- 1 INSERM, U1092, Limoges, France
- 2 Université de Limoges, UMR-S1092, Limoges, France
- 3 Université de Limoges, GRESE EA4330, ENSIL, Limoges, France
The spread of antibiotic-resistant bacteria is a growing problem and a public health issue. In recent decades, various genetic mechanisms involved in the spread of resistance genes among bacteria have been identified. Integrons – genetic elements that acquire, exchange, and express genes embedded within gene cassettes (GC) – are one of these mechanisms. Integrons are widely distributed, especially in Gram-negative bacteria; they are carried by mobile genetic elements, plasmids, and transposons, which promote their spread within bacterial communities. Initially studied mainly in the clinical setting for their involvement in antibiotic resistance, their role in the environment is now an increasing focus of attention. The aim of this review is to provide an in-depth analysis of recent studies of antibiotic-resistance integrons in the environment, highlighting their potential involvement in antibiotic-resistance outside the clinical context. We will focus particularly on the impact of human activities (agriculture, industries, wastewater treatment, etc.).
Bacterial evolution has largely been shaped by the high plasticity of bacterial genomes, leading to their adaptation to most ecosystems. This ability to exchange and rearrange genomic sequences to gain new traits has been extensively demonstrated with the bacterial resistance to antibiotics. Today, the increasing rate of antibiotic resistant bacteria is a major public health issue (Davies and Davies, 2010). During the last decade, several studies have underlined the environmental resistome as a source of resistance genes of clinical interest (D’Costa et al., 2006; Aminov and Mackie, 2007; Martínez, 2008; Wright, 2010). While mutation events contribute to the bacterial adaptation, horizontal gene transfer seems to be the main cause of the rapid proliferation of antibiotic-resistance genes across a wide diversity of bacteria. Much of this horizontal gene transfers have been shown to occur in the environment (Aminov, 2011). Nevertheless, the diversity of mobile genetic elements currently described (Wozniak and Waldor, 2010; Bertels and Rainey, 2011), shows that beyond horizontal gene transfer, the loss and acquisition of functional modules are an important part in the processes of rapid bacterial adaptation and development of resistance. Integrons are one of the genetic elements involved in the adaptation of bacteria. We address the question of the involvement of integrons in the environmental spread of antibiotic resistance. More specifically, the anthropogenic impacts, which have been shown to be involved in the antibiotic-resistance spread in the environment, and the role of integrons in this process.
Integrons: Generalities
Integrons are bacterial genetic elements able to promote acquisition and expression of genes embedded within gene cassettes (GCs; Stokes and Hall, 1989). The definition of an integron is based on a functional platform (also called 5′ conserved segment, 5′CS), composed of three key elements: the intI gene, a specific recombination site attI, and a promoter, Pc (Hall and Collis, 1995; Boucher et al., 2007; Figure 1). The intI gene encodes an integrase protein IntI, which belongs to the family of tyrosine recombinases (Nunes-Düby et al., 1998).
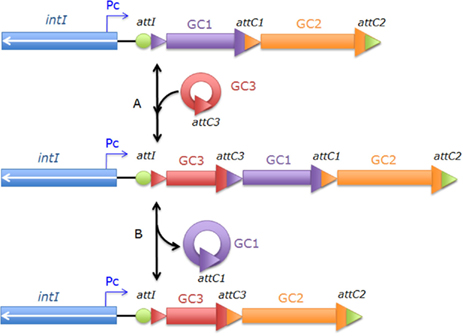
Figure 1. General organization of an integron and gene cassette (GC) recombination mechanism. The IntI1 protein catalyzes the insertion (A) and excision (B) of the GC in the integron, with GC integration occurring at the attI recombination site. In example (A), the circularized GC3 is integrated in linear form inside the integron platform via a specific recombination mechanism between the attI site and the attC3 site of the GC3. GC excision preferentially occurs between two attC sites. In example (B), the GC1 is excised following the recombination between the two attC1 and attC3 sites. Pc: gene cassette promoter; attI: integron recombination site; attC1, attC2, and attC3: attC GC recombination sites; intI: the integrase gene; GC1, GC2, GC3 are the gene cassettes, and arrows indicate the direction of coding sequences (Barraud and Ploy, 2011).
The GCs are non-replicative mobile elements, which generally couple an open reading frame (ORF) with an attC site. GCs are integrated or excised from the functional platform by a site-specific recombination mechanism catalyzed by the IntI integrase. Two types of recombination can occur (Figure 1): (i) between attI and attC sites, resulting in the insertion of GCs at the attI site, and (ii) between two attC sites, leading to excision of the GCs (Mazel, 2006). The GCs can be found either as a linear form, included in an integron, or as a covalently closed circular free intermediate (Collis and Hall, 1992). GCs are usually promoterless and require the Pc promoter for their expression as in an operon. The consequence of this system is that the last integrated cassette is the closest to the Pc promoter (Collis et al., 1993; Collis and Hall, 2004), leading to the highest level of expression in the integron.
Two major groups of integrons have been described: “chromosomal integrons” (CIs), and “mobile integrons” (MIs). CIs are located on the chromosome of hundreds of bacterial species; in silico analysis showed that 17% of sequenced bacterial genomes exhibited such genetic arrangements (Cambray et al., 2010). CIs are often described in bacteria from marine or terrestrial ecosystems, such as Vibrio spp. and Xanthomonas spp., CIs have also been termed “super-integrons” (SIs) as they can carry up to 200 cassettes that mainly encode proteins with unknown functions. CIs may also carry cassettes without functional reading frames. MIs are not self-transposable elements but are located on mobile genetic elements such as transposons and plasmids, which promote their dissemination among bacteria. MIs contain a limited number of GCs (less than 10 GCs; Naas et al., 2001; GenBank DQ112222). The GCs described to date in these MIs usually encode antibiotic-resistance determinants. MIs are therefore sometimes also called “resistant integrons” (RIs) or “multidrug resistance integrons” (MRIs).
In this review, we will focus on MIs.
Classes of MIs
Most MIs have been described in a wide range of Gram-negative bacteria, and only sporadically in Gram-positive bacteria (Martin et al., 1990; Nesvera et al., 1998; Nandi et al., 2004; Shi et al., 2006; Xu et al., 2010; Barraud et al., 2011). Based on the amino-acid sequence of the IntI protein, five classes of MIs have been described (Cambray et al., 2010). Classes 1, 2, and 3 are the most commonly detected. Classes 4 and 5 have only been detected once (Hochhut et al., 2001; GenBank AJ277063).
Class 1 MIs have been extensively studied due to their broad distribution among Gram-negative bacteria of clinical interest and are the most reported in human and animals. They have been described to be mainly associated with functional and non-functional transposons derived from Tn402. The non-functional type is the main common structural organization described in clinical isolates, and led some authors to call these class 1 MIs, “clinical integrons” (Gillings et al., 2008c). In addition, these structures are frequently embedded in plasmids or larger transposons, such as those of the Tn3 family (Tn21, Tn1696) allowing their dispersion (Labbate et al., 2009). The intI1 gene sequence is highly conserved among MIs found in clinical isolates, while it shows variability in MIs-containing environmental isolates (Gillings et al., 2008b). Furthermore, many class 1 MIs exhibit a 3′ region usually called 3′-conserved segment (3′CS). However, some authors consider using this 3′CS to detect MIs could create a bias in detection, since some MIs lack this sequence (Betteridge et al., 2011). The 3′CS is composed of a qacEΔ1 gene, a functional deletion of the qacE gene still conferring resistance to quaternary ammonium compounds (QACs; Paulsen et al., 1993), followed by a sul1 gene conferring resistance to sulfonamides, and orf5 encoding a protein of unknown function.
Class 2 MIs are the second most described group. In most class 2 MIs, the intI2 gene is interrupted by a stop codon, resulting in a truncated and non-functional protein. This results in a stable GCs array, mainly composed of the GC dfrA1 (involved in the resistance to trimethoprim), sat2 (involved in the resistance to streptothricin), aadA1 (involved in the resistance to streptomycin and spectinomycin), and orfX (unknown function; Hansson et al., 2002). However, some class 2 MIs with a different GCs array have been described, probably resulting from the ability of the integrase of class 1 MIs to catalyze recombination at the attI2 site (Biskri and Mazel, 2003; Ahmed et al., 2005; Ramírez et al., 2005, 2010; Gassama Sow et al., 2010). Class 2 MIs are almost always associated with the Tn7 transposon and their derivatives, hence promoting their dissemination. Two class 2 MIs have been described recently with a functional integrase, one containing nine GCs encoding unknown function and the second one harbored the dfrA14 GC followed by a second novel GC in which a lipoprotein signal peptidase gene has been predicted (Barlow and Gobius, 2006; Márquez et al., 2008).
Only few class 3 MIs have been described. Although their role in clinical antimicrobial resistance is less important, environmental ecosystems could harbor an important pool of these elements (see below).
MIs Dissemination and Their Involvement in Antibiotic Resistance
Antibiotic pressure has probably played an important role in the MIs selection and dissemination in bacteria. More than 130 GCs conferring resistance to antibiotics and more than 60 GCs of unknown functions have been described in MIs (Partridge et al., 2009). Genes involved in resistance to almost all antibiotic families are embedded in GCs, including beta-lactams, aminoglycosides, trimethoprim, chloramphenicol, fosfomycin, macrolides, lincosamides, rifampicin, and quinolones. In addition, the qac GCs, encoding resistance determinants to antiseptics of the QACs family, are commonly found in MIs. Studies have suggested that MIs were more prevalent in bacterial communities subjected to direct or indirect antibiotic pressure in clinical, agricultural, and environmental settings (Skurnik et al., 2005; Daikos et al., 2007; Barlow et al., 2009; Luo et al., 2010; Kristiansson et al., 2011). Other factors, such as QACs or heavy metals have also been shown to be involved in the MIs dissemination, and thus probably playing a role in their spread before the antibiotic era (see below). More generally, it has been shown, when studying animal fecal E. coli, that human activity in the near vicinity increased the prevalence of MIs in these bacteria (Skurnik et al., 2006). Concerning the role of the antibiotic selective pressure, no published studies have demonstrated the direct in vivo selection of resistance through the acquisition of an integron. One study has demonstrated the in vivo selection of resistance through a rearrangement of the GCs array within a class 1 MI under antibiotic selective pressure (Hocquet et al., 2011).
Recent in vitro studies have shown that antibiotics are able to induce integrase transcription, both in CIs and MIs, via the SOS response. The SOS response is a global regulatory network controlled by the transcriptional repressor LexA and induced by stress leading to direct or indirect DNA damage, such as damage resulting from exposure to some widely used antibiotics (fluoroquinolones, beta-lactams, trimethoprim, aminoglycosides; Guerin et al., 2009; Baharoglu et al., 2010; Cambray et al., 2011). The activation of the SOS response in bacteria results in integrase overexpression, which leads to the raise of GCs recombination events.
Clinical, veterinary, and environmental surveys have shown that bacteria harboring MIs are often associated with multidrug-resistant (MDR) phenotypes (Bass et al., 1999; Leverstein-van Hall et al., 2003; Biyela et al., 2004; Nijssen et al., 2005; Laroche et al., 2009). However, the MDR profile could not be linked only to the antibiotic-resistance GCs carried by the MIs, but also to other resistance genes located on MIs-containing plasmids and transposons. This way, MIs could be co-selected with the plasmid- and/or transposon-associated antibiotic-resistance genes (Laroche et al., 2009; Li et al., 2010). For example, co-selection of class 1 MIs on plasmids harboring a tet gene (involved in the tetracycline resistance) in oxytetracycline-contaminated environments has been reported (Li et al., 2010).
The link between MIs and antibiotic resistance is still controversial since several studies present divergent conclusions (Hoyle et al., 2006; Smith et al., 2007). Furthermore many data have to be interpreted with caution. Indeed, biases in the study of links between MIs and antibiotic resistance could be generated by the selective choice of antibiotic-specific resistant strains, leading to misinterpretation. Finally, this relationship between MIs and antibiotic resistance has mainly been studied in bacteria of clinical or veterinary interest, such as those within the family Enterobacteriaceae.
Otherwise, the environment contains a wide range of bacterial species and cultivation methods only permit the isolation of a small fraction (around 1%; Amann et al., 1995). Techniques based on the study of the metagenome have thus been developed to avoid this limitation. The combination of culturing and metagenomics approaches on environmental ecosystems has highlighted the roles of MIs in antibiotic-resistance dissemination. Tables 1 and 2 present an extensive list of the studies that have quantified the occurrence of MIs in the environment, using either cultivation methods (Table 1) or cultivation independent methods (Table 2). Genetic methods presented in this review quantify the abundance of integrase genes in the total DNA from different ecosystems. In order to normalize the quantity of gene to the total bacterial communities, most authors have used quantification of the ubiquitous bacterial 16S rRNA encoding genes. By dividing the abundance of integrase genes by the number of 16S rRNA genes, authors were able to demonstrate relative abundance. This ratio corresponds to the integrase genes proportion in the total bacterial communities. However, some authors have multiplying the ratio by the average number of copies of the 16S rRNA encoding genes per bacteria; which is approximately four (Klappenbach et al., 2001), and other authors present their results as percentages. In order to integrate all relative abundance data from diverse studies, results have been normalized to the same ratio for the purpose of this review and the relative abundance corresponds to the percentage of MIs per bacterial cell (Table 2).
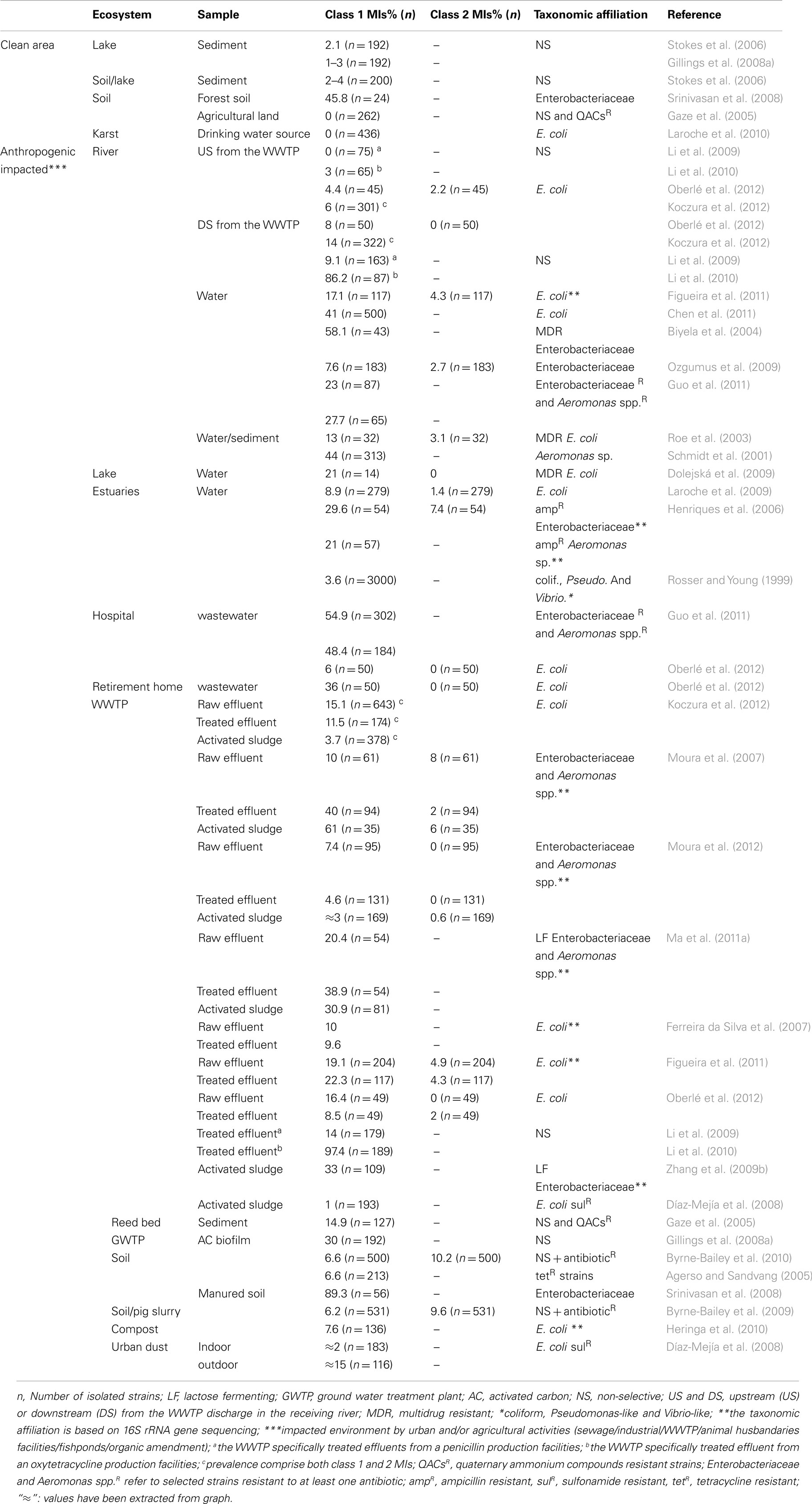
Table 1. Bacterial prevalence of class 1 and 2 MIs in different ecosystems (results from cultivation-dependent studies).
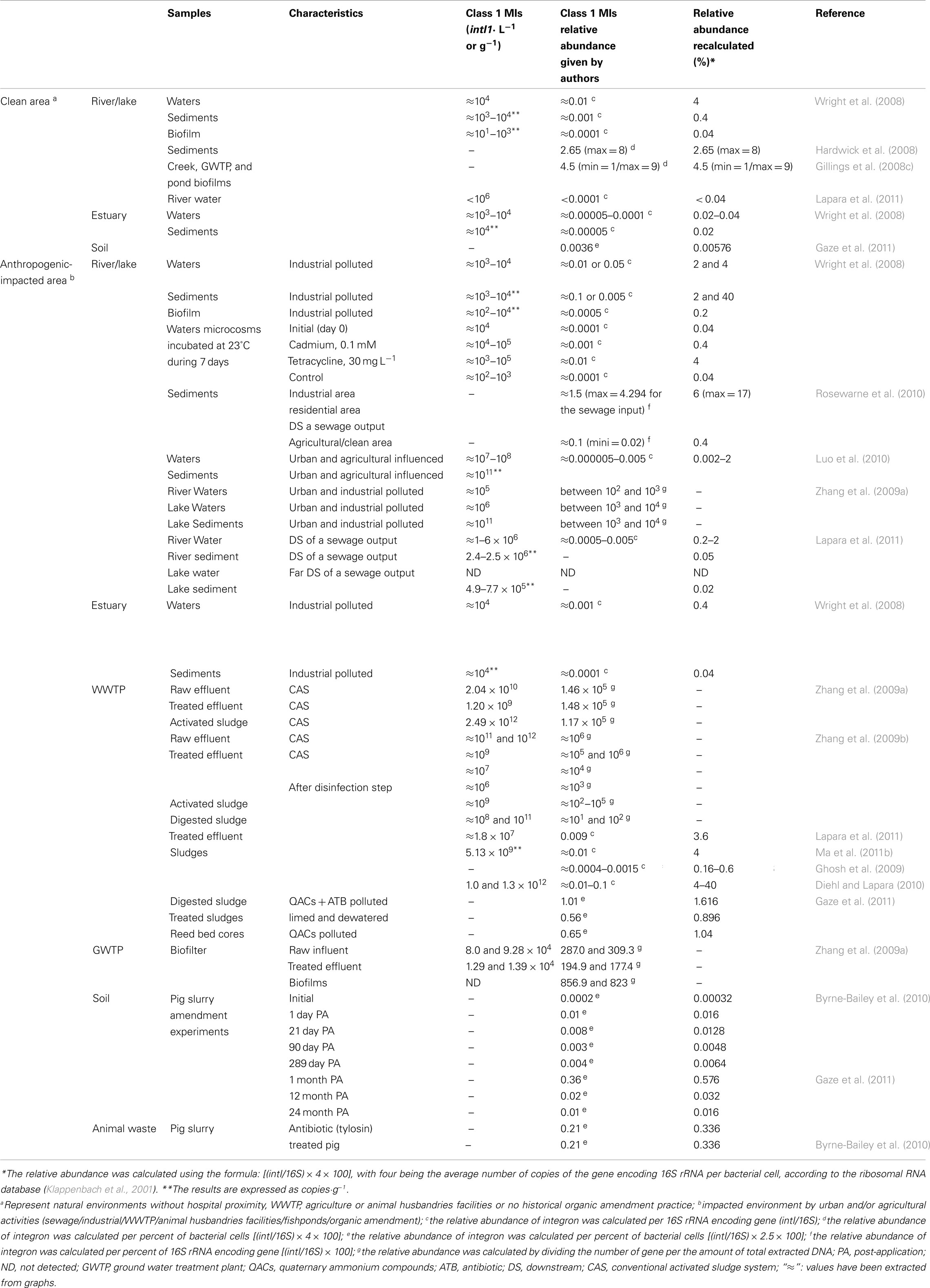
Table 2. Concentration and relative abundance of class 1 MIs in total community DNA from different ecosystems.
MIs in the Environment
There is growing evidence that the environment plays a role in the spread of antibiotic resistance among pathogenic strains. Many questions have been raised concerning the impact of the release of antibiotics and antibiotic-resistant bacteria on the environment or on human and animal health (Aminov, 2010). The distribution of MIs, and especially the class 1 MIs, in the environment is a growing focus of attention, as illustrated by the recent publications presented in Tables 1 and 2.
MIs have been described in a wide range of natural ecosystems, both aquatic (e.g., lakes, rivers, estuaries) and terrestrial. However, their distribution has been investigated mainly in human-impacted environments such as amended soils and aquatic ecosystems influenced by urbanization, agriculture, aquaculture, industrial waste, and even in indoor and outdoor dust.
MI Occurrence in “Natural” Environments
Different authors have investigated the occurrence of class 1 MIs in ecosystems considered to be untouched or barely affected by anthropogenic influence, these are often termed “reference sites” and correspond in Tables 1 and 2 to the “clean area.”
Only a few teams have studied MIs abundance in soils. Gaze et al. (2011) reported a class 1 MIs relative abundance of 0.00576% (Table 2) by a metagenomic approach in soils with no history of organic amendment, whereas the same authors previously found no class 1 MIs in the bacterial culturable fraction, which was composed of Bacillaceae, Paenibacillaceae, and Pseudomonadaceae (Gaze et al., 2005). In a study on forest soils, 11 out of 24 isolated Enterobacteriaceae strains (45%) were found to contain class 1 MIs, but these MIs harbored no GCs (Srinivasan et al., 2008).
In aquatics environments, Wright et al. (2008) and Hardwick et al. (2008) found, using metagenomics approaches, a relative class 1 MIs abundance recovery from 0.02 to 4%, in estuarine and stream water/sediments/biofilms, and 2.65% in creek sediments (Table 2). Using cultivation-dependent methods, class 1 MIs were found in lake sediments, with a prevalence of 1–4% (Stokes et al., 2006; Gillings et al., 2008a). Some studies investigated the GCs content of class 1 MIs. More often, one to three GCs were present, mainly encoding unknown function. GCs implied in the resistance to QACs (qac alleles) were also frequently described and antibiotic-resistance GCs were rarely found (Gillings et al., 2008c, 2009a).
Environmental Source of MIs
The class 1 MIs are ubiquitous elements naturally occurring in the environment, and different studies suggest that these elements emerged from ancestral environmental CIs (Rowe-Magnus et al., 2001; Mazel, 2006). Following the discovery of several class 1 MIs lacking resistance genes in environmental samples and located on the bacterial chromosome (Stokes et al., 2006; Gillings et al., 2008a), an evolutionary model was proposed and is now well documented (Gillings et al., 2008a; Labbate et al., 2009; Cambray et al., 2010; Stokes and Gillings, 2011). This model involves a succession of evolutionary recombination events, which facilitated the spread of class 1 MIs among pathogenic bacteria. These events led to the association of an “ancient” chromosomal class 1 MI with mobile functions of a Tn402-like transposon, and the acquisition of a qacE and sul1 genes. During this evolution, deletions, insertions, and other rearrangements finally shaped the 3′CS of current class 1 MIs found in clinical isolates, as well as their inclusion in larger mobile platforms (plasmids and transposons), resulting in the spread of these elements among a broad range of bacteria, including pathogenic species (Gillings et al., 2008a; Labbate et al., 2009). Finally, it has been suggested that the class 1 MIs were probably widely distributed in Proteobacteria before the antibiotic era (Stokes and Gillings, 2011). These authors suggested that these class 1 MIs were unlikely to have GCs encoding antibiotic resistant determinants, and that they further evolved by acquisition of the 3′CS and antibiotic-resistance GCs. Nevertheless, a class 1 MI found in a Pseudomonas isolate recently recovered from 15,000- to 40,000-years-old Siberian permafrost with all the characteristics of a typical clinical class 1 MI, i.e., 5′CS and 3′CS, an antibiotic resistant GC (aadA2 encoding resistance determinants to streptomycin and spectinomycin), localization on a mobile element (Tn5045 transposon), contradicts this hypothesis (Petrova et al., 2011).
Anthropogenic Impact on MIs Distribution
Rivers, seas, and lakes
Water is the main vector of pollutants in the environment and thus has received most attention. Furthermore, water bodies have been underlined as ideal vectors for the antibiotic-resistance dissemination (Lupo et al., 2012). Indeed, compared to the “natural” waters previously described, the prevalence of class 1 MIs-containing strains is higher in known polluted waters (Table 1). The variation of results observed among studies may depend on many factors, such as the selected bacterial species, the applied culture method (selective or not selective), as well as the sample characteristics (e.g., sediment or water, occurrence of rain events before sampling, close location of a wastewater discharged site). Using metagenomic approaches, urban and agricultural activities were positively associated with class 1 MIs. High concentrations of class 1 MIs were found in a Chinese river located in an urban and agriculturally influenced region, with around 107–108 copies · L−1 and 1011 copies · g−1 of sediment (Luo et al., 2010), whereas in a clean area, concentrations of class 1 MIs were found to be around 104 copies · L−1 and 103–104 copies · g−1 of sediments (Wright et al., 2008). Zhang et al. (2009b) found a significant enrichment of class 1 MIs into the Yangtze river along its course through the Nanjing city, highlighting the impact of urban areas on rivers. Also, the relative abundance of class 1 MIs has been strongly correlated with the contribution of treated sewage output flow in the receiving river sediment (Rosewarne et al., 2010). This has been confirmed in a recent study carried out by Lapara et al. (2011), underlining the role of the wastewater treatment plant (WWTP) in the dissemination of class 1 MIs in the environment. Otherwise, fish farming has been shown to significantly elevate the prevalence of class 1 MIs in motile Aeromonads in river waters. The MIs identified contained dfr GCs encoding trimethoprim determinants, and their occurrence correlated with the administration of combined sulfonamide/trimethoprim drugs in freshwater fish farms (Schmidt et al., 2001). In polluted estuaries, the prevalence of class 1 MIs appears to be less important than in the aquatic ecosystems previously described, with values ranging between 2.7 and 14.7% (Laroche et al., 2009). Nevertheless, it has been observed that in anthropogenically impacted estuaries the relative abundance was around 10 times more than in an unpolluted reference estuary (Wright et al., 2008; Table 2). The authors did not show any influence of the tide, the relative MIs abundance being similar during ebb or flood tides.
Studies involving effluents of factories which produce antibiotics showed that antibiotic production could have an effect on the prevalence of MI-containing bacteria in the receiving river (Li et al., 2009, 2010). In these two studies, the impact differed according to the industry production, although the effluent treatment processes were equivalent in the two industries (anaerobic digestion following by activated sludge process without disinfection step). Indeed, the penicillin production effluents elevated the prevalence of class 1 MIs-harboring strains in the river, from 0% upstream of the discharge to 9.1% after the treated effluent was discharged whereas the oxytetracycline production effluents elevated the MIs prevalence in the river from 3% upstream of the discharge to 86.2% downstream (Li et al., 2009, 2010). Moreover, the authors suggested that some Pseudomonas sp. and Bacillus sp. isolates harbored simultaneously up to seven different class 1 MIs per bacteria, from the effluent of the oxytetracycline factory, as well as in the receiving river. In comparison, the bacteria from upstream of the WWTP harbored only one class 1 MI. More recently in a metagenomics study, authors observed a 6.7-fold enrichment of class 1 MIs in river sediments downstream of a treated WWTP effluent discharge point from an antibiotic production complex (Kristiansson et al., 2011).
However, the impact of anthropogenic activities is not limited to antibiotic pressure alone, since similar observations have been made in environments without sources of antibiotics input. An Australian study has correlated the rise of the relative abundance of class 1 MIs with environmental parameters (Hardwick et al., 2008). When the environmental conditions were more stressful to the bacteria, the relative abundance of class 1 MIs was higher. Industrial activities (mainly resulting in heavy metal contamination) also have been shown specifically to contribute to the increase of class 1 MIs relative abundance (Wright et al., 2008; Rosewarne et al., 2010). It has been shown that adding tetracycline or cadmium to a water stream in microcosm experiments increased the MIs relative abundance by a factor of between 10- and 100-fold (Wright et al., 2008). The co-selection of resistance genes with heavy metal such as mercury resistance has been previously described (Aminov and Mackie, 2007). Class 1 MIs have been described on the Tn21 transposon which also contains a mercury resistance operon (Liebert et al., 1999). Antiseptic agents as QACs have also been shown to be associated with a higher prevalence of class 1 MIs (Gillings et al., 2008c). In QACs contaminated reed bed, it was shown that 95% of the isolated strains with class 1 MIs harbored a qac gene (Gaze et al., 2005). Heavy metals and QACs are thus probably involved in MIs dissemination and may have contributed to the MIs selection before the antibiotic era (Stokes and Gillings, 2011).
In anthropogenic-impacted waters, an important diversity of GCs has been recovered (Rosser and Young, 1999; Roe et al., 2003; Henriques et al., 2006; Taviani et al., 2008; Laroche et al., 2009; Li et al., 2009; Ozgumus et al., 2009; Verner-Jeffreys et al., 2009; Kumar et al., 2010; Rosewarne et al., 2010; Chen et al., 2011). Resistance to almost all families of antimicrobials has been recovered with various GCs: aad, aac (conferring resistance to aminoglycosides); blaCARB-2, blaOXA, blaP1 (conferring resistance to beta-lactams); dfr (conferring resistance to trimethoprim); catB (conferring resistance to chloramphenicol); ereA (conferring resistance to erythromycin); arr (conferring resistance to rifampicin); and qac (conferring resistance to QACs). Moreover, GCs with unknown function have been also commonly found. Several studies have characterized the total pool of integron GCs from environmental samples by using a PCR approach targeting only the GCs (attC sites) and not the integrase genes. They showed a huge GCs diversity mostly encoding unknown functions, and underlined the effect of both environmental and anthropogenic conditions on the GCs pool composition (Koenig et al., 2008, 2009, 2011; Huang et al., 2009; Elsaied et al., 2011). Anthropogenic activity thus increases the prevalence of class 1 MIs in microbial communities. These anthropogenic environmental changes result in an increase in transferable genetic elements potentially harboring resistance genes, and an ability to capture new resistance genes from autochthonous hosts. Antibiotic-resistance genes located in mobile genetic elements (plasmids, transposons, integrons) have been suggested to be “genetic pollutants” representative of human activities (Martinez, 2009a). Moreover, anthropogenic stresses has been suggested to facilitate the possible transfer of chromosomal resistance genes to the mobile gene pool accelerating the evolution and the possible spread in human-pathogenic strains (Cattoir et al., 2008; Picão et al., 2008; Martinez, 2009a,b; Rahube and Yost, 2010).
Class 2 MIs are less prevalent than class 1 in polluted waters (0–7.4%), Table 1. In a culture-independent method survey, the low relative abundance rate of class 2 MIs from river has been underlined (Luo et al., 2010). These results suggest that their role in aquatic ecosystems is probably minor.
Sewage and wastewater treatment plants
Wastewater treatment plants are the interface between human waste and both the aquatic and soil environments (Figure 2). They collect effluents from diverse sources (such as hospital, private household, industries, animal husbandries), which contribute to the final ecosystem of the WWTP. These include the organics, chemicals, and microbiological wastes. Finally the WWTP ecosystem constitutes a “broth” where each element interacts with each other under a physical and chemical constraint resulting mainly in an organic degradation in the aqueous and solid phase. Microorganisms are key to the process resulting in organic and chemical degradation or transformation. The bacterial communities are organized in free biofilm entities (called bacterial flocs), which constitute the total biomass (the sludge). As suggested by many authors, the high bacterial density, due to the nutritional richness, indicates that WWTP are hot spots for horizontal gene transfer (Tennstedt et al., 2003). Moreover, the antibiotics potentially present in the WWTP could select antibiotic-resistant bacteria, as shown for erythromycin (Louvet et al., 2010), thereby enabling the persistence of antibiotic-resistance plasmids. It has been shown that sulfamethoxazole or amoxicillin at sub-inhibitory concentrations in activated sludge improved the stability of the pB10 plasmid in E. coli (Merlin et al., 2011). This co-existence of bacteria and antibiotics in WWTP increases the frequency of genetic variations (as recombination events) and the possible emergence of novel mechanisms of resistance (Baquero et al., 2008).
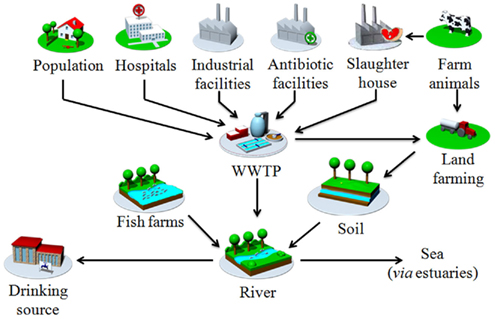
Figure 2. Main route of MIs dissemination from anthropogenic sources to the environment (pictures derived from PILLS project: http://www.pills-project.eu).
The highest concentrations of class 1 MIs ever described have been recovered from raw effluents with values comprised between 1010 and 1012 copies · L−1 (Table 2). Class 1 MIs have been described at all stages of the WWTP process with variable prevalence or relative abundance (Tables 1 and 2), nevertheless their relative abundance in the final treated effluents highlights the inefficiency of the process to remove bacteria harboring these genetic elements (this will be described below in more details). In activated sludge, 3–61% of the isolated strains harbored a class 1 MI (Table 1). By metagenomics approach, the relative abundance of class 1 MIs varied (Table 2). These variations could be explained by the different methods of nucleic acids extraction as well as the primers used to detect the MIs. Nevertheless relative abundance up to 40% has been found suggesting that the activated sludge is a hot spot for class 1 MIs selection and/or dissemination. In addition, two studies showed that 12% of isolated plasmids from WWTP sludge carried MIs (Tennstedt et al., 2003; Moura et al., 2007), among which more than half were broad-host-range plasmids displaying very high transfer frequencies (Tennstedt et al., 2003).
As previously described in the aquatic ecosystems, the low prevalence of class 2 MIs in WWTP suggests that their role is probably minor. Although less than 10 publications have reported class 3 MIs, 2 of them have been described in Delftia sp. (D. acidovorans and D. tsuruhatensis) isolated from activated sludge (Xu et al., 2007). These class 3 MIs contained GCs of unknown function. Moreover, using molecular approach, class 3 MIs were detected in effluents from an urban WWTP and a slaughter house WWTP (Moura et al., 2010). These findings suggest that even if class 3 MIs play a minor role in clinical microbiology, their role in the environment is probably more extensive.
The analysis of GCs content from wastewater ecosystems showed a huge diversity of genes encoding antibiotic resistance: resistances to aminoglycosides with aad, aacA GCs; to beta-lactams with blaOXA, blaVIM-2, blaIMP, blaP1, blaGES-5, and blaGES-7 GCs; to trimethoprim with dfr gene GCs; to chloramphenicol with cat and cml GCs; to erythromycin with ereA and estX GCs; to rifampicin with arr GCs; and to quinolones with qnrVC4 GC (Tennstedt et al., 2003; Ferreira da Silva et al., 2007; Moura et al., 2007, 2012; Taviani et al., 2008; Li et al., 2009, 2010; Pellegrini et al., 2009, 2011; Zhang et al., 2009b; Xia et al., 2010; Girlich et al., 2011; Guo et al., 2011; Ma et al., 2011a; Scotta et al., 2011). A molecular approach describing the global pool of GCs in WWTP have shown a great diversity of GCs, mainly encoding for determinants implied in metabolic functions or unknown functions, suggesting the wide potential reservoir of GCs in WWTP (Moura et al., 2010).
Efficiency of WWTP process to remove MIs
While the WWTP reduced the bacterial load, it appears that the treatment is inefficient to remove both antibiotic resistant bacteria (Novo and Manaia, 2010; Luczkiewicz et al., 2010), and MIs-harboring bacteria.
As presented in Tables 1 and 2, the prevalence or relative abundance of MIs after the activated sludge process is not reduced, and is even often higher than in the raw effluent (Ferreira da Silva et al., 2007; Moura et al., 2007; Figueira et al., 2011; Ma et al., 2011a). These authors often concluded that activated process can remove bacteria, but do not reduce significantly the bacteria harboring class 1 MIs. When using abundance normalized to the total DNA amount, same observation have been done (Zhang et al., 2009a), however in another study, these authors found that the effluent treatment process decreased the MIs rate (Zhang et al., 2009b). Nevertheless, normalization to DNA amount is critical as total community DNA usually contains DNA of non-bacterial origin. The removal of bacteria bearing antibiotic-resistance genetics elements by the WWTP is a new challenge for the future. Several studies have investigated the efficiency of different advanced processes such as UV treatment, membrane biological reactors, and chlorination, to remove bacteria carrying antibiotic-resistance genes (Auerbach et al., 2007; Garcia et al., 2007; Kim et al., 2010; Huang et al., 2011; Munir et al., 2011), but no studies have examined the effects on MIs. Recently, hospital effluents were shown to be potential sources of dissemination of MIs in the sewage network (Guo et al., 2011). Oberlé et al. (2012) noted a decrease of the prevalence of class 1 MIs in E. coli along the effluents treatment from the hospital to the WWTP and the receiving river continuum. As treatment of hospital effluents onsite is a growing and controversial question (Pauwels and Verstraete, 2006; Kümmerer, 2008; Ort et al., 2010; Escher et al., 2011), these data need confirmation by further studies in order to assess the impact of these specific effluents on the release of MIs in the WWTP.
Activated sludges are often used in agriculture as organic amendment (Figure 2). However, before their use, treatments in order to reduce their volume and improve stability are applied. Several studies have specifically investigated the potential of aerobic and anaerobic treatments to reduce class 1 MIs in activated sludges, demonstrating a better performance of anaerobic thermophilic process (50–60°C) to decrease the relative abundance of class 1 MIs (Ghosh et al., 2009; Diehl and Lapara, 2010). However, dissimilar results have been obtained in same conditions by Ma et al. (2011b), suggesting that other factors may influence the MIs occurrence during the sludge digestion. Evidence of horizontal gene transfers in WWTP sludge has been shown by Merlin et al. (2011). They have shown that, horizontal transfer of pB10 plasmid occurred in sludge from the anaerobic digesters or from fixed biofilm reactors, with higher efficiency in fixed biofilm conditions.
Soil ecosystem and the animal wastes as sources of MIs
While the soil “resistome” is a vast original reservoir of resistance genes (D’Costa et al., 2006; Allen et al., 2010), manure has been shown to significantly increase the mobile genetic resistance elements pool (Heuer et al., 2011). Recent studies have highlighted the role of the amendment practice on the input of MIs in soil (Heuer and Smalla, 2007; Binh et al., 2009; Byrne-Bailey et al., 2010; Gaze et al., 2011), see Table 2. Moreover, studies on sewage sludge and pig slurry amendment showed that even if the prevalence of class 1 MIs decreased after the particular amendment (2 years and 10 months, respectively), the prevalence was still higher than in control soils without amendment (around 100 times more; Byrne-Bailey et al., 2010; Gaze et al., 2011). Some authors studied the GCs array of class 1 MIs introduced in soil via manure amendment and mainly found streptomycin and spectinomycin resistance aadA GCs (Heuer and Smalla, 2007; Binh et al., 2009). Class 2 MIs have been also identified from amended soils with relative high rates (Byrne-Bailey et al., 2009, 2010; Rodríguez-Minguela et al., 2009). The high antibiotics consumption in some animal husbandries, and their systemic application as food additives in the past, had probably significantly contributed to MIs dissemination in amended soils. Tschäpe (1994) showed that the streptothricin usage as food additive contributed to the dissemination of sat genes in amended soils via mobile genetic structures, such as the Tn7 transposon carrying a class 2 MI usually bearing a streptothricin-resistant sat2 GC.
Animal wastes (e.g., manure, poultry litter, and slurry) are the main vectors of MIs dissemination in soil. As recently reviewed by Heuer et al. (2011), only a few studies have investigated the reduction of some resistance genes following different processes, such as storage, composting, and anaerobic digestion (Chen et al., 2007, 2010; Heuer et al., 2008). Only composting was efficient in reducing the prevalence and absolute amount of erythromycin resistance genes (Chen et al., 2007). Concerning the MIs, one study reported that after 57 days of storage of manure at 20°C, the class 1 MIs GCs array electrophoresis gel profiles were almost identical to that at the beginning of the experiment; however the GCs contents was not investigated (Heuer et al., 2008).
Role of the Food Chain
The food chain probably also takes place in the transit of MIs from the environments to the human. Indeed, bacteria harboring MIs have been recovered from a variety of aquatic living organisms, such as in prawns, with an Enterobacter cloacae harboring a class 1 MI (Gillings et al., 2009b); or in Corbicula with a class 1 MIs relative abundance of 4% (Wright et al., 2008); and in oysters where the uncommon class 3 MIs prevailed (Barkovskii et al., 2010). Transfers of MIs between animals and human occur and have been well reviewed by Stokes and Gillings (2011). Class 1 MIs have been also reported from biofilms of drinking water supplies (Tables 1 and 2; Gillings et al., 2008a; Zhang et al., 2009a). All these results underline the link, via the food chain, between the environmental MIs and the human or animal MIs.
Conclusion
As described in this review, MIs are efficient tools for bacterial adaptation and play a significant role in antibiotic resistance. Environmental studies demonstrated that anthropogenic impact lead to enrichment of class 1 MIs. More specifically, all factors leading to bacterial stress, such as antibiotics, QACs, or high concentrations of heavy metals resulted in the selection of class 1 MIs-harboring bacteria. Several hot spots of class 1 MIs dissemination have been identified, as agricultural manure amendment, WWTP, or industrial effluents. While these wastes are treated in varying degrees before their discharge, it appears that the current processes are inefficient to reduce MIs dissemination. This uncontrolled dissemination of MIs in the environment could represent a risk for human health.
Conflict of Interest Statement
The authors declare that the research was conducted in the absence of any commercial or financial relationships that could be construed as a potential conflict of interest.
Acknowledgments
This work was supported by the regional council of Limousin. The authors wish to thank Colin Hunter and William Rawlinson for their help in critical reading of the manuscript.
References
Agerso, Y., and Sandvang, D. (2005). Class 1 integrons and tetracycline resistance genes in Alcaligenes, Arthrobacter, and Pseudomonas spp. isolated from pigsties and manured soil. Appl. Environ. Microbiol. 71, 7941–7947.
Ahmed, A. M., Nakano, H., and Shimamoto, T. (2005). Molecular characterization of integrons in non-typhoid Salmonella serovars isolated in Japan: description of an unusual class 2 integron. J. Antimicrob. Chemother. 55, 371–374.
Allen, H. K., Donato, J., Wang, H. H., Cloud-Hansen, K. A., Davies, J., and Handelsman, J. (2010). Call of the wild: antibiotic resistance genes in natural environments. Nat. Rev. Microbiol. 8, 251–259.
Amann, R. I., Ludwig, W., and Schleifer, K. H. (1995). Phylogenetic identification and in situ detection of individual microbial cells without cultivation. Microbiol. Rev. 59, 143–169.
Aminov, R. I. (2010). A brief history of the antibiotic era: lessons learned and challenges for the future. Front. Microbiol. 1:134. doi:10.3389/fmicb.2010.00134
Aminov, R. I. (2011). Horizontal gene exchange in environmental microbiota. Front. Microbiol. 2:158. doi:10.3389/fmicb.2011.00158
Aminov, R. I., and Mackie, R. I. (2007). Evolution and ecology of antibiotic resistance genes. FEMS Microbiol. Lett. 271, 147–161.
Auerbach, E. A., Seyfried, E. E., and McMahon, K. D. (2007). Tetracycline resistance genes in activated sludge wastewater treatment plants. Water Res. 41, 1143–1151.
Baharoglu, Z., Bikard, D., and Mazel, D. (2010). Conjugative DNA transfer induces the bacterial SOS response and promotes antibiotic resistance development through integron activation. PLoS Genet. 6, e1001165. doi:10.1371/journal.pgen.1001165
Baquero, F., Martínez, J. L., and Cantón, R. (2008). Antibiotics and antibiotic resistance in water environments. Curr. Opin. Biotechnol. 19, 260–265.
Barkovskii, A. L., Green, C., and Hurley, D. (2010). The occurrence, spatial and temporal distribution, and environmental routes of tetracycline resistance and integrase genes in Crassostrea virginica beds. Mar. Pollut. Bull. 60, 2215–2224.
Barlow, R. S., Fegan, N., and Gobius, K. S. (2009). Integron-containing bacteria in faeces of cattle from different production systems at slaughter. J. Appl. Microbiol. 107, 540–545.
Barlow, R. S., and Gobius, K. S. (2006). Diverse class 2 integrons in bacteria from beef cattle sources. J. Antimicrob. Chemother. 58, 1133–1138.
Barraud, O., Badell, E., Denis, F., Guiso, N., and Ploy, M.-C. (2011). Antimicrobial drug resistance in Corynebacterium diphtheriae mitis. Emerging Infect. Dis. 17, 2078–2080.
Barraud, O., and Ploy, M.-C. (2011). Actualités sur les intégrons de résistance aux antibiotiques: mise au point. J. Anti-infectieux 13, 133–144.
Bass, L., Liebert, C. A., Lee, M. D., Summers, A. O., White, D. G., Thayer, S. G., and Maurer, J. J. (1999). Incidence and characterization of integrons, genetic elements mediating multiple-drug resistance, in avian Escherichia coli. Antimicrob. Agents Chemother. 43, 2925–2929.
Bertels, F., and Rainey, P. B. (2011). Within-genome evolution of REPINs: a new family of miniature mobile DNA in bacteria. PLoS Genet. 7, e1002132. doi:10.1371/journal.pgen.1002132
Betteridge, T., Partridge, S. R., Iredell, J. R., and Stokes, H. W. (2011). Genetic context and structural diversity of class 1 integrons from human commensal bacteria in a hospital intensive care unit. Antimicrob. Agents Chemother. 55, 3939–3943.
Binh, C. T. T., Heuer, H., Kaupenjohann, M., and Smalla, K. (2009). Diverse aadA gene cassettes on class 1 integrons introduced into soil via spread manure. Res. Microbiol. 160, 427–433.
Biskri, L., and Mazel, D. (2003). Erythromycin esterase gene ere(A) is located in a functional gene cassette in an unusual class 2 integron. Antimicrob. Agents Chemother. 47, 3326–3331.
Biyela, P. T., Lin, J., and Bezuidenhout, C. C. (2004). The role of aquatic ecosystems as reservoirs of antibiotic resistant bacteria and antibiotic resistance genes. Water Sci. Technol. 50, 45–50.
Boucher, Y., Labbate, M., Koenig, J. E., and Stokes, H. W. (2007). Integrons: mobilizable platforms that promote genetic diversity in bacteria. Trends Microbiol. 15, 301–309.
Byrne-Bailey, K. G., Gaze, W. H., Kay, P., Boxall, A. B. A., Hawkey, P. M., and Wellington, E. M. H. (2009). Prevalence of sulfonamide resistance genes in bacterial isolates from manured agricultural soils and pig slurry in the United Kingdom. Antimicrob. Agents Chemother. 53, 696–702.
Byrne-Bailey, K. G., Gaze, W. H., Zhang, L., Kay, P., Boxall, A., Hawkey, P. M., and Wellington, E. M. H. (2010). Integron prevalence and diversity in manured soil. Appl. Environ. Microbiol. 77, 684–687.
Cambray, G., Sanchez-Alberola, N., Campoy, S., Guerin, E., Da Re, S., Gonzalez-Zorn, B., Ploy, M.-C., Barbe, J., Mazel, D., and Erill, I. (2011). Prevalence of SOS-mediated control of integron integrase expression as an adaptive trait of chromosomal and mobile integrons. Mob. DNA 2, 6.
Cattoir, V., Poirel, L., Aubert, C., Soussy, C.-J., and Nordmann, P. (2008). Unexpected occurrence of plasmid-mediated quinolone resistance determinants in environmental Aeromonas spp. Emerging Infect. Dis. 14, 231–237.
Chen, B., Zheng, W., Yu, Y., Huang, W., Zheng, S., Zhang, Y., Guan, X., Zhuang, Y., Chen, N., and Topp, E. (2011). Class 1 integrons, selected virulence genes, and antibiotic resistance in Escherichia coli isolates from the Minjiang River, Fujian Province, China. Appl. Environ. Microbiol. 77, 148–155.
Chen, J., Michel, F. C. Jr, Sreevatsan, S., Morrison, M., and Yu, Z. (2010). Occurrence and persistence of erythromycin resistance genes (erm) and tetracycline resistance genes (tet) in waste treatment systems on swine farms. Microb. Ecol. 60, 479–486.
Chen, J., Yu, Z., Michel, F. C. Jr, Wittum, T., and Morrison, M. (2007). Development and application of real-time PCR assays for quantification of erm genes conferring resistance to macrolides-lincosamides-streptogramin B in livestock manure and manure management systems. Appl. Environ. Microbiol. 73, 4407–4416.
Collis, C. M., Grammaticopoulos, G., Briton, J., Stokes, H. W., and Hall, R. M. (1993). Site-specific insertion of gene cassettes into integrons. Mol. Microbiol. 9, 41–52.
Collis, C. M., and Hall, R. M. (1992). Gene cassettes from the insert region of integrons are excised as covalently closed circles. Mol. Microbiol. 6, 2875–2885.
Collis, C. M., and Hall, R. M. (2004). Comparison of the structure-activity relationships of the integron-associated recombination sites attI3 and attI1 reveals common features. Microbiology 150, 1591–1601.
Daikos, G. L., Kosmidis, C., Tassios, P. T., Petrikkos, G., Vasilakopoulou, A., Psychogiou, M., Stefanou, I., Avlami, A., and Katsilambros, N. (2007). Enterobacteriaceae Bloodstream infections: presence of integrons, risk factors, and outcome. Antimicrob. Agents Chemother. 51, 2366–2372.
Davies, J., and Davies, D. (2010). Origins and evolution of antibiotic resistance. Microbiol. Mol. Biol. Rev. 74, 417–433.
D’Costa, V. M., McGrann, K. M., Hughes, D. W., and Wright, G. D. (2006). Sampling the antibiotic resistome. Science 311, 374–377.
Díaz-Mejía, J. J., Amábile-Cuevas, C. F., Rosas, I., and Souza, V. (2008). An analysis of the evolutionary relationships of integron integrases, with emphasis on the prevalence of class 1 integrons in Escherichia coli isolates from clinical and environmental origins. Microbiology 154, 94–102.
Diehl, D. L., and Lapara, T. M. (2010). Effect of temperature on the fate of genes encoding tetracycline resistance and the integrase of class 1 integrons within anaerobic and aerobic digesters treating municipal wastewater solids. Environ. Sci. Technol. 44, 9128–9133.
Dolejská, M., Bierosová, B., Kohoutová, L., Literák, I., and Cízek, A. (2009). Antibiotic-resistant i and Escherichia coli isolates with integrons and extended-spectrum beta-lactamases in surface water and sympatric black-headed gulls. J. Appl. Microbiol. 106, 1941–1950.
Elsaied, H., Stokes, H. W., Kitamura, K., Kurusu, Y., Kamagata, Y., and Maruyama, A. (2011). Marine integrons containing novel integrase genes, attachment sites, attI, and associated gene cassettes in polluted sediments from Suez and Tokyo Bays. ISME J. 5, 1162–1177.
Escher, B. I., Baumgartner, R., Koller, M., Treyer, K., Lienert, J., and McArdell, C. S. (2011). Environmental toxicology and risk assessment of pharmaceuticals from hospital wastewater. Water Res. 45, 75–92.
Ferreira da Silva, M., Vaz-Moreira, I., Gonzalez-Pajuelo, M., Nunes, O. C., and Manaia, C. M. (2007). Antimicrobial resistance patterns in Enterobacteriaceae isolated from an urban wastewater treatment plant. FEMS Microbiol. Ecol. 60, 166–176.
Figueira, V., Serra, E., and Manaia, C. M. (2011). Differential patterns of antimicrobial resistance in population subsets of Escherichia coli isolated from waste- and surface waters. Sci. Total Environ. 409, 1017–1023.
Garcia, S., Wade, B., Bauer, C., Craig, C., Nakaoka, K., and Lorowitz, W. (2007). The effect of wastewater treatment on antibiotic resistance in Escherichia coli and Enterococcus sp. Water Environ. Res. 79, 2387–2395.
Gassama Sow, A., Aïdara-Kane, A., Barraud, O., Gatet, M., Denis, F., and Ploy, M. C. (2010). High prevalence of trimethoprim-resistance cassettes in class 1 and 2 integrons in Senegalese Shigella spp isolates. J. Infect. Dev. Ctries. 4, 207–212.
Gaze, W. H., Abdouslam, N., Hawkey, P. M., and Wellington, E. M. H. (2005). Incidence of class 1 integrons in a quaternary ammonium compound-polluted environment. Antimicrob. Agents Chemother. 49, 1802–1807.
Gaze, W. H., Zhang, L., Abdouslam, N. A., Hawkey, P. M., Calvo-Bado, L., Royle, J., Brown, H., Davis, S., Kay, P., Boxall, A. B. A., and Wellington, E. M. (2011). Impacts of anthropogenic activity on the ecology of class 1 integrons and integron-associated genes in the environment. ISME J. 5, 1253–1261.
Ghosh, S., Ramsden, S. J., and LaPara, T. M. (2009). The role of anaerobic digestion in controlling the release of tetracycline resistance genes and class 1 integrons from municipal wastewater treatment plants. Appl. Microbiol. Biotechnol. 84, 791–796.
Gillings, M. R., Boucher, Y., Labbate, M., Holmes, A., Krishnan, S., Holley, M., and Stokes, H. W. (2008a). The evolution of class 1 integrons and the rise of antibiotic resistance. J. Bacteriol. 190, 5095–5100.
Gillings, M. R., Krishnan, S., Worden, P. J., and Hardwick, S. A. (2008b). Recovery of diverse genes for class 1 integron-integrases from environmental DNA samples. FEMS Microbiol. Lett. 287, 56–62.
Gillings, M. R., Xuejun, D., Hardwick, S. A., Holley, M. P., and Stokes, H. W. (2008c). Gene cassettes encoding resistance to quaternary ammonium compounds: a role in the origin of clinical class 1 integrons? ISME J. 3, 209–215.
Gillings, M. R., Holley, M. P., and Stokes, H. W. (2009a). Evidence for dynamic exchange of qac gene cassettes between class 1 integrons and other integrons in freshwater biofilms. FEMS Microbiol. Lett. 296, 282–288.
Gillings, M. R., Labbate, M., Sajjad, A., Giguère, N. J., Holley, M. P., and Stokes, H. W. (2009b). Mobilization of a Tn402-like class 1 integron with a novel cassette array via flanking miniature inverted-repeat transposable element-like structures. Appl. Environ. Microbiol. 75, 6002–6004.
Girlich, D., Poirel, L., Szczepanowski, R., Schlüter, A., and Nordmann, P. (2011). Carbapenem-hydrolyzing GES-5-encoding gene on different plasmid types from a sewage treatment plant. Appl. Environ. Microbiol. 78, 1292–1295.
Guerin, E., Cambray, G., Sanchez-Alberola, N., Campoy, S., Erill, I., Da Re, S., Gonzalez-Zorn, B., Barbé, J., Ploy, M.-C., and Mazel, D. (2009). The SOS response controls integron recombination. Science 324, 1034.
Guo, X., Xia, R., Han, N., and Xu, H. (2011). Genetic diversity analyses of class 1 integrons and their associated antimicrobial resistance genes in Enterobacteriaceae strains recovered from aquatic habitats in China. Lett. Appl. Microbiol. 52, 667–675.
Hall, R. M., and Collis, C. M. (1995). Mobile gene cassettes and integrons: capture and spread of genes by site-specific recombination. Mol. Microbiol. 15, 593–600.
Hansson, K., Sundström, L., Pelletier, A., and Roy, P. H. (2002). IntI2 integron integrase in Tn7. J. Bacteriol. 184, 1712–1721.
Hardwick, S. A., Stokes, H. W., Findlay, S., Taylor, M., and Gillings, M. R. (2008). Quantification of class 1 integron abundance in natural environments using real-time quantitative PCR. FEMS Microbiol. Lett. 278, 207–212.
Henriques, I. S., Fonseca, F., Alves, A., Saavedra, M. J., and Correia, A. (2006). Occurrence and diversity of integrons and beta-lactamase genes among ampicillin-resistant isolates from estuarine waters. Res. Microbiol. 157, 938–947.
Heringa, S., Kim, J., Shepherd, M. W. Jr, Singh, R., and Jiang, X. (2010). The presence of antibiotic resistance and integrons in Escherichia coli isolated from compost. Foodborne Pathog. Dis. 7, 1297–1304.
Heuer, H., Focks, A., Lamshöft, M., Smalla, K., Matthies, M., and Spiteller, M. (2008). Fate of sulfadiazine administered to pigs and its quantitative effect on the dynamics of bacterial resistance genes in manure and manured soil. Soil Biol. Biochem. 40, 1892–1900.
Heuer, H., Schmitt, H., and Smalla, K. (2011). Antibiotic resistance gene spread due to manure application on agricultural fields. Curr. Opin. Microbiol. 14, 236–243.
Heuer, H., and Smalla, K. (2007). Manure and sulfadiazine synergistically increased bacterial antibiotic resistance in soil over at least two months. Environ. Microbiol. 9, 657–666.
Hochhut, B., Lotfi, Y., Mazel, D., Faruque, S. M., Woodgate, R., and Waldor, M. K. (2001). Molecular analysis of antibiotic resistance gene clusters in Vibrio cholera O139 and O1 SXT constins. Antimicrob. Agents Chemother. 45, 2991–3000.
Hocquet, D., Llanes, C., Thouverez, M., Kulasekara, H., Legrand, F., Bertrand, X., Plésiat, P., Mazel, D., and Miller, S. (2011). “A Pseudomonas aeruginosa clinical isolate with antibiotic resistance promoted by the SOS response in a patient,” in ASM 111th General Meeting, New Orleans, LA.
Hoyle, D. V., Davison, H. C., Knight, H. I., Yates, C. M., Dobay, O., Gunn, G. J., Amyes, S. G. B., and Woolhouse, M. E. J. (2006). Molecular characterisation of bovine faecal Escherichia coli shows persistence of defined ampicillin resistant strains and the presence of class 1 integrons on an organic beef farm. Vet. Microbiol. 115, 250–257.
Huang, J.-J., Hu, H.-Y., Tang, F., Li, Y., Lu, S.-Q., and Lu, Y. (2011). Inactivation and reactivation of antibiotic-resistant bacteria by chlorination in secondary effluents of a municipal wastewater treatment plant. Water Res. 45, 2775–2781.
Huang, L., Cagnon, C., Caumette, P., and Duran, R. (2009). First gene cassettes of integrons as targets in finding adaptive genes in metagenomes. Appl. Environ. Microbiol. 75, 3823–3825.
Kim, S., Park, H., and Chandran, K. (2010). Propensity of activated sludge to amplify or attenuate tetracycline resistance genes and tetracycline resistant bacteria: a mathematical modeling approach. Chemosphere 78, 1071–1077.
Koczura, R., Mokracka, J., Jabłońska, L., Gozdecka, E., Kubek, M., and Kaznowski, A. (2012). Antimicrobial resistance of integron-harboring Escherichia coli isolates from clinical samples, wastewater treatment plant and river water. Sci. Total Environ. 414, 680–685.
Klappenbach, J. A., Saxman, P. R., Cole, J. R., and Schmidt, T. M. (2001). rrndb: the Ribosomal RNA operon copy number database. Nucleic Acids Res. 29, 181–184.
Koenig, J. E., Boucher, Y., Charlebois, R. L., Nesbø, C., Zhaxybayeva, O., Bapteste, E., Spencer, M., Joss, M. J., Stokes, H. W., and Doolittle, W. F. (2008). Integron-associated gene cassettes in Halifax Harbour: assessment of a mobile gene pool in marine sediments. Environ. Microbiol. 10, 1024–1038.
Koenig, J. E., Bourne, D. G., Curtis, B., Dlutek, M., Stokes, H. W., Doolittle, W. F., and Boucher, Y. (2011). Coral-mucus-associated Vibrio integrons in the Great Barrier Reef: genomic hotspots for environmental adaptation. ISME J. 5, 962–972.
Koenig, J. E., Sharp, C., Dlutek, M., Curtis, B., Joss, M., Boucher, Y., and Doolittle, W. F. (2009). Integron gene cassettes and degradation of compounds associated with industrial waste: the case of the Sydney Tar Ponds. PLoS ONE 4, e5276. doi:10.1371/journal.pone.0005276
Kristiansson, E., Fick, J., Janzon, A., Grabic, R., Rutgersson, C., Weijdegård, B., Söderström, H., and Larsson, D. G. J. (2011). Pyrosequencing of antibiotic-contaminated river sediments reveals high levels of resistance and gene transfer elements. PLoS ONE 6, e17038. doi:10.1371/journal.pone.0017038
Kumar, A., Mukherjee, S., and Chakraborty, R. (2010). Characterization of a novel trimethoprim resistance gene, dfrA28, in class 1 integron of an oligotrophic Acinetobacter johnsonii strain, MB52, isolated from River Mahananda, India. Microb. Drug Resist. 16, 29–37.
Kümmerer, K. (2008). Pharmaceuticals in the Environment Sources, Fate, Effects, and Risks, 3rd Edn. Heidelberg: Springer.
Labbate, M., Case, R. J., and Stokes, H. W. (2009). The integron/gene cassette system: an active player in bacterial adaptation. Methods Mol. Biol. 532, 103–125.
Lapara, T. M., Burch, T. R., McNamara, P. J., Tan, D. T., Yan, M., and Eichmiller, J. J. (2011). Tertiary-treated municipal wastewater is a significant point source of antibiotic resistance genes into Duluth-Superior Harbor. Environ. Sci. Technol. 45, 9543–9549.
Laroche, E., Pawlak, B., Berthe, T., Skurnik, D., and Petit, F. (2009). Occurrence of antibiotic resistance and class 1, 2 and 3 integrons in Escherichia coli isolated from a densely populated estuary (Seine, France). FEMS Microbiol. Ecol. 68, 118–130.
Laroche, E., Petit, F., Fournier, M., and Pawlak, B. (2010). Transport of antibiotic-resistant Escherichia coli in a public rural karst water supply. J. Hydrol. 392, 12–21.
Leverstein-van Hall, M. A., Blok, H. E. M., Donders, A. R. T., Paauw, A., Fluit, A. C., and Verhoef, J. (2003). Multidrug resistance among Enterobacteriaceae is strongly associated with the presence of integrons and is independent of species or isolate origin. J. Infect. Dis. 187, 251–259.
Li, D., Yang, M., Hu, J., Zhang, J., Liu, R., Gu, X., Zhang, Y., and Wang, Z. (2009). Antibiotic-resistance profile in environmental bacteria isolated from penicillin production wastewater treatment plant and the receiving river. Environ. Microbiol. 11, 1506–1517.
Li, D., Yu, T., Zhang, Y., Yang, M., Li, Z., Liu, M., and Qi, R. (2010). Antibiotic resistance characteristics of environmental bacteria from an oxytetracycline production wastewater treatment plant and the receiving river. Appl. Environ. Microbiol. 76, 3444–3451.
Liebert, C. A., Hall, R. M., and Summers, A. O. (1999). Transposon Tn21, flagship of the floating genome. Microbiol. Mol. Biol. Rev. 63, 507–522.
Louvet, J. N., Giammarino, C., Potier, O., and Pons, M. N. (2010). Adverse effects of erythromycin on the structure and chemistry of activated sludge. Environ. Pollut. 158, 688–693.
Luczkiewicz, A., Jankowska, K., Fudala-Ksiazek, S., and Olanczuk-Neyman, K. (2010). Antimicrobial resistance of fecal indicators in municipal wastewater treatment plant. Water Res. 44, 5089–5097.
Luo, Y., Mao, D., Rysz, M., Zhou, Q., Zhang, H., Xu, L., and Alvarez, P. J. J. (2010). Trends in antibiotic resistance genes occurrence in the Haihe River, China. Environ. Sci. Technol. 44, 7220–7225.
Lupo, A., Coyne, S., and Berendonk, T. U. (2012). Origin and evolution of antibiotic resistance: the common mechanisms of emergence and spread in water bodies. Front. Microbiol. 3:18. doi:10.3389/fmicb.2012.00018
Ma, L., Zhang, X.-X., Cheng, S., Zhang, Z., Shi, P., Liu, B., Wu, B., and Zhang, Y. (2011a). Occurrence, abundance and elimination of class 1 integrons in one municipal sewage treatment plant. Ecotoxicology 20, 968–973.
Ma, Y., Wilson, C. A., Novak, J. T., Riffat, R., Aynur, S., Murthy, S., and Pruden, A. (2011b). Effect of various sludge digestion conditions on sulfonamide, macrolide, and tetracycline resistance genes and class I integrons. Environ. Sci. Technol. 45, 7855–7861.
Márquez, C., Labbate, M., Ingold, A. J., Roy Chowdhury, P., Ramírez, M. S., Centrón, D., Borthagaray, G., and Stokes, H. W. (2008). Recovery of a functional class 2 integron from an Escherichia coli strain mediating a urinary tract infection. Antimicrob. Agents Chemother. 52, 4153–4154.
Martin, C., Timm, J., Rauzier, J., Gomez-Lus, R., Davies, J., and Gicquel, B. (1990). Transposition of an antibiotic resistance element in mycobacteria. Nature 345, 739–743.
Martínez, J. L. (2008). Antibiotics and antibiotic resistance genes in natural environments. Science 321, 365–367.
Martinez, J. L. (2009a). Environmental pollution by antibiotics and by antibiotic resistance determinants. Environ. Pollut. 157, 2893–2902.
Martinez, J. L. (2009b). The role of natural environments in the evolution of resistance traits in pathogenic bacteria. Proc. Biol. Sci. 276, 2521–2530.
Merlin, C., Bonot, S., Courtois, S., and Block, J.-C. (2011). Persistence and dissemination of the multiple-antibiotic-resistance plasmid pB10 in the microbial communities of wastewater sludge microcosms. Water Res. 45, 2897–2905.
Moura, A., Henriques, I., Ribeiro, R., and Correia, A. (2007). Prevalence and characterization of integrons from bacteria isolated from a slaughterhouse wastewater treatment plant. J. Antimicrob. Chemother. 60, 1243–1250.
Moura, A., Henriques, I., Smalla, K., and Correia, A. (2010). Wastewater bacterial communities bring together broad-host range plasmids, integrons and a wide diversity of uncharacterized gene cassettes. Res. Microbiol. 161, 58–66.
Moura, A., Pereira, C., Henriques, I., and Correia, A. (2012). Novel gene cassettes and integrons in antibiotic-resistant bacteria isolated from urban wastewaters. Res. Microbiol. 163, 92–100.
Munir, M., Wong, K., and Xagoraraki, I. (2011). Release of antibiotic resistant bacteria and genes in the effluent and biosolids of five wastewater utilities in Michigan. Water Res. 45, 681–693.
Naas, T., Mikami, Y., Imai, T., Poirel, L., and Nordmann, P. (2001). Characterization of In53, a class 1 plasmid- and composite transposon-located integron of Escherichia coli which carries an unusual array of gene cassettes. J. Bacteriol. 183, 235–249.
Nandi, S., Maurer, J. J., Hofacre, C., and Summers, A. O. (2004). Gram-positive bacteria are a major reservoir of class 1 antibiotic resistance integrons in poultry litter. Proc. Natl. Acad. Sci. U.S.A. 101, 7118–7122.
Nesvera, J., Hochmannová, J., and Pátek, M. (1998). An integron of class 1 is present on the plasmid pCG4 from Gram-positive bacterium Corynebacterium glutamicum. FEMS Microbiol. Lett. 169, 391–395.
Nijssen, S., Florijn, A., Top, J., Willems, R., Fluit, A., and Bonten, M. (2005). Unnoticed spread of integron-carrying Enterobacteriaceae in intensive care units. Clin. Infect. Dis. 41, 1–9.
Novo, A., and Manaia, C. (2010). Factors influencing antibiotic resistance burden in municipal wastewater treatment plants. Appl. Microbiol. Biotechnol. 87, 1157–1166.
Nunes-Düby, S. E., Kwon, H. J., Tirumalai, R. S., Ellenberger, T., and Landy, A. (1998). Similarities and differences among 105 members of the Int family of site-specific recombinases. Nucleic Acids Res. 26, 391–406.
Oberlé, K., Capdeville, M.-J., Berthe, T., Budzinski, H., and Petit, F. (2012). Evidence for a complex relationship between antibiotics and antibiotic-resistant Escherichia coli: from medical center patients to a receiving environment. Environ. Sci. Technol. 46, 1859–1868.
Ort, C., Lawrence, M. G., Reungoat, J., Eaglesham, G., Carter, S., and Keller, J. (2010). Determining the fraction of pharmaceutical residues in wastewater originating from a hospital. Water Res. 44, 605–615.
Ozgumus, O. B., Sandalli, C., Sevim, A., Celik-Sevim, E., and Sivri, N. (2009). Class 1 and class 2 integrons and plasmid-mediated antibiotic resistance in coliforms isolated from ten rivers in northern Turkey. J. Microbiol. 47, 19–27.
Partridge, S. R., Tsafnat, G., Coiera, E., and Iredell, J. R. (2009). Gene cassettes and cassette arrays in mobile resistance integrons. FEMS Microbiol. Rev. 33, 757–784.
Paulsen, I. T., Littlejohn, T. G., Rådström, P., Sundström, L., Sköld, O., Swedberg, G., and Skurray, R. A. (1993). The 3′ conserved segment of integrons contains a gene associated with multidrug resistance to antiseptics and disinfectants. Antimicrob. Agents Chemother. 37, 761–768.
Pauwels, B., and Verstraete, W. (2006). The treatment of hospital wastewater: an appraisal. J. Water Health 4, 405–416.
Pellegrini, C., Celenza, G., Segatore, B., Bellio, P., Setacci, D., Amicosante, G., and Perilli, M. (2011). Occurrence of class 1 and 2 integrons in resistant Enterobacteriaceae collected from a urban wastewater treatment plant: first report from central Italy. Microb. Drug Resist. 17, 229–234.
Pellegrini, C., Mercuri, P. S., Celenza, G., Galleni, M., Segatore, B., Sacchetti, E., Volpe, R., Amicosante, G., and Perilli, M. (2009). Identification of bla(IMP-22) in Pseudomonas spp. in urban wastewater and nosocomial environments: biochemical characterization of a new IMP metallo-enzyme variant and its genetic location. J. Antimicrob. Chemother. 63, 901–908.
Petrova, M., Gorlenko, Z., and Mindlin, S. (2011). Tn5045, a novel integron-containing antibiotic and chromate resistance transposon isolated from a permafrost bacterium. Res. Microbiol. 162, 337–345.
Picão, R. C., Poirel, L., Demarta, A., Silva, C. S. F., Corvaglia, A. R., Petrini, O., and Nordmann, P. (2008). Plasmid-mediated quinolone resistance in Aeromonas allosaccharophila recovered from a Swiss lake. J. Antimicrob. Chemother. 62, 948–950.
Rahube, T. O., and Yost, C. K. (2010). Antibiotic resistance plasmids in wastewater treatment plants and their possible dissemination into the environment. Afr. J. Biotechnol. 9, 9183–9190.
Ramírez, M. S., Piñeiro, S., and Centrón, D. (2010). Novel insights about class 2 integrons from experimental and genomic epidemiology. Antimicrob. Agents Chemother. 54, 699–706.
Ramírez, M. S., Quiroga, C., and Centrón, D. (2005). Novel rearrangement of a class 2 integron in two non-epidemiologically related isolates of Acinetobacter baumannii. Antimicrob. Agents Chemother. 49, 5179–5181.
Rodríguez-Minguela, C. M., Apajalahti, J. H. A., Chai, B., Cole, J. R., and Tiedje, J. M. (2009). Worldwide prevalence of class 2 integrases outside the clinical setting is associated with human impact. Appl. Environ. Microbiol. 75, 5100–5110.
Roe, M. T., Vega, E., and Pillai, S. D. (2003). Antimicrobial resistance markers of class 1 and class 2 integron-bearing Escherichia coli from irrigation water and sediments. Emerging Infect. Dis. 9, 822–826.
Rosewarne, C. P., Pettigrove, V., Stokes, H. W., and Parsons, Y. M. (2010). Class 1 integrons in benthic bacterial communities: abundance, association with Tn402-like transposition modules and evidence for coselection with heavy-metal resistance. FEMS Microbiol. Ecol. 72, 35–46.
Rosser, S. J., and Young, H. K. (1999). Identification and characterization of class 1 integrons in bacteria from an aquatic environment. J. Antimicrob. Chemother. 44, 11–18.
Rowe-Magnus, D. A., Guerout, A. M., Ploncard, P., Dychinco, B., Davies, J., and Mazel, D. (2001). The evolutionary history of chromosomal super-integrons provides an ancestry for multiresistant integrons. Proc. Natl. Acad. Sci. U.S.A. 98, 652–657.
Schmidt, A. S., Bruun, M. S., Dalsgaard, I., and Larsen, J. L. (2001). Incidence, distribution, and spread of tetracycline resistance determinants and integron-associated antibiotic resistance genes among motile aeromonads from a fish farming environment. Appl. Environ. Microbiol. 67, 5675–5682.
Scotta, C., Juan, C., Cabot, G., Oliver, A., Lalucat, J., Bennasar, A., and Albertí, S. (2011). Environmental microbiota represents a natural reservoir for dissemination of clinically relevant metallo-{beta}-lactamases. Antimicrob. Agents Chemother. 55, 5376–5379.
Shi, L., Zheng, M., Xiao, Z., Asakura, M., Su, J., Li, L., and Yamasaki, S. (2006). Unnoticed spread of class 1 integrons in Gram-positive clinical strains isolated in Guangzhou, China. Microbiol. Immunol. 50, 463–467.
Skurnik, D., Le Menac’h, A., Zurakowski, D., Mazel, D., Courvalin, P., Denamur, E., Andremont, A., and Ruimy, R. (2005). Integron-associated antibiotic resistance and phylogenetic grouping of Escherichia coli isolates from healthy subjects free of recent antibiotic exposure. Antimicrob. Agents Chemother. 49, 3062–3065.
Skurnik, D., Ruimy, R., Andremont, A., Amorin, C., Rouquet, P., Picard, B., and Denamur, E. (2006). Effect of human vicinity on antimicrobial resistance and integrons in animal faecal Escherichia coli. J. Antimicrob. Chemother. 57, 1215–1219.
Smith, J. L., Drum, D. J. V., Dai, Y., Kim, J. M., Sanchez, S., Maurer, J. J., Hofacre, C. L., and Lee, M. D. (2007). Impact of antimicrobial usage on antimicrobial resistance in commensal Escherichia coli strains colonizing broiler chickens. Appl. Environ. Microbiol. 73, 1404–1414.
Srinivasan, V., Nam, H.-M., Sawant, A. A., Headrick, S. I., Nguyen, L. T., and Oliver, S. P. (2008). Distribution of tetracycline and streptomycin resistance genes and class 1 integrons in Enterobacteriaceae isolated from dairy and nondairy farm soils. Microb. Ecol. 55, 184–193.
Stokes, H. W., and Gillings, M. R. (2011). Gene flow, mobile genetic elements and the recruitment of antibiotic resistance genes into Gram negative pathogens. FEMS Microbiol. Rev. 35, 790–819.
Stokes, H. W., and Hall, R. M. (1989). A novel family of potentially mobile DNA elements encoding site-specific gene-integration functions: integrons. Mol. Microbiol. 3, 1669–1683.
Stokes, H. W., Nesbø, C. L., Holley, M., Bahl, M. I., Gillings, M. R., and Boucher, Y. (2006). Class 1 integrons potentially predating the association with tn402-like transposition genes are present in a sediment microbial community. J. Bacteriol. 188, 5722–5730.
Taviani, E., Ceccarelli, D., Lazaro, N., Bani, S., Cappuccinelli, P., Colwell, R. R., and Colombo, M. M. (2008). Environmental Vibrio spp., isolated in Mozambique, contain a polymorphic group of integrative conjugative elements and class 1 integrons. FEMS Microbiol. Ecol. 64, 45–54.
Tennstedt, T., Szczepanowski, R., Braun, S., Pühler, A., and Schlüter, A. (2003). Occurrence of integron-associated resistance gene cassettes located on antibiotic resistance plasmids isolated from a wastewater treatment plant. FEMS Microbiol. Ecol. 45, 239–252.
Tschäpe, H. (1994). The spread of plasmids as a function of bacterial adaptability. FEMS Microbiol. Ecol. 15, 23–31.
Verner-Jeffreys, D. W., Welch, T. J., Schwarz, T., Pond, M. J., Woodward, M. J., Haig, S. J., Rimmer, G. S. E., Roberts, E., Morrison, V., and Baker-Austin, C. (2009). High prevalence of multidrug-tolerant bacteria and associated antimicrobial resistance genes isolated from ornamental fish and their carriage water. PLoS ONE 4, e8388. doi:10.1371/journal.pone.0008388
Wozniak, R. A. F., and Waldor, M. K. (2010). Integrative and conjugative elements: mosaic mobile genetic elements enabling dynamic lateral gene flow. Nat. Rev. Microbiol. 8, 552–563.
Wright, G. D. (2010). Antibiotic resistance in the environment: a link to the clinic? Curr. Opin. Microbiol. 13, 589–594.
Wright, M. S., Baker-Austin, C., Lindell, A. H., Stepanauskas, R., Stokes, H. W., and McArthur, J. V. (2008). Influence of industrial contamination on mobile genetic elements: class 1 integron abundance and gene cassette structure in aquatic bacterial communities. ISME J. 2, 417–428.
Xia, R., Guo, X., Zhang, Y., and Xu, H. (2010). qnrVC-like gene located in a novel complex class 1 integron harboring the ISCR1 element in an Aeromonas punctata strain from an aquatic environment in Shandong Province, China. Antimicrob. Agents Chemother. 54, 3471–3474.
Xu, H., Davies, J., and Miao, V. (2007). Molecular characterization of class 3 integrons from Delftia spp. J. Bacteriol. 189, 6276–6283.
Xu, Z., Li, L., Shirtliff, M. E., Peters, B. M., Peng, Y., Alam, M. J., Yamasaki, S., and Shi, L. (2010). First report of class 2 integron in clinical Enterococcus faecalis and class 1 integron in Enterococcus faecium in South China. Diagn. Microbiol. Infect. Dis. 68, 315–317.
Zhang, X., Wu, B., Zhang, Y., Zhang, T., Yang, L., Fang, H. H. P., Ford, T., and Cheng, S. (2009a). Class 1 integronase gene and tetracycline resistance genes tetA and tetC in different water environments of Jiangsu Province, China. Ecotoxicology 18, 652–660.
Keywords: integron, antibiotic resistance, soil, aquatic ecosystems, wastewater, agriculture, water
Citation: Stalder T, Barraud O, Casellas M, Dagot C and Ploy M-C (2012) Integron involvement in environmental spread of antibiotic resistance. Front. Microbio. 3:119. doi: 10.3389/fmicb.2012.00119
Received: 29 November 2011; Accepted: 13 March 2012;
Published online: 09 April 2012.
Edited by:
Rustam I. Aminov, University of Aberdeen, UKReviewed by:
Kornelia Smalla, Julius Kühn-Institut – Federal Research Centre for Cultivated Plants, GermanyVincent Burrus, Université de Sherbrooke, Canada
Laurent Poirel, French Institute of Health, France
Henning Sørum, Norwegian School of Veterinary Science, Norway
Holger Heuer, Julius Kühn-Institut – Federal Research Centre for Cultivated Plants, Germany
Copyright: © 2012 Stalder, Barraud, Casellas, Dagot and Ploy. This is an open-access article distributed under the terms of the Creative Commons Attribution Non Commercial License, which permits non-commercial use, distribution, and reproduction in other forums, provided the original authors and source are credited.
*Correspondence: Marie-Cécile Ploy, UMR Inserm 1092, Faculté de Médecine, 2 rue du docteur Marcland, Limoges, France. e-mail:bWFyaWUtY2VjaWxlLnBsb3lAdW5pbGltLmZy