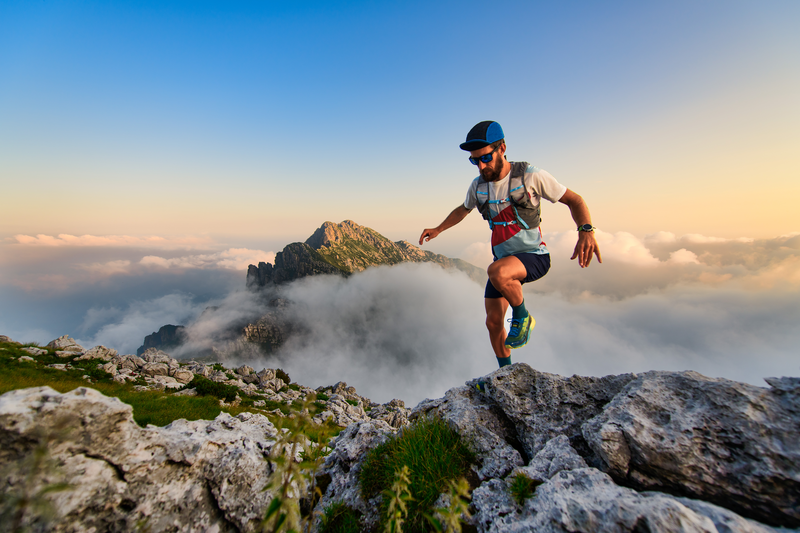
95% of researchers rate our articles as excellent or good
Learn more about the work of our research integrity team to safeguard the quality of each article we publish.
Find out more
METHODS article
Front. Microbiol. , 28 May 2015
Sec. Infectious Agents and Disease
Volume 6 - 2015 | https://doi.org/10.3389/fmicb.2015.00532
This article is part of the Research Topic Development of new diagnostic techniques, treatment approaches and control of cure of infectious diseases View all 8 articles
A large number of viral and bacterial organisms are responsible for community-acquired pneumonia (CAP) which contributes to substantial burden on health management. A new resequencing microarray (RPM-IVDC1) associated with targeted multiplex PCR was recently developed and validated for multiple respiratory viruses detection and discrimination. In this study, we evaluated the capability of RPM-IVDC1 for simultaneous identification of multiple viral and bacterial organisms. The nasopharyngeal aspirates (NPAs) of 110 consecutive CAP patients, aged from 1 month to 96 years old, were collected from five distinct general hospitals in Beijing during 1-year period. The samples were subjected to the RPM-IVDC1 established protocol as compared to a real-time PCR (qRT-PCR), which was used as standard. The results of virus detection were consistent with those previously described. A total of 37 of Streptococcus pneumoniae, 14 of Haemophilus influenzae, 10 of Mycoplasma pneumoniae, two of Klebsiella pneumoniae and one of Moraxella catarrhalis were detected by RPM-IVDC1. The sensitivities and specificities were compared with those of qRT-PCR for S. pneumoniae (100, 100%, respectively), H. influenzae (92.3, 97.9%, respectively), M. pneumoniae (69.2, 99.0%, respectively), K. pneumoniae (100, 100%, respectively), and M. catarrhalis (100, 100%, respectively). Additional 22 of Streptococcus spp., 24 of Haemophilus spp. and 16 of Neisseria spp. were identified. In addition, methicillin-resistant and carbapenemases allele were also found in nine of Staphylococcus spp. and one of K. pneumoniae, respectively. These results demonstrated the capability of RPM-IVDC1 for simultaneous detection of broad-spectrum respiratory pathogens in complex backgrounds and the advantage of accessing to the actual sequences, showing great potential use of epidemic outbreak investigation. The detection results should be carefully interpreted when introducing this technique in the clinical diagnostics.
Acute respiratory infection (ARI) is a leading cause of morbidity and mortality worldwide. The community-acquired pneumonia (CAP) resulted from ARI remains to be a common and serious illness and contributes to a substantial burden on health services (Bartlett and Mundy, 1995; Welte et al., 2012). A large and growing number of viral and bacterial organisms are responsible for CAP (Juven et al., 2000; Johansson et al., 2010). In addition to the predominant causative agents (most commonly Streptococcus pneumoniae, Haemophilus influenzae, Mycoplasma pneumoniae, respiratory syncytial virus, and influenza virus) (Rudan et al., 2008, 2013), new pathogens including Coronaviruses (CoV-NL63 and CoV-HKU1) (Moes et al., 2005; Woo et al., 2005), human metapneumovirus (hPMV) (Hamelin et al., 2005), human bocavirus (hBoV) (Fry et al., 2007) and H7N9 (Uyeki and Cox, 2013) are frequently being reported. Some of these pathogens can cause severe cases of pneumonia and pandemic (Uyeki and Cox, 2013).
A large number of studies have been carried out to detect the etiology of CAP, showing that high prevalence of mixed viral-bacterial infections occured in CAP patients (Tsolia et al., 2004; Kumar et al., 2008). In addition, some bacterial infections were associated with critically illness (Trouillet et al., 1998; Gruson et al., 2000). Because of the difficulty in differentiating between pathogens based on clinical signs or radiology, the identification of pathogens in severe respiratory tract infections would be of great value to assist medical treatment. Therefore, to improve the capability of diagnosis of serious respiratory illnesses a powerful assay that is capable of broad-spectrum pathogen detection and identification is urgently needed.
The resequencing microarray, depending on hybridization between probes attached to the array and complementary fragments of nucleic acids in the specimens, offered detailed microbial characterization and broad-range pathogen detection (Leski et al., 2012). An important feature of this approach was that it generated actual sequences of the detected targets. The target sequence generated by the hybridization between closely overlapping probe sets and complementary fragments was compared with a sequence database. Benefit from the advantage of obtaining reliable sequence information, the microarray-based resequencing assay achieved high-resolution target discrimination and identification of target mixtures (Leski et al., 2012). Thus, this technology has been applied for high-resolution multiplexed diagnostics for clinical syndromes with similar symptoms (Lin et al., 2006, 2007; Leski et al., 2009).
We previously described a new resequencing pathogen microarray based assay (RPM-IVDC1) showing excellent capability for detecting broad-range CAP-associated viruses (Shen et al., 2013). The RPM-IVDC1 assay could sequence 47,974 bp of both strands of targeted gene sequences, distributed across 183 detector tiles (each 224-bp in length), representing 86 types/subtypes of viral pathogens and 21 other respiratory tract pathogens that cause respiratory infection (Shen et al., 2013). But the capacity of RPM-IVDC1 for simultaneous detection of viral and bacterial pathogens with improved application in microbial diagnostics has not been validated. In this study, 110 Nasopharynx aspirates (NPAs) from CAP patients in five district hospitals in Beijing were selected and tested by RPM-IVDC1 assay. The bacterial detection results were additionally confirmed by specific real-time PCR assays which is the gold standard in this case.
From January 2011 to December 2011, participants for radiologically verified CAP were enrolled from Beijing Pneumonia Surveillance. The data on all hospital admissions, other important medical events, culture results of mycoplasma and viral infections were collected. Among these participants, the patients with complete information regarding demographic character, radiological results and clinical manifestations were enrolled in this study. A total of 110 CAP patients (62 of whom were female) meeting the criteria, aged from 1 month to 96 years, were selected in this study (Table 1).
NPAs were collected from all patients admitting hospitals. Five milliliters of sterile saline was squirted into one nostril, and a 6Fr suction catheter was placed into the nasopharynx using the opposite nostril, pointing the end of the tube directly backward, with the patient aspirating while the suction catheter was gently removed. After ensuring that the specimen was cloudy and had mucus and cells, it was immediately placed on ice and mixed with a universal transport medium (UTM, Copan Diagnostic, Inc., Murrieta, CA) before transporting to ultra-low temperature freezer in district hospital. Next, batches of specimens were transported to Beijing CDC in 5 days, on dry ice, where they were divided into aliquots for culture and PCR, and the remainder was stored at −80°C.
The complete nucleic acid of microorganisms and human cells from NPAs was extracted and purified using the MasterPure Complete DNA and RNA Purification kit (Epicenter Technologies, Madison, WI) according to the manufacture's recommended protocols. Purified DNA and RNA were frozen at −80°C in 15 μl aliquots for the following PCR. A reverse transcription PCR using SuperScript III (Invitrogen Life Technologies, Carlsbad, CA) associated with five targeted multiplex PCR assays (assays A-E) were performed before subjecting to RPM-IVDC1 process. Each of the multiplex PCR tubes contained primers representing different pathogens and internal controls as previously described (Shen et al., 2013). Primer mixes A-D were previously described (Shen et al., 2013) (see Supplementary Tables 1–4 in the Supplemental Material), while primer mix E contained 37 primer pairs corresponding to 35 target genes of Bordetella pertussis, Chlamydia trachomatis, Chlamydophila pneumoniae, Chlamydophila psittaci, Corynebacterium diphtheriae, H. influenzae, K. pneumoniae, Legionella pneumophila, Moraxella catarrhalis, M. pneumoniae, Pseudomonas aeruginosa, Pseudomonas spp., Staphylococcus aureus, S. pneumoniae, Sreptococcus agalactiae, Streptococcus pyogenes (group A Streptococcus, GAS), Francisella tularensis, Neisseria meningitides, Neisseria gonorrhoeae, Neisseria spp. and one internal control (TIM-2) (see Supplementary Table 5 in the Supplemental Material). The amplification reactions were carried out in a Px2 Thermo Cycler (Thermo Electron Corp, Vantaa, Finland) with the same reaction conditions as previously described (Shen et al., 2013).
The products from five multiplex PCR assays were pooled together and subjected to purification (Qiagen, Germany), processing, and hybridization in a GeneChip Hybridization Oven 640 (Affymetrix Inc, Santa Clara, CA) according to the manufacture's recommended protocol(Malanoski et al., 2006; Lin et al., 2007). However, the hybridization was performed at 49°C for 12 h, which was much shorter than the time previously reported resequencing microarray adopted(Lin et al., 2007, 2009). The process of staining and scanning followed by hybridization were performed in a GeneChip Fluidics Station 450 (Affymetrix Inc, Santa Clara, CA). The data collected by microarray were transferred to a computer to create scanned images. A FASTA output file produced by processing the scanned image using TessArray Sequence Analysis (TSEQ) (Malanoski et al., 2006) was subjected to alignment with corresponding detector tile sequences. The alignment results were evaluated by “C3 Score,” which means the total number of TSEQ-identified nucleotides that appear in runs of three or more consecutive (non-N) base calls, expressed as percentage of the length (nucleotides) of each RPM-IVDC1 detector tile sequence (Lin et al., 2009).
Detection and quantification of the targets identified by RPM-IVDC1 were confirmed by using qRT-PCR (7500 real-time PCR system; Applied Biosystems) as described previously. These targets included S. pneumoniae, H. influenzae, M. catarrhalis, K. pneumoniae, and M. pneumoniae (Table 2). Compared with the targets identified by RPM-IVDC1, these qRT-PCRs targeted other regions of the same gene or other genes different from RPM-IVDC1 targets. A positive and negative control in each set PCR assay was included to survey the possibility of laboratory contamination. All the qRT-PCR methods were reported previously (Table 2) and validated in the lab of National Institute for Communicable Disease Control and Prevention, Chinese Center for Disease Control and Prevention. For culture of M. pneumoniae, a 50 μl aliquot of NPAs was plated on SP4 agar and the putative M. pneumoniae colonies were verified by nested PCR (Dorigo-Zetsma et al., 1999).
For others potential respiratory bacteria including S. aureus, P. aeruginosa, Mycobacterium tuberculosis, Neisseria meningitides, and GAS not covered by RPM-IVDC1, an additional set of qRT-PCRs targeting these organisms were performed in consideration of probability of false-negative results. All the qRT-PCR methods were reported previously (Table 2) and validated in our laboratory.
Eligibility and classification of the clinical syndromes of CAP were determined from the original record of each item on the medical history and examination in the database. RPM-IVDC1-identified results were compared with qRT-PCRs to evaluate its sensitivity, specificity, and overall diagnostic accuracy. The frequency distributions of the important pathogens among different ages of CAP patients and among different seasons were described and compared with those reported in the literatures. The C3 score, expressed as percentage of the length (nucleotides) of each RPM-IVDC1 detector tile sequence, was set to 40 in this study. The alignment results of targeted bacterial sequences with C3 score above 40 were tested as positive (Table 3).
All aspects of this study were performed in accordance with national ethics regulations and approved by the Institutional Review Boards of the Center for Disease Control and Prevention of China and the Ethics Committee of the Beijing Center for Disease Control and Prevention (Beijing CDC). The participants received written information regarding the purpose of the study and of their right to confidentiality. Individual written informed consent was obtained from adult participants or children's parents or guardians.
The simultaneous detection results of viral pathogens were the same as previously described (Shen et al., 2013), demonstrating that the high specificity of RPM-IVDC1 for viruses detection was not interfered by the complex background. A viral etiology was found in 53 patients (48%), 12 of whom had more than one viral species identified. It was noted that the housekeeping genes were targeted and the corresponding bacteria could only be identified at species-level (e.g., gyrase A subunit gene of Neisseria spp.).
M. pneumoniae (8/10) and S. pneumoniae (21/37) were mainly detected in virus-negative samples, indicating that these organisms may be the causative agent of CAP. S. pneumoniae (4/4) and H. influenzae (3/4) were frequently found in the coronavirus (CoV)-infected samples, which may be the result of secondary infection induced by CoV or co-infection. A similar situation occurred in parainfluenza virus (PIV) and respiratory syncytial virus (RSV) infections, where four of seven PIV-positive and three of five RSV-positive samples were positive for S. pneumoniae, compared with detection rate of 24% (6/25) for S. pneumoniae among others virus infections (data not shown).
The chosen cutoff value resulted in 37 samples positive for S. pneumoniae, 14 positive for H. influenzae, 10 positive for M. pneumoniae, one positive for M. catarrhalis, and two positive for K. pneumoniae. Additional 22 specimens were positive for Streptococcus spp., 24 were positive for Haemophilus spp., 16 were positive for Neisseria spp., and 10 were positive for Staphylococcus spp. mecA (Table 3). According to the results of confirmatory qRT-PCR, two of 14 H. influenzae microarray-positive specimens were false positive. The RPM-IVDC1 assay failed to identify 4 specimens that were positive for M. pneumoniae by qRT-PCR. One NPAs sample with a true-positive result for M. pneumoniae, as confirmed by mycoplasma culture, was not detected by qRT-PCR (Table 4). The specimens that were positive for Streptococcus spp., Haemophilus spp., and Neisseria spp. were confirmed by 16s rRNA sequencing(Klindworth et al., 2013) (data not shown). Of these, nine of 10 Staphylococcus spp. mecA positive samples were confirmed by qRT-PCR and one was false positive (Table 5).
Table 4. Comparison of RPM-IVDC1 with specific qRT-PCR assays for detection of M. pneumoniae, S. pneumoniae, and H. influenzaea.
Table 5. Comparison of RPM-IVDC1 with specific qRT-PCR assays for detection of other respiratory bacteria.
The sensitivities, specificities, and PPVs for the RPM-IVDC1 assay were shown in Table 6. The sensitivities for the three most important bacterial pathogens, S. pneumoniae, H. influenzae, and M. pneumoniae, were 100, 92.3, and 69.2%, respectively. The number of specimens positive for K. pneumoniae and M. catarrhalis was too small to allow for a realistic evaluation of sensitivities. The resequencing assay showed excellent specificities for these organisms, ranging from 97.9 to 100%. The PPVs for the five common pathogens varied from 85.7 to 100%.
Due to the commensal and asymptomatic colonization of the upper respiratory tract, it was difficult to distinguish colonization from pathogen infection. Another hurdle for determining the etiology of these CAP patients was that the RPM-IVDC1 could only identify some organisms at species-level by conserved targeting (e.g., Neisseria spp.). Therefore, the distributions of only the four most important pathogens were analyzed (Table 7). The detection rate of S. pneumoniae and H. influenzae in autumn (September–November) was 41.4 and 17.2%, compared with 30.9 and 8.6% of the other seasons, respectively. Among the children aged less than 5 years, S. pneumoniae and H. influenzae were detected in 68.2 and 22.7% of this age group, compared with 25 (22/88) and 8.0% (7/88) of the other groups, respectively. The positive results for M. pneumoniae were mainly found in age group of 5 to 14 years (26.3%, 5/19) and of 15 to 24 years (15.0%, 3/20). Interestingly, the detection rate of M. pneumoniae among the male (14.6%) was obviously higher than the female patients (4.8%). The two positive results for K. pneumoniae were detected from two male patients aged 72 and 53 years, respectively.
Table 7. The distribution of four important respiratory bacteria among 110 CAP patients (age, 0.1–96 years) detected by RPM-IVDC1.
The simultaneous detection of broad-spectrum pathogens causing similar clinical syndromes is beneficial to public health management. The previously reported RPM-IVDC1 assay offered a sensitive, accurate, and specific identification for multiple respiratory tract viruses infection (Shen et al., 2013). However, the ability of this assay to diagnose bacterial infection was not validated. Therefore, the purpose of this study is to evaluate the use of RPM-IVDC1 assay for detecting both respiratory viruses and bacteria using the same batch of NPAs samples of CAP patients.
To achieve high sensitivity of pathogen detection, both the pathogenicity-related genes and conserved genes were selected and the corresponding sequences were tiled on RPM-IVDC1. In this study, the combination of highly multiplexed PCR with resequencing array allowed for accurate detection of S. pneumoniae, K. pneumoniae, H. influenzae, and M. pneumoniae. The comparison between RPM-IVDC1 and established qRT-PCR methods demonstrated a concordance for two of two samples in K. pneumoniae, nine of 13 samples in M. pneumoniae, 12 of 13 samples in H. influenzae, and 37 of 37 samples in S. pneumoniae. Among the inconsistent results, one sample that was negative for M. pneumoniae by qRT-PCR was detected in microarray analysis and confirmed by mycoplasma culture. The disagreement over one sample in H. influenzae and five samples in M. pneumoniae identification may have resulted from the differences in sensitivity and specificity of the two methods.
The RPM-IVDC1 assay maintained coverage of a large number of pathogens. Due to this advantage, some uncommon types of Haemophilus spp., Streptococcus spp., and Neisseria spp. were identified. As an example, the targeted sequence of adenylate cyclase (adk) gene could be used to identify H. influenzae by the sequence alignment, while the targeted sequence of outer membrane protein (skp) gene could be used to detect Haemophilus spp. at species-level. The specific qRT-PCR confirmed 12 of 14 RPM-positive results for H. influenzae which might be the causative agents of CAP (Barnes et al., 1987) and was of particular importance for respiratory infections in children (Nascimento-Carvalho, 2001). Haemophilus parainfluenzae and Haemophilus haemolyticus, the other two types of Haemophilus spp., were commonly considered as commensals colonizing the upper respiratory tract asymptomatically (Mukundan et al., 2007; Murphy et al., 2007). However, recent data suggested that occasional clinical disease might be caused by the two intimately related species of H. influenzae (Pillai et al., 2000; Anderson et al., 2012). A similar situation occurred in the detection of Neisseria spp. where only species-level identification could be achieved by RPM-IVDC1. Interestingly, the Neisseria spp. positive results were mainly found among children aged less than 5 years (9/16). Among the children aged under 2 years, the detection rate was highest (38.5%, 5/13), which was similar to the carriage prevalence of Neisseria lactamica, a N. meningitides related species but living as harmless commensal (Kristiansen et al., 2012). Although RPM-IVDC1 could not identify these organisms at type-level, the species detection information may be valuable for further analysis in the context of outbreak investigation.
The wide coverage of respiratory pathogens by RPM-IVDC1 assay enabled it an ideal tool for real-world diagnostics and surveillance. Besides the pathogens that were frequently detected in the upper airways (e.g., S. pneumoniae, H. influenzae), some well-recognized causes of CAP but rarely found in NPAs were detected by RPM-IVDC1. The detection of these atypical bacteria (e.g., M. pneumoniae, K. pneumoniae) might be of great predictive value for diagnosing the etiologic agent, which had an essential role in guiding the development of treatment and prevention strategies. K. pneumoniae has been a recognized cause of bacterial pneumonia since its discovery (Ko et al., 2002), but the incidence of community-acquired Klebsiella pneumonia has apparently declined in China in recent years (Liu et al., 2006). The detection of K. pneumoniae in the upper respiratory tract may help the etiologic diagnosis and thus initiate most appropriate and effective therapy in the context of pneumonia. In this study, a total of two samples were identified as K. pneumoniae positive by the alignment of two housekeeping loci sequences, fumC and mdh, which indicated the possibility of Klebsiella pneumonia. The subsequent specific real-time confirmed a Klebsiella pneumoniae carbapenemases (KPCs)-positive strain from a 53 years old CAP patient. The combination of RPM-IVDC1 result and antibiotic resistance test would be helpful for clinical therapy.
An additional advantage of RPM-IVDC1 assay was the capability of acquiring the actual sequences of the detected targets. The specificity of RPM-IVDC1 could be improved by the automated alignment of target sequences by TESQ. Of the 39 ply-positive samples of which C3 score meeting the cutoff value, a total of two samples were identified as Streptococcus pseudopneumoniae, which were confirmed by qRT-PCR (Sistek et al., 2012) (data not shown). The advantage of sequence alignment avoided resulting in false-positive for S. pneumoniae. As for the Staphylococcus spp. detection, a total of 10 samples were detected positive for mecA allele, a conserved gene encoded penicillin-binding protein causing methicillin-resistant of S. aureus (MRSA). The classical mecA gene was not restricted to S. aureus but was commonly found in other Staphylococci such as, S. epidermidis, S. haemolyticus, and S. pseudintermedius (Murakami et al., 1991). Our alignment results showed that these mecA-positive samples were Staphylococcus spp. positive but non- S. aureus. The following S. aureus specific qRT-PCR confirmed the detection results of RPM-IVDC1, except for one false-positive sample. Therefore, the access to the actual sequences of the targets and automated alignment performed by TESQ could avoid false-positive and improve the specificity of RPM-IVDC1 array.
Some characteristic distributions of the detected pathogens were found by RPM-IVDC1. S. pneumoniae, H. influenzae, and M. pneumoniae were the most significant pathogens detected among these patients and the detection rate of these pathogens were relatively higher among children below the age of 5 years than other age groups, which were in concordance with other studies (Mundy et al., 1995). The pathogen detection rate was relatively higher in winter than in other seasons, which may result from the cold weather (Young, 1924). With the additional four false-negative samples and one sample confirmed by culture, eight of 14 samples positive for M. pneumoniae were found in the age group of two to 14 years and nine of 14 positive results were found among male patients. However, there were no statistically significant correlations between the prevalence of M. pneumoniae and age or gender in both symptomatic (p = 0.91, p = 0.22, respectively) and asymptomatic group (p = 0.36, p = 0.84, respectively) in the study conducted by Spuesens et al. (2013). The phenomena found in our study may be attributed to the limited sample size. The absence of S. aureus and lower detection rate of M. catarrhalis than that in other studies (Vaneechoutte et al., 1990; Mundy et al., 1995) may be due to the small sample size, sample quality, and the use of antibiotics prior to sampling.
The RPM-IVDC1 assay provided a sensitive and comprehensive alternative to conventional approaches to the diagnosis and surveillance of respiratory infections. However, some issues must be noted. First, a total of three false-positive results were found among these detections. Of these, two specimens were false-positive for H. influenzae, one specimen was false-positive for Streptococcus spp. The false-positive results were due to the procedures involving opened PCR plates in this assay, such as multiplex PCR, purification, processing, hybridization, and scanning. The three false positive results were not found after retesting. The handling of unsealed specimens for testing by RPM-IVDC1 was one of the major concerns regarding the assay. The application of decontamination procedures, such as dUTP uracil glycosylase treatment, may be operable in preventing from contamination. Notably, our routine use of the assay after the study period for outbreak investigation has not revealed any contamination.
In addition, when this RPM-IVDC1 assay is applied in pathogen detection for severe respiratory infections or outbreak investigation, carefully considerations must be given. The interpretation of testing results may be complicated due to the commensal colonization of the upper respiratory tract and the difficulty of obtaining a high-quality specimen (Blaschke, 2011). In this study, although RPM-IVDC1 detected S. pneumoniae in most samples, it was difficult to distinguish colonization from invasive infection. The quantitative assay might be necessary to accurately identify patients with true pneumococcal disease because most commensal microflora appeared to be present at low titers in the samples. On the other hand, although the RPM assay did not determine the titers of the pathogens detected, a negative nasopharyngeal test might be useful to exclude pneumococcal CAP. The species-level identifications of Staphylococcus spp., Haemophilus spp., Streptococcus spp., and Neisseria spp. may be of great significance in outbreak investigation when the colonization could be excluded.
In conclusion, the RPM-IVDC1 assay described here demonstrated capability of simultaneous detection of broad-spectrum respiratory pathogens. The mixing of specific and conserved targets, association with highly multiplexed PCR, as well as acquiring actual targeted sequence improved its sensitivity and specificity while maintaining coverage of a large number of pathogens. When introducing this method in clinical diagnostic for severe respiratory infections or epidemic outbreak investigation, carefully considerations about the results interpretation must be given.
The views expressed in this article are those of the authors and do not necessarily represent the views of the Chinese Center for Disease Control and Prevention.
The authors declare that the research was conducted in the absence of any commercial or financial relationships that could be construed as a potential conflict of interest.
This work was supported by the China Mega-Project for Infectious Disease (2013ZX10004-001, 2012ZX10004-215, 2013ZX10004-202, and 2013ZX10004804-007).
The Supplementary Material for this article can be found online at: http://journal.frontiersin.org/article/10.3389/fmicb.2015.00532/abstract
Anderson, R., Wang, X., Briere, E. C., Katz, L. S., Cohn, A. C., Clark, T. A., et al. (2012). Haemophilus haemolyticus isolates causing clinical disease. J. Clin. Microbiol. 50, 2462–2465. doi: 10.1128/JCM.06575-11
Barnes, D. J., Naraqi, S., and Igo, J. D. (1987). Haemophilus influenzae pneumonia in Melanesian adults: report of 15 cases. Thorax 42, 889–891.
Bartlett, J. G., and Mundy, L. M. (1995). Community-acquired pneumonia. N. Engl. J. Med. 333, 1618–1624.
Blaschke, A. J. (2011). Interpreting assays for the detection of Streptococcus pneumoniae. Clin. Infect. Dis. 52(Suppl 4), S331–S337. doi: 10.1093/cid/cir048
Boving, M. K., Pedersen, L. N., and Moller, J. K. (2009). Eight-plex PCR and liquid-array detection of bacterial and viral pathogens in cerebrospinal fluid from patients with suspected meningitis. J. Clin. Microbiol. 47, 908–913. doi: 10.1128/JCM.01966-08
Cole, J. M., Schuetz, A. N., Hill, C. E., and Nolte, F. S. (2009). Development and evaluation of a real-time PCR assay for detection of Klebsiella pneumoniae carbapenemase genes. J. Clin. Microbiol. 47, 322–326. doi: 10.1128/JCM.01550-08
Dorigo-Zetsma, J. W., Zaat, S. A., Wertheim-van Dillen, P. M., Spanjaard, L., Rijntjes, J., van Waveren, G., et al. (1999). Comparison of PCR, culture, and serological tests for diagnosis of Mycoplasma pneumoniae respiratory tract infection in children. J. Clin. Microbiol. 37, 14–17.
Feizabadi, M. M., Majnooni, A., Nomanpour, B., Fatolahzadeh, B., Raji, N., Delfani, S., et al. (2010). Direct detection of Pseudomonas aeruginosa from patients with healthcare associated pneumonia by real time PCR. Infect. Genet. Evol. 10, 1247–1251. doi: 10.1016/j.meegid.2010.08.008
Fry, A. M., Lu, X., Chittaganpitch, M., Peret, T., Fischer, J., Dowell, S. F., et al. (2007). Human bocavirus: a novel parvovirus epidemiologically associated with pneumonia requiring hospitalization in Thailand. J. Infect. Dis. 195, 1038–1045. doi: 10.1086/512163
Greiner, O., Day, P. J., Altwegg, M., and Nadal, D. (2003). Quantitative detection of Moraxella catarrhalis in nasopharyngeal secretions by real-time PCR. J. Clin. Microbiol. 41, 1386–1390. doi: 10.1128/JCM.41.4.1386-1390.2003
Greiner, O., Day, P. J., Bosshard, P. P., Imeri, F., Altwegg, M., and Nadal, D. (2001). Quantitative detection of Streptococcus pneumoniae in nasopharyngeal secretions by real-time PCR. J. Clin. Microbiol. 39, 3129–3134. doi: 10.1128/JCM.39.9.3129-3134.2001
Gruson, D., Hilbert, G., Vargas, F., Valentino, R., Bebear, C., Allery, A., et al. (2000). Rotation and restricted use of antibiotics in a medical intensive care unit: impact on the incidence of ventilator-associated pneumonia caused by antibiotic-resistant gram-negative bacteria. Am. J. Respir. Crit. Care Med. 162, 837–843. doi: 10.1164/ajrccm.162.3.9905050
Hamelin, M. E., Cote, S., Laforge, J., Lampron, N., Bourbeau, J., Weiss, K., et al. (2005). Human metapneumovirus infection in adults with community-acquired pneumonia and exacerbation of chronic obstructive pulmonary disease. Clin. Infect. Dis. 41, 498–502. doi: 10.1086/431981
Johansson, N., Kalin, M., Tiveljung-Lindell, A., Giske, C. G., and Hedlund, J. (2010). Etiology of community-acquired pneumonia: increased microbiological yield with new diagnostic methods. Clin. Infect. Dis. 50, 202–209. doi: 10.1086/648678
Juven, T., Mertsola, J., Waris, M., Leinonen, M., Meurman, O., Roivainen, M., et al. (2000). Etiology of community-acquired pneumonia in 254 hospitalized children. Pediatr. Infect. Dis. J. 19, 293–298. doi: 10.1097/00006454-200004000-00006
Klindworth, A., Pruesse, E., Schweer, T., Peplies, J., Quast, C., Horn, M., et al. (2013). Evaluation of general 16S ribosomal RNA gene PCR primers for classical and next-generation sequencing-based diversity studies. Nucleic Acids Res. 41:e1. doi: 10.1093/nar/gks808
Ko, W.-C., Paterson, D. L., Sagnimeni, A. J., Hansen, D. S., Von Gottberg, A., Mohapatra, S., et al. (2002). Community-acquired Klebsiella pneumoniae bacteremia: global differences in clinical patterns. Emerg. Infect. Dis. 8, 160–166. doi: 10.3201/eid0802.010025
Kristiansen, P. A., Diomande, F., Ouedraogo, R., Sanou, I., Sangare, L., Ouedraogo, A. S., et al. (2012). Carriage of Neisseria lactamica in 1- to 29-year-old people in Burkina Faso: epidemiology and molecular characterization. J. Clin. Microbiol. 50, 4020–4027. doi: 10.1128/JCM.01717-12
Kumar, S., Wang, L., Fan, J., Kraft, A., Bose, M. E., Tiwari, S., et al. (2008). Detection of 11 common viral and bacterial pathogens causing community-acquired pneumonia or sepsis in asymptomatic patients by using a multiplex reverse transcription-PCR assay with manual (enzyme hybridization) or automated (electronic microarray) detection. J. Clin. Microbiol. 46, 3063–3072. doi: 10.1128/JCM.00625-08
Leski, T. A., Lin, B., Malanoski, A. P., and Stenger, D. A. (2012). Application of resequencing microarrays in microbial detection and characterization. Future Microbiol. 7, 625–637. doi: 10.2217/fmb.12.30
Leski, T. A., Lin, B., Malanoski, A. P., Wang, Z., Long, N. C., Meador, C. E., et al. (2009). Testing and validation of high density resequencing microarray for broad range biothreat agents detection. PLoS ONE 4:e6569. doi: 10.1371/journal.pone.0006569
Lin, B., Blaney, K. M., Malanoski, A. P., Ligler, A. G., Schnur, J. M., Metzgar, D., et al. (2007). Using a resequencing microarray as a multiple respiratory pathogen detection assay. J. Clin. Microbiol. 45, 443–452. doi: 10.1128/JCM.01870-06
Lin, B., Malanoski, A. P., Wang, Z., Blaney, K. M., Long, N. C., Meador, C. E., et al. (2009). Universal detection and identification of avian influenza virus by use of resequencing microarrays. J. Clin. Microbiol. 47, 988–993. doi: 10.1128/JCM.01346-08
Lin, B., Wang, Z., Vora, G. J., Thornton, J. A., Schnur, J. M., Thach, D. C., et al. (2006). Broad-spectrum respiratory tract pathogen identification using resequencing DNA microarrays. Genome Res. 16, 527–535. doi: 10.1101/gr.4337206
Liu, Y., Chen, M., Zhao, T., Wang, H., Wang, R., Liu, Q., et al. (2006). [A multicentre study on the pathogenic agents in 665 adult patients with community-acquired pneumonia in cities of China]. Zhonghua Jie He He Hu Xi Za Zhi 29, 3–8.
Louie, L., Simor, A. E., Louie, M., McGeer, A., and Low, D. E. (1998). Diagnosis of group A streptococcal necrotizing fasciitis by using PCR to amplify the streptococcal pyrogenic exotoxin B gene. J. Clin. Microbiol. 36, 1769–1771.
Luo, R. F., Scahill, M. D., and Banaei, N. (2010). Comparison of single-copy and multicopy real-time PCR targets for detection of Mycobacterium tuberculosis in paraffin-embedded tissue. J. Clin. Microbiol. 48, 2569–2570. doi: 10.1128/JCM.02449-09
Malanoski, A. P., Lin, B., Wang, Z., Schnur, J. M., and Stenger, D. A. (2006). Automated identification of multiple micro-organisms from resequencing DNA microarrays. Nucleic Acids Res. 34, 5300–5311. doi: 10.1093/nar/gkl565
Moes, E., Vijgen, L., Keyaerts, E., Zlateva, K., Li, S., Maes, P., et al. (2005). A novel pancoronavirus RT-PCR assay: frequent detection of human coronavirus NL63 in children hospitalized with respiratory tract infections in Belgium. BMC Infect. Dis. 5:6. doi: 10.1186/1471-2334-5-6
Mukundan, D., Ecevit, Z., Patel, M., Marrs, C. F., and Gilsdorf, J. R. (2007). Pharyngeal colonization dynamics of Haemophilus influenzae and Haemophilus haemolyticus in healthy adult carriers. J. Clin. Microbiol. 45, 3207–3217. doi: 10.1128/JCM.00492-07
Mundy, L. M., Auwaerter, P. G., Oldach, D., Warner, M. L., Burton, A., Vance, E., et al. (1995). Community-acquired pneumonia: impact of immune status. Am. J. Respir. Crit. Care Med. 152(4 Pt 1), 1309–1315.
Murakami, K., Minamide, W., Wada, K., Nakamura, E., Teraoka, H., and Watanabe, S. (1991). Identification of methicillin-resistant strains of staphylococci by polymerase chain reaction. J. Clin. Microbiol. 29, 2240–2244.
Murphy, T. F., Brauer, A. L., Sethi, S., Kilian, M., Cai, X., and Lesse, A. J. (2007). Haemophilus haemolyticus : a human respiratory tract commensal to be distinguished from Haemophilus influenzae. J. Infect. Dis. 195, 81–89. doi: 10.1086/509824
Nascimento-Carvalho, C. M. (2001). Etiology of childhood community acquired pneumonia and its implications for vaccination. Braz. J. Infect. Dis. 5, 87–97. doi: 10.1590/S1413-86702001000200007
Pillai, A., Mitchell, J. L., Hill, S. L., and Stockley, R. A. (2000). A case of Haemophilus parainfluenzae pneumonia. Thorax 55, 623–624. doi: 10.1136/thorax.55.7.623
Rudan, I., Boschi-Pinto, C., Biloglav, Z., Mulholland, K., and Campbell, H. (2008). Epidemiology and etiology of childhood pneumonia. Bull. World Health Organ. 86, 408–416. doi: 10.2471/BLT.07.048769
Rudan, I., O'Brien, K. L., Nair, H., Liu, L., Theodoratou, E., Qazi, S., et al. (2013). Epidemiology and etiology of childhood pneumonia in 2010: estimates of incidence, severe morbidity, mortality, underlying risk factors and causative pathogens for 192 countries. J. Glob. Health 3:010401. doi: 10.7189/jogh.03.010401
Ruimy, R., Dos-Santos, M., Raskine, L., Bert, F., Masson, R., Elbaz, S., et al. (2008). Accuracy and potential usefulness of triplex real-time PCR for improving antibiotic treatment of patients with blood cultures showing clustered gram-positive cocci on direct smears. J. Clin. Microbiol. 46, 2045–2051. doi: 10.1128/JCM.02250-07
Shen, H., Shi, W., Wang, J., Wang, M., Li, J., Zhang, C., et al. (2013). Development of a new resequencing pathogen microarray based assay for detection of broad-spectrum respiratory tract viruses in patients with community-acquired pneumonia. PLoS ONE 8:e75704. doi: 10.1371/journal.pone.0075704
Sistek, V., Boissinot, M., Boudreau, D. K., Huletsky, A., Picard, F. J., and Bergeron, M. G. (2012). Development of a real-time PCR assay for the specific detection and identification of Streptococcus pseudopneumoniae using the recA gene. Clin. Microbiol. Infect. 18, 1089–1096. doi: 10.1111/j.1469-0691.2011.03684.x
Spuesens, E. B., Fraaij, P. L., Visser, E. G., Hoogenboezem, T., Hop, W. C., van Adrichem, L. N., et al. (2013). Carriage of Mycoplasma pneumoniae in the upper respiratory tract of symptomatic and asymptomatic children: an observational study. PLoS Med. 10:e1001444. doi: 10.1371/journal.pmed.1001444
Trouillet, J. L., Chastre, J., Vuagnat, A., Joly-Guillou, M. L., Combaux, D., Dombret, M. C., et al. (1998). Ventilator-associated pneumonia caused by potentially drug-resistant bacteria. Am. J. Respir. Crit. Care Med. 157, 531–539.
Tsolia, M. N., Psarras, S., Bossios, A., Audi, H., Paldanius, M., Gourgiotis, D., et al. (2004). Etiology of community-acquired pneumonia in hospitalized school-age children: evidence for high prevalence of viral infections. Clin. Infect. Dis. 39, 681–686. doi: 10.1086/422996
Uyeki, T. M., and Cox, N. J. (2013). Global concerns regarding novel influenza A (H7N9) virus infections. N. Engl. J. Med. 368, 1862–1864. doi: 10.1056/NEJMp1304661
Vaneechoutte, M., Verschraegen, G., Claeys, G., Weise, B., and Van den Abeele, A. M. (1990). Respiratory tract carrier rates of Moraxella (Branhamella) catarrhalis in adults and children and interpretation of the isolation of M. catarrhalis from sputum. J. Clin. Microbiol. 28, 2674–2680.
van Ketel, R. J., de Wever, B., and van Alphen, L. (1990). Detection of Haemophilus influenzae in cerebrospinal fluids by polymerase chain reaction DNA amplification. J. Med. Microbiol. 33, 271–276.
Welte, T., Torres, A., and Nathwani, D. (2012). Clinical and economic burden of community-acquired pneumonia among adults in Europe. Thorax 67, 71–79. doi: 10.1136/thx.2009.129502
Wessels, E., Schelfaut, J. J., Bernards, A. T., and Claas, E. C. (2012). Evaluation of several biochemical and molecular techniques for identification of Streptococcus pneumoniae and Streptococcus pseudopneumoniae and their detection in respiratory samples. J. Clin. Microbiol. 50, 1171–1177. doi: 10.1128/JCM.06609-11
Winchell, J. M., Thurman, K. A., Mitchell, S. L., Thacker, W. L., and Fields, B. S. (2008). Evaluation of three real-time PCR assays for detection of Mycoplasma pneumoniae in an outbreak investigation. J. Clin. Microbiol. 46, 3116–3118. doi: 10.1128/JCM.00440-08
Woo, P. C., Lau, S. K., Chu, C. M., Chan, K. H., Tsoi, H. W., Huang, Y., et al. (2005). Characterization and complete genome sequence of a novel coronavirus, coronavirus HKU1, from patients with pneumonia. J. Virol. 79, 884–895. doi: 10.1128/JVI.79.2.884-895.2005
Keywords: resequencing microarray, RPM-IVDC1, community-acquired pneumonia, multiple respiratory pathogens, detection
Citation: Shen H, Zhu B, Wang S, Mo H, Wang J, Li J, Zhang C, Zeng H, Guan L, Shi W, Zhang Y and Ma X (2015) Association of targeted multiplex PCR with resequencing microarray for the detection of multiple respiratory pathogens. Front. Microbiol. 6:532. doi: 10.3389/fmicb.2015.00532
Received: 13 January 2015; Accepted: 14 May 2015;
Published: 28 May 2015.
Edited by:
Rafaella Fortini Grenfell E. Queiroz, Fundação Oswaldo Cruz—Fiocruz, BrazilReviewed by:
Malgorzata Anna Mikaszewska-Sokolewicz, The Medical University of Warsaw, PolandCopyright © 2015 Shen, Zhu, Wang, Mo, Wang, Li, Zhang, Zeng, Guan, Shi, Zhang and Ma. This is an open-access article distributed under the terms of the Creative Commons Attribution License (CC BY). The use, distribution or reproduction in other forums is permitted, provided the original author(s) or licensor are credited and that the original publication in this journal is cited, in accordance with accepted academic practice. No use, distribution or reproduction is permitted which does not comply with these terms.
*Correspondence: Yong Zhang, Futian District Center for Disease Control and Prevention, Hongli West Rd. 8043, Futian District, Shenzhen, Guangdong 518040, China,MTAzMzEzMzY4MEBxcS5jb20=;
Xuejun Ma, Key Laboratory of Medical Virology, Ministry of Health, Chinese Center for Disease Control and Prevention, National Institute for Viral Disease Control and Prevention, Changbai Rd. 155, Changping District, Beijing 102206, China,bWF4akBpdmRjLmNoaW5hY2RjLmNu
†These authors have contributed equally to this work.
Disclaimer: All claims expressed in this article are solely those of the authors and do not necessarily represent those of their affiliated organizations, or those of the publisher, the editors and the reviewers. Any product that may be evaluated in this article or claim that may be made by its manufacturer is not guaranteed or endorsed by the publisher.
Research integrity at Frontiers
Learn more about the work of our research integrity team to safeguard the quality of each article we publish.