- 1CNRS UMR 7267, Laboratoire Ecologie et Biologie des Interactions, Université de Poitiers, Poitiers, France
- 2Genetics Department, Texas Biomedical Research Institute, San Antonio, TX, USA
- 3Institut des Sciences de l’Evolution de Montpellier (UMR CNRS-IRD-UM 5554), Université de Montpellier, Montpellier, France
Wolbachia is an intracellular α-proteobacterium which is transmitted vertically from mother to offspring but also frequently switches horizontally from one host to another. Our hypothesis is based on the role of immune cells and the organs that produce them, the hematopoietic organs (HOs), as primordial niches for the propagation of Wolbachia via hemocytes both (i) within hosts: to initiate and maintain the systemic infection and (ii) between hosts: to promote both vertical and horizontal transmission of Wolbachia. Therefore, we review some fundamental ideas underlying this hypothesis and go further with new empirical data that lead to a first close-up analysis of the potential role of HOs in Wolbachia propagation. The monitoring of the first steps of Wolbachia infection in horizontally infected host organs by transmission electron microscopy and qPCR suggests that (i) HOs are colonized early and extensively as soon as they are in contact with Wolbachia which find in these cells a favorable niche to multiply and (ii) infected HOs which expel hemocytes all lifelong can generate and maintain a systemic infection that could contribute to increase both vertical and horizontal propagation of these symbionts.
Introduction
The kind of symbiotic interactions formed between a symbiont and its host depends on the virulence of the first and the resistance of the latter (Combes, 2001). If the symbiont is horizontally transmitted, optimal transmission can be directly linked to virulence as higher multiplication can allow better dispersion. Thus, horizontally transmitted symbionts can maintain high virulence level due to extensive colonization of the host. Contrariwise, vertically transmitted symbionts depend on their host reproduction to transmit. Hence, one could expect an alignment of the interests of both partners that could lead to an attenuated virulence of the symbiont and restriction of symbiont location to gonads. These two extreme situations are observed and documented in nature. However, some symbionts perform both vertical and horizontal transmission in order to optimize their fitness (Ebert, 2013). Such symbiotic systems should present intermediate situation both in terms of colonization and virulence patterns.
Wolbachia pipientis is a non-culturable intracellular α-proteobacteria that colonizes ovaries of their arthropod hosts to be vertically transmitted. To increase vertical transmission, Wolbachia manipulates its hosts’ reproduction by several ways leading to an increase either in the host female ratio or in fitness for infected females compared to uninfected ones (Werren et al., 2008). In addition to the major vertical transmission path, Wolbachia can also quite frequently horizontally pass from one host species to another and adapt to the new host species (Mercot and Poinsot, 2009). Such colonization after horizontal transfer certainly contributes to the large host range of Wolbachia which is considered to date as being the most widespread symbiont in the animal kingdom. The most recent meta-analyses of Wolbachia pandemics revealed that around 50% of terrestrial arthropod species could be infected with this symbiont (Weinert et al., 2015).
The vertical transmission of Wolbachia from mother to offspring is dependent on the symbiont tropism to germ cells (Frydman et al., 2006). However Wolbachia frequently exhibits wide intra-host distributions outside of the gonads: they have been observed in the cells of major organs including the brain, nervous chain, muscles, fat body, Malpighian tubules, salivary glands, gnathosoma, midgut and hemolymph in insects, mites and crustaceans (reviewed in Sicard et al., 2014). This intensive extra-gonadal colonization leads to a systemic infection which might be necessary for Wolbachia to develop their diverse host manipulations and to spread within host population by other routes than vertical transmission (reviewed in Sicard et al., 2014).
Many terrestrial isopods species were found infected with Wolbachia (Bouchon et al., 2008). Phylogenies of both symbionts and hosts appeared incongruent suggesting that Wolbachia can transfer horizontally between and within species (Cordaux et al., 2001, 2012; Zimmermann et al., 2015). Such horizontal transfers are readily performed experimentally: (i) by injection of ovary suspension (Rigaud et al., 1991; Juchault et al., 1994; Le Clec’h et al., 2012, 2013, 2014), (ii) by transplantation of infected tissues (Juchault et al., 1974) (iii) via hemolymph (injection or hemolymph contact between injured isopods) (Rigaud and Juchault, 1995; Le Clec’h et al., 2014) and (iv) cannibalism (Le Clec’h et al., 2013). Both hemolymphatic contact and cannibalism were closely investigated as potential ecological paths for horizontal transfers between terrestrial isopods (Rigaud and Juchault, 1995; Le Clec’h et al., 2013, 2014). Cannibalism experiments demonstrated that Wolbachia was able to pass the intestinal wall and contaminate organs of the predator (Le Clec’h et al., 2013). However, predator exhibited only really low infection loads and the infection was eventually lost for many of them (Le Clec’h et al., 2013). Contrariwise, hemolymph contact led to the systemic infection of all recipient animals which exhibited Wolbachia loads similar to vertically infected individuals demonstrating that this path leads to successful horizontal transfers (Rigaud and Juchault, 1995; Le Clec’h et al., 2014).
After introduction of Wolbachia in a naïve host by injection of hemolymph or ovary suspension many somatic cells including the hemocytes (i.e., the immune cells) of recipient hosts were extensively infected with Wolbachia as observed in naturally infected animals (Braquart-Varnier et al., 2008; Chevalier et al., 2011; Le Clec’h et al., 2014). This observation raises the question of the selective advantage, for Wolbachia, of colonizing somatic cells. In Armadillidium vulgare, Genty et al. (2014) have hypothesized that many oocytes would be infected secondarily by Wolbachia coming from somatic cell sources during isopod life. Indeed, an optimal vertical transmission would not be dependent only on germ line primary tropism but also on the harvesting of some Wolbachia cells coming from somatic tissues external to the gonads (Faria and Sucena, 2013; Genty et al., 2014). It is thus possible that Wolbachia vertical propagation may rely on distant reservoir requiring mobile vectors, such as hemocytes, to shuttle Wolbachia from a source organ to a sink one (i.e., the ovaries) (Rigaud et al., 1991; Rigaud and Juchault, 1995; Chevalier et al., 2011). Hemocytes are good candidates for Wolbachia propagation both (i) between organs of an individual and (ii) between individuals during horizontal transfer via hemolymph contact. In both terrestrial isopods and insects such as anopheles, Wolbachia were spotted in the hemocytes suggesting that the utilization of these cells as “cellular taxis” could be quite common in the arthropod world (Chevalier et al., 2011; Hughes et al., 2011). However, the presence of alive and infectious Wolbachia is only demonstrated in the terrestrial isopods. Indeed, the comparison of Wolbachia colonization dynamics in terrestrial isopods after the injection of a similar dose of Wolbachia from ovary suspension or hemolymph revealed that the latter allowed a quicker colonization of somatic and reproductive organs (Le Clec’h et al., 2014). Such ability of hemocytes to act as “cellular taxis” could be linked to the fact that 40% of them are colonized by around seven Wolbachia on average (Chevalier et al., 2011). As the hemocyte density in hemolymph is around 20,000 cells/μL, in the 30 μL of hemolymph contained in an adult, at least 1.106 Wolbachia are expected to circulate permanently in the whole host body.
Colonization of immune cells could constitute a primordial step for the propagation of Wolbachia both (i) within hosts, to initiate and maintain the systemic infection, and (ii) between hosts, to promote both vertical and horizontal transmission of Wolbachia. Addressing the question of the production and renewing of infected immune cells during host life contributes to the understanding of Wolbachia-host interactions at both organismal and ecological scales. We present in this paper empirical elements that led to a first close-up analysis of the potential role of Hematopoietic Organs (HOs), involved in the hemocyte production, in Wolbachia propagation. To investigate the first step of host colonization after introduction of contaminated hemocytes, we performed injection of hemolymph and HOs transplantations from animals infected with Wolbachia to naive ones. The monitoring of the Wolbachia infection in host recipient organs by transmission electron microscopy (TEM) and qPCR suggests that (i) HOs are colonized early and extensively as soon as they are in contact with Wolbachia which find in these cells a ‘favorable niche’ to multiply and (ii) infected HOs expelling hemocytes all lifelong can then generate and maintain a systemic infection contributing to increase both vertical and horizontal propagation of these symbionts.
Materials and Methods
Animals
Armadillidium vulgare individuals used in these experiments were either infected with a feminizing Wolbachia strain (wVulC) (i.e., symbiotic) [lines originating from Helsingör (Denmark) or Celles-sur-Belle (France) (Rigaud et al., 1991; Cordaux et al., 2004)] or uninfected (i.e., asymbiotic) animals [lines originating from Helsingör (Denmark) or Nice (France) (Bouchon et al., 1998; Sicard et al., 2010)]. As A. vulgare males are not usually infected with Wolbachia (due to the feminizing nature of these Wolbachia), only females were used in this study. All animal lines were reared at 20°C under natural photoperiod with food provided ad libitum.
Introduction of Wolbachia by Hemolymph Injection
Cuticles of all animals (donors and recipients) used in these experiments were disinfected by immersing individuals for 30 s in a 10% sodium hypochlorite solution followed by a 30 s immersion in distilled water to avoid bacterial contamination from the cuticle. Transinfection was performed by injecting hemolymph, carrying hemocytes infected with Wolbachia (∼104 Wolbachia/μL). To collect hemolymph, the cuticle of each of symbiotic A. vulgare females used as donors was pierced dorsally between the sixth and the seventh pereion segments using a needle and 10 μL of hemolymph from 5 infected females were collected with a micropipette and pooled in a microtube kept on ice, in order to limit coagulation of hemocytes. Then, one microliter of hemolymph was injected into the general cavity of recipient asymbiotic individuals with help of a Hamilton syringe after having pierced laterally the sixth pereion segment.
Introduction of Wolbachia by HOs Transplantation
The HOs which are localized between the sixth and seventh pereion segment and the first telson segment, along the dorsal vessel, were dissected from animals (either infected with Wolbachia or not). Then, they were sucked up with a thin glass Pasteur pipette and grafted in the recipient host (either infected with Wolbachia or not) for which a small square of 2 mm × 2 mm of cuticle was cut off to allow the introduction of the Pasteur pipette containing the graft.
Wolbachia Quantification in Recipient Hosts
After dissections, total DNA was extracted from the different organs of each recipient individual as described by Kocher et al. (1989). For each sample, the concentration and quality (ratios OD 260/280 nm and 260/230 nm) of the extracted DNA were measured using the Nanodrop 1000 spectrophotometer (Thermo). The quantification of Wolbachia was performed by qPCR on DNA samples from ovaries, nervous chain, hemocyte and HO for each treatment collected 15 days after transinfection (either by hemolymph injection or HO transplantation). Wolbachia quantification was also performed for all inocula in order to measure the injection dose. All qPCR reactions were performed using a LightCycler 480 system (Roche) as described in Le Clec’h et al. (2012).
Transmission Electron Microscopy
To analyze the colonization of HOs and other organs by Wolbachia using TEM, tissues were fixed (9% glutaraldehyde, 0.3M sodium cacodylate, 3% NaCl, v/v/v) for 2 h at 4°C then washed (0.3M sodium cacodylate, 3% NaCl, 0.8M sucrose, v/v/v) for 2 h at 4°C. Post-fixation was performed in 4% OsO4, 0.3M sodium cacodylate, 5.5% NaCl for 45 min at room temperature. Tissues were subsequently dehydrated though a graded series of acetone solutions, infiltrated and embedded in resin (Spurr, Polyscience Inc.). First sections (0.5 μm) were stained with 1% toluidin blue for light microscopy observation. Thinner sections (90 nm) were contrasted by incubation in 1% uranyl acetate in 50% ethanol for 1 min, and then stained with lead citrate. Sections were observed using a transmission electron microscope (JEM 1010).
Results
The Trafficking of Hemocytes Into Several Organs
In several A. vulgare’s organs, notably in nervous chain and in ovaries, we observed in TEM some hemocytes trafficking directly in the organs. These observations reveal a direct contact between hemocytes and surrounding cells which can promote the passage of Wolbachia from one cell type to another (Figure 1).
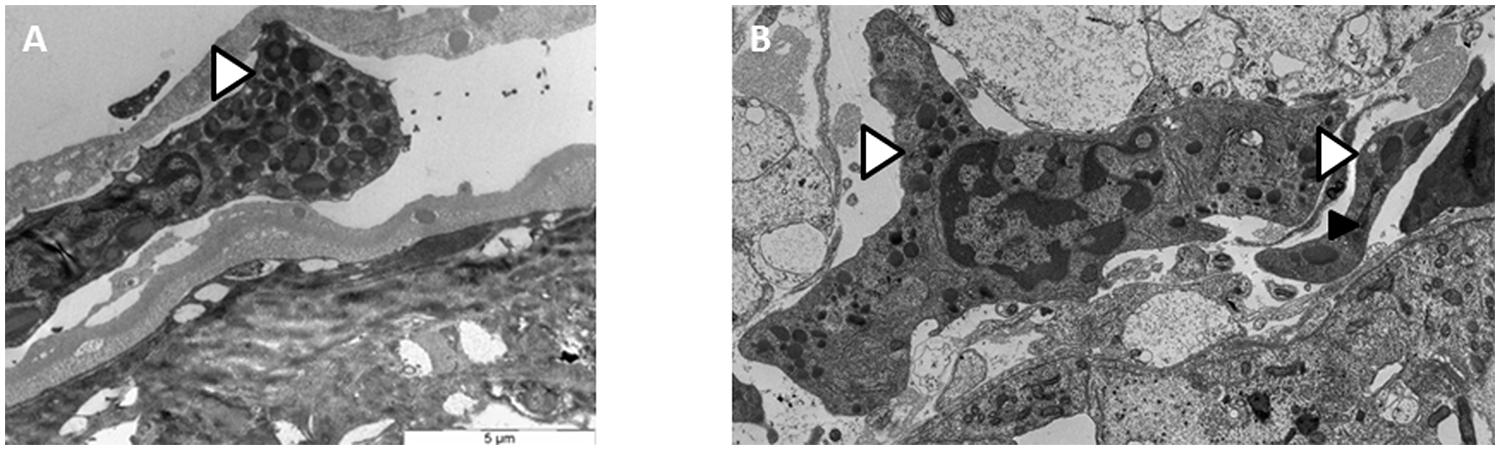
FIGURE 1. Transmission electron microscopy (TEM) observations of hemocytes trafficking in the ovary (A) and in the nervous chain (B). Wolbachia
Hemocytes trafficking.
HOs of Recipient Hosts are Highly Colonized After Horizontal Transfers Via Hemolymph Injection or HOs Transplantation
The quantification of Wolbachia by qPCR in 12 asymbiotic recipient animals 15 days after their transinfection with hemolymph from symbiotic animals revealed that all tissues collected (ovaries, nervous chain, hemocytes and HOs) in the recipient animals were infected with Wolbachia (Figure 2A). The HOs were overall not significantly more colonized than other organs (Kruskal-Wallis test Non-significant). However, the highest densities of Wolbachia were registered in HOs (some individuals showed more than 2000 Wolbachia/ng DNA in HOs while other tissues never exhibit more than 200 Wolbachia/ng DNA in any recipient individuals).
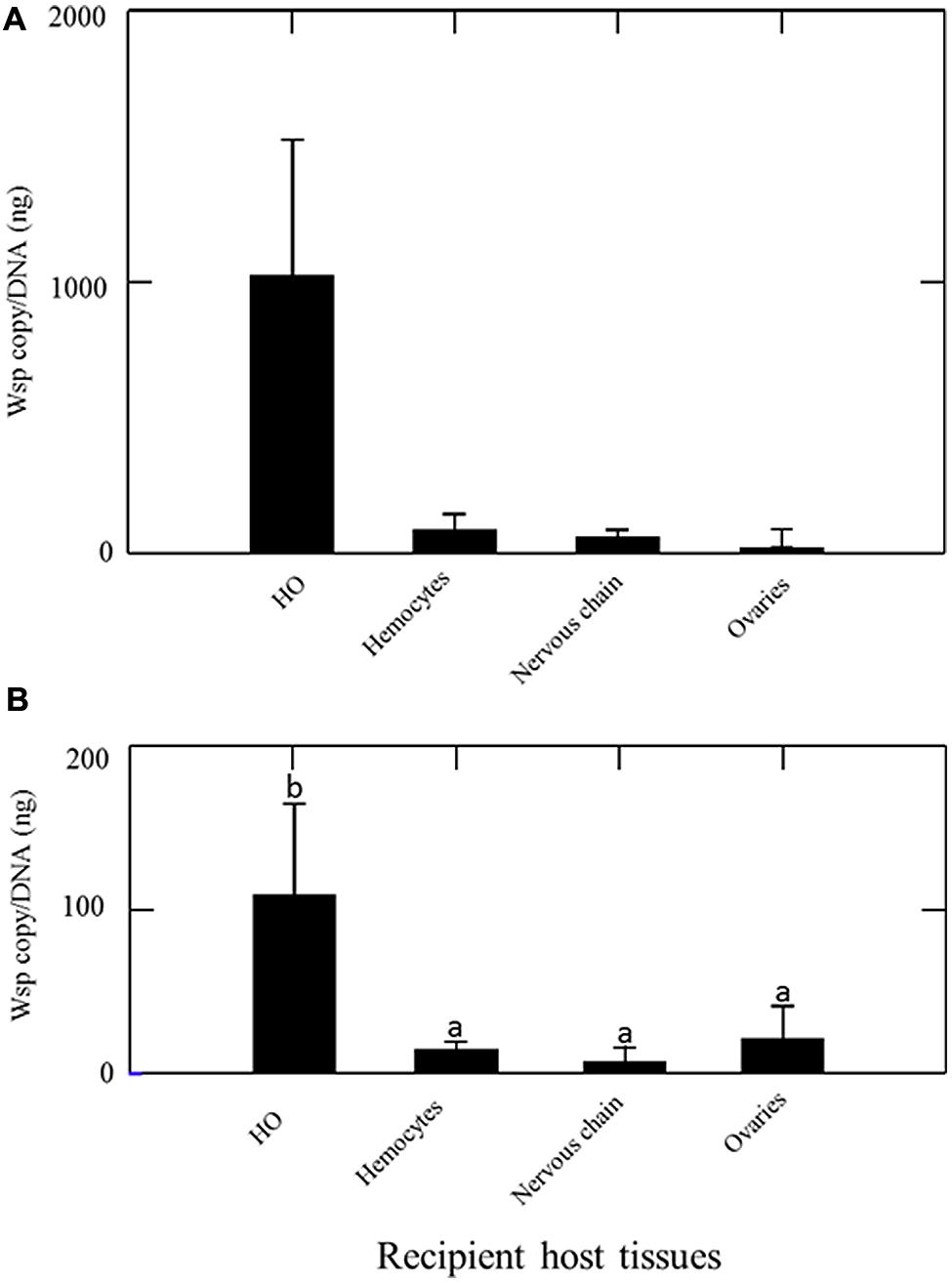
FIGURE 2. Quantification of Wolbachia [wsp copy/DNA (ng)] in asymbiotic recipient animals 15 days after their transinfection (A) by injection of hemolymph from symbiotic animals (B) by transplantation of HOs from symbiotic animals. Different letters indicate significant differences.
The quantification of Wolbachia in nine asymbiotic recipient animals 15 days after they received a HOs transplantation from symbiotic animals revealed that all tissues collected (ovaries, nervous chain, hemocytes, HOs) in the recipient animals were infected with Wolbachia (Figure 2B). The infection patterns observed is close to the one observed after transinfection with hemolymph (Figure 2A). However, in this experiment Wolbachia density was significantly higher in the HOs of recipient animals than in any other organ (Kruskal–Wallis: 14,620; P = 0.002).
HOs: A Privileged Environment for Wolbachia Multiplication?
To monitor the behavior of Wolbachia in HOs’ cells, HOs coming from 16 naive animals were transplanted to 16 animals infected with Wolbachia. The grafted organs were resampled in recipient hosts 15, 30, and 60 days after the transplantation and observed in TEM (Figures 3, 4, and 5). The transplantation of HOs was used here as an experimental procedure to assess the relevance of this organ as a reservoir for Wolbachia. As in all transplantation experiments, there are some limitations in the interpretation as the grafted HOs might have lost their normal function, and could be detected and attacked by the immune response of the recipient. However, we did not observed immune reaction such as encapsulation toward grafted HOs. Starting from 15 days after transplantation, Wolbachia were only observed in adipocytes surrounding the HOs but not in the HO itself (Figure 3A). Only qPCR allowed the detection of Wolbachia at this stage within HOs cells. However, histological observation of HOs showed that the organization of these organs coming from asymbiotic animals changed when they were introduced in symbiotic animals: the compactness of cells is reduced. Such pattern of reduced compactness was also observed in HOs of symbiotic animals. We hypothesize a putative link between colonization by Wolbachia and loss of compactness in HOs (Figures 3B,C; Chevalier et al., 2011). Then, 30 days after transplantation more cells of the grafted HOs were colonized by Wolbachia (Figure 4A). The colonized cells were localized at the surface of the HOs. Sixty days after transplantation, all the cells of the transplanted HOs from central area to the surface were infected with several Wolbachia cells per host cell (Figures 4B,C). We observed Wolbachia cells dividing in the same vacuole suggesting a high rate of multiplication in HOs (Figures 5A,B). Interestingly, some Wolbachia cells appeared to be localized extracellularly between the cells of the HOs suggesting that they could pass from one cell to another in an extracellular manner (Figure 5C). These TEM observations showed that naive HOs when transplanted in a symbiotic environment were progressively colonized by Wolbachia. The hemocytes produced by the HOs are thus infected with Wolbachia (at least 40% of them in Chevalier et al., 2011) and can propagate the infection in the whole organism.
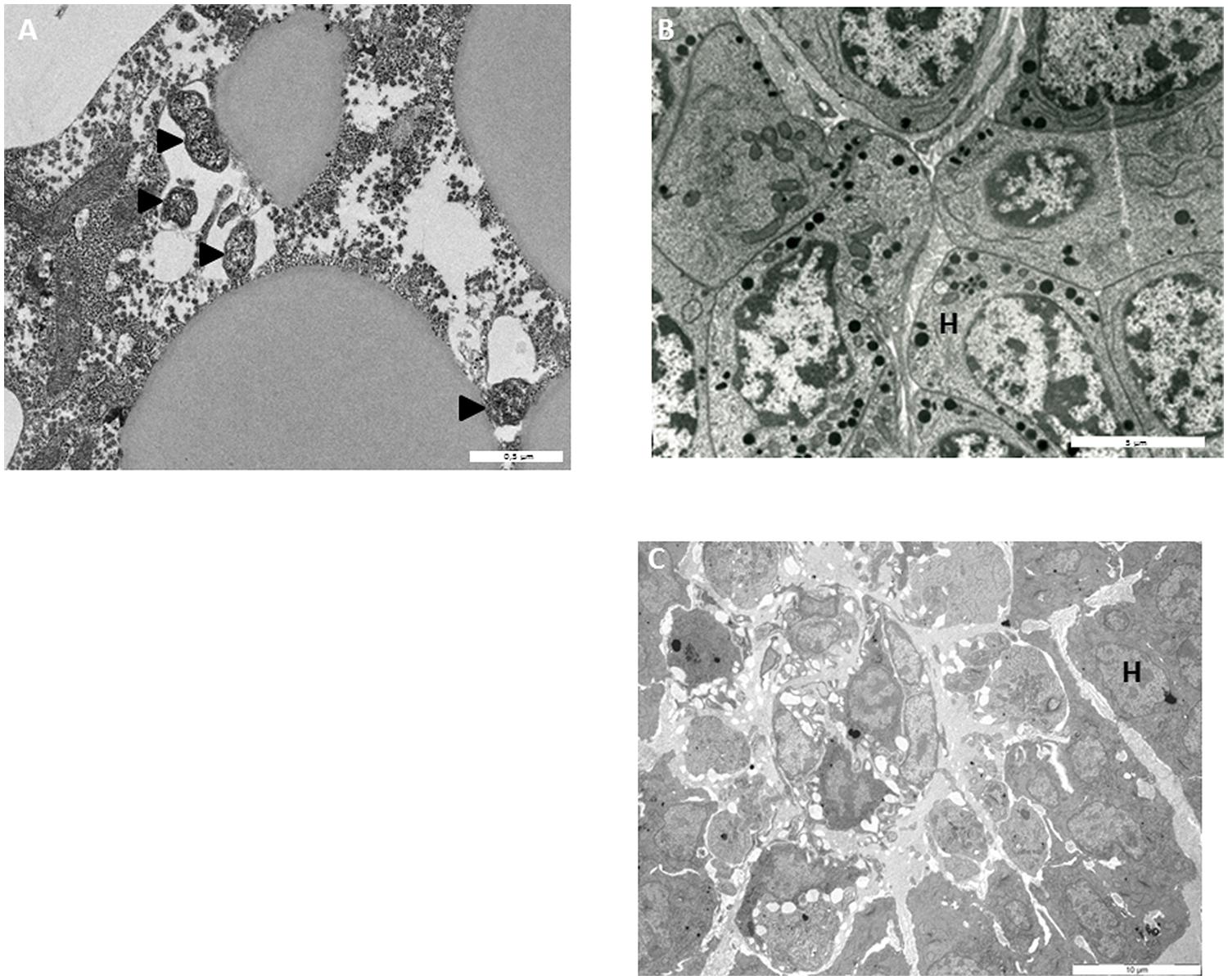
FIGURE 3. Transmission electron microscopy observations (A) Wolbachia in adipocyte surrounding grafted HOs 15 days after transplantation; (B) HO from asymbiotic animals (C) asymbiotic HO grafted in symbiotic environment (15 days after transplantation). H, hemocytes Wolbachia.
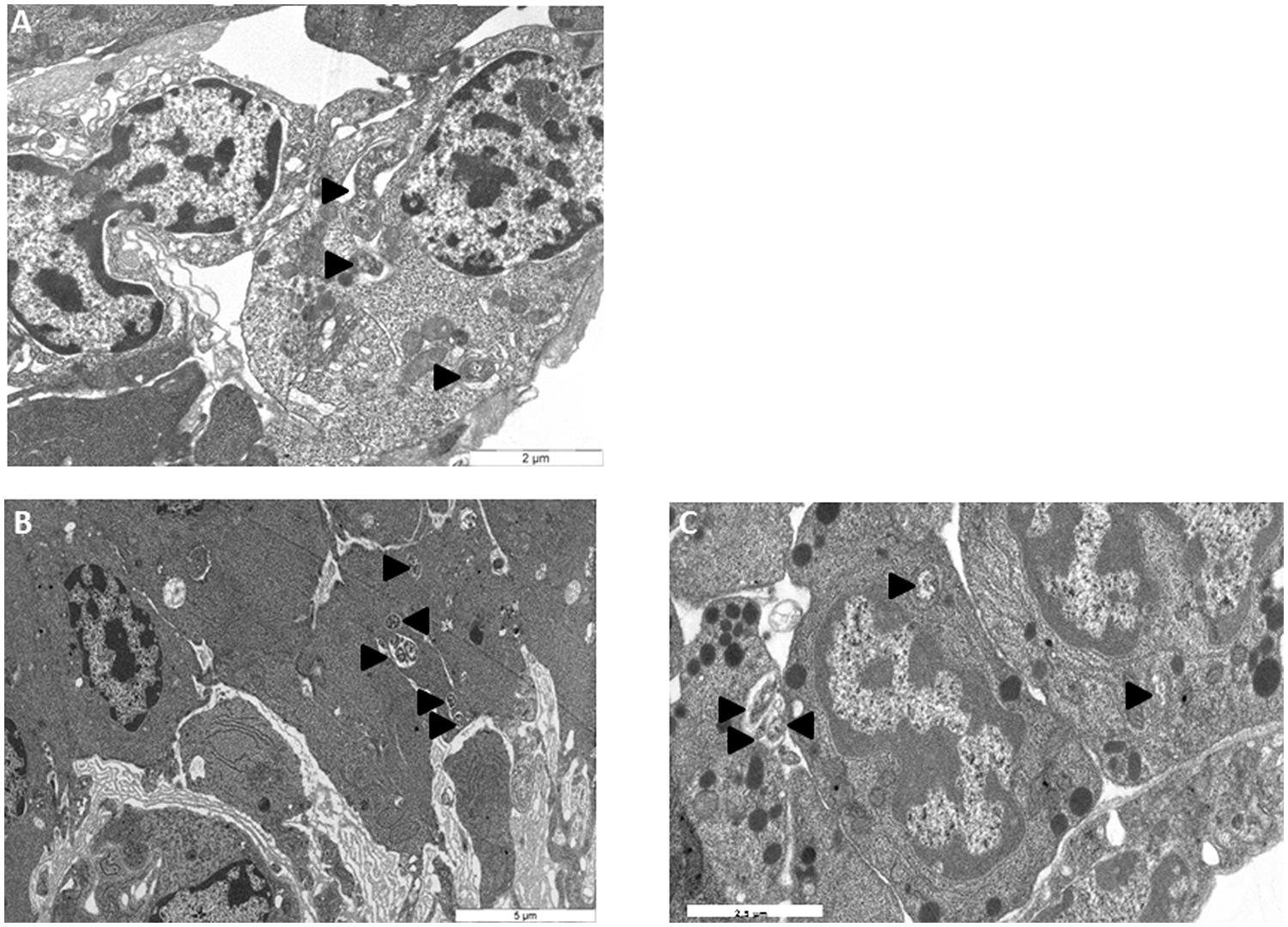
FIGURE 4. Transmission electron microscopy observations of asymbiotic HO grafted in symbiotic animals (A) 30 days post transplantation (B,C) 60 days post transplantation. H, hemocytes Wolbachia.
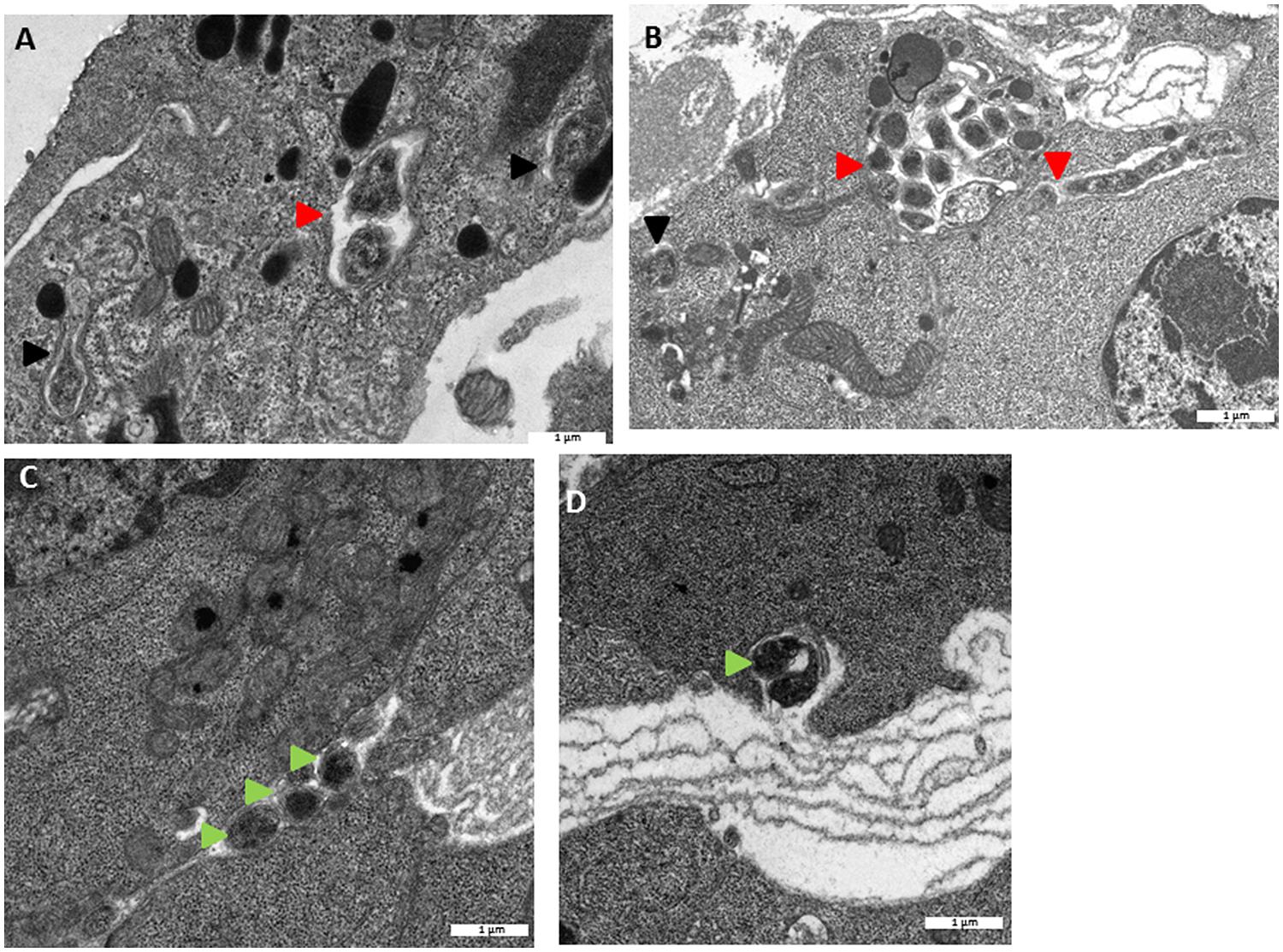
FIGURE 5. Transmission electron microscopy observations of Wolbachia intracellularly (A,B) and extracellularly (C,D) in originally asymbiotic HOs 60 days post transplantation. Wolbachia
Wolbachia dividing,
Extracellular Wolbachia.
Discussion
Wolbachia is responsible for the most widespread pandemics in the animal kingdom (LePage and Bordenstein, 2013). This secondary facultative symbiont manipulates its hosts reproduction to promote its vertical propagation (Werren et al., 2008). However, for several years, it has been demonstrated that Wolbachia, additionally to vertical transmission, can also pass horizontally from one host to another (Sicard et al., 2014). The vision of Wolbachia being not only a reproductive parasite has been raised since its impact on many physiological aspects outside reproduction have been revealed (Saridaki and Bourtzis, 2010; Sicard et al., 2014). Previous studies on Wolbachia-terrestrial isopod interactions demonstrated that the presence of Wolbachia influences the immune phenotype by changing many immune parameters including PO activity, hemocyte density and phagocytosis (Braquart-Varnier et al., 2008; Sicard et al., 2010; Chevalier et al., 2011; Pigeault et al., 2014). Moreover, it has also been demonstrated that the immune cells are infected with Wolbachia which (i) live in a vacuole delimitated by several membranes (Braquart-Varnier et al., 2008; Chevalier et al., 2011), (ii) are metabolically active (Chevalier et al., 2011), and (iii) are able to infect new hosts after blood contact (Rigaud and Juchault, 1995; Le Clec’h et al., 2014). For intracellular bacteria, to live inside the cells, is a good way to be protected from both cellular and humoral immune effectors. By living in the immune cells, Wolbachia, would not only be preserved from the immune system, but would also benefit from this location to spread. Such ability to colonize actively and survive in immune cells is similar to what was described for Anaplasma, a vertebrate pathogen phylogenetically close to Wolbachia (Rikihisa, 2010; Pruneau et al., 2014).
Our hypothesis is that the localization of Wolbachia in hemocytes would be an adaptive feature. Indeed, this colonization can contribute to the maintenance of a systemic infection that could increase vertical transmission of Wolbachia by chronic ovary infections (Figure 6). In the context of horizontal transmission, the presence of Wolbachia in hemocytes constitutes an adaptation for horizontal transfers to new hosts via hemolymph contact, a path that should frequently occur in natura due to the gregarious behavior of terrestrial isopods and the high frequency of wounded animals (Rigaud and Juchault, 1995; Hornung, 2011). To be able to reach the hemocytes that act as ‘taxis’ for transfer within and among individuals, we suggest that Wolbachia could target the hemocyte sources: the HOs. Our empirical data showed that HOs tend to be more colonized than other organs in only a short time after Wolbachia introduction.
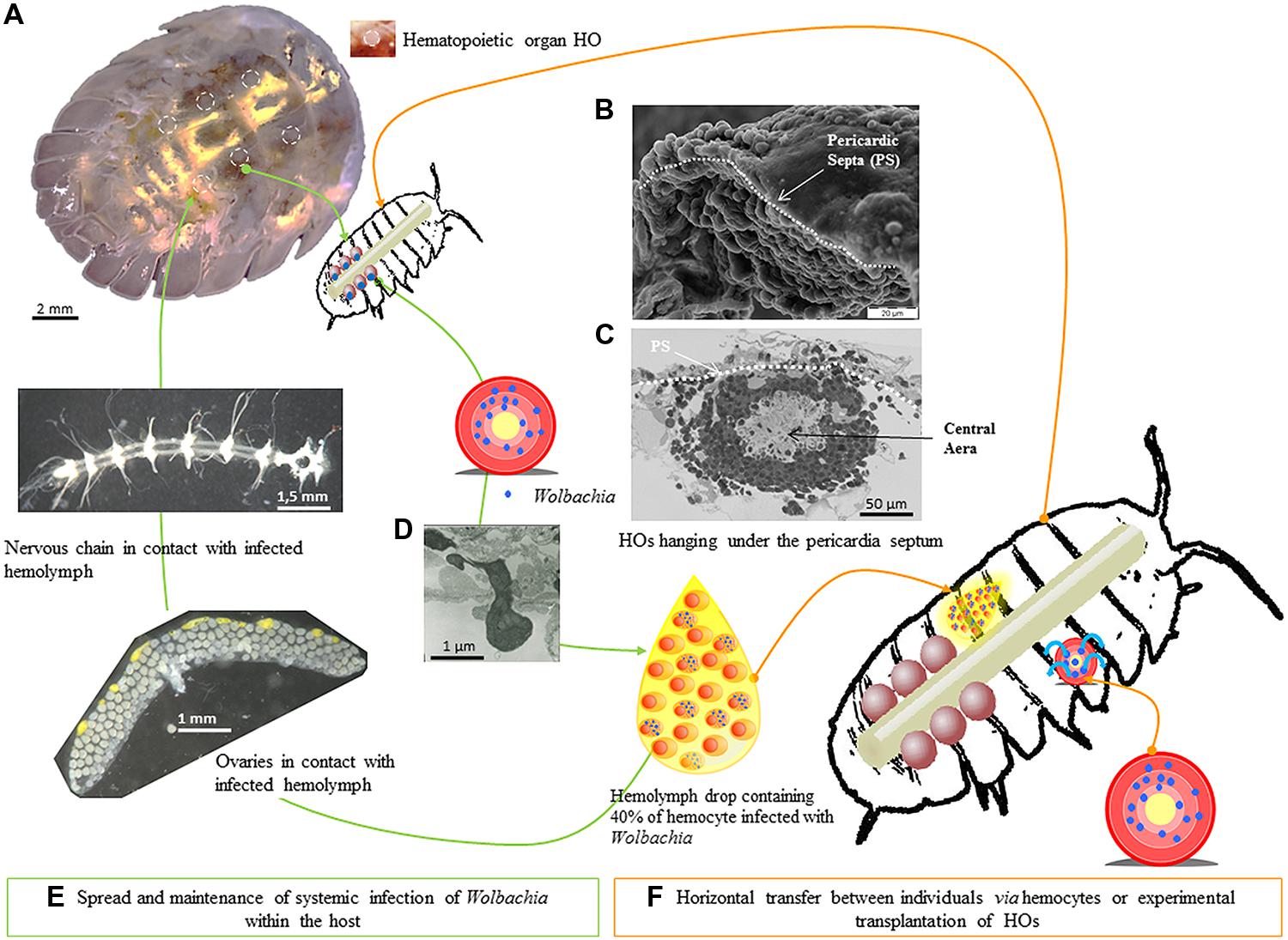
FIGURE 6. Hematopoietic organs as cornerstone for Wolbachia propagation. The six hematopoietic organs are localized between the sixth and seventh pereion segments and the first telson segment (A), along the dorsal vessel and hanging under the pericardia septum (B,C). The six HOs were 150 μm long, 150 μm large and 50–60 μm thick (C). Each HO contained only hemocytes presenting different maturation stages, the least mature being in the central area (C). The HOs discharge hemocytes in the hemolymph probably by diapedesis (D). In terrestrial isopod, hemolymph is a vital biological fluid notably implied in the transportation of oxygen and the circulation of immune cells in the general cavity (E). Transmission of Wolbachia can also happen when a hemolymph contact occurs between two wounded animals (F).
Conclusion
As the hemolymph is circulating throughout the isopod body due to the regular contraction of the dorsal vessel, all the isopod organs hosted in the general cavity are permanently in contact with hemocytes (Figure 6). As hemocytes are able to traffic between the organ cells such as ovaries, nervous chain, etc., the Wolbachia infecting the hemocytes can contaminate cells in many organs including HOs. In this hypothesis, HOs which produce hemocytes all lifelong (up to 3 years for A. vulgare) are the cornerstone for the maintenance and propagation of Wolbachia between and within terrestrial isopods (Figure 6).
Funding
This work was supported by the Ministère de l’Enseignement Supérieur et de la Recherche and the Agence Nationale de la Recherche (ADaWOL ANR-09-JCJC-0109-01 coordinated by Mathieu Sicard and ImmunSymbArt ANR-10-BLAN-1701 coordinated by Didier Bouchon).
Conflict of Interest Statement
The authors declare that the research was conducted in the absence of any commercial or financial relationships that could be construed as a potential conflict of interest.
Acknowledgments
We thank Carine Delaunay, Catherine Debenest for their technical supports and ImageUP, the microscopy platform of the University of Poitiers.
References
Bouchon, D., Cordaux, R., and Grève, P. (2008). “Feminizing Wolbachia and the evolution of sex determination in isopods,” in Insect Symbiosis, eds K. Bourtzis and T. A. Miller (Boca Raton, FL: Taylor and Francis Group), 276–294.
Bouchon, D., Rigaud, T., and Juchault, P. (1998). Evidence for widespread Wolbachia infection in isopod crustaceans: molecular identification and host feminization. Proc. Biol. Sci. 265, 1081–1090. doi: 10.1098/rspb.1998.0402
Braquart-Varnier, C., Lachat, M., Herbiniere, J., Johnson, M., Caubet, Y., Bouchon, D., et al. (2008). Wolbachia mediate variation of host immunocompetence. PLoS ONE 3:e3286. doi: 10.1371/journal.pone.0003286
Chevalier, F., Herbiniere-Gaboreau, J., Bertaux, J., Raimond, M., Morel, F., Bouchon, D., et al. (2011). The immune cellular effectors of terrestrial isopod Armadillidium vulgare: meeting with their invaders, Wolbachia. PLoS ONE 6:e18531. doi: 10.1371/journal.pone.0018531
Combes, C. (2001). Parasitism: The Ecology and Evolution of the Intimate Interactions. Paris: Masson.
Cordaux, R., Michel-Salzat, A., and Bouchon, D. (2001). Wolbachia infection in crustaceans: novel hosts and potential routes for horizontal transmission. J. Evol. Biol. 14, 237–243. doi: 10.1046/j.1420-9101.2001.00279.x
Cordaux, R., Michel-Salzat, A., Frelon-Raimond, M., Rigaud, T., and Bouchon, D. (2004). Evidence for a new feminizing Wolbachia strain in the isopod Armadillidium vulgare: evolutionary implications. Heredity 93, 78–84. doi: 10.1038/sj.hdy.6800482
Cordaux, R., Pichon, S., Hatira, H. B., Doublet, V., Greve, P., Marcade, I., et al. (2012). Widespread Wolbachia infection in terrestrial isopods and other crustaceans. ZooKeys 176, 123–131. doi: 10.3897/zookeys.176.2284
Ebert, D. (2013). The epidemiology and evolution of symbionts with mixed-mode transmission. Annu. Rev. Ecol. Syst. 44, 623–643. doi: 10.1146/annurev-ecolsys-032513-100555
Faria, V. G., and Sucena, E. (2013). Wolbachia in the Malpighian tubules: evolutionary dead-end or adaptation? J. Exp. Zool. B Mol. Dev. Evol. 320, 195–199. doi: 10.1002/jez.b.22498
Frydman, H. M., Li, J. M., Robson, D. N., and Wieschaus, E. (2006). Somatic stem cell niche tropism in Wolbachia. Nature 441, 509–512. doi: 10.1038/nature04756
Genty, L. M., Bouchon, D., Raimond, M., and Bertaux, J. (2014). Wolbachia infect ovaries in the course of their maturation: last minute passengers and priority travellers? PLoS ONE 9:e94577. doi: 10.1371/journal.pone.0094577
Hornung, E. (2011). Evolutionary adaptation of oniscidean isopods to terrestrial life: structure, physiology and behavior. Terr. Arthropod Rev. 4, 95–130. doi: 10.1163/187498311X576262
Hughes, G. L., Koga, R., Xue, P., Fukatsu, T., and Rasgon, J. L. (2011). Wolbachia infections are virulent and inhibit the human malaria parasite Plasmodium falciparum in Anopheles gambiae. PLoS Pathog. 7:e1002043. doi: 10.1371/journal.ppat.1002043
Juchault, P., Bouchon, D., and Rigaud, T. (1994). New evidence for feminizing bacteria in terrestrial isopods: evolutionary implications. C. R. Acad. Sci. Paris III 317, 225–230.
Juchault, P., Legrand, J., and Martin, G. (1974). Action interspécifique du facteur épigénétique féminisant responsable de la thélygénie et de l’intersexualité du crustacé Armadillidium vulgare (Isopode oniscoïde). Ann. Embr. Morph. 7, 265–276.
Kocher, T. D., Thomas, W. K., Meyer, A., Edwards, S. V., Paabo, S., Villablanca, F. X., et al. (1989). Dynamics of mitochondrial-DNA evolution in animals – amplification and sequencing with conserved primers. Proc. Natl. Acad. Sci. U.S.A. 86, 6196–6200. doi: 10.1073/pnas.86.16.6196
Le Clec’h, W., Braquart-Varnier, C., Raimond, M., Ferdy, J. B., Bouchon, D., and Sicard, M. (2012). High virulence of Wolbachia after host switching: when autophagy hurts. PLoS Pathog. 8:e1002844. doi: 10.1371/journal.ppat.1002844
Le Clec’h, W., Chevalier, F. D., Genty, L., Bertaux, J., Bouchon, D., and Sicard, M. (2013). Cannibalism and predation as paths for horizontal passage of Wolbachia between terrestrial isopods. PLoS ONE 8:e60232. doi: 10.1371/journal.pone.0060232
Le Clec’h, W., Raimond, M., Bouchon, D., and Sicard, M. (2014). Strength of the pathogenicity caused by feminizing Wolbachia after transfer in a new host: strain or dose effect? J. Invertebr. Pathol. 116, 18–26. doi: 10.1016/j.jip.2013.12.003
LePage, D., and Bordenstein, S. R. (2013). Wolbachia: can we save lives with a great pandemic? Trends Parasitol 29, 385–393. doi: 10.1016/j.pt.2013.06.003
Mercot, H., and Poinsot, D. (2009). Infection by Wolbachia: from passengers to residents. C. R. Biol. 332, 284–297. doi: 10.1016/j.crvi.2008.09.010
Pigeault, R., Braquart-Varnier, C., Marcade, I., Mappa, G., Mottin, E., and Sicard, M. (2014). Modulation of host immunity and reproduction by horizontally acquired Wolbachia. J. Insect Physiol. 70, 125–133. doi: 10.1016/j.jinsphys.2014.07.005
Pruneau, L., Moumène, A., Meyer, D., Marcelino, A., Lefrançois, T., and Vachiéry, N. (2014). Understanding Anaplasmataceae pathogenesis using “Omics” approaches. Front. Cell. Infect. Microbiol. 4:86. doi: 10.3389/fcimb.2014.00086
Rigaud, T., and Juchault, P. (1995). Success and failure of horizontal transfers of feminizing Wolbachia endosymbionts in woodlice. J. Evol. Biol. 8, 249–255. doi: 10.1046/j.1420-9101.1995.8020249.x
Rigaud, T., Mocquard, J. P., Juchault, P., Souty-Grosset, C., and Raimond, R. (1991). Feminizing endocytobiosis in the terrestrial crustacean Armadillidium vulgare Latr. (Isopoda): recent acquisitions. Endocytol. Cell Res. 7, 259–273.
Rikihisa, Y. (2010). Anaplasma phagocytophilum and Ehrlichia chaffeensis: subversive manipulators of host cells. Nat. Rev. Microbiol. 8, 328–339. doi: 10.1038/nrmicro2318
Saridaki, A., and Bourtzis, K. (2010). Wolbachia: more than just a bug in insects genitals. Curr. Opin. Microbiol. 13, 67–72. doi: 10.1016/j.mib.2009.11.005
Sicard, M., Chevalier, F., De Vlechouver, M., Bouchon, D., Greve, P., and Braquart-Varnier, C. (2010). Variations of immune parameters in terrestrial isopods: a matter of gender, aging and Wolbachia. Naturwissenschaften 97, 819–826. doi: 10.1007/s00114-010-0699-2
Sicard, M., Dittmer, J., Greve, P., Bouchon, D., and Braquart-Varnier, C. (2014). A host as an ecosystem: Wolbachia coping with environmental constraints. Environ. Microbiol. 16, 3583–3607. doi: 10.1111/1462-2920.12573
Weinert, L. A., Araujo-Jnr, E. V., Ahmed, M. Z., and Welch, J. J. (2015). The incidence of bacterial endosymbionts in terrestrial arthropods. Proc. R. Soc. B Biol. Sci. 282, 20150249. doi: 10.1098/rspb.2015.0249
Werren, J. H., Baldo, L., and Clark, M. E. (2008). Wolbachia: master manipulators of invertebrate biology. Nat. Rev. Microbiol. 6, 741–751. doi: 10.1038/nrmicro1969
Keywords: Wolbachia, intracellular endosymbiont, hematopoietic organs, hemocytes, terrestrial isopod, symbiosis, mobile vector
Citation: Braquart-Varnier C, Raimond M, Mappa G, Chevalier FD, Le Clec’h W and Sicard M (2015) The Hematopoietic Organ: A Cornerstone for Wolbachia Propagation Between and Within Hosts. Front. Microbiol. 6:1424. doi: 10.3389/fmicb.2015.01424
Received: 26 June 2015; Accepted: 30 November 2015;
Published: 18 December 2015.
Edited by:
Puttaraju H. P., Bangalore University, IndiaReviewed by:
Martin Zimmer, Leibniz Center for Tropical Marine Ecology, GermanyMiguel Moreno-García, Colorado State University, USA
Copyright © 2015 Braquart-Varnier, Raimond, Mappa, Chevalier, Le Clec’h and Sicard. This is an open-access article distributed under the terms of the Creative Commons Attribution License (CC BY). The use, distribution or reproduction in other forums is permitted, provided the original author(s) or licensor are credited and that the original publication in this journal is cited, in accordance with accepted academic practice. No use, distribution or reproduction is permitted which does not comply with these terms.
*Correspondence: Christine Braquart-Varnier, christine.braquart@univ-poitiers.fr