimen, the following PK/PD parameters were obtained: ratio of area under the curve of cefquinome concentration versus time to MIC (AUC0-∞/MIC) or (AUC0-24 h/MIC), ratio of area under the curve of cefquinome concentration exceeding MIC to MIC (AUCC > MIC/MIC), time of concentration above MIC (T > MIC) or MPC (T > MPC), time of concentration within MPC and MIC (TMSW) or expressed as percentage (TMSW%), ratio of MIC72 to MICinitial (MIC72/MICinitial).
Gaussian function was used to simulate the correlation of MIC72 h/MICinitial with TMSW% (percentage of the time during which cefquinome concentrations were inside the MSW). The formula was as follows:
where A was the area under curve and upper baseline; x0 was the minimum value of TMSW% which resulted in the maximum MIC72 h/MICinitial.
Results
MIC99 and MPC
The MIC99 and MPC of cefquinome against S. aureus ATCC 25923 were 0.4 and 4.096 μg/mL, respectively. So the SI was 10.24 (MPC/MIC99).
In Vitro Simulated Pharmacokinetics
The in vitro simulated time-concentration curves for cefquinome with terminal half-lives of 3 and 6 h are shown in Figure 2. This study used time-concentration curves of the unbound fractions of cefquinome in Mueller-Hinton broth. The maximum concentrations were approximately equal to 1/2MIC to 16-fold MIC of cefquinome against S. aureus ATCC 25923 after three consecutive administrations. The MPC and MIC levels were also indicated in the simulated time-concentration curves.
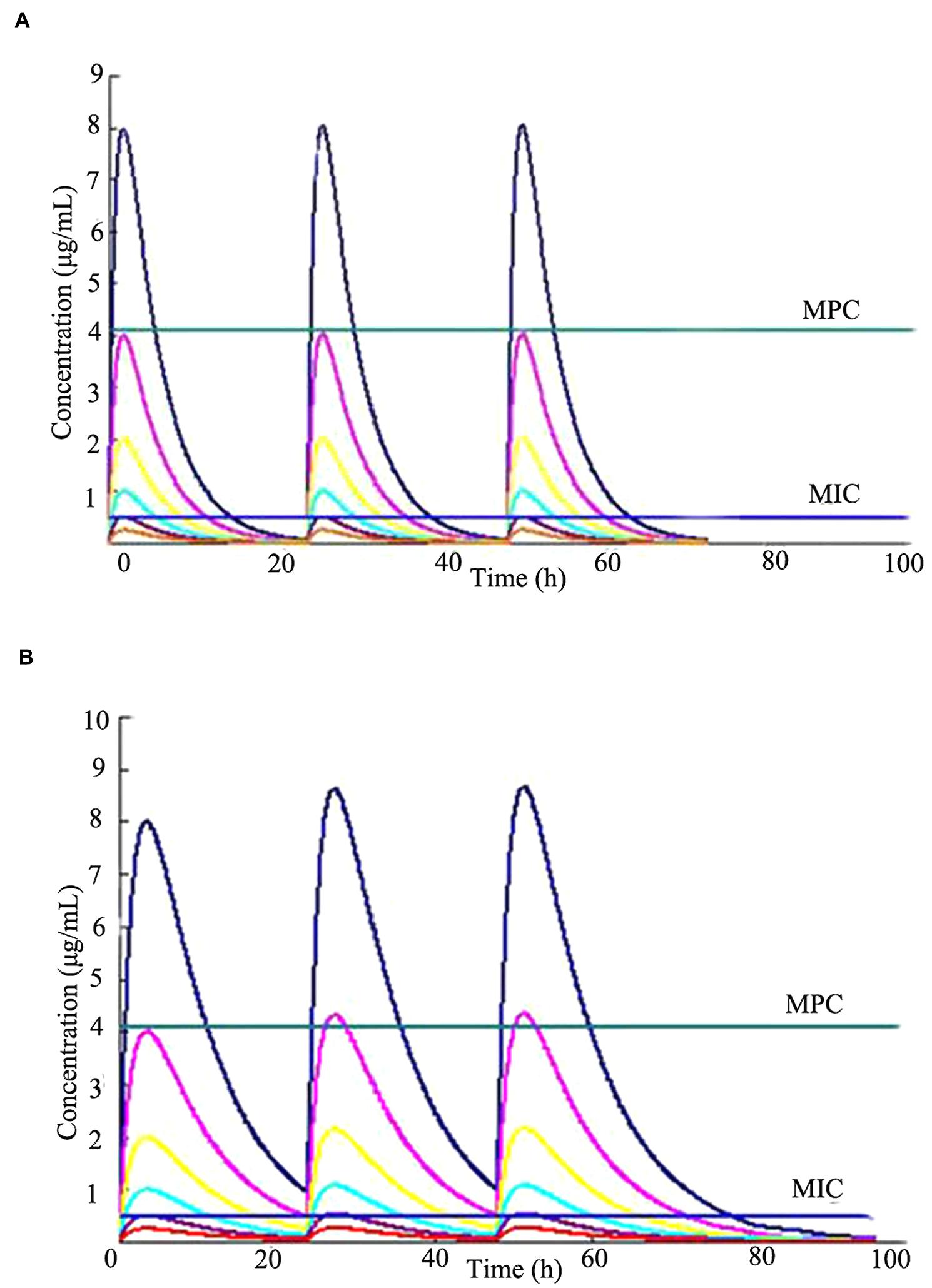
FIGURE 2. Concentration-time curves of cefquinome in the in vitro pharmacokinetics model of two terminal half-lives (t1/2β). The horizontal lines indicate the MPC (4.096 μg/mL) and MIC (0.4 μg/mL) for Staphylococcus aureus ATCC 25923, respectively. (A) t1/2β = 3 h, (B) t1/2β = 6 h.
The Bacteria Killing Curves in In Vitro Models
Time-killing curves of S. aureus ATCC 25923 in the in vitro model under different dosing regimens are shown in the following Figure 3. For a t1/2β of 3 h multiple dosages, bacteriostatic or bactericidal action was observed when maximum cefquinome concentrations were equal to MIC or over MIC levels (dosages ranged from 0.672 to 10.767 mg). For a t1/2β of 6 h multiple dosages, continuous bactericidal action was observed when maximum cefquinome concentrations were at 4MIC, 8MIC, 16MIC (dose ranged from 2.691 to 10.767 mg), and the continuous time of bactericidal action at 16MIC was the longest among three concentrations.
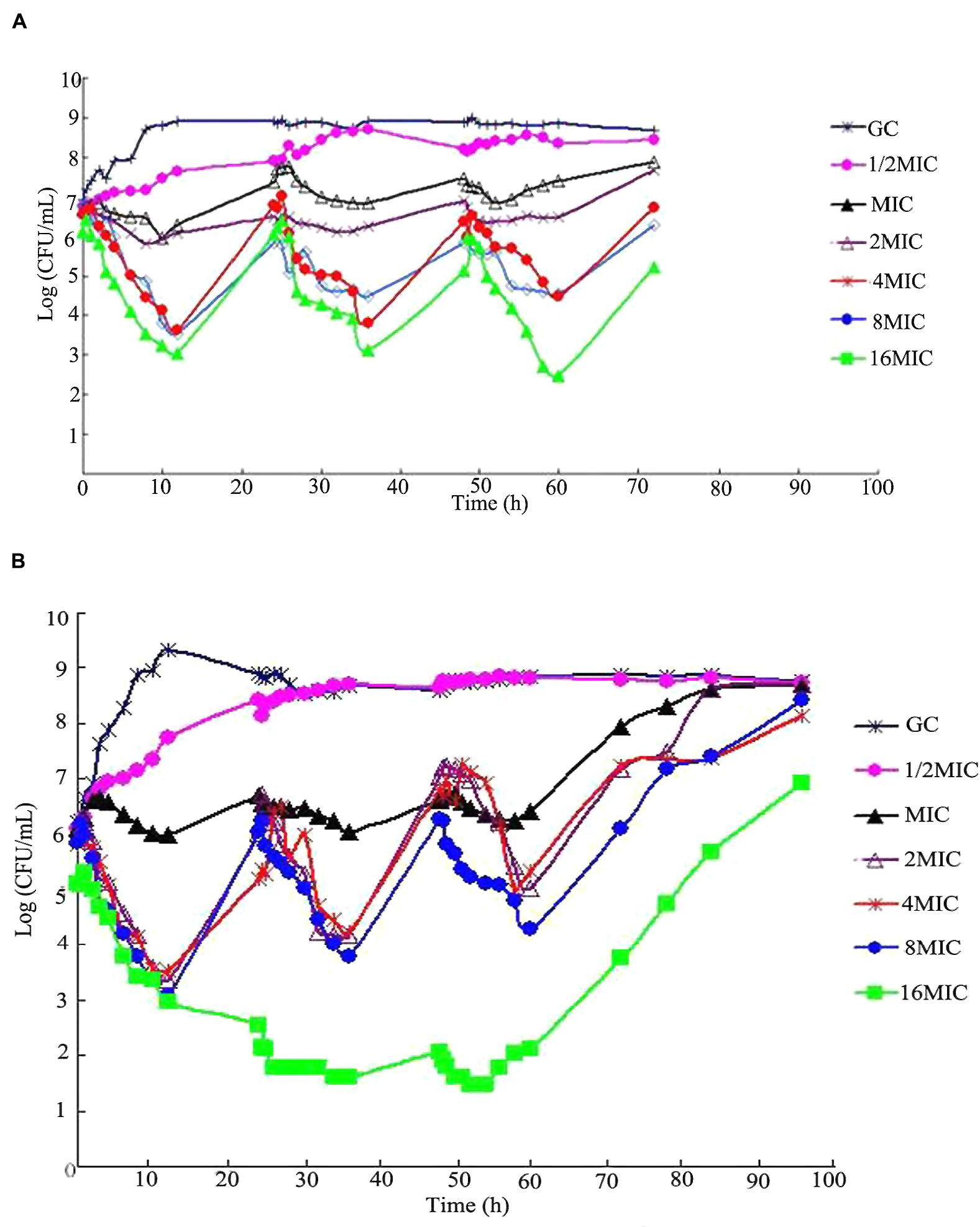
FIGURE 3. Viable counts of S. aureus ATCCC 25923 in time-kill assays with cefquinome in different terminal half-lives (t1/2β) at cefquinome concentrations from a half to 16-fold the minimum inhibitory concentration (MIC), in comparison with a cefquinome-free control. (A) t1/2β = 3 h, (B) t1/2β = 6 h.
Loss of Susceptibility to Cefquinome
Compared to drug-free group, loss of susceptibility was observed in MIC, 2MIC, and 4MIC concentration groups after administrations, as shown in Figure 4. For multiple dosages with t1/2β of 3 h, the bacterial MICs after administration increased to 4.0, 8.0, and 1.0 μg/mL for MIC, 2MIC, and 4MIC groups, respectively. For multiple dosages with t1/2β of 6 h, the bacterial MICs after administration increased to 2.0, 4.0, and 0.8 μg/mL for MIC, and 2MIC, and 4MIC concentration administrations, respectively. The MIC values did not change through the whole experiment period in 8MIC and 16MIC administration groups with two different terminal half-lives.
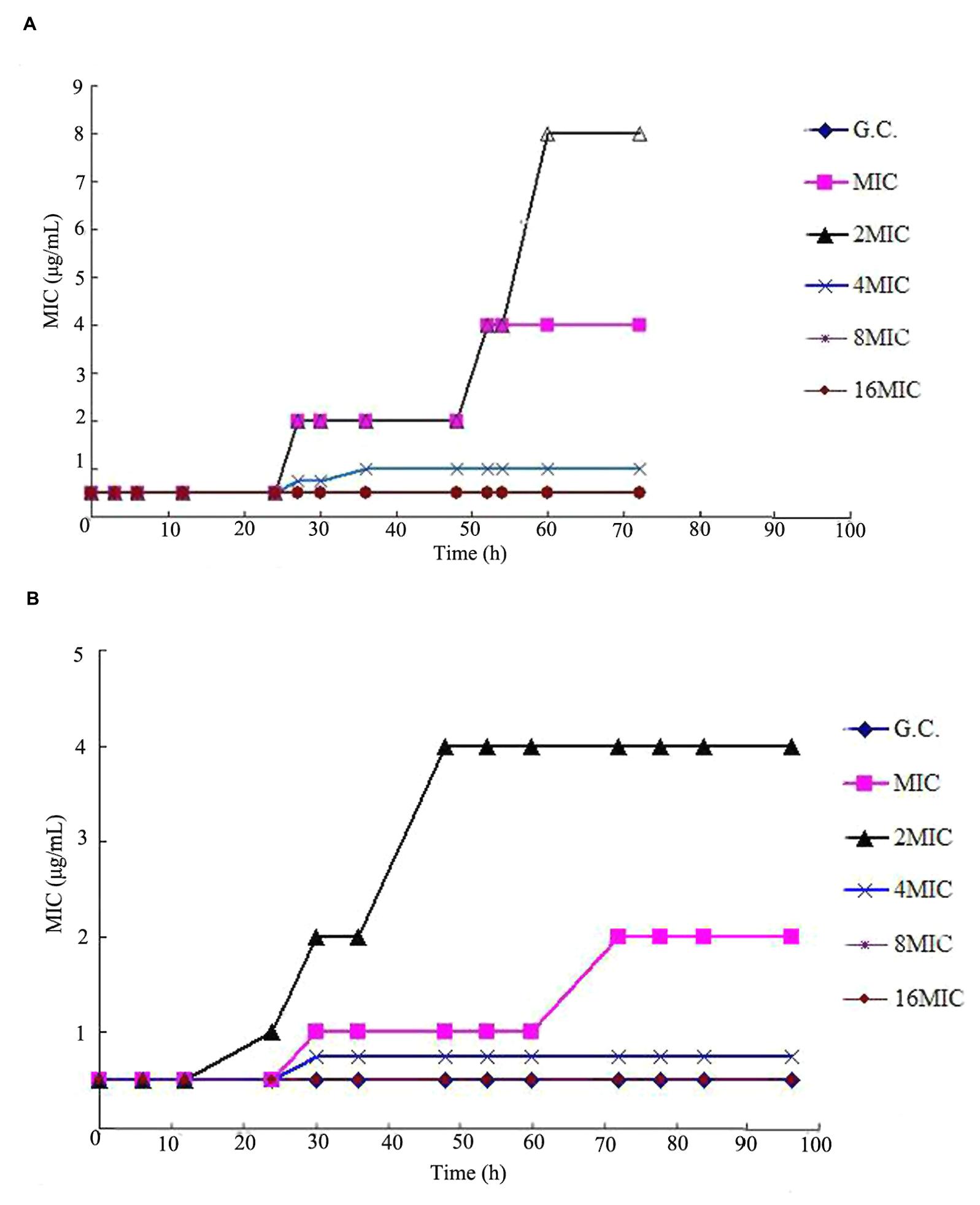
FIGURE 4. Typical susceptibility responses of S. aureus ATCCC 25923 observed in the in vitro PK/PD model simulating the pharmacokinetics of cefquinome with different terminal half-lives (t1/2β).(A) t1/2β = 3 h, (B) t1/2β = 6 h.
Correlation of PK/PD Indices with Resistance Selection
Pharmacokinetic/pharmacodynamic indices, such as AUC24 h/MIC (where AUC24h is the area under the drug concentration time curve in a 24 h interval) and time above the MIC, provide an empirical way to relate antimicrobial dose to favor the treatment effect of bactericidal agents. Relationships between PK/PD indices and loss of susceptibility are shown in Table 1. For cephalosporin, T > MIC was the index most commonly associated with restricting susceptible cell growth. When T > MIC attained 17.14 h or T > MIC% attained 24%, the MIC value of cefquinome against S. aureus increased to 16-fold of initial value at 72 h after multiple administrations in groups with t1/2β of 3 h. Similarly, in groups with t1/2β of 6 h, when T > MIC = 34.28 h or T > MIC% = 48%, the value of cefquinome MIC increased to eightfold of initial value after multiple dosages.
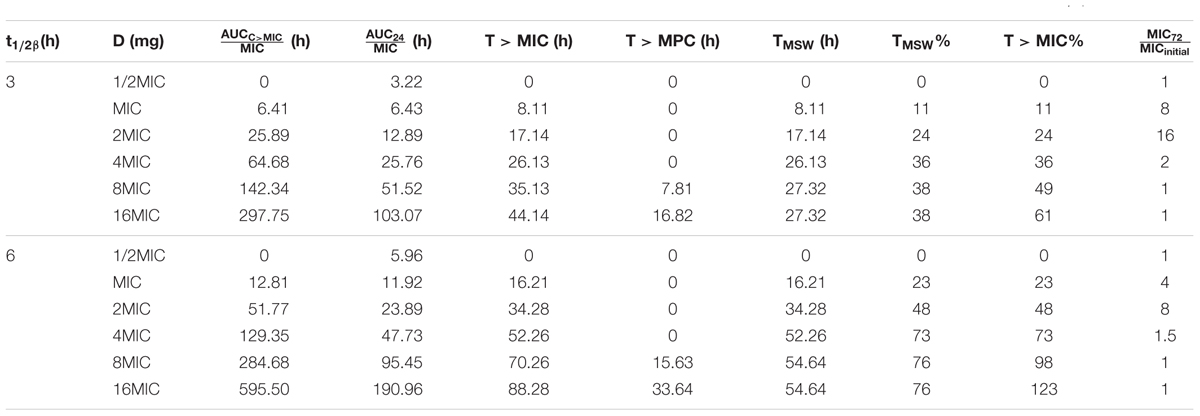
TABLE 1. Pharmacokinetic/pharmacodynamic (PK/PD) indices of antimicrobial efficacy and risk of resistance selection amongst Staphylococcus aureus ATCC 25923 over the complete dosing interval following PK simulations of three consecutive administrations of cefquinome in t1/2β of 3 and 6 h.
Other PK/PD indices also showed correlation with the selection of resistance (Table 1). When TMSW and TMSW% were 17.14 h and 24%, the MIC increased to 16-fold of initial value in groups with t1/2β of 3 h after multiple dosages, and the MIC increased to eightfold of initial value When TMSW and TMSW% were 34.28 h and 48% with t1/2β of 6 h after multiple dosages, respectively.
Correlation Analysis of MIC Increase with TMSW%
According to Gaussian function and regression analysis results, the bacteria was prone to develop resistance when TMSW% was about 20% (x0 = 0.2027) in groups with t1/2β of 3 h after multiple dosages (R2 = 0.9989), and TMSW% was about 40% (x0 = 0.4102) in groups with t1/2β of 6 h after multiple dosages (R2 = 0.9986), respectively, as shown in Figure 5. Those results were consistent with the data from Table 1.
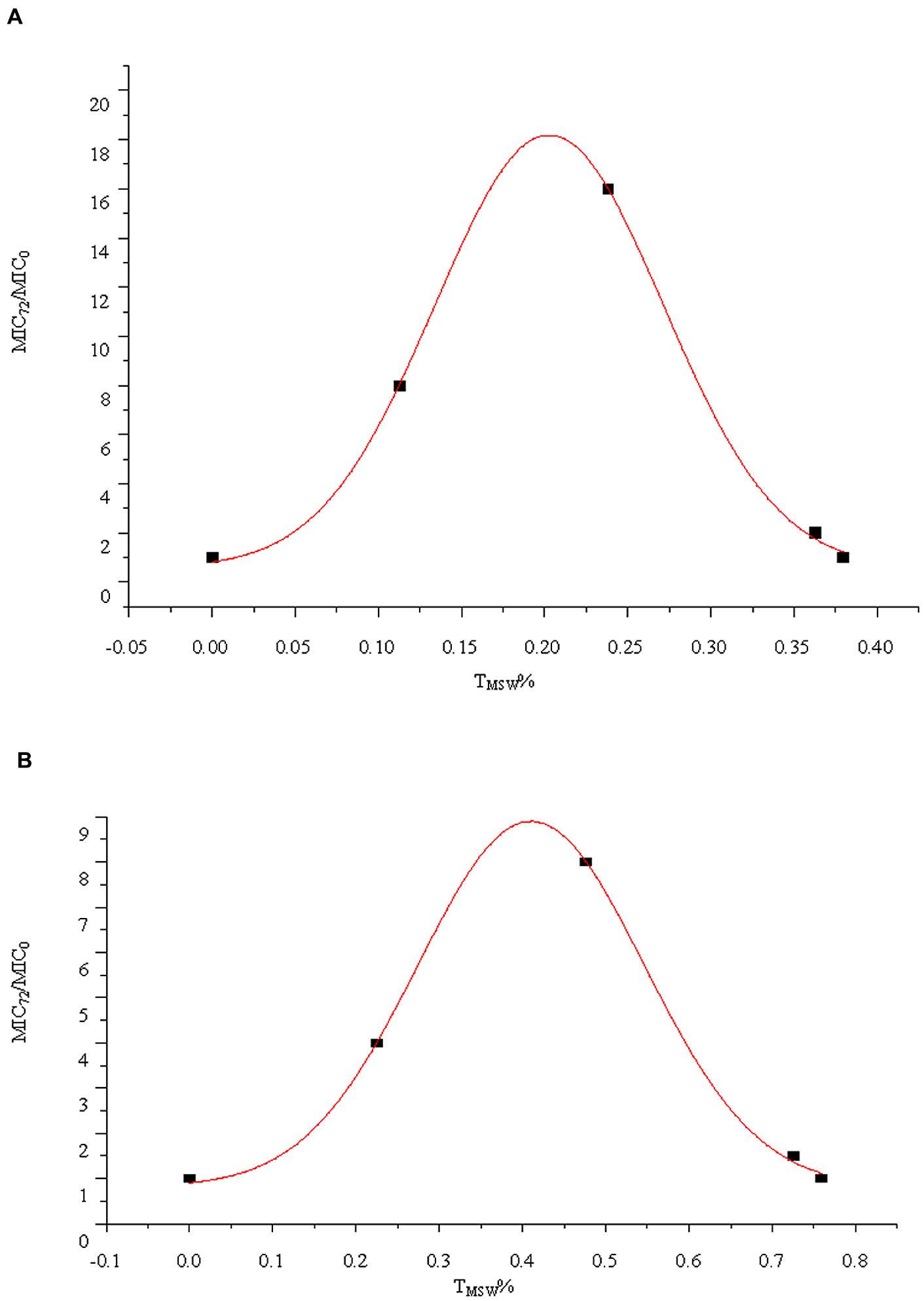
FIGURE 5. Relationship between the TMSW% and MIC72/MIC0 for S. aureus ATCC 25293 exposed to cefquinome in the in vitro model with two terminal half-lives (t1/2β). (A) t1/2β = 3 h, (B) t1/2β = 6 h.
Discussion
Antimicrobial resistance has been a global problem and a great number of strategies have been proposed to slow the emergence of resistance (Stratton, 2003; Levy and Marshall, 2004). In vivo or in vitro PK/PD models have previously been applied to optimize the dosage regimen of various antibiotics in the literature (Croisier et al., 2004b; Ferran et al., 2009; Andraud et al., 2011; Gebru et al., 2012). However, PK/PD model has been renewably used to investigate relationship of bacterial resistance with AUC/MIC, Cmax/MIC and T > MIC, recently. In the present study, we simulated the cefquinome pharmacokinetic profiles with different terminal half-lives using this dynamic model and tried to predict the selection of resistant S. aureus with some indices, such as T > MIC and TMSW%.
It is believed that integration of drug pharmacokinetics, mutant prevention concentration is helpful in slowing the emergence of resistance. In this experiment, the MPC and MSW of cefquinome against S. aureus ATCC25923 were determined. The SI, ratio of MPC to MIC99, is the ability of a drug to select resistant mutants (Zhao and Drlica, 2001). In other word, the bigger the SI is, the easier the drug is to induce resistance. The SI of cefquinome against S. aureus ATCC25923 was 10.24 in our study, which suggested that cefquinome could induce S. aureus resistant mutation easily and effectively.
T > MIC was regarded as a promising predictor for the efficacy of cephalosporins. Previous pharmacodynamic studies have addressed separate issues around the relationships between T > MIC and efficacy for cephalosporins. Craig (1995) has reviewed the data of efficacy for cephalosporins against Enterbacteriaceae, streptococci and S. aureus in several animal infection models and found that the time above MIC required for a bacteriostatic effect against strains of Enterbacteriaceae, Streptococci were generally 60–70%, and 40–50% for S. aureus, respectively. In our study, the value of T > MIC for maximal killing was 40~60% for cefquinome against S. aureus reference strain. In consistent with the widespread idea that antibacterial efficacy of β-lactams depend on T > MIC, the effect of cefquinome against S. aureus in our study exhibited similar correlation. As observed in Figure 3, killing curves of S. aureus exposed to each dose of cefquinome showed the typical pattern of time-dependent bactericidal action. The similar observation was also reported for other β-lactam drugs in previous studies (Lindecrona et al., 2000; Burgess and Hall, 2004). Considering that the T > MIC was one of the most important parameters for optimal dosage regimen, T > MIC was selected as a parameter to evaluate resistant mutation in this article. In the present study, the values of T > MIC ranging from 8.11 to 17.14 h were dangerous zones for inducing resistant mutation in groups with t1/2β of 3 h after multiple dosages, and values ranging from 16.21 to 34.28 h were dangerous zones for inducing resistant mutation in groups with t1/2β of 6 h multiple dosages. Compared these values with previously reported study data that T > MIC value ranged from 42 to 54 h for cefquinome in dairy cows (Zonca et al., 2011), the prevention of selecting resistant S. aureus strains seems to be achieved by the present dosage regimens approved by the European Medicines Agency (EMA) for the treatment of dairy cow mastitis.
TMSW is another important parameter used for evaluating resistant mutation. A previous study conducted in a rabbit lung infection model with Streptococcus pneumonia showed that the selection of resistant bacteria occurred systematically when concentrations of gatifloxacin were within the MSW (TMSW) for more than 45% of the treatment duration (Croisier et al., 2004b). Another experiment in rabbits infected by S. aureus also showed that time in the MSW > 33% was preferable to select mutants (Cui et al., 2006). When the abilities of the indices TMSW, AUC24 h/MIC, Cmax/MIC to predict the selection of resistant bacteria were compared, only TMSW appeared to be a good predictor of the prevention of resistance (Ferran et al., 2009). In this study, TMSW was also used to predict selection of resistant S. aureus against cefquinome. It was clearly determined that when TMSW was above 36% in groups with t1/2β of 3 h after multiple dosages or above 73% in groups with t1/2β of 6 h after multiple dosages, cefquinome could restrict the resistant mutation.
In the present investigation, the bacterial resistant mutation was affected by different terminal half-lives and dosages. Within the group of the same terminal half-life (3 or 6 h), the bacterial resistant mutation happened under the condition of relative low dosage (maximum concentrations were 2MIC and 4MIC). When the maximum concentration was over 8MIC, resistant mutation was prevented. In the same dosages (maximum concentration was 2MIC or 4MIC), the MIC72 h increased from 8- to 16-fold in groups with t1/2β of 3 h after multiple dosages and increased from 4- to 8-fold in groups with t1/2β of 6 h after multiple dosages, respectively. Those results suggested that the bacterial resistant mutation might happen at a low concentration. In high dosages or long terminal half-lives, the drug concentrations might exceed the MPC value, the upper boundary of MSW, and the resistant mutation could be inhibited. The conclusion from this study was consistent with other similar studies (Cui et al., 2006; Ferran et al., 2009; Zhu et al., 2012).
The limitation of the study was that only one reference strain was used to simulate the efficacy of cefquinome in this in vitro model; however, the multi-resistance is becoming an urgent trend all over the world. It may be reasonable that more clinical isolates of S. aureus could be used for further study. Firsov et al. (2008) used two methicillin-resistant strains of S. aureus, ATCC 6538 and ATCC 43300, to study the enrichment of ciprofloxacin resistant mutation in an in vitro dynamic model. Liang et al. (2011), chose three clinical isolates of S. aureus, SA99, RN450 and RN450-A1, to analyze the enrichment of levofloxacin resistant mutation with in vitro dynamic model. From the results obtained in the dynamic model, more clinical isolates could be used to study resistant mutation easily based on the substantial method. Moreover, to our knowledge, evaluation of antimicrobial activity for cefquinome with different terminal half-lives is scarce. Considering the fact that cefquinome is the time-dependent drug, it is positive to study the correlation resistant mutation with different terminal half-life conditions. Another limitation of the current study was the absence of resistance mechanisms of S. aureus observed in vitro. Therefore, the pattern of S. aureus resistant to cefquinome, such as production of β-lactamases, alteration of drug targets, porin-mediated resistance, and/or efflux-mediated resistance, needs confirmation in the further study.
Conclusion
The present study of resistant mutation with S aureus ATCC 25923 exposed to cefquinome with two different terminal half-lives in in vitro dynamic models supports the MSW hypothesis and provides some useful parameters to predict the resistant mutation, especially T > MIC and TMSW%. Further study will concentrate on more clinical isolates which could be used for verification of these results and resistance patterns of S. aureus resistant to cefquinome.
Author Contributions
All authors listed, have made substantial, direct and intellectual contribution to the work, and approved it for publication.
Conflict of Interest Statement
The authors declare that the research was conducted in the absence of any commercial or financial relationships that could be construed as a potential conflict of interest.
Acknowledgments
The authors would like to thank the financial support by the Natural Science Foundation of China (grant number 31172366) and 973 program (grant number 2013CB127200/2013CB127203).
References
Allen, G. P., and Hankins, C. D. (2009). Evaluation of the mutant selection window for fluoroquinolones against Neisseria gonorrhoeae. J. Antimicrob. Chemother. 64, 359–363. doi: 10.1093/jac/dkp172
Allen, G. P., Kaatz, G. W., and Rybak, M. J. (2004). In vitro activities of mutant prevention concentration-targeted concentrations of fluoroquinolones against Staphylococcus aureus in a pharmacodynamic model. Int. J. Antimicrob. Agents 24, 150–160. doi: 10.1016/j.ijantimicag.2004.03.011
Al-Taher, A. (2010). Pharmacokinetics of cefquinome in camels. J. Animal Vet. Adv. 9, 848–852. doi: 10.3923/javaa.2010.848.852
Andraud, M., Chauvin, C., Sanders, P., and Laurentie, M. (2011). Pharmacodynamic modeling of in vitro activity of marbofloxacin against Escherichia coli strains. Antimicrob. Agents Chemother. 55, 756–761. doi: 10.1128/AAC.00865-10
Archer, G. L. (1998). Staphylococcus aureus: a well-armed pathogen. Clin. Infect. Dis. 26, 1179–1181. doi: 10.1086/520289
Azizoglu, R. O., Lyman, R., and Anderson, K. L. (2013). Bovine Staphylococcus aureus: dose response to iodine and chlorhexidine and effect of iodine challenge on antibiotic susceptibility. J. Dairy Sci. 96, 993–999. doi: 10.3168/jds.2012-5857
Blondeau, J., Borsos, S., Blondeau, L., Blondeau, B., and Hesje, C. (2012). Comparative minimum inhibitory and mutant prevention drug concentrations of enrofloxacin, ceftiofur, florfenicol, tilmicosin and tulathromycin against bovine clinical isolates of Mannheimia haemolytica. Vet. Microbiol. 160, 85–90. doi: 10.1016/j.vetmic.2012.05.006
Bronner, S., Murbach, V., Peter, J.-D., Levêque, D., Elkhaïli, H., Salmon, Y., et al. (2002). Ex vivo pharmacodynamics of amoxicillin-clavulanate against β-lactamase-producing Escherichia coli in a Yucatan miniature pig model that mimics human pharmacokinetics. Antimicrob. Agents Chemother. 46, 3782–3789. doi: 10.1128/AAC.46.12.3782-3789.2002
Burgess, D. S., and Hall, R. G. II, (2004). In vitro killing of parenteral beta-lactams against standard and high inocula of extended-spectrum beta-lactamase and non-esbl producing Klebsiella pneumoniae. Diagn. Microbiol. Infect. Dis. 49, 41–46. doi: 10.1016/j.diagmicrobio.2003.11.007
Cavaco, L., Abatih, E., Aarestrup, F. M., and Guardabassi, L. (2008). Selection and persistence of CTX-M-producing Escherichia coli in the intestinal flora of pigs treated with amoxicillin, ceftiofur, or cefquinome. Antimicrob. Agents Chemother. 52, 3612–3616. doi: 10.1128/AAC.00354-08
Clinical Laboratory Standards Institute (CLSI) (2013). Performance Standards for Antimicrobial Disk, and Dilution Susceptibility Tests for Bacteria Isolated From Animals, Approved Standard-Fourth Edn. Wayne, PA: Clinical Laboratory Standards Institute.
Craig, W. A. (1995). Interrelationship between pharmacokinetics and pharmacodynamics in determining dosage regimens for broad-spectrum cephalosporins. Diagn. Microbiol. Infect. Dis. 22, 89–96. doi: 10.1016/0732-8893(95)00053-D
Croisier, D., Etienne, M., Bergoin, E., Charles, P.-E., Lequeu, C., Piroth, L., et al. (2004a). Mutant selection window in levofloxacin and moxifloxacin treatments of experimental pneumococcal pneumonia in a rabbit model of human therapy. Antimicrob. Agents Chemother. 48, 1699–1707. doi: 10.1128/AAC.48.5.1699-1707.2004
Croisier, D., Etienne, M., Piroth, L., Bergoin, E., Lequeu, C., Portier, H., et al. (2004b). In vivo pharmacodynamic efficacy of gatifloxacin against Streptococcus pneumoniae in an experimental model of pneumonia: impact of the low levels of fluoroquinolone resistance on the enrichment of resistant mutants. J. Antimicrob. Chemother. 54, 640–647. doi: 10.1093/jac/dkh393
Cui, J., Liu, Y., Wang, R., Tong, W., Drlica, K., and Zhao, X. (2006). The mutant selection window in rabbits infected with Staphylococcus aureus. J. Infect. Dis. 194, 1601–1608. doi: 10.1086/508752
Dong, Y., Zhao, X., Domagala, J., and Drlica, K. (1999). Effect of fluoroquinolone concentration on selection of resistant mutants of Mycobacterium bovis BCG and Staphylococcus aureus. Antimicrob. Agents Chemother. 43, 1756–1758.
Dumka, V. K., Dinakaran, V., Ranjan, B., and Rampal, S. (2013). Comparative pharmacokinetics of cefquinome following intravenous and intramuscular administration in goats. Small Ruminant Res. 113, 273–277. doi: 10.1016/j.smallrumres.2013.02.010
Ferran, A., Dupouy, V., Toutain, P.-L., and Bousquet-Mélou, A. (2007). Influence of inoculum size on the selection of resistant mutants of Escherichia coli in relation to mutant prevention concentrations of marbofloxacin. Antimicrob. Agents Chemother. 51, 4163–4166. doi: 10.1128/AAC.00156-07
Ferran, A. A., Kesteman, A.-S., Toutain, P.-L., and Bousquet-Mélou, A. (2009). Pharmacokinetic/pharmacodynamic analysis of the influence of inoculum size on the selection of resistance in Escherichia coli by a quinolone in a mouse thigh bacterial infection model. Antimicrob. Agents Chemother. 53, 3384–3390. doi: 10.1128/AAC.01347-08
Firsov, A. A., Lubenko, I. Y., Smirnova, M. V., Strukova, E. N., and Zinner, S. H. (2008). Enrichment of fluoroquinolone-resistant Staphylococcus aureus: oscillating ciprofloxacin concentrations simulated at the upper and lower portions of the mutant selection window. Antimicrob. Agents Chemother. 52, 1924–1928. doi: 10.1128/AAC.01371-07
Gebru, E., Damte, D., Choi, M.-J., Lee, S.-J., Kim, Y.-H., and Park, S. C. (2012). Mutant prevention concentration and phenotypic and molecular basis of fluoroquinolone resistance in clinical isolates and in vitro-selected mutants of Escherichia coli from dogs. Vet. Microbiol. 154, 384–394. doi: 10.1016/j.vetmic.2011.07.033
Grasso, S., Meinardi, G., De Carneri, I., and Tamassia, V. (1978). New in vitro model to study the effect of antibiotic concentration and rate of elimination on antibacterial activity. Antimicrob. Agents Chemother. 13, 570–576. doi: 10.1128/AAC.13.4.570
Guardabassi, L., Schwarz, S., and Lloyd, D. H. (2004). Pet animals as reservoirs of antimicrobial-resistant bacteria review. J. Antimicrob. Chemother. 54, 321–332. doi: 10.1093/jac/dkh332
Harris, S. R., Feil, E. J., Holden, M. T., Quail, M. A., Nickerson, E. K., Chantratita, N., et al. (2010). Evolution of MRSA during hospital transmission and intercontinental spread. Science 327, 469–474. doi: 10.1126/science.1182395
Le Loir, Y., Baron, F., and Gautier, M. (2003). Staphylococcus aureus and food poisoning. Genet. Mol. Res. 2, 63–76.
Levy, S. B., and Marshall, B. (2004). Antibacterial resistance worldwide: causes, challenges and responses. Nat. Med. 10, S122–S129. doi: 10.1038/nm1145
Li, X., Wu, W., Su, D., Wang, Z., Jiang, H., and Shen, J. (2008). Pharmacokinetics and bioavailability of cefquinome in healthy piglets. J. Vet. Pharmacol. Ther. 31, 523–527. doi: 10.1111/j.1365-2885.2008.00989.x
Liang, B., Bai, N., Cai, Y., Wang, R., Drlica, K., and Zhao, X. (2011). Mutant prevention concentration-based pharmacokinetic/pharmacodynamic indices as dosing targets for suppressing the enrichment of levofloxacin-resistant subpopulations of Staphylococcus aureus. Antimicrob. Agents Chemother. 55, 2409–2412. doi: 10.1128/AAC.00975-10
Limbert, M., Isert, D., Klesel, N., Markus, A., Seeger, K., Seibert, G., et al. (1991). Antibacterial activities in vitro and in vivo and pharmacokinetics of cefquinome (HR 111V), a new broad-spectrum cephalosporin. Antimicrob. Agents Chemother. 35, 14–19. doi: 10.1128/AAC.35.1.14
Lindecrona, R., Friis, C., and Aarestrup, F. M. (2000). The pharmacodynamic effect of amoxicillin and danofloxacin against Salmonella typhimurium in an in-vitro pharmacodynamic model. Res. Vet. Sci. 68, 261–264. doi: 10.1053/rvsc.2000.0374
Lowy, F. D. (1998). Staphylococcus aureus infections. New Engl. J. Med. 339, 520–532. doi: 10.1056/NEJM199808203390806
Mohammed Fayaz, A., Girilal, M., Mahdy, S. A., Somsundar, S., Venkatesan, R., and Kalaichelvan, P. (2011). Vancomycin bound biogenic gold nanoparticles: a different perspective for development of anti VRSA agents. Process Biochem. 46, 636–641. doi: 10.1016/j.procbio.2010.11.001
Stratton, C. W. (2003). Dead bugs don’t mutate: susceptibility issues in the emergence of bacterial resistance. Emerg. Infect. Dis. 9:10. doi: 10.3201/eid0901.020172
Tauxe, R. V. (2002). Emerging foodborne pathogens. Int. J. Food Microbiol. 78, 31–41. doi: 10.1016/S0168-1605(02)00232-5
Tohamy, M. (2011). Age-related intramuscular pharmacokinetics of cefquinome in sheep. Small Ruminant Res. 99, 72–76. doi: 10.1016/j.smallrumres.2011.03.004
Wang, L., Yuanshu, Z., Yuhan, Z., and Yingxia, L. (2010). Mutant prevention concentrations of fluoroquinolones against Campylobacter jejuni isolated from chicken. Vet. Microbiol. 144, 409–414. doi: 10.1016/j.vetmic.2010.02.020
Yuan, L., Sun, J., Wang, R., Sun, L., Zhu, L., Luo, X., et al. (2011). Pharmacokinetics and bioavailability of cefquinome in healthy ducks. Am. J. Vet. Res. 72, 122–126. doi: 10.2460/ajvr.72.1.122
Zhao, X., and Drlica, K. (2001). Restricting the selection of antibiotic-resistant mutants: a general strategy derived from fluoroquinolone studies. Clin. Infect. Dis. 33, S147–S156. doi: 10.1086/321841
Zhao, X., and Drlica, K. (2002). Restricting the selection of antibiotic-resistant mutant bacteria: measurement and potential use of the mutant selection window. J. Infect. Dis. 185, 561–565. doi: 10.1086/338571
Zhu, Y.-L., Hu, L.-F., Mei, Q., Cheng, J., Liu, Y.-Y., Ye, Y., et al. (2012). Testing the mutant selection window in rabbits infected with methicillin-resistant Staphylococcus aureus exposed to vancomycin. J. Antimicrob. Chemother. 67, 2700–2706. doi: 10.1093/jac/dks280
Keywords: cefquinome, Staphylococcus aureus, PK/PD, MPC, in vitro model
Citation: Li Y, Feng B, Gu X, Yang D, Zeng Z, Zhang B and Ding H (2016) Correlation of PK/PD Indices with Resistance Selection for Cefquinome against Staphylococcus aureus in an In Vitro Model. Front. Microbiol. 7:466. doi: 10.3389/fmicb.2016.00466
Received: 30 August 2015; Accepted: 21 March 2016;
Published: 12 April 2016.
Edited by:
Alexandre Gonçalves, Universidade de Trás-os-Montes e Alto Douro, PortugalReviewed by:
Dmitri Debabov, NovaBay Pharmaceuticals, Inc., USAWilliam Farias Porto, Universidade Católica de Brasília, Brazil
Copyright © 2016 Li, Feng, Gu, Yang, Zeng, Zhang and Ding. This is an open-access article distributed under the terms of the Creative Commons Attribution License (CC BY). The use, distribution or reproduction in other forums is permitted, provided the original author(s) or licensor are credited and that the original publication in this journal is cited, in accordance with accepted academic practice. No use, distribution or reproduction is permitted which does not comply with these terms.
*Correspondence: Huanzhong Ding, hzding@scau.edu.cn
†These authors have contributed equally to this work.