- 1SIBS-UGENT-SJTU Joint Laboratory of Mycotoxin Research, Key Laboratory of Food Safety Research, Institute for Nutritional Sciences, Shanghai Institutes for Biological Sciences, Chinese Academy of Sciences, Shanghai, China
- 2College of Plant Science and Technology, Huazhong Agricultural University, Wuhan, China
- 3Academy of State Administration of Grain, Beijing, China
Mycotoxins, as microbial secondary metabolites, frequently contaminate cereal grains and pose a serious threat to human and animal health around the globe. Deoxynivalenol (DON), a commonly detected Fusarium mycotoxin, has drawn utmost attention due to high exposure levels and contamination frequency in the food chain. Biological control is emerging as a promising technology for the management of DON contamination. Functional biological control agents (BCAs), which include antagonistic microbes, natural fungicides derived from plants and detoxification enzymes, can be used to control DON contamination at different stages of grain production. In this review, studies regarding different biological agents for DON control in recent years are summarized for the first time. Furthermore, this article highlights the significance of BCAs for controlling DON contamination, as well as the need for more practical and efficient BCAs concerning food safety.
Introduction
Mycotoxins are secondary metabolites produced by fungi and may exert toxic effects on plants, animals and humans (Goswami and Kistler, 2004; Yu and Keller, 2005). Trichothecenes, a group of sesquiterpenoid mycotoxins, are commonly found in grains worldwide. B type trichothecenes that are common contaminants of grains, are characterized by a keto functional group at C-8 in their molecular structures (Richard, 2007; McCormick et al., 2011; Mohamed Anwar et al., 2014). Some common B type trichothecenes include deoxynivalenol (DON), 3-acetyldeoxynivalenol (3ADON), 15-acetyldeoxynivalenol (15ADON), nivalenol (NIV) and fusarenon X (FUSX) (Figure 1A) (Arunachalam and Doohan, 2013). Among them, DON, also known as vomitoxin, is the most frequently detected and economically important mycotoxin in cereal grains. DON contamination in cereal grains is a global problem. DON is predominantly produced by Fusarium graminearum and Fusarium culmorum. These phytopathogens can infect crops in the field and cause a destructive disease called Fusarium head blight (FHB) or scab (McMullen et al., 2012). As a virulence factor of these phytopathogens, mycotoxin DON facilitates the spread of Fusarium strains within infected tissue and contributes to the symptoms of FHB disease (Bai et al., 2002).
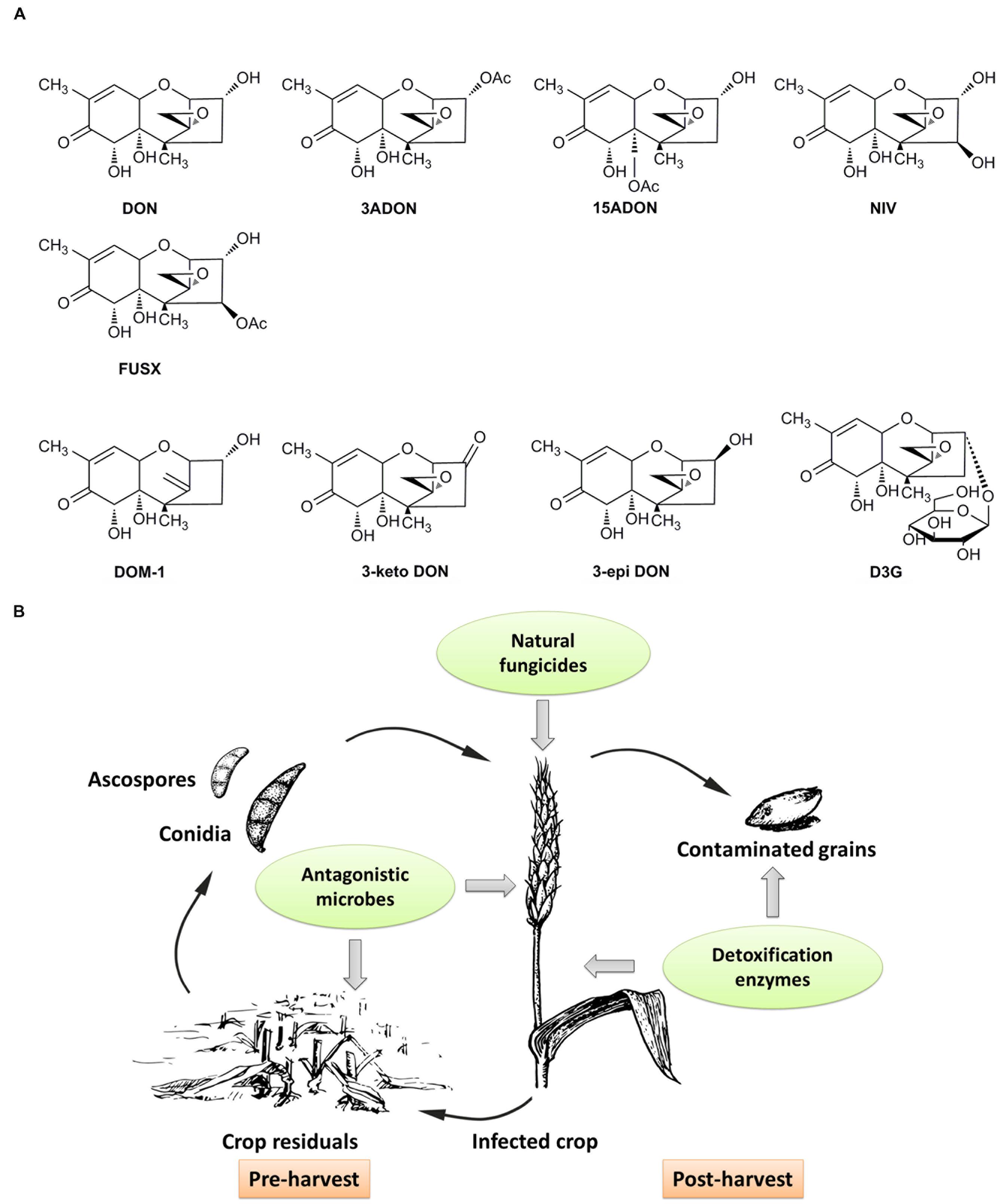
FIGURE 1. Chemical structures of major type B trichothecenes and the detoxification products of DON, and a schematic of BCAs on control of DON contamination at different stages of grain production. (A) Major B type trichothecenes include DON, 3ADON, 15ADON, NIV and FUSX. Detoxification products of DON mainly include DOM-1, 3-keto DON, 3-epi DON and D3G. (B) Antagonistic microbes can be applied to crop residuals to inhibit sporulation, or to spikes with natural fungicides to inhibit the growth and DON production of pathogens. Contaminated grains can be treated with enzymes to detoxification after harvest, and the enzymes also can be expressed in genetically modified crops to detoxify DON and increase crop resistance to pathogens.
FHB caused by Fusarium strains can lead to enormous losses of yield and quality in cereal grains. Moreover, DON contamination poses a great threat to public health and food safety (Wagacha and Muthomi, 2008). Consumption of DON-contaminated food can cause serious gastroenteritis including diarrhea, nausea, vomition, and abdominal pain in humans (Pestka, 2010; Sobrova et al., 2010; Pinton et al., 2012; da Rocha et al., 2014). Thus, DON contamination control is a crucial issue for mitigating economic losses and improving food safety in the food chain. Currently, some effective measures including crop rotation, selection of resistant wheat lines, fungicides application and biological control agents (BCAs) have been put into action to control DON contamination in grain production (Dill-Macky and Jones, 2000; Edwards and Godley, 2010; Wegulo et al., 2015). Of the measures mentioned above, application of synthetic fungicides is relatively successful for control of these phytopathogens and mycotoxin production. However, synthetic fungicides are not economical for long-term use, as well as causing a series of undesirable effects on the environment (Mesterhazy et al., 2011; Schoneberg et al., 2015). Biological control of DON contamination is emerging as a green approach. Functional BCAs include antagonistic microbes, natural fungicides derived from plants which inhibit the development and mycotoxin production, and enzymes from beneficial organisms for DON detoxification after production. Until now, several reviews on managing FHB or mycotoxins detoxification with different strategies have been published (Yuen and Schoneweis, 2007; Awad et al., 2010; He et al., 2010; Karlovsky, 2011; McCormick, 2013; Wegulo et al., 2015). Here we are focusing on recent progresses in various BCAs to achieve DON contamination control (Table 1), which will be reviewed briefly in the next section. This will be beneficial to understand the exploration and application of BCAs in the field of DON contamination control.
Functional BCAs Against DON Contamination
Antagonistic Microbes
Previous results have demonstrated the positive linear relationship between the occurrence of FHB and DON contamination (Wegulo et al., 2011; Hernandez Nopsa et al., 2012; Wegulo, 2012). Therefore, managing FHB plays a key role in controlling DON contamination (Yuen and Schoneweis, 2007). Selection of available antagonistic microbes that suppress mycelia growth, sporulation and mycotoxin production of pathogens is indispensable for the purpose of managing FHB (Pfliegler et al., 2015), of which antagonistic fungi and bacteria have gained significant attention in the past.
Major fungal antagonists comprise Trichoderma strains, Clonostachys rosea, Cladosporium cladosporioides (Schoneberg et al., 2015), Aureobasidium pullulans (Wachowska and Głowacka, 2014) and Cryptococcus strains (Schisler et al., 2011). Trichoderma strains have been widely investigated, because they grow fast as competitors to significantly reduce the colony areas of Fusarium strains, and inhibit the pathogen spread by antibiotic production (Matarese et al., 2012; Schoneberg et al., 2015). Another important control mechanism is mycoparasitism mediated by production of cell wall degrading enzymes including cellulases, chitinase and glucanases (Vinale et al., 2008; Mukherjee et al., 2013). On the other hand, during competition between Fusarium and Trichoderma, DON production, as a negative signal against antagonism, can repress one chitinase gene (nag1) expression in a Trichoderma atroviride strain P1 (Lutz et al., 2003).
Bacterial strains in the genus of Bacillus and Pseudomonas have also been widely explored as potential BCAs against FHB in recent years (Yoshida et al., 2012). Most antagonistic bacterial strains belong to endophytic microbes inhabiting plant or rhizosphere without leading to diseases or adverse effects (Dal Bello et al., 2002). Bacillus subtilis SG6 isolated from wheat anthers showed a remarkable inhibitory effect on mycelial growth, sporulation and DON production of F. graminearum (Zhao et al., 2014). Moreover, B. subtilis RC 218 and Brevibacillus sp. RC 263 isolated from wheat anthers could effectively reduce the incidence and severity of FHB and DON accumulation under semi controlled field conditions (Palazzini et al., 2016). In another study, bacterial strains isolated from peanut shells exhibited potent inhibition to the growth and DON production of F. graminearum, and the tested strains with the strongest inhibitory effect were identified as B. amyloliquefaciens (Shi et al., 2014). Besides, a Shewanella algae strain YM8 isolated from sea sediment, which can produce volatile organic compounds, has a broad spectrum of inhibition activity against nine agronomically important phytopathogens including F. graminearum (Gong et al., 2015). This research indicates that marine bacteria can be a potential source for effective agents to control the growth and mycotoxin production of pathogens in the field and during storage.
The above mentioned antagonistic microbes can be applied to crop residuals to inhibit ascospores and conidia production, or directly used on spikes to restrict the development and mycotoxin production of pathogens (Figure 1B) (Xue et al., 2014; Schoneberg et al., 2015; Wegulo et al., 2015).
Natural Fungicides
In order to decrease the use of synthetic fungicides, a green alternative strategy with natural fungicides can be used to inhibit pathogens (da Cruz Cabral et al., 2013). The restriction on applications of chemical fungicides has increased the demand of natural fungicides (Terzi et al., 2014). As potential sources of natural fungicides, metabolites from plants, including phenolic compounds and essential oils, have been researched for activities that inhibit pathogen development and mycotoxin production in recent years (Esper et al., 2014; Pagnussatt et al., 2014).
Phenolic compounds derived from Spirulina strains exerted efficient antifungal activity against F. graminearum (Pagnussatt et al., 2013, 2014). Moreover, a recent work has indicated that chlorogenic acid, a common phenolic acid, can be transformed by F. graminearum, generating some forms of metabolites which are even more efficient in limiting mycelial growth and DON production (Gauthier et al., 2016). This study provides a new understanding on the role of phenolic compounds in their antifungal activities.
Essential oils extracted from plants usually contain some antimicrobial or antioxidant compounds, and they are regarded as good choices of natural fungicides (Bakkali et al., 2008). For instance, essential oils extracted from cinnamon, clove, oregano, palmarosa and lemongrass were selected to test their anti-mycotoxigenic activity. All these essential oils could prevent DON accumulation in F. graminearum-infected grains, and the clove essential oil was the most effective (Marín et al., 2004). In addition, another study found that environmental factors, such as water activity and temperature, could influence the anti-mycotoxigenic activity of essential oils (Velluti et al., 2004). Recent research shows that Ocimum sanctum essential oil has a prominent antagonistic activity on the growth of F. graminearum (Kalagatur et al., 2015). All these studies show that natural fungicides that are sourced from functional plant metabolites have great potentials in controlling DON contamination.
Detoxification Enzymes
As complementary to management of the incidence and severity of FHB by antagonistic microbes and natural fungicides, detoxification of DON in contaminated grains could also reduce food safety risk and economic losses effectively (Awad et al., 2010; Jard et al., 2011; Karlovsky, 2011; McCormick, 2013). A number of conventional physical and chemical approaches have been used to remove DON from contaminated grains, but the loss of nutritional value or potential safety problems should not be ignored (He et al., 2010). Therefore, detoxifying DON by enzymatic reactions can be an attractive approach for controlling DON contamination.
Enzymatic reactions of DON detoxification may include deepoxidation, oxidation, epimerization and glycosylation. The detoxification products from these reactions, such as DOM-1, 3-keto DON, 3-epi DON and DON-3-glucoside (D3G), are shown in Figure 1A. In addition, some studies with unknown detoxification products were also reported. For example, B. licheniformis and B. subtilis strains were proved to degrade DON under anaerobic conditions, but the detoxification products in this transformation remained unknown (Cheng et al., 2010). A strain of Aspergillus (NJA1) isolated from soil could convert DON to an unknown product with a molecule weight of 18.1 kDa (He et al., 2008). In another study, strains of Rhizopus oryzae and Aspergillus oryzae can degrade DON in submerged fermentation, but the degradation mechanism was mainly explained by toxin absorption (Garda-Buffon and Badiale-Furlong, 2010). Therefore, deepoxidation, oxidation, epimerization and glycosylation are available enzymatic detoxification processes.
Deepoxidation
The active epoxide group in DON determines its toxicity for interrupting protein synthesis. DON can be deepoxidated to deepoxy DON (DOM-1) which is much less toxic. This process exhibits a promising detoxification approach in contaminated grains (Karlovsky, 2011; Li et al., 2011). Bacteria from the intestines of chicken could convert DON to DOM-1 under oxygen free conditions (Young et al., 2007). In aerobic conditions, a Bacillus strain isolated from intestinal track of fish can also deepoxidate DON in contaminated corn (Guan et al., 2009). A mixed microbial culture including six bacterial genera found in soil was capable of converting DON to DOM-1 under aerobic conditions with a higher transformation efficiency compared to anaerobic conditions (Islam et al., 2012). Interestingly, the human fecal microbiota from one volunteer in an experiment were found to detoxify DON to DOM-1, although the efficiency was relatively low (Gratz et al., 2013).
Oxidation and Epimerization
With the aid of bacteria, other detoxification processes converting DON into low-toxic products, such as oxidation of DON to 3-keto DON and epimerization of DON to 3-epi DON, have been reported (McCormick, 2013). For instance, the Gram-positive genus Nocardioides and the Gram-negative genus Devosia could achieve the detoxification processes (Ikunaga et al., 2011; Sato et al., 2012). A recent study reported that a bacterium Devosia mutans 17-2-E-8 could completely detoxify DON into 3-epi DON and 3-keto DON, and 3-epi DON was the major product, meanwhile the authors confirmed that 3-epi DON was much less toxic than DON by both in vitro and in vivo studies (He et al., 2015).
Glycosylation
Mycotoxin glycosides known as detoxification products in plants, are generally termed as masked mycotoxin, since their conjugate structures may escape routine detection by conventional analytical methods. Plants have the capacity to detoxify harmful compounds like mycotoxin by conjugation with sugars (Berthiller et al., 2007). The first UDP-glucosyltransferase (DOGT1) that can convert DON to D3G was identified from Arabidopsis thaliana in 2003 (Poppenberger et al., 2003). It has been verified that resistant wheat lines are capable of converting more DON to D3G, so the D3G/DON ratios of different wheat lines could give a clear indication of their resistance against DON (Cirlini et al., 2013). Results have illustrated that it is a feasible way to convert DON to D3G in transgenic cereal crops by a high-efficiency and stable UDP-glucosyltransferase to against DON contamination (Lulin et al., 2010; Schweiger et al., 2010; Shin et al., 2012; Li et al., 2015).
Generally, the detoxification enzymes can be applied after harvest to manage contaminated cereal grains, or be expressed in genetically modified crops by transgenic technologies to detoxify DON in infected grains and increase crop resistance against pathogens (He et al., 2010). It is expected that these approaches will be of great significance to reduce DON contamination in years to come.
Conclusion and Perspectives
Functional BCAs offer alternative strategies to control DON contamination in a green and environment-friendly way. As an emerging technique, biological control including the application of beneficial organisms and their functional products such as enzymes or metabolites, has gained more and more attention in recent years (Vinale et al., 2008). In this review, we summarize different types of functional BCAs used to achieve control of DON contamination (Figure 1B). These control strategies mainly include prevention before harvest and detoxification after harvest. What is more, for the purpose of controlling DON contamination, it seems more effective to integrate all available BCAs flexibly throughout grain production and storage.
Researches on DON contamination control by BCAs are still developing and ongoing, since few of them are commercially available (Wegulo et al., 2015). More in-depth studies should be conducted in this field. For instance, the stability and toxicity of detoxified DON should be studied and assessed for providing food safety assurance. The detoxification mechanisms need further investigation as well. With regard to antagonistic microbes and natural fungicides, laboratory-scale studies are insufficient, so systematic field tests should be carried out to establish a comprehensive safety evaluation. In addition, with the rapid development of molecular biology and transgenic techniques, there is a need to seek and identify the genes coding effective and applicable detoxification enzymes in both microbes and plants. And detoxification genes and related enzyme products could be modified in a highly efficient, stable and safe way. With the development of emerging BCAs, there is no doubt that the application of biological control would be a promising strategy to control DON contamination in cereal grains and reduce the risk of food safety in the food chain.
Author Contributions
AW and YT designed the work plan of this review and initiated it, and YT drafted this review with YLT, NL, CS, YL, and SW. AW and YT reviewed the final version before submission.
Conflict of Interest Statement
The authors declare that the research was conducted in the absence of any commercial or financial relationships that could be construed as a potential conflict of interest.
Acknowledgments
This work was supported by the Public Science and Technology Research Funds Projects of State Grains Bureau (No. 201313005-01-2), National Basic Research Program of China (Grant 2013CB127801) and National Natural Science Foundation of China (31471661).
References
Arunachalam, C., and Doohan, F. M. (2013). Trichothecene toxicity in eukaryotes: cellular and molecular mechanisms in plants and animals. Toxicol. Lett. 217, 149–158. doi: 10.1016/j.toxlet.2012.12.003
Awad, W. A., Ghareeb, K., Böhm, J., and Zentek, J. (2010). Decontamination and detoxification strategies for the Fusarium mycotoxin deoxynivalenol in animal feed and the effectiveness of microbial biodegradation. Food Addit. Contam. Part A Chem. Anal. Control Expo. Risk Assess. 27, 510–520. doi: 10.1080/19440040903571747
Bai, G.-H., Desjardins, A., and Plattner, R. (2002). Deoxynivalenol-nonproducing Fusarium graminearum causes initial infection, but does not cause Disease spread in wheat spikes. Mycopathologia 153, 91–98. doi: 10.1023/A:1014419323550
Bakkali, F., Averbeck, S., Averbeck, D., and Idaomar, M. (2008). Biological effects of essential oils – a review. Food Chem. Toxicol. 46, 446–475. doi: 10.1016/j.fct.2007.09.106
Berthiller, F., Lemmens, M., Werner, U., Krska, R., Hauser, M., Adam, G., et al. (2007). Short review: metabolism of the Fusarium mycotoxins deoxynivalenol and zearalenone in plants. Mycotoxin Res. 23, 68–72. doi: 10.1007/BF02946028
Cheng, B., Wan, C., Yang, S., Xu, H., Wei, H., Liu, J., et al. (2010). Detoxification of deoxynivalenol by Bacillus strains. J. Food Safety 30, 599–614. doi: 10.1111/j.1745-4565.2010.00228.x
Cirlini, M., Generotti, S., Dall’Erta, A., Lancioni, P., Ferrazzano, G., Massi, A., et al. (2013). Durum wheat (Triticum Durum Desf.) lines show different abilities to form masked mycotoxins under greenhouse conditions. Toxins 6, 81–95. doi: 10.3390/toxins6010081
da Cruz Cabral, L., Fernández Pinto, V., and Patriarca, A. (2013). Application of plant derived compounds to control fungal spoilage and mycotoxin production in foods. Int. J. Food Microbiol. 166, 1–14. doi: 10.1016/j.ijfoodmicro.2013.05.026
da Rocha, M. E. B., Freire, F. D. C. O., Maia, F. E. F., Guedes, M. I. F., and Rondina, D. (2014). Mycotoxins and their effects on human and animal health. Food Control 36, 159–165. doi: 10.1016/j.foodcont.2013.08.021
Dal Bello, G., Monaco, C., and Simon, M. (2002). Biological control of seedling blight of wheat caused by Fusarium graminearum with beneficial rhizosphere microorganisms. World J. Microbiol. Biotechnol. 18, 627–636. doi: 10.1023/A:1016898020810
Dill-Macky, R., and Jones, R. (2000). The effect of previous crop residues and tillage on Fusarium head blight of wheat. Plant Dis. 84, 71–76. doi: 10.1094/PDIS.2000.84.1.71
Edwards, S. G., and Godley, N. P. (2010). Reduction of Fusarium head blight and deoxynivalenol in wheat with early fungicide applications of prothioconazole. Food Addit. Contam. Part A Chem. Anal. Control Expo. Risk Assess. 27, 629–635. doi: 10.1080/19440040903515942
Esper, R. H., Goncalez, E., Marques, M. O., Felicio, R. C., and Felicio, J. D. (2014). Potential of essential oils for protection of grains contaminated by aflatoxin produced by Aspergillus flavus. Front. Microbiol. 5:269. doi: 10.3389/fmicb.2014.00269
Garda-Buffon, J., and Badiale-Furlong, E. (2010). Kinetics of deoxynivalenol degradation by Aspergillus oryzae and Rhizopus oryzae in submerged fermentation. J. Braz. Chem. Soc. 21, 710–714. doi: 10.1590/S0103-50532010000400018
Gauthier, L., Bonnin-Verdal, M.-N., Marchegay, G., Pinson-Gadais, L., Ducos, C., Richard-Forget, F., et al. (2016). Fungal biotransformation of chlorogenic and caffeic acids by Fusarium graminearum: new insights in the contribution of phenolic acids to resistance to deoxynivalenol accumulation in cereals. Int. J. Food Microbiol. 221, 61–68. doi: 10.1016/j.ijfoodmicro.2016.01.005
Gong, A.-D., Li, H.-P., Shen, L., Zhang, J.-B., Wu, A.-B., He, W.-J., et al. (2015). The Shewanella algae strain YM8 produces volatiles with strong inhibition activity against Aspergillus pathogens and aflatoxins. Front. Microbiol. 6:1091. doi: 10.3389/fmicb.2015.01091
Goswami, R. S., and Kistler, H. C. (2004). Heading for disaster: Fusarium graminearum on cereal crops. Mol. Plant Pathol. 5, 515–525. doi: 10.1111/j.1364-3703.2004.00252.x
Gratz, S. W., Duncan, G., and Richardson, A. J. (2013). The human fecal microbiota metabolizes deoxynivalenol and deoxynivalenol-3-glucoside and may be responsible for urinary deepoxy-deoxynivalenol. Appl. Environ. Microbiol. 79, 1821–1825. doi: 10.1128/AEM.02987-12
Guan, S., He, J., Young, J. C., Zhu, H., Li, X.-Z., Ji, C., et al. (2009). Transformation of trichothecene mycotoxins by microorganisms from fish digesta. Aquaculture 290, 290–295. doi: 10.1016/j.aquaculture.2009.02.037
He, C., Fan, Y., Liu, G., and Zhang, H. (2008). Isolation and identification of a strain of Aspergillus tubingensis with deoxynivalenol biotransformation capability. Int. J. Mol. Sci. 9, 2366–2375. doi: 10.3390/ijms9122366
He, J., Zhou, T., Young, J. C., Boland, G. J., and Scott, P. M. (2010). Chemical and biological transformations for detoxification of trichothecene mycotoxins in human and animal food chains: a review. Trends Food Sci. Technol. 21, 67–76. doi: 10.1016/j.tifs.2009.08.002
He, J. W., Bondy, G. S., Zhou, T., Caldwell, D., Boland, G. J., and Scott, P. M. (2015). Toxicology of 3-epi-deoxynivalenol, a deoxynivalenol-transformation product by Devosia mutans 17-2-E-8. Food Chem. Toxicol. 84, 250–259. doi: 10.1016/j.fct.2015.09.003
Hernandez Nopsa, J. F., Baenziger, P. S., Eskridge, K. M., Peiris, K. H., Dowell, F. E., Harris, S. D., et al. (2012). Differential accumulation of deoxynivalenol in two winter wheat cultivars varying in FHB phenotype response under field conditions. Can. J. Plant Pathol. 34, 380–389. doi: 10.1080/07060661.2012.695751
Ikunaga, Y., Sato, I., Grond, S., Numaziri, N., Yoshida, S., Yamaya, H., et al. (2011). Nocardioides sp. strain WSN05-2, isolated from a wheat field, degrades deoxynivalenol, producing the novel intermediate 3-epi-deoxynivalenol. Appl. Microbiol. Biotechnol. 89, 419–427. doi: 10.1007/s00253-010-2857-z
Islam, R., Zhou, T., Young, J. C., Goodwin, P. H., and Pauls, K. P. (2012). Aerobic and anaerobic de-epoxydation of mycotoxin deoxynivalenol by bacteria originating from agricultural soil. World J. Microbiol. Biotechnol. 28, 7–13. doi: 10.1007/s11274-011-0785-4
Jard, G., Liboz, T., Mathieu, F., Guyonvarc’h, A., and Lebrihi, A. (2011). Review of mycotoxin reduction in food and feed: from prevention in the field to detoxification by adsorption or transformation. Food Addit. Contam. Part A Chem. Anal. Control Expo. Risk Assess. 28, 1590–1609. doi: 10.1080/19440049.2011.595377
Kalagatur, N. K., Mudili, V., Siddaiah, C., Gupta, V. K., Natarajan, G., Sreepathi, M. H., et al. (2015). Antagonistic activity of Ocimum sanctum L. essential oil on growth and zearalenone production by Fusarium graminearum in maize grains. Front. Microbiol. 6:892. doi: 10.3389/fmicb.2015.00892
Karlovsky, P. (2011). Biological detoxification of the mycotoxin deoxynivalenol and its use in genetically engineered crops and feed additives. Appl. Microbiol. Biotechnol. 91, 491–504. doi: 10.1007/s00253-011-3401-5
Li, X., Shin, S., Heinen, S., Dill-Macky, R., Berthiller, F., Nersesian, N., et al. (2015). Transgenic wheat expressing a barley UDP-glucosyltransferase detoxifies deoxynivalenol and provides high levels of resistance to Fusarium graminearum. Mol. Plant Microbe Interact. 28, 1237–1246. doi: 10.3389/fmicb.2015.00892
Li, X.-Z., Zhu, C., de Lange, C., Zhou, T., He, J., Yu, H., et al. (2011). Efficacy of detoxification of deoxynivalenol-contaminated corn by Bacillus sp. LS100 in reducing the adverse effects of the mycotoxin on swine growth performance. Food Addit. Contam. Part A Chem. Anal. Control Expo. Risk Assess. 28, 894–901. doi: 10.1080/19440049.2011.576402
Lulin, M., Yi, S., Aizhong, C., Zengjun, Q., Liping, X., Peidu, C., et al. (2010). Molecular cloning and characterization of an up-regulated UDP-glucosyltransferase gene induced by DON from Triticum aestivum L. cv. Wangshuibai. Mol. Biol. Rep. 37, 785–795. doi: 10.1007/s11033-009-9606-3
Lutz, M. P., Feichtinger, G., Defago, G., and Duffy, B. (2003). Mycotoxigenic Fusarium and deoxynivalenol production repress chitinase gene expression in the biocontrol agent Trichoderma atroviride P1. Appl. Environ. Microbiol. 69, 3077–3084. doi: 10.1128/AEM.69.6.3077-3084.2003
Marín, S., Velluti, A., Ramos, A. J., and Sanchis, V. (2004). Effect of essential oils on zearalenone and deoxynivalenol production by Fusarium graminearum in non-sterilized maize grain. Food Microbiol. 21, 313–318. doi: 10.1016/j.fm.2003.08.002
Matarese, F., Sarrocco, S., Gruber, S., Seidl-Seiboth, V., and Vannacci, G. (2012). Biocontrol of Fusarium head blight: interactions between Trichoderma and mycotoxigenic Fusarium. Microbiology 158, 98–106. doi: 10.1099/mic.0.052639-0
McCormick, S. P. (2013). Microbial detoxification of mycotoxins. J. Chem. Ecol. 39, 907–918. doi: 10.1007/s10886-013-0321-0
McCormick, S. P., Stanley, A. M., Stover, N. A., and Alexander, N. J. (2011). Trichothecenes: from simple to complex mycotoxins. Toxins 3, 802–814. doi: 10.3390/toxins3070802
McMullen, M., Bergstrom, G., De Wolf, E., Dill-Macky, R., Hershman, D., Shaner, G., et al. (2012). A unified effort to fight an enemy of wheat and barley: Fusarium head blight. Plant Dis. 96, 1712–1728. doi: 10.1094/PDIS-03-12-0291-FE
Mesterhazy, A., Toth, B., Varga, M., Bartok, T., Szabo-Hever, A., Farady, L., et al. (2011). Role of fungicides, application of nozzle types, and the resistance level of wheat varieties in the control of Fusarium head blight and deoxynivalenol. Toxins (Basel) 3, 1453–1483. doi: 10.3390/toxins3111453
Mohamed Anwar, B. U., Mclaughlin, J. E., Butterly, M. S., Susan, M. C., and Tumer, N. E. (2014). Elimination of damaged mitochondria through mitophagy reduces mitochondrial oxidative stress and increases tolerance to trichothecenes. Proc. Natl. Acad. Sci. U.S.A. 111, 11798–11803. doi: 10.1073/pnas.1403145111
Mukherjee, P. K., Horwitz, B. A., Herrera-Estrella, A., Schmoll, M., and Kenerley, C. M. (2013). Trichoderma research in the genome era. Annu. Rev. Phytopathol. 51, 105–129. doi: 10.1146/annurev-phyto-082712-102353
Pagnussatt, F. A., Del Ponte, E. M., Garda-Buffon, J., and Badiale-Furlong, E. (2014). Inhibition of Fusarium graminearum growth and mycotoxin production by phenolic extract from Spirulina sp. Pestic Biochem. Physiol. 108, 21–26. doi: 10.1016/j.pestbp.2013.11.002
Pagnussatt, F. A., Kupski, L., Darley, F. T., Filoda, P. F., Del Ponte,É. M., Garda-Buffon, J., et al. (2013). Fusarium graminearum growth inhibition mechanism using phenolic compounds from Spirulina sp. Food Sci. Technol. (Campinas) 33, 75–80. doi: 10.1590/S0101-20612013000500012
Palazzini, J. M., Alberione, E., Torres, A., Donat, C., Köhl, J., and Chulze, S. (2016). Biological control of Fusarium graminearum sensu stricto, causal agent of Fusarium head blight of wheat, using formulated antagonists under field conditions in Argentina. Biol. Control 94, 56–61. doi: 10.1016/j.biocontrol.2015.12.009
Pestka, J. J. (2010). Deoxynivalenol: mechanisms of action, human exposure, and toxicological relevance. Arch. Toxicol. 84, 663–679. doi: 10.1007/s00204-010-0579-8
Pfliegler, W. P., Pusztahelyi, T., and Pócsi, I. (2015). Mycotoxins–prevention and decontamination by yeasts. J. Basic Microbiol. 55, 805–818. doi: 10.1007/s10142-010-0177-0
Pinton, P., Tsybulskyy, D., Lucioli, J., Laffitte, J., Callu, P., Lyazhri, F., et al. (2012). Toxicity of deoxynivalenol and its acetylated derivatives on the intestine: differential effects on morphology, barrier function, tight junctions proteins and MAPKinases. Toxicol. Sci. 130, 180–190. doi: 10.1093/toxsci/kfs239
Poppenberger, B., Berthiller, F., Lucyshyn, D., Sieberer, T., Schuhmacher, R., Krska, R., et al. (2003). Detoxification of the Fusarium mycotoxin deoxynivalenol by a UDP-glucosyltransferase from Arabidopsis thaliana. J. Biol. Chem. 278, 47905–47914. doi: 10.1016/S2095-3119(14)60857-1
Richard, J. L. (2007). Some major mycotoxins and their mycotoxicoses—An overview. Int. J. Food Microbiol. 119, 3–10. doi: 10.1016/j.ijfoodmicro.2007.07.019
Sato, I., Ito, M., Ishizaka, M., Ikunaga, Y., Sato, Y., Yoshida, S., et al. (2012). Thirteen novel deoxynivalenol-degrading bacteria are classified within two genera with distinct degradation mechanisms. FEMS Microbiol. Lett. 327, 110–117. doi: 10.1111/j.1574-6968.2011.02461.x
Schisler, D., Slininger, P., Boehm, M., and Paul, P. (2011). Co-culture of yeast antagonists of Fusarium head blight and their effect on disease development in wheat. Plant Pathol. J. 10, 128–137. doi: 10.3923/ppj.2011.128.137
Schoneberg, A., Musa, T., Voegele, R. T., and Vogelgsang, S. (2015). The potential of antagonistic fungi for control of Fusarium graminearum and Fusarium crookwellense varies depending on the experimental approach. J. Appl. Microbiol. 118, 1165–1179. doi: 10.1111/jam.12775
Schweiger, W., Boddu, J., Shin, S., Poppenberger, B., Berthiller, F., Lemmens, M., et al. (2010). Validation of a candidate deoxynivalenol-inactivating UDP-glucosyltransferase from barley by heterologous expression in yeast. Mol. Plant Microbe Interact. 23, 977–986. doi: 10.1094/MPMI-23-7-0977
Shi, C., Yan, P., Li, J., Wu, H., Li, Q., and Guan, S. (2014). Biocontrol of Fusarium graminearum growth and deoxynivalenol production in wheat kernels with bacterial antagonists. Int. J. Environ. Res. Public Health 11, 1094–1105. doi: 10.3390/ijerph110101094
Shin, S., Torres-Acosta, J. A., Heinen, S. J., McCormick, S., Lemmens, M., Paris, M. P. K., et al. (2012). Transgenic Arabidopsis thaliana expressing a barley UDP-glucosyltransferase exhibit resistance to the mycotoxin deoxynivalenol. J. Exp. Bot. 63, 4731–4740. doi: 10.1093/jxb/ers141
Sobrova, P., Adam, V., Vasatkova, A., Beklova, M., Zeman, L., and Kizek, R. (2010). Deoxynivalenol and its toxicity. Interdiscipl. Toxicol. 3, 94–99. doi: 10.2478/v10102-010-0019-x
Terzi, V., Tumino, G., Stanca, A. M., and Morcia, C. (2014). Reducing the incidence of cereal head infection and mycotoxins in small grain cereal species. J. Cereal Sci. 59, 284–293. doi: 10.1016/j.jcs.2013.10.005
Velluti, A., Sanchis, V., Ramos, A. J., Turon, C., and Marín, S. (2004). Impact of essential oils on growth rate, zearalenone and deoxynivalenol production by Fusarium graminearum under different temperature and water activity conditions in maize grain. J. Appl. Microbiol. 96, 716–724. doi: 10.1111/j.1365-2672.2004.02212.x
Vinale, F., Sivasithamparam, K., Ghisalberti, E. L., Marra, R., Woo, S. L., and Lorito, M. (2008). Trichoderma plant pathogen interactions. Soil Biol. Biochem. 40, 1–10. doi: 10.1016/j.soilbio.2007.07.002
Wachowska, U., and Głowacka, K. (2014). Antagonistic interactions between Aureobasidium pullulans and Fusarium culmorum, a fungal pathogen of winter wheat. BioControl 59, 635–645. doi: 10.1007/s10526-014-9596-5
Wagacha, J., and Muthomi, J. (2008). Mycotoxin problem in Africa: current status, implications to food safety and health and possible management strategies. Int. J. Food Microbiol. 124, 1–12. doi: 10.1016/j.ijfoodmicro.2008.01.008
Wegulo, S. N. (2012). Factors influencing deoxynivalenol accumulation in small grain cereals. Toxins 4, 1157–1180. doi: 10.1094/PDIS-07-10-0495
Wegulo, S. N., Baenziger, P. S., Hernandez Nopsa, J., Bockus, W. W., and Hallen-Adams, H. (2015). Management of Fusarium head blight of wheat and barley. Crop Protect. 73, 100–107. doi: 10.1016/j.cropro.2015.02.025
Wegulo, S. N., Bockus, W. W., Nopsa, J. H., De Wolf, E. D., Eskridge, K. M., Peiris, K. H., et al. (2011). Effects of integrating cultivar resistance and fungicide application on Fusarium head blight and deoxynivalenol in winter wheat. Plant Dis. 95, 554–560. doi: 10.1094/PDIS-07-10-0495
Xue, A. G., Chen, Y., Voldeng, H. D., Fedak, G., Savard, M. E., Längle, T., et al. (2014). Concentration and cultivar effects on efficacy of CLO-1 biofungicide in controlling Fusarium head blight of wheat. Biol. Control 73, 2–7. doi: 10.1016/j.biocontrol.2014.02.010
Yoshida, S., Ohba, A., Liang, Y.-M., Koitabashi, M., and Tsushima, S. (2012). Specificity of Pseudomonas isolates on healthy and Fusarium head blight-infected spikelets of wheat heads. Microb. Ecol. 64, 214–225. doi: 10.1007/s00248-012-0009-y
Young, J. C., Zhou, T., Yu, H., Zhu, H., and Gong, J. (2007). Degradation of trichothecene mycotoxins by chicken intestinal microbes. Food Chem. Toxicol. 45, 136–143. doi: 10.1016/j.fct.2006.07.028
Yu, J.-H., and Keller, N. (2005). Regulation of secondary metabolism in filamentous fungi. Annu. Rev. Phytopathol. 43, 437–458. doi: 10.1146/annurev.phyto.43.040204.140214
Yuen, G. Y., and Schoneweis, S. D. (2007). Strategies for managing Fusarium head blight and deoxynivalenol accumulation in wheat. Int. J. Food Microbiol. 119, 126–130. doi: 10.1016/j.ijfoodmicro.2007.07.033
Keywords: mycotoxin, deoxynivalenol (DON), Fusarium, biological agents, control
Citation: Tian Y, Tan Y, Liu N, Liao Y, Sun C, Wang S and Wu A (2016) Functional Agents to Biologically Control Deoxynivalenol Contamination in Cereal Grains. Front. Microbiol. 7:395. doi: 10.3389/fmicb.2016.00395
Received: 29 January 2016; Accepted: 14 March 2016;
Published: 30 March 2016.
Edited by:
Sahdeo Prasad, The University of Texas MD Anderson Cancer Center, USAReviewed by:
Masoomeh Shams-Ghahfarokhi, Tarbiat Modares University, IranRobert Lawrence Brown, United States Department of Agriculture, Agricultural Research Service, USA
Aparna Ramachandran, Rutgers University, USA
Copyright © 2016 Tian, Tan, Liu, Liao, Sun, Wang and Wu. This is an open-access article distributed under the terms of the Creative Commons Attribution License (CC BY). The use, distribution or reproduction in other forums is permitted, provided the original author(s) or licensor are credited and that the original publication in this journal is cited, in accordance with accepted academic practice. No use, distribution or reproduction is permitted which does not comply with these terms.
*Correspondence: Aibo Wu, abwu@sibs.ac.cn