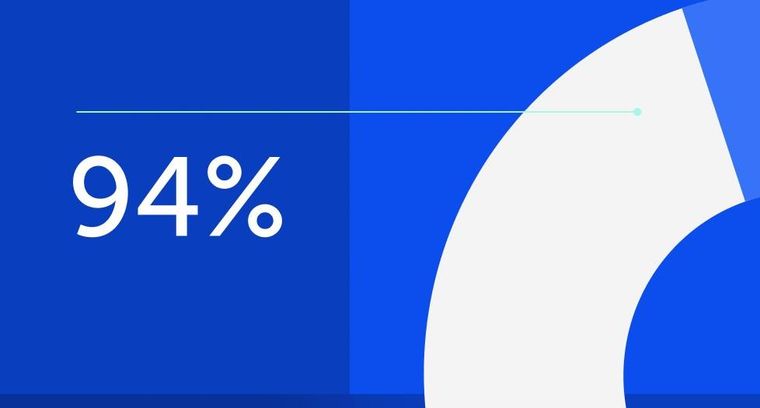
94% of researchers rate our articles as excellent or good
Learn more about the work of our research integrity team to safeguard the quality of each article we publish.
Find out more
MINI REVIEW article
Front. Microbiol., 03 January 2012
Sec. Virology
volume 2 - 2011 | https://doi.org/10.3389/fmicb.2011.00269
This article is part of the Research TopicStructural biology for virus researchView all 16 articles
Influenza A virus is an enveloped virus with a segmented, single-strand, negative-sense RNA genome. Its virions show spherical or filamentous shapes of about 100 nm in diameter and occasionally irregular morphology, which exemplifies the pleomorphic nature of these virions. Each viral RNA segment forms a ribonucleoprotein complex (RNP), along with an RNA-dependent RNA polymerase complex and multiple copies of nucleoproteins; the RNPs reside in the enveloped virions. Here, we focus on electron microscopic analyses of influenza virions and RNPs. Based on the morphological and structural observations obtained by using electron microscopic techniques, we present a model of the native morphology of the influenza virion.
Influenza A, B, and C viruses are members of the Orthomyxoviridae, which is a family of enveloped viruses with segmented, single-stranded, negative-sense RNA genomes (Palese, 2007). They are classified according to antigenic differences in their nucleoprotein (NP) and matrix protein (M1), which are major structural components of their virions. Of the three members, Influenza A viruses, which are further classified into 16 hemagglutinin (HA) subtypes and 9 neuraminidase (NA) subtypes on the basis of their antigenic properties, cause annual epidemics in humans and recurring pandemics that spread globally with severe consequences for human health and the economy.
The genome of influenza A virus consists of eight single-stranded, negative-sense RNA segments that form ribonucleoprotein complexes (RNPs) together with the viral RNA (vRNA)-dependent RNA polymerase complex [basic polymerase 1 (PB1), basic polymerase 2 (PB2), and acidic polymerase (PA)] and many nucleoprotein (NP) molecules. The genome encodes at least 12 viral proteins, most of which are necessary for efficient virus replication in host cells and for virion formation. During virus infection, the virus attaches to receptors (i.e., sialic acid linked to galactose) on the cell surface via HA virion surface proteins and enters the cell by clathrin-mediated endocytosis. The RNPs within the virion are released into the cytoplasm by acidic pH-dependent membrane fusion, again via the HA proteins, and are transported to the nucleus of the infected cells. Unlike most negative-sense RNA viruses, transcription and replication of the influenza virus genome take place in the nucleus. After the RNPs are synthesized in the nucleus, they are exported to the cytoplasm with the help of two viral proteins, M1 (Bui et al., 1996, 2000) and nuclear export protein (NEP/NS2; O’Neill et al., 1998; Ye et al., 1999; Baudin et al., 2001), via the cellular chromosome region maintenance 1 (Crm1) protein-dependent pathway (Neumann et al., 2000; Elton et al., 2001; Ma et al., 2001; Watanabe et al., 2001; Akarsu et al., 2003; Iwatsuki-Horimoto et al., 2004). They are then transported to the budding site of the apical plasma membrane of polarized cells, through a Rab 11-dependent vesicular transport pathway (Amorim et al., 2011; Eisfeld et al., 2011; Momose et al., 2011). The transmembrane proteins HA, NA, and an ion channel protein (M2) are conveyed to the budding site by a standard exocytic pathway through the endoplasmic reticulum and Golgi apparatus. M1 is presumed to interact with both the RNPs and the cytoplasmic tails of HA, NA, and M2 and to act as a bridge between them at the budding site (Zhang et al., 2000; McCown and Pekosz, 2005). Eventually, all of the viral components, including the viral transmembrane proteins, M1, and RNPs, are orderly assembled into progeny virions. The progeny virions are released from the apical plasma membrane into the extracellular environment, where NA plays an important role in cleaving sialic acids bound to receptors on the cell surface and HA proteins on the progeny virions.
Although the morphology of influenza A virions, whether budding virions or purified virions, has been investigated extensively for many years by using various electron microscopic methods, knowledge of the virion structure remains limited, mainly due to pleomorphism, which complicates morphological and structural analyses and impedes investigators efforts to draw conclusions about the native structure of the virion. However, recent electron microscopic analyses have revealed several important morphological and structural features of influenza virions, which provide valuable information about the native morphology of these structures. This review summarizes the current progress in virion morphology studies and presents a model of the likely native morphology of influenza A virion.
Virions possess a lipid envelope that is derived from the host cellular membrane during the budding. They are generally spherical or elliptical in shape, ranging from approximately 80–120 nm in diameter and are occasionally filamentous, reaching more than 20 μm in length. Sometimes, however, they take on an irregular shape. Virions are covered with numerous membrane-spanning glycoproteins, HA and NA, and small amounts of M2. The peripheral membrane protein, M1, which is one of the most abundant viral proteins in the virion, binds to the lipid envelope to maintain virion morphology. The segmented genome is enclosed in the virion in the form of the RNP. Although small amounts of NEP are present within the virion (Yasuda et al., 1993), its localization is unknown.
Most laboratory-adapted strains of influenza A virus are predominantly spherical or elliptical, whereas clinical isolates are predominantly filamentous and their adaptation in eggs results in the loss of this filamentous phenotype (Chu et al., 1949; Kilbourne and Murphy, 1960). The filamentous phenotype is thought to be a genetic trait, as shown by virus gene reassortment experiments (Smirnov et al., 1991) and reverse genetics studies (Bourmakina and Garcia-Sastre, 2003; Elleman and Barclay, 2004). Such studies have demonstrated that the M1 and M2 proteins are important determinants of filamentous virion formation (Hughey et al., 1995; Roberts et al., 1998; Bourmakina and Garcia-Sastre, 2003). In addition, the polarized cell phenotype and actin cytoskeleton network also play important roles in the formation of filamentous virions (Roberts and Compans, 1998), suggesting that several factors are involved in determining virion morphology. The significance of the filamentous morphology for virus replication and pathogenesis remains uncertain; however, the fact that clinical isolates, independent of HA and NA subtype, consistently form filamentous shapes strongly suggests that this filamentous phenotype is essential for virus survival in nature. The filamentous morphology may, for example, facilitate cell-to-cell transmission of progeny virions in the respiratory mucosa of infected individuals.
In addition to the spherical, elliptical, and filamentous virions, irregular-shaped virions are sometimes observed when using negative-staining electron microscopy (negative-staining EM) of purified virions (Figure 1; Stevenson and Biddle, 1966; Almeida and Waterson, 1967; Wrigley, 1979). Interestingly, such virions have never been reported when ultrathin-section transmission electron microscopy (ultrathin-section EM) or scanning electron microscopy (SEM) is used to view budding virions. This discrepancy is thought to be partially due to artifacts caused during sample preparation for negative-staining, since artifacts can be introduced into fragile biological specimens on air drying (Nermut and Frank, 1971; Ruigrok et al., 1992). However, other artifacts may also be included, because such irregular-shaped virions are also reported when using cryoelectron microscopy (cryoEM) of purified virions (Harris et al., 2006; Nayak et al., 2009), which does not involve air drying. Earlier reports suggested that the pleomorphism is introduced during the storage of virions at 4°C after they are harvested (Choppin et al., 1961; Nermut and Frank, 1971). More importantly, it was recently demonstrated that virion morphology is substantially affected by ultracentrifugation, which is often involved in the negative-staining process, resulting in the appearance of morphologically irregular virions (Sugita et al., 2011). When virions are chemically fixed with glutaraldehyde (GLA), which presumably creates cross-links among the membrane-bound M1 proteins that form the layer underneath the viral envelope as well as among M1 proteins and the cytoplasmic tails of the HA, NA, and M2 transmembrane proteins, the virion morphology is relatively conserved even after ultracentrifugation. This finding is consistent with observations made when using ultrathin-section TEM and SEM of budding virions, where morphologically irregular virions have never been found; sample processing of the ultrathin-section TEM and SEM usually begins with GLA fixation, and does not involve ultracentrifugation. Taken together, the pleomorphism of influenza virions observed when using negative-staining EM and cryoEM is an artifact that is mainly introduced during sample preparation procedures that involve ultracentrifugation and storage.
Figure 1. Electron micrograph of influenza virions. The virions were purified by ultracentrifugation, and visualized by negative-staining EM. Bars, 200 nm. (A) Spherical virions [A/WSN/33 (H1N1)], (B) filamentous virions [A/Udorn/307/72 (H3N2)], and (C) irregular-shaped virions [A/Puerto Rico/8/34 (H1N1)].
The eight vRNA segments, which contain conserved sequences at their 3′ and 5′ ends that are partially complimentary to each other and form the core promoter (Skehel and Hay, 1978; Robertson, 1979; Desselberger et al., 1980), range in size from 890 to 2341 bases. Each vRNA segment is separately encapsidated by NP to form the RNP, which has a flexible, twisted rod-like structure that is folded back and coiled on itself. The rod-like RNPs are approximately 12 nm in diameter, but vary in length from approximately 30–110 nm (Pons et al., 1969; Compans et al., 1972). The length of each rod-like RNP correlates with the length of each vRNA segment (Compans et al., 1972).
Although the morphology of the purified RNPs has been well characterized, the conformation of RNPs inside virions had been largely unknown for a long time. When virions were examined by using negative-staining EM or cryoEM, a continuous strand of 7–8 nm in diameter, which was regularly packed in the form of a helix, was observed within disrupted virions (Hoyle et al., 1961; Apostolov and Flewett, 1965; Schulze, 1972; Almeida and Brand, 1975; Murti et al., 1980, 1992; Booy et al., 1985; Harris et al., 2006). Although the strands were observed only at a low frequency, they were the sole structure clearly visualized by using negative-staining EM and cryoEM. Therefore, it was proposed that RNPs exist as a single continuous helix, which would be fragmented into multiple rod-like RNPs during the purification process of RNPs (Almeida and Brand, 1975; Murti et al., 1980). Later, however, it was demonstrated by use of negative-staining EM (Ruigrok et al., 1989) and cryoEM (Calder et al., 2010), that the continuous helix found within disrupted virions was a layer of M1 proteins underneath the lipid envelope.
How, then, are the RNP fragments arranged within the virion? By using thin-section EM, it is shown that progeny virions budding from the plasma membrane of infected cells contain a distinct arrangement of RNPs (Oxford and Hockley, 1987; Noda et al., 2006). The RNPs are always suspended from the top of the budding virions and are oriented perpendicular to the budding tip. Many transversely sectioned budding virions contain eight RNPs, in which a central RNP is consistently surrounded by seven others. Serial transverse ultrathin-sections of whole budding virions have revealed that the eight RNPs within a virion differ in length, suggesting that the budding virion contains different kinds of vRNAs (Compans et al., 1972). Filamentous virions also contain eight RNPs arranged in this distinct pattern. Interestingly, the eight RNPs are confined to the top of the filamentous virion and the reminder of the filamentous virion is empty, indicating that such filamentous virions do not randomly incorporate multiple sets of eight RNPs (Noda et al., 2006). Recent cryoEM analyses of purified virions confirmed that they contain eight RNPs arranged in a distinct pattern (Harris et al., 2006; Calder et al., 2010). Furthermore, the RNPs are restricted to one end of the filamentous particles that are released from infected cells, which is consistent with the observations made by using ultrathin-section EM of budding virions (Noda et al., 2006). Thus, virions, regardless of their shape, incorporate an organized set of eight RNPs.
Based on recent microscopic analyses, a schematic diagram of a native influenza virion is depicted in Figure 2. Whether spherical, elliptical (Figure 2 left), or filamentous (Figure 2 right), the lipid-enveloped virions are regular in shape and covered with HA and NA glycoproteins and M2 proteins. Abundant HA proteins are distributed over the virion surface. Single NA proteins can be observed anywhere on the virion surface, whereas a cluster of NA proteins is also found at the end of the virion that is opposite to the end where the RNPs are located (Calder et al., 2010). Because the RNPs bind to the inner leaflet of the envelope at the distal end of budding virion (Noda et al., 2006; Calder et al., 2010), the NA cluster would play a role in release from the infected cell by destroying the HA binding to receptors on the cell surface. Given that membrane scission is caused by M2 proteins (Rossman et al., 2010), M2 proteins are likely located at the proximal end of the budding virion, on the same side as the NA cluster. In addition, the M2 protein plays an important role in RNP incorporation (McCown and Pekosz, 2005; Iwatsuki-Horimoto et al., 2006), suggesting that it should also be present at the distal end of the budding virion, where the M2 protein and RNPs could associate with each other. The M1 protein forms a matrix layer underneath the lipid envelope that is arranged as an ordered helix composed of multiple copies of M1 proteins that contribute to the virion morphology (Calder et al., 2010).
Figure 2. Schematic diagram of influenza virions. Left, an elliptical virion; right, a filamentous virion. Note that both virions contain an organized set of eight RNPs at the end of the virion.
The eight rod-like RNPs, which differ in length, are arranged in a distinct pattern, in which seven peripheral RNPs surround a central RNP (Oxford and Hockley, 1987; Noda et al., 2006). Such an arrangement is observed not only in budding virions, but also in isolated virions (Harris et al., 2006; Calder et al., 2010), suggesting that there are interactions among the RNPs to maintain this organization. The eight RNPs are consistently associated with the inner envelope at the distal end of the budding virion and oriented almost perpendicular to the budding tip (Oxford and Hockley, 1987; Noda et al., 2006), indicating a polar organization of the virion.
Electron microscopic approaches are useful methods for investigating morphological and structural features of viruses. However, when fragile, lipid-enveloped viruses, including influenza viruses, are examined by using electron microscopy, artifactual effects such as ultracentrifugation, storage, and air drying should be considered. If not, misleading information can be visualized. Recent electron microscopic analyses of influenza virions clearly show that the native virion morphology is uniformly spherical, elliptical, or filamentous. Irregularly shaped virus particles are mainly artifacts caused during sample processing, indicating that such virus particles unlikely reflect the true virion structure. Accordingly, it is important to pay close attention to sample processing, when structurally examining influenza virions with electron microscopic techniques.
Despite the fact that various electron microscopic analyses have contributed to our knowledge of influenza virion morphology, much about the internal structure of the virion remains unknown. For instance, how do the RNPs associate with the envelope at the tip of the virion? How are the eight RNPs arranged in their distinct pattern? Do they interact with each other? If so, where and how do they interact? Although solving these issues by using conventional two-dimensional electron microscopic methods, such as thin-section EM and negative-staining EM would be difficult, if not impossible, they can be addressed by using three-dimensional analysis of RNPs within the virion by means of electron tomography, which provides higher resolution images.
The author declares that the research was conducted in the absence of any commercial or financial relationships that could be construed as a potential conflict of interest.
I thank Susan Watson for editing the manuscript. This work is supported by a Grant-in-Aid for Young Scientists from the Japan Society for the Promotion of Science.
Akarsu, H., Burmeister, W. P., Petosa, C., Petit, I., Müller, C. W., Ruigrok, R. W., and Baudin, F. (2003). Crystal structure of the M1 protein-binding domain of the influenza A virus nuclear export protein (NEP/NS2). EMBO J. 22, 4646–4655.
Almeida, J. D., and Brand, C. M. A. (1975). Morphological study of the internal component of influenza virus. J. Gen. Virol. 27, 313–318.
Almeida, J. D., and Waterson, A. P. (1967). Some observations on the envelope of an influenza virus. J. Gen. Microbiol. 46, 107–110.
Amorim, M. J., Bruce, E. A., Reed, E. K., Foeglein, A., Mahen, R., Stuart, A. D., and Digard, P. (2011). A Rab11- and microtubule-dependent mechanism for cytoplasmic transport of influenza A virus viral RNA. J. Virol. 85, 4134–4156.
Apostolov, K., and Flewett, T. H. (1965). Internal structure of influenza virus. Virology 26, 506–508.
Baudin, F., Petit, I., Weissenhorn, W., and Ruigrok, R. W. (2001). In vitro dissection of the membrane and RNP binding activities of influenza virus M1 protein. Virology 281, 102–108.
Booy, F. P., Ruigrok, R. W., and van Bruggen, E. F. (1985). Electron microscopy of influenza virus. A comparison of negatively stained and ice-embedded particles. J. Mol. Biol. 184, 667–676.
Bourmakina, S. V., and Garcia-Sastre, A. (2003). Reverse genetics studies on the filamentous morphology of influenza A virus. J. Gen. Virol. 84, 517–527.
Bui, M., Whittaker, G., and Helenius, A. (1996). Effect of M1 protein and low pH on nuclear transport of influenza virus ribonucleoproteins. J. Virol. 70, 8391–8401.
Bui, M., Wills, E. G., Helenius, A., and Whittaker, G. R. (2000). Role of the influenza virus M1 protein in nuclear export of viral ribonucleoproteins. J. Virol. 74, 1781–1786.
Calder, L. J., Wasilewski, S., Berriman, J. A., and Rosenthal, P. B. (2010). Structural organization of a filamentous influenza A virus. Proc. Natl. Acad. Sci. U.S.A. 107, 10685–10690.
Choppin, P. W., Murphy, J. S., and Stoeckenius, W. (1961). The surface structure of influenza virus filaments. Virology 13, 548–550.
Chu, C. M., Dawson, L. M., and Elford, W. J. (1949). Filamentous forms associated with newly isolated influenza virus. Lancet 1, 602.
Compans, R. W., Content, J., and Duesberg, P. H. (1972). Structure of the ribonucleoprotein of influenza virus. J. Virol. 10, 795–800.
Desselberger, U., Racaniello, V. R., Zazra, J. J., and Palese, P. (1980). The 3′ and 5′-terminal sequences of influenza A, B and C virus RNA segments are highly conserved and show partial inverted complementarity. Gene 8, 315–328.
Eisfeld, A. J., Kawakami, E., Watanabe, T., Neumann, G., and Kawaoka, Y. (2011). RAB11A is essential for transport of the influenza virus genome to the plasma membrane. J. Virol. 85, 6117–6126.
Elleman, C. J., and Barclay, W. S. (2004). The M1 matrix protein controls the filamentous phenotype of influenza A virus. Virology 321, 144–153.
Elton, D., Simpson-Holley, M., Archer, K., Medcalf, L., Hallam, R., McCauley, J., and Digard, P. (2001). Interaction of the influenza virus nucleoprotein with the cellular CRM1-mediated nuclear export pathway. J. Virol. 75, 408–419.
Harris, A., Cardone, G., Winkler, D. C., Heymann, J. B., Brecher, M., White, J. M., and Steven, A. C. (2006). Influenza virus pleiomorphy characterized by cryoelectron tomography. Proc. Natl. Acad. Sci. U.S.A. 103, 19123–19127.
Hoyle, L., Horne, R. W., and Waterson, A. P. (1961). The structure and composition of the myxoviruses. II. Components released from the influenza virus particle by ether. Virology 13, 448–459.
Hughey, P. G., Roberts, P. C., Holsinger, L. J., Zebedee, S. L., Lamb, R. A., and Compans, R. W. (1995). Effects of antibody to the influenza A virus M2 protein on M2 surface expression and virus assembly. Virology 212, 411–421.
Iwatsuki-Horimoto, K., Horimoto, T., Fujii, Y., and Kawaoka, Y. (2004). Generation of influenza A virus NS2 (NEP) mutants with an altered nuclear export signal sequence. J. Virol. 78, 10149–10155.
Iwatsuki-Horimoto, K., Horimoto, T., Noda, T., Kiso, M., Maeda, J., Watanabe, S., Muramoto, Y., Fujii, K., and Kawaoka, Y. (2006). The cytoplasmic tail of the influenza A virus M2 protein plays a role in viral assembly. J. Virol. 80, 5233–5240.
Kilbourne, E. D., and Murphy, J. S. (1960). Genetic studies of influenza viruses. 1. Viral morphology and growth capacity as exchangeable genetic traits. Rapid in ovo adaptation of early passage Asian strain isolates by combination with PR8. J. Exp. Med. 111, 387–406.
Ma, K., Roy, A. M., and Whittaker, G. R. (2001). Nuclear export of influenza virus ribonucleoproteins: identification of an export intermediate at the nuclear periphery. Virology 282, 215–220.
McCown, M. F., and Pekosz, A. (2005). The influenza A virus M2 cytoplasmic tail is required for infectious virus production and efficient genome packaging. J. Virol. 79, 3595–3605.
Momose, F., Sekimoto, T., Ohkura, T., Jo, S., Kawaguchi, A., Nagata, K., and Morikawa, Y. (2011). Apical transport of influenza A virus ribonucleoprotein requires Rab11-positive recycling endosome. PLoS ONE 6, e21123. doi:10.1371/journal.pone.0021123
Murti, K. G., Bean, W. J. Jr., and Webster, R. G. (1980). Helical ribonucleoproteins of influenza virus: an electron microscopic analysis. Virology 104, 224–229.
Murti, K. G., Brown, P. S., Bean, W. J. Jr., and Webster, R. G. (1992). Composition of the helical internal components of influenza virus as revealed by immunogold labeling/electron microscopy. Virology 186, 294–299.
Nayak, D. P., Balogun, R. A., Yamada, H., Zhou, Z. H., and Barman, S. (2009). Influenza virus morphogenesis and budding. Virus Res. 143, 147–161.
Nermut, M. V., and Frank, H. (1971). Fine structure of influenza A2 (Singapore) as revealed by negative staining, freeze-drying and freeze-etching. J. Gen. Virol. 10, 37–51.
Neumann, G., Hughes, M. T., and Kawaoka, Y. (2000). Influenza A virus NS2 protein mediates vRNP nuclear export through NES-independent interaction with hCRM1. EMBO J. 19, 6751–6758.
Noda, T., Sagara, H., Yen, A., Takada, A., Kida, H., Cheng, R. H., and Kawaoka, Y. (2006). Architecture of ribonucleoprotein complexes in influenza A virus particles. Nature 439, 490–492.
O’Neill, R. E., Talon, J., and Palese, P. (1998). The influenza virus NEP (NS2 protein) mediates the nuclear export of viral ribonucleoproteins. EMBO J. 17, 288–296.
Oxford, J. S., and Hockley, D. J. (1987). Orthomyxoviridae. Animal Virus Structure. Amsterdam: Elsevier, 213–232.
Palese, P. (2007). “Orthomyxoviridae,” in Fields Virology, 5th Edn, eds D. M. Knipe, P. M. Howley, D. E. Griffin, R. A. Lamb, M. A. Martin, B. Roizman, and S. E. Straus (Philadelphia: Lippincott Williams & Wilkins), 1647–1689.
Pons, M. W., Schulze, I. T., Hirst, G. K., and Hauser, R. (1969). Isolation and characterization of the ribonucleoprotein of influenza virus. Virology 39, 250–259.
Roberts, P. C., and Compans, R. W. (1998). Host cell dependence of viral morphology. Proc. Natl. Acad. Sci. U.S.A. 95, 5746–5751.
Roberts, P. C., Lamb, R. A., and Compans, R. W. (1998). The M1 and M2 proteins of influenza A virus are important determinants in filamentous particle formation. Virology 240, 127–137.
Robertson, J. S. (1979). 5′ and 3′ terminal nucleotide sequences of the RNA genome segments of influenza virus. Nucleic Acids Res. 6, 3745–3757.
Rossman, J. S., Jing, X., Leser, G. P., and Lamb, R. A. (2010). Influenza virus M2 protein mediates ESCRT-independent membrane scission. Cell 142, 902–913.
Ruigrok, R. W., Calder, L. J., and Wharton, S. A. (1989). Electron microscopy of the influenza virus submembranal structure. Virology 173, 311–316.
Ruigrok, R. W., Hewat, E. A., and Wade, R. H. (1992). Low pH deforms the influenza virus envelope. J. Gen. Virol. 73, 995–998.
Schulze, I. T. (1972). The structure of influenza virus. II. A model based on the morphology and composition of subviral particles. Virology 47, 181–196.
Skehel, J. J., and Hay, A. J. (1978). Nucleotide sequences at the 5′ termini of influenza virus RNAs and their transcripts. Nucleic Acids Res. 5, 1207–1219.
Smirnov, Y. U. A., Kuznetsova, M. A., and Kaverin, N. V. (1991). The genetic aspects of influenza virus filamentous particle formation. Arch. Virol. 118, 279–284.
Stevenson, J. P., and Biddle, F. (1966). Pleomorphism of influenza virus particles under the electron microscope. Nature 212, 619–621.
Sugita, Y., Noda, T., Sagara, H., and Kawaoka, Y. (2011). Ultracentrifugation deforms unfixed influenza A virions. J. Gen. Virol. 92, 2485–2493.
Watanabe, K., Takizawa, N., Katoh, M., Hoshida, K., Kobayashi, N., and Nagata, K. (2001). Inhibition of nuclear export of ribonucleoprotein complexes of influenza virus by leptomycin B. Virus Res. 77, 31–42.
Yasuda, J., Nakada, S., Kato, A., Toyoda, T., and Ishihama, A. (1993). Molecular assembly of influenza virus: association of the NS2 protein with virion matrix. Virology 196, 249–255.
Ye, Z., Liu, T., Offringa, D. P., Mclnnis, J., and Levandowski, R. A. (1999). Association of influenza virus matrix protein with ribonucleoproteins. J. Virol. 73, 7467–7473.
Keywords: influenza virus, morphology, electron microscopy
Citation: Noda T (2012) Native morphology of influenza virions. Front. Microbio. 2:269. doi: 10.3389/fmicb.2011.00269
Received: 02 December 2011; Accepted: 17 December 2011;
Published online: 03 January 2012.
Edited by:
Akio Adachi, The University of Tokushima Graduate School, JapanReviewed by:
Kiyoko Iwatsuki-Horimoto, University of Tokyo, JapanCopyright: © 2012 Noda. This is an open-access article distributed under the terms of the Creative Commons Attribution Non Commercial License, which permits non-commercial use, distribution, and reproduction in other forums, provided the original authors and source are credited.
*Correspondence: Takeshi Noda, Division of Virology, Department of Microbiology and Immunology, Institute of Medical Science, University of Tokyo, 4-6-1 Shirokanedai, Minatoku, Tokyo 108-8639, Japan. e-mail:dC1ub2RhQGltcy51LXRva3lvLmFjLmpw
Disclaimer: All claims expressed in this article are solely those of the authors and do not necessarily represent those of their affiliated organizations, or those of the publisher, the editors and the reviewers. Any product that may be evaluated in this article or claim that may be made by its manufacturer is not guaranteed or endorsed by the publisher.
Research integrity at Frontiers
Learn more about the work of our research integrity team to safeguard the quality of each article we publish.