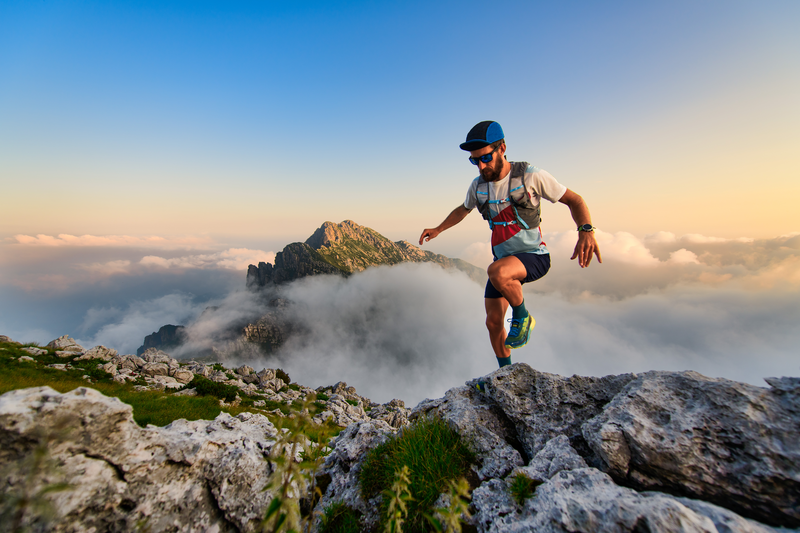
94% of researchers rate our articles as excellent or good
Learn more about the work of our research integrity team to safeguard the quality of each article we publish.
Find out more
ORIGINAL RESEARCH article
Front. Microbiol. , 18 June 2012
Sec. Antimicrobials, Resistance and Chemotherapy
Volume 3 - 2012 | https://doi.org/10.3389/fmicb.2012.00220
This article is part of the Research Topic Antimicrobial compounds from natural sources View all 14 articles
Candida albicans forms oral biofilms that cause disease and are difficult to treat with conventional antifungal agents. Tea tree oil (TTO) is a natural compound with reported antimicrobial and immunomodulatory activities. The aims of the study were to evaluate the antifungal efficacy of TTO and key derivatives against C. albicans biofilms, to assess the toxicological effects of TTO on a clinically relevant oral cell line, and to investigate its impact on inflammation. TTO and its derivatives were examined against 100 clinical strains of C. albicans. Planktonic minimum inhibitory concentrations (MICs) were determined using the CLSI M-27A broth microdilution method. Sessile MICs were determined using an XTT reduction assay. Inhibition, time-kill, and mode of action studies were performed. OKF6-TERT2 epithelial cells were used for cytotoxicity and cytokine expression assays. Planktonic C. albicans isolates were susceptible to TTO, terpinen-4-ol (T-4-ol), and α-terpineol, with an MIC50 of 0.5, 0.25, and 0.25%, respectively. These three compounds also displayed potent activity against the 69 biofilm-forming strains, of which T-4-ol and α-terpineol displayed rapid kill kinetics. For all three compounds, 1 × MIC50 effectively inhibited biofilm growth when C. albicans were treated at 0, 1, and 2 h post adhesion. By scanning electron microscopy analysis and PI uptake, TTO and derivative components were shown to be cell membrane active. TTO and T-4-ol were cytotoxic at 1 × MIC50, whereas at 0.5 × MIC50 T-4-ol displayed no significant toxicity. Transcript and protein analysis showed a reduction of IL-8 when treated with TTO and T-4-ol. These data provide further in vitro evidence that TTO and its derivative components, specifically T-4-ol, exhibit strong antimicrobial properties against fungal biofilms. T-4-ol has safety advantages over the complete essential oil and may be suitable for prophylaxis and treatment of established oropharyngeal candidosis. A clinical trial of T-4-ol is worthy of consideration.
Many cancer therapies have a profound negative impact on oral health, causing serious complications (Dreizen, 1990). These include both non-infectious and infectious side-effects with potential for systemic spread (Wingard, 1990). Oral fungal infections, caused predominantly by Candida species, are common in patients suffering from cancer at all stages of the disease, especially those receiving palliative care (Sweeney et al., 1998; Sweeney and Bagg, 2000; Davies et al., 2006). Candida albicans is known to form complex biofilms (Ramage et al., 2005; Ganguly and Mitchell, 2011) and other Candida spp. also have this ability to varying degrees, though it is strain-dependent (Silva et al., 2009). Such biofilms form on the oral epithelium or on the surfaces of intra-oral prostheses (Williams et al., 2011), and can result in pseudomembranous or erythematous candidosis.
The effectiveness of most antifungal agents is significantly reduced if yeasts are in a biofilm as opposed to the planktonic state (Kuhn and Ghannoum, 2004; Lamfon et al., 2004; d’Enfert, 2006). Furthermore, in recent years there has been growing concern about the increasing prevalence of infections caused by yeasts that are resistant to commonly used antifungal drugs (Bagg et al., 2003), with the emergence of strains of C. albicans that are resistant to the azole antifungals (Casalinuovo et al., 2004), in addition to the inherently reduced drug susceptibility of many non-albicans yeasts that can be selected through over-use of antifungal drugs (Davies et al., 2002; Bagg et al., 2003). There is a need to identify new methods of preventing and treating oral candidosis among immunocompromised patients, including those with cancer, both to improve treatment of established infections and to limit further development of drug resistance. Given the extensive evolutionary interaction that exists between plants and microorganisms it is unlikely that resistance would be cause for concern, nevertheless, clinically we would anticipate natural compounds to augment existing antifungal agents opposed to direct replacement.
One agent which merits consideration is tea tree oil (TTO; Hartford and Zug, 2005; Carson et al., 2006), which has recently reported to have minimal impact on developing resistance (Hammer et al., 2012). TTO is produced as a distillate of leaves of the Melaleuca alternifolia shrub, which grows in New South Wales, Australia. It is a complex mixture of essential oils, comprising approximately 100 components, most of which are monoterpenes, sesquiterpenes, and their related alcohols (Carson et al., 2006). TTO has been shown to possess a number of therapeutic properties, including anti-inflammatory activities (Hart et al., 2000; Koh et al., 2002; Pearce et al., 2005) and there is current interest in its possible anti-tumor properties (Bozzuto et al., 2011). However, it is best known for its antimicrobial activity against a wide spectrum of microorganisms, for example Staphylococcus aureus (including MRSA; Thompson et al., 2008; Kwiecinski et al., 2009), a range of oral bacteria (Hammer et al., 2003b), and certain viruses, including herpes simplex and influenza viruses (Carson et al., 2001; Garozzo et al., 2011). TTO also has potent activity against many fungi (Hammer et al., 2003a, 2004), including some azole-resistant yeasts (Mondello et al., 2003; Bagg et al., 2006) and there is some evidence for its efficacy in treating fluconazole refractory oral candidosis in AIDS patients. This raises the possibility of using TTO preparations for the prevention and treatment of oral candidal infections. Oral care products are now available containing TTO (Soukoulis and Hirsch, 2004), including an alcohol-free mouthwash. However, hypersensitivity reactions to TTO have been reported (Knight and Hausen, 1994; Mozelsio et al., 2003; Hammer et al., 2006; Rutherford et al., 2007) and the palatability of the agent as an oral preparation is poor. Moreover, given the complex chemical composition of TTO, which results in batch-to-batch variability, then the ability to accurately interpret its clinical utility is limited. Some of the individual components of TTO are believed to have antimicrobial properties (Mondello et al., 2006) and may be more appropriate for development into oral care products with respect to safety and consistency.
This study had three aims. The first was to evaluate the efficacy of TTO and two of its key derivatives [terpinen-4-ol (T-4-ol) and α-terpineol] against biofilms formed by a clinically diverse panel of C. albicans isolates. Secondly, the toxicological effects of TTO and derivative components were assessed by means of a clinically relevant oral cell line. Finally, the study aimed to investigate further the previously reported anti-inflammatory effects of TTO.
One-hundred clinical strains of C. albicans were used in the course of this study, which were isolated from a wide variety of patient groups, including those with denture stomatitis (Glasgow Dental School, n = 26), those receiving palliative care for advanced cancer (Accord Hospice, Paisley, n = 30), neonates (Royal Hospital for Sick Children, Glasgow, n = 36), and those with bloodstream infections (Glasgow Royal Infirmary, n = 8). All strains were maintained routinely on Sabouraud dextrose (SAB) agar (Oxoid, Basingstoke, UK) stored at 4°C, and stored indefinitely on Microbank beads (Prolab Diagnostics) at −80°C.
Tea tree oil and seven HPLC-grade derivatives of the oil obtained from Sigma-Aldrich (Poole, Dorset) were used in the study. The derivatives included terpinen-4-ol (T-4-ol), α-terpineol, 1-8-cineole, terpinolene, α-terpinene, γ-terpinene, and p-cymene. Stock concentrations of each component were prepared in RPMI-1640 AutoMod™ medium (Sigma, UK), containing 0.5% (v/v) Tween® 80 (ICI Americas, Inc.). Each compound was prepared as a 4% v/v solution for testing and serially diluted for both planktonic and sessile susceptibility testing. All procedures were carried out in a laminar flow cabinet (Hera Safe laminar flow cabinet, Kendro, Model K515).
Antifungal testing to determine planktonic minimum inhibitory concentrations (PMICs) was performed using the CLSI M-27A broth microdilution method (Clinical Laboratory Standards Institute, 2008). For sessile susceptibility testing, C. albicans biofilms were formed as previously described on polystyrene, flat-bottomed, 96-well microtiter plates (Corning Incorporated, Corning, NY, USA; Ramage et al., 2001). These were treated with a range of concentrations of each antifungal compound (0.0625–2%) in RPMI-1640 containing 0.5% (v/v) TWEEN 80®. After 24 h challenge the sessile minimum fungicidal concentrations (SMFCs) were determined as a 50% inhibition compared to the untreated control using a XTT reduction assay, as described previously (Ramage et al., 2001). For time-kill studies C. albicans biofilms (n = 3) were grown for 24 h, then exposed to TTO, T-4-ol, or α-terpineol at a concentration of 2 × MIC90 for 2, 5, 15, and 60 min. Metabolic activity was quantified by the XTT assay and biofilm viability assessed as a relative reduction in absorbance in comparison to untreated controls. For inhibition, C. albicans isolates (n = 4) were plated in 96-well microtiter plates for biofilm growth (1 × 106 cells/ml) then treated at 0, 1, 2, or 4 h after adhesion with TTO, T-4-ol, and α-terpineol at 0.5 and 1 × MIC50. The cells were then incubated for a further 24 h at 37°C. The levels of biofilm inhibition were compared to an untreated control using a crystal violet biomass assay, as previously described (Mowat et al., 2007). All experiments outlined above were performed on two independent occasions with a minimum of three clinical isolates in triplicate.
We investigated whether TTO or T-4-ol interacted with the membrane using a propidium iodide (PI) uptake assay, as previously reported (Sherry et al., 2012). PI is a fluorescent dye that diffuses through the membranes of dead cells and is incorporated within nuclear DNA, therefore increased fluorescence directly correlates with reduced membrane integrity. C. albicans (3153A) was grown in an overnight broth as described previously, and standardized to 1 × 107 cells/ml in RPMI-1640 and treated with TTO, T-4-ol, or α-terpineol at a concentration of 2 × MIC50 for 10, 20, 30, 40, 50, and 60 min. Following treatment, cells were centrifuged at 10,000 rpm to remove the compounds, washed with PBS, stained with 20 μM of propidium iodide (PI; Sigma-Aldrich, UK), and incubated at 37°C for 15 min to allow the dye to bind to DNA. One-hundred microliters of each sample were transferred to a black microtiter plate (Corning Incorporated, NY, USA) and fluorescence measured using a microtiter plate reader (FluoStar Omega, BMG Labtech) at excitation and emission wavelengths 535/617 nm, respectively. Raw data were corrected for background fluorescence and the assay was carried out in triplicate. In addition, the physical effect of these compounds was assessed microscopically using scanning electron microscopy (SEM). C. albicans was adhered to Thermanox™ coverslips (Nunc Inc., Thermo Fisher Scientific) for 2 h within 24 well tissue culture plates then treated with TTO and T-4-ol at 2 × MIC50 for 24 h. The cells were fixed using 2% w/v para-formaldehyde, 2% v/v glutaraldehyde, 0.15 M sodium cacodylate, and 0.15% Alcian Blue (pH 7.4), and prepared for SEM as previously described (Erlandsen et al., 2004). The fixed and dried biofilm samples were sputter-coated with gold and viewed under a JEOL JSM-6400 scanning electron microscope.
OKF6-TERT2 epithelial cells were used as previously described (Ramage et al., 2012). This is an immortalized human oral keratinocyte cell line provided by the Rheinwald Laboratory (Brigham and Woman’s Hospital, Boston). These cells were immortalized by forced expression of telomerase, and resemble primary oral keratinocytes in studies of cytotoxicity or inducible cytokine and beta-defensin expression (Dongari-Bagtzoglou and Kashleva, 2003). The cells were cultured in keratinocyte serum-free medium (KSFM) supplemented with 100 IU penicillin, 100 μg/ml streptomycin, 25 μg/ml bovine pituitary extract (BPE), 0.2 ng/ml epidermal growth factor (EGF), and 0.3 mM CaCl2 (0.4 mM total Ca2+). Primary periradicular fibroblasts (PRF, kind gift of Professor Colin Murray, University of Glasgow) were cultured in standard Dulbecco’s Modified Eagles Medium (DMEM) with 10% fetal calf serum (FCS), 2 mM L-glutamine, 100 IU penicillin, and 100 μg/ml streptomycin. Both cell lines were passaged at approximately 90% confluence, using 0.05% Trypsin EDTA. Experiments were used with cells between passages 5 and 10.
TTO and T-4-ol dilutions were prepared in KSFM or DMEM with 0.25% (v/v) Tween® 80 for OKF6 or PRF cells, respectively. To assess cytotoxicity, cells were seeded at 1 × 105 cells per well per ml in KSFM or DMEM in a 24 well culture plate and grown until 90–100% confluent. Medium was then removed and cells exposed to 0.5 and 1 × MIC50 of each compound for 2 min, then cells were washed gently (×3) in Hanks balanced salt solution. The viability of the cells was assessed using an XTT assay (0.25 mg/ml XTT and 1μM of menadione in KSFM or DMEM), in which the cells were incubated in 5% CO2 at 37°C for 2 h, and the absorbance quantified at 490 nm in an automated microtiter plate reader (Tecan Sunrise, Jencons, UK). Viability was calculated based on unexposed control cells.
OKF6 cells were grown in 12 well tissue culture trays were seeded and grown as described above and treated with TTO and T-4-ol at 0.5 × MIC50 for 2 min, washed then stimulated with zymosan (50 μg/ml). Media and zymosan controls were also included. Supernatants were collected after 4 and 24 h and RNA extracted using TRIzol® according to the manufacturer’s instructions (Invitrogen, Paisley, Scotland). Total RNA was treated with DNase I (Promega, Southampton, UK) at 37°C for 30 min. RNA quality and quantity was assessed using a NanoDrop™ spectrophotometer ND-1000 (Labtech International Ltd., Ringmer, East Sussex, UK), prior to cDNA production using Superscript II Reverse Transcriptase (Invitrogen). qPCR was then performed using an MxPro MX3000P Quantitative PCR machine (Stratagene, Amsterdam, Netherlands) with the following primers: IL-8 (F: 5′-CAGAGACAGCAGAGCACACAA-3′; R: 5′-TTAGCACTCCTTGGCAAAAC-3′; 170 bp), and the housekeeping gene GAPDH (F: 5′-GGTGGTGAAGACGCCAGT-3′; R: 5′-CAAGGCTGAGAACGGGAAG-3′). Each duplicate PCR reaction contained the following: 0.5 μl of 1 μg/μl of cDNA, 0.5 μl forward and reverse primer (10 nmol), 0.25 μl ROX reference dye, 12.5 μl SYBR® Green (Invitrogen), and 10.5 μl dH2O. PCR reaction conditions were as follows: 95°C – 10 min, 40× (94°C – 30 s, 58°C –30 s, 72°C – 30 s) and 72°C – 10 min. Analysis was performed using the Mx3000P software (Stratagene) and gene expression normalized to β-tublin gene according to the 2−ΔΔCT method. To assess IL-8 protein levels an ELISA was performed on retained supernatants. ELISA kits (R&D Systems, Abingdon, UK) were used in accordance with the manufacturer’s instructions. All assays were optimized and validated prior to use, with standards and samples performed in at least duplicate.
For assessing the statistical significance of observed changes in metabolic activity and biomass of C. albicans and IL-8 protein levels in oral keratinocyte culture fluids (data that conformed to a near normal distribution) a one-way ANOVA with Bonferroni multiple comparison post-test was performed. ANOVA and post hoc tests were also used on natural log transformations (to normalize) of proportional data for the analysis of IL-8 mRNA abundance in these cells. p < 0.05 was considered significant. The analyses were performed using GraphPad Prism version 4.0 for Windows (GraphPad Software, CA, USA).
The planktonic C. albicans isolates tested were susceptible to TTO, T-4-ol, and α-terpineol, with an MIC50 of 0.5, 0.25, and 0.25%, respectively, and an MIC90 of 1.0, 0.5, and 0.5%, respectively (Table 1). There was minimal variation in susceptibility as determined by the concentration ranges for the 100 strains tested. These three compounds also displayed potent activity against the 69 strains capable of forming biofilms, with the SMIC50 for T-4-ol and α-terpineol showing no change when compared to median effective planktonic concentration (SMFC50 = 0.25%). However, the concentration of TTO required to show a similar effect was double that required to inhibit planktonic cells (SMFC50 = 1%). All other compounds tested showed relatively poor activity against the isolates tested.
The biofilm rate of kill for the three most effective components (TTO, T-4-ol, and α-terpineol) at 2 × SMIC50 was performed, where time dependent killing was observed (Figure 1). TTO was shown to reduce the viability of the biofilms within 2 min by 44%, but after 5, 15, and 60 min this had declined only slightly to 46, 56, and 65%, respectively. However, both T-4-ol and α-terpineol displayed comparable and more effective killing, reducing the viability rapidly after 2 min by approximately 55%. This anti-biofilm activity remained, reducing biofilm viability by approximately 61, 75, and 94% after 5, 15, and 60 min, respectively. Both T-4-ol and α-terpineol showed significantly better activity than TTO only at 60 min (p < 0.001).
Figure 1. Candida albicans biofilm killing by TTO, T-4-ol, and α-terpineol is time dependent. Standardized C. albicans (1 × 106 cells/ml) were incubated in flat-bottomed 96-well plates for 24 h, washed in PBS, and treated with 2 × SMIC50 at 2, 5, 10, 15, and 60 min. Metabolic activity of treated biofilms was then quantified using the XTT assay by reading at 492 nm in a microtiter plate reader (FluoStar Omega, BMG Labtech). Three isolates were used for each assay, and this was performed on two independent occasions in triplicate. **p < 0.001. Error bars represent the ±standard error of the mean.
Inhibition of biofilm formation was assessed for these three compounds at 0.5 and 1 × MIC50 by assessing biofilm biomass. It was shown for all compounds that 1 × MIC50 was able to inhibit biofilm growth effectively when treated at 0, 1, and 2 h post adhesion (8.5−12% biomass compared to control). At 4 h post adhesion, by which time cells had began to form hyphae, treatment with TTO, T-4-ol, and α-terpineol further suppressed biofilm proliferation by 71, 74, and 82% that of the control (Figure 2A). No significant differences were observed between the treatments at each time point. Treatment with 0.5 × MIC50 was overall less effective at inhibiting biofilm growth most notably with TTO, which displayed a time dependent reduction in inhibition, showing a 44% inhibition of cells treated at 4 h (Figure 2B). Both T-4-ol and α-terpineol were significantly more effective against 1 and 2 h cells (p < 0.05), inhibiting biofilm formation by >80%. However, this inhibition was reduced against the 4 h cells (54 and 58%, respectively).
Figure 2. Candida albicans biofilm development is inhibited by TTO, T-4-ol, and α-terpineol. Standardized C. albicans (1 × 106 cells/ml) were added to a flat-bottomed 96-well plate and treated with (A) 0.5 and (B) 1 × SMIC50 for 0, 1, 2, and 4 h post incubation. Biofilms were subsequently allowed to develop for 24 h. Resultant biofilms were quantified using a crystal violet assay read at 570 nm in a microtiter plate reader (FluoStar Omega, BMG Labtech). Four isolates were used for each assay, and this was performed on two independent occasions in triplicate. *p < 0.05. Error bars represent the ±standard error of the mean.
Scanning electron microscopy analysis of TTO and T-4-ol treated cells was performed. It was noted that compared to the control (untreated) cells (Figure 3Ai), both compounds had ruptured the cells, allowing the cell contents to leak out, giving a punctured appearance (Figures 3Aii,iii). The cell damage was shown to be more extensive for T-4-ol treated cells. Given this appearance we hypothesized that cell membrane integrity had been compromised. We therefore undertook PI uptake experiments, as previously reported (Sherry et al., 2012). For TTO, PI uptake was shown to be relatively slow, with maximal fluorescence obtained at 30 min (Figure 3B). In comparison, for T-4-ol fluorescence was shown to increase in a time dependent manner up to 40 min, twice that of TTO, after which time this reached a plateau. These data show similar kinetics to the time-kill data presented.
Figure 3. Tea tree oil and T-4-ol are cell membrane active. (A) Adherent cells (3153A) were adhered for 2 h on Thermanox™ coverslips and were (i) untreated (control), or treated with (ii) TTO, or (iii) T-4-ol at 2 × MIC50 for 24 h. These were then processed and viewed on an SEM. (B) C. albicans (3153A) planktonic cells (5 × 107 cells/ml) were treated with TTO, T-4-ol, or α-terpineol at a concentration of 2 × MIC50 for 10, 20, 30, 40, 50, and 60 min. The cells were washed by centrifugation, resuspended in PI (20 μM in PBS), and incubated for 15 min at 37°C. These were then transferred to a black 96-well plate for quantification in a fluorescent plate reader (Ex485/Em620). Each assay was performed on at least two independent occasions in triplicate. Error bars represent the ±standard error of the mean.
The effect of a short exposure (2 min) of TTO and T-4-ol on cellular toxicity was investigated. It was shown that TTO and T-4-ol were toxic to both fibroblast and epithelial cells at 1 × MIC50, reducing the viability of PRF to approximately 12% and OKF6 cells to 35 and 15%, respectively (Figure 4A). At 0.5 × MIC50 T-4-ol displayed no toxicity (98% viable) against PRF compared with TTO (63% viable). Both 0.5× T-4-ol and 0.5× TTO were non-toxic to OKF6 cells (>100% viable).
Figure 4. Tea tree oil and T-4-ol are biologically active against mammalian cells. (A) PR fibroblast and OKF6 epithelial cells were grown until 90–100% confluent. These were exposed to 0.5 and 1 × MIC50 of each compound for 2 min. Cell viability was assessed using an XTT assay after incubation in 5% CO2 at 37°C for 2 h. Viability was calculated based on unexposed control cells. For cytokine studies OKF6 cells were grown in 12 well tissue culture trays treated with TTO and T-4-ol at 0.5 × MIC50 for 2 min, washed then stimulated with zymosan (50 μg/ml). Cells were processed for (B) qPCR analysis and (C) supernatant was processed for protein analysis of IL-8. Each assay was performed on three independent occasions in triplicate. *p < 0.05. Error bars represent the ±standard error of the mean.
Given that the concentration of 1 × MIC50 is cytotoxic, all subsequent work was performed with 0.5 × MIC50. Transcriptional expression of IL-8 was assessed by qPCR, using zymosan as a potent cell inflammatory agonist. Generally, greater IL-8 mRNA levels were observed at 4 h compared to 24 h. TTO and T-4-ol pre-treatment of the cells had no effect on the induction of the IL-8 gene when compared to the control (Figure 4B). In zymosan-stimulated cells pre-treatment with TTO did not reduce IL-8 expression significantly at either 4 or 24 h (p > 0.05). Although T-4-ol showed up to a twofold reduction in IL-8 expression in cells at both 4 and 24 h, though this reduction was not statistically significant (p > 0.05).
Analysis of IL-8 protein levels after 4 and 24 h stimulation with zymosan showed that neither TTO or T-4-ol were able to significantly affect IL-8 levels in culture fluids (p > 0.05) of untreated and zymosan-stimulated oral keratinocytes (data shown for zymosan only). Nevertheless, a significant (50%) reduction in IL-8 expression was observed in culture fluids of TTO treated zymosan-stimulated cells (p < 0.05), and a modest reduction of approximately 14% of IL-8 was noted for T-4-ol treated zymosan-stimulated cells (Figure 4C). These compounds had no effect on the IL-8 ELISA (data not shown).
Increasing use of conventional antifungal agents, such as azoles, in parallel with larger groups of susceptible individuals (aging population and more common immunosuppressive therapies) has resulted in the emergence of multidrug-resistant Candida strains (Sanglard and Odds, 2002; Akins, 2005; Cannon et al., 2007; Niimi et al., 2010). Non-compliance due to toxic side-effects and palatability may also exacerbate this escalating trend. Consequently, there is a demand for novel therapies to manage these infections. Our current and previous data investigating the effect of TTO on a wide variety of yeast species clearly demonstrate that it is effective against C. albicans planktonic and biofilm cells (Bagg et al., 2006).
Given the complex chemical composition of TTO we aimed to investigate key individual components to assess specific activities. T-4-ol and α-terpineol exhibited the greatest and comparable antifungal activity against both planktonic and sessile cells, which has been confirmed from reports elsewhere (Hammer et al., 2003a; Mondello et al., 2006; Terzi et al., 2007). Moreover, time-kill studies showed a rapid and sustained level of activity of both compounds, significantly superior to TTO at 2 × MIC90 after 60 min. This superior activity was less evident during the early biofilm inhibition studies (0–2 h), however, at 4 h TTO showed a significant decline in its ability to inhibit biofilm growth. Both T-4-ol and α-terpineol have hydroxyl groups in their chemical structures, making them moderately water-soluble. This allows them to diffuse through water, enter, and destabilize cell membranes, resulting in osmotic shock (Straede et al., 2007). This was evident from analysis of cell membrane integrity using a PI uptake assay and microscopic examination by SEM.
T-4-ol and α-terpineol both showed excellent activity, but the remainder of the studies compared TTO with T-4-ol, primarily because of the high bioavailability of the latter within TTO and its overall antifungal profile (Carson et al., 2006). Cytotoxicity was observed at MIC50 levels for both TTO and T-4-ol, whereas at 0.5 × MIC50 (concentrations able to inhibit C. albicans growth) OKF6 cells remained viable. Previous reports have demonstrated varying levels of cytotoxicity to primary fibroblasts and primary epithelial cells, with a 1 h exposure to 0.03%TTO shown to be toxic (Soderberg et al., 1996). Further reports indicated that a 4 h exposure to 0.28% TTO was required to inhibit the HeLa epithelial cell line (Hayes et al., 1997). In addition, it was shown previously that TTO was highly toxic to monocytes and neutrophils, but after a prolonged 20 h exposure (Hart et al., 2000). The relevance of this length of exposure is difficult to interpret. Collectively, these studies highlight the importance of the cell line being tested and in what context. Given that our primary interests lay in developing a mouthwash to prevent candidal growth as opposed to treating an active infection, a 2 min exposure of sub-inhibitory concentrations was deemed optimal.
These data were used to assess the impact of TTO-based compounds on inflammation, using IL-8 as a biomarker. Both transcriptional and protein analysis showed that IL-8 was regulated by both compounds, with TTO showing protein reduction of approximately 50% 24 h post treatment, whereas T-4-ol only caused a 14% reduction. However, at 4 h post treatment T-4-ol inhibited IL-8 protein production by 53% opposed to 16% for TTO, although neither was significant. The differences observed may be accounted for by the quantitative composition of TTO, which contains approximately 40% T-4-ol. A previous study of LPS stimulated monocytes demonstrated a significant IL-8 suppression by 0.052% T-4-ol after 40 h (Hart et al., 2000). The discordance with our data can be explained through differences in concentration and exposure time. Irrespective, both studies indicate that these molecules have the potential capacity to suppress inflammatory mediators, which are common within the oral cavity of OPC sufferers. Indeed, there is in vivo evidence to support this. Several murine studies have shown inhibitory effects on inflammatory processes, including reduced contact hypersensitivity (Brand et al., 2002a), reduced histamine-induced edema (Brand et al., 2002b), and blocking of zymosan-induced inflammation by inhaled TTO (Golab and Skwarlo-Sonta, 2007). In humans, nickel-induced contact hypersensitivity has been reduced by the topical application of 100% TTO (Pearce et al., 2005). Both TTO (Koh et al., 2002) and T-4-ol (Khalil et al., 2004) have also been shown to reduce histamine-induced weal and flare reaction in human skin. Conversely, other studies have implicated TTO as being pro-inflammatory (de Groot and Weyland, 1992; Rutherford et al., 2007). Given the apparent contradictory reports in the literature it seems prudent to focus on utilizing the most biologically active and abundant compound from TTO, i.e., T-4-ol. This will enable investigators to determine accurately the medicinal benefits of pure T-4-ol and exclude deleterious effects caused by the other terpenes that comprise TTO.
In summary, these studies have added to the body of in vitro evidence indicating that TTO and some of its individual components, specifically T-4-ol, exhibit strong antimicrobial efficacy against fungal biofilms. Furthermore, this has also demonstrated a potential biofilm inhibiting activity, suggesting that this agent may be suitable for use in prophylactic oral hygiene products such as mouth rinses and denture cleansers, as well as treatment for established OPC infections. The use of T-4-ol, a single component from TTO, has advantages over the complete essential oil in terms of product safety and consistency. Oral candidosis is a continuing problem for cancer patients, as well as other groups of immunocompromised hosts. In the face of increasing resistance to azoles and other established antifungal drugs, the need for novel preventive and therapeutic agents has never been greater. The weight of laboratory data that has now accumulated and the anecdotal reports of clinical efficacy suggest that clinical trials of TTO components, particularly T-4-ol, would be merited.
The authors declare that the research was conducted in the absence of any commercial or financial relationships that could be construed as a potential conflict of interest.
We acknowledge the support for Gordon Ramage through the International Association for Dental Research and GlaxoSmithKline Research Fellowship.
Akins, R. A. (2005). An update on antifungal targets and mechanisms of resistance in Candida albicans. Med. Mycol. 43, 285–318.
Bagg, J., Jackson, M. S., Petrina Sweeney, M., Ramage, G., and Davies, A. N. (2006). Susceptibility to Melaleuca alternifolia (tea tree) oil of yeasts isolated from the mouths of patients with advanced cancer. Oral Oncol. 42, 487–492.
Bagg, J., Sweeney, M. P., Lewis, M. A., Jackson, M. S., Coleman, D., Al, M. A., Baxter, W., McEndrick, S., and McHugh, S. (2003). High prevalence of non-albicans yeasts and detection of anti-fungal resistance in the oral flora of patients with advanced cancer. Palliat. Med. 17, 477–481.
Bozzuto, G., Colone, M., Toccacieli, L., Stringaro, A., and Molinari, A. (2011). Tea tree oil might combat melanoma. Planta Med. 77, 54–56.
Brand, C., Grimbaldeston, M. A., Gamble, J. R., Drew, J., Finlay-Jones, J. J., and Hart, P. H. (2002a). Tea tree oil reduces the swelling associated with the efferent phase of a contact hypersensitivity response. Inflamm. Res. 51, 236–244.
Brand, C., Townley, S. L., Finlay-Jones, J. J., and Hart, P. H. (2002b). Tea tree oil reduces histamine-induced oedema in murine ears. Inflamm. Res. 51, 283–289.
Cannon, R. D., Lamping, E., Holmes, A. R., Niimi, K., Tanabe, K., Niimi, M., and Monk, B. C. (2007). Candida albicans drug resistance another way to cope with stress. Microbiology 153, 3211–3217.
Carson, C. F., Ashton, L., Dry, L., Smith, D. W., and Riley, T. V. (2001). Melaleuca alternifolia (tea tree) oil gel (6%) for the treatment of recurrent herpes labialis. J. Antimicrob. Chemother. 48, 450–451.
Carson, C. F., Hammer, K. A., and Riley, T. V. (2006). Melaleuca alternifolia (tea tree) oil: a review of antimicrobial and other medicinal properties. Clin. Microbiol. Rev. 19, 50–62.
Casalinuovo, I. A., Di Francesco, P., and Garaci, E. (2004). Fluconazole resistance in Candida albicans: a review of mechanisms. Eur. Rev. Med. Pharmacol. Sci. 8, 69–77.
Clinical Laboratory Standards Institute. (2008). Reference Method for Broth Dilution Antifungal Susceptibility Testing of Yeast, Approved Standard – 3rd Edn, CLSI document M27-A3. Wayne: CLSI.
Davies, A. N., Brailsford, S., Broadley, K., and Beighton, D. (2002). Oral yeast carriage in patients with advanced cancer. Oral Microbiol. Immunol. 17, 79–84.
Davies, A. N., Brailsford, S. R., and Beighton, D. (2006). Oral candidosis in patients with advanced cancer. Oral Oncol. 42, 698–702.
de Groot, A. C., and Weyland, J. W. (1992). Systemic contact dermatitis from tea tree oil. Contact Derm. 27, 279–280.
d’Enfert, C. (2006). Biofilms and their role in the resistance of pathogenic Candida to antifungal agents. Curr. Drug Targets 7, 465–470.
Dongari-Bagtzoglou, A., and Kashleva, H. (2003). Candida albicans triggers interleukin-8 secretion by oral epithelial cells. Microb. Pathog. 34, 169–177.
Dreizen, S. (1990). Oral complications of cancer therapies. Description and incidence of oral complications. NCI Monogr. 11–15.
Erlandsen, S. L., Kristich, C. J., Dunny, G. M., and Wells, C. L. (2004). High-resolution visualization of the microbial glycocalyx with low-voltage scanning electron microscopy: dependence on cationic dyes. J. Histochem. Cytochem. 52, 1427–1435.
Ganguly, S., and Mitchell, A. P. (2011). Mucosal biofilms of Candida albicans. Curr. Opin. Microbiol. 14, 380–385.
Garozzo, A., Timpanaro, R., Stivala, A., Bisignano, G., and Castro, A. (2011). Activity of Melaleuca alternifolia (tea tree) oil on influenza virus A/PR/8: study on the mechanism of action. Antiviral Res. 89, 83–88.
Golab, M., and Skwarlo-Sonta, K. (2007). Mechanisms involved in the anti-inflammatory action of inhaled tea tree oil in mice. Exp. Biol. Med. (Maywood) 232, 420–426.
Hammer, K. A., Carson, C. F., and Riley, T. V. (2003a). Antifungal activity of the components of Melaleuca alternifolia (tea tree) oil. J. Appl. Microbiol. 95, 853–860.
Hammer, K. A., Dry, L., Johnson, M., Michalak, E. M., Carson, C. F., and Riley, T. V. (2003b). Susceptibility of oral bacteria to Melaleuca alternifolia (tea tree) oil in vitro. Oral Microbiol. Immunol. 18, 389–392.
Hammer, K. A., Carson, C. F., and Riley, T. V. (2004). Antifungal effects of Melaleuca alternifolia (tea tree) oil and its components on Candida albicans, Candida glabrata and Saccharomyces cerevisiae. J. Antimicrob. Chemother. 53, 1081–1085.
Hammer, K. A., Carson, C. F., and Riley, T. V. (2012). Effects of Melaleuca alternifolia (tea tree) essential oil and the major monoterpene component terpinen-4-ol on the development of single- and multistep antibiotic resistance and antimicrobial susceptibility. Antimicrob. Agents Chemother. 56, 909–915.
Hammer, K. A., Carson, C. F., Riley, T. V., and Nielsen, J. B. (2006). A review of the toxicity of Melaleuca alternifolia (tea tree) oil. Food Chem. Toxicol. 44, 616–625.
Hart, P. H., Brand, C., Carson, C. F., Riley, T. V., Prager, R. H., and Finlay-Jones, J. J. (2000). Terpinen-4-ol, the main component of the essential oil of Melaleuca alternifolia (tea tree oil), suppresses inflammatory mediator production by activated human monocytes. Inflamm. Res. 49, 619–626.
Hayes, A. J., Leach, D. N., and Markham, J. L. (1997). In vitro cytotoxicity of Australian tea tree oil using human cell lines. J. Essent. Oil Res. 9, 572–582.
Khalil, Z., Pearce, A. L., Satkunanathan, N., Storer, E., Finlay-Jones, J. J., and Hart, P. H. (2004). Regulation of wheal and flare by tea tree oil: complementary human and rodent studies. J. Invest. Dermatol. 123, 683–690.
Knight, T. E., and Hausen, B. M. (1994). Melaleuca oil (tea tree oil) dermatitis. J. Am. Acad. Dermatol. 30, 423–427.
Koh, K. J., Pearce, A. L., Marshman, G., Finlay-Jones, J. J., and Hart, P. H. (2002). Tea tree oil reduces histamine-induced skin inflammation. Br. J. Dermatol. 147, 1212–1217.
Kuhn, D. M., and Ghannoum, M. A. (2004). Candida biofilms: antifungal resistance and emerging therapeutic options. Curr. Opin. Investig. Drugs 5, 186–197.
Kwiecinski, J., Eick, S., and Wojcik, K. (2009). Effects of tea tree (Melaleuca alternifolia) oil on Staphylococcus aureus in biofilms and stationary growth phase. Int. J. Antimicrob. Agents 33, 343–347.
Lamfon, H., Porter, S. R., McCullough, M., and Pratten, J. (2004). Susceptibility of Candida albicans biofilms grown in a constant depth film fermentor to chlorhexidine, fluconazole and miconazole: a longitudinal study. J. Antimicrob. Chemother. 53, 383–385.
Mondello, F., De Bernardis, F., Girolamo, A., Cassone, A., and Salvatore, G. (2006). In vivo activity of terpinen-4-ol, the main bioactive component of Melaleuca alternifolia Cheel (tea tree) oil against azole-susceptible and -resistant human pathogenic Candida species. BMC Infect. Dis. 6, 158. doi:10.1186/1471-2334-6-158
Mondello, F., De Bernardis, F., Girolamo, A., Salvatore, G., and Cassone, A. (2003). In vitro and in vivo activity of tea tree oil against azole-susceptible and -resistant human pathogenic yeasts. J. Antimicrob. Chemother. 51, 1223–1229.
Mowat, E., Butcher, J., Lang, S., Williams, C., and Ramage, G. (2007). Development of a simple model for studying the effects of antifungal agents on multicellular communities of Aspergillus fumigatus. J. Med. Microbiol. 56, 1205–1212.
Mozelsio, N. B., Harris, K. E., McGrath, K. G., and Grammer, L. C. (2003). Immediate systemic hypersensitivity reaction associated with topical application of Australian tea tree oil. Allergy Asthma Proc. 24, 73–75.
Niimi, M., Firth, N. A., and Cannon, R. D. (2010). Antifungal drug resistance of oral fungi. Odontology 98, 15–25.
Pearce, A. L., Finlay-Jones, J. J., and Hart, P. H. (2005). Reduction of nickel-induced contact hypersensitivity reactions by topical tea tree oil in humans. Inflamm. Res. 54, 22–30.
Ramage, G., Saville, S. P., Thomas, D. P., and Lopez-Ribot, J. L. (2005). Candida biofilms: an update. Eukaryot. Cell 4, 633–638.
Ramage, G., Vande Walle, K., Wickes, B. L., and Lopez-Ribot, J. L. (2001). Standardized method for in vitro antifungal susceptibility testing of Candida albicans biofilms. Antimicrob. Agents Chemother. 45, 2475–2479.
Ramage, G., Zalewska, A., Cameron, D., Sherry, L., Murray, C., Finnegan, M. B., Lowey, Z., and Jagger, D. C. (2012). A comparative in vitro study of two denture cleaning techniques as an effective strategy for inhibiting Candida albicans biofilms on denture surfaces and inducing inflammation. J. Prosthodont. doi: 10.1111/j.1532-849X.2012.00865.x
Rutherford, T., Nixon, R., Tam, M., and Tate, B. (2007). Allergy to tea tree oil: retrospective review of 41 cases with positive patch tests over 4.5 years. Australas. J. Dermatol. 48, 83–87.
Sanglard, D., and Odds, F. C. (2002). Resistance of Candida species to antifungal agents: molecular mechanisms and clinical consequences. Lancet. Infect. Dis. 2, 73–85.
Sherry, L., Jose, A., Murray, C., Williams, C., Jones, B., Millington, O., Bagg, J., and Ramage, G. (2012). Carbohydrate derived fulvic acid: an in vitro investigation of a novel membrane active antiseptic agent against Candida albicans biofilms. Front. Microbiol. 3:116. doi:10.3389/fmicb.2012.00116
Silva, S., Henriques, M., Martins, A., Oliveira, R., Williams, D., and Azeredo, J. (2009). Biofilms of non-Candida albicans Candida species: quantification, structure and matrix composition. Med. Mycol. 47, 681–689.
Soderberg, T. A., Johansson, A., and Gref, R. (1996). Toxic effects of some conifer resin acids and tea tree oil on human epithelial and fibroblast cells. Toxicology 107, 99–109.
Soukoulis, S., and Hirsch, R. (2004). The effects of a tea tree oil-containing gel on plaque and chronic gingivitis. Aust. Dent. J. 49, 78–83.
Straede, A., Corran, A., Bundy, J., and Heinisch, J. J. (2007). The effect of tea tree oil and antifungal agents on a reporter for yeast cell integrity signalling. Yeast 24, 321–334.
Sweeney, M. P., and Bagg, J. (2000). The mouth and palliative care. Am. J. Hosp. Palliat. Care 17, 118–124.
Sweeney, M. P., Bagg, J., Baxter, W. P., and Aitchison, T. C. (1998). Oral disease in terminally ill cancer patients with xerostomia. Oral Oncol. 34, 123–126.
Terzi, V., Morcia, C., Faccioli, P., Vale, G., Tacconi, G., and Malnati, M. (2007). In vitro antifungal activity of the tea tree (Melaleuca alternifolia) essential oil and its major components against plant pathogens. Lett. Appl. Microbiol. 44, 613–618.
Thompson, G., Blackwood, B., McMullan, R., Alderdice, F. A., Trinder, T. J., Lavery, G. G., and McAuley, D. F. (2008). A randomized controlled trial of tea tree oil (5%) body wash versus standard body wash to prevent colonization with methicillin-resistant Staphylococcus aureus (MRSA) in critically ill adults: research protocol. BMC Infect. Dis. 8, 161. doi:10.1186/1471-2334-8-161
Williams, D. W., Kuriyama, T., Silva, S., Malic, S., and Lewis, M. A. (2011). Candida biofilms and oral candidosis: treatment and prevention. Periodontol. 2000 55, 250–265.
Keywords: tea tree oil, oral candidosis, oral cancer, antifungal agent, terpinen-4-ol
Citation: Ramage G, Milligan S, Lappin DF, Sherry L, Sweeney P, Williams C, Bagg J and Culshaw S (2012) Antifungal, cytotoxic, and immunomodulatory properties of tea tree oil and its derivative components: potential role in management of oral candidosis in cancer patients. Front. Microbio. 3:220. doi: 10.3389/fmicb.2012.00220
Received: 02 May 2012; Paper pending published: 19 May 2012;
Accepted: 29 May 2012; Published online: 18 June 2012.
Edited by:
Pedro Ismael Da Silva Jr., Butantan Institute, BrazilCopyright: © 2012 Ramage, Milligan, Lappin, Sherry, Sweeney, Williams, Bagg and Culshaw. This is an open-access article distributed under the terms of the Creative Commons Attribution Non Commercial License, which permits non-commercial use, distribution, and reproduction in other forums, provided the original authors and source are credited.
*Correspondence: Gordon Ramage, Infection and Immunity Research Group, Glasgow Dental School, School of Medicine, College of Medical, Veterinary and Life Sciences, University of Glasgow, 378 Sauchiehall Street, Glasgow G2 3JZ, UK. e-mail:Z29yZG9uLnJhbWFnZUBnbGFzZ293LmFjLnVr
Disclaimer: All claims expressed in this article are solely those of the authors and do not necessarily represent those of their affiliated organizations, or those of the publisher, the editors and the reviewers. Any product that may be evaluated in this article or claim that may be made by its manufacturer is not guaranteed or endorsed by the publisher.
Research integrity at Frontiers
Learn more about the work of our research integrity team to safeguard the quality of each article we publish.