- 1Department of Medical Mycology, Vallabhbhai Patel Chest Institute, University of Delhi, Delhi, India
- 2Department of Medical Microbiology and Infectious Diseases, Canisius-Wilhelmina Hospital, Nijmegen, Netherlands
- 3Department of Medical Microbiology, Radboud University Medical Center, Nijmegen, Netherlands
Aspergillus fumigatus causes varied clinical syndromes ranging from colonization to deep infections. The mainstay of therapy of Aspergillus diseases is triazoles but several studies globally highlighted variable prevalence of triazole resistance, which hampers the management of aspergillosis. We studied the prevalence of resistance in clinical A. fumigatus isolates during 4 years in a referral Chest Hospital in Delhi, India and reviewed the scenario in Asia and the Middle East. Aspergillus species (n = 2117) were screened with selective plates for azole resistance. The isolates included 45.4% A. flavus, followed by 32.4% A. fumigatus, 15.6% Aspergillus species and 6.6% A. terreus. Azole resistance was found in only 12 (1.7%) A. fumigatus isolates. These triazole resistant A. fumigatus (TRAF) isolates were subjected to (a) calmodulin and β tubulin gene sequencing (b) in vitro antifungal susceptibility testing against triazoles using CLSI M38-A2 (c) sequencing of cyp51A gene and real-time PCR assay for detection of mutations and (d) microsatellite typing of the resistant isolates. TRAF harbored TR34/L98H mutation in 10 (83.3%) isolates with a pan-azole resistant phenotype. Among the remaining two TRAF isolates, one had G54E and the other had three non-synonymous point mutations. The majority of patients were diagnosed as invasive aspergillosis followed by allergic bronchopulmonary aspergillosis and chronic pulmonary aspergillosis. The Indian TR34/L98H isolates had a unique genotype and were distinct from the Chinese, Middle East, and European TR34/L98H strains. This resistance mechanism has been linked to the use of fungicide azoles in agricultural practices in Europe as it has been mainly reported from azole naïve patients. Reports published from Asia demonstrate the same environmental resistance mechanism in A. fumigatus isolates from two highly populated countries in Asia, i.e., China and India and also from the neighboring Middle East.
Introduction
Among the Aspergillus species, Aspergillus fumigatus is the leading etiologic agent of all forms of aspergillosis, which could be attributed to the ubiquitous presence of its thermo-tolerant spores that are refractory to adverse environmental conditions (Kwon-Chung and Sugui, 2013). A. fumigatus, in contrast to Candida albicans, has no reservoir in the immunocompetent population; thus, infections with A. fumigatus are generally environmentally acquired (Verweij et al., 2009; Chowdhary et al., 2013b). Furthermore, aspergillosis is associated with high morbidity and mortality in both immunocompetent and immunosuppressed populations primarily due to difficulties in early diagnosis or delay in recovery of the immune system (Kosmidis and Denning, 2015). Triazole antifungals, the competitive inhibitors of cyp51A, are preferred for prophylaxis and treatment of aspergillosis. However, failure of treatment with azoles and a steady increase in the occurrence of triazole resistant A. fumigatus (TRAF) isolates from environment as well as clinical settings has been reported (Denning et al., 1997; Mellado et al., 2007; Verweij et al., 2007; Rodriguez-Tudela et al., 2008; Snelders et al., 2008, 2009; Baddley et al., 2009; Howard et al., 2009; Arendrup et al., 2010; Mortensen et al., 2010, 2011; van der Linden et al., 2011, 2013; Burgel et al., 2012; Chowdhary et al., 2012a,b, 2013b, 2014a,b; Alastruey-Izquierdo et al., 2013; Badali et al., 2013; Bader et al., 2013; Escribano et al., 2013; Seyedmousavi et al., 2013; Prigitano et al., 2014; Spiess et al., 2014; Lavergne et al., 2015; Steinmann et al., 2015). The most common mechanism of triazole resistance has been linked to theTR34/L98H mutation with tandem repeat in the cyp51A promoter region combined with a single amino acid exchange of leucine 98 to histidine (Chowdhary et al., 2014c). Apparently, this mutated allele has spread throughout the A. fumigatus population and thus has been reported worldwide from patients as well as the environment (Mellado et al., 2007; Verweij et al., 2007; Rodriguez-Tudela et al., 2008; Snelders et al., 2008, 2009; Baddley et al., 2009; Howard et al., 2009; Mortensen et al., 2010, 2011; Lockhart et al., 2011; van der Linden et al., 2011; Burgel et al., 2012; Chowdhary et al., 2012a,b; Hamprecht et al., 2012; Jeurissen et al., 2012; Morio et al., 2012; Rath et al., 2012; Alastruey-Izquierdo et al., 2013; Bader et al., 2013; Escribano et al., 2013; Rocchi et al., 2014; Kidd et al., 2015; Steinmann et al., 2015). In addition several point mutations such as G54, G138, or M220 lead to disturbances in the docking of azole drugs to cyp51A protein rendering azole resistant A. fumigatus phenotype (Diaz-Guerra et al., 2003; Mann et al., 2003; Mellado et al., 2004; Chen et al., 2005; Howard et al., 2006, 2009, 2013; Rodriguez-Tudela et al., 2008; Snelders et al., 2008; Albarrag et al., 2011; van der Linden et al., 2011). These mutations have been previously reported to occur de novo due to prolonged exposure of A. fumigatus isolates to azole antifungal drugs in clinical settings (Chen et al., 2005; Howard et al., 2009; Escribano et al., 2012). However, a recent study reports presence of A. fumigatus carrying G54 point mutation in the environment of Tanzania, Romania, and India suggesting that environment may predominately be the cause in acquisition of azole resistant isolates (Sharma et al., 2015). Also, non-cyp51A mediated mutations have been increasingly recognized in the development of azole resistance and are mainly reported from Manchester, UK (Bueid et al., 2010). We conducted a prospective study for the assessment of prevalence of TRAF and the underlying cyp51A mutations in clinical isolates of Aspergillus species collected during a 4-year (2011–2014) period in a referral Chest Hospital in Delhi, India and reviewed the reports on TRAF isolates from environmental and clinical sources from Asia and the neighboring Middle East.
Materials and Methods
Fungal Isolates and Their Phenotypic Characterization
During 2011–2014 a total of 8222 clinical samples were processed for fungal culture and microscopy collected from patients of our hospital and three neighboring referral hospitals included in Table 1. The clinical specimens included sputum, endotracheal aspirates, bronchial aspirates, bronchoalveolar lavages (BAL), bronchial tissues, fine needle aspiration biopsies, lung biopsies, nasal polyps, bone marrow aspirations, pleural fluid, bronchial plugs, and cerebrospinal fluid. The study was approved by the Institute’s Ethics Committee. All Aspergillus species cultured from the specimens were preliminarily identified based on colony color and morphology of the isolates on Czapek dox agar plates incubated at 28°C for 7 days. In order to investigate azole resistance in all of the Aspergillus species, they were screened on itraconazole (ITC, 4 μg/ml) and voriconazole (VRC, 1 μg/ml) supplemented Sabouraud dextrose agar (SDA) plates. Aspergillus isolates that exhibited growth on either of the antifungal plate were confirmed as Aspergillus species by amplification and sequencing of β-tubulin and calmodulin genes.
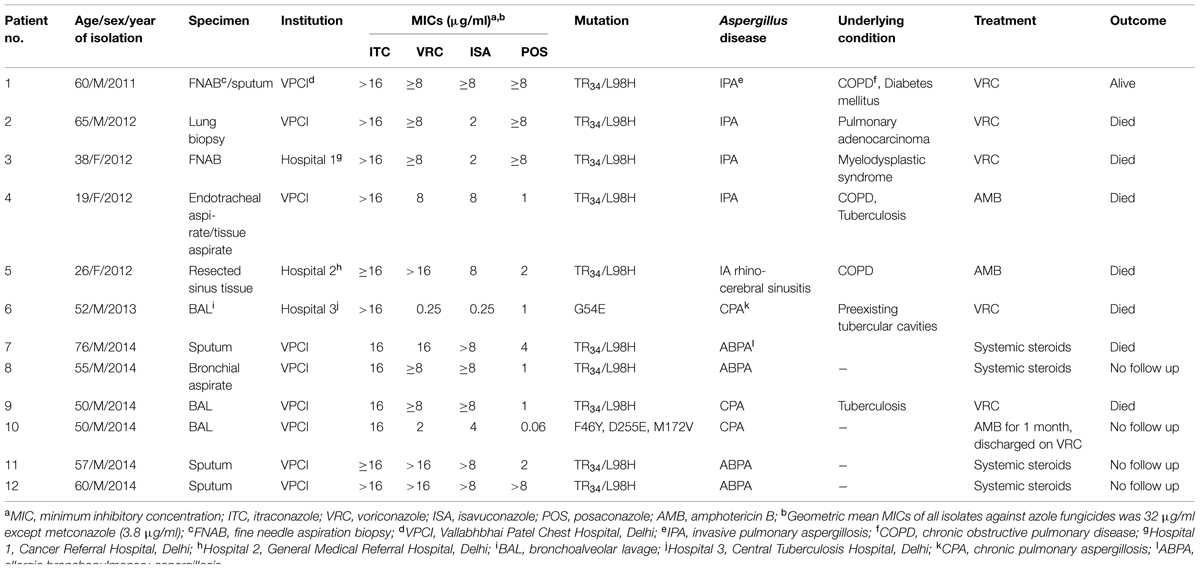
TABLE 1. Clinical characteristics of 12 patients with triazole resistant Aspergillus fumigatus (TRAF) isolates.
Antifungal Susceptibility Testing (AFST)
All resistant A. fumigatus were subjected to AFST against four standard medical triazoles, amphotericin B (AMB), echinocandins, and 10 commonly used azole fungicides using CLSI M38-A2 broth microdilution Clinical and Laboratory Standards Institute [CLSI] (2008). The drugs tested included ITC (Lee Pharma, Hyderabad, India, and Janssen Research Foundation, Beerse, Belgium), VRC (Pfizer Central Research, Sandwich, Kent, UK), isavuconazole (ISA, Basilea Pharmaceutica International AG, Basel, Switzerland), posaconazole (POS, Merck, Whitehouse Station, NJ, USA), AMB (Sigma–Aldrich, Germany), caspofungin (CAS, Merck), micafungin (MFG, Astellas Toyama Co. Ltd., Japan), and anidulafungin (AFG, Pfizer). The tested azole fungicides were bromuconazole, cyproconazole, difenoconazole, epoxiconazole, penconazole, tebuconazole, triadimefon, metconazole, hexaconazole (Rallis India, Mumbai, India), and tricyclazole (Cheminova India, Mumbai, India). The AFST results were analyzed by using epidemiological cutoff values (ECVs) proposed by Espinel-Ingroff et al. (2011a,b, 2013) ITC, 1 μg/ml; VRC, 1 μg/ml; POS, 0.5 μg/ml; ISA, 1 μg/ml, AMB, 4 μg/ml, and CAS, 0.25 μg/ml (Pfaller et al., 2011).
Mutation Analysis
To gain insight into the mechanisms responsible for azole resistance in A. fumigatus, isolates were subjected to amplification and sequencing of cyp51A gene along with the promoter region (Sharma et al., 2015). The amplified product was purified followed by sequencing on an ABI 3130XL genetic analyzer (Applied Biosystems, Foster City, CA, USA) using the BigDye terminator kit (v3.1, RR-100; Applied Biosystems; Chowdhary et al., 2013a). DNA sequences were analyzed with Sequencing Analysis software version 5.3.1 (Applied Biosystems). Consensus sequences were made using BioEdit software (version 7.0.5.3; Hall, 1999). The sequences of the resistant A. fumigatus strains were compared with the wild type susceptible reference A. fumigatus strain (Af293). The mutations in the resistant strains were further confirmed by mixed format real time PCR analysis as described previously (Klaassen et al., 2010).
Microsatellite Typing
The genotypic relationship of the Indian resistant A. fumigatus isolates with the isolates from Asia, Middle East, and Europe harboring various mutations in cyp51A gene was determined by microsatellite typing using a panel of nine short tandem repeats (STR) as described previously (de Valk et al., 2005). The amplification of three di-, tri-, or tetranucleotide repeat markers was carried out using three multiplex PCRs, namely, M2, M3, and M4, respectively, (de Valk et al., 2005). Repeat numbers in each marker was assigned using A. fumigatus Af293 as reference. The STR data was imported to BioNumerics v5.1 software (Applied Maths, Sint-Martens-Latem, Belgium) for phylogenetic analysis. The dendrogram based on unweighted pair-group method with arithmetic mean (UPGMA) clustering using the Pearson correlation coefficient was generated. A total of 49 A. fumigatus isolates from Asia, Middle East, Europe, and Australia were included as controls to evaluate the genetic differences with the A. fumigatus isolates from India. The isolates from outside India were from following countries: China (clinical resistant, n = 8), Iran (environmental resistant, n = 5; environmental susceptible, n = 4), Kuwait (clinical resistant, n = 2; clinical susceptible, n = 2; environmental resistant, n = 8; environmental susceptible, n = 2), France (clinical resistant, n = 1), Germany (clinical resistant, n = 7; clinical susceptible, n = 1), the Netherlands (clinical resistant, n = 2; environmental resistant, n = 3), and Australia (clinical resistant, n = 2; clinical susceptible, n = 2). Also, Indian isolates (environmental resistant n = 3; environmental susceptible n = 6 and clinical susceptible n = 3) collected during the previous study on resistant A. fumigatus were included for comparison with the present clinical TRAF isolates from India (Chowdhary et al., 2012b).
Results
Overall, during a 4-year survey period, 25.7% of clinical samples harbored Aspergillus species (n = 2117). Out of these 2117 isolates, 45.4% (n = 963) were A. flavus, followed by 32.4% (n = 685) A. fumigatus, 15.6% (n = 329) Aspergillus species and 6.6% (n = 140) A. terreus (Kathuria et al., 2015). Barring 12 isolates of A. fumigatus none of the other Aspergillus species grew on SDA plates supplemented with ITC and/or VRC. Of these, 11 A. fumigatus grew on both ITC and VRC supplemented SDA plates while a solitary isolate grew only on ITC plate. All the 12 resistant isolates were identified as A. fumigatus sensu stricto by β-tubulin and calmodulin genes sequencing.
Of the 12 resistant A. fumigatus, 11 showed a pan-azole resistant phenotype exhibiting high MIC of all the triazoles, namely, ITC [geometric mean (GM) MIC, 16 μg/ml], VRC (GM MIC, 8 μg/ml), ISA (GM MIC, 6.34 μg/ml), and POS (GM MIC, 2.82 μg/ml). In contrast, a solitary A. fumigatus isolate exhibited high MIC of only ITC (>16 μg/ml) and POS (1 μg/ml; Table 1). However, AMB (GM MIC, 0.4 μg/ml) and three echinocandins, namely, CAS, MFG, and AFG were active against all the resistant A. fumigatus isolates with GM MICs of 0.13 μg/ml, 0.017 μg/ml, and 0.02 μg/ml, respectively. Further all TRAF uniformly revealed cross-resistance to all the azole fungicides (MICs,>32 μg/ml) tested excepting metconazole (MICs, 3.80 μg/ml).
Overall, 1.75% (12/685) of the clinical A. fumigatus isolates were resistant. The major resistance mechanism observed among the eleven pan-azole resistant phenotype was TR34/L98H mutation (n = 10) and a solitary isolate exhibited three non-synonymous point mutations, namely, F46Y, D255E, and M172V. Another single point mutation, G54E, was observed in a solitary isolate that had high MICs to both ITC and POS.
The STR typing data revealed a single microsatellite complex (MC) among all the TR34/L98H genotypes whereas solitary isolate each of G54E and non-synonymous mutant of A. fumigatus represented two distinct genotypes (Figure 1). The TR34/L98H MC was homogenous and shared all the nine loci except one isolate that differed at two loci (4A and 4C). Further to determine the genetic relatedness among the present Indian TR34/L98H clinical isolates (n = 10) comparison with Indian TR34/L98H environmental isolates (n = 3) collected from the previous study was done (Chowdhary et al., 2012b). Both the environmental and clinical A. fumigatus isolates had an identical STR pattern. The genotypes of all Indian TR34/L98H isolates were distinct from the TR34/L98H strains of Chinese, Kuwait, Iran, and European isolates (Lockhart et al., 2011; Badali et al., 2013; Ahmad et al., 2014, 2015; Steinmann et al., 2015). However, environmental A. fumigatus isolates from Kuwait (n = 4) and Iran (n = 5) were more closely related with Indian TRAF isolates with similarity observed at 5–6 of the nine loci studied. Although the Chinese clinical TRAF isolates (n = 8) also formed a separate cluster but unlike Indian isolates they were markedly heterogeneous exhibiting variable STR patterns. The STR typing data of the clinical Kuwait TRAF isolates (n = 6) revealed homogeneity among them, however, when compared to Indian TRAF clinical isolates they showed differences at 6–7 loci (Ahmad et al., 2015).
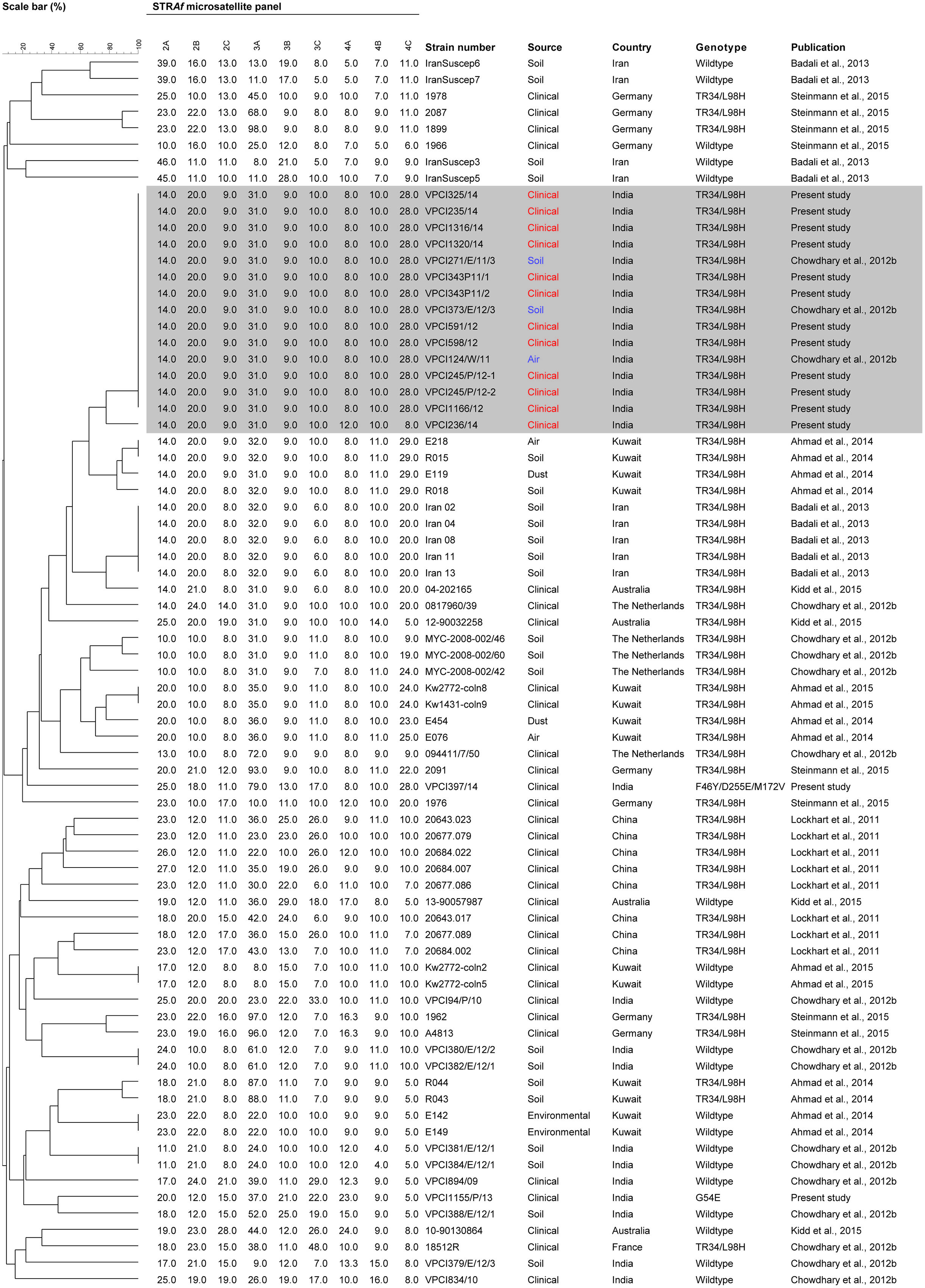
FIGURE 1. Genotypicrelationship of Indian Aspergillus fumigatus isolates (clinical resistant, n = 14; clinical susceptible, n = 3; environmental resistant, n = 3; environmental susceptible, n = 6) with isolates from Asia including China (clinical resistant, n = 8), Middle East including Iran (environmental resistant, n = 5; environmental susceptible, n = 4), and Kuwait (clinical resistant, n = 2; clinical susceptible, n = 2; environmental resistant, n = 8; environmental susceptible, n = 2), and Europe including France (clinical resistant, n = 1), Germany (clinical resistant, n = 7; clinical susceptible, n = 1), the Netherlands (clinical resistant, n = 2; environmental susceptible, n = 3), and Australia (clinical resistant, n = 2; clinical susceptible, n = 2). The dendrogram is based on a categorical analysis of nine microsatellite markers in combination with Unweighted Pair Group Method with arithmetic mean clustering. The scale bar indicates the percentage identity. The isolates VPCI 343/P/11/1, VPCI 343/P/11/2, and VPCI 245/P/12-1, VPCI 245/P/12-2 were serial isolates from two individual patients, respectively.
The detailed clinical record of all patients whose clinical samples yielded TRAF was retrieved from the database. The majority of patients were diagnosed as invasive aspergillosis (IA, n = 5) followed by allergic bronchopulmonary aspergillosis (ABPA, n = 4) and chronic pulmonary aspergillosis (CPA, n = 3). The most common underlying condition among the IA patients was chronic obstructive pulmonary disease (COPD) in three patients and myelodysplastic syndrome and pulmonary adenocarcinoma in the remaining two patients, respectively. IA was defined as probable or proven according to the criteria of the European Organization for Research and Treatment of Cancer/Mycoses Study Group (Ascioglu et al., 2002; De Pauw et al., 2008). All cases of IA in the present study were proven cases and FNAB/lung biopsy or resected sinus tissue was positive for fungus and yielded A. fumigatus in culture. IPA (n = 4) was the most common manifestation and a solitary case had invasive rhino-cerebral sinusitis. All COPD patients who finally developed IA during the course of 5–10 years of illness had been admitted to the medical units or ICU repeatedly (4–9 episodes per year) for respiratory symptoms with severe airflow obstruction. All these patients were on systemic and inhaled steroids for a long period ranging from 10 to 12 years. Associated co-morbidities like diabetes mellitus and tuberculosis were observed in two of the three COPD patients. Allergic aspergillosis manifesting as ABPA was diagnosed in four patients by a combination of clinical, mycoserologic, and radiological features (Agarwal et al., 2013). None of the ABPA patients diagnosed in the present study were on azoles when their culture grew TRAF. The third clinical entity was CPA, which was diagnosed by chronic duration of clinical symptoms (>3 months), progressive pulmonary lesions with or without cavitation, precipitating antibodies to A. fumigatus in serum, and mycological evidence of fungal presence. Further, none of the patients harboring identical TR34/L98H genotype (n = 10) admitted to the Chest hospital (n = 8) in the present study had an overlapping time frame during their admission. Also, two patients harboring the same genotype were from two different hospitals in Delhi (Table 1).
Overall, the mortality was highest in IA (four of five patients) and CPA (two of three patients) and a solitary case of ABPA also had a fatal outcome. Among IA patients, VRC (6 mg/kg intravenous 12 hourly followed by 4 mg/kg and switched to oral 200 mg twice a day when clinically stable) was administered in three patients and two were started on AmB deoxycholate (1 mg/kg/day). All CPA patients were given VRC for 7–10 days before the antifungal susceptibility results were conveyed. The two CPA patients had severe lung involvement and the disease was fatal in both of them within 1 week. However, in one case therapy was switched to amphotericin B for one month followed by repeat BAL culture that yielded negative results for Aspergillus and the patient was discharged on oral VRC on follow up. However, patient failed to report for the follow up visits. The patients with ABPA in the present study were managed symptomatically and systemic steroids were the mainstay of therapy.
Discussion
In the present study, we examined azole susceptibility and resistant mechanisms among A. fumigatus isolates from patients with bronchopulmonary aspergillosis in a referral Chest Institute, which caters to a vast population of Delhi and adjoining states of Uttar Pradesh, Haryana and also to far remote regions of India. The rate of azole resistance in A. fumigatus isolates in our study was 1.75% (12/685) during a 4-year period, which is remarkably low compared to the high prevalence in Europe including UK (6.6–27.8%), the Netherlands (3.1–4.6%), and Germany (3.2%; Snelders et al., 2008; Howard et al., 2009; Bueid et al., 2010; van der Linden et al., 2011; Bader et al., 2013). In contrast in Spain, a lower prevalence rate (2.5%) of azole resistance in A. fumigatus complex has been reported suggesting that azole resistance has not yet uniformly spread in Europe (Escribano et al., 2013). TR34/L98H was the predominant resistance mechanism in 83.3% of the Indian TRAF isolates followed by G54E (n = 1) and a non-synonymous mutation (n = 1).
Short tandem repeats typing of the TRAF isolates demonstrated a single cluster, with a homogenous population. It is also worth mentioning here that the patients’ positive for TRAF isolates in the present study were both from Delhi, and other states namely West Bengal, Haryana, and Uttar Pradesh. These states previously have been found to harbor A. fumigatus isolates carrying TR34/L98H in soil samples (Chowdhary et al., 2012b). Notably the genotype detected in clinical isolates in this study was identical to the TR34/L98H genotype reported earlier from environmental samples from India and shared the identical nine loci, suggesting environmental origin of this major resistance mechanism (Chowdhary et al., 2012b). This is the first study outside the Netherlands revealing the possibility of acquisition of clinical isolates linked with the environment. However, in contrast to the heterogeneity observed in environmental and clinical isolates in the Netherlands (Klaassen et al., 2012), the Indian TRAF in the present study had a homogenous population which was recently also confirmed with whole genome sequencing (Abdolrasoulia et al., 2015).
Table 2 summarizes the reports of TRAF isolation harboring mutations in the cyp51A gene from clinical and environmental sources in Asia and the neighboring Middle East. The initial reports describing azole resistance in clinical A. fumigatus isolates originated from Europe in the late 1990s followed by systematical investigations in several European countries which, reported prevalence, mechanism, and genomic aspects of TRAF (Mellado et al., 2007; Verweij et al., 2007; Rodriguez-Tudela et al., 2008; Howard et al., 2009; Mortensen et al., 2010; Burgel et al., 2012; Morio et al., 2012; Bader et al., 2013; Astvad et al., 2014; Fischer et al., 2014; Spiess et al., 2014; van der Linden et al., 2015). However, the first comprehensive report on the occurrence of TRAF isolates in Asia originated from China during 2008–2009 from the ARTEMIS global sentinel surveillance program demonstrating TR34/L98H resistance mechanism in 27.5% (8/29) A. fumigatus isolates (Lockhart et al., 2011; Table 2). In contrast, more recently few studies from Japan, described TRAF in clinical isolates but interestingly none of them exhibited TR34/L98H resistance mechanism, instead several SNPs and novel mutations, F332K and P216L were reported (Asano et al., 2011; Hagiwara et al., 2014). The single center study from Japan reported 5.2% (1/19) TRAF isolates harboring only G54E/R/W and I266N mutation (Tashiro et al., 2012a,b). Notably, a recent study on environmental sampling of air from a pumpkin farm sprayed with azole fungicides, at Nihon University, Japan was carried out by using an outdated and less efficacious settle plate method and reported no azole resistance in 50 A. fumigatus isolates (Kano et al., 2015). The lack of isolation of TRAF could be attributed to not adopting a highly efficacious sampling method such as using an air sampler and to the low number of isolates tested for resistance resulting in false rates of TRAF prevalence in the environment. The possibility of missing resistance was recently also highlighted in the SCARE study (van der Linden et al., 2015). Further, planned studies with wider coverage areas and different sources such as soil samples, air, wooden debris etc., should be undertaken for finding out the true prevalence of TRAF isolates. In India, a comprehensive wider environmental survey covering north, south, and eastern India analyzed 486 environmental samples comprising soil from flowerbeds of nurseries, surrounding parks of hospitals, cotton trees, tea gardens, paddy fields, soil containing bird excreta, and decayed wood of tree trunks revealed 7% TRAF isolates in the environment carrying TR34/L98H mutation (Chowdhary et al., 2012b). Also, a recently described VRC resistant TR46/Y121F/T289A mechanism was observed in environmental A. fumigatus isolates from agricultural fields in India (Chowdhary et al., 2014a). Barring the solitary environmental report on TR46/Y121F/T289A mechanism from India none of the clinical or environmental samples from Asia has yet documented the occurrence of A. fumigatus isolates carrying this mechanism so far. However, considering the presence of this new resistant mechanism in the environment of India it may be anticipated that in future it may spread to the neighboring Asian countries. In addition to the above-discussed reports on triazole resistance in Asia, the neighboring Middle East countries, Iran, and Kuwait had reported 12.2 and 7% TRAF carrying TR34/L98H resistance in the environment, respectively, (Badali et al., 2013; Ahmad et al., 2014). A similar range of resistance prevalence of 3.2 and 12.5% TR34/L98H A. fumigatus isolates was observed in clinical samples from CPA and ABPA patients from Iran and Kuwait, respectively, (Seyedmousavi et al., 2013; Ahmad et al., 2015). Based on the fact that the Indian TRAF isolates exhibited a distinct cluster away from the Chinese TR34/L98H isolates, it may be suggested that resistance among the A. fumigatus strains across Asia has evolved from separate strains and not from a common resistant ancestor, which may have spread worldwide.
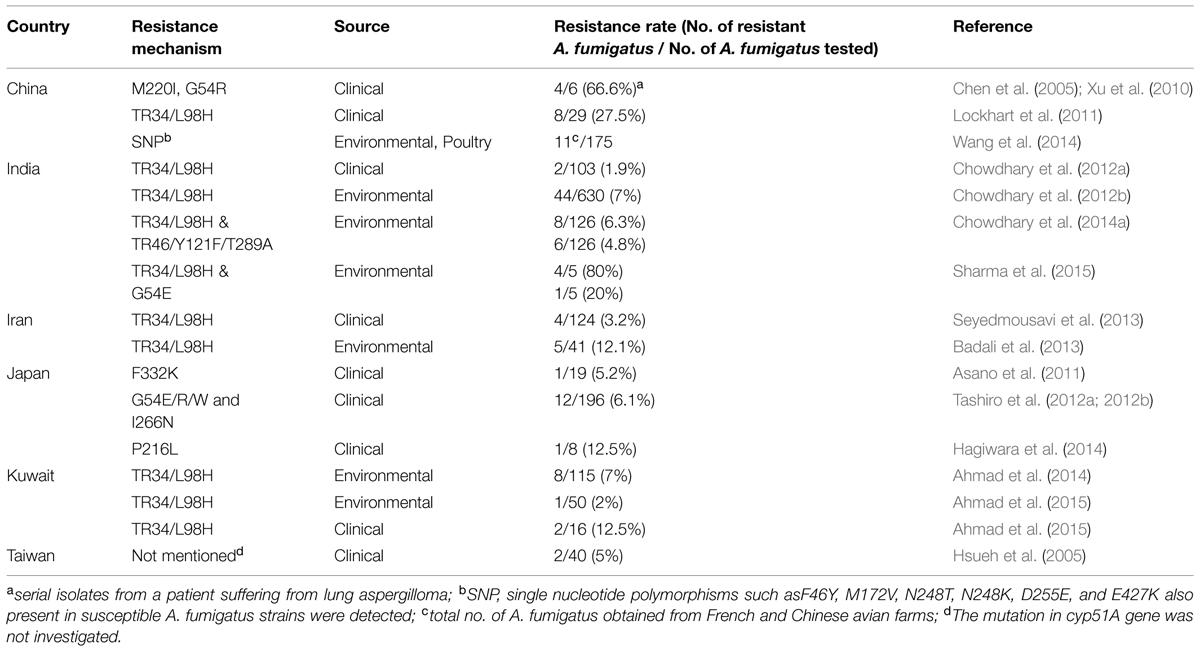
TABLE 2. Distribution of TRAF in clinical and environmental samples in Asia and Middle East harboring mutations in the Cyp51A gene.
It is also pertinent to mention that the high rates of triazole resistance in Europe as compared to Asia could be due to environmental factors or more so by frequent use of azoles in clinical settings and in the environment. Azole fungicides are extensively used in agriculture for crop protection to control mildews and rusts of grains, fruits, vegetables, and also for preservation of materials like wood etc. Geographically, Europe is the dominant market where fungicide usage is significantly high in production of fruits and vegetables along with wheat and vineyard (Stensvold et al., 2012). Thus, the reports of high resistance rates in environmental A. fumigatus isolates from the Netherlands, Belgium, UK, and Germany can be attributed to higher fungicide usage. Similarly, the absence or very low prevalence of triazole resistance in A. fumigatus in the USA could possibly be due to the low usage of fungicides in the USA as compared to Europe1. Notably, triazole resistance was screened among 1026 A. fumigatus isolates from 22 states of USA and none had TR34/L98H mutation (Pham et al., 2014). In India, the crop protection chemicals account for ∼2% of the total chemicals market and currently India is the second largest manufacturer of pesticides in Asia, second only to Japan2. Among different classes of pesticides used in India the percent share of insecticides (60%) is highest followed by fungicides (19%), herbicides (16%), biopesticides (3%), and others (3%). Per capita consumption of crop protection products in India is 0.6 kg/ha compared to 16 kg/ha in Taiwan, 13 kg/ha in China, and 12 kg/ha in Japan2. Finally, the environmental resistance mechanism in A. fumigatus isolates have been reported from two highly populated countries in Asia, i.e., China and India. Further, the environment of agricultural fields in India has been harboring variable cyp51A mediated resistant patterns in A. fumigatus isolates, which are continuously exposed to agricultural azole fungicides. It is emphasized that in depth analysis of azole resistance in A. fumigatus isolates in both clinical laboratories and environmental settings is required to prevent its spread and emergence in neighboring Asian countries.
Acknowledgments
This work was supported in part by a research grant from University Grants Commission Research Fellowship, India (F.2-15/2003 SA-I) and Indian Council of Medical Research (5/3/3/26/2010-ECD-I), Government of India, New Delhi, India. We are grateful to Paul E. Verweij (Radboud University Medical Centre, Nijmegen, the Netherlands) for providing several fungicides (bromuconazole, cyproconazole, difenoconazole, epoxiconazole, penconazole, tebuconazole, triadimefon, metconazole). We acknowledge Rallis India, India, and Cheminova India, for kindly providing us hexaconazole and tricyclazole fungicides.
Conflict of Interest Statement
Jacques F. Meis received grants from Astellas, Basilea, and Merck. He has been a consultant to Astellas, Basilea, and Merck and received speaker’s fees fromMerck and Gilead. All other authors: no potential conflicts of interest. The authors alone are responsible for the content and writing of the paper.
Footnotes
- ^ http://ec.europa.eu/food/fs/sc/ssc/out278_en.pdf
- ^ http://www.ficci.com/spdocument/20292/petro1.pdf
References
Abdolrasoulia, A., Rhodes, J., Beale, M. A., Hagen, F., Rogers, T. R., Chowdhary, A., et al. (2015). Genomic context of azole-resistance mutations in Aspergillus fumigatus using whole-genome sequencing. mBio 6, (in press).
Agarwal, R., Chakrabarti, A., Shah, A., Gupta, D., Meis, J. F., Guleria, R., et al. (2013). Allergic bronchopulmonary aspergillosis: review of literature and proposal of new diagnostic and classification criteria. Clin. Exp. Allergy 43, 850–873. doi: 10.1111/cea.12141
PubMed Abstract | Full Text | CrossRef Full Text | Google Scholar
Ahmad, S., Joseph, L., Hagen, F., Meis, J. F., and Khan, Z. (2015). Concomitant occurrence of itraconazole-resistant and -susceptible strains of Aspergillus fumigatus in routine cultures. J. Antimicrob. Chemother. 70, 412–415. doi: 10.1093/jac/dku410
PubMed Abstract | Full Text | CrossRef Full Text | Google Scholar
Ahmad, S., Khan, Z., Hagen, F., and Meis, J. F. (2014). Occurrence of triazole-resistant Aspergillus fumigatus with TR34/L98H mutations in outdoor and hospital environment in Kuwait. Environ. Res. 133, 20–26. doi: 10.1016/j.envres.2014.05.009
PubMed Abstract | Full Text | CrossRef Full Text | Google Scholar
Alastruey-Izquierdo, A., Mellado, E., Peláez, T., Pemán, J., Zapico, S., Alvarez, M., et al. (2013). Population-based survey of filamentous fungi and antifungal resistance in Spain (FILPOP Study). Antimicrob. Agents Chemother. 57, 3380–3387. doi: 10.1128/AAC.00383-13
PubMed Abstract | Full Text | CrossRef Full Text | Google Scholar
Albarrag, A. M., Anderson, M. J., Howard, S. J., Robson, G. D., Warn, P. A., Sanglard, D., et al. (2011). Interrogation of related clinical pan-azole-resistant Aspergillus fumigatus strains: G138C, Y431C, and G434C single nucleotide polymorphisms in cyp51A, upregulation of cyp51A, and integration and activation of transposon Atf1 in the cyp51A promoter. Antimicrob. Agents Chemother. 55, 5113–5121. doi: 10.1128/AAC.00517-11
PubMed Abstract | Full Text | CrossRef Full Text | Google Scholar
Arendrup, M. C., Mavridou, E., Mortensen, K. L., Snelders, E., Frimodt-Møller, N., Khan, H., et al. (2010). Development of azole resistance in Aspergillus fumigatus during azole therapy associated with change in virulence. PLoS ONE 5:e10080. doi: 10.1371/journal.pone.0010080
PubMed Abstract | Full Text | CrossRef Full Text | Google Scholar
Asano, M., Kano, R., Makimura, K., Hasegawa, A., and Kamata, H. (2011). Molecular typing and in-vitro activity of azoles against clinical isolates of Aspergillus fumigatus and A. niger in Japan. J. Infect. Chemother. 17, 483–486. doi: 10.1007/s10156-010-0202-1
PubMed Abstract | Full Text | CrossRef Full Text | Google Scholar
Ascioglu, S., Rex, J. H., de Pauw, B., Bennett, J. E., Bille, J., Crokaert, F., et al. (2002). Defining opportunistic invasive fungal infections in immunocompromised patients with cancer and hematopoietic stem cell transplants: an international consensus. Clin. Infect. Dis. 34, 7–14. doi: 10.1086/323335
PubMed Abstract | Full Text | CrossRef Full Text | Google Scholar
Astvad, K. M., Jensen, R. H., Hassan, T. M., Mathiasen, E. G., Thomsen, G. M., Pedersen, U. G., et al. (2014). First detection of TR46/Y121F/T289A and TR34/L98H alterations in Aspergillus fumigatus isolates from azole-naive patients in Denmark despite negative findings in the environment. Antimicrob. Agents Chemother. 58, 5096–5101. doi: 10.1128/AAC.02855-14
PubMed Abstract | Full Text | CrossRef Full Text | Google Scholar
Badali, H., Vaezi, A., Haghani, I., Yazdanparast, S. A., Hedayati, M. T., Mousavi, B., et al. (2013). Environmental study of azole-resistant Aspergillus fumigatus with TR34/L98H mutations in the cyp51A gene in Iran. Mycoses 56, 659–663. doi: 10.1111/myc.12089
PubMed Abstract | Full Text | CrossRef Full Text | Google Scholar
Baddley, J. W., Marr, K. A., Andes, D. R., Walsh, T. J., Kauffman, C. A., Kontoyiannis, D. P., et al. (2009). Patterns of susceptibility of Aspergillus isolates recovered from patients enrolled in the Transplant-Associated Infection Surveillance Network. J. Clin. Microbiol. 47, 3271–3275. doi: 10.1128/JCM.00854-09
PubMed Abstract | Full Text | CrossRef Full Text | Google Scholar
Bader, O., Weig, M., Reichard, U., Lugert, R., Kuhns, M., Christner, M., et al. (2013). cyp51A-based mechanisms of Aspergillus fumigatus azole drug resistance present in clinical samples from Germany. Antimicrob. Agents Chemother. 57, 3513–3517. doi: 10.1128/AAC.00167-13
PubMed Abstract | Full Text | CrossRef Full Text | Google Scholar
Bueid, A., Howard, S. J., Moore, C. B., Richardson, M. D., Harrison, E., Bowyer, P., et al. (2010). Azole antifungal resistance in Aspergillus fumigatus: 2008 and 2009. J. Antimicrob. Chemother. 65, 2116–2118. doi: 10.1093/jac/dkq279
PubMed Abstract | Full Text | CrossRef Full Text | Google Scholar
Burgel, P. R., Baixench, M. T., Amsellem, M., Audureau, E., Chapron, J., Kanaan, R., et al. (2012). High prevalence of azole-resistant Aspergillus fumigatus in adults with cystic fibrosis exposed to itraconazole. Antimicrob. Agents Chemother. 56, 869–874. doi: 10.1128/AAC.05077-11
PubMed Abstract | Full Text | CrossRef Full Text | Google Scholar
Chen, J., Li, H., Li, R., Bu, D., and Wan, Z. (2005). Mutations in the cyp51A gene and susceptibility to itraconazole in Aspergillus fumigatus serially isolated from a patient with lung aspergilloma. J. Antimicrob. Chemother. 55, 31–37. doi: 10.1093/jac/dkh507
PubMed Abstract | Full Text | CrossRef Full Text | Google Scholar
Chowdhary, A., Agarwal, K., Kathuria, S., Singh, P. K., Roy, P., Gaur, S. N., et al. (2013a). Clinical significance of filamentous basidiomycetes illustrated by isolates of the novel opportunist Ceriporia lacerata from the human respiratory tract. J. Clin. Microbiol. 51, 585–590. doi: 10.1128/JCM.02943-12
PubMed Abstract | Full Text | CrossRef Full Text | Google Scholar
Chowdhary, A., Kathuria, S., Xu, J., and Meis, J. F. (2013b). Emergence of azole-resistant Aspergillus fumigatus strains due to agricultural azole use creates an increasing threat to human health. PLoS Pathog. 9:e1003633. doi: 10.1371/journal.ppat.1003633
PubMed Abstract | Full Text | CrossRef Full Text | Google Scholar
Chowdhary, A., Kathuria, S., Randhawa, H. S., Gaur, S. N., Klaassen, C. H., and Meis, J. F. (2012a). Isolation of multiple-triazole-resistant Aspergillus fumigatus strains carrying the TR/L98H mutations in the cyp51A gene in India. J. Antimicrob. Chemother. 67, 362–366. doi: 10.1093/jac/dkr443
PubMed Abstract | Full Text | CrossRef Full Text | Google Scholar
Chowdhary, A., Kathuria, S., Xu, J., Sharma, C., Sundar, G., Singh, P. K., et al. (2012b). Clonal expansion and emergence of environmental multiple-triazole-resistant Aspergillus fumigatus strains carrying the TR34/L98H mutations in the cyp51A gene in India. PLoS ONE 7:e52871. doi: 10.1371/journal.pone.0052871
PubMed Abstract | Full Text | CrossRef Full Text | Google Scholar
Chowdhary, A., Sharma, C., Kathuria, S., Hagen, F., and Meis, J. F. (2014a). Azole-resistant Aspergillus fumigatus with the environmental TR46/Y121F/T289A mutation in India. J. Antimicrob. Chemother. 69, 555–557. doi: 10.1093/jac/dkt397
PubMed Abstract | Full Text | CrossRef Full Text | Google Scholar
Chowdhary, A., Sharma, C., van den Boom, M., Yntema, J. B., Hagen, F., Verweij, P. E., et al. (2014b). Multi-azole-resistant Aspergillus fumigatus in the environment in Tanzania. J. Antimicrob. Chemother. 69, 2979–2983. doi: 10.1093/jac/dku259
PubMed Abstract | Full Text | CrossRef Full Text | Google Scholar
Chowdhary, A., Sharma, C., Hagen, F., and Meis, J. F. (2014c). Exploring azole antifungal drug resistance in Aspergillus fumigatus with special reference to resistance mechanisms. Future Microbiol. 9, 697–711. doi: 10.2217/fmb.14.27
PubMed Abstract | Full Text | CrossRef Full Text | Google Scholar
Clinical and laboratory Standards Institute [CLSI]. (2008). Reference Method for Broth Dilution. (Antifungal) Susceptibility Testing of Filamentous Fungi; Approved Standard-Second Edition, M38- A2. Wayne, PA: CLSI.
Denning, D. W., Venkateswarlu, K., Oakley, K. L., Anderson, M. J., Manning, N. J., Stevens, D. A., et al. (1997). Itraconazole resistance in Aspergillus fumigatus. Antimicrob. Agents Chemother. 41, 1364–1368.
De Pauw, B., Walsh, T. J., Donnelly, J. P., Stevens, D. A., Edwards, J. E., Calandra, T., et al. (2008). Revised definitions of invasive fungal disease from the European Organization for Research and Treatment of Cancer/Invasive Fungal Infections Cooperative Group and the National Institute of Allergy and Infectious Diseases Mycoses Study Group (EORTC/MSG) Consensus Group. Clin. Infect. Dis. 46, 1813–1821. doi: 10.1086/588660
PubMed Abstract | Full Text | CrossRef Full Text | Google Scholar
de Valk, H. A., Meis, J. F., Curfs, I. M., Muehlethaler, K., Mouton, J. W., and Klaassen, C. H. (2005). Use of a novel panel of nine short tandem repeats for exact and high-resolution fingerprinting of Aspergillus fumigatus isolates. J. Clin. Microbiol. 43, 4112–4120. doi: 10.1128/JCM.43.8.4112-4120.2005
PubMed Abstract | Full Text | CrossRef Full Text | Google Scholar
Diaz-Guerra, T. M., Mellado, E., Cuenca-Estrella, M., and Rodriguez-Tudela, J. L. (2003). A point mutation in the 14-alpha-sterol demethylase gene cyp51A contributes to itraconazole resistance in Aspergillus fumigatus. Antimicrob. Agents Chemother. 47, 1120–1124. doi: 10.1128/AAC.47.3.1120-1124.2003
Escribano, P., Peláez, T., Muñoz, P., Bouza, E., and Guinea, J. (2013). Is azole resistance in Aspergillus fumigatus a problem in Spain? Antimicrob. Agents Chemother. 57, 2815–2820. doi: 10.1128/AAC.02487-12
PubMed Abstract | Full Text | CrossRef Full Text | Google Scholar
Escribano, P., Recio, S., Peláez, T., González-Rivera, M., Bouza, E., and Guinea, J. (2012). In vitro acquisition of secondary azole resistance in Aspergillus fumigatus isolates after prolonged exposure to itraconazole: presence of heteroresistant populations. Antimicrob. Agents Chemother. 56, 174–178. doi: 10.1128/AAC.00301-11
PubMed Abstract | Full Text | CrossRef Full Text | Google Scholar
Espinel-Ingroff, A., Chowdhary, A., Gonzalez, G. M., Lass-Flörl, C., Martin-Mazuelos, E., Meis, J. F., et al. (2013). Multicenter study of isavuconazole MIC distributions and epidemiological cutoff values for Aspergillus spp. for the CLSI M38-A2 broth microdilution method. Antimicrob. Agents Chemother. 57, 3823–3828. doi: 10.1128/AAC.00636-13
PubMed Abstract | Full Text | CrossRef Full Text | Google Scholar
Espinel-Ingroff, A., Fothergill, A., Fuller, J., Johnson, E., Pelaez, T., and Turnidge, J. (2011a). Wild-type MIC distributions and epidemiological cutoff values for caspofungin and Aspergillus spp. for the CLSI broth microdilution method (M38-A2 document). Antimicrob. Agents Chemother. 55, 2855–2859. doi: 10.1128/AAC.01730-10
PubMed Abstract | Full Text | CrossRef Full Text | Google Scholar
Espinel-Ingroff, A., Cuenca-Estrella, M., Fothergill, A., Fuller, J., Ghannoum, M., Johnson, E., et al. (2011b). Wild-type MIC distributions and epidemiological cutoff values for amphotericin B and Aspergillus spp. for the CLSI broth microdilution method (M38-A2 document). Antimicrob. Agents Chemother. 55, 5150–5154. doi: 10.1128/AAC.00686-11
PubMed Abstract | Full Text | CrossRef Full Text | Google Scholar
Fischer, J., van Koningsbruggen-Rietschel, S., Rietschel, E., Vehreschild, M. J., Wisplinghoff, H., Krönke, M., et al. (2014). Prevalence and molecular characterization of azole resistance in Aspergillus spp. isolates from German cystic fibrosis patients. J. Antimicrob. Chemother. 69, 1533–1536. doi: 10.1093/jac/dku009
PubMed Abstract | Full Text | CrossRef Full Text | Google Scholar
Hagiwara, D., Takahashi, H., Watanabe, A., Takahashi-Nakaguchi, A., Kawamoto, S., Kamei, K., et al. (2014). Whole-genome comparison of Aspergillus fumigatus strains serially isolated from patients with aspergillosis. J. Clin. Microbiol. 52, 4202–4209. doi: 10.1128/JCM.01105-14
PubMed Abstract | Full Text | CrossRef Full Text | Google Scholar
Hall, T. A. (1999). BioEdit: a user-friendly biological sequence alignment editor and analysisprogram for Windows 95/98/NT. Nucleic Acids. Symp. Ser. 41, 95–98. doi: 10.14601/Phytopathol_Mediterr-14998ů1.29
Hamprecht, A., Buchheidt, D., Vehreschild, J. J., Cornely, O. A., Spiess, B., Plum, G., et al. (2012). Azole-resistant invasive aspergillosis in a patient with acute myeloid leukaemia in Germany. Euro. Surveill. 17, 20262.
Howard, S. J., Cerar, D., Anderson, M. J., Albarrag, A., Fisher, M. C., Pasqualotto, A. C., et al. (2009). Frequency and evolution of azole resistance in Aspergillus fumigatus associated with treatment failure. Emerg. Infect. Dis. 15, 1068–1076. doi: 10.3201/eid1507.090043
PubMed Abstract | Full Text | CrossRef Full Text | Google Scholar
Howard, S. J., Lass-Flörl, C., Cuenca-Estrella, M., Gomez-Lopez, A., and Arendrup, M. C. (2013). Determination of isavuconazole susceptibility of Aspergillus and Candida species by the EUCAST method. Antimicrob. Agents Chemother. 57, 5426–5431. doi: 10.1128/AAC.01111-13
PubMed Abstract | Full Text | CrossRef Full Text | Google Scholar
Howard, S. J., Webster, I., Moore, C. B., Gardiner, R. E., Park, S., Perlin, D. S., et al. (2006). Multi-azole resistance in Aspergillus fumigatus. Int. J. Antimicrob. Agents 28, 450–453. doi: 10.1016/j.ijantimicag.2006.08.017
PubMed Abstract | Full Text | CrossRef Full Text | Google Scholar
Hsueh, P. R., Lau, Y. J., Chuang, Y. C., Wan, J. H., Huang, W. K., and Shyr, J. M. (2005). Antifungal susceptibilities of clinical isolates of Candida species, Cryptococcus neoformans, and Aspergillus species from Taiwan: surveillance of multicenter antimicrobial resistance in Taiwan program data from 2003. Antimicrob. Agents Chemother. 49, 512–517. doi: 10.1128/AAC.49.2.512-517.2005
PubMed Abstract | Full Text | CrossRef Full Text | Google Scholar
Jeurissen, A., Cooreman, S., Van Kerckhoven, W., Van Leemput, J., Vanhove, P., Lagrou, K., et al. (2012). Invasive pulmonary aspergillosis due to a multi-azole resistant Aspergillus fumigatus. Acta Clin. Belg. 67, 46–48.
Kano, R., Kohata, E., Tateishi, A., Murayama, S. Y., Hirose, D., Shibata, Y., et al. (2015). Does farm fungicide use induce azole resistance in Aspergillus fumigatus? Med. Mycol. 53, 174–177. doi: 10.1093/mmy/myu076
PubMed Abstract | Full Text | CrossRef Full Text | Google Scholar
Kathuria, S., Sharma, C., Singh, P. K., Agarwal, P., Agarwal, K., Hagen, F., et al. (2015). Molecular epidemiology and in-vitro antifungal susceptibility of Aspergillus terreus species complex isolates in Delhi, India: evidence of genetic diversity by amplified fragment length polymorphism and microsatellite typing. PLoS ONE 10:e0118997. doi: 10.1371/journal.pone.0118997
PubMed Abstract | Full Text | CrossRef Full Text | Google Scholar
Kidd, S. E., Goeman, E., Meis, J. F., Slavin, M. A., and Verweij, P. E. (2015). Multi-triazole-resistant Aspergillus fumigatus infections in Australia. Mycoses 58, 350–355. doi: 10.1111/myc.12324
PubMed Abstract | Full Text | CrossRef Full Text | Google Scholar
Klaassen, C. H., de Valk, H. A., Curfs-Breuker, I. M., and Meis, J. F. (2010). Novel mixed-format real-time PCR assay to detect mutations conferring resistance to triazoles in Aspergillus fumigatus and prevalence of multi-triazole resistance among clinical isolates in the Netherlands. J. Antimicrob. Chemother. 65, 901–905. doi: 10.1093/jac/dkq041
PubMed Abstract | Full Text | CrossRef Full Text | Google Scholar
Klaassen, C. H., Gibbons, J. G., Fedorova, N. D., Meis, J. F., and Rokas, A. (2012). Evidence for genetic differentiation and variable recombination rates among Dutch populations of the opportunistic human pathogen Aspergillus fumigatus. Mol. Ecol. 21, 57–70. doi: 10.1111/j.1365-294X.2011.05364.x
PubMed Abstract | Full Text | CrossRef Full Text | Google Scholar
Kosmidis, C., and Denning, D. W. (2015). The clinical spectrum of pulmonary aspergillosis. Thorax 70, 270–277. doi: 10.1136/thoraxjnl-2014-206291
PubMed Abstract | Full Text | CrossRef Full Text | Google Scholar
Kwon-Chung, K. J., and Sugui, J. A. (2013). Aspergillus fumigatus-what makes the species a ubiquitous human fungal pathogen? PLoS Pathog. 9:e1003743. doi: 10.1371/journal.ppat.1003743
PubMed Abstract | Full Text | CrossRef Full Text | Google Scholar
Lavergne, R. A., Morio, F., Favennec, L., Dominique, S., Meis, J. F., Gargala, G., et al. (2015). First description of azole-resistant Aspergillus fumigatus due to TR46/Y121F/T289A mutation in France. Antimicrob. Agents Chemother. (Accepted). doi: 10.1128/AAC.00127-15
PubMed Abstract | Full Text | CrossRef Full Text | Google Scholar
Lockhart, S. R., Frade, J. P., Etienne, K. A., Pfaller, M. A., Diekema, D. J., and Balajee, S. A. (2011). Azole resistance in Aspergillus fumigatus isolates from the ARTEMIS global surveillance study is primarily due to the TR/L98H mutation in the cyp51A gene. Antimicrob. Agents Chemother. 55, 4465–4468. doi: 10.1128/AAC.00185-11
PubMed Abstract | Full Text | CrossRef Full Text | Google Scholar
Mann, P. A., Parmegiani, R. M., Wei, S. Q., Mendrick, C. A., Li, X., Loebenberg, D., et al. (2003). Mutations in Aspergillus fumigatus resulting in reduced susceptibility to posaconazole appear to be restricted to a single amino acid in the cytochrome P450 14-alpha-demethylase. Antimicrob. Agents Chemother. 47, 577–581. doi: 10.1128/AAC.47.2.577-581.2003
Mellado, E., Garcia-Effron, G., Alcazar-Fuoli, L., Cuenca-Estrella, M., and Rodriguez-Tudela, J. L. (2004). Substitutions at methionine 220 in the 14-alpha-sterol demethylase (cyp51A) of Aspergillus fumigatus are responsible for resistance in vitro to azole antifungal drugs. Antimicrob. Agents Chemother. 48, 2747–2750. doi: 10.1128/AAC.48.7.2747-2750.2004
PubMed Abstract | Full Text | CrossRef Full Text | Google Scholar
Mellado, E., Garcia-Effron, G., Alcázar-Fuoli, L., Melchers, W. J., Verweij, P. E., Cuenca-Estrella, M., et al. (2007). A new Aspergillus fumigatus resistance mechanism conferring in vitro cross-resistance to azole antifungals involves a combination of cyp51A alterations. Antimicrob. Agents Chemother. 51, 1897–1904. doi: 10.1128/AAC.01092-06
PubMed Abstract | Full Text | CrossRef Full Text | Google Scholar
Morio, F., Aubin, G. G., Danner-Boucher, I., Haloun, A., Sacchetto, E., Garcia-Hermoso, D., et al. (2012). High prevalence of triazole resistance in Aspergillus fumigatus, especially mediated by TR/L98H, in a French cohort of patients with cystic fibrosis. J. Antimicrob. Chemother. 67, 1870–1873. doi: 10.1093/jac/dks160
PubMed Abstract | Full Text | CrossRef Full Text | Google Scholar
Mortensen, K. L., Jensen, R. H., Johansen, H. K., Skov, M., Pressler, T., Howard, S. J., et al. (2011). Aspergillus species and other molds in respiratory samples from patients with cystic fibrosis: a laboratory-based study with focus on Aspergillus fumigatus azole resistance. J. Clin. Microbiol. 49, 2243–2251. doi: 10.1128/JCM.00213-11
PubMed Abstract | Full Text | CrossRef Full Text | Google Scholar
Mortensen, K. L., Mellado, E., Lass-Flörl, C., Rodriguez-Tudela, J. L., Johansen, H. K., and Arendrup, M. C. (2010). Environmental study of azole-resistant Aspergillus fumigatus and other aspergilli in Austria, Denmark, and Spain. Antimicrob. Agents Chemother. 54, 4545–4549. doi: 10.1128/AAC.00692-10
PubMed Abstract | Full Text | CrossRef Full Text | Google Scholar
Pfaller, M., Boyken, L., Hollis, R., Kroeger, J., Messer, S., Tendolkar, S., et al. (2011). Use of epidemiological cutoff values to examine 9-year trends in susceptibility of Aspergillus species to the triazoles. J. Clin. Microbiol. 49, 586–590. doi: 10.1128/JCM.02136-10
PubMed Abstract | Full Text | CrossRef Full Text | Google Scholar
Pham, C. D., Reiss, E., Hagen, F., Meis, J. F., and Lockhart, S. R. (2014). Passivesurveillance for azole-resistant Aspergillus fumigatus, United States, 2011–2013. Emerg. Infect. Dis. 20, 1498–1503. doi: 10.3201/eid2009.140142
PubMed Abstract | Full Text | CrossRef Full Text | Google Scholar
Prigitano, A., Venier, V., Cogliati, M., De Lorenzis, G., Esposto, M. C., and Tortorano, A. M. (2014). Azole-resistant Aspergillus fumigatus in the environment of northern Italy, May 2011 to June 2012. Euro. Surveill. 19, 20747. doi: 10.2807/1560-7917.ES2014.19.12.20747
PubMed Abstract | Full Text | CrossRef Full Text | Google Scholar
Rath, P. M., Buchheidt, D., Spiess, B., Arfanis, E., Buer, J., and Steinmann, J. (2012). First reported case of azole-resistant Aspergillus fumigatus due to the TR/L98H mutation in Germany. Antimicrob. Agents Chemother. 56, 6060–6061. doi: 10.1128/AAC.01017-12
PubMed Abstract | Full Text | CrossRef Full Text | Google Scholar
Rocchi, S., Daguindau, E., Grenouillet, F., Deconinck, E., Bellanger, A. P., Garcia-Hermoso, D., et al. (2014). Azole-resistant Aspergillus fumigatus isolate with the TR34/L98H mutation in both a fungicide-sprayed field and the lung of a hematopoietic stem cell transplant recipient with invasive aspergillosis. J. Clin. Microbiol. 52, 1724–1726. doi: 10.1128/JCM.03182-13
PubMed Abstract | Full Text | CrossRef Full Text | Google Scholar
Rodriguez-Tudela, J. L., Alcazar-Fuoli, L., Mellado, E., Alastruey-Izquierdo, A., Monzon, A., and Cuenca-Estrella, M. (2008). Epidemiological cutoffs and cross-resistance to azole drugs in Aspergillus fumigatus. Antimicrob. Agents Chemother. 52, 2468–2472. doi: 10.1128/AAC.00156-08
PubMed Abstract | Full Text | CrossRef Full Text | Google Scholar
Seyedmousavi, S., Hashemi, S. J., Zibafar, E., Zoll, J., Hedayati, M. T., Mouton, J. W., et al. (2013). Azole-resistant Aspergillus fumigatus, Iran. Emerg. Infect. Dis. 19, 832–834. doi: 10.3201/eid1905.130075
PubMed Abstract | Full Text | CrossRef Full Text | Google Scholar
Sharma, C., Hagen, F., Moroti, R., Meis, J. F., and Chowdhary, A. (2015). Triazole resistant Aspergillus fumigatus harbouring G54 mutation: is it de novo or environmentally acquired? J. Glob. Antimicrob. Resist. 3, (in press). doi: 10.1016/j.jgar.2015.01.005
Snelders, E., Huis In ’t Veld, R. A., Rijs, A. J., Kema, G. H., Melchers, W. J., and Verweij, P. E. (2009). Possible environmental origin of resistance of Aspergillus fumigatus to medical triazoles. Appl. Environ. Microbiol. 75, 4053–4057. doi: 10.1128/AEM.00231-09
PubMed Abstract | Full Text | CrossRef Full Text | Google Scholar
Snelders, E., van der Lee, H. A., Kuijpers, J., Rijs, A. J., Varga, J., Samson, R. A., et al. (2008). Emergence of azole resistance in Aspergillus fumigatus and spread of a single resistance mechanism. PLoS Med. 5:e219. doi: 10.1371/journal.pmed.0050219
PubMed Abstract | Full Text | CrossRef Full Text | Google Scholar
Spiess, B., Postina, P., Reinwald, M., Cornely, O. A., Hamprecht, A., Hoenigl, M., et al. (2014). Incidence of Cyp51A key mutations in Aspergillus fumigatus-A study on primary clinical samples of immunocompromised patients in the period of 1995-2013. PLoS ONE 9:e103113. doi: 10.1371/journal.pone.0103113
PubMed Abstract | Full Text | CrossRef Full Text | Google Scholar
Steinmann, J., Hamprecht, A., Vehreschild, M. J., Cornely, O. A., Buchheidt, D., Spiess, B., et al. (2015). Emergence of azole-resistant invasive aspergillosis in HSCT recipients in Germany. J. Antimicrob. Chemother. 70, 1522–1526. doi: 10.1093/jac/dku566
PubMed Abstract | Full Text | CrossRef Full Text | Google Scholar
Stensvold, C., Jørgensen, L., and Arendrup, M. (2012). Azole-resistant invasive aspergillosis: relationship to agriculture. Curr. Fungal Infect. Rep. 6, 178–191. doi: 10.1007/s12281-012-0097-7
Tashiro, M., Izumikawa, K., Hirano, K., Ide, S., Mihara, T., Hosogaya, N., et al. (2012a). Correlation between triazole treatment history and susceptibility in clinically isolated Aspergillus fumigatus. Antimicrob. Agents Chemother. 56, 4870–4875. doi: 10.1128/AAC.00514-12
PubMed Abstract | Full Text | CrossRef Full Text | Google Scholar
Tashiro, M., Izumikawa, K., Minematsu, A., Hirano, K., Iwanaga, N., Ide, S., et al. (2012b). Antifungal susceptibilities of Aspergillus fumigatus clinical isolates obtained in Nagasaki, Japan. Antimicrob. Agents Chemother. 56, 584–587. doi: 10.1128/AAC.05394-11
PubMed Abstract | Full Text | CrossRef Full Text | Google Scholar
van der Linden, J. W., Arendrup, M. C., Warris, A., Lagrou, K., Pelloux, H., Hauser, P. M., et al. (2015). Prospective multicentre international surveillance of azole resistance in Aspergillus fumigatus. Emerg. Infect. Dis. (in press). doi: 10.3201/eid2106.140717
van der Linden, J. W., Camps, S. M., Kampinga, G. A., Arends, J. P., Debets-Ossenkopp, Y. J., Haas, P. J., et al. (2013). Aspergillosis due to voriconazole highly resistant Aspergillus fumigatus and recovery of genetically related resistant isolates from domiciles. Clin. Infect. Dis. 57, 513–520. doi: 10.1093/cid/cit320
PubMed Abstract | Full Text | CrossRef Full Text | Google Scholar
van der Linden, J. W., Snelders, E., Kampinga, G. A., Rijnders, B. J., Mattsson, E., Debets-Ossenkopp, Y. J., et al. (2011). Clinical implications of azole resistance in Aspergillus fumigatus, The Netherlands, 2007-2009. Emerg. Infect. Dis. 17, 1846–1854. doi: 10.3201/eid1710.110226
PubMed Abstract | Full Text | CrossRef Full Text | Google Scholar
Verweij, P. E., Mellado, E., and Melchers, W. J. (2007). Multiple-triazole-resistant aspergillosis. N. Engl. J. Med. 356, 1481–1483. doi: 10.1056/NEJMc061720
PubMed Abstract | Full Text | CrossRef Full Text | Google Scholar
Verweij, P. E., Snelders, E., Kema, G. H., Mellado, E., and Melchers, W. J. (2009). Azole resistance in Aspergillus fumigatus: a side-effect of environmental fungicide use? Lancet Infect. Dis. 9, 789–795. doi: 10.1016/S1473-3099(09)70265-8
PubMed Abstract | Full Text | CrossRef Full Text | Google Scholar
Wang, D. Y., Gricourt, M., Arné, P., Thierry, S., Seguin, D., Chermette, R., et al. (2014). Mutations in the Cyp51A gene and susceptibility to itraconazole in Aspergillus fumigatus isolated from avian farms in France and China. Poult. Sci. 93, 12–15. doi: 10.3382/ps.2013-03541
PubMed Abstract | Full Text | CrossRef Full Text | Google Scholar
Xu, H., Chen, W., Li, L., Wan, Z., Li, R., and Liu, W. (2010). Clinical itraconazole-resistant strains of Aspergillus fumigatus, isolated serially from a lung aspergilloma patient with pulmonary tuberculosis, can be detected with real-time PCR method. Mycopathologia 169, 193–199. doi: 10.1007/s11046-009-9249-x
PubMed Abstract | Full Text | CrossRef Full Text | Google Scholar
Keywords: triazole resistant A. fumigatus, TR34/L98H, G54E, microsatellite typing, India, Asia
Citation: Chowdhary A, Sharma C, Kathuria S, Hagen F and Meis JF (2015) Prevalence and mechanism of triazole resistance in Aspergillus fumigatus in a referral chest hospital in Delhi, India and an update of the situation Front. Microbiol. 6:428. doi: 10.3389/fmicb.2015.00428
Received: 04 March 2015; Accepted: 22 April 2015;
Published online: 08 May 2015.
Edited by:
Frederic Lamoth, Lausanne University Hospital, SwitzerlandReviewed by:
Dominique Sanglard, University of Lausanne and University Hospital Center, SwitzerlandJesus Guinea, Hospital Gregorio Marañón, Spain
Copyright © 2015 Chowdhary, Sharma, Kathuria, Hagen and Meis. This is an open-access article distributed under the terms of the Creative Commons Attribution License (CC BY). The use, distribution or reproduction in other forums is permitted, provided the original author(s) or licensor are credited and that the original publication in this journal is cited, in accordance with accepted academic practice. No use, distribution or reproduction is permitted which does not comply with these terms.
*Correspondence: Anuradha Chowdhary, Department of Medical Mycology, Vallabhbhai Patel Chest Institute, University of Delhi, Delhi 110 007, India, dranuradha@hotmail.com