- 1Departamento de Biología, División de Ciencias Naturales y Exactas, Campus Guanajuato, Universidad de Guanajuato, Guanajuato, México
- 2Centro de Investigacion y de Estudios Avanzados del Instituto Politécnico Nacional, Irapuato, México
- 3Department of Microbiology, University of Szeged, Szeged, Hungary
The systemic infections caused by members of the Candida parapsilosis complex are currently associated to high morbility and mortality rates, and are considered as relevant as those caused by Candida albicans. Since the fungal cell wall is the first point of contact with the host cells, here we performed a comparison of this organelle in members of the C. parapsilosis complex, and its relevance during interaction with human peripheral blood mononuclear cells (PBMCs). We found that the wall of the C. parapsilosis complex members is similar in composition, but differs to that from C. albicans, with less mannan content and more β-glucan and porosity levels. Furthermore, lectin-based analysis showed increased chitin and β1,3-glucan exposure at the surface of C. parapsilosis sensu lato when compared to C. albicans. Yeast cells of members of the C. parapsilosis complex stimulated more cytokine production by human PBMCs than C. albicans cells; and this significantly changed upon removal of O-linked mannans, indicating this wall component plays a significant role in cytokine stimulation by C. parapsilosis sensu lato. When inner wall components were exposed on the wall surface, C. parapsilosis sensu stricto and C. metapsilosis, but not C. orthopsilosis, stimulated higher cytokine production. Moreover, we found a strong dependency on β1,3-glucan recognition for the members of the C. parapsilosis complex, but not for live C. albicans cells; whereas TLR4 was required for TNFα production by the three members of the complex, and stimulation of IL-6 by C. orthopsilosis. Mannose receptor had a significant role during TNFα and IL-1β stimulation by members of the complex. Finally, we demonstrated that purified N- and O-mannans from either C. parapsilosis sensu lato or C. albicans are capable to block the recognition of these pathogens by human PBMCs. Together; our results suggest that the innate immune recognition of the members of the C. parapsilosis complex is differential of that reported for C. albicans. In addition, we propose that purified cell wall mannans can be used as antagonist to block specific receptors on innate immune cells.
Introduction
Fungal infections are currently a burden for most of the health systems worldwide, and among them, superficial and invasive candidiasis are of special interest, since the latter has a mortality rate higher than 45% in infected patients (Brown et al., 2012). Candida albicans is the most frequent causative agent of candidiasis, being responsible of about 50% of total invasive candidiasis, while other members of the Candida genus, named emerging species, contribute together to the rest of the reported cases (Trofa et al., 2008). Candida parapsilosis sensu lato is a species that is mostly found in neonate patients, causing more than 33% of invasive candidiasis in this group (Pammi et al., 2013). It is a versatile yeast-like organism that, at difference of other pathogenic Candida species, can be found colonizing non-human organisms and inert material from the environment (Trofa et al., 2008). This organism is in fact a complex of three closely related species: C. parapsilosis sensu stricto, Candida orthopsilosis and Candida metapsilosis (Tavanti et al., 2005); which have subtle, but key differences in terms of virulence (Nemeth et al., 2013; Gago et al., 2014), drug sensitivity (Spreghini et al., 2012; Szenzenstein et al., 2013), and secretion of hydrolytic enzymes (Trevino-Rangel Rde et al., 2013).
The establishment of a protective anti-Candida immune response in the host relays on a proper activation of the innate immune branch, and significant efforts have been done to understand this host–pathogen interaction, using C. albicans as a model (Netea et al., 2015). In C. parapsilosis sensu lato, there is currently limited information about the mechanisms underlying its interaction with components of the innate immunity. Thus far, it has been demonstrated that galectin 3 expressed by human neutrophils drives an increased C. parapsilosis phagocytosis, but not when challenged against C. albicans yeast cells (Linden et al., 2013). In addition, human peripheral blood mononuclear cells (PBMCs) stimulated with heat-killed (HK) C. parapsilosis sensu stricto yeast cells produced lower Interleukin (IL) 1β, interferon γ, IL-17 and IL-22, but higher levels of IL-10, when compared to cells confronted with C. albicans (Toth et al., 2013). Despite this progress, there are not reports dealing with the interaction of immune cells with members of the C. parapsilosis complex.
The fungal cell wall contains most of the pathogen-associated molecular patterns recognized by pattern recognition receptors (PRRs) on innate immune cells, and again, the C. albicans cell wall is the best model thus far characterized (Díaz-Jiménez et al., 2012). This structure is composed of four main polysaccharides arranged in two well defined layers: the outermost layer composed of glycoproteins, bearing N- and O-linked mannans, and the components of the inner layer, chitin, β1,6- and β1,3-glucans (Díaz-Jiménez et al., 2012). The participation of this organelle in the activation of the innate immune response has been thoroughly studied. It is now well established that β1,3-glucan is normally hidden from the recognition of dectin-1 and TLR2, and if accessible, plays a major role in the induction of pro-inflammatory cytokines and phagocytosis by macrophages (Gantner et al., 2005; Gow et al., 2007; Heinsbroek et al., 2008). The N-linked mannans play also a significant role in both cytokine stimulation and macrophage uptake, via the mannose receptor (MR), dectin-2, and DC-SIGN (Netea et al., 2006; Mora-Montes et al., 2007, 2010; Cambi et al., 2008; McKenzie et al., 2010; Saijo and Iwakura, 2011). On the contrary, O-linked mannans play a dispensable role in both immunological processes, although it engages to TLR4 (Netea et al., 2006), a potent PRRs that plays a significant role in controlling bacteria (Miyake, 2007). Thus far, there is limited information about the cell wall of members of the C. parapsilosis complex, and the particular contribution of PRRs in the activation of cytokine production. Here, we performed a comparative study of the cell wall composition of C. albicans, C. parapsilosis sensu stricto, C. orthopsilosis, and C. metapsilosis and found that, although the composition is similar, the arrangement of the components has significant differences that impact their ability to activate human PBMCs. Moreover, we demonstrated that purified N- and O-linked mannans from either C. albicans, C. parapsilosis sensu stricto, C. orthopsilosis or C. metapsilosis are capable to block the recognitions of these pathogens by human PBMCs.
Materials and Methods
Strains and Culturing Conditions
Candida albicans SC5314 (Gillum et al., 1984), C. parapsilosis sensu stricto SZMC 8110, C. orthopsilosis SZMC 1545, and C. metapsilosis SZMC 1548 (Szenzenstein et al., 2013) were used in this study. Cells were propagated at 30°C in Sabouraud broth [1% (w/v) mycological peptone, 4% (w/v) glucose], and maintained in plates containing medium added with 2% (w/v) agar. For all the experiments here reported, 500 μL of overnight-grown cells were used to inoculated 100 mL of fresh medium and incubated at 30°C with shaking at 200 rpm, until reach the mid-log growth phase (typically 5–6 h). Cells were incubated at 56°C for 1 h for heat inactivation as reported (Mora-Montes et al., 2007). For all the cases, inactivation was confirmed by loss of fungal growth in Sabouraud medium at 30°C for 72 h. To remove O-linked mannans, cells were incubated overnight with 100 mM NaOH as described (Diaz-Jimenez et al., 2012). Under these conditions, more than 94% cells kept viability, as tested by CFU/mL before and after treatment with NaOH.
Cell Wall Analysis
Cell homogenates were obtained in a Braun homogenizer, with 5 cycles of 30 s and cooling on ice in-between (Mora-Montes et al., 2007). The cell walls were pelleted by centrifugation and thoroughly washed with deionized water. Further wall cleansing was performed with hot 2% (v/v) SDS, 0.3 M β-mercaptoethanol, and 1 M NaCl as described (Mora-Montes et al., 2007). Freeze-dried cell walls were hydrolyzed by adding 2 M trifluoroacetic acid and boiling for 3 h; then, the acid was evaporated, and samples were suspended in deionized water. The hydrolysates were analyzed by High Performance Anion Exchange Chromatography coupled to Pulsed Amperometric Detection (HPAEC-PAD), using a gradient of sodium acetate in 150 mM NaOH (flow 0.5 mL/min) as follows: 0–5 min = 45–75 mM NaOH, 5.1–15.0 min = 90 mM NaOH, 15.1–17.0 min = 105 mM NaOH + 75 mM sodium acetate, 17.1–20.0 min = 75 mM NaOH + 150 mM sodium acetate, and 20.1–25.0 min = 45 mM NaOH, at a column temperature of 25°C. Applied potentials for detection by the amperometric pulse were: E1 (400 ms), E2 (20 ms), E3 (20 ms), and E4 (60 ms) of +0.1, -2.0, +0.6, and -0.1 V, respectively. Protein content was determined upon lyophilized cell walls were alkali-hydrolyzed (Mora-Montes et al., 2007), using the Bradford protein assay.
The cell wall porosity was assessed using the polycation method previously reported (De Nobel et al., 1990). Aliquots containing 1 × 108 cells were pelleted, the supernatant discarded and cells suspended in either 10 mM Tris-HCl, pH 7.4 (buffer A), buffer A plus 30 μg/mL poly-L-lysine (MW 30–70 kDa, Sigma Cat. No. P-2636) or buffer A plus 30 μg/mL DEAE-dextran (MW 500 kDa, Sigma Cat. No. D-9885). Then, cells were incubated at 30°C for 30 min, and shaking (200 rpm), pelleted by centrifuging, the supernatant saved, and further centrifuged before reading the absorbance at 260 nm. The relative cell wall porosity to DEAE-dextran was calculated as described (De Nobel et al., 1990).
The level of cell wall phosphomannan was determined by the ability of cells to bind the cationic dye Alcian Blue as described (Hobson et al., 2004).
Analysis of Chitin and β1,3-Glucan Exposure at the Cell Surface
Cells were labeled with 1 mg/mL fluorescein isothiocyanate-wheat germ agglutinin conjugate (WGA-FITC; Sigma) (Mora-Montes et al., 2011) for chitin staining; while β1,3-glucan was labeled with 5 μg/mL IgG Fc-Dectin-1 chimera (Graham et al., 2006) for 40 min at room temperature, followed by incubating with 1 μg/mL donkey anti Fc IgG-FITC for 40 min at room temperature (Marakalala et al., 2013). In both cases, samples were examined by fluorescence microscopy using a Zeiss Axioscope-40 microscope and an Axiocam MRc camera. Pixels associated to 100 fluorescent cells were obtained with Adobe PhotoshopTM CS6 and the following formula: [(total of green pixels-background green pixels) × 100]/total pixels.
Ethics Statement
Universidad de Guanajuato, though the Ethics Committee, approved the use of human cells in this study (permission number 17082011). Blood samples from healthy adult volunteers were obtained upon information about the study was provided and written informed consent was signed.
Human PBMCs-Candida Interaction
Human PBMCs were isolated by density centrifugation using Histopaque-1077 (Sigma) as reported (Endres et al., 1988). The immune cell-fungus interaction was performed in 96-well microplates with 5 × 105 PBMCs, in 100-μL RPMI 1640 Dutch modification (Sigma), and 100 μL with 1 × 105 fungal cells. When required, PBMCs were pre-incubated for 60 min at 37°C with either 200 μg/mL purified mannan, laminarin (200 μg/mL), anti-MR (10 μg/mL, Invitrogen, Cat. No. Mab-Hmr) or anti-TLR4 (10 μg/mL, Santa Cruz Biotechnology, Cat. No. sc-293072) prior to stimulation with yeast cells. Isotype matched, irrelevant IgG1 antibodies (10 μg/mL, Santa Cruz Biotechnology, Cat. No. sc-52003) were used as controls for experiments assessing MR and TLR4. Despite all the reagents used for the pre-incubation experiments were negative to contamination by LPS (tested with the Limulus amebocyte lysate from Sigma) all reactions were performed in presence of 5 μg/mL polymyxin B (Sigma) (Schwartz et al., 1972). In all cases, the interactions were incubated for 24 h at 37°C with 5% (v/v) CO2, plates were centrifuged for 10 min at 3000 × g and 4°C and supernatants saved and kept at -20°C until used. ELISA kits from Peprotech were used to measure the concentration of TNFα, IL-6, and IL-10; while IL-1β levels were measured using an ELISA kit from R&D Systems. Mock interactions with only human PBMCs incubated with RPMI 1640 Dutch modification were included as negative controls. For all the cases, the amount of cytokine quantified in the negative controls was subtracted before data analysis.
N- and O-Linked Mannan Isolation
The N- and O-linked mannans were isolated, and purified using the endoglycosidase H and β-elimination strategy previously reported by our group (Mora-Montes et al., 2012). Absence of protein and other wall polysaccharides was confirmed by the Bradford method and HPAEC-PAD, respectively (Mora-Montes et al., 2012); while lack of bacterial and fungal contamination was assessed by negative detection of LPS and no growth in LB and Sabouraud broth for 72 h at 37 and 28°C, respectively.
Statistical Analysis
Statistical analysis was performed using GraphPad Prism 6 software. Cytokine stimulation using human PMBCs was performed in duplicate with six healthy donors, whereas the rest of the experiments were performed at least thrice in duplicate. Data represent cumulative results of all experiments performed and are showed as mean ± SD. The Mann–Whitney U test or unpaired t-test was used to establish statistical significance (see figure legends for details), with a significance level set at P < 0.05.
Results
Members of the C. parapsilosis Complex have Similar Composition in the Cell Wall but Differ from that Present in C. albicans
In order to assess the proportions of the main cell wall components in the three members of the C. parapsilosis complex, cell walls where isolated, acid-hydrolyzed and the proportion of N-acetylglucosamine, mannose and glucose, the basic unit of chitin, mannan and β-glucan, respectively (Mora-Montes et al., 2007, 2010, 2012), were quantified by HPAEC-PAD. C. parapsilosis sensu stricto, C. orthopsilosis and C. metapsilosis showed similar levels of the three polysaccharides analyzed (Table 1), but when compared with C. albicans, the species belonging to the complex showed significantly less mannan and increased β-glucan content (Table 1). In terms of chitin content, only C. metapsilosis wall displayed higher content than the C. albicans cell wall (Table 1). No differences were observed for protein content in the four yeast species analyzed (Table 1). Next, in order to confirm the lower mannan content in the members of the C. parapsilosis complex, we assessed the level of phosphomannan content and the wall porosity, parameters that suffer alterations depending on the status of the protein mannosylation pathways (De Nobel et al., 1990; Bates et al., 2005, 2006; Mora-Montes et al., 2007, 2010; West et al., 2013; Lopes-Bezerra et al., 2015). Interestingly, the cell wall from C. parapsilosis sensu stricto, C. orthopsilosis and C. metapsilosis showed lower phosphomannan content and higher porosity, when compared with C. albicans cells (Table 1). To assess whether the β1,3-glucan and chitin fibers in the members of the complex are underneath the mannan layer, as reported in C. albicans (Gow et al., 2007; Mora-Montes et al., 2011), yeast cells were labeled with the IgG Fc-Dectin-1 chimera (Graham et al., 2006), and the lectin-β1,3-glucan interaction revealed with FITC-conjugated IgG; whereas chitin was labeled with WGA-FITC. The analysis of the fluorescence associated to 100 cells indicated that, C. parapsilosis sensu stricto, C. orthopsilosis, and C. metapsilosis have β1,3-glucan and chitin more exposed the at the cell surface than C. albicans cells (Figure 1). However, when compared the three members of the complex, C. orthopsilosis displayed significantly higher levels of both polysaccharides at the wall surface than the other two species (Figure 1). As control, a similar experiment was conducted with HK yeast cells, where the components of the inner wall layer were artificially exposed at the cell wall surface and as consequence, higher labeling with the lectins was observed, with exception of C. orthopsilosis, where HK and live cells had similar content of both polysaccharides at the surface (Figure 1). Overall, these data indicate that the cell wall composition and organization among members of the C. parapsilosis complex differ to that described in C. albicans.
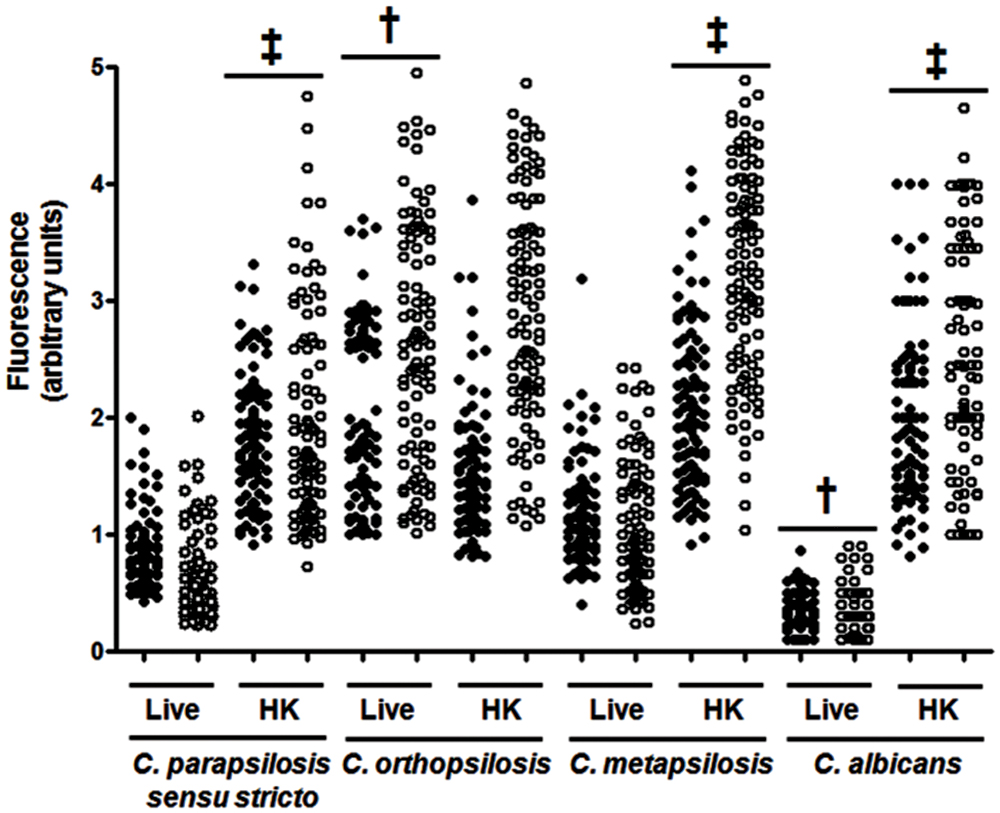
FIGURE 1. Analysis of β1,3-glucan and chitin exposure at the cell wall surface. Live (L) yeast cells were incubated with IgG Fc-Dectin-1and then with FITC-conjugated IgG to label β1,3-glucan (closed circles). Alternatively, chitin was labeled with WGA-FITC (open circles). The fluorescence associated to each cell is plotted. In addition, the experiment was conducted with cells previously heat-inactivated (HK). Strains used are Candida parapsilosis sensu stricto SZMC 8110, C. orthopsilosis SZMC 1545, C. metapsilosis SZMC 1548, and C. albicans SC5314. One hundred cells were analyzed for each group. ‡P < 0.05, when compared with the live cells; †P < 0.05 when compared to the other groups with live cells.
C. albicans and C. parapsilosis sensu lato Differentially Stimulate Cytokine Production by Human PBMCs
The differences in composition and organization of the cell wall above described may suggest that the fungus-immune cell interaction differs between member of the C. parapsilosis complex and C. albicans. Thus, in order to get some insights about such interaction, yeast cells were co-incubated with human PBMCs and secreted cytokines levels were measured as a read out of such interaction. Live C. albicans cells barely stimulated the production of either TNFα, IL-1β, IL-6 or IL-10 (Figure 2); however, C. parapsilosis sensu stricto, C. orthopsilosis, and C. metapsilosis stimulated higher and similar levels of TNFα, IL-1β, and IL-10 (Figure 2). Although IL-6 stimulation was significantly higher during the interaction with any of the three members of the C. parapsilosis complex, C. orthopsilosis stimulated the highest IL-6 production, when compared with the other species analyzed (Figure 2). Next, we compared the cytokine profile stimulated with either live or HK cells with or without β-elimination treatment to remove O-linked mannans from the cell wall (Diaz-Jimenez et al., 2012). As previously reported for C. albicans (Gow et al., 2007), HK yeast cells stimulated significant levels of TNFα, IL-1β, IL-6, and IL-10, when compared to live cells (Figure 3). A subtle, not significant reduction was observed in the production of the cytokines upon stimulation of human PBMCs with β-eliminated HK C. albicans cells (Figure 3). Upon heat-inactivation, C. parapsilosis sensu stricto and C. metapsilosis stimulated increased levels of TNFα, IL-6 and IL-10, and these cytokine levels were similar when live yeast cells were O-deglycosylated, i.e., β-eliminated (Figure 3). No further changes in the cytokine stimulation were observed when this treatment was applied to HK cells (Figure 3). For stimulation of IL-1β, modest levels of this cytokine were obtained, being not as abundant as those stimulated with HK C. albicans cells, as reported (Toth et al., 2013), and displaying no significant changes even though cells were both HK and β-eliminated (Figure 3). For C. orthopsilosis, the heat killing and β-elimination did not affect production of TNFα, IL-1β, IL-6 and IL-10 by human PBMCs. Therefore, C. parapsilosis sensu lato is capable to stimulate a different cytokine production than that observed in C. albicans, when incubated with human PBMCs. Furthermore, these data indicate that C. parapsilosis sensu stricto and C. metapsilosis but not C. orthopsilosis, have a similar interaction with these immune cells.
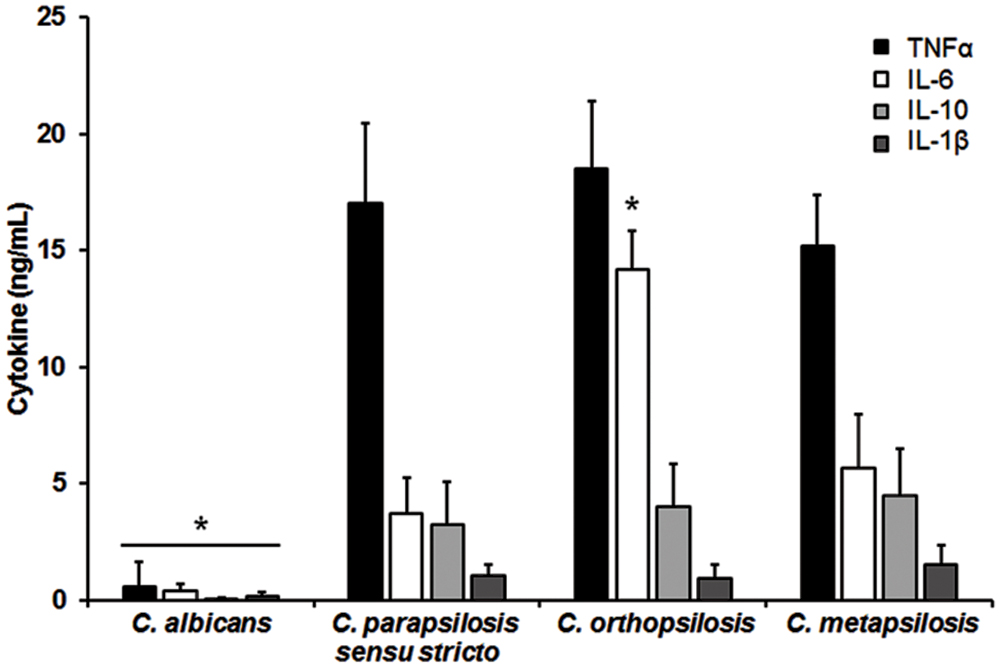
FIGURE 2. Candida albicans and members of the C. parapsilosis complex differentially stimulate the cytokine production by human PBMCs. Human PBMCs were co-incubated 24 h with live yeast cells, the supernatant collected and used to quantify the cytokine levels. Strains used are C. albicans SC5314, C. parapsilosis sensu stricto SZMC 8110, C. orthopsilosis SZMC 1545, and C. metapsilosis SZMC 1548. ∗P < 0.05, when compared with the cytokine level stimulated by other species.
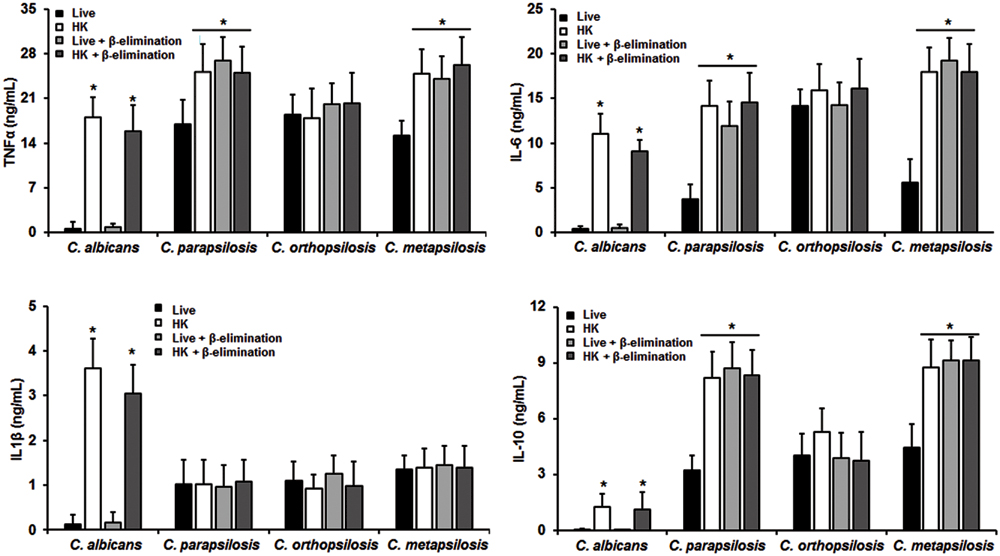
FIGURE 3. Cytokine production by human PBMCs stimulated with either live, heat-killed (HK) or β-eliminated yeast cells. Human PBMCs were co-incubated 24 h with either live, HK, live and β-eliminated, or HK and β-eliminated yeast cells, supernatant collected and used to quantify the cytokine levels. Strains used are C. albicans SC5314, C. parapsilosis sensu stricto SZMC 8110, C. orthopsilosis SZMC 1545, and C. metapsilosis SZMC 1548. ∗P < 0.05, when compared with live cells from the same species.
Role of PRRs in the Sensing of Members of the C. parapsilosis Complex
Next, in order to assess the importance of some PRRs during the fungus-immune cell interaction, human PBMCs were pre-incubated with antagonist of specific PRRs before the interaction with fungal cells. To evaluate the relevance of dectin-1 during this interaction, human PBMCs were pre-incubated with the specific antagonist laminarin (Maneu et al., 2011; Huang et al., 2012; Cohen-Kedar et al., 2014). Results showed in Figure 4 indicate that stimulation of TNFα, IL-1β, IL-6, and IL-10 by HK C. albicans cells was significantly dependent on the engagement of dectin-1 with β1,3-glucan, but not for live cells. When similar experiments were conducted with members of the C. parapsilosis complex, levels of TNFα, IL-6, and IL-10 stimulated with the three species were significantly reduced upon pre-incubation with laminarin, indicating a strong dependence on this receptor for their stimulation (Figure 4). At difference of C. albicans, stimulation of cytokines by live C. parapsilosis sensu lato cells was significantly sensitive to the presence of the dectin-1 antagonist. Interestingly, when human PBMCs were stimulated with C. parapsilosis sensu lato the IL-1β production was insensitive to the dectin-1 blocking, suggesting this receptor does not play a significant role for IL-1β stimulation by members of the C. parapsilosis complex.
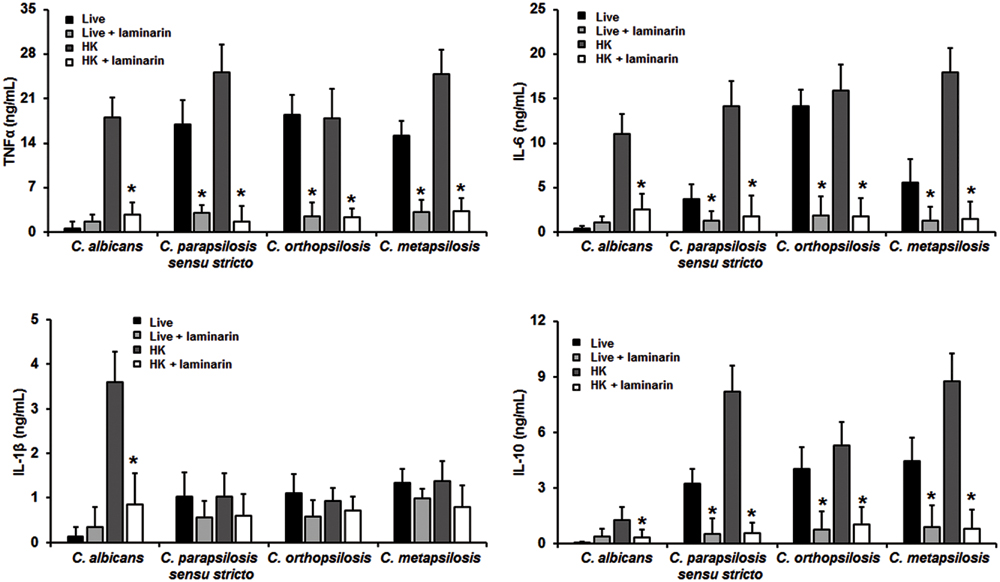
FIGURE 4. Cytokine production by human PBMCs pre-incubated with laminarin. Human PBMCs were pre-incubated 60 min with 200 μg/mL laminarin before challenged for 24 h with either live or HK yeast cells, supernatant were collected and used to quantify the cytokine levels. Strains used are C. albicans SC5314, C. parapsilosis sensu stricto SZMC 8110, C. orthopsilosis SZMC 1545, and C. metapsilosis SZMC 1548. ∗P < 0.05, when compared with untreated cells from the same organism.
To assess the relevance of TLR4 receptor during recognition of C. parapsilosis sensu lato, human PBMCs were pre-incubated with blocking anti-TLR4 antibodies before challenged with yeast cells. Results shown in Figure 5 indicate that blocking of TLR4 has no impact on the cytokine production stimulated by live C. albicans cells. Similar experiments conducted with HK cells did not show significant differences in the levels of cytokine production (not shown). TNFα production stimulated by the three members of the C. parapsilosis complex was significantly reduced when TLR4 was blocked (Figure 5); while IL-6 stimulation was significantly diminished only when human PBMCs were co-incubated with C. orthopsilosis (Figure 5). Experiments conducted in presence of an irrelevant antibody of the same isotype gave no significant differences in the cytokine level (Figure 5). No significant changes where observed when IL-10 and IL-1β were quantified after pre-incubation with the TLR4 blocking antibodies (Figure 5). Therefore, TLR4 is required for TNFα production but not for IL-10 nor IL-1β, when human PBMCs are challenged with C. parapsilosis sensu lato. Furthermore, production of IL-6 was specifically affected when TLR4 engagement was disrupted in cells stimulated with C. orthopsilosis.
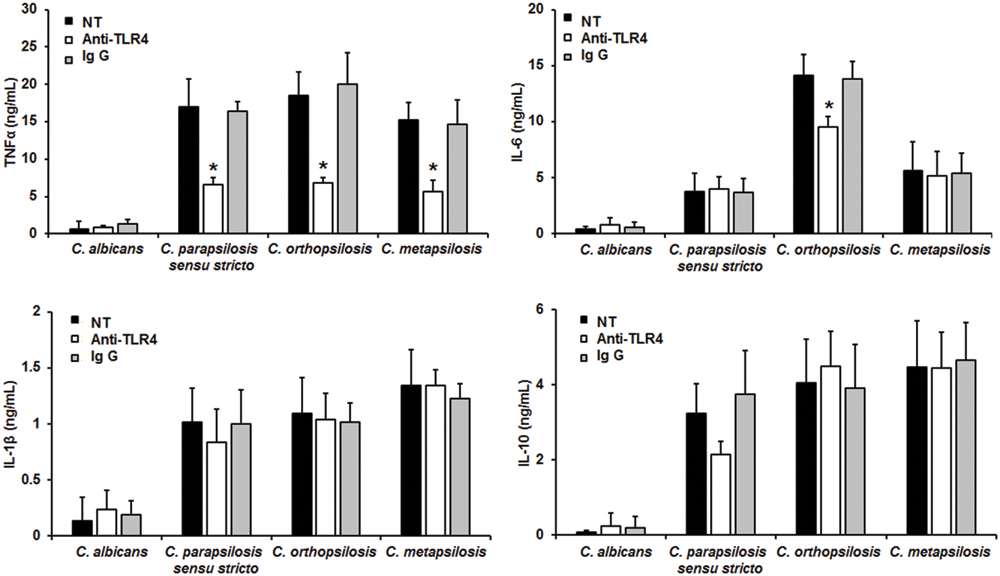
FIGURE 5. Cytokine production by human PBMCs pre-incubated with anti-TLR4 antibodies. Human PBMCs were pre-incubated 60 min with 10 μg/mL either anti-TLR4 antibodies or IgG1 before challenged for 24 h with live cells, supernatant were collected and used to quantify the cytokine levels. Strains used are C. albicans SC5314, C. parapsilosis sensu stricto SZMC 8110, C. orthopsilosis SZMC 1545, and C. metapsilosis SZMC 1548. ∗P < 0.05, when compared with untreated cells from the same organism. NT, non-treated cells.
When the yeast-PBMC interaction was performed with human cells pre-treated with anti-MR antibodies, there were no significant changes in the cytokine production stimulated by C. albicans cells (Figure 6). However, TNFα, and IL-1β stimulation by C. parapsilosis sensu lato was significantly reduced upon MR blocking (Figure 6). IL-6 levels were only significantly reduced in anti-MR-pre-incubated human PBMCs stimulated with either C. orthopsilosis or C. metapsilosis, but not with C. parapsilosis sensu stricto (Figure 6); while blocking of MR only affected IL-10 production in cells stimulated with C. orthopsilosis (Figure 6). Control interactions with an irrelevant antibody did not show changes in the cytokine production stimulated by all tested yeast cells (Figure 6). Taken together, these results indicate the role of dectin-1, TLR4 and MR in the recognition of members of the C. parapsilosis complex and C. albicans is different.
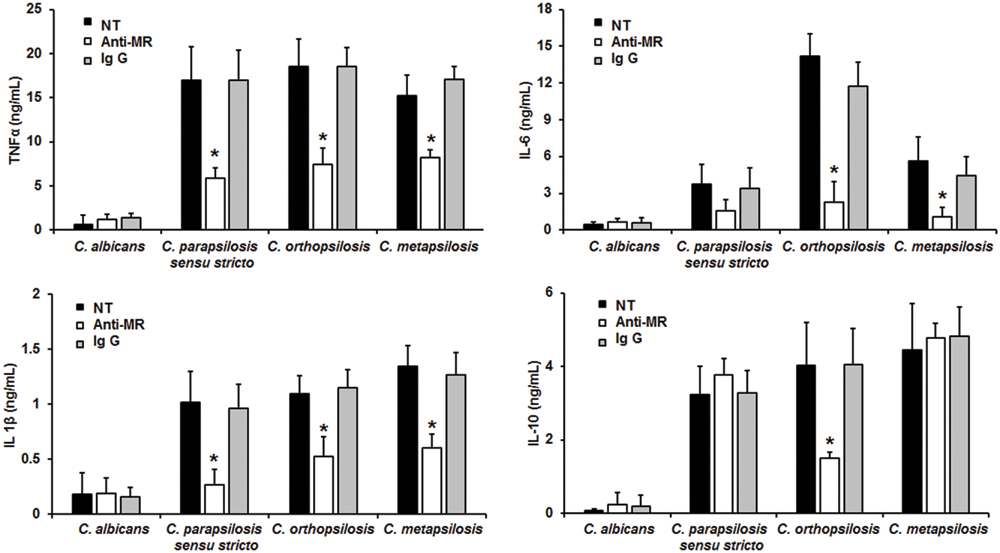
FIGURE 6. Cytokine production by human PBMCs pre-incubated with anti-MR antibodies. Human PBMCs were pre-incubated 60 min with 10 μg/mL either anti-MR antibodies or IgG1 before challenged for 24 h with live cells, supernatant were collected and used to quantify the cytokine levels. Strains used are C. albicans SC5314, C. parapsilosis sensu stricto SZMC 8110, C. orthopsilosis SZMC 1545, and C. metapsilosis SZMC 1548. ∗P < 0.05, when compared with untreated cells from the same organism. NT, non-treated cells.
Purified Mannans from C. albicans and C. parapsilosis sensu lato are Capable to Mimic the Effect of Anti-TLR4 and Anti-MR Antibodies on Human PMBCs
Purified cell wall components from C. albicans have been previously used to block its proper recognition by human PBMCs (Mora-Montes et al., 2011), thus we aimed to isolate either N-linked or O-linked mannan using well-established protocols (Mora-Montes et al., 2012). Purified mannans from any of the three members of the C. parapsilosis complex or C. albicans were negative to fungal, bacteria and protein or other wall polysaccharide contamination, and did not stimulate any cytokine production when used at concentration up to 400 μg/mL (not shown). When human PBMCs were pre-incubated with 200 μg/mL N- or O-linked mannans from C. albicans and then stimulated with C. parapsilosis sensu stricto, TNFα stimulation was significantly reduced, but not IL-6 production (Table 2). Similar results were observed when mannans from either C. parapsilosis sensu lato, C. orthopsilosis or C. metapsilosis were used in the pre-incubation step (Table 2), or when mannans were used to block the proper recognition of C. orthopsilosis or C. metapsilosis (not shown). Therefore, N- and O-linked mannans are able to block the recognition of C. parapsilosis sensu lato as the anti-MR and anti TLR4 antibodies, respectively.
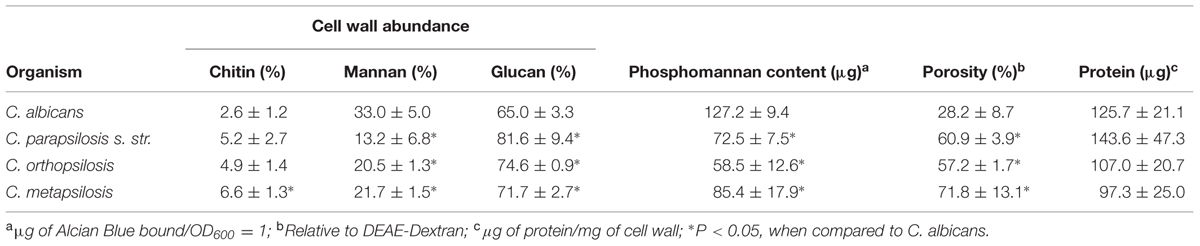
TABLE 2. Blocking of cytokine production by N- and O-linked mannans isolated from either C. albicans or C. parapsilosis sensu lato cell wall.
Discussion
Members of the Candida genus are frequently regarded as similar to C. albicans; however, it is possible to demonstrate they have both genetic and metabolic differences that can affect the interaction with the host (Butler et al., 2009). Here, our comparative analysis of cell wall composition showed that members of the C. parapsilosis complex displayed lower mannan quantity, with similar protein level, when compared to C. albicans cells. In order to explain these data, we hypothesize that the mannans displayed on the wall surface have lower content of mannose per oligosaccharide unit, i.e., they are shorter in C. parapsilosis sensu lato. Indeed, it has been demonstrated C. parapsilosis has shorter N-linked mannans than those from the C. albicans cell wall (Shibata et al., 1995). The presence of short mannans is likely to affect the cell wall porosity, offering higher ability to DEAE-dextran, a bulky polycation, to reach the plasma membrane with the consequent increment in nucleic acid leakage (De Nobel et al., 1990). Moreover, these shorter mannans could be responsible of the increased content of both chitin and β1,3-glucan exposed at the wall surface. Our hypothesis of shorter mannans on the surface of C. parapsilosis sensu lato cells is also supported by the reduced phosphomannan content, which is an indirect measurement of both N- and O-linked mannan length (Bates et al., 2005, 2006; Mora-Montes et al., 2007, 2010; West et al., 2013; Lopes-Bezerra et al., 2015). This lower content of cell wall mannan seems to be naturally compensated by increasing the β-glucan content. This is a well-documented compensatory mechanism driven by the activation of the calcineurin and the protein kinase C signaling pathways, where disruption of the synthesis of one cell wall component usually affects the levels of other components to avoid wall weakness (Bates et al., 2005, 2006; Mora-Montes et al., 2007; Walker et al., 2013). Our results also point out to subtle, but significant differences in the wall organization between members of the C. parapsilosis complex. In C. orthopsilosis all the β1,3-glucan and chitin is exposed in live cells, which suggest its cell wall proteins have even shorter mannans than C. parapsilosis sensu stricto and C. metapsilosis, but with more glycosylation sites occupied per protein. Alternatively, it could also be possible that mannans attached to C. orthopsilosis wall proteins are larger than those on the surface of other members of the complex, occupying less number of sites in the proteins, making the inner wall polysaccharides highly exposed at the cell surface. Nevertheless, further experiments are required to provide a conclusive explanation to these observations.
Results presented here clearly demonstrate that the human PMBCs-C. parapsilosis sensu lato interaction is different to that previously characterized in C. albicans. While yeast cells of C. albicans are a poor stimulus for cytokine production, the three members of the C. parapsilosis complex induced a strong dectin-1-dependent cytokine production. This is in line with the higher cell wall β1,3-glucan content in live C. parapsilosis sensu lato, but not in C. albicans. It is noteworthy to mention that results obtained here with C. albicans cells contrast with those previously reported (Netea et al., 2006), where strong cytokine production was stimulated by yeast cells of this species. This apparent discrepancy relies in the fact that here we used live yeast cells, while in the previous work the main conclusions were based on HK organisms (Netea et al., 2006). When cells were heat killed we observed and increment in the chitin and the β1,3-glucan content, as previously reported (Gantner et al., 2005; Gow et al., 2007; Mora-Montes et al., 2011), and this correlated with a significant increment in cytokine production stimulated by C. albicans, C. parapsilosis sensu stricto and C. metapsilosis, stressing the importance of the dectin-1-β1,3-glucan interaction for a strong cytokine induction. In the case of C. orthopsilosis, we did not notice a significant increment in cytokine production upon heat killing, which support our result indicating most of the β1,3-glucan is naturally exposed on the C. orthopsilosis cell surface. Our results also suggest that O-linked mannans from yeast cells play a major role in the recognition of C. parapsilosis sensu lato than in C. albicans, where they are dispensable and redundant wall elements for cytokine stimulation by human PBMCs (Netea et al., 2006). It has been reported though, that O-linked mannans from C. albicans hypha have a major participation in cytokine stimulation by epithelial cells (Murciano et al., 2011), suggesting that the proposed redundant role during interaction with components of the immune system may be restricted to PBMCs. Here, we have evidence indicating that in the case of C. parapsilosis sensu lato, β-eliminated and HK cells stimulated similar cytokine levels, which suggest that upon removal of O-linked mannans, inner wall components are exposed and available to engage with PRRs, i.e., O-linked mannans have a role hiding inner wall components from immune receptors. Thus far, it has been reported that N-linked mannans mask the recognition of inner wall components and when disrupted, a significant cytokine production can be stimulated (Wheeler and Fink, 2006; Gow et al., 2007). Similar strategies, where a pro-inflammatory wall components is masked to avoid recognition by elements of the immune system, have been reported in Histoplasma capsulatum and Aspergillus fumigatus, where α-glucans and rodlet hydrophobins hide β1,3-glucan, respectively (Rappleye et al., 2007; Aimanianda et al., 2009). Therefore, it is feasible to conceive that in the case of C. parapsilosis sensu lato, both N- and O-linked mannans provide a disguising strategy to avoid recognition of β1,3-glucan by dectin-1. More experiments are required to demonstrate whether this structures are more abundant on the C. parapsilosis sensu lato cell wall or contain more mannose residues than those reported in C. albicans.
In this study we assessed the relevance of dectin-1, TLR4 and MR in the recognition of C. parapsilosis sensu lato. We chose these receptors because they are the main PRRs involved in the C. albicans immune recognition (Netea et al., 2002, 2006, 2015). TLR 4 is thus far the unique receptor for C. albicans O-linked mannans (Netea et al., 2006), and it is likely also participating in the recognition of the same cell wall component on C. parapsilosis sensu lato. The blocking experiments with either antibodies against receptors or laminarin, showed that TNFα stimulation by members of the C. parapsilosis complex, but not with C. albicans cells, depends on the engagement of TLR4, dectin-1 and MR with their ligands. These results contrast with our previous observation with β-eliminated cells, where TNFα levels upon disrupting the O-linked mannan-TLR4 interaction increased. The hypothesis to explain this apparent paradoxical response is that when the TLR4 receptor is blocked with anti-TLR4 antibodies, the O-linked mannans are still present on the surface of the fungal cell wall, hiding β1,3-glucan from the recognition by dectin-1. Interestingly, IL-6 production was only affected when human PBMCs were treated with anti-TLR4 antibodies and then stimulated with C. orthopsilosis, suggesting the O-linked mannans from this organism are different from those present on the surface of C. parapsilosis sensu stricto and C. metapsilosis. IL-10 production was strongly depend on engagement of dectin-1, as previously reported (Reid et al., 2009). It is noteworthy to mention that the IL-1β production by C. parapsilosis sensu lato was partially dependent on MR recognition, but not dectin-1 nor TLR4. This observation contrast with that reported earlier (Toth et al., 2013), where IL-1β stimulation by C. parapsilosis sensu stricto was dectin-1 dependent. Since the C. albicans immune recognition via dectin-1 is fungal strain-specific (Marakalala et al., 2013), it is feasible to conceive that this discrepancy can be explained because the genetic background of the strain used here (SZMC 8110) and in the previous report (GA-1) (Toth et al., 2013). In cells stimulated by C. albicans, IL-1β production is dependent on the activation of dectin-1, TLR2 and MR (van de Veerdonk et al., 2009); thus, different PRRs or downstream signaling components after C. parapsilosis sensu lato recognition could be responsible of this difference.
It has been previously demonstrated that cell wall preparations or non-purified wall components, such as zymosan, are potent inductors of cytokines (Brown et al., 2003; Netea et al., 2006); while purified wall components are unable to stimulated cytokine production (Dennehy et al., 2008; van de Veerdonk et al., 2009), and can block cytokine production when immune cells are stimulated (Maneu et al., 2011; Mora-Montes et al., 2011; Huang et al., 2012; Cohen-Kedar et al., 2014). These observations have been the base to propose the co-stimulatory theory, where a strong cytokine response involves the engagement of not only one but two or more PRRs at once (Netea et al., 2015). Although it is likely mannans from C. albicans and C. parapsilosis sensu lato are different, here it was demonstrated that purified N- and O-linked mannan preparations do not stimulate cytokine production and are capable to block proper Candida recognition, in a similar way that anti-MR and anti-TLR4 antibodies, respectively. Thus, they can be a reliable and alternative tool to assess the contribution of these PRRs during the innate immune recognition of fungal cells.
Overall, our data clearly demonstrate that the current knowledge about C. albicans biology and interaction with the host cannot be extrapolated to other members of the genus. C. parapsilosis sensu lato displays significant differences in the cell wall that impact the recognition by human PBMCs, stimulating stronger cytokine production, via the increased exposure of β1,3-glucan at the cell surface. Finally, our results suggest different contribution of dectin-1, MR and TLR4 in the recognition of the members of the C. parapsilosis complex, which has to be taken in consideration when analyzing the C. parapsilosis sensu lato-host interaction.
Author Contributions
ML, AG, and HM-M conceived the study. EE-M, MN-A, LP-G, EM-M, and KC performed experiments. EE-M, MN-A, LP-G, EM-M, ML, KC, AG, and HM-M analyzed data. HM-M drafted the manuscript. EE-M, MN-A, LP-G, EM-M, ML, KC, AG, and HM-M revised and approved the manuscript.
Conflict of Interest Statement
The authors declare that the research was conducted in the absence of any commercial or financial relationships that could be construed as a potential conflict of interest.
Acknowledgments
This work was supported by Consejo Nacional de Ciencia y Tecnología (ref. CB2011/166860), Universidad de Guanajuato (ref. 0025/11) and Programa de Mejoramiento de Profesorado (ref. UGTO-PTC-261).
References
Aimanianda, V., Bayry, J., Bozza, S., Kniemeyer, O., Perruccio, K., Elluru, S. R., et al. (2009). Surface hydrophobin prevents immune recognition of airborne fungal spores. Nature 460, 1117–1121. doi: 10.1038/nature08264
Bates, S., Hughes, H. B., Munro, C. A., Thomas, W. P., MacCallum, D. M., Bertram, G., et al. (2006). Outer chain N-glycans are required for cell wall integrity and virulence of Candida albicans. J. Biol. Chem. 281, 90–98. doi: 10.1074/jbc.M510360200
Bates, S., MacCallum, D. M., Bertram, G., Munro, C. A., Hughes, H. B., Buurman, E. T., et al. (2005). Candida albicans Pmr1p, a secretory pathway P-type Ca2+/Mn2+-ATPase, is required for glycosylation and virulence. J. Biol. Chem. 280, 23408–23415. doi: 10.1074/jbc.M502162200
Brown, G. D., Denning, D. W., Gow, N. A., Levitz, S. M., Netea, M. G., and White, T. C. (2012). Hidden killers: human fungal infections. Sci. Transl. Med. 4, 165rv13. doi: 10.1126/scitranslmed.3004404
Brown, G. D., Herre, J., Williams, D. L., Willment, J. A., Marshall, A. S., and Gordon, S. (2003). Dectin-1 mediates the biological effects of beta-glucans. J. Exp. Med. 197, 1119–1124. doi: 10.1084/jem.20021890
Butler, G., Rasmussen, M. D., Lin, M. F., Santos, M. A., Sakthikumar, S., Munro, C. A., et al. (2009). Evolution of pathogenicity and sexual reproduction in eight Candida genomes. Nature 459, 657–662. doi: 10.1038/nature08064
Cambi, A., Netea, M. G., Mora-Montes, H. M., Gow, N. A., Hato, S. V., Lowman, D. W., et al. (2008). Dendritic cell interaction with Candida albicans critically depends on N-linked mannan. J. Biol. Chem. 283, 20590–20599. doi: 10.1074/jbc.M709334200
Cohen-Kedar, S., Baram, L., Elad, H., Brazowski, E., Guzner-Gur, H., and Dotan, I. (2014). Human intestinal epithelial cells respond to beta-glucans via Dectin-1 and Syk. Eur. J. Immunol. 44, 3729–3740. doi: 10.1002/eji.201444876
De Nobel, J. G., Klis, F. M., Munnik, T., Priem, J., and Van Den Ende, H. (1990). An assay of relative cell wall porosity in Saccharomyces cerevisiae, Kluyveromyces lactis and Schizosaccharomyces pombe. Yeast 6, 483–490. doi: 10.1002/yea.320060605
Dennehy, K. M., Ferwerda, G., Faro-Trindade, I., Pyz, E., Willment, J. A., Taylor, P. R., et al. (2008). Syk kinase is required for collaborative cytokine production induced through Dectin-1 and Toll-like receptors. Eur. J. Immunol. 38, 500–506. doi: 10.1002/eji.200737741
Diaz-Jimenez, D. F., Mora-Montes, H. M., Hernandez-Cervantes, A., Luna-Arias, J. P., Gow, N. A., and Flores-Carreon, A. (2012). Biochemical characterization of recombinant Candida albicans mannosyltransferases Mnt1, Mnt2 and Mnt5 reveals new functions in O- and N-mannan biosynthesis. Biochem. Biophys. Res. Commun. 419, 77–82. doi: 10.1016/j.bbrc.2012.01.131
Díaz-Jiménez, D., Pérez-García, L., Martínez-Álvarez, J., and Mora-Montes, H. (2012). Role of the fungal cell wall in pathogenesis and antifungal resistance. Curr. Fungal Infect. Rep. 6, 275–282. doi: 10.1007/s12281-012-0109-7
Endres, S., Ghorbani, R., Lonnemann, G., van der Meer, J. W., and Dinarello, C. A. (1988). Measurement of immunoreactive interleukin-1 beta from human mononuclear cells: optimization of recovery, intrasubject consistency, and comparison with interleukin-1 alpha and tumor necrosis factor. Clin. Immunol. Immunopathol. 49, 424–438. doi: 10.1016/0090-1229(88)90130-4
Gago, S., Garcia-Rodas, R., Cuesta, I., Mellado, E., and Alastruey-Izquierdo, A. (2014). Candida parapsilosis, Candida orthopsilosis, and Candida metapsilosis virulence in the non-conventional host Galleria mellonella. Virulence 5, 278–285. doi: 10.4161/viru.26973
Gantner, B. N., Simmons, R. M., and Underhill, D. M. (2005). Dectin-1 mediates macrophage recognition of Candida albicans yeast but not filaments. EMBO J. 24, 1277–1286. doi: 10.1038/sj.emboj.7600594
Gillum, A. M., Tsay, E. Y., and Kirsch, D. R. (1984). Isolation of the Candida albicans gene for orotidine-5′-phosphate decarboxylase by complementation of S. cerevisiae ura3 and E. coli pyrF mutations. Mol. Gen. Genet. 198, 179–182. doi: 10.1007/BF00328721
Gow, N. A. R., Netea, M. G., Munro, C. A., Ferwerda, G., Bates, S., Mora-Montes, H. M., et al. (2007). Immune recognition of Candida albicans beta-glucan by dectin-1. J. Infect. Dis. 196, 1565–1571. doi: 10.1086/523110
Graham, L. M., Tsoni, S. V., Willment, J. A., Williams, D. L., Taylor, P. R., Gordon, S., et al. (2006). Soluble Dectin-1 as a tool to detect beta-glucans. J. Immunol. Methods 314, 164–169. doi: 10.1016/j.jim.2006.05.013
Heinsbroek, S. E., Taylor, P. R., Martinez, F. O., Martinez-Pomares, L., Brown, G. D., and Gordon, S. (2008). Stage-specific sampling by pattern recognition receptors during Candida albicans phagocytosis. PLoS Pathog. 4:e1000218. doi: 10.1371/journal.ppat.1000218
Hobson, R. P., Munro, C. A., Bates, S., MacCallum, D. M., Cutler, J. E., Heinsbroek, S. E., et al. (2004). Loss of cell wall mannosylphosphate in Candida albicans does not influence macrophage recognition. J. Biol. Chem. 279, 39628–39635. doi: 10.1074/jbc.M405003200
Huang, H., Ostroff, G. R., Lee, C. K., Agarwal, S., Ram, S., Rice, P. A., et al. (2012). Relative contributions of dectin-1 and complement to immune responses to particulate beta-glucans. J. Immunol. 189, 312–317. doi: 10.4049/jimmunol.1200603
Linden, J. R., Kunkel, D., Laforce-Nesbitt, S. S., and Bliss, J. M. (2013). The role of galectin-3 in phagocytosis of Candida albicans and Candida parapsilosis by human neutrophils. Cell. Microbiol. 15, 1127–1142. doi: 10.1111/cmi.12103
Lopes-Bezerra, L. M., Lozoya-Perez, N. E., Lopez-Ramirez, L. A., Martinez-Alvarez, J. A., Teixeira, M. M., Felipe, M. S., et al. (2015). Functional characterization of Sporothrix schenckii glycosidases involved in the N-linked glycosylation pathway. Med. Mycol. 53, 60–68. doi: 10.1093/mmy/myu057
Maneu, V., Yanez, A., Murciano, C., Molina, A., Gil, M. L., and Gozalbo, D. (2011). Dectin-1 mediates in vitro phagocytosis of Candida albicans yeast cells by retinal microglia. FEMS Immunol. Med. Microbiol. 63, 148–150. doi: 10.1111/j.1574-695X.2011.00829.x
Marakalala, M. J., Vautier, S., Potrykus, J., Walker, L. A., Shepardson, K. M., Hopke, A., et al. (2013). Differential adaptation of Candida albicans in vivo modulates immune recognition by dectin-1. PLoS Pathog. 9:e1003315. doi: 10.1371/journal.ppat.1003315
McKenzie, C. G., Koser, U., Lewis, L. E., Bain, J. M., Mora-Montes, H. M., Barker, R. N., et al. (2010). Contribution of Candida albicans cell wall components to recognition by and escape from murine macrophages. Infect. Immun. 78, 1650–1658. doi: 10.1128/IAI.00001-10
Miyake, K. (2007). Innate immune sensing of pathogens and danger signals by cell surface Toll-like receptors. Semin. Immunol. 19, 3–10. doi: 10.1016/j.smim.2006.12.002
Mora-Montes, H. M., Bates, S., Netea, M. G., Castillo, L., Brand, A., Buurman, E. T., et al. (2010). A multifunctional mannosyltransferase family in Candida albicans determines cell wall mannan structure and host-fungus interactions. J. Biol. Chem. 285, 12087–12095. doi: 10.1074/jbc.M109.081513
Mora-Montes, H. M., Bates, S., Netea, M. G., Diaz-Jimenez, D. F., Lopez-Romero, E., Zinker, S., et al. (2007). Endoplasmic reticulum alpha-glycosidases of Candida albicans are required for N glycosylation, cell wall integrity, and normal host-fungus interaction. Eukaryot. Cell 6, 2184–2193. doi: 10.1128/EC.00350-07
Mora-Montes, H. M., McKenzie, C., Bain, J. M., Lewis, L. E., Erwig, L. P., and Gow, N. A. (2012). Interactions between macrophages and cell wall oligosaccharides of Candida albicans. Methods Mol. Biol. 845, 247–260. doi: 10.1007/978-1-61779-539-8_16
Mora-Montes, H. M., Netea, M. G., Ferwerda, G., Lenardon, M. D., Brown, G. D., Mistry, A. R., et al. (2011). Recognition and blocking of innate immunity cells by Candida albicans chitin. Infect. Immun. 79, 1961–1970. doi: 10.1128/IAI.01282-10
Murciano, C., Moyes, D. L., Runglall, M., Islam, A., Mille, C., Fradin, C., et al. (2011). Candida albicans cell wall glycosylation may be indirectly required for activation of epithelial cell proinflammatory responses. Infect. Immun. 79, 4902–4911. doi: 10.1128/IAI.05591-11
Nemeth, T., Toth, A., Szenzenstein, J., Horvath, P., Nosanchuk, J. D., Grozer, Z., et al. (2013). Characterization of virulence properties in the C. parapsilosis sensu lato species. PLoS ONE 8:e68704. doi: 10.1371/journal.pone.0068704
Netea, M. G., Gow, N. A., Munro, C. A., Bates, S., Collins, C., Ferwerda, G., et al. (2006). Immune sensing of Candida albicans requires cooperative recognition of mannans and glucans by lectin and Toll-like receptors. J. Clin. Invest. 116, 1642–1650. doi: 10.1172/JCI27114
Netea, M. G., Joosten, L. A., van der Meer, J. W., Kullberg, B. J., and van de Veerdonk, F. L. (2015). Immune defence against Candida fungal infections. Nat. Rev. Immunol. 15, 630–642. doi: 10.1038/nri3897
Netea, M. G., Van Der Graaf, C. A., Vonk, A. G., Verschueren, I., Van Der Meer, J. W., and Kullberg, B. J. (2002). The role of toll-like receptor (TLR) 2 and TLR4 in the host defense against disseminated candidiasis. J. Infect. Dis. 185, 1483–1489. doi: 10.1086/340511
Pammi, M., Holland, L., Butler, G., Gacser, A., and Bliss, J. M. (2013). Candida parapsilosis is a significant neonatal pathogen: a systematic review and meta-analysis. Pediatr. Infect. Dis. J. 32, e206–e216. doi: 10.1097/INF.0b013e3182863a1c
Rappleye, C. A., Eissenberg, L. G., and Goldman, W. E. (2007). Histoplasma capsulatum alpha-(1,3)-glucan blocks innate immune recognition by the beta-glucan receptor. Proc. Natl. Acad. Sci. U.S.A. 104, 1366–1370. doi: 10.1073/pnas.0609848104
Reid, D. M., Gow, N. A. R., and Brown, G. D. (2009). Pattern recognition: recent insights from Dectin-1. Curr. Opin. Immunol. 21, 30–37. doi: 10.1016/j.coi.2009.01.003
Saijo, S., and Iwakura, Y. (2011). Dectin-1 and Dectin-2 in innate immunity against fungi. Int. Immunol. 23, 467–472. doi: 10.1093/intimm/dxr046
Schwartz, S. N., Medoff, G., Kobayashi, G. S., Kwan, C. N., and Schlessinger, D. (1972). Antifungal properties of polymyxin B and its potentiation of tetracycline as an antifungal agent. Antimicrob. Agents Chemother. 2, 36–40. doi: 10.1128/AAC.2.1.36
Shibata, N., Ikuta, K., Imai, T., Satoh, Y., Satoh, R., Suzuki, A., et al. (1995). Existence of branched side chains in the cell wall mannan of pathogenic yeast, Candida albicans. Structure-antigenicity relationship between the cell wall mannans of Candida albicans and Candida parapsilosis. J. Biol. Chem. 270, 1113–1122. doi: 10.1074/jbc.270.3.1113
Spreghini, E., Orlando, F., Tavanti, A., Senesi, S., Giannini, D., Manso, E., et al. (2012). In vitro and in vivo effects of echinocandins against Candida parapsilosis sensu stricto, Candida orthopsilosis and Candida metapsilosis. J. Antimicrob. Chemother. 67, 2195–2202. doi: 10.1093/jac/dks180
Szenzenstein, J., Gacser, A., Grozer, Z., Farkas, Z., Nagy, K., Vagvolgyi, C., et al. (2013). Differential sensitivity of the species of Candida parapsilosis sensu lato complex against statins. Mycopathologia 176, 211–217. doi: 10.1007/s11046-013-9689-1
Tavanti, A., Davidson, A. D., Gow, N. A., Maiden, M. C., and Odds, F. C. (2005). Candida orthopsilosis and Candida metapsilosis spp. nov. to replace Candida parapsilosis groups II and III. J. Clin. Microbiol. 43, 284–292. doi: 10.1128/JCM.43.1.284-292.2005
Toth, A., Csonka, K., Jacobs, C., Vagvolgyi, C., Nosanchuk, J. D., Netea, M. G., et al. (2013). Candida albicans and Candida parapsilosis induce different T-cell responses in human peripheral blood mononuclear cells. J. Infect. Dis. 208, 690–698. doi: 10.1093/infdis/jit188
Trevino-Rangel Rde, J., Gonzalez, J. G., and Gonzalez, G. M. (2013). Aspartyl proteinase, phospholipase, esterase and hemolysin activities of clinical isolates of the Candida parapsilosis species complex. Med. Mycol. 51, 331–335. doi: 10.3109/13693786.2012.712724
Trofa, D., Gacser, A., and Nosanchuk, J. D. (2008). Candida parapsilosis, an emerging fungal pathogen. Clin. Microbiol. Rev. 21, 606–625. doi: 10.1128/CMR.00013-08
van de Veerdonk, F. L., Joosten, L. A., Devesa, I., Mora-Montes, H. M., Kanneganti, T. D., Dinarello, C. A., et al. (2009). Bypassing pathogen-induced inflammasome activation for the regulation of interleukin-1beta production by the fungal pathogen Candida albicans. J. Infect. Dis. 199, 1087–1096. doi: 10.1086/597274
Walker, L. A., Gow, N. A., and Munro, C. A. (2013). Elevated chitin content reduces the susceptibility of Candida species to caspofungin. Antimicrob. Agents Chemother. 57, 146–154. doi: 10.1128/AAC.01486-12
West, L., Lowman, D. W., Mora-Montes, H. M., Grubb, S., Murdoch, C., Thornhill, M. H., et al. (2013). Differential virulence of Candida glabrata glycosylation mutants. J. Biol. Chem. 288, 22006–22018. doi: 10.1074/jbc.M113.478743
Keywords: Candida parapsilosis, Candida albicans host-fungus interaction, cell wall, cytokine, mononuclear cells
Citation: Estrada-Mata E, Navarro-Arias MJ, Pérez-García LA, Mellado-Mojica E, López MG, Csonka K, Gacser A and Mora-Montes HM (2016) Members of the Candida parapsilosis Complex and Candida albicans are Differentially Recognized by Human Peripheral Blood Mononuclear Cells. Front. Microbiol. 6:1527. doi: 10.3389/fmicb.2015.01527
Received: 30 October 2015; Accepted: 18 December 2015;
Published: 13 January 2016.
Edited by:
Marcio L. Rodrigues, Oswaldo Cruz Foundation, BrazilReviewed by:
Claudia Monari, Università degli Studi di Perugia, ItalyAnamelia Lorenzetti Bocca, University of Brasilia, Brazil
Copyright © 2016 Estrada-Mata, Navarro-Arias, Pérez-García, Mellado-Mojica, López, Csonka, Gacser and Mora-Montes. This is an open-access article distributed under the terms of the Creative Commons Attribution License (CC BY). The use, distribution or reproduction in other forums is permitted, provided the original author(s) or licensor are credited and that the original publication in this journal is cited, in accordance with accepted academic practice. No use, distribution or reproduction is permitted which does not comply with these terms.
*Correspondence: Héctor M. Mora-Montes, aG1vcmFAdWd0by5teA==