- 1College of Veterinary Medicine, Shandong Agricultural University, Tai'an, China
- 2Sino-German Cooperative Research Centre for Zoonosis of Animal Origin Shandong Province, Tai'an, China
- 3Key Laboratory of Animal Biotechnology and Disease Control and Prevention of Shandong Province, Tai'an, China
- 4College of Life Sciences, Taishan Medical University, Tai'an, China
The prevalence of extended-spectrum beta-lactamase (ESBL)-producing Escherichia coli (E. coli) is increasing rapidly in both hospital environments and animal farms. A lot of animal manure has been directly applied into arable fields in developing countries. But the impact of ESBL-positive bacteria from animal manure on the agricultural fields is sparse, especially in the rural regions of Tai'an, China. Here, we collected 29, 3, and 10 ESBL-producing E. coli from pig manure, compost, and soil samples, respectively. To track ESBL-harboring E. coli from agricultural soil, these isolates of different sources were analyzed with regard to antibiotic resistance profiles, ESBL genes, plasmid replicons, and enterobacterial repetitive intergenic consensus (ERIC)-polymerase chain reaction (PCR) typing. The results showed that all the isolates exhibited multi-drug resistant (MDR). CTX-M gene was the predominant ESBL gene in the isolates from pig farm samples (30/32, 93.8%) and soil samples (7/10, 70.0%), but no SHV gene was detected. Twenty-five isolates contained the IncF-type replicon of plasmid, including 18 strains (18/32, 56.3%) from the pig farm and 7 (7/10, 70.0%) from the soil samples. ERIC-PCR demonstrated that 3 isolates from soil had above 90% genetic similarity with strains from pig farm samples. In conclusion, application of animal manure carrying drug-resistant bacteria on agricultural fields is a likely contributor to antibiotic resistance gene spread.
Introduction
The rapid increase of extended-spectrum beta-lactamases (ESBLs)-producing Enterobacteriaceae has attracted worldwide concern. ESBLs are enzymes that make bacteria, especially Escherichia coli and Klebsiella pneumoniae, resistant to beta-lactam antibiotics including 3rd and 4rd generation cephalosporins to decrease antibiotics available in clinical practices (Bradford, 2001). ESBL-producing E. coli were widely found in hospital environments and farms (Edelstein et al., 2003; Smet et al., 2008) and they are able to survive in various natural environments such as feces, soils, and water bodies (Koczura et al., 2012; Blaak et al., 2014; Haque et al., 2014), so ESBL-producing E. coli is usually regarded as an indicator bacterium to trace the spread of antibiotic resistance gene (Gao et al., 2014). At present, numerous studies have focused on the occurrence and spread of ESBL genes in hospital environments, waste water treatment plants, water bodies, and food-producing animals (Edelstein et al., 2003; Agerso et al., 2012; Blaak et al., 2014). ESBL-producing E. coli have been shown to be able to transmit from animal farms to surrounding environments, even rural water reservoirs (Laube et al., 2014; Von Salviati et al., 2015; Zhang et al., 2015).
In rural areas of China, animal manure has been used to feed agricultural fields as fertilizers in the production of crops, fruits, and vegetables (Huang et al., 2014). But animal feces contain large amounts of antimicrobial-resistant bacteria, including certain pathogenic bacteria such as Listeria monocytogenes and Salmonella spp. and certain drug-resistant bacteria, such as ESBL-producing E. coli, vancomycin-resistant Enterococcus and methicillin-resistant Staphylococcus aureus (Guber et al., 2007). Animal manure fertilization has been found to increase the abundance of drug-resistant bacteria and the frequency of antibiotic resistance genes in soils (Venglovsky et al., 2009; Marti et al., 2013). Zhu et al. (2013) found diverse and abundant antibiotic resistance genes in compost and soil treated with manure. When animal manure is added into soil in the form of solid or liquid, antibiotic resistance genes can transfer between the same species and even different ones through genetic elements, especially plasmids (D'Costa et al., 2006; Guber et al., 2007; Heuer et al., 2011a). Moreover, antibiotic resistance genes or bacteria in soils could enter into other environments and food chain through various ways to threaten public health. Researchers have found polluted soil could contaminate surface water by over land flow or rainfall (Tate et al., 2000; Curriero et al., 2001). There are also reports about the contamination of resistance gene in vegetables (Ruimy et al., 2010; Reuland et al., 2014). Therefore, investigations on the influence of animal manure application on agriculture are of great significance.
In China, a large number of arable soils have been amended with commercial fertilizers and animal manures (Ju et al., 2007). But, up to date, little information about whether the fertilization model enhances the dissemination of drug-resistant bacteria of animal origins was provided. Previous studies have shown that manure application contributed to the accumulation of resistance genes in soil, mainly sulfonamide resistance genes (Sengeløv et al., 2003; Zhou et al., 2010; Heuer et al., 2011b). In the last decade, the prevalence of ESBL genes has increased rapidly. Meanwhile, ESBL genes that were found to be located on mobile genetic elements, and often associated with other resistance genes, could transfer horizontally between bacteria (Allen, 2014). Thus, this study was performed to assess the dissemination of ESBL-producing E. coli of animal origins into agricultural fields.
Materials and Methods
Sampling Sites and Collection of Samples
Manure samples and soil samples were collected from a pig farm with an intensity of 5000 pigs and its surrounding lands fed with manure between May and July 2014. The farm is located in rural region of Tai'an, China, far away from villages, surrounded by farm land where crops (corn and beans) were planted. Pig manure was piled up and then used as compost into the soil or directly fed the soil by local farmers instead of chemical fertilizer. The soil samples were collected from the cropland which has been receiving compost for at least 3 years.
The pig farm was visited four times. Each time, 10 fecal samples, five composts were collected. At the same time, 20 soil samples were collected from different locations in the surrounding field. Forty soil samples collected from soil treated with chemical fertilizer were used as control. All samples were immediately transported to the lab with an ice box and processed in 6 h.
Isolation and Confirmation of ESBL-producing E. coli
About 0.5 g sample soil/feces was dissolved in 5 ml phosphate buffered saline and homogenized. Then 1 ml solution was mixed with 9 ml BHI broth (Haibo, Qingdao, China) for enrichment at 37°C overnight under aerobic conditions. Hundred microliters soil enrichment or 1 loop of the fecal samples enrichment solution was spread onto MacConkey agar (Oxoid, Basingstoke, England) plates with 2 mg/l cefotaxime and then incubated overnight at 37°C.
A presumptive ESBL-producing E. coli colony with bright pink or red color was identified by traditional biochemical test including indole, Methyl red-Voges-Proskauer, and citrate biochemical testing and API 20E (bioMerieux, Marcy l'Etoile, France) (Chang et al., 2015).
The screened ESBL-producing E. coli isolates were further confirmed by phenotypic confirmatory tests using cefotaxime (30 μg), cefotaxime + clavulanic acid (30 μg/10 μg), ceftazidime (30 μg), ceftazidime + clavulanic acid (30 μg/10 μg) (CLSI, 2013).
Antimicrobial Susceptibility
The confirmed ESBL-producing E. coli were subjected to antimicrobial susceptibility tests according to the Clinical and Laboratory Standards Institute (CLSI, 2013) guidelines. Disk diffusion method was used to determine resistance profile of the isolates on Mueller-Hinton agar (Haibo, Qingdao, China). Eight drug classes containing 14 antibiotics (Oxoid, England) were included representing the most frequently used antibiotics in animals. Penicillins were represented by amoxicillin (AML). Cephalosporins included ceftiofur (CET), cephalothin (KF), cefotaxime (CTX), and ceftriaxone (CRO). Carbopenems were represented by imipenem (IPM). Aminoglycosides were represented by gentamicin (GM), kanamycin (K), and amikacin (AK). Quinolones included nalidixic acid (NA) and ciprofloxacin (CIP). Tetracyclines were represented by tetracycline (TE). Chloramphenicol (C) and florfenicol (FFC) were also included. The standard E. coli ATCC 25922 was used as quality control strain (Zhang et al., 2014). E. coli isolates resistant to three or more categories were regarded as multi-drug resistant (MDR), resistant to one or two categories regarded as extensively drug-resistant (XDR) and resistant to all antimicrobial categories considered as pandrug-resistant (PDR) (Magiorakos et al., 2012).
Detection of ESBL Genes
Major β-lactamase genes detected in food-producing animals in China, blaTEM, blaSHV, blaCTX−M, blaCMY−2, and blaOXA, were amplified by PCR (Zhao et al., 2001; Monstein et al., 2007; Shaheen et al., 2011). Groups of CTX-M-positive E. coli were further determined using specific group primers for CTX-M-1, CTX-M-2, and CTX-M-9 (Batchelor et al., 2005). The PCR products were purified using a PCR purification kit (TianGen, Beijing, China) and then sequenced bi-directionally with the same primers by ABI 3730 DNA sequencer (Applied Biosystems, Foster City, CA, USA) (Zhang et al., 2014). All gene sequences were subjected to BLASTn analysis in GenBank (http://www.ncbi.nlm.nih.gov/) or β-lactamase classification system (http://www.lahey.org/studies/webt.asp) to confirm subtypes of ESBL-encoding genes (Zheng et al., 2012).
Plasmid Replicon Typing
The plasmid replicon types of the ESBL-producing E. coli were detected using the inc/rep PCR method (a PCR method based on replicons of major plasmid incompatibility groups) (Carattoli et al., 2005). Multiplex- and simplex-PCR were used to recognize 18 plasmid incompatibility groups among Enterobacteriaceae including FIA, FIB, FIC, HI1, HI2, I1-Ig, L/M, N, P, W, T, A/C, K, B/O, X, Y, F, and FIIA and performed as previously described (Carattoli et al., 2005).
ERIC-PCR Analysis
Twenty-one ESBL-producing E. coli isolates from pig farm samples and seven isolates from treated soil samples were selected randomly and subjected to enterobacterial repetitive intergenic consensus sequence (ERIC-PCR) to analyze the similarity of the strains from manure, compost and treated soil. The PCR procedure and conditions were performed as previously described (Koczura et al., 2012). The amplified products were separated in 2% agarose gel by electrophoresis. The presence or absence of the amplified bands on the gel were recorded as 1 or 0 for further analysis. NTSYS-pc (Version 2.10) was used to do cluster analysis based on Dice's similarity coefficient with a 1% position tolerance and the unweighted pair group method using arithmetic averages (UPGMA). Isolates of different origins or the same origin with above 90% similarity were treated as the same strain (Edelstein et al., 2003; Yuan et al., 2010).
Results
Isolation of ESBL-producing E. coli
A total of 42 ESBL-producing E. coli were isolated from the 180 samples. There were 32 isolates from pig farm samples, including 29 from manure and three from compost samples. From the soil samples treated with compost or manure, 10 ESBL-producing E. coli were isolated. However, no ESBL-producing isolate was obtained from soil treated with chemical fertilizer (Table 1).
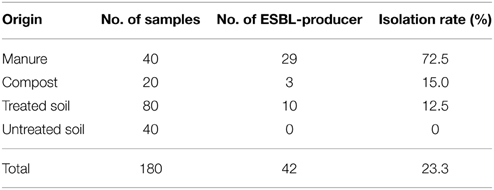
Table 1. Isolation of ESBL-producing E. coli from manure, compost, treated soil, and untreated soil.
Antimicrobial Susceptibility
All the 42 ESBL-producing E. coli from manure, compost, and treated soil showed multiple drug resistance to antibiotics tested and the isolates from different origins had similar drug-resistant spectrum against the 14 antibiotics. Forty-two isolates were all 100% resistant to AML and KF, but susceptible to IMP. Additionally, isolates from different sources had above 60% resistance to CET, K, TE, C, and FFC (Table 2 and Table S1).
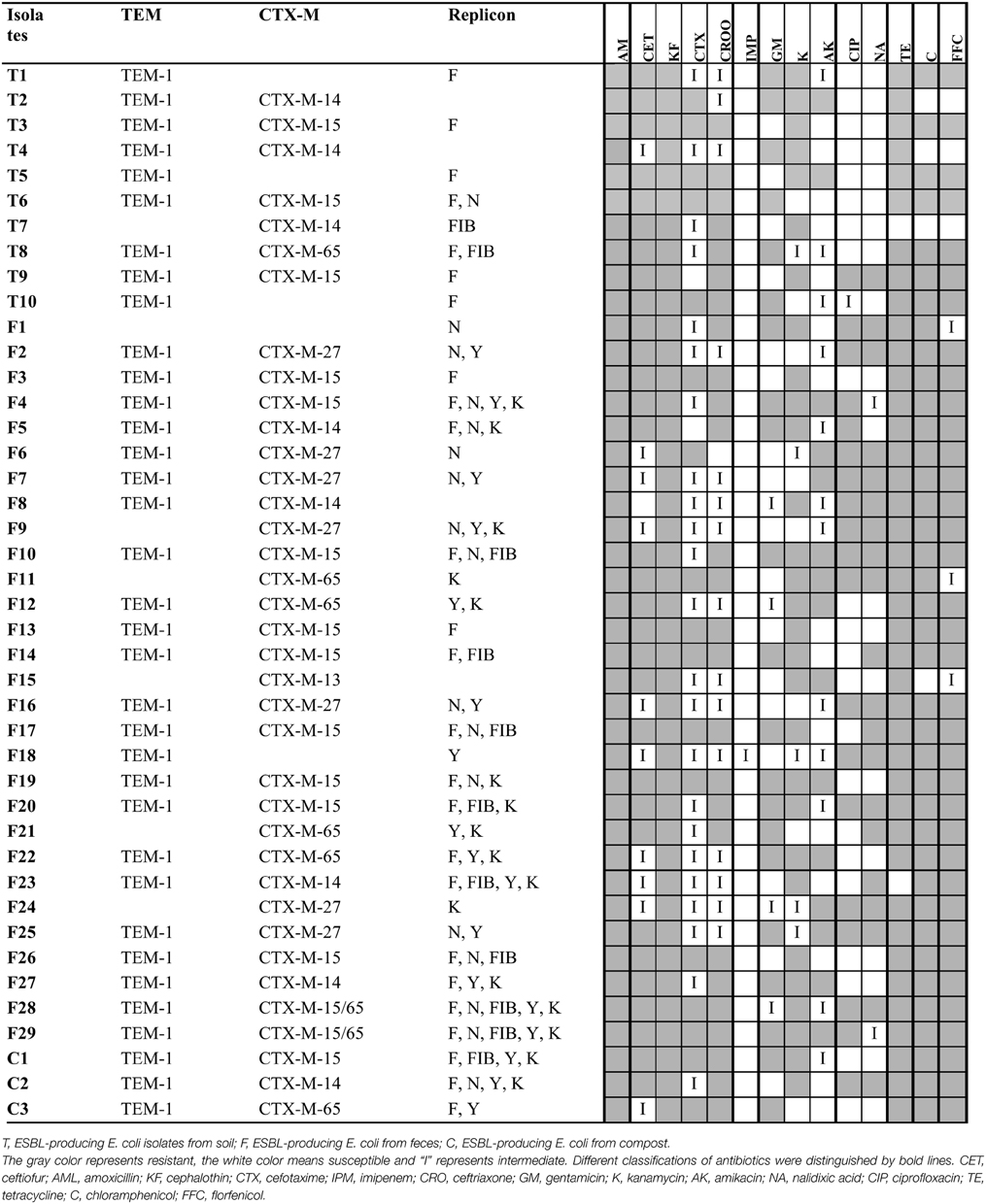
Table 2. Characteristics of the 42 ESBL-producing E. coli from different samples including β-lactamase genes, replicon type, and resistance profile.
Characterization of β-Lactamase Genes
Among the 42 ESBL-producing E. coli, 37 isolates carried blaCTX−M(37/42, 86.0%), including 30 isolates from the pig farm (30/32, 93.8%), and seven from treated soil (7/10, 70.0%). Thirty-one isolates carried blaCTX−M+TEM gene, including 25 isolates (25/32, 78.1%) from the pig farm and six (6/10, 60.0%) from treated soil. The other three genes (SHV, CMY-2 and OXA) were all not detected.
Sequence and blast results showed that the most prevalent ESBL gene in pig farm samples was blaCTX−M−15, which was found in 12 isolates, followed by blaCTX−M−27 and blaCTX−M−65 respectively detected in seven isolates. Five isolates from the pig farm carried blaCTX−M−14 and one isolate harbored blaCTX−M−13. Two isolates from fecal samples carried both blaCTX−M−15 and blaCTX−M−65 gene. Both blaCTX−M−15 and blaCTX−M−14 genes were the common ESBL genes in isolates from soil samples and respectively detected in three strains as shown in Table 2.
Five isolates of the 42 ESBL-producing E. coli did not carry ESBL genes (three from soil samples and two from pig farm samples). Three isolates from soil samples and one from manure only carried blaTEM−1 gene. No β-lactamase gene was found in one isolate from manure.
Plasmid Replicon Type
Among the 42 ESBL-producing E. coli of different origins, IncF was the most prevalent replicon type in the isolates from the pig farm (18/32, 56.3%) and treated soil samples (7/10, 70%). IncN was detected in 16 ESBL-producers from pig farm samples and one from soil samples. IncFIB was found in nine isolates from pig farm samples and two from soil samples. Four isolates were not typed including both two from pig farm samples and soil samples as shown in Table 2.
Isolates from pig farm samples showed a higher diversity of inc/rep than that from soil samples. IncK and Y were detected in 16 and 17 isolates from pig farm samples respectively, while none of them was found in soil samples.
Similarity Analysis of ESBL-producing E. coli from Manure, Compost, and Treated Soil
The similarity between ESBL-producing E. coli from pig farm and treated soil was between 70 and 100%. Three isolates (T2, T4, and T7) from treated soil had above 90% similarity with those from pig manure and compost samples (F8 and F1) (Figure 1), which suggested that they might come from the same strain.
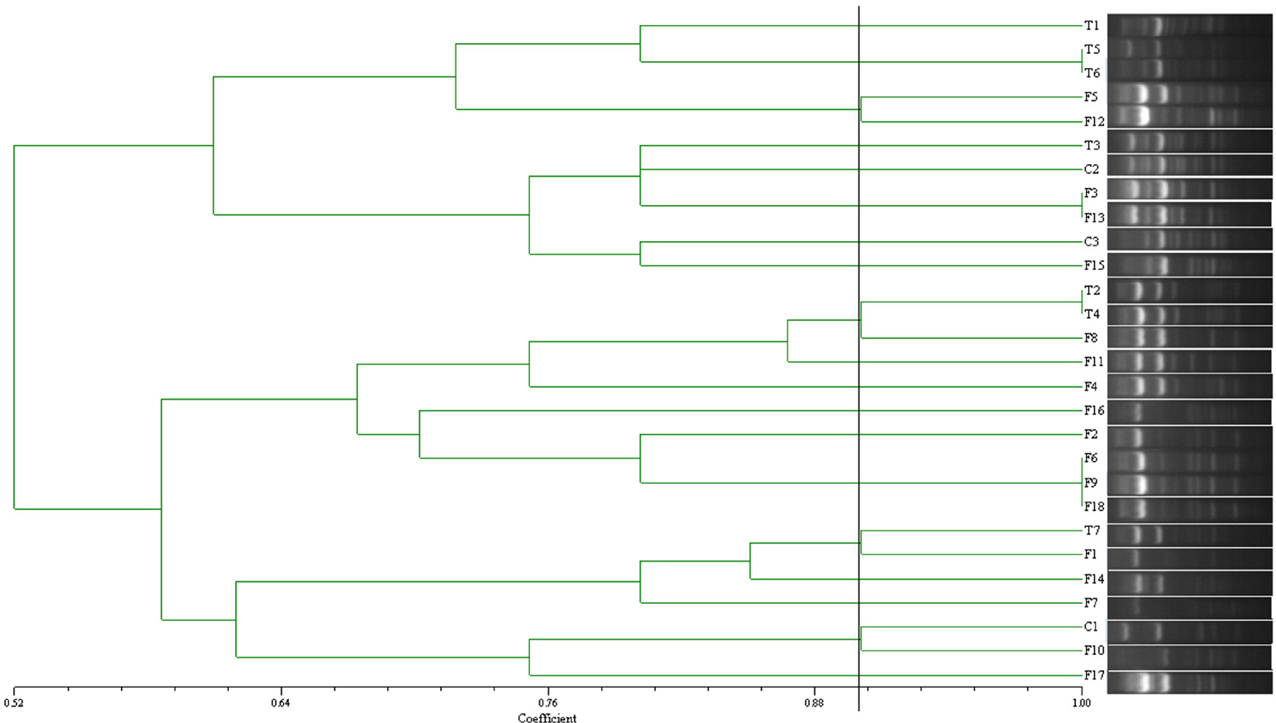
Figure 1. Dendrogram of ERIC-PCR results of 28 ESBL-producing E. coli originating from manure (F), compost (C), and soil (T).
Discussion
Animal wastes without composting process have been widely used in agricultural fields, especially in developing countries to improve the soil fertility and structure (Kumar et al., 2005; Hu et al., 2010). However, animal original bacteria carrying antibiotic resistance genes can regrow to high levels under favorable condition (Ghosh and LaPara, 2007; Marti et al., 2013; Chen and Jiang, 2014). Wide application of animal manure to agricultural fields has raised a concern about antibiotic resistance genes spread. Once antimicrobial-resistant bacteria from animal feces enter into arable soils, mobile genetic elements can transmit between the same or different species of bacteria under some conditions, especially between Enterobacteriaceae (Rensing et al., 2002; Heuer and Smalla, 2007). Additionally, these bacteria can spread to other environments through rainwater or other routes (Khaleel et al., 1982; Curriero et al., 2001).
Soil harbored a vast diversity of microorganisms, which was considered as a potential reservoir for antibiotic resistance (Forsberg et al., 2012). When drug-resistant bacteria of animal origins enter into this community, they could exchange resistance genes with soil-dwelling organisms. In this study, we isolated ESBL-producing E. coli from one pig farm as an indicator to better understand the pollution of ESBL-producing E. coli from animal manure on agricultural fields. We found that ESBL producers from manure, compost, and soil showed a remarkable similarity in terms of resistance phenotypes, ESBL genes, plasmid replicon type, and genomic characterization.
Previous studies have demonstrated that animal feces carrying drug-resistant bacteria can lead to antibiotic resistance genes spread, which attracted wide concern worldwide (Heuer et al., 2011a). In China, a large of arable soils have been amended with commercial fertilizers and animal manure (Huang et al., 2014), therefore it is of importance to investigate whether this fertilization model can enhance the dissemination of drug-resistant bacteria of animal origins can disseminate into the surrounding soil. In this study, we isolated ESBL-producing E. coli from pig farm samples (manure and compost) and soil treated with pig manure, but no isolate was detected in control soil samples only treated with chemical fertilizer. These results indicated that pig manure may be a likely contributor of drug-resistant bacteria or genes, and antibiotic selection may be another reason. Additionally, the presence of ESBL-producers in soils may be related with the sampling time and the frequency of fertilization (Jensen et al., 2001; Sengeløv et al., 2003; Riber et al., 2014), which is required to be further studied.
The 42 ESBL-producing E. coli all showed multi-drug resistance to 14 antibiotics commonly used in the local clinics, and the isolates both from pig farm samples and treated soil had the similar characteristics of resistance to a large extend. The close relatedness also indicated that application of pig manure to agricultural fields may play an important role in the dissemination of drug-resistant bacteria or genes of animal origins. ESBL-producers from different sources showed high resistance to the β-lactam antibiotics. It may be related with the use of ceftiofur that was allowed to be used in veterinary medicine in China (Guo et al., 2014).
CTX-M gene was the predominant ESBL genes in pig farm samples, which was also the main ESBL gene detected in soil samples as previous studies (Pitout and Laupland, 2008; Ewers et al., 2012). CTX-M-15, CTX-M-14, and CTX-M-65 genes were found in ESBL-producing E. coli of different origins. CTX-M-15 was detected in 12 isolates in pig farms accounting for 37.5%, similar prevalence 30% was observed in treated soil samples. IncF was the dominant replicon type in samples from pig farms and soil samples detected in 18 isolates from pig farm samples and seven from soil. Additionally, ERIC-PCR of the ESBL-producing E. coli showed four isolates from treated soil exhibited above 90% similarity with fecal isolates. The similar characteristics suggested that isolates in soil may mainly come from the manure or compost. The result showed that pig manure may be a likely contributor of drug-resistant bacteria or genes, including ESBL producers. However, isolates from the pig farm showed a high diversity of replicon or ESBL genes, which may be due to the different evolution and selective pressure of those isolates.
The bacteria community of arable soil was closely related with organic fertilizer applications. Previous studies have demonstrated that drug-resistant genes were found in the arable soil amended with manures (Sengeløv et al., 2003; Heuer et al., 2011a) and the repeated application of manure could increase the abundance of sulfonamide resistance gene (Heuer et al., 2011b). ESBL-producing E. coli have been detected on the surface of the ground in the vicinity of the animal farms (Walsh and Duffy, 2013; Laube et al., 2014).
In summary, ESBL-producing isolates from compost, treated soil, and manure showed high overlaps in terms of resistance phenotypes, ESBL genes, plasmid replicon type, and genomic backbone characterization, which implies the dissemination of resistance bacteria or genes of animal origins to soil that treated with animal manure.
Conflict of Interest Statement
The authors declare that the research was conducted in the absence of any commercial or financial relationships that could be construed as a potential conflict of interest.
Acknowledgments
This study was sponsored by the project of National Natural Science Foundation of China [31270172]; the project of Natural Science Foundation of Shandong Province [ZR2012CM041]; Shandong Natural Science Foundation [ZR2013CM030]; and Taishan Medical University Grand [2013GCC07].
Supplementary Material
The Supplementary Material for this article can be found online at: http://www.frontiersin.org/journal/10.3389/fmicb.2015.00313/abstract
References
Agerso, Y., Aarestrup, F. M., Pedersen, K., Seyfarth, A. M., Struve, T., and Hasman, H. (2012). Prevalence of extended-spectrum cephalosporinase (ESC)-producing Escherichia coli in Danish slaughter pigs and retail meat identified by selective enrichment and association with cephalosporin usage. J. Antimicrob. Chemother. 67, 582–588. doi: 10.1093/jac/dkr507
Allen, H. K. (2014). Antibiotic resistance gene discovery in food-producing animals. Curr. Opin. Microbiol. 19, 25–29. doi: 10.1016/j.mib.2014.06.001
PubMed Abstract | Full Text | CrossRef Full Text | Google Scholar
Batchelor, M., Hopkins, K., Threlfall, E. J., Clifton-Hadley, F. A., Stallwood, A. D., Davies, R. H., et al. (2005). bla(CTX-M) genes in clinical Salmonella isolates recovered from humans in England and Wales from 1992 to 2003. Antimicrob. Agents Chemother. 49, 1319–1322.
Blaak, H., de Kruijf, P., Hamidjaja, R. A., van Hoek, A. H., de Roda, H. A., and Schets, F. M. (2014). Prevalence and characteristics of ESBL-producing E. coli in Dutch recreational waters influenced by wastewater treatment plants. Vet. Microbiol. 171, 448–459. doi: 10.1016/j.vetmic.2014.03.007
PubMed Abstract | Full Text | CrossRef Full Text | Google Scholar
Bradford, P. A. (2001). Extended-spectrum beta-lactamases in the 21st century: characterization, epidemiology, and detection of this important resistance threat. Clin. Microbiol. Rev. 14, 933–951. doi: 10.1128/CMR.14.4.933-951.2001
PubMed Abstract | Full Text | CrossRef Full Text | Google Scholar
Carattoli, A., Bertini, A., Villa, L., Falbo, V., Hopkins, K. L., and Threlfall, E. J. (2005). Identification of plasmids by PCR-based replicon typing. J. Microbiol. Methods 63, 219–228. doi: 10.1016/j.mimet.2005.03.018
PubMed Abstract | Full Text | CrossRef Full Text | Google Scholar
Chang, S. K., Lo, D. Y., Wei, H. W., and Kuo, H. C. (2015). Antimicrobial resistance of Escherichia coli isolates from canine urinary tract infections. J. Vet. Med. Sci. 77, 59–65. doi: 10.1292/jvms.13-0281
PubMed Abstract | Full Text | CrossRef Full Text | Google Scholar
Chen, Z., and Jiang, X. P. (2014). Microbiological safety of chicken litter or chicken litter-based organic fertilizers: a review. Agriculture 4, 1–29. doi: 10.3390/agriculture4010001
CLSI. (2013). Performance Standards for Antimicrobial Susceptibility Testing: 23rd Informational Supplement (M100-S23). Wayne, PA: Clinical and Laboratory Standards Institute.
Curriero, F. C., Patz, J. A., Rose, J. B., and Lele, S. (2001). The association between extreme precipitation and waterborne disease outbreaks in the United States, 1948-1994. Am. J. Public Health 91, 1194–1199. doi: 10.2105/AJPH.91.8.1194
PubMed Abstract | Full Text | CrossRef Full Text | Google Scholar
D'Costa, V. M., McGrann, K. M., Hughes, D. W., and Wright, G. D. (2006). Sampling the antibiotic resistome. Science 311, 374–377. doi: 10.1126/science.1120800
PubMed Abstract | Full Text | CrossRef Full Text | Google Scholar
Edelstein, M., Pimkin, M., Palagin, I., Edelstein, I., and Stratchounski, L. (2003). Prevalence and molecular epidemiology of CTX-M extended-spectrum beta-lactamase-producing Escherichia coli and Klebsiella pneumoniae in Russian hospitals. Antimicrob. Agents Chemother. 47, 3724–3732. doi: 10.1128/AAC.47.12.3724-3732.2003
PubMed Abstract | Full Text | CrossRef Full Text | Google Scholar
Ewers, C., Bethe, A., Semmler, T., Guenther, S., and Wieler, L. H. (2012). Extended-spectrum beta-lactamase-producing and AmpC-producing Escherichia coli from livestock and companion animals, and their putative impact on public health: a global perspective. Clin. Microbiol. Infect. 18, 646–655. doi: 10.1111/j.1469-0691.2012.03850.x
PubMed Abstract | Full Text | CrossRef Full Text | Google Scholar
Forsberg, K. J., Reyes, A., Wang, B., Selleck, E. M., Sommer, M. O., and Dantas, G. (2012). The shared antibiotic resistome of soil bacteria and human pathogens. Science 337, 1107–1111. doi: 10.1126/science.1220761
PubMed Abstract | Full Text | CrossRef Full Text | Google Scholar
Gao, L., Hu, J., Zhang, X., Ma, R., Gao, J., Li, S., et al. (2014). Dissemination of ESBL-Producing Escherichia coli of Chicken Origin to the Nearby River Water. J. Mol. Microbiol. Biotechnol. 24, 279–285. doi: 10.1159/000365786
PubMed Abstract | Full Text | CrossRef Full Text | Google Scholar
Ghosh, S., and LaPara, T. M. (2007). The effects of subtherapeutic antibiotic use in farm animals on the proliferation and persistence of antibiotic resistance among soil bacteria. ISME J. 1, 191–203. doi: 10.1038/ismej.2007.31
PubMed Abstract | Full Text | CrossRef Full Text | Google Scholar
Guber, A. K., Pachepsky, Y. A., Shelton, D. R., and Yu, O. (2007). Effect of bovine manure on fecal coliform attachment to soil and soil particles of different sizes. Appl. Environ. Microbiol. 73, 3363–3370. doi: 10.1128/AEM.02434-06
PubMed Abstract | Full Text | CrossRef Full Text | Google Scholar
Guo, Y. F., Zhang, W. H., Ren, S. Q., Yang, L., Lu, D. H., Zeng, Z. L., et al. (2014). IncA/C plasmid-mediated spread of CMY-2 in multidrug-resistant Escherichia coli from food animals in China. PLoS ONE 9:e96738. doi: 10.1371/journal.pone.0096738
PubMed Abstract | Full Text | CrossRef Full Text | Google Scholar
Haque, A., Yoshizumi, A., Saga, T., Ishii, Y., and Tateda, K. (2014). ESBL-producing Enterobacteriaceae in environmental water in Dhaka, Bangladesh. J. Infect. Chemother. 20, 735–737. doi: 10.1016/j.jiac.2014.07.003
PubMed Abstract | Full Text | CrossRef Full Text | Google Scholar
Heuer, H., Schmitt, H., and Smalla, K. (2011a). Antibiotic resistance gene spread due to manure application on agricultural fields. Curr. Opin. Microbiol. 14, 236–243. doi: 10.1016/j.mib.2011.04.009
PubMed Abstract | Full Text | CrossRef Full Text | Google Scholar
Heuer, H., and Smalla, K. (2007). Manure and sulfadiazine synergistically increased bacterial antibiotic resistance in soil over at least two months. Environ. Microbiol. 9, 657–666. doi: 10.1111/j.1462-2920.2006.01185.x
PubMed Abstract | Full Text | CrossRef Full Text | Google Scholar
Heuer, H., Solehati, Q., Zimmerling, U., Kleineidam, K., Schloter, M., Müller, T., et al. (2011b). Accumulation of sulfonamide resistance genes in arable soils due to repeated application of manure containing sulfadiazine. Appl. Environ. Microbiol. 77, 2527–2530. doi: 10.1128/AEM.02577-10
PubMed Abstract | Full Text | CrossRef Full Text | Google Scholar
Hu, X., Zhou, Q., and Luo, Y. (2010). Occurrence and source analysis of typical veterinary antibiotics in manure, soil, vegetables and groundwater from organic vegetable bases, northern China. Environ. Pollut. 158, 2992–2998. doi: 10.1016/j.envpol.2010.05.023
PubMed Abstract | Full Text | CrossRef Full Text | Google Scholar
Huang, L., Yao, L., He, Z., Zhou, C., Li, G., Yang, B., et al. (2014). Roxarsone and its metabolites in chicken manure significantly enhance the uptake of As species by vegetables. Chemosphere 100, 57–62. doi: 10.1016/j.chemosphere.2013.12.074
PubMed Abstract | Full Text | CrossRef Full Text | Google Scholar
Jensen, L. B., Baloda, S., Boye, M., and Aarestrup, F. M. (2001). Antimicrobial resistance among Pseudomonas spp. and the Bacillus cereus group isolated from Danish agricultural soil. Environ. Int. 26, 581–587. doi: 10.1016/S0160-4120(01)00045-9
PubMed Abstract | Full Text | CrossRef Full Text | Google Scholar
Ju, X. T., Kou, C. L., Christie, P., Dou, Z. X., and Zhang, F. S. (2007). Changes in the soil environment from excessive application of fertilizers and manures to two contrasting intensive cropping systems on the North China Plain. Environ. Pollut. 145, 497–506. doi: 10.1016/j.envpol.2006.04.017
PubMed Abstract | Full Text | CrossRef Full Text | Google Scholar
Khaleel, R., Reddy, K. R., and Overcash, M. R. (1982). Transport of potential pollutants in runoff water from areas receiving animal waste. Water Res. 14, 421–436. doi: 10.1016/0043-1354(80)90206-7
Koczura, R., Mokracka, J., Jablonska, L., Gozdecka, E., Kubek, M., and Kaznowski, A. (2012). Antimicrobial resistance of integron-harboring Escherichia coli isolates from clinical samples, wastewater treatment plant and river water. Sci. Total Environ. 414, 680–685. doi: 10.1016/j.scitotenv.2011.10.036
PubMed Abstract | Full Text | CrossRef Full Text | Google Scholar
Kumar, K., Gupta, S. C., Baidoo, S. K., Chander, Y., and Rosen, C. J. (2005). Antibiotic uptake by plants from soil fertilized with animal manure. J. Environ. Qual. 34, 2082–2085. doi: 10.2134/jeq2005.0026
PubMed Abstract | Full Text | CrossRef Full Text | Google Scholar
Laube, H., Friese, A., von Salviati, C., Guerra, B., and Rosler, U. (2014). Transmission of ESBL/AmpC-producing Escherichia coli from broiler chicken farms to surrounding areas. Vet. Microbiol. 172, 519–527. doi: 10.1016/j.vetmic.2014.06.008
PubMed Abstract | Full Text | CrossRef Full Text | Google Scholar
Magiorakos, A. P., Srinivasan, A., Carey, R. B., Carmeli, Y., Falagas, M. E., Giske, C. G., et al. (2012). Multidrug-resistant, extensively drug-resistant and pandrug-resistant bacteria: an international expert proposal for interim standard definitions for acquired resistance. Clin. Microbiol. Infect. 18, 268–281. doi: 10.1111/j.1469-0691.2011.03570.x
PubMed Abstract | Full Text | CrossRef Full Text | Google Scholar
Marti, R., Scott, A., Tien, Y. C., Murray, R., Sabourin, L., Zhang, Y., et al. (2013). Impact of manure fertilization on the abundance of antibiotic-resistant bacteria and frequency of detection of antibiotic resistance genes in soil and on vegetables at harvest. Appl. Environ. Microbiol. 79, 5701–5709. doi: 10.1128/AEM.01682-13
PubMed Abstract | Full Text | CrossRef Full Text | Google Scholar
Monstein, H. J., Ostholm-Balkhed, A., Nilsson, M. V., Nilsson, M., Dornbusch, K., and Nilsson, L. E. (2007). Multiplex PCR amplification assay for the detection of blaSHV, blaTEM and blaCTX-M genes in Enterobacteriaceae. APMIS 115, 1400–1408. doi: 10.1111/j.1600-0463.2007.00722.x
PubMed Abstract | Full Text | CrossRef Full Text | Google Scholar
Pitout, J. D., and Laupland, K. B. (2008). Extended-spectrum beta-lactamase-producing Enterobacteriaceae: an emerging public-health concern. Lancet Infect. Dis. 8, 159–166. doi: 10.1016/S1473-3099(08)70041-0
PubMed Abstract | Full Text | CrossRef Full Text | Google Scholar
Rensing, C., Newby, D. T., and Pepper, I. L. (2002). The role of selective pressure and selfish DNA in horizontal gene transfer and soil microbial community adaptation. Soil Biol. Biochem. 34, 285–296. doi: 10.1016/S0038-0717(01)00183-3
Reuland, E. A., Al, N. N., Raadsen, S. A., Savelkoul, P. H., Kluytmans, J. A., and Vandenbroucke-Grauls, C. M. (2014). Prevalence of ESBL-producing Enterobacteriaceae in raw vegetables. Eur. J. Clin. Microbiol. Infect. Dis. 33, 1843–1846. doi: 10.1007/s10096-014-2142-7
PubMed Abstract | Full Text | CrossRef Full Text | Google Scholar
Riber, L., Poulsen, P. H., Al-Soud, W. A., Skov Hansen, L. B., Bergmark, L., Brejnrod, A., et al. (2014). Exploring the immediate and long-term impact on bacterial communities in soil amended with animal and urban organic waste fertilizers using pyrosequencing and screening for horizontal transfer of antibiotic resistance. FEMS Microbiol. Ecol. 90, 206–224. doi: 10.1111/1574-6941.12403
PubMed Abstract | Full Text | CrossRef Full Text | Google Scholar
Ruimy, R., Brisabois, A., Bernede, C., Skurnik, D., Barnat, S., Arlet, G., et al. (2010). Organic and conventional fruits and vegetables contain equivalent counts of Gram-negative bacteria expressing resistance to antibacterial agents. Environ. Microbiol. 12, 608–615. doi: 10.1111/j.1462-2920.2009.02100.x
PubMed Abstract | Full Text | CrossRef Full Text | Google Scholar
Sengeløv, G., Agersø, Y., Halling-Sørensen, B., Baloda, S. B., Andersen, J. S., and Jensen, L. B. (2003). Bacterial antibiotic resistance levels in Danish farmland as a result of treatment with pig manure slurry. Environ. Int. 28, 587–595. doi: 10.1016/S0160-4120(02)00084-3
PubMed Abstract | Full Text | CrossRef Full Text | Google Scholar
Shaheen, B. W. R., Nayak, S. L., Foley, O., Kweon, J., Deck, M., Boothe, et al. (2011). Molecular characterization of resistance to extended-spectrum cephalosporins in clinical Escherichia coli isolates from companion animals in the United States. Antimicrob. Agents Chemother. 55, 5666–5675. doi: 10.1128/AAC.00656-11
Smet, A., Martel, A., Persoons, D., Dewulf, J., Heyndrickx, M., Catry, B., et al. (2008). Diversity of extended-spectrum beta-lactamases and class C beta-lactamases among cloacal Escherichia coli Isolates in Belgian broiler farms. Antimicrob. Agents Chemother. 52, 1238–1243. doi: 10.1128/AAC.01285-07
PubMed Abstract | Full Text | CrossRef Full Text | Google Scholar
Tate, K. W., Atwill, E. R., George, M. R., McDougald, N. K., and Larsen, R. E. (2000). Cryptosporidium parvum transport from cattle fecal deposits on California rangelands. J. Range Manage. 53, 295–299. doi: 10.2307/4003435
Venglovsky, J., Sasakova, N., and Placha, I. (2009). Pathogens and antibiotic residues in animal manures and hygienic and ecological risks related to subsequent land application. Bioresour. Technol. 100, 5386–5391. doi: 10.1016/j.biortech.2009.03.068
PubMed Abstract | Full Text | CrossRef Full Text | Google Scholar
Von Salviati, C., Laube, H., Guerra, B., Roesler, U., and Friese, A. (2015). Emission of ESBL/AmpC producing Escherichia coli from pig fattening farms to surrounding areas. Vet. Microbiol. 30, 77–84.
Walsh, F., and Duffy, B. (2013). The culturable soil antibiotic resistome: a community of multi-drug resistant bacteria. PLoS ONE 8:e65567. doi: 10.1371/journal.pone.0065567
PubMed Abstract | Full Text | CrossRef Full Text | Google Scholar
Yuan, W., Chai, T. J., and Miao, Z. M. (2010). ERIC-PCR identification of the spread of airborne Escherichia coli in pig houses. Sci. Total Environ. 408, 1446–1450. doi: 10.1016/j.scitotenv.2009.12.019
PubMed Abstract | Full Text | CrossRef Full Text | Google Scholar
Zhang, H., Zhou, Y., Guo, S., and Chang, W. (2015). Multidrug resistance found in extended-spectrum beta-lactamase-producing Enterobacteriaceae from rural water reservoirs in Guantao, China. Front. Microbiol. 6:267. doi: 10.3389/fmicb.2015.00267
Zhang, J., Zheng, B., Zhao, L., Wei, Z., Ji, J., Li, L., et al. (2014). Nationwide high prevalence of CTX-M and an increase of CTX-M-55 in Escherichia coli isolated from patients with community-onset infections in Chinese county hospitals. BMC Infect. Dis. 14, 659. doi: 10.1186/s12879-014-0659-0
PubMed Abstract | Full Text | CrossRef Full Text | Google Scholar
Zhao, S., White, D. G., McDermott, P. F., Friedman, S., English, L., Ayers, S., et al. (2001). Identification and expression of cephamycinase bla(CMY) genes in Escherichia coli and Salmonella isolates from food animals and ground meat. Antimicrob. Agents Chemother. 45, 3647–3650. doi: 10.1128/AAC.45.12.3647-3650.2001
PubMed Abstract | Full Text | CrossRef Full Text | Google Scholar
Zheng, H., Zeng, Z., Chen, S., Liu, Y., Yao, Q., Deng, Y., et al. (2012). Prevalence and characterisation of CTX-M beta-lactamases amongst Escherichia coli isolates from healthy food animals in China. Int. J. Antimicrob. Agents 39, 305–310. doi: 10.1016/j.ijantimicag.2011.12.001
PubMed Abstract | Full Text | CrossRef Full Text | Google Scholar
Zhou, Z., Raskin, L., and Zilles, J. L. (2010). Effects of Swine manure on macrolide, lincosamide, and streptogramin B antimicrobial resistance in soils. Appl. Environ. Microbiol. 76, 2218–2224. doi: 10.1128/AEM.02183-09
PubMed Abstract | Full Text | CrossRef Full Text | Google Scholar
Zhu, Y. G., Johnson, T. A., Su, J. Q., Qiao, M., Guo, G. X., Stedtfeld, R. D., et al. (2013). Diverse and abundant antibiotic resistance genes in Chinese swine farms. Proc. Natl. Acad. Sci. U.S.A. 110, 3435–3440. doi: 10.1073/pnas.1222743110
PubMed Abstract | Full Text | CrossRef Full Text | Google Scholar
Keywords: ESBL-producing E. coli, animal manure, ERIC-PCR, agricultural fields, antibiotic resistance gene spread
Citation: Gao L, Hu J, Zhang X, Wei L, Li S, Miao Z and Chai T (2015) Application of swine manure on agricultural fields contributes to extended-spectrum β-lactamase-producing Escherichia coli spread in Tai'an, China. Front. Microbiol. 6:313. doi: 10.3389/fmicb.2015.00313
Received: 06 December 2014; Accepted: 29 March 2015;
Published: 14 April 2015.
Edited by:
Ghassan M. Matar, American University of Beirut, LebanonReviewed by:
Yuji Morita, Aichi Gakuin University, JapanMarco Rinaldo Oggioni, University of Leicester, UK
Copyright © 2015 Gao, Hu, Zhang, Wei, Li, Miao and Chai. This is an open-access article distributed under the terms of the Creative Commons Attribution License (CC BY). The use, distribution or reproduction in other forums is permitted, provided the original author(s) or licensor are credited and that the original publication in this journal is cited, in accordance with accepted academic practice. No use, distribution or reproduction is permitted which does not comply with these terms.
*Correspondence: Zengmin Miao, College of Life Sciences, Taishan Medical University, Changcheng Road 619, Tai'an 271000, ChinaemVuZ21pbm1pYW9AMTI2LmNvbQ==;
Tongjie Chai, College of Animal Science and Veterinary Medicine, Shandong Agricultural University, Daizong Road 61, Tai'an 271000, ChinaY2hhaXRqMTE3QDE2My5jb20=