- 1ISVV, Unité de Recherche Œnologie EA 4577, USC 1366 Institut National de la Recherche Agronomique, Bordeaux INP, University Bordeaux, Villenave d'Ornon, France
- 2Bordeaux Sciences Agro, Gradignan, France
- 3Biolaffort, Bordeaux, France
- 4ENSCBP, Bordeaux INP, Pessac, France
Saccharomyces cerevisiae is by far the most widely used yeast in oenology. However, during the last decade, several other yeasts species has been purposed for winemaking as they could positively impact wine quality. Some of these non-conventional yeasts (Torulaspora delbrueckii, Metschnikowia pulcherrima, Pichia kluyveri, Lachancea thermotolerans, etc.) are now proposed as starters culture for winemakers in mixed fermentation with S. cerevisiae, and several others are the subject of various studies (Hanseniaspora uvarum, Starmerella bacillaris, etc.). Along with their biotechnological use, the knowledge of these non-conventional yeasts greatly increased these last 10 years. The aim of this review is to describe the last updates and the current state-of-art of the genetics of non-conventional yeasts (including S. uvarum, T. delbrueckii, S. bacillaris, etc.). We describe how genomics and genetics tools provide new data into the population structure and biodiversity of non-conventional yeasts in winemaking environments. Future challenges will lie on the development of selection programs and/or genetic improvement of these non-conventional species. We discuss how genetics, genomics and the advances in next-generation sequencing will help the wine industry to develop the biotechnological use of non-conventional yeasts to improve the quality and differentiation of wines.
Introduction
In oenology, alcoholic fermentation is generally performed by Saccharomyces cerevisiae yeast, the “conventional” wine yeast. Currently, the winemakers have the choice between hundreds of S. cerevisiae starters that have been selected for various characteristics including their ability to complete alcoholic fermentation in oenological conditions, their low release of off-flavor compounds, their positive impact on wine aromas, etc., (Pretorius, 2000; Marullo and Dubourdieu, 2010). The growing demand for more diversified wines or for specific characteristics (low ethanol content, etc.) has led to the exploration of new species for winemaking. These non-conventional yeasts may contribute to the wine's flavor and taste by producing a broad range of secondary metabolites and extracellular enzymes (Hong and Park, 2013; Ciani et al., 2014; Wang et al., 2015). Some species could be interesting for alcohol level reduction in wine (Masneuf-Pomarede et al., 2010; Bely et al., 2013) or for greater fermentative ability in harsh conditions due to enhanced fructophily (Sutterlin, 2010; Magyar and Tóth, 2011). It has to be noted that, as only some Saccharomyces species (i.e., S. cerevisiae, S. uvarum, and some interspecific hybrids) are able to consume all the sugar contained in grape must, non-Saccharomyces yeasts must be used in co- or sequential-fermentation with a Saccharomyces spp. able to secure AF completion (Jolly et al., 2006; Bely et al., 2013).
The wine industry currently proposes starters of a few non-conventional yeasts (Torulaspora delbrueckii, Metschnikowia pulcherrima, Pichia kluyveri, Lachancea thermotolerans, etc.), while several other species (Hanseniaspora uvarum, Starmerella bacillaris, etc.) are the subject of various studies to assess both positive contribution (Table 1) and negative impact (if any) on wine quality (Bely et al., 2013; Maturano et al., 2015). These non-conventional yeasts are widely distributed amongst the Saccharomycetales (Figure 1). In order to evaluate the oenological potential of a given species, several strains are usually compared for phenotypes of interest like fermentation ability (Renault et al., 2009) or glycerol production (Magyar and Tóth, 2011). However, in most cases, neither the relationships between the tested strains are described, nor the genetic structuration of the species is known. This lack of genetic knowledge is clearly detrimental, since we are not able to determine whether the phenotypic diversity described is representative of the species or not. The recent advances in next-generation sequencing (NGS) have triggered the development of genomic and genetic tools for some of these non-conventional yeasts, but the field is still in its infancy. The objective of this paper is thus to review the current state-of-art of the genetics of non-conventional wine yeasts and to discuss the future prospects and challenges from an oenological viewpoint.
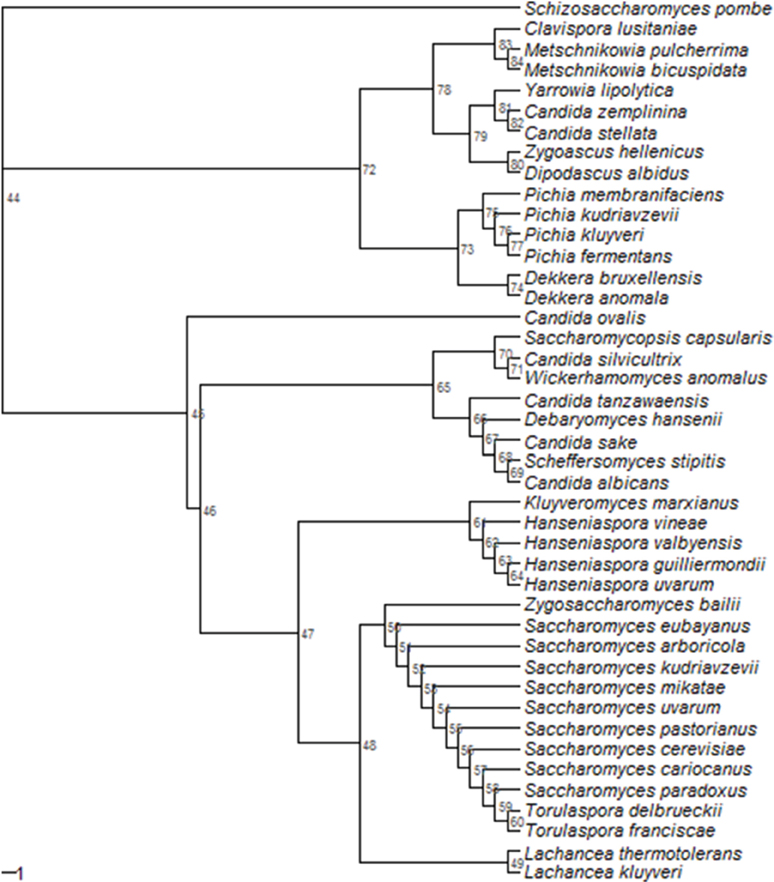
Figure 1. Phylogeny of 41 species of Saccharomycetales on the basis of 18S ribosomal DNA sequence. Multiple sequence alignment (1951 bases) was performed by Clustal Omega (EMBL-EBI website). Genetic distance was computed using the K80 Kimura model (Kimura, 1980), phylogenetic tree was built using Neighbor joining clustering method and bootstrapping (1000 replicates) was used to assess the robustness of the nodes by means of R package ape (Paradis et al., 2004). Schizosaccharomyces pombe was used as outgroup species. The following sequences and strains (mostly type strains) were used: AB000642.1|Dipodascus albidus IFO 1984; AB013504.1|C. tanzawaensis JCM 1648; AB018175.1|C. stellata JCM 9476; AB023473.1|M. pulcherrima IFO 1678; AB040997.1|S. kudriavzevii IFO 1802; AB040998.1|S. mikatae IFO 1815; AB054561.1|C. silvicultrix JCM 9831; AB013529.1|C. sake JCM 2951; AF548094.1|S. cerevisiae CBS 1171; AJ271813.1|S. cariocanus UFRJ 50816; AY046254.1|H. valbyensis NRRL Y-1626; AY046256.1|H. guilliermondii NRRL Y-1625; AY046257.1|H. uvarum NRRL Y-1614; AY046258.1|H. vineae NRRL Y-17529; S. bacillaris CBS 9494; EF550365.1|P. membranifaciens NRRL Y-2026; EF550372.1|P. fermentans Y-1619; EF550389.1|P. kluyveri NRRL Y-11519; EF550396.1|D. anomala NRRL Y-17522; EF550479.1|Wickerhamomyces anomalus NRRL Y-366; EU011714.1|C. ovalis NRRL Y-17662; EU011734.1|D. bruxellensis NRRL Y-12961; EU348783.1|C. albicans NRRL Y-12983; FJ153136.1|L. thermotolerans NRRL Y-8284; FJ153143.1|T. franciscae NRRL Y-6686; GU266277.1|S. arboricola AS 2.3317; GU597328.1|Zygoascus hellenicus CBS 5839; HQ651939.1|Scheffersomyces stipitis ATCC 58376; JQ698884.1|Saccharomycopsis capsularis NRRL Y-17639; JQ698900.1|Clavispora lusitaniae NRRL Y-11827; JQ698910.1|Debaryomyces hansenii NRRL Y-7426; JQ698926.1|Yarrowia lipolytica NRRL YB-423; JQ698936.1|Schizosaccharomyces pombe NRRL Y-12796; M55528.1|P. kudriavzevii MUCL 29849; S. eubayanus FM1318; S. uvarum CBS7001; X69846.1|M. bicuspidata MUCL 31145; X89523.1|L. marxianus CBS 712; X91083.1|Zygosaccharomyces bailii NCYC 1416; X97805.1|S. pastorianus NCYC 392; X97806.1|S. paradoxus CBS 432; X98120.1|T. delbrueckii CBS 1146; Z75580.1|L. kluyveri NCYC 543.
Basic Genetic Knowledge of Wine Yeasts
As a model organism, the genomic outline of S. cerevisiae is well-known: its genome size is around 12 Mb organized in 16 chromosomes, with a mitochondrial genome of 85 Kb (Table 1). The genome sequences of several hundreds of strains of various origins are available, and much more sequences are produced easily using NGS technology and subsequently assembled even by lab with moderate bioinformatics skills. The population genomics of S. uvarum has been improved recently with the sequencing of more than 50 strains of various origins (Almeida et al., 2014). The type strain CBS7001T has a genome size of 11.5 Mb and 16 chromosomes (Cliften et al., 2003). By contrast, such basic knowledge (genome size, chromosome number, etc.) is available only for a small number of non-conventional wine species: T. delbrueckii has a genome of 9–11 Mb distributed on eight chromosomes; L. thermotolerans has a 10.4 Mb genome with eight chromosomes. Other wine yeast species usually have genome size ranging from 8 to 12 Mb, with chromosomes number unknown yet (P. kluyveri, M. pulcherrima, etc.). Moreover, there is still a lack of reference genome sequence for several non-conventional wine yeasts of interest like S. bacillaris, P. fermentans, etc., (Table 1). Disparities exist also for the mitochondrial genome, with full sequences available for some species like L. thermotolerans or H. uvarum, and partial sequences for other species (C. stellata, P. membranifaciens, etc.). Thus, although the genomic data of non-conventional wine yeast greatly increased this last decade, there is still a lot of work to achieve in this field.
The Life-Cycle of Wine Yeasts
The life cycle of Saccharomyces wine species is well-known: both S. cerevisiae and S. uvarum are diploid species that divide asexually by mitosis. They are able to enter meiosis and form asci containing generally four haploid spores (tetrads). While haploid cells can undergo mitosis, the haploid level is generally transient and crosses between haploid spores of opposite mating types are readily observed, leading to diploid zygote formation. Moreover, haploid cells are usually able to switch mating type at mitosis (homothallism). The physical proximity between mother and daughter haploid cells of opposite mating type usually results in high level of inbreeding (Ruderfer et al., 2006; Cubillos et al., 2009; Warringer et al., 2011). Variations in this breeding system were described for S. cerevisiae like near-dioecy or higher level of outcrossing, but seemed quite rare and associated with environmental specificities (Knop, 2006; Al Safadi et al., 2010; Murphy and Zeyl, 2010).
By comparison, the precise life-cycle of most non-Saccharomyces yeasts is unknown yet. Sporulation was observed for most non-conventional yeast, albeit forming non-tetrad asci in many cases (T. delbrueckii, D. hansenii, H. vinae, etc., Table 1). No evidence of sporulation ability was recorded to date for Starmerella/Candida species. Data regarding the occurrence of sexual reproduction is usually scarce for most non-Saccharomyces yeasts, so classical genetic manipulations are impossible to date. To circumvent this limitation, both intra and inter specific hybridizations by protoplast fusion can be achieved as demonstrated in the past (Ball, 1984; Pina et al., 1986).
The basic ploidy level is also usually unresolved (Table 1): T. delbrueckii has been considered as a haploid species for a long time, but the detection of several strains harboring several loci with two alleles (26.4% of strains showing heterozygosity), its ability to sporulate and the presence of mating type genes is more congruent with a diploid status (Albertin et al., 2014a). Conversely, for S. bacillaris, the proportion of heterozygous strains was almost null (0.01%). This, combined with its inability to sporulate, is more consistent with an hypothesis of an haploid status (Masneuf-Pomarede et al., 2015) but has still to be formally demonstrated. Finally, despite its fully sequenced genome, the ploidy status of L. thermotolerans is controversial: haploid or diploid depending on the authors (Souciet et al., 2009; Freel et al., 2014). In conclusion, the biological life-cycle of many non-Saccharomyces yeasts remains to be elucidated.
Ecology of Wine Yeast
Most wine yeasts can colonize several ecological niches, including wine-related environments like grape, must, winery equipment and premise (Table 1). Moreover, many of them can be isolated from other human-associated processes (brewery, bakery, dairy, bioethanol, distillery, etc.) and also from wild substrates (soil, insect, plant, etc.). Isolation from clinical specimens is rarely described yet possible (yeasts being opportunistic microorganisms), and most wine yeasts are Generally Recognized As Safe (GRAS). Dissemination and transfer between the different ecological reservoirs could be performed through insects (Parle and Di Menna, 1966; Stefanini et al., 2012; Palanca et al., 2013), but also through human activities like material exchanges, etc., (Goddard et al., 2010). Indeed, although most wine yeasts are described as ubiquitous from an ecological viewpoint, some species have a restricted substrate range. This is the case of H. guillermondii and Starmerella species for example, which are very rarely isolated from non-wine-related substrates (Masneuf-Pomarede et al., 2015). Thus, the study of most wine yeast should consider not only wine strains but also isolates from other technological processes and substrates in order to assess their biodiversity.
Adaptation to Winemaking Environments and Evolutionary Mechanisms
Wine environments are particularly harsh and inconstant: winemaking is a seasonal practice, so that yeasts present at the surface of grape berries at harvest suddenly have to survive in grape must containing high sugar concentrations, usually with sulfur dioxide content. Moreover, from an ecological viewpoint, the ensuing alcoholic fermentation is a rapidly fluctuating ecosystem: within a few days, grape must is depleted of nitrogen nutrients, while ethanol concentration and temperature increase steadily thanks to Saccharomyces spp. metabolism, thus conferring a fitness advantage for Saccharomyces spp. over the other wine yeasts (Goddard, 2008; Salvadó et al., 2011). In addition, the range of temperature can be quite high, with either short-term variations (daily variations) or long-term evolution (seasonal variations). As a result, within wine yeast species, some strains show specific wine-adaptation (Steensels and Verstrepen, 2014) like sulphite resistance (Divol et al., 2012), ethanol tolerance (García-Ríos et al., 2014), low pH adaptation (Pretorius, 2000), temperature adaptation (Naumov et al., 2000), etc. The underlying adaptive mechanisms vary greatly from one species to another: in S. cerevisiae, molecular approaches identified allelic variations as molecular causes of adaptation to the winemaking process (Aa et al., 2006; Marullo et al., 2007; Ambroset et al., 2011; Salinas et al., 2012; Jara et al., 2014). At the chromosome level, translocations were shown to be responsible for adaptation to sulfite (Zimmer et al., 2014). Polyploidy and hybridization are also major evolutionary processes that probably triggered adaptation to wine environments (Borneman et al., 2012; Erny et al., 2012) and are currently explored for biotechnological application (Timberlake et al., 2011; Plech et al., 2014; Blein-Nicolas et al., 2015; da Silva et al., 2015). Large genomic introgressions were evidenced in S. uvarum strains associated with human-driven fermentations, suggesting a link between introgressions and domestication (Almeida et al., 2014). Various horizontal gene transfers were also evidenced for wine S. cerevisiae strains (Novo et al., 2009), and were shown to favor adaptation to the nitrogen-limited wine fermentation environment (Marsit et al., 2015). Other evolutionary mechanisms were described (Dujon et al., 2004; Barrio et al., 2006; Scannell et al., 2007), and it is highly probable that further investigations will allow the identification of additional adaptation processes in wine yeasts. In particular, it could be interesting to focus on transposon families and their possible implication in environmental adaptation (Zeyl, 2004; Liti et al., 2005; Sarilar et al., 2015), to explore the impact of mitochondrial genome variation regarding adaptation to wine environments and practices (Picazo et al., 2015; Wu et al., 2015) or to describe the landscape of gene duplication and prion involvement in fitness issues (Landry et al., 2006; Jarosz et al., 2014). However, to date, most of these data were obtained from Saccharomyces species and could now be obtained from non-Saccharomyces of interest.
Population Genetics of Yeast Species Associated with Winemaking
Within a given species, the colonization of different ecosystems can led to the evolutionary differentiation of the subpopulations, in relationship with their adaptation to environmental specificities. This is the case of S. cerevisiae species that shows genetic subgroups of wild and domestic strains associated with human activities like wine, bread, beer, sake, etc., (Fay and Benavides, 2005; Liti et al., 2009; Sicard and Legras, 2011; Almeida et al., 2015), that probably originated through multiple domestication events (Schacherer et al., 2009). In a recent study, Almeida et al. (2014) showed that S. uvarum was also divided in genetic subgroups, one of domestic strains used in both winemaking and cidermaking and associated with the northern hemisphere, while others subgroups were composed of wild isolates from South America and Australasia. The current hypothesis is that a Patagonian “wild” sub-population gave rise to the domestic subpopulation through a recent bottleneck (Almeida et al., 2014). Another wine species was recently described as domesticated: T. delbrueckii is also divided in genetic subgroups of wild and domestic strains (Albertin et al., 2014a). Moreover, the wine/grape-related group showed an increase ability to ferment sugar in oenological condition, confirming the occurrence of phenotypic domestication (Albertin et al., 2015). By contrast, no hint of domestication was recorded to date for S. bacillaris and H. uvarum whose genetic diversity is shaped by geographical localization and/or time variation (Masneuf-Pomarede et al., 2015; Albertin et al., 2016).
Biodiversity in Winemaking Conditions
Several molecular methods were developed in order to perform intra-specific discrimination, like pulsed field electrophoresis, RAPD-PCR fingerprinting, tandem repeat-tRNA, Fourier transform infrared spectroscopy, RFLP, etc., (Barquet et al., 2012; Tofalo et al., 2013, 2014; Pfliegler et al., 2014; Grangeteau et al., 2015). However, these approaches do not allow the establishment of the genetic relationships within a given species and subsequent population genetics studies. An alternative is the use of microsatellite genotyping. It has been successfully applied to S. cerevisiae (Legras et al., 2005; Richards et al., 2009), S. uvarum (Masneuf-Pomarede et al., 2009), T. delbrueckii (Albertin et al., 2014a), S. bacillaris (Masneuf-Pomarede et al., 2015), H. uvarum (Albertin et al., 2016) as well as to the spoilage wine yeast Brettanomyces bruxellensis (Albertin et al., 2014c), and is currently developed for additional wine species like Meyerozyma guilliermondii (Wrent et al., 2015). In addition to population genetic clustering, microsatellites allow measuring the genetic diversity of a given species in specific conditions. In S. cerevisiae, the genetic diversity varied greatly, from 0 (fully clonal populations) to 1 (fully diversified population, Table 1). The precise impact of S. cerevisiae diversity (or absence of diversity) on wine quality is still debated/studied (Egli et al., 1998; Howell et al., 2006; King et al., 2008) and the direct link between microbial diversity and wine complexity should be considered with caution. S. uvarum and T. delbrueckii showed also a large range of diversity (0.35–1 and 0–0.62). By contrast, other species show systematic high diversity (>0.9 for H. uvarum or S. bacillaris), suggesting that they are not under selective pressure in winemaking environments (Masneuf-Pomarede et al., 2015; Albertin et al., 2016).
Future Challenges
Definite progresses in the genetics of non-conventional yeasts were made in the last decade. However, there is still a great lack of data compared to the conventional wine yeast S. cerevisiae. Such knowledge is nowadays within reach thanks to the NGS revolution (Solieri et al., 2013). NGS allows the development of genome-assisted approaches like whole genome sequencing and resequencing, transcriptome profiling, ChIP-sequencing to identify DNA-structure, etc., (Solieri et al., 2013). De novo sequencing is greatly needed as some wine species still lack of nuclear and mitochondrial reference genomes (S. bacillaris, P. fermentans, M. pulcherrima, etc.). However, de novo assembly is sometimes difficult to conduct due to high heterozygosity level or sequence repeat, and led to draft genome with high number of contigs or scaffolds. For example, H. uvarum DSM 2768 genome displays 335 contigs, P. kudriavzevii M12 has 621 scaffolds, and P. anomala NRRL Y-366 shows 1932 scaffolds. Thus, the first aim of non-conventional wine yeast studies should be the completion of robust genomic sequences. Then, additional genome sequencing could be performed: genome re-sequencing using NGS captures individual genotypes and allows population genetics and ecologic studies within species. Such comparative genomics approaches were successfully applied to S. cerevisiae (Liti et al., 2009) and S. uvarum (Almeida et al., 2014), and could now address non-Saccharomyces yeasts of technological interest. In addition to intraspecific genomics, comparative genomics between yeast species is particularly useful to understand genome evolution (Liti and Louis, 2005). The identification of specific metabolic pathways, gene duplications or functions between species may increase our appreciation of adaptation's mechanisms and their biotechnological interest (Blein-Nicolas et al., 2015). It has to be noted that several species genetically close to wine yeasts show no peculiar affinity with winemaking environment (Figure 1). This is the case of S. paradoxus: despite being the most closely related species to S. cerevisiae, S. paradoxus is essentially associated with wild environments and particularly trees (Sniegowski et al., 2002; Johnson et al., 2004). Comparative genomics of wine vs. non-wine yeast species could thus increase our knowledge of the common genomic requirement for grape/wine colonization, if any. Finally, NGS technologies have greatly improved genome-assisted approaches aiming at detecting genetic variants associated with phenotypes in S. cerevisiae (Ehrenreich et al., 2010). In particular, QTL-seq or genome-wide association studies (GWAS) could now be applied to non-conventional yeasts depending on whether classical breeding is possible (QTL-seq) or not (GWAS). These fields are blank pages waiting to be filled in the next future of oenology microbial research.
The use of mixed-cultures, combining both non-conventional yeasts and one Saccharomyces species able to complete AF, is increasing in winemaking. Thus, another challenge lies in understanding yeast-yeast interactions and their underlying mechanisms (Ciani et al., 2010; Ciani and Comitini, 2015). Indeed, several types of yeast-yeast interactions have been described in enological conditions: competition for nutriments, release of toxic compounds (Fleet, 2003), and even “quorum-sensing” like mechanisms (Nissen and Arneborg, 2003; Nissen et al., 2003; Renault et al., 2013). Understanding these complex interactions is of first importance as the combination of some yeast strains seems condemned to failure: for example, cell-cell contact was recently shown to be involved in the death of strains of T. delbrueckii and L. thermotolerans during mixed-culture alcoholic fermentation with S. cerevisiae (Renault et al., 2013; Kemsawasd et al., 2015). In some cases, yeast death was associated with the release of metabolites or killer toxin (Pérez-Nevado et al., 2006; Albergaria et al., 2010; Branco et al., 2015; Ramírez et al., 2015). The precise impact of such interactions regarding wine quality and aromas is still unclear (Ciani et al., 2006), but will have to be considered to control and optimize complex mixed oenological fermentation.
Finally, in addition to NGS-assisted approaches and interactions studies, another prospect in the field of non-conventional wine yeast lies in classical genetic approaches: indeed, one of the limits of the previously detailed approaches is their low ability in elucidating the basic life-cycle of wine yeasts, particularly regarding the occurrence and control of sexual reproduction. Still, classical breeding is one of the key issues for genetic improvement of industrial strains of S. cerevisiae (Pretorius, 2000; Giudici et al., 2005; Marullo et al., 2006; Steensels et al., 2014) and represents a technological barrier that must be overcome for actual improvement of non-Saccharomyces wine yeasts. There is an important need for traditional sporulation assays, spore microdissection attempts, subsequent segregant analyses, breeding assays, etc. In addition, genetic transformation of non-conventional wine yeasts would be a welcomed tool for subsequent functional studies (Pacheco et al., 2009; Roberts and Oliver, 2011). These classical approaches are time-consuming and necessitate traditional yeast-manipulation know-how, sometimes viewed as old-fashioned and therefore neglected. However, these old approaches are essential for our future understanding of the genetics of non-conventional wine yeast, and are complementary to the more en vogue NGS-assisted approaches.
Funding
This work was funded by the European Union's Seventh Framework Programme (FP7/2007–2013) under grant agreement n° 315065 WILDWINE project: “Multi-strain indigenous Yeast and Bacterial starters for “Wild-ferment” wine production.”
Conflict of Interest Statement
The authors declare that the research was conducted in the absence of any commercial or financial relationships that could be construed as a potential conflict of interest.
References
Aa, E., Townsend, J. P., Adams, R. I., Nielsen, K. M., and Taylor, J. W. (2006). Population structure and gene evolution in Saccharomyces cerevisiae. FEMS Yeast Res. 6, 702–715. doi: 10.1111/j.1567-1364.2006.00059.x
Albergaria, H., Francisco, D., Gori, K., Arneborg, N., and Gírio, F. (2010). Saccharomyces cerevisiae CCMI 885 secretes peptides that inhibit the growth of some non-Saccharomyces wine-related strains. Appl. Microbiol. Biotechnol. 86, 965–972. doi: 10.1007/s00253-009-2409-6
Albertin, W., Chasseriaud, L., Comte, G., Panfili, A., Delcamp, A., Salin, F., et al. (2014a). Winemaking and bioprocesses strongly shaped the genetic diversity of the ubiquitous yeast Torulaspora delbrueckii. PLoS ONE 9:e94246. doi: 10.1371/journal.pone.0094246
Albertin, W., Marullo, P., Aigle, M., Bourgais, A., Bely, M., Dillmann, C., et al. (2009). Evidence for autotetraploidy associated with reproductive isolation in Saccharomyces cerevisiae: towards a new domesticated species. J. Evol. Biol. 22, 2157–2170. doi: 10.1111/j.1420-9101.2009.01828.x
Albertin, W., Marullo, P., Peltier, E., Windholtz, S., Bely, M., and Masneuf-Pomarede, I. (2015). Biodiversity of Wine Yeasts: New Insights from Population Genetics. Oeno2015.
Albertin, W., Miot-Sertier, C., Bely, M., Marullo, P., Coulon, J., Moine, V., et al. (2014b). Oenological prefermentation practices strongly impact yeast population dynamics and alcoholic fermentation kinetics in Chardonnay grape must. Int. J. Food Microbiol. 178, 87–97. doi: 10.1016/j.ijfoodmicro.2014.03.009
Albertin, W., Miot-Sertier, C., Bely, M., Mostert, T. T., Colonna-Ceccaldi, B., Coulon, J., et al. (2016). Hanseniaspora uvarum from winemaking environments show spatial and temporal genetic clustering. Front. Microbiol. 6:1569. doi: 10.3389/fmicb.2015.01569
Albertin, W., Panfili, A., Miot-Sertier, C., Goulielmakis, A., Delcamp, A., Salin, F., et al. (2014c). Development of microsatellite markers for the rapid and reliable genotyping of Brettanomyces bruxellensis at strain level. Food Microbiol. 42, 188–195. doi: 10.1016/j.fm.2014.03.012
Almeida, P., Barbosa, R., Zalar, P., Imanishi, Y., Shimizu, K., Turchetti, B., et al. (2015). A population genomics insight into the mediterranean origins of wine yeast domestication. Mol. Ecol. 24, 5412–5427. doi: 10.1111/mec.13341
Almeida, P., Gonçalves, C., Teixeira, S., Libkind, D., Bontrager, M., Masneuf-Pomarède, I., et al. (2014). A Gondwanan imprint on global diversity and domestication of wine and cider yeast Saccharomyces uvarum. Nat. Commun. 5:4044. doi: 10.1038/ncomms5044
Al Safadi, R., Weiss-Gayet, M., Briolay, J., and Aigle, M. (2010). A polyploid population of Saccharomyces cerevisiae with separate sexes (dioecy). FEMS Yeast Res. 10, 757–768. doi: 10.1111/j.1567-1364.2010.00660.x
Ambroset, C., Petit, M., Brion, C., Sanchez, I., Delobel, P., Guérin, C., et al. (2011). Deciphering the molecular basis of wine yeast fermentation traits using a combined genetic and genomic approach. G3 1, 263–281. doi: 10.1534/g3.111.000422
Andorrà, I., Berradre, M., Mas, A., Esteve-Zarzoso, B., and Guillamón, J. M. (2012). Effect of mixed culture fermentations on yeast populations and aroma profile. LWT Food Sci. Technol. 49, 8–13. doi: 10.1016/j.lwt.2012.04.008
Anfang, N., Brajkovich, M., and Goddard, M. R. (2009). Co-fermentation with Pichia kluyveri increases varietal thiol concentrations in Sauvignon Blanc. Aust. J. Grape Wine Res. 15, 1–8. doi: 10.1111/j.1755-0238.2008.00031.x
Azzolini, M., Fedrizzi, B., Tosi, E., Finato, F., Vagnoli, P., Scrinzi, C., et al. (2012). Effects of Torulaspora delbrueckii and Saccharomyces cerevisiae mixed cultures on fermentation and aroma of Amarone wine. Eur. Food Res. Technol. 235, 303–313. doi: 10.1007/s00217-012-1762-3
Barnett, J. A., Payne, R. W., and Yarrow, D. (2000). Yeasts: Characteritics and Identification. Cambridge, UK: Cambridge University Press.
Barquet, M., Martín, V., Medina, K., Pérez, G., Carrau, F., and Gaggero, C. (2012). Tandem repeat-tRNA (TRtRNA) PCR method for the molecular typing of non-Saccharomyces subspecies. Appl. Microbiol. Biotechnol. 93, 807–814. doi: 10.1007/s00253-011-3714-4
Barrio, E., Gonzalez, S., Arias, A., Belloch, C., and Querol, A. (2006). “Molecular mechanisms involved in the adaptive evolution of industrial yeasts,” in Yeasts in Food and Beverages, eds A. Querol and G. Fleet (Berlin; Heildeberg: Springer-Verlag), 153–174.
Bely, M., Renault, P., da Silva, T., Masneuf-Pomarede, I., Albertin, W., Moine, V., et al. (2013). “Non-conventional yeasts and alcohol level reduction,” in Alcohol Level Reduction in Wine (Vigne et Vin Publications Internationales), 33–37.
Bely, M., Stoeckle, P., Masneuf-Pomarède, I., and Dubourdieu, D. (2008). Impact of mixed Torulaspora delbrueckii-Saccharomyces cerevisiae culture on high-sugar fermentation. Int. J. Food Microbiol. 122, 312–320. doi: 10.1016/j.ijfoodmicro.2007.12.023
Blein-Nicolas, M., Albertin, W., da Silva, T., Valot, B., Balliau, T., Masneuf-Pomarede, I., et al. (2015). A systems approach to elucidate heterosis of protein abundances in yeast. Mol. Cell. Proteomics 14, 2056–2071. doi: 10.1074/mcp.M115.048058
Borneman, A. R., Desany, B. A., Riches, D., Affourtit, J. P., Forgan, A. H., Pretorius, I. S., et al. (2012). The genome sequence of the wine yeast VIN7 reveals an allotriploid hybrid genome with Saccharomyces cerevisiae and Saccharomyces kudriavzevii origins. FEMS Yeast Res. 12, 88–96. doi: 10.1111/j.1567-1364.2011.00773.x
Borneman, A. R., Forgan, A. H., Pretorius, I. S., and Chambers, P. J. (2008). Comparative genome analysis of a Saccharomyces cerevisiae wine strain. FEMS Yeast Res. 8, 1185–1195. doi: 10.1111/j.1567-1364.2008.00434.x
Branco, P., Viana, T., Albergaria, H., and Arneborg, N. (2015). Antimicrobial peptides (AMPs) produced by Saccharomyces cerevisiae induce alterations in the intracellular pH, membrane permeability and culturability of Hanseniaspora guilliermondii cells. Int. J. Food Microbiol. 205, 112–118. doi: 10.1016/j.ijfoodmicro.2015.04.015
Breuer, U., and Harms, H. (2006). Debaryomyces hansenii–an extremophilic yeast with biotechnological potential. Yeast 23, 415–437. doi: 10.1002/yea.1374
Chan, G. F., Gan, H. M., Ling, H. L., and Rashid, N. A. (2012). Genome sequence of Pichia kudriavzevii M12, a potential producer of bioethanol and phytase. Eukaryot. Cell 11, 1300–1301. doi: 10.1128/EC.00229-12
Ciani, M., Beco, L., and Comitini, F. (2006). Fermentation behaviour and metabolic interactions of multistarter wine yeast fermentations. Int. J. Food Microbiol. 108, 239–245. doi: 10.1016/j.ijfoodmicro.2005.11.012
Ciani, M., Canonico, L., Oro, L., and Comitini, F. (2014). Sequential fermentation using non-Saccharomyces yeasts for the reduction of alcohol content in wine. BIO Web Conf. 3:02015. doi: 10.1051/bioconf/20140302015
Ciani, M., and Comitini, F. (2015). Yeast interactions in multi-starter wine fermentation. Curr. Opin. Food Sci. 1, 1–6. doi: 10.1016/j.cofs.2014.07.001
Ciani, M., Comitini, F., Mannazzu, I., and Domizio, P. (2010). Controlled mixed culture fermentation: a new perspective on the use of non-Saccharomyces yeasts in winemaking. FEMS Yeast Res. 10, 123–133. doi: 10.1111/j.1567-1364.2009.00579.x
Ciani, M., and Maccarelli, F. (1998). Oenological properties of non-Saccharomyces yeasts associated with wine-making. World J. Microbiol. Biotechnol. 14, 199–203. doi: 10.1023/A:1008825928354
Clemente-Jimenez, J. M., Mingorance-Cazorla, L., Martıìnez-Rodrıìguez, S., Heras-Vázquez, F. J. L., and Rodrıìguez-Vico, F. (2004). Molecular characterization and oenological properties of wine yeasts isolated during spontaneous fermentation of six varieties of grape must. Food Microbiol. 21, 149–155. doi: 10.1016/S0740-0020(03)00063-7
Clemente-Jimenez, J. M., Mingorance-Cazorla, L., Martínez-Rodríguez, S., Las Heras-Vázquez, F. J., and Rodríguez-Vico, F. (2005). Influence of sequential yeast mixtures on wine fermentation. Int. J. Food Microbiol. 98, 301–308. doi: 10.1016/j.ijfoodmicro.2004.06.007
Cliften, P., Sudarsanam, P., Desikan, A., Fulton, L., Fulton, B., Majors, J., et al. (2003). Finding functional features in Saccharomyces genomes by phylogenetic footprinting. Science 301, 71–76. doi: 10.1126/science.1084337
Comitini, F., Gobbi, M., Domizio, P., Romani, C., Lencioni, L., Mannazzu, I., et al. (2011). Selected non-Saccharomyces wine yeasts in controlled multistarter fermentations with Saccharomyces cerevisiae. Food Microbiol. 28, 873–882. doi: 10.1016/j.fm.2010.12.001
Comitini, F., De Ingeniis, J., Pepe, L., Mannazzu, I., and Ciani, M. (2004). Pichia anomala and Kluyveromyces wickerhamii killer toxins as new tools against Dekkera/Brettanomyces spoilage yeasts. FEMS Microbiol. Lett. 238, 235–240. doi: 10.1111/j.1574-6968.2004.tb09761.x
Csoma, H., and Sipiczki, M. (2008). Taxonomic reclassification of Candida stellata strains reveals frequent occurrence of Candida zemplinina in wine fermentation. FEMS Yeast Res. 8, 328–336. doi: 10.1111/j.1567-1364.2007.00339.x
Cubillos, F. A., Vásquez, C., Faugeron, S., Ganga, A., and Martínez, C. (2009). Self-fertilization is the main sexual reproduction mechanism in native wine yeast populations. FEMS Microbiol. Ecol. 67, 162–170. doi: 10.1111/j.1574-6941.2008.00600.x
da Silva, T., Albertin, W., Dillmann, C., Bely, M., la Guerche, S., Giraud, C., et al. (2015). Hybridization within Saccharomyces Genus Results in Homoeostasis and Phenotypic Novelty in Winemaking Conditions. PLoS ONE 10:e0123834. doi: 10.1371/journal.pone.0123834
Diezmann, S., and Dietrich, F. S. (2009). Saccharomyces cerevisiae: population Divergence and Resistance to Oxidative Stress in Clinical, Domesticated and Wild Isolates. PLoS ONE 4:e5317. doi: 10.1371/journal.pone.0005317
Di Maio, S., Genna, G., Gandolfo, V., Amore, G., Ciaccio, M., and Oliva, D. (2012). Presence of Candida zemplinina in sicilian musts and selection of a strain for wine mixed fermentations. S. Afr. J. Enol. Viticult. 33, 80–87.
Divol, B., du Toit, M., and Duckitt, E. (2012). Surviving in the presence of sulphur dioxide: strategies developed by wine yeasts. Appl. Microbiol. Biotechnol. 95, 601–613. doi: 10.1007/s00253-012-4186-x
Domizio, P., Liu, Y., Bisson, L. F., and Barile, D. (2014). Use of non-Saccharomyces wine yeasts as novel sources of mannoproteins in wine. Food Microbiol. 43, 5–15. doi: 10.1016/j.fm.2014.04.005
Domizio, P., Romani, C., Comitini, F., Gobbi, M., Lencioni, L., Mannazzu, I., et al. (2011a). Potential spoilage non-Saccharomyces yeasts in mixed cultures with Saccharomyces cerevisiae. Ann. Microbiol. 61, 137–144. doi: 10.1007/s13213-010-0125-1
Domizio, P., Romani, C., Lencioni, L., Comitini, F., Gobbi, M., Mannazzu, I., et al. (2011b). Outlining a future for non-Saccharomyces yeasts: Selection of putative spoilage wine strains to be used in association with Saccharomyces cerevisiae for grape juice fermentation. Int. J. Food Microbiol. 147, 170–180. doi: 10.1016/j.ijfoodmicro.2011.03.020
Dujon, B., Sherman, D., Fischer, G., Durrens, P., Casaregola, S., Lafontaine, I., et al. (2004). Genome evolution in yeasts. Nature 430, 35–44. doi: 10.1038/nature02579
Egli, C. M., Edinger, W. D., Mitrakul, C. M., and Henick-Kling, T. (1998). Dynamics of indigenous and inoculated yeast populations and their effect on the sensory character of Riesling and Chardonnay wines. J. Appl. Microbiol. 85, 779–789. doi: 10.1046/j.1365-2672.1998.00521.x
Ehrenreich, I. M., Torabi, N., Jia, Y., Kent, J., Martis, S., Shapiro, J. A., et al. (2010). Dissection of genetically complex traits with extremely large pools of yeast segregants. Nature 464, 1039–1042. doi: 10.1038/nature08923
Englezos, V., Rantsiou, K., Torchio, F., Rolle, L., Gerbi, V., and Cocolin, L. (2015). Exploitation of the non-Saccharomyces yeast Starmerella bacillaris (synonym Candida zemplinina) in wine fermentation: physiological and molecular characterizations. Int. J. Food Microbiol. 199, 33–40. doi: 10.1016/j.ijfoodmicro.2015.01.009
Erny, C., Raoult, P., Alais, A., Butterlin, G., Delobel, P., Matei-Radoi, F., et al. (2012). Ecological success of a group of Saccharomyces cerevisiae/Saccharomyces kudriavzevii hybrids in the Northern European wine making environment. Appl. Environ. Microbiol. 8, 3256–3265. doi: 10.1128/AEM.06752-11
Esteve-Zarzoso, B., Peris-Torán, M., Ramón, D., and Querol, A. (2001). Molecular characterisation of Hanseniaspora species. Antonie van Leeuwenhoek 80, 85–92. doi: 10.1023/A:1012268931569
Fay, J. C., and Benavides, J. A. (2005). Evidence for domesticated and wild populations of Saccharomyces cerevisiae. PLoS Genet. 1, 66–71. doi: 10.1371/journal.pgen.0010005
Fleet, G. H. (2003). Yeast interactions and wine flavour. Int. J. Food Microbiol. 86, 11–22. doi: 10.1016/S0168-1605(03)00245-9
Foury, F., Roganti, T., Lecrenier, N., and Purnelle, B. (1998). The complete sequence of the mitochondrial genome of Saccharomyces cerevisiae. FEBS Lett. 440, 325–331. doi: 10.1016/S0014-5793(98)01467-7
Freel, K. C., Friedrich, A., Hou, J., and Schacherer, J. (2014). Population genomic analysis reveals highly conserved mitochondrial genomes in the yeast species Lachancea thermotolerans. Genome Biol. Evol. 6, 2586–2594. doi: 10.1093/gbe/evu203
Friel, D., Vandenbol, M., and Jijakli, M. H. (2005). Genetic characterization of the yeast Pichia anomala (strain K), an antagonist of postharvest diseases of apple. J. Appl. Microbiol. 98, 783–788. doi: 10.1111/j.1365-2672.2004.02520.x
García-Ríos, E., Gutiérrez, A., Salvadó, Z., Arroyo-López, F. N., and Guillamon, J. M. (2014). The fitness advantage of commercial wine yeasts in relation to the nitrogen concentration, temperature, and ethanol content under microvinification conditions. Appl. Environ. Microbiol. 80, 704–713. doi: 10.1128/AEM.03405-13
Giaramida, P., Ponticello, G., Di Maio, S., Squadrito, M., Genna, G., Barone, E., et al. (2013). Candida zemplinina for production of wines with less alcohol and more glycerol. S. Afr. J. Enol. Viticult. 34, 204–211. doi: 10.1128/AEM.06768-11
Giorello, F. M., Berna, L., Greif, G., Camesasca, L., Salzman, V., Medina, K., et al. (2014). Genome sequence of the native apiculate wine yeast Hanseniaspora vineae T02/19AF. Genome Announc 2, e00530–e00614. doi: 10.1128/genomeA.00530-14
Giudici, P., Solieri, L., Pulvirenti, A. M., and Cassanelli, S. (2005). Strategies and perspectives for genetic improvement of wine yeasts. Appl. Microbiol. Biotechnol. 66, 622–628. doi: 10.1007/s00253-004-1784-2
Goddard, M. R. (2008). Quantifying the complexities of Saccharomyces cerevisiae's ecosystem engineering via fermentation. Ecology 89, 2077–2082. doi: 10.1890/07-2060.1
Goddard, M. R., Anfang, N., Tang, R., Gardner, R. C., and Jun, C. (2010). A distinct population of Saccharomyces cerevisiae in New Zealand: evidence for local dispersal by insects and human-aided global dispersal in oak barrels. Environ. Microbiol. 12, 63–73. doi: 10.1111/j.1462-2920.2009.02035.x
Goffeau, A., Barrell, B. G., Bussey, H., Davis, R. W., Dujon, B., Feldmann, H., et al. (1996). Life with 6000 genes. Science 274, 546; 563–547. doi: 10.1126/science.274.5287.546
Gomez-Angulo, J., Vega-Alvarado, L., Escalante-García, Z., Grande, R., Gschaedler-Mathis, A., Amaya-Delgado, L., et al. (2015). Genome sequence of Torulaspora delbrueckii NRRL Y-50541, isolated from mezcal fermentation. Genome Announc. 3, e00438–e00515. doi: 10.1128/genomeA.00438-15
Gordon, J. L., Armisén, D., Proux-Wéra, E., ÓhÉigeartaigh, S. S., Byrne, K. P., and Wolfe, K. H. (2011). Evolutionary erosion of yeast sex chromosomes by mating-type switching accidents. Proc. Natl. Acad. Sci. U.S.A. 108, 20024–20029. doi: 10.1073/pnas.1112808108
Grangeteau, C., Gerhards, D., Rousseaux, S., von Wallbrunn, C., Alexandre, H., and Guilloux-Benatier, M. (2015). Diversity of yeast strains of the genus Hanseniaspora in the winery environment: what is their involvement in grape must fermentation? Food Microbiol. 50, 70–77. doi: 10.1016/j.fm.2015.03.009
Hall, B., Durall, D. M., and Stanley, G. (2011). Population dynamics of Saccharomyces cerevisiae during spontaneous fermentation at a British Columbia Winery. Am. J. Enol. Vitic. 62, 66–72. doi: 10.5344/ajev.2010.10054
Hong, Y.-A., and Park, H.-D. (2013). Role of non-Saccharomyces yeasts in Korean wines produced from campbell early grapes: potential use of Hanseniaspora uvarum as a starter culture. Food Microbiol. 34, 207–214. doi: 10.1016/j.fm.2012.12.011
Howell, K. S., Cozzolino, D., Bartowsky, E. J., Fleet, G. H., and Henschke, P. A. (2006). Metabolic profiling as a tool for revealing Saccharomyces interactions during wine fermentation. FEMS Yeast Res. 6, 91–101. doi: 10.1111/j.1567-1364.2005.00010.x
Jara, M., Cubillos, F. A., García, V., Salinas, F., Aguilera, O., Liti, G., et al. (2014). Mapping genetic variants underlying differences in the central nitrogen metabolism in fermenter yeasts. PLoS ONE 9:e86533. doi: 10.1371/journal.pone.0086533
Jarosz, D. F., Brown, J. C., Walker, G. A., Datta, M. S., Ung, W. L., Lancaster, A. K., et al. (2014). Cross-kingdom chemical communication drives a heritable, mutually beneficial prion-based transformation of metabolism. Cell 158, 1083–1093. doi: 10.1016/j.cell.2014.07.025
Johnson, L. J., Koufopanou, V., Goddard, M. R., Hetherington, R., Schäfer, S. M., and Burt, A. (2004). Population genetics of the wild yeast Saccharomyces paradoxus. Genetics 166, 43–52. doi: 10.1534/genetics.166.1.43
Jolly, N. P., Augustyn, O. P. H., and Pretorius, I. S. (2006). The role and use of non-Saccharomyces yeasts in wine production. S. Afr. J. Enol. Viticult. 27, 15–39.
Jung, P. P., Friedrich, A., Reisser, C., Hou, J., and Schacherer, J. (2012). Mitochondrial genome evolution in a single protoploid yeast species. G3 2, 1103–1111. doi: 10.1534/g3.112.003152
Kemsawasd, V., Branco, P., Almeida, M. G., Caldeira, J., Albergaria, H., and Arneborg, N. (2015). Cell-to-cell contact and antimicrobial peptides play a combined role in the death of Lachanchea thermotolerans during mixed-culture alcoholic fermentation with Saccharomyces cerevisiae. FEMS Microbiol. Lett. 362:fnv103. doi: 10.1093/femsle/fnv103
Kimura, M. (1980). A simple method for estimating evolutionary rates of base substitutions through comparative studies of nucleotide sequences. J. Mol. Evol. 16, 111–120. doi: 10.1007/BF01731581
King, E. S., Swiegers, J. H., Travis, B., Francis, I. L., Bastian, S. E. P., and Pretorius, I. S. (2008). Coinoculated fermentations using Saccharomyces yeasts affect the volatile composition and sensory properties of Vitis vinifera L. cv. Sauvignon Blanc Wines. J. Agric. Food Chem. 56, 10829–10837. doi: 10.1021/jf801695h
Knop, M. (2006). Evolution of the hemiascomycete yeasts: on life styles and the importance of inbreeding. Bioessays 28, 696–708. doi: 10.1002/bies.20435
Kreger-van Rij, N. J. W. (1977). Ultrastructure of Hanseniaspora ascospores. Antonie Van Leeuwenhoek 43, 225–232. doi: 10.1007/BF00395677
Kumar, S., Randhawa, A., Ganesan, K., Raghava, G. P. S., and Mondal, A. K. (2012). Draft genome sequence of salt-tolerant yeast Debaryomyces hansenii var. hansenii MTCC 234. Eukaryotic Cell 11, 961–962. doi: 10.1128/EC.00137-12
Kurtzman, C. P., Fell, J. W., and Boekhout, T. (2011). The Yeasts: A Taxonomic Study. Amsterdam: Elsevier.
Kvitek, D. J., Will, J. L., and Gasch, A. P. (2008). Variations in Stress Sensitivity and Genomic Expression in Diverse, S. cerevisiae Isolates. PLoS Genet. 4:e1000223. doi: 10.1371/journal.pgen.1000223
Landry, C. R., Oh, J., Hartl, D. L., and Cavalieri, D. (2006). Genome-wide scan reveals that genetic variation for transcriptional plasticity in yeast is biased towards multi-copy and dispensable genes. Gene 366, 343–351. doi: 10.1016/j.gene.2005.10.042
Legras, J. L., Merdinoglu, D., Cornuet, J. M., and Karst, F. (2007). Bread, beer and wine: Saccharomyces cerevisiae diversity reflects human history. Mol. Ecol. 16, 2091–2102. doi: 10.1111/j.1365-294X.2007.03266.x
Legras, J. L., Ruh, O., Merdinoglu, D., and Karst, F. (2005). Selection of hypervariable microsatellite loci for the characterization of Saccharomyces cerevisiae strains. Int. J. Food Microbiol. 102, 73–83. doi: 10.1016/j.ijfoodmicro.2004.12.007
Liti, G., Carter, D. M., Moses, A. M., Warringer, J., Parts, L., James, S. A., et al. (2009). Population genomics of domestic and wild yeasts. Nature 458, 337–341. doi: 10.1038/nature07743
Liti, G., and Louis, E. J. (2005). Yeast evolution and comparative genomics. Annu. Rev. Microbiol. 59, 135–153. doi: 10.1146/annurev.micro.59.030804.121400
Liti, G., Peruffo, A., James, S. A., Roberts, I. N., and Louis, E. J. (2005). Inferences of evolutionary relationships from a population survey of LTR-retrotransposons and telomeric-associated sequences in the Saccharomyces sensu stricto complex. Yeast 22, 177–192. doi: 10.1002/yea.1200
Magyar, I., Nyitrai-Sárdy, D., Leskó, A., Pomázi, A., and Kállay, M. (2014). Anaerobic organic acid metabolism of Candida zemplinina in comparison with Saccharomyces wine yeasts. Int. J. Food Microbiol. 178, 1–6. doi: 10.1016/j.ijfoodmicro.2014.03.002
Magyar, I., and Tóth, T. (2011). Comparative evaluation of some oenological properties in wine strains of Candida stellata, Candida zemplinina, Saccharomyces uvarum and Saccharomyces cerevisiae. Food Microbiol. 28, 94–100. doi: 10.1016/j.fm.2010.08.011
Malpertuy, A., Llorente, B., Blandin, G., Artiguenave, F., Wincker, P., and Dujon, B. (2000). Genomic exploration of the hemiascomycetous yeasts: 10. Kluyveromyces thermotolerans. FEBS Lett. 487, 61–65. doi: 10.1016/S0014-5793(00)02281-X
Mangani, S., Buscioni, G., Collina, L., Bocci, E., and Vincenzini, M. (2011). Effects of microbial populations on anthocyanin profile of sangiovese wines produced in Tuscany, Italy. Am. J. Enol. Vitic. 62, 487–494. doi: 10.5344/ajev.2011.11047
Marsit, S., Mena, A., Bigey, F., Sauvage, F. X., Couloux, A., Guy, J., et al. (2015). Evolutionary advantage conferred by an eukaryote-to-eukaryote gene transfer event in wine yeasts. Mol. Biol. Evol. 32, 1695–1707. doi: 10.1093/molbev/msv057
Marullo, P., Aigle, M., Bely, M., Masneuf-Pomarède, I., Durrens, P., Dubourdieu, D., et al. (2007). Single QTL mapping and nucleotide-level resolution of a physiologic trait in wine Saccharomyces cerevisiae strains. FEMS Yeast Res. 7, 941–952. doi: 10.1111/j.1567-1364.2007.00252.x
Marullo, P., Bely, M., Masneuf-Pomarède, I., Pons, M., Aigle, M., and Dubourdieu, D. (2006). Breeding strategies for combining fermentative qualities and reducing off-flavor production in a wine yeast model. FEMS Yeast Res. 6, 268–279. doi: 10.1111/j.1567-1364.2006.00034.x
Marullo, P., and Dubourdieu, D. (2010). “Yeast selection for wine flavour modulation,” in Managing Wine Quality, Vol. 2, ed A. G. Reynolds (Cambridge: Woodhead Publishing), 293–345.
Masneuf-Pomarede, I., Bely, M., Marullo, P., Lonvaud-Funel, A., and Dubourdieu, D. (2010). Reassessment of phenotypic traits for Saccharomyces bayanus var. uvarum wine yeast strains. Int. J. Food Microbiol. 139, 79–86. doi: 10.1016/j.ijfoodmicro.2010.01.038
Masneuf-Pomarede, I., Juquin, E., Miot-Sertier, C., Renault, P., Laizet, Y. H., Salin, F., et al. (2015). The yeast Starmerella bacillaris (synonym Candida zemplinina) shows high genetic diversity in winemaking environments. 15:fov045. FEMS Yeast Res. doi: 10.1093/femsyr/fov045
Masneuf-Pomarede, I., Le Jeune, C., Durrens, P., Lollier, M., Aigle, M., and Dubourdieu, D. (2007). Molecular typing of wine yeast strains Saccharomyces bayanus var. uvarum using microsatellite markers. Syst. Appl. Microbiol. 30, 75–82. doi: 10.1016/j.syapm.2006.02.006
Masneuf-Pomarede, I., Salin, F., Coton, E., Coton, M., Le Jeune, C., Lonvaud-Funel, A., et al. (2009). “First insight into the genetic structure of S. bayanus var. uvarum revealed by microsatellite markers analysis,” in 27th ISSY (Paris).
Maturano, Y. P., Mestre, M. V., Esteve-Zarzoso, B., Nally, M. C., Lerena, M. C., Toro, M. E., et al. (2015). Yeast population dynamics during prefermentative cold soak of Cabernet Sauvignon and Malbec wines. Int. J. Food Microbiol. 199, 23–32. doi: 10.1016/j.ijfoodmicro.2015.01.005
Miller, M., Kock, J. L. F., Pretorius, G. H. J., and Coetzee, D. J. (1989). The value of orthogonal-field-alternation gel electrophoresis and other criteria in the taxonomy of the Genus Pichia Hansen emend. Kurtzman. Syst. Appl. Microbiol. 12, 191–202. doi: 10.1016/S0723-2020(89)80014-1
Mira, N. P., Münsterkötter, M., Dias-Valada, F., Santos, J., Palma, M., Roque, F. C., et al. (2014). The genome sequence of the highly acetic acid-tolerant Zygosaccharomyces bailii-derived interspecies hybrid strain ISA1307, isolated from a sparkling wine plant. DNA Res. 21, 299–313. doi: 10.1093/dnares/dst058
Moreira, N., Mendes, F., Guedes de Pinho, P., Hogg, T., and Vasconcelos, I. (2008). Heavy sulphur compounds, higher alcohols and esters production profile of Hanseniaspora uvarum and Hanseniaspora guilliermondii grown as pure and mixed cultures in grape must. Int. J. Food Microbiol. 124, 231–238. doi: 10.1016/j.ijfoodmicro.2008.03.025
Muller, L. A. H., and McCusker, J. H. (2009). Microsatellite analysis of genetic diversity among clinical and nonclinical Saccharomyces cerevisiae isolates suggests heterozygote advantage in clinical environments. Mol. Ecol. 18, 2779–2786. doi: 10.1111/j.1365-294X.2009.04234.x
Murphy, H. A., and Zeyl, C. W. (2010). Yeast sex: surprisingly high rates of outcrossing between asci. PLoS ONE 5:e10461. doi: 10.1371/journal.pone.0010461
Naumov, G. I., Masneuf, I., Naumova, E. S., Aigle, M., and Dubourdieu, D. (2000). Association of Saccharomyces bayanus var. uvarum with some French wines: genetic analysis of yeast populations. Res. Microbiol. 151, 683–691. doi: 10.1016/S0923-2508(00)90131-1
Naumov, G. I., and Naumova, E. S. (2009). Chromosomal differentiation of the sibling species Pichia membranifaciens and Pichia manshurica. Microbiology 78, 214–217. doi: 10.1134/S002626170902012X
Nissen, P., and Arneborg, N. (2003). Characterization of early deaths of non-Saccharomyces yeasts in mixed cultures with Saccharomyces cerevisiae. Arch. Microbiol. 180, 257–263. doi: 10.1007/s00203-003-0585-9
Nissen, P., Nielsen, D., and Arneborg, N. (2003). Viable Saccharomyces cerevisiae cells at high concentrations cause early growth arrest of non-Saccharomyces yeasts in mixed cultures by a cell–cell contact-mediated mechanism. Yeast 20, 331–341. doi: 10.1002/yea.965
Novo, M., Bigey, F., Beyne, E., Galeote, V., Gavory, F., Mallet, S., et al. (2009). Eukaryote-to-eukaryote gene transfer events revealed by the genome sequence of the wine yeast Saccharomyces cerevisiae EC1118. Proc. Natl. Acad. Sci. U.S.A. 106, 16333–16338. doi: 10.1073/pnas.0904673106
Pacheco, A., Almeida, M. J., and Sousa, M. J. (2009). Improved gene disruption method for Torulaspora delbrueckii. FEMS Yeast Res. 9, 158–160. doi: 10.1111/j.1567-1364.2008.00452.x
Palanca, L., Gaskett, A. C., Günther, C. S., Newcomb, R. D., and Goddard, M. R. (2013). Quantifying variation in the ability of yeasts to attract Drosophila melanogaster. PLoS ONE 8:e75332. doi: 10.1371/journal.pone.0075332
Paradis, E., Claude, J., and Strimmer, K. (2004). APE: analyses of phylogenetics and evolution in R language. Bioinformatics 20, 289–290. doi: 10.1093/bioinformatics/btg412
Parapouli, M., Hatziloukas, E., Drainas, C., and Perisynakis, A. (2010). The effect of Debina grapevine indigenous yeast strains of Metschnikowia and Saccharomyces on wine flavour. J. Indust. Microbiol. Biotechnol. 37, 85–93. doi: 10.1007/s10295-009-0651-7
Parle, J. N., and Di Menna, M. E. (1966). The source of yeasts in New Zealand wines. N.Z. J. Agric. Res. 9, 98–107. doi: 10.1080/00288233.1966.10418122
Pérez-Nevado, F., Albergaria, H., Hogg, T., and Girio, F. (2006). Cellular death of two non-Saccharomyces wine-related yeasts during mixed fermentations with Saccharomyces cerevisiae. Int. J. Food Microbiol. 108, 336–345. doi: 10.1016/j.ijfoodmicro.2005.12.012
Pfliegler, W. P., Horváth, E., Kallai, Z., and Sipiczki, M. (2014). Diversity of Candida zemplinina isolates inferred from RAPD, micro/minisatellite and physiological analysis. Microbiol. Res. 169, 402–410. doi: 10.1016/j.micres.2013.09.006
Picazo, C., Gamero-Sandemetrio, E., Orozco, H., Albertin, W., Marullo, P., Matallana, E., et al. (2015). Mitochondria inheritance is a key factor for tolerance to dehydration in wine yeast production. Lett. Appl. Microbiol. 60, 217–222. doi: 10.1111/lam.12369
Pina, A., Calderón, I. L., and Benítez, T. (1986). Intergeneric hybrids of Saccharomyces cerevisiae and Zygosaccharomyces fermentati obtained by protoplast fusion. Appl. Environ. Microbiol. 51, 995–1003.
Plech, M., de Visser, J. A., and Korona, R. (2014). Heterosis is prevalent among domesticated but not wild strains of Saccharomyces cerevisiae. G3 4, 315–323. doi: 10.1534/g3.113.009381
Pramateftaki, P. V., Kouvelis, V. N., Lanaridis, P., and Typas, M. A. (2006). The mitochondrial genome of the wine yeast Hanseniaspora uvarum: a unique genome organization among yeast/fungal counterparts. FEMS Yeast Res. 6, 77–90. doi: 10.1111/j.1567-1364.2005.00018.x
Pramateftaki, P. V., Kouvelis, V. N., Lanaridis, P., and Typas, M. A. (2008). Complete mitochondrial genome sequence of the wine yeast Candida zemplinina: intraspecies distribution of a novel group-IIB1 intron with eubacterial affiliations. FEMS Yeast Res. 8, 311–327. doi: 10.1111/j.1567-1364.2007.00332.x
Pretorius, I. S. (2000). Tailoring wine yeast for the new millennium: novel approaches to the ancient art of winemaking. Yeast 16, 675–729. doi: 10.1002/1097-0061(20000615)16:8<675::AID-YEA585>3.0.CO;2-B
Ramírez, M., Velázquez, R., Maqueda, M., López-Piñeiro, A., and Ribas, J. C. (2015). A new wine Torulaspora delbrueckii killer strain with broad antifungal activity and its toxin-encoding double-stranded RNA virus. Front. Microbiol. 6:983. doi: 10.3389/fmicb.2015.00983
Renault, P. E., Albertin, W., and Bely, M. (2013). An innovative tool reveals interaction mechanisms among yeast populations under oenological conditions. Appl. Microbiol. Biotechnol. 97, 4105–4119. doi: 10.1007/s00253-012-4660-5
Renault, P., Miot-Sertier, C., Marullo, P., Hernández-Orte, P., Lagarrigue, L., Lonvaud-Funel, A., et al. (2009). Genetic characterization and phenotypic variability in Torulaspora delbrueckii species: potential applications in the wine industry. Int. J. Food Microbiol. 134, 201–210. doi: 10.1016/j.ijfoodmicro.2009.06.008
Richards, K. D., Goddard, M. R., and Gardner, R. C. (2009). A database of microsatellite genotypes for Saccharomyces cerevisiae. Antonie Van Leeuwenhoek 96, 355–359. doi: 10.1007/s10482-009-9346-3
Roberts, I. N., and Oliver, S. G. (2011). The yin and yang of yeast: biodiversity research and systems biology as complementary forces driving innovation in biotechnology. Biotechnol. Lett. 33, 477–487. doi: 10.1007/s10529-010-0482-7
Rodrigues, F., Ludovico, P., Sousa, M. J., Steensma, H. Y., Côrte-Real, M., and Leão, C. (2003). The spoilage yeast Zygosaccharomyces bailii forms mitotic spores: a screening method for haploidization. Appl. Environ. Microbiol. 69, 649–653. doi: 10.1128/AEM.69.1.649-653.2003
Rojas, V., Gil, J. V., Piñaga, F., and Manzanares, P. (2001). Studies on acetate ester production by non-Saccharomyces wine yeasts. Int. J. Food Microbiol. 70, 283–289. doi: 10.1016/S0168-1605(01)00552-9
Ruderfer, D. M., Pratt, S. C., Seidel, H. S., and Kruglyak, L. (2006). Population genomic analysis of outcrossing and recombination in yeast. Nat. Genet. 38, 1077–1081. doi: 10.1038/ng1859
Sadoudi, M., Tourdot-Maréchal, R., Rousseaux, S., Steyer, D., Gallardo-Chacón, J. J., Ballester, J., et al. (2012). Yeast-yeast interactions revealed by aromatic profile analysis of Sauvignon Blanc wine fermented by single or co-culture of non-Saccharomyces and Saccharomyces yeasts. Food Microbiol. 32, 243–253. doi: 10.1016/j.fm.2012.06.006
Salinas, F., Cubillos, F. A., Soto, D., Garcia, V., Bergström, A., Warringer, J., et al. (2012). The genetic basis of natural variation in oenological traits in Saccharomyces cerevisiae. PLoS ONE 7:e49640. doi: 10.1371/journal.pone.0049640
Salvadó, Z., Arroyo-López, F. N., Barrio, E., Querol, A., and Guillamón, J. M. (2011). Quantifying the individual effects of ethanol and temperature on the fitness advantage of Saccharomyces cerevisiae. Food Microbiol. 28, 1155–1161. doi: 10.1016/j.fm.2011.03.008
Sarilar, V., Bleykasten-Grosshans, C., and Neuvéglise, C. (2015). Evolutionary dynamics of hAT DNA transposon families in Saccharomycetaceae. Genome Biol. Evol. 7, 172–190. doi: 10.1093/gbe/evu273
Scannell, D. R., Butler, G., and Wolfe, K. H. (2007). Yeast genome evolution–the origin of the species. Yeast 24, 929–942. doi: 10.1002/yea.1515
Schacherer, J., Shapiro, J. A., Ruderfer, D. M., and Kruglyak, L. (2009). Comprehensive polymorphism survey elucidates population structure of Saccharomyces cerevisiae. Nature 458, 342–345. doi: 10.1038/nature07670
Schuller, D., Cardoso, F., Sousa, S., Gomes, P., Gomes, A. C., Santos, M. A., et al. (2012). Genetic diversity and population structure of Saccharomyces cerevisiae strains isolated from different grape varieties and winemaking regions. PLoS ONE 7:e32507. doi: 10.1371/journal.pone.0032507
Sicard, D., and Legras, J. L. (2011). Bread, beer and wine: yeast domestication in the Saccharomyces sensu stricto complex. C. R. Biol. 334, 229–236. doi: 10.1016/j.crvi.2010.12.016
Sipiczki, M. (2004). Species identification and comparative molecular and physiological analysis of Candida zemplinina and Candida stellata. J. Basic Microbiol. 44, 471–479. doi: 10.1002/jobm.200410449
Sniegowski, P. D., Dombrowski, P. G., and Fingerman, E. (2002). Saccharomyces cerevisiae and Saccharomyces paradoxus coexist in a natural woodland site in North America and display different levels of reproductive isolation from European conspecifics. FEMS Yeast Res. 1, 299–306. doi: 10.1111/j.1567-1364.2002.tb00048.x
Solieri, L., Dakal, T., and Giudici, P. (2013). Next-generation sequencing and its potential impact on food microbial genomics. Ann. Microbiol. 63, 21–37. doi: 10.1007/s13213-012-0478-8
Souciet, J. L., Dujon, B., Gaillardin, C., Johnston, M., Baret, P. V., Cliften, P., et al. (2009). Comparative genomics of protoploid Saccharomycetaceae. Genome Res. 19, 1696–1709. doi: 10.1101/gr.091546.109
Starmer, W. T., Ganter, P. F., and Aberdeen, V. (1992). Geographic distribution and genetics of killer phenotypes for the yeast Pichia kluyveri across the United States. Appl. Environ. Microbiol. 58, 990–997.
Steensels, J., Snoek, T., Meersman, E., Picca Nicolino, M., Voordeckers, K., and Verstrepen, K. J. (2014). Improving industrial yeast strains: exploiting natural and artificial diversity. FEMS Microbiol. Rev. 38, 947–995. doi: 10.1111/1574-6976.12073
Steensels, J., and Verstrepen, K. J. (2014). Taming wild yeast: potential of conventional and nonconventional yeasts in industrial fermentations. Annu. Rev. Microbiol. 68, 61–80. doi: 10.1146/annurev-micro-091213-113025
Stefanini, I., Dapporto, L., Legras, J. L., Calabretta, A., Di Paola, M., De Filippo, C., et al. (2012). Role of social wasps in Saccharomyces cerevisiae ecology and evolution. Proc. Natl. Acad. Sci. U.S.A. 109, 13398–13403. doi: 10.1073/pnas.1208362109
Strope, P. K., Skelly, D. A., Kozmin, S. G., Mahadevan, G., Stone, E. A., Magwene, P. M., et al. (2015). The 100-genomes strains, an S. cerevisiae resource that illuminates its natural phenotypic and genotypic variation and emergence as an opportunistic pathogen. Genome Res. 25, 762–774. doi: 10.1101/gr.185538.114
Sutterlin, K. A. (2010). Fructophilic Yeasts to Cure Stuck Fermentations in Alcoholic Beverages. Ph.D. thesis, University of Stellenbosch.
Talla, E., Anthouard, V., Bouchier, C., Frangeul, L., and Dujon, B. (2005). The complete mitochondrial genome of the yeast Kluyveromyces thermotolerans. FEBS Lett. 579, 30–40. doi: 10.1016/j.febslet.2004.10.106
Timberlake, W. E., Frizzell, M. A., Richards, K. D., and Gardner, R. C. (2011). A new yeast genetic resource for analysis and breeding. Yeast 28, 63–80. doi: 10.1002/yea.1821
Tofalo, R., Perpetuini, G., Fasoli, G., Schirone, M., Corsetti, A., and Suzzi, G. (2014). Biodiversity study of wine yeasts belonging to the “terroir” of Montepulciano d'Abruzzo “Colline Teramane” revealed Saccharomyces cerevisiae strains exhibiting atypical and unique 5.8S-ITS restriction patterns. Food Microbiol. 39, 7–12. doi: 10.1016/j.fm.2013.10.001
Tofalo, R., Perpetuini, G., Schirone, M., Fasoli, G., Aguzzi, I., Corsetti, A., et al. (2013). Biogeographical characterization of Saccharomyces cerevisiae wine yeast by molecular methods. Front. Microbiol. 4:166. doi: 10.3389/fmicb.2013.00166
Tofalo, R., Schirone, M., Torriani, S., Rantsiou, K., Cocolin, L., Perpetuini, G., et al. (2012). Diversity of Candida zemplinina strains from grapes and Italian wines. Food Microbiol. 29, 18–26. doi: 10.1016/j.fm.2011.08.014
Viana, F., Belloch, C., Vallés, S., and Manzanares, P. (2011). Monitoring a mixed starter of Hanseniaspora vineae–Saccharomyces cerevisiae in natural must: impact on 2-phenylethyl acetate production. Int. J. Food Microbiol. 151, 235–240. doi: 10.1016/j.ijfoodmicro.2011.09.005
Viana, F., Gil, J. V., Genovés, S., Vallés, S., and Manzanares, P. (2008). Rational selection of non-Saccharomyces wine yeasts for mixed starters based on ester formation and enological traits. Food Microbiol. 25, 778–785. doi: 10.1016/j.fm.2008.04.015
Wang, C., and Liu, Y. (2013). Dynamic study of yeast species and Saccharomyces cerevisiae strains during the spontaneous fermentations of Muscat blanc in Jingyang, China. Food Microbiol. 33, 172–177. doi: 10.1016/j.fm.2012.09.014
Wang, C., Mas, A., and Esteve-Zarzoso, B. (2015). Interaction between Hanseniaspora uvarum and Saccharomyces cerevisiae during alcoholic fermentation. Int. J. Food Microbiol. 206, 67–74. doi: 10.1016/j.ijfoodmicro.2015.04.022
Wang, Q.-M., Liu, W.-Q., Liti, G., Wang, S.-A., and Bai, F.-Y. (2012). Surprisingly diverged populations of Saccharomyces cerevisiae in natural environments remote from human activity. Mol. Ecol. 21, 5404–5417. doi: 10.1111/j.1365-294X.2012.05732.x
Warringer, J., Zörgö, E., Cubillos, F. A., Zia, A., Gjuvsland, A., Simpson, J. T., et al. (2011). Trait variation in yeast is defined by population history. PLoS Genet. 7:e1002111. doi: 10.1371/journal.pgen.1002111
Wrent, P., Rivas, E. M., Peinado, J. M., and de Silóniz, M. I. (2015). Development of an affordable typing method for Meyerozyma guilliermondii using microsatellite markers. Int. J. Food Microbiol. 217, 1–6. doi: 10.1016/j.ijfoodmicro.2015.10.008
Wu, B., Buljic, A., and Hao, W. (2015). Extensive horizontal transfer and homologous recombination generate highly chimeric mitochondrial genomes in yeast. Mol. Biol. Evol. 32, 2559–2570. doi: 10.1093/molbev/msv127
Yanai, T., and Sato, M. (1999). Isolation and properties of β-glucosidase produced by Debaryomyces hansenii and its application in winemaking. Am. J. Enol. Vitic. 50, 231–235.
Zara, G., Mannazzu, I., Del Caro, A., Budroni, M., Pinna, M. B., Murru, M., et al. (2014). Wine quality improvement through the combined utilisation of yeast hulls and Candida zemplinina/Saccharomyces cerevisiae mixed starter cultures. Aust. J. Grape Wine Res. 20, 199–207. doi: 10.1111/ajgw.12078
Zeyl, C. (2004). Capturing the adaptive mutation in yeast. Res. Microbiol. 155, 217–223. doi: 10.1016/j.resmic.2003.12.006
Zimmer, A., Durand, C., Loira, N., Durrens, P., Sherman, D. J., and Marullo, P. (2014). QTL dissection of lag phase in wine fermentation reveals a new translocation responsible for Saccharomyces cerevisiae adaptation to sulfite. PLoS ONE 9:e86298. doi: 10.1371/journal.pone.0086298
Keywords: non-conventional yeast, non-Saccharomyces, wine, enology, oenology, microsatellite
Citation: Masneuf-Pomarede I, Bely M, Marullo P and Albertin W (2016) The Genetics of Non-conventional Wine Yeasts: Current Knowledge and Future Challenges. Front. Microbiol. 6:1563. doi: 10.3389/fmicb.2015.01563
Received: 06 November 2015; Accepted: 23 December 2015;
Published: 11 January 2016.
Edited by:
Gemma Beltran, Universitat Rovira i Virgili, SpainReviewed by:
Aspasia Nisiotou, Technological Educational Institute of Athens, GreecePilar Morales, Consejo Superior de Investigaciones Científicas, Spain
Copyright © 2016 Masneuf-Pomarede, Bely, Marullo and Albertin. This is an open-access article distributed under the terms of the Creative Commons Attribution License (CC BY). The use, distribution or reproduction in other forums is permitted, provided the original author(s) or licensor are credited and that the original publication in this journal is cited, in accordance with accepted academic practice. No use, distribution or reproduction is permitted which does not comply with these terms.
*Correspondence: Warren Albertin, d2FycmVuLmFsYmVydGluQHUtYm9yZGVhdXguZnI=