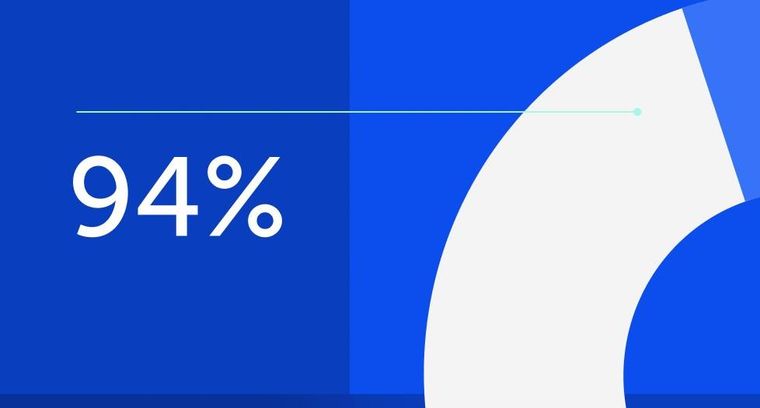
94% of researchers rate our articles as excellent or good
Learn more about the work of our research integrity team to safeguard the quality of each article we publish.
Find out more
REVIEW article
Front. Med. Technol., 01 February 2024
Sec. Medtech Data Analytics
Volume 6 - 2024 | https://doi.org/10.3389/fmedt.2024.1320690
This article is part of the Research TopicThe Novel Progress in Tumor Immunology, Cancer Nano Immunotherapy and Cellular Immunotherapy (CAR T cells)View all 4 articles
Sex hormones play a pivotal role in modulating various physiological processes, with emerging evidence underscoring their influence on cancer progression and treatment outcomes. This review delves into the intricate relationship between sex hormones and cancer, elucidating the underlying biological mechanisms and their clinical implications. We explore the multifaceted roles of estrogen, androgens, and progesterone, highlighting their respective influence on specific cancers such as breast, ovarian, endometrial, and prostate. Special attention is given to estrogen receptor-positive (ER+) and estrogen receptor-negative (ER−) tumors, androgen receptor signaling, and the dual role of progesterone in both promoting and inhibiting cancer progression. Clinical observations reveal varied treatment responses contingent upon hormonal levels, with certain therapies like tamoxifen, aromatase inhibitors, and anti-androgens demonstrating notable success. However, disparities in treatment outcomes between males and females in hormone-sensitive cancers necessitate further exploration. Therapeutically, the utilization of hormone replacement therapy (HRT) during cancer treatments presents both potential risks and benefits. The promise of personalized therapies, tailored to an individual’s hormonal profile, offers a novel approach to optimizing therapeutic outcomes. Concurrently, the burgeoning exploration of new drugs and interventions targeting hormonal pathways heralds a future of more effective and precise treatments for hormone-sensitive cancers. This review underscores the pressing need for a deeper understanding of sex hormones in cancer therapy and the ensuing implications for future therapeutic innovations.
Sex hormones, such as androgens and estrogens, are pivotal in dictating the physiological and morphological differences between males and females (1). These hormones extend their influence beyond reproduction, significantly modulating a myriad of pathological processes, including cancer (2). Over time, the conventional understanding of steroid hormones as transcription factors that predominantly regulate reproductive organs has changed (3). Sex steroid receptors, including estrogen (ER), progesterone (PR), and androgen (AR) receptors, were initially perceived as transcription factors governing physiological and pathological responses in reproductive organs (4). Upon hormone binding, these receptors were thought to translocate to the nucleus, recognizing hormone-responsive elements (HREs) and regulating gene transcription (5). However, recent studies have revealed different roles for steroid receptors that includes both non-genomic and genomic pathways (6). Beyond their classical transcriptional functions, steroid receptors can rapidly activate transduction pathways, such as PI3K/AKT or MAPKs, influencing various physiological and pathological processes in diverse anatomical sites (7). This paradigm shift in understanding suggests that steroid receptors play a broader role in regulating key genes, impacting organ development, function, and, notably, contributing to the development and progression of cancers (8, 9). While classical hormone-related cancers like breast, prostate, and ovary have been extensively studied, a growing body of research investigates the influence of sex steroid receptors in various cancers (10). Researchers are exploring the complex relationships between steroid hormones and their receptors and the prevalence of cancer in both men and women (6).
Looking further into the extensive literature surrounding sex hormones and cancer, reveals that these biological factors have profound influence beyond their well-established roles in sexual development (2, 11, 12). Sex hormones emerging as key players in the complex landscape of cancer. As illustrated in Figure 1, the incidence of specific cancers varies between males and females. Such disparities are often attributed to the influence of sex hormones (13). The nuanced interplay between sex hormones and cellular pathways in different tissues can either augment or suppress cancer's onset, progression, and response to therapies (14). Consequently, this interaction often manifests as marked differences in cancer outcomes across sexes.
Figure 1. A visual representation comparing the prevalence of select cancer types in males and females. The associated key hormones with potential influence on each cancer are also indicated.
Androgens, predominantly testosterone, produced mainly by the testes and in smaller quantities by the adrenal glands, drive the development of male reproductive tissues and the manifestation of secondary sexual characteristics (15). Notably, prostate cancer exhibits a profound association with androgen levels, rendering androgen deprivation therapy a therapeutic mainstay for affected individuals (16). Elevated androgen levels also harbor implications for liver cancer (17, 18) and have hinted at roles in bladder, kidney, lung, and breast malignancies (19, 20).
Conversely, estrogens, chiefly estradiol, produced in the ovaries, with contributions from the adrenal glands and adipose tissues, are essential for female reproductive organ growth, menstrual cycle regulation, and secondary sexual characteristic manifestation (21). In oncology, heightened estrogen levels are unequivocally linked to breast tumor proliferation (22). Additionally, the coordinated action of estrogens and their specific receptors plays a critical role in the pathogenesis of ovarian and endometrial cancers, highlighting their multifaceted influence on female-centric malignancies (23–25).
However, the scope of these hormones transcends sex-specific cancers. Intriguingly, estrogen-related pathways have been identified as potential targets for improving immunotherapy responses in melanoma, a skin cancer unconfined to sex-specific occurrence (26). Moreover, novel insights suggest that estrogen signaling, particularly in myeloid cells, fosters immune suppression in melanoma, and targeting ERα could further enhance immunotherapeutic outcomes (23). Concurrently, interventions combining radiation therapy and Fulvestrant, an estrogen receptor antagonist, have shown to bolster immunotherapy responses in ER + breast cancer (27). Such revelations accentuate the expanding horizon of hormonal influences in cancer therapy (28).
The literature also highlights the importance of the immune microenvironment and its interactions with estrogens and their receptors in shaping therapeutic outcomes in breast cancer (25, 29). Hormone receptor antagonism has been shown to amplify the expression of immunotherapeutic targets on breast tumors, suggesting a potential synergistic approach for treatment (30). Furthermore, emerging evidence underscores the role of β-estradiol in non-small cell lung cancer’s tumorigenesis, prognosis, and therapeutic responses (31, 32). Sex differences in immunotherapy responses are evident, necessitating exploratory endeavors for novel therapeutic combinations (33, 34). The synthesis, secretion, and equilibrium of these hormones can be swayed by a constellation of factors including age, metabolic status, and environmental exposures (35, 36). As we navigate the intricate nexus of sex hormones and their omnipresence in the oncological landscape, it becomes palpably clear that a comprehensive understanding of these dynamics is not just requisite but instrumental in paving the way for tailored, sex-specific therapeutic paradigms, heralding a new epoch in cancer care (37–39). Recognizing the profound influence of sex hormones on immune modulation, researchers are harnessing immunotherapy's potential to tailor treatments based on hormonal profiles. Such strategies have shown promise, particularly when targeting hormone-responsive cancers, underscoring the significance of understanding hormone-immune system interplay (40–47).
Estrogen, often used as a collective term for several related hormones, plays crucial roles in numerous physiological functions, particularly within the reproductive and cardiovascular systems (48). Beyond these functions, estrogen's interactions at cellular levels have implications in oncology (49). Estrogen exerts its effects primarily through its binding with specific intracellular receptors, specifically estrogen receptor alpha (ERα) and estrogen receptor beta (ERβ) (50). Such interactions initiate a cascade of gene transcription events that in turn regulate protein synthesis and dictate cellular actions vital for tissue morphogenesis, cellular proliferation, differentiation, and apoptosis; processes intrinsically linked with oncogenesis (2, 25).
Estrogen acts via both non-genomic and genomic signaling mechanisms (51). The actions can be mediated either by processes that do not involve direct binding to DNA (non-genomic effects) or by direct binding of estrogen receptor complexes to particular regions in gene promoters (genomic effects). Direct genomic signaling is considered the classical mechanism of estrogen signaling, and non-genomic signaling is considered the non-classic mechanism (52).
In genomic signaling, estrogen binds to nuclear receptors (ERα and ERβ), inducing conformational changes that lead to dimerization and translocation to the nucleus. There, the receptors bind to estrogen response elements (EREs) on DNA, regulating gene expression directly (53). However, not all estrogen-regulated genes have EREs, and approximately 35% of estrogen-responsive genes lack them. When it comes to indirect genomic signaling, estrogen receptors interact with transcription factors like the activator protein (AP)-1, nuclear factor-κB (NF-κB) and stimulating protein-1 (Sp-1), stimulating gene expression without direct DNA binding. This mechanism involves protein-protein interactions and influences target genes such as LDL receptor, progesterone receptor, and endothelial nitric oxide synthase (54).
Non-genomic signaling, on the other hand, operates outside the nucleus and involves rapid responses (51). The GPER1 receptor and variants of ERα and ERβ are associated with this pathway. Estrogen activates signal-transduction cascades, including PLC/PKCs, Ras/Raf/MAPK, PI3K/Akt, and cAMP/PKA pathways. These cascades result in the phosphorylation of transcription factors, affecting gene expression indirectly. The membrane receptors ERα and ERβ may interact with scaffold proteins and membrane receptors, activating signaling cascades and influencing transcriptional regulation (50, 55).
The crosstalk between genomic and non-genomic pathways involves complex interactions between nuclear receptors, membrane receptors, and various transcription factors. Two proposed mechanisms include the dimerization of nuclear estrogen receptor complexes with phosphorylated transcription factors and the activation of protein kinase cascades at the plasma membrane, leading to enhanced transcriptional activity (56). Furthermore, estrogen receptors can be activated independently of ligands, triggered by phosphorylation events involving protein kinases, inflammatory cytokines, cell adhesion molecules, and growth factors. In summary, estrogen signaling is a multifaceted process involving both genomic and non-genomic pathways, with intricate crosstalk mechanisms contributing to the regulation of gene expression in various cellular contexts (50).
Breast cancer serves as a prime example of estrogen's significant influence on oncogenesis. Estrogen-responsive breast cancer cells utilize the hormone's interaction with ERα to promote cell cycle progression and inhibit apoptosis (57). Furthermore, estrogen boosts the expression of certain growth factors, including insulin-like growth factor (IGF) and vascular endothelial growth factor (VEGF), enhancing tumor growth and angiogenesis (58, 59). About 75% of breast cancer tumors express ERα (60). Treatments for patients with these ERα-positive tumors typically include selective ER modulators (e.g., Tamoxifen) (61), selective ER degraders (e.g., Fulvestrant) (62), or aromatase inhibitors (63). Even though these treatments can effectively restrict ER-positive breast cancer cell growth, prolonged ERα inhibition may stimulate immunosuppressive activities in these cells, triggering several immune checkpoint processes (64, 65). Hormonal therapies, especially those targeting ER+, are used in treating tumors that predominantly rely on estrogenic signaling. On the contrary, ER- tumors, lacking these receptors, tend to be more aggressive and require tailored therapeutic strategies (66–68).
Elevated estrogen levels correlate with an increased risk of ovarian cancer. The oncogenic properties of estradiol, a form of estrogen, are particularly concerning when considering the adverse effects of estrogen-only treatments during menopause (69). Several studies have also indicated that estrogen may promote the proliferation and migration of ovarian cancer cells (70). The deeper mechanistic relationship between elevated estrogen levels and increased ovarian cancer risk is still under investigation (53, 54). Estrogen’s influence is also evident in endometrial cancer. Prolonged exposure to estrogen without the balancing effect of progesterone can heighten the risk of this type of cancer (71, 72). In the context of prostate cancer, prevailing treatments can sometimes result in castration-resistant forms of the disease. Targeting specific ER subtypes (α or β) offers a promising avenue for mitigating the growth and spread of prostate cancer cells. Notably, ERβ has been identified as a potential tumor suppressor, suggesting its activation could be leveraged in therapeutic strategies against prostate cancer (73).
Androgens, primarily testosterone and its derivatives, play foundational roles in male physiology, governing secondary sexual features, reproductive capacities, and bone health (74). Testosterone, produced by Leydig cells in response to luteinizing hormone, activates androgen receptor (AR) signaling in Sertoli cells (SCs). The AR signaling pathway can be categorized into classical and non-classical pathways (75).
In the classical pathway, androgen binding to cytoplasmic AR leads to nuclear translocation, dimerization, and binding to androgen response elements (AREs) to regulate gene transcription. This process is relatively slow, requiring 30–45 min for transcriptional changes (75).
The non-classical pathway involves membrane-bound AR, rapid activation within 1 min, and subsequent activation of kinases such as Src, ERK, and Akt. These pathways play roles in regulating gene expression, spermatogenesis, and cellular processes (76). Three distinct non-classical signaling pathways associated with testosterone action have been identified in the testis. The first and main pathway involves the binding of testosterone to membrane-bound AR, interacts with the SH3 domain of the SRC proto-oncogene (Src), and triggers a cascade involving EGFR, Ras kinase, MAPK cascades (Ras-Raf-MEK-ERK), and downstream transcription factors like cAMP-response element-binding protein (CREB) (77). Another non-classical pathway involves the PI3K/Akt pathway, activated by the PI3K subunit p85α. An additional non-classical pathway specific to immature Sertoli cells induces depolarization within the K+ ATP channels, mediated by G protein-induced activation of phospholipase C, resulting in a quick influx of Ca2+ and subsequent activation of signaling molecules (75, 78, 79).
Beyond these primary functions, androgens have a pronounced footprint in oncology, especially in prostate and breast cancers, and are further implicated in other malignancies (80, 81). The prostate gland is intricately sensitive to androgens, with cellular interactions predominantly mediated through the AR. When androgens bind to the AR, it triggers the activation of the receptor, facilitating its transportation into the cell nucleus (82). Consequently, this process plays a crucial role in modulating gene transcription, which is vital for cellular growth and survival. This physiologically essential pathway can, however, shift to pathological roles, especially in early stages of prostate cancer that exhibit androgen-driven growth. Such observations underlie the therapeutic approach of androgen deprivation therapy (ADT) for prostate cancer (83).
Beyond the prostate, the story of androgens grows more complex. Dihydrotestosterone (DHT), a powerful derivative of testosterone, has been shown to stimulate certain types of breast cancer cells, suggesting an androgenic influence in breast tumor biology (80, 81). Post-menopausal increases in systemic androgen levels have also been linked to heightened risks of endometrial cancer, possibly via indirect pathways that bolster estrogen synthesis (84, 85). The AR has been meticulously studied in cancers that display hormone sensitivity, like the prostate and breast cancers mentioned. Cancers that are AR-positive may depend on androgens for growth and progression. Research hints at the significance of AR signaling even in some hormone-independent cancers. For instance, male prevalence in liver and stomach cancers exceeds that of females, suggesting potential hormonal influence (86). This observation extends to other malignancies like bladder, kidney, pancreas, liver, endometrial, specific lymphomas, and salivary gland cancers, in which AR signaling might display varied effects (19, 87).
With advancing research uncovering the diverse roles of AR, there's potential for developing treatments that target either AR directly or its downstream signaling pathways. This perspective offers promising avenues for cancer therapy (88). Additionally, recent clinical insights suggest that ADT might enhance the effectiveness of some immunotherapies, including immune checkpoint inhibitors (89).
A cornerstone for female reproductive processes, progesterone also occupies a pivotal position in cancer biology. Progesterone acts through both genomic (classical) and nongenomic (non-classical) signaling pathways, affecting different tissues and potentially having an impact on the development of cancer (90).
Classical progesterone receptors (PRs), including PR-A and PR-B isoforms, operate as nuclear receptors, initiating transcriptional changes upon ligand binding. These receptors undergo conformational changes, dimerize, translocate to the nucleus, and bind to progesterone response elements (PREs) to regulate target gene transcription (91). Various PR isoforms, such as PR-A, PR-B, PR-C, PR-M, PR-S, and PR-T, exhibit tissue-selective signaling with distinct roles in organs like the mammary gland and uterus. In classical signaling, progesterone plays a crucial role in mammary gland proliferation, impacting pathways like RANKL, CCND1 (Cyclin D1), and WNT-1. PR-B signaling is predominant in normal mammary gland function, while PR-A is crucial for uterine and ovarian functions. Prolonged exposure to progesterone, as observed during the luteal phase, may lead to dysregulated pathways and contribute to breast cancer (92, 93).
The non-classical progesterone signaling, highlighting rapid progestin-activated pathways that involve membrane-associated actions on EGFR, c-Src, and MAPK (94). Membrane progesterone receptors (mPRs), a class of proteins that resemble G protein-coupled receptors (GPCRs) in structure, mediate non-classical signaling. The five mPRs (mPRα, mPRβ, mPRɣ, mPRε, and mPRδ) activate MAPKs, ERK1/2, and intracellular Ca2+ influx. Additionally, membrane-associated progesterone receptors, PGRMC1 and PGRMC2, contribute to non-classical signaling, influencing cholesterol synthesis, cytochrome P450 (CYP) enzymes, and intracellular heme transport (93, 95).
Progesterone roles in oncology are multifaceted, often marked by a duality wherein it can either support or counteract cancer progression, contingent upon its complex signaling interplay (96). In the realm of breast cancer, progesterone occasionally stimulates cellular growth, particularly when its receptors engage with certain growth factors (97). However, in a contrasting light, there are scenarios in which progesterone acts against breast cancer by predominantly steering breast cell differentiation (39). Interactions with other hormonal pathways, especially estrogen, further complicate progesterone’s role. Often, it acts to temper estrogen-driven cell proliferation in tissues, especially in the endometrium. Any disturbances in this intricate balance, be it due to a deficit of progesterone or an excess of estrogen, can amplify risks associated with endometrial cancer (98).
A pivotal aspect of progesterone's interaction with cancer involves its interplay with other signaling pathways. Notably, its communication with growth factors like insulin-like growth factor (IGF) can modify cellular hormonal responses, a comprehension of which can inform targeted therapeutic approaches (38). When discussing colon cancer, combined actions of estradiol and progesterone appear to be instrumental. Their collective activity is posited to inhibit tumor proliferation and induce apoptosis, perhaps through the activation of ERβ (99). Research into progesterone's effects on prostate cancer (100), breast cancer (97), and ovarian cancer (101) paints a complex picture with findings that are, at times, contradictory or multifarious in interpretation.
As depicted in Table 1, a summary of the sex hormone signaling pathways, focusing on estrogen, androgen, and progesterone was provided.
Cancer treatment outcomes can vary greatly. Although numerous elements play a role, recent research has highlighted the crucial role of sex hormone levels in determining treatment efficacy (37). This intersection of hormones and therapeutic responses has rapidly emerged as a key area of focus. Table 2 offers a detailed summary of various sex hormones and their links with particular cancers.
Hormonal status can be a major determinant in how patients respond to cancer treatment. This is observed in hormone-sensitive cancers like breast and prostate, but also others, such as melanoma (121) and colon cancer (122, 123). Breast cancer's deep-rooted connection to hormones serves as a clear illustration. Therapies that target estrogen pathways, such as Tamoxifen or aromatase inhibitors, are beneficial for patients with ER+ tumors. However, their efficacy diminishes in those with lower estrogen levels (124). Additionally, combination strategies like radiation therapy and Fulvestrant have shown improved immunotherapy responses in ER+ breast cancer (27). In prostate cancer, the success of androgen deprivation therapy (ADT) is built on the understanding that these tumors often rely on androgens (125). However, outcomes with ADT can vary significantly depending on baseline testosterone levels. Additionally, long-term ADT usage has been linked with an increased risk of colorectal cancer (16, 126). As personalized medicine becomes a standard, understanding how sex hormones influence treatment efficacy is paramount (127). Additionally, advancements in biomedical imaging, nanotechnology, COVID-19 research, and immunotherapy hold potential to enhance the efficacy of sex-specific cancer therapies. These fields, while not yet fully integrated into sex-specific treatment paradigms, offer promising avenues for exploring and understanding the impact of hormones on cancer. While their direct contribution to sex-specific therapy is still emerging, there is no doubt that these interdisciplinary domains will significantly influence future developments in this area (42–46, 128–164).
Sex hormones are undeniably influential in targeted cancer treatments. Over the years, strategies that modulate these hormones or their receptors have shown promise across various cancers. Tamoxifen, for instance, has become a cornerstone for ER+ breast cancer due to its ability to antagonize estrogen receptors (165), significantly reducing recurrence rates. Aromatase inhibitors, such as letrozole and anastrozole, by decreasing estrogen production, hinder tumor growth (166). In prostate cancer, anti-androgens like bicalutamide and enzalutamide have revolutionized treatment by interrupting vital growth signals (39, 167). Despite their efficacy, these therapies are not without challenges. Tamoxifen might elevate the risk of endometrial cancer in post-menopausal women, while aromatase inhibitors can lead to bone loss (31, 168). While anti-androgens are transformative, they come with cardiovascular risks and might eventually lead to resistance (25, 169). Ongoing studies, such as the development of selective estrogen receptor degraders (SERDs) that interact with ER-positive immune cells, show promise in enhancing the response to immune checkpoint inhibitors in breast cancer (24).
Sex hormones intricately link to the differences observed in treatment outcomes between males and females (170). Although breast cancer is mainly found in women, its rare occurrence in men brings unique challenges (38). Similarly, prostate cancer’s androgen signaling complexities provide insights that can be compared with certain breast cancers in females. Furthermore, research in lung cancer has unveiled potential gender differences in treatment responses. Some studies suggest women may have more favorable outcomes with specific targeted therapies than men (171, 172). Additionally, hormone-targeting agents can induce gender-specific side effects; for instance, women on aromatase inhibitors might deal with symptoms of estrogen deficiency, whereas men on anti-androgen treatments might develop gynecomastia (173). Conclusively understanding the interplay among gender, sex hormones, and cancer treatments is vital for optimizing patient care.
The therapeutic landscape for hormone-sensitive cancers is vast and continually evolving. HRT, which is used in the management of menopausal symptoms in women and conditions such as hypogonadism in men, remains a contentious subject in oncology (174). Although HRT has been seen to offer protective effects against conditions like osteoporosis and improve quality of life, its relationship with cancer is complex (175). Some studies, like the Women’s Health Initiative, have shown an increased risk of breast cancer with combined estrogen-progestin therapy, while estrogen-only therapy might have a protective effect. Additionally, other studies have highlighted an increased ovarian cancer risk with prolonged HRT (165, 176), whereas evidence suggests a protective effect against colorectal cancer (37). The effects of estrogen and its receptors on the immune tumor microenvironment in breast cancer (25) further complicate this dynamic. For breast cancer survivors, the use of HRT has been a concern, especially in potentially reactivating dormant cancer cells or progressing undetected micrometastases. When discussing testosterone replacement therapy (TRT) in men, concerns analogous to HRT in women arise, particularly regarding prostate cancer risk, which is further complicated with evidence suggesting that long-term androgen deprivation therapy for prostate cancer might increase colorectal cancer risk (16). In summary, the correlation between HRT and cancer remains intricate, highlighting the necessity for individualized treatment plans.
The trend in oncological treatments is shifting from a one-size-fits-all approach to personalized strategies, recognizing the importance of individual variations. For instance, the role of hormones in influencing anticancer immune surveillance elements underscores the importance of tailoring therapies based on hormonal profiles (38). Treatments for breast cancer, like the combination of radiation therapy and Fulvestrant, which has shown enhanced immunotherapy response in ER+ breast cancers (27), are reliant on the patient's hormonal environment. Similar strategies are evident in other cancers. For instance, estrogen signaling plays a role in myeloid cells promoting immune suppression in melanoma, suggesting that targeting these pathways might improve immunotherapy response (23). As we move forward, treatments might integrate hormonal profiling with genetic and molecular markers, paving the way for more personalized and effective therapies. Advanced biomedical imaging techniques have shed light on the nuanced interactions between sex hormones and tumor microenvironments, providing invaluable insights into differential therapy responses in male and female patients and paving the way for individualized treatment regimens (45, 141, 153, 154).
The recognition of the interplay between hormones and cancer progression has provided an avenue for new therapeutic breakthroughs. There is increasing evidence suggesting potential new treatments, such as the ERβ agonist LY500307 that suppresses lung cancer metastasis by activating antitumor neutrophils (58), or the utilization of newly designed SERDs that interact with ER-positive immune cells to improve the response to immune checkpoint inhibitors in breast cancer (24). The growing interest extends to targeting enzymes involved in hormone synthesis and metabolism. As biotechnological advancements like antibody-drug conjugates emerge, these allow for a more targeted delivery to hormone-sensitive tumors. The potential of gene therapy in modulating specific hormonal pathways also presents promising avenues for treatment, especially when considering how sex steroid hormones might interact with DNA repair components affecting genotoxic therapy response (165). Additionally, insights into sex differences in immunotherapy responses (33) highlight the potential of novel combinations that might be more tailored to the individual’s hormonal landscape. As our comprehension of the hormonal pathways in cancer deepens, it opens the door to more innovative treatments, promising better outcomes for patients. In the realm of personalized medicine, biomaterials stand out by creating hormone-responsive matrices and scaffolds for tissue engineering, enabling a more in-depth study of hormone-tumor interactions and contributing to the development of innovative therapeutic interventions tailored to specific hormonal environments (132, 150, 157).
Sex hormones, as important regulators of numerous physiological processes, wield profound influence on the landscape of cancer therapy outcomes. This review underscores the nuanced relationship between these hormones and various cancers, revealing the intricate web of interactions that determine therapeutic efficacy. From the direct implications of estrogen, androgens, and progesterone on tumors such as those of the breast, prostate, ovaries, and endometrium to the broader effects on cancer treatment outcomes, the role of sex hormones is indisputably significant. Clinically, it is paramount to consider hormonal levels as influential determinants in therapeutic decision-making. Their influence on treatment responsiveness, especially in hormone-sensitive cancers, emphasizes the need for a tailored approach in therapeutic strategies. Moreover, the disparities witnessed in treatment outcomes between males and females further accentuate the criticality of this consideration. Yet, while strides have been made in recognizing and acting upon these insights, much remains to be explored. Personalized therapies based on an individual's hormonal profile and future treatments targeting hormonal pathways are avenues that hold immense promise. We strongly advocate for intensified research efforts in this domain, aiming to refine therapeutic approaches and develop strategies that cater to the individual intricacies of each patient. By delving deeper into the realm of sex hormones and their connections with cancer, the medical community stands poised to usher in an era of enhanced therapeutic precision and efficacy.
PB: Writing – review & editing. JH: Writing – review & editing. SZ: Writing – review & editing.
The author(s) declare that no financial support was received for the research, authorship, and/or publication of this article.
SZ and PB were employed by the company MetasFree LLC.
The remaining author declares that the research was conducted in the absence of any commercial or financial relationships that could be construed as a potential conflict of interest.
All claims expressed in this article are solely those of the authors and do not necessarily represent those of their affiliated organizations, or those of the publisher, the editors and the reviewers. Any product that may be evaluated in this article, or claim that may be made by its manufacturer, is not guaranteed or endorsed by the publisher.
1. Kroon J, Pereira AM, Meijer OC. Glucocorticoid sexual dimorphism in metabolism: dissecting the role of sex hormones. Trends Endocrinol Metab. (2020) 31(5):357–67. doi: 10.1016/j.tem.2020.01.010
2. Folkerd EJ, Dowsett M. Influence of sex hormones on cancer progression. J Clin Oncol. (2010) 28(26):4038–44. doi: 10.1200/JCO.2009.27.4290
3. Strauss III JF, FitzGerald GA. Steroid hormones and other lipid molecules involved in human reproduction, In: Yen and Jaffe's reproductive endocrinology. Amesterdam: Elsevier (2019). p. 75–114. e7. doi: 10.1016/B978-0-323-47912-7.00004-4
4. Whirledge S, Cidlowski JA. Steroid hormone action. In: Yen and jaffe's reproductive endocrinology. Amesterdam: Elsevier (2019). p. 115–31. e4. doi: 10.1016/B978-0-323-47912-7.00005-6
5. Hayakawa A, Kurokawa T, Kanemoto Y, Sawada T, Mori J, Kato S. Skeletal and gene-regulatory functions of nuclear sex steroid hormone receptors. J Bone Miner Metab. (2022) 40(3):361–74. doi: 10.1007/s00774-021-01306-2
6. Giovannelli P, Ramaraj P, Williams C. Role of sex steroids and their receptor in cancers. Front Endocrinol (Lausanne). (2022) 13:883229. doi: 10.3389/fendo.2022.883229
7. Klinge CM. Steroid hormone receptors and signal transduction processes. Princ Endocrinol Hormone Act. (2018):187–232. doi: 10.1007/978-3-319-44675-2_9
8. Mahadik N, Bhattacharya D, Padmanabhan A, Sakhare K, Narayan KP, Banerjee R. Targeting steroid hormone receptors for anti-cancer therapy—a review on small molecules and nanotherapeutic approaches. Wiley Interdiscip Rev Nanomed Nanobiotechnol. (2022) 14(2):e1755. doi: 10.1002/wnan.1755
9. Font-Díaz J, Jiménez-Panizo A, Caelles C, Vivanco MD, Pérez P, Aranda A, et al. Nuclear receptors: lipid and hormone sensors with essential roles in the control of cancer development. Semin Cancer Biol. (2021) 73:58–75. doi: 10.1016/j.semcancer.2020.12.007
10. Thiebaut C, Vlaeminck-Guillem V, Trédan O, Poulard C, Le Romancer M. Non-genomic signaling of steroid receptors in cancer. Mol Cell Endocrinol. (2021) 538:111453. doi: 10.1016/j.mce.2021.111453
11. Auchus RJ, Sharifi N. Sex hormones and prostate cancer. Annu Rev Med. (2020) 71:33–45. doi: 10.1146/annurev-med-051418-060357
12. Folkerd E, Dowsett M. Sex hormones and breast cancer risk and prognosis. Breast. (2013) 22:S38–43. doi: 10.1016/j.breast.2013.07.007
13. Vera R, Juan-Vidal O, Safont-Aguilera MJ, de la Peña FA, Del Alba AG. Sex differences in the diagnosis, treatment and prognosis of cancer: the rationale for an individualised approach. Clin Transl Oncol. (2023) 45:1–8. doi: 10.1007/s12094-023-03112-w
14. Rubin JB, Lagas JS, Broestl L, Sponagel J, Rockwell N, Rhee G, et al. Sex differences in cancer mechanisms. Biol Sex Differ. (2020) 11:1–29. doi: 10.1186/s13293-020-00291-x
15. Smith LB, Walker WH. The regulation of spermatogenesis by androgens. Semin Cell Dev Biol. (2014) 30:2–13. doi: 10.1016/j.semcdb.2014.02.012
16. Gillessen S, Templeton A, Marra G, Kuo YF, Valtorta E, Shahinian VB. Risk of colorectal cancer in men on long-term androgen deprivation therapy for prostate cancer. J Natl Cancer Inst. (2010) 102(23):1760–70. doi: 10.1093/jnci/djq419
17. Li H, Li Y, Lu JW, Huo X, Gong Z. Liver-specific androgen receptor knockout attenuates early liver tumor development in zebrafish. Sci Rep. (2019) 9(1):10645. doi: 10.1038/s41598-019-46378-3
18. Zhang H, Spencer K, Burley SK, Zheng XS. Toward improving androgen receptor-targeted therapies in male-dominant hepatocellular carcinoma. Drug Discov Today. (2021) 26(6):1539–46. doi: 10.1016/j.drudis.2021.02.001
19. Chang C, Lee S, Yeh S, Chang T. Androgen receptor (AR) differential roles in hormone-related tumors including prostate, bladder, kidney, lung, breast and liver. Oncogene. (2014) 33(25):3225–34. doi: 10.1038/onc.2013.274
20. Li P, Chen J, Miyamoto H. Androgen receptor signaling in bladder cancer. Cancers (Basel). (2017) 9(2):20. doi: 10.3390/cancers9020020
21. Hamilton KJ, Hewitt SC, Arao Y, Korach KS. Estrogen hormone biology. Curr Top Dev Biol. (2017) 125:109–46. doi: 10.1016/bs.ctdb.2016.12.005
22. Maharjan CK, Mo J, Wang L, Kim MC, Wang S, Borcherding N, et al. Natural and synthetic estrogens in chronic inflammation and breast cancer. Cancers. (2021) 14(1):206. doi: 10.3390/cancers14010206
23. Chakraborty B, Byemerwa J, Shepherd J, Haines CN, Baldi R, Gong W, et al. Inhibition of estrogen signaling in myeloid cells increases tumor immunity in melanoma. J Clin Invest. (2021) 131(23). doi: 10.1172/JCI151347
24. Márquez-Garbán DC, Deng G, Comin-Anduix B, Garcia AJ, Xing Y, Chen HW, et al. Antiestrogens in combination with immune checkpoint inhibitors in breast cancer immunotherapy. J Steroid Biochem Mol Biol. (2019) 193:105415. doi: 10.1016/j.jsbmb.2019.105415
25. Segovia-Mendoza M, Morales-Montor J. Immune tumor microenvironment in breast cancer and the participation of estrogen and its receptors in cancer physiopathology. Front Immunol. (2019) 10:348. doi: 10.3389/fimmu.2019.00348
26. Lin M, Du T, Tang X, Liao Y, Cao L, Zhang Y, et al. An estrogen response-related signature predicts response to immunotherapy in melanoma. Front Immunol. (2023) 14:1109300. doi: 10.3389/fimmu.2023.1109300
27. O'Leary KA, Bates AM, Jin WJ, Burkel BM, Sriramaneni RN, Emma SE, et al. Estrogen receptor blockade and radiation therapy cooperate to enhance the response of immunologically cold ER+ breast cancer to immunotherapy. Breast Cancer Res. (2023) 25(1):1–15. doi: 10.1186/s13058-022-01559-3
28. Haupt S, Caramia F, Klein SL, Rubin JB, Haupt Y. Sex disparities matter in cancer development and therapy. Nat Rev Cancer. (2021) 21(6):393–407. doi: 10.1038/s41568-021-00348-y
29. Zhang Y, Chan HL, Garcia-Martinez L, Karl DL, Weich N, Slingerland JM, et al. Estrogen induces dynamic ERα and RING1B recruitment to control gene and enhancer activities in luminal breast cancer. Sci Adv. (2020) 6(23):eaaz7249. doi: 10.1126/sciadv.aaz7249
30. Jaini R, Loya MG, Eng C. Immunotherapeutic target expression on breast tumors can be amplified by hormone receptor antagonism: a novel strategy for enhancing efficacy of targeted immunotherapy. Oncotarget. (2017) 8(20):32536. doi: 10.18632/oncotarget.15812
31. Smida T, Bruno TC, Stabile LP. Influence of estrogen on the NSCLC microenvironment: a comprehensive picture and clinical implications. Front Oncol. (2020) 10:137. doi: 10.3389/fonc.2020.00137
32. Deng S, Ramos-Castaneda M, Velasco WV, Clowers MJ, Gutierrez BA, Noble O, et al. Interplay between estrogen and Stat3/NF-κB-driven immunomodulation in lung cancer. Carcinogenesis. (2020) 41(11):1529–42. doi: 10.1093/carcin/bgaa064
33. Botticelli A, Onesti CE, Zizzari I, Cerbelli B, Sciattella P, Occhipinti M, et al. The sexist behaviour of immune checkpoint inhibitors in cancer therapy? Oncotarget. (2017) 8(59):99336. doi: 10.18632/oncotarget.22242
34. Bakhshi P, Nourizadeh M, Sharifi L, Farajollahi MM, Mohsenzadegan M. Development of dendritic cell loaded MAGE-A2 long peptide; a potential target for tumor-specific T cell-mediated prostate cancer immunotherapy. Cancer Cell Int. (2023) 23(1):270. doi: 10.1186/s12935-023-03108-0
35. Fucic A, Duca RC, Galea KS, Maric T, Garcia K, Bloom MS, et al. Reproductive health risks associated with occupational and environmental exposure to pesticides. Int J Environ Res Public Health. (2021) 18(12):6576. doi: 10.3390/ijerph18126576
36. Peter A, Kantartzis K, Machann J, Schick F, Staiger H, Machicao F, et al. Relationships of circulating sex hormone-binding globulin with metabolic traits in humans. Diabetes. (2010) 59(12):3167–73. doi: 10.2337/db10-0179
37. Rakshith HT, Lohita S, Rebello AP, Goudanavar PS, Naveen NR. Sex differences in drug effects and/or toxicity in oncology. Curr Res Pharmacol Drug Discov. (2023) 4:100152. doi: 10.1016/j.crphar.2022.100152
38. Orzołek I, Sobieraj J, Domagała-Kulawik J. Estrogens, cancer and immunity. Cancers (Basel). (2022) 14(9):2265. doi: 10.3390/cancers14092265
39. Özdemir BC, Dotto G-P. Sex hormones and anticancer immunity. Clin Cancer Res. (2019) 25(15):4603–10. doi: 10.1158/1078-0432.CCR-19-0137
40. Shekarian T, Zinner CP, Bartoszek EM, Duchemin W, Wachnowicz AT, Hogan S, et al. Immunotherapy of glioblastoma explants induces interferon-γ responses and spatial immune cell rearrangements in tumor center, but not periphery. Sci Adv. (2022) 8(26):eabn9440. doi: 10.1126/sciadv.abn9440
41. Sepand MR, Bigdelou B, Maghsoudi AS, Sanadgol N, Ho JQ, Chauhan P, et al. Ferroptosis: environmental causes, biological redox signaling responses, cancer and other health consequences. Coord Chem Rev. (2023) 480:215024. doi: 10.1016/j.ccr.2023.215024
42. Ho JQ, Sepand MR, Bigdelou B, Shekarian T, Esfandyarpour R, Chauhan P, et al. The immune response to COVID-19: does sex matter? Immunology. (2022) 166(4):429–43. doi: 10.1111/imm.13487
43. Pauliah M, Zanganeh S, Erfanzadeh M, Ho J. Tumor-targeted Therapy, in Iron Oxide Nanoparticles for Biomedical Applications. Elsevier (2018). p. 273–90.
44. Zanganeh S, Ho JQ, Spitler R, Jafari T, Khakpash N, Erfanzadeh M, et al. Cancer Therapy, in Iron Oxide Nanoparticles for Biomedical Applications. Amsterdam, Netherlands: Elsevier (2018). p. 291–307.
45. Zanganeh S, Spitler R, Hutter G, Ho JQ, Pauliah M, Mahmoudi M. Tumor-associated macrophages, nanomedicine and imaging: the axis of success in the future of cancer immunotherapy. Immunotherapy. (2017) 9(10):819–35. doi: 10.2217/imt-2017-0041
46. Alvim RG, Georgala P, Nogueira L, Somma AJ, Nagar K, Thomas J, et al. Combined OX40 agonist and PD-1 inhibitor immunotherapy improves the efficacy of vascular targeted photodynamic therapy in a urothelial tumor model. Molecules. (2021) 26(12):3744. doi: 10.3390/molecules26123744
47. Rao RR, Long JZ, White JP, Svensson KJ, Lou J, Lokurkar I, et al. Meteorin-like is a hormone that regulates immune-adipose interactions to increase beige fat thermogenesis. Cell. (2014) 157(6):1279–91. doi: 10.1016/j.cell.2014.03.065
48. Chambliss K, Mineo C, Shaul PW. Endothelial biology of estrogen and cardiovascular disease. Endocrinology. (2023) 164(10):bqad122. doi: 10.1210/endocr/bqad122
49. Yaşar P, Ayaz G, User SD, Güpür G, Muyan M. Molecular mechanism of estrogen–estrogen receptor signaling. Reprod Med Biol. (2017) 16(1):4–20. doi: 10.1002/rmb2.12006
50. Fuentes N, Silveyra P. Estrogen receptor signaling mechanisms. Adv Protein Chem Struct Biol. (2019) 116:135–70. doi: 10.1016/bs.apcsb.2019.01.001
51. Azuma K, Inoue S. Genomic and non-genomic actions of estrogen: recent developments. Biomol Concepts. (2012) 3(4):365–70. doi: 10.1515/bmc-2012-0002
52. Vrtačnik P, Ostanek B, Mencej-Bedrač S, Marc J. The many faces of estrogen signaling. Biochem Med (Zagreb). (2014) 24(3):329–42. doi: 10.11613/BM.2014.035
53. Song D, He H, Indukuri R, Huang Z, Stepanauskaite L, Sinha I, et al. ERα and ERβ homodimers in the same cellular context regulate distinct transcriptomes and functions. Front Endocrinol (Lausanne). (2022) 13:930227. doi: 10.3389/fendo.2022.930227
54. Marino M, Galluzzo P, Ascenzi P. Estrogen signaling multiple pathways to impact gene transcription. Curr Genomics. (2006) 7(8):497–508. doi: 10.2174/138920206779315737
55. Guo RX, Wei LH, Tu Z, Sun PM, Wang JL, Zhao D, et al. 17β-estradiol activates PI3K/akt signaling pathway by estrogen receptor (ER)-dependent and ER-independent mechanisms in endometrial cancer cells. J Steroid Biochem Mol Biol. (2006) 99(1):9–18. doi: 10.1016/j.jsbmb.2005.11.013
56. Levin ER, Hammes SR. Nuclear receptors outside the nucleus: extranuclear signalling by steroid receptors. Nat Rev Mol Cell Biol. (2016) 17(12):783–97. doi: 10.1038/nrm.2016.122
57. Khan MZI, Uzair M, Nazli A, Chen J-Z. An overview on estrogen receptors signaling and its ligands in breast cancer. Eur J Med Chem. (2022) 241:114658. doi: 10.1016/j.ejmech.2022.114658
58. Zhao L, Huang S, Mei S, Yang Z, Xu L, Zhou N, et al. Pharmacological activation of estrogen receptor beta augments innate immunity to suppress cancer metastasis. Proc Natl Acad Sci USA. (2018) 115(16):E3673–81. doi: 10.1073/pnas.1803291115
59. Avino S, De Marco P, Cirillo F, Santolla MF, De Francesco EM, Perri MG, et al. Stimulatory actions of IGF-I are mediated by IGF-IR cross-talk with GPER and DDR1 in mesothelioma and lung cancer cells. Oncotarget. (2016) 7(33):52710. doi: 10.18632/oncotarget.10348
60. Siersbæk R, Kumar S, Carroll JS. Signaling pathways and steroid receptors modulating estrogen receptor α function in breast cancer. Genes Dev. (2018) 32(17-18):1141–54. doi: 10.1101/gad.316646.118
61. Cao L, Xiang G, Liu F, Xu C, Liu J, Meng Q, et al. A high AR: eRα or PDEF: eRα ratio predicts a sub-optimal response to tamoxifen therapy in ERα-positive breast cancer. Cancer Chemother Pharmacol. (2019) 84:609–20. doi: 10.1007/s00280-019-03891-6
62. Yeh WL, Shioda K, Coser KR, Rivizzigno D, McSweeney KR, Shioda T. Fulvestrant-induced cell death and proteasomal degradation of estrogen receptor α protein in MCF-7 cells require the CSK c-src tyrosine kinase. PLoS One. (2013) 8(4):e60889. doi: 10.1371/journal.pone.0060889
63. Almeida CF, Teixeira N, Oliveira A, Augusto TV, Correia-da-Silva G, Ramos MJ, et al. Discovery of a multi-target compound for estrogen receptor-positive (ER+) breast cancer: involvement of aromatase and ERs. Biochimie. (2021) 181:65–76. doi: 10.1016/j.biochi.2020.11.023
64. Hühn D, Martí-Rodrigo P, Mouron S, Hansel C, Tschapalda K, Porebski B, et al. Prolonged estrogen deprivation triggers a broad immunosuppressive phenotype in breast cancer cells. Mol Oncol. (2022) 16(1):148–65. doi: 10.1002/1878-0261.13083
65. Kajihara N, Ge Y, Seino K-i. Blocking of oestrogen signals improves anti-tumour effect regardless of oestrogen receptor alpha expression in cancer cells. Br J Cancer. (2023) 129(6):935–46. doi: 10.1038/s41416-023-02381-0
66. Rizzo P, Miao H, D'Souza G, Osipo C, Yun J, Zhao H, et al. Cross-talk between notch and the estrogen receptor in breast cancer suggests novel therapeutic approaches. Cancer Res. (2008) 68(13):5226–35. doi: 10.1158/0008-5472.CAN-07-5744
67. Switzer CH, Glynn SA, Ridnour LA, Cheng RY, Vitek MP, Ambs S, et al. Nitric oxide and protein phosphatase 2A provide novel therapeutic opportunities in ER-negative breast cancer. Trends Pharmacol Sci. (2011) 32(11):644–51. doi: 10.1016/j.tips.2011.07.001
68. Skor MN, Wonder EL, Kocherginsky M, Goyal A, Hall BA, Cai Y, et al. Glucocorticoid receptor antagonism as a novel therapy for triple-negative breast cancer. Clin Cancer Res. (2013) 19(22):6163–72. doi: 10.1158/1078-0432.CCR-12-3826
69. Johansson Å, Schmitz D, Höglund J, Hadizadeh F, Karlsson T, Ek WE. Investigating the effect of estradiol levels on the risk of breast, endometrial, and ovarian cancer. J Endocr Soc. (2022) 6(8):bvac100. doi: 10.1210/jendso/bvac100
70. Li S, Jiang K, Li J, Hao X, Chu W, Luo C, et al. Estrogen enhances the proliferation and migration of ovarian cancer cells by activating transient receptor potential channel C3. J Ovarian Res. (2020) 13:1–11. doi: 10.1186/s13048-019-0602-5
71. Jeon S-Y, Hwang K-A, Choi K-C. Effect of steroid hormones, estrogen and progesterone, on epithelial mesenchymal transition in ovarian cancer development. J Steroid Biochem Mol Biol. (2016) 158:1–8. doi: 10.1016/j.jsbmb.2016.02.005
72. Langdon SP, Herrington CS, Hollis RL, Gourley C. Estrogen signaling and its potential as a target for therapy in ovarian cancer. Cancers (Basel). (2020) 12(6):1647. doi: 10.3390/cancers12061647
73. Di Zazzo E, Galasso G, Giovannelli P, Di Donato M, Castoria G. Estrogens and their receptors in prostate cancer: therapeutic implications. Front Oncol. (2018) 8:2. doi: 10.3389/fonc.2018.00002
74. Schiffer L, Arlt W, Storbeck K-H. Intracrine androgen biosynthesis, metabolism and action revisited. Mol Cell Endocrinol. (2018) 465:4–26. doi: 10.1016/j.mce.2017.08.016
75. Wang J-M, Li Z-F, Yang W-X. What does androgen receptor signaling pathway in sertoli cells during normal spermatogenesis tell US? Front Endocrinol (Lausanne). (2022) 13:838858. doi: 10.3389/fendo.2022.838858
76. Davey RA, Grossmann M. Androgen receptor structure, function and biology: from bench to bedside. Clin Biochem Rev. (2016) 37(1):3. 4810760.27057074
77. Shihan M, Bulldan A, Scheiner-Bobis G. Non-classical testosterone signaling is mediated by a G-protein-coupled receptor interacting with Gnα11. Biochim Biophys Acta. (2014) 1843(6):1172–81. doi: 10.1016/j.bbamcr.2014.03.002
78. Santiago J, Patrício D, Silva JV. Testicular signaling: team work in sperm production. In: Tissue-specific cell signaling. Cham: Springer International Publishing (2020). p. 225–55. doi: 10.1007/978-3-030-44436-5_8
79. Smith LB. Nonclassical testosterone signaling: a new pathway controlling spermatogenesis? Biol Reprod. (2016) 94(2):43. 1–2. doi: 10.1095/biolreprod.115.137950
80. Anestis A, Zoi I, Papavassiliou AG, Karamouzis MV. Androgen receptor in breast cancer—clinical and preclinical research insights. Molecules. (2020) 25(2):358. doi: 10.3390/molecules25020358
81. Giovannelli P, Di Donato M, Galasso G, Di Zazzo E, Bilancio A, Migliaccio A. The androgen receptor in breast cancer. Front Endocrinol (Lausanne). (2018) 9:492. doi: 10.3389/fendo.2018.00492
82. Smith LB, Walker WH, O’Donnell L. Hormonal regulation of spermatogenesis through Sertoli cells by androgens and estrogens. In: Sertoli cell biology. Amesterdam: Elsevier (2015). p. 175–200. doi: 10.1016/B978-0-12-417047-6.00006-5
83. Gravis G, Boher JM, Joly F, Soulié M, Albiges L, Priou F, et al. Androgen deprivation therapy (ADT) plus docetaxel versus ADT alone in metastatic non castrate prostate cancer: impact of metastatic burden and long-term survival analysis of the randomized phase 3 GETUG-AFU15 trial. Eur Urol. (2016) 70(2):256–62. doi: 10.1016/j.eururo.2015.11.005
84. Maček P, Molinari N, Sobočan M, Knez J. What role do androgens play in endometrial cancer? J Pers Med. (2023) 13(2):341. doi: 10.3390/jpm13020341
85. Kostakis EK, Gkioni LN, Macut D, Mastorakos G. Androgens in menopausal women: not only polycystic ovary syndrome. Hyperandrogenism Women. (2019) 53:135–61. doi: 10.1159/000494909
86. Siegel RL, Miller KD, Jemal A. Cancer statistics, 2018. CA Cancer J Clin. (2018) 68(1):7–30. doi: 10.3322/caac.21442
87. Antonarakis ES. AR Signaling in Human Malignancies: Prostate Cancer and Beyond. Basel, Switzerland: MDPI (2018). p. 22.
88. Hu C, Fang D, Xu H, Wang Q, Xia H. The androgen receptor expression and association with patient’s survival in different cancers. Genomics. (2020) 112(2):1926–40. doi: 10.1016/j.ygeno.2019.11.005
89. Siewe N, Friedman A. Combination therapy for mCRPC with immune checkpoint inhibitors, ADT and vaccine: a mathematical model. PLoS One. (2022) 17(1):e0262453. doi: 10.1371/journal.pone.0262453
90. Medina-Laver Y, Rodríguez-Varela C, Salsano S, Labarta E, Domínguez F. What do we know about classical and non-classical progesterone receptors in the human female reproductive tract? A review. Int J Mol Sci. (2021) 22(20):11278. doi: 10.3390/ijms222011278
91. Islam MS, Afrin S, Jones SI, Segars J. Selective progesterone receptor modulators—mechanisms and therapeutic utility. Endocr Rev. (2020) 41(5):bnaa012. doi: 10.1210/endrev/bnaa012
92. Mani SK, Oyola MG. Progesterone signaling mechanisms in brain and behavior. Front Endocrinol (Lausanne). (2012) 3:7. doi: 10.3389/fendo.2012.00007
93. Pedroza DA, Subramani R, Lakshmanaswamy R. Classical and non-classical progesterone signaling in breast cancers. Cancers (Basel). (2020) 12(9):2440. doi: 10.3390/cancers12092440
94. Garg D, Ng SS, Baig KM, Driggers P, Segars J. Progesterone-mediated non-classical signaling. Trends Endocrinol Metab. (2017) 28(9):656–68. doi: 10.1016/j.tem.2017.05.006
95. Mauvais-Jarvis F, Lange CA, Levin ER. Membrane-initiated estrogen, androgen, and progesterone receptor signaling in health and disease. Endocr Rev. (2022) 43(4):720–42. doi: 10.1210/endrev/bnab041
96. Brisken C, Scabia V. 90 years of progesterone: progesterone receptor signaling in the normal breast and its implications for cancer. J Mol Endocrinol. (2020) 65:81–94. doi: 10.1530/JME-20-0091
97. Horwitz KB, Sartorius CA. Progesterone and progesterone receptors in breast cancer: past, present, future. J Mol Endocrinol. (2020) 65(1):T49. doi: 10.1530/JME-20-0104
98. Wang C, Tran DA, Fu MZ, Chen W, Fu SW, Li X. Estrogen receptor, progesterone receptor, and HER2 receptor markers in endometrial cancer. J Cancer. (2020) 11(7):1693. doi: 10.7150/jca.41943
99. Sasso CV, Santiano FE, Arboccó FC, Zyla LE, Semino SN, Guerrero-Gimenez ME, et al. Estradiol and progesterone regulate proliferation and apoptosis in colon cancer. Endocr Connect. (2019) 8(3):217. doi: 10.1530/EC-18-0374
100. Grindstad T, Richardsen E, Andersen S, Skjefstad K, Rakaee khanehkenari M, Donnem T, et al. Progesterone receptors in prostate cancer: progesterone receptor B is the isoform associated with disease progression. Sci Rep. (2018) 8(1):11358. doi: 10.1038/s41598-018-29520-5
101. Mauro LJ, Spartz A, Austin JR, Lange CA. Re-evaluating the role of progesterone in ovarian cancer: is progesterone always protective? Endocr Rev. (2023) 44:bnad018. doi: 10.1210/endrev/bnad018
102. Sun YS, Zhao Z, Yang ZN, Xu F, Lu HJ, Zhu ZY, et al. Risk factors and preventions of breast cancer. Int J Biol Sci. (2017) 13(11):1387. doi: 10.7150/ijbs.21635
103. Slobodníková J, Meluš V, Krajčovičová Z, Kašlíková K. Endogenous oestrogen as a possible risk factor for breast carcinoma genesis. Acupunct Nat Med. (20171/2) 2017:52.
104. Lax SF. Pathology of endometrial carcinoma. Mol Genet Endometrial Carcinoma. (2017) 943:75–96. doi: 10.1007/978-3-319-43139-0_3
105. Manjani S. Histopathological Study of Endometrial Curettings in Women with Abnormal Uterine Bleeding and Immunohistochemical Study of Estrogen and Progesterone Receptor Expression in Perimenopausal Age Group. Madhuranthagam: Karpaga Vinayaga Institute of Medical Sciences (2016).
106. Pejčić T, Todorović Z, Đurašević S, Popović L. Mechanisms of prostate cancer cells survival and their therapeutic targeting. Int J Mol Sci. (2023) 24(3):2939. doi: 10.3390/ijms24032939
107. Zakari S, Ekenwaneze CC, Amadi EC, and Ogunlana OO. Unlocking the Secrets of Androgen Receptors in the Susceptibility, Progression, and Treatment of Prostate Cancer. (2023).
109. Bulletti C, Bulletti FM, Sciorio R, Guido M. Progesterone: the key factor of the beginning of life. Int J Mol Sci. (2022) 23(22):14138. doi: 10.3390/ijms232214138
110. Diep CH, Daniel AR, Mauro LJ, Knutson TP, Lange CA. Progesterone action in breast, uterine, and ovarian cancers. J Mol Endocrinol. (2015) 54(2):R31. doi: 10.1530/JME-14-0252
111. Trabert B, Sherman ME, Kannan N, Stanczyk FZ. Progesterone and breast cancer. Endocr Rev. (2020) 41(2):320–44. doi: 10.1210/endrev/bnz001
112. Aranha AF, Dos Anjos LG, Turri JA, Simoes RS, Maciel GA, Baracat EC, et al. Impact of the prolactin levels in breast cancer: a systematic review and meta-analysis. Gynecol Endocrinol. (2022) 38(5):385–90. doi: 10.1080/09513590.2022.2047173
113. Li Y, Kong X, Xuan L, Wang Z, Huang YH. Prolactin and endocrine therapy resistance in breast cancer: the next potential hope for breast cancer treatment. J Cell Mol Med. (2021) 25(22):10327–48. doi: 10.1111/jcmm.16946
114. Schuler LA, O’Leary KA. Prolactin: the third hormone in breast cancer. Front Endocrinol (Lausanne). (2022) 13:910978. doi: 10.3389/fendo.2022.910978
115. Wang M, Wu X, Chai F, Zhang Y, Jiang J. Plasma prolactin and breast cancer risk: a meta-analysis. Sci Rep. (2016) 6(1):25998. doi: 10.1038/srep25998
116. Cheng L, Albers P, Berney DM, Feldman DR, Daugaard G, Gilligan T, et al. Testicular cancer. Nat Rev Dis Primers. (2018) 4(1):29. doi: 10.1038/s41572-018-0029-0
117. Bandak M, Jørgensen N, Juul A, Lauritsen J, Kier MG, Mortensen MS, et al. Longitudinal changes in serum levels of testosterone and luteinizing hormone in testicular cancer patients after orchiectomy alone or bleomycin, etoposide, and cisplatin. Eur Urol Focus. (2018) 4(4):591–8. doi: 10.1016/j.euf.2016.11.018
118. Perales-Puchalt A, Wojtak K, Duperret EK, Yang X, Slager AM, Yan J, et al. Follicle-stimulating hormone receptor is expressed by most ovarian cancer subtypes and is a safe and effective immunotherapeutic target. Clin Cancer Res. (2017) 23(2):441–53. doi: 10.1158/1078-0432.CCR-16-0492
119. Perales-Puchalt A, Wojtak K, Duperret EK, Yang X, Slager AM, Yan J, et al. Engineered DNA vaccination against follicle-stimulating hormone receptor delays ovarian cancer progression in animal models. Mol Ther. (2019) 27(2):314–25. doi: 10.1016/j.ymthe.2018.11.014
120. Bi WK, Luan SS, Wang J, Wu SS, Jin XC, Fu YL, et al. FSH Signaling is involved in affective disorders. Biochem Biophys Res Commun. (2020) 525(4):915–20. doi: 10.1016/j.bbrc.2020.03.039
121. Miller M, Schoenfield L, Abdel-Rahman M, Cebulla CM. Is uveal melanoma a hormonally sensitive cancer? A review of the impact of sex hormones and pregnancy on uveal melanoma. Ocul Oncol Pathol. (2021) 7(4):239–50. doi: 10.1159/000514650
122. Abancens M, Bustos V, Harvey H, McBryan J, Harvey BJ. Sexual dimorphism in colon cancer. Front Oncol. (2020) 10:607909. doi: 10.3389/fonc.2020.607909
123. Li S, Chen Y, Xie L, Meng Y, Zhu L, Chu H, et al. Sex hormones and genetic variants in hormone metabolic pathways associated with the risk of colorectal cancer. Environ Int. (2020) 137:105543. doi: 10.1016/j.envint.2020.105543
124. Svoronos N, Perales-Puchalt A, Allegrezza MJ, Rutkowski MR, Payne KK, Tesone AJ, et al. Tumor cell–independent estrogen signaling drives disease progression through mobilization of myeloid-derived suppressor cells. Cancer Discov. (2017) 7(1):72–85. doi: 10.1158/2159-8290.CD-16-0502
125. Choi E, Buie J, Camacho J, Sharma P, de Riese WT. Evolution of androgen deprivation therapy (ADT) and its new emerging modalities in prostate cancer: an update for practicing urologists, clinicians and medical providers. Res Rep Urol. (2022) 14:87–108. doi: 10.2147/RRU.S303215
126. Shore R, Zhang J, Ye W, Stattin P, Lindblad M. Risk of colorectal adenocarcinoma in men receiving androgen deprivation therapy for prostate cancer; a nationwide cohort study. Cancer Causes Control. (2023) 34:1–13. doi: 10.1007/s10552-023-01736-5
127. Moyer AM, Matey ET, Miller VM. Individualized medicine: sex, hormones, genetics, and adverse drug reactions. Pharmacol Res Perspect. (2019) 7(6):e00541. doi: 10.1002/prp2.541
128. Abuteen A, Zanganeh S, Akhigbe J, Samankumara LP, Aguirre A, Biswal N, et al. The evaluation of NIR-absorbing porphyrin derivatives as contrast agents in photoacoustic imaging. Phys Chem Chem Phys. (2013) 15(42):18502–9. doi: 10.1039/c3cp52193a
129. Alqasemi U, Li H, Yuan G, Kumavor P, Zanganeh S, Zhu Q. Interlaced photoacoustic and ultrasound imaging system with real-time coregistration for ovarian tissue characterization. J Biomed Opt. (2014) 19(7):76020. doi: 10.1117/1.JBO.19.7.076020
130. Arabi L, Ho JQ, Javdani N, Sharaf M, Lam M, Aieneravaie M, et al. Nanoparticulate Systems for Delivery of Biomolecules and Cells to the Injured Myocardium, in Nanomedicine for Ischemic Cardiomyopathy. Amsterdam, Netherlands: Elsevier (2020). p. 143–56.
131. Assali A, Akhavan O, Adeli M, Razzazan S, Dinarvand R, Zanganeh S, et al. Multifunctional core-shell nanoplatforms (gold@graphene oxide) with mediated NIR thermal therapy to promote miRNA delivery. Nanomedicine. (2018) 14(6):1891–903. doi: 10.1016/j.nano.2018.05.016
132. Atashgah RB, Ghasemi A, Raoufi M, Abdollahifar MA, Zanganeh S, Nejadnik H, et al. Restoring endogenous repair mechanisms to heal chronic wounds with a multifunctional wound dressing. Mol Pharm. (2021) 18(8):3171–80. doi: 10.1021/acs.molpharmaceut.1c00400
133. Bigdelou B, Sepand MR, Najafikhoshnoo S, Negrete JA, Sharaf M, Ho JQ, et al. COVID-19 and preexisting comorbidities: risks, synergies, and clinical outcomes. Front Immunol. (2022) 13:890517. doi: 10.3389/fimmu.2022.890517
134. Biswal NC, Pavlik C, Smith MB, Aguirre A, Xu Y, Zanganeh S, et al. Imaging tumor hypoxia by near-infrared fluorescence tomography. J Biomed Opt. (2011) 16(6):066009. doi: 10.1117/1.3589348
135. Bradbury MS, Zanganeh SS, Madajewski B, Campesato LF, Marghoub T, Overholtzer M, et al. Inducing Favorable Effects on Tumor Microenvironment via Administration of Nanoparticle Compositions. Google Patents. (2022).
136. Farvadi F, Ghahremani MH, Hashemi F, Hormozi-Nezhad MR, Raoufi M, Zanganeh S, et al. Cell shape affects nanoparticle uptake and toxicity: an overlooked factor at the nanobio interfaces. J Colloid Interface Sci. (2018) 531:245–52. doi: 10.1016/j.jcis.2018.07.013
137. Hajipour MJ, Mehrani M, Abbasi SH, Amin A, Kassaian SE, Garbern JC, et al. Nanoscale technologies for prevention and treatment of heart failure: challenges and opportunities. Chem Rev. (2019) 119(21):11352–90. doi: 10.1021/acs.chemrev.8b00323
138. Javdani N, Ho JQ, Arabi L, Le A, Ghegeliu D, Aieneravaie M, et al. Nanoparticulate Systems for Monitoring of Therapeutic Cells, in Nanomedicine for Ischemic Cardiomyopathy. Amsterdam, Netherlands: Elsevier (2020). p. 113–23.
139. Kumavor PD, Aguirre A, Xu C, Gamelin J, Ardeshirpour Y, Tavakoli B, et al. Target detection and quantification using a hybrid hand-held diffuse optical tomography and photoacoustic tomography system. J Biomed Opt. (2011) 16(4):046010. doi: 10.1117/1.3563534
140. Martins TA, Schmassmann P, Shekarian T, Boulay JL, Ritz MF, Zanganeh S, et al. Microglia-Centered combinatorial strategies against glioblastoma. Front Immunol. (2020) 11:571951. doi: 10.3389/fimmu.2020.571951
141. Nejadnik H, Taghavi-Garmestani SM, Madsen SJ, Li K, Zanganeh S, Yang P, et al. The protein Corona around nanoparticles facilitates stem cell labeling for clinical MR imaging. Radiology. (2018) 286(3):938–47. doi: 10.1148/radiol.2017170130
142. Pauliah M, Zanganeh S, Erfanzadeh M, Ho J. Tumor-targeted Therapy Iron Oxide Nanoparticles for Biomedical Applications. Amsterdam, Netherlands: Elsevier (2018).
143. Raoufi E, Hosseini F, Onagh B, Salehi-Shadkami M, Mehrali M, Mohsenzadegan M, et al. Designing and developing a sensitive and specific SARS-CoV-2 RBD IgG detection kit for identifying positive human samples. Clin Chim Acta. (2023) 542:117279. doi: 10.1016/j.cca.2023.117279
144. Sepand MR, Aghsami M, Keshvadi MH, Bigdelou B, Behzad R, Zanganeh S, et al. The role of macrophage polarization and function in environmental toxicant-induced cancers. Environ Res. (2021) 196:110933. doi: 10.1016/j.envres.2021.110933
145. Sepand MR, Bigdelou B, Ho JQ, Sharaf M, Lannigan AJ, Sullivan IM, et al. Long-term immunity and antibody response: challenges for developing efficient COVID-19 vaccines. Antibodies (Basel). (2022) 11(2):35. doi: 10.3390/antib11020035
146. Sepand MR, Ghavami M, Zanganeh S, Stacks S, Ghasemi F, Montazeri H, et al. Impact of plasma concentration of transferrin on targeting capacity of nanoparticles. Nanoscale. (2020) 12(8):4935–44. doi: 10.1039/C9NR08784B
147. Spitler R, Zanganeh S, Jafari T, Khakpash N, Erfanzadeh M, Ho JQ, et al. Drug delivery systems: possibilities and challenges. Drug Deliv. Syst. (2017):1–52. ISBN: 978913201040.
148. Tavakol M, Hajipour MJ, Ferdousi M, Zanganeh S, Maurizi L. Competition of opsonins and dysopsonins on the nanoparticle surface. Nanoscale. (2023) 15(43):17342–9. doi: 10.1039/D3NR03823H
149. Tavakol M, Montazeri A, Naghdabadi R, Hajipour MJ, Zanganeh S, Caracciolo G, et al. Disease-related metabolites affect protein-nanoparticle interactions. Nanoscale. (2018) 10(15):7108–15. doi: 10.1039/C7NR09502C
150. Tavares-Negrete JA, Das P, Najafikhoshnoo S, Zanganeh S, Esfandyarpour R. Recent advances in lung-on-a-chip technology for modeling respiratory disease. Bio-Design and Manufacturing. (2023) 6:1–23. doi: 10.1007/s42242-023-00241-7
151. Xu C, Kumavor PD, Alqasemi U, Li H, Xu Y, Zanganeh S, et al. Indocyanine green enhanced co-registered diffuse optical tomography and photoacoustic tomography. J Biomed Opt. (2013) 18(12):126006. doi: 10.1117/1.JBO.18.12.126006
152. Xu Y, Zanganeh S, Mohammad I, Aguirre A, Wang T, Yang Y, et al. Targeting tumor hypoxia with 2-nitroimidazole-indocyanine green dye conjugates. J Biomed Opt. (2013) 18(6):66009. doi: 10.1117/1.JBO.18.6.066009
153. Zanganeh S, Aieneravaie M, Erfanzadeh M, Ho JQ, Spitler R. Magnetic Particle Imaging (MPI), in Iron Oxide Nanoparticles for Biomedical Applications. Amsterdam, Netherlands: Elsevier (2018). p. 115–33.
154. Zanganeh S, Georgala P, Corbo C, Arabi L, Ho JQ, Javdani N, et al. Immunoengineering in glioblastoma imaging and therapy. Wiley Interdiscip Rev Nanomed Nanobiotechnol. (2019) 11(6):e1575. doi: 10.1002/wnan.1575
155. Zanganeh S, Ho JQ, Aieneravaie M, Erfanzadeh M, Pauliah M, Spitler R. Drug Delivery, in Iron Oxide Nanoparticles for Biomedical Applications. Amsterdam, Netherlands: Elsevier (2018). p. 247–71.
156. Zanganeh S, Ho JQ, Aieneravaie M, Erfanzadeh M, Spitler R. Protein Corona: the Challenge at the Nanobiointerfaces, in Iron Oxide Nanoparticles for Biomedical Applications. Amsterdam, Netherlands: Elsevier (2018). p. 91–104.
157. Zanganeh S, Hutter G, Spitler R, Lenkov O, Mahmoudi M, Shaw A, et al. Iron oxide nanoparticles inhibit tumour growth by inducing pro-inflammatory macrophage polarization in tumour tissues. Nat Nanotechnol. (2016) 11(11):986–94. doi: 10.1038/nnano.2016.168
158. Zanganeh S, Jafari T, Khakpash N, Erfanzadeh M, Ho JQ. Nanoparticles in circulation: blood stability. Drug Delivery Systems. (2017) 1:53. doi: 10.1142/9789813201057_0002
159. Zanganeh S, Li H, Kumavor PD, Alqasemi U, Aguirre A, Mohammad I, et al. Photoacoustic imaging enhanced by indocyanine green-conjugated single-wall carbon nanotubes. J Biomed Opt. (2013) 18(9):096006. doi: 10.1117/1.JBO.18.9.096006
160. Zanganeh S, Spitler R, Erfanzadeh M, Alkilany AM, Mahmoudi M. Protein corona: opportunities and challenges. Int J Biochem Cell Biol. (2016) 75:143–7. doi: 10.1016/j.biocel.2016.01.005
161. Zanganeh S, Spitler R, Javdani N, Ho JQ. Nanocytotoxicity, in Iron Oxide Nanoparticles for Biomedical Applications. Amsterdam, Netherlands: Elsevier (2018). p. 105–14.
162. Zanganeh S, Spitler R, Javdani N, Ho JQ. How do Nanoparticles (NPs) Pass Barriers. Drug Delivery Systems. Singapore: World Scientific (2017). p. 89–111.
163. Zanganeh S, Xu Y, Hamby CV, Backer MV, Backer JM, Zhu Q. Enhanced fluorescence diffuse optical tomography with indocyanine green-encapsulating liposomes targeted to receptors for vascular endothelial growth factor in tumor vasculature. J Biomed Opt. (2013) 18(12):126014. doi: 10.1117/1.JBO.18.12.126014
164. Zhou F, Zanganeh S, Mohammad I, Dietz C, Abuteen A, Smith MB, et al. Targeting tumor hypoxia: a third generation 2-nitroimidazole-indocyanine dye-conjugate with improved fluorescent yield. Org Biomol Chem. (2015) 13(46):11220–7. doi: 10.1039/C5OB01460C
165. Rangsrikitphoti P, Marquez-Garban DC, Pietras RJ, McGowan E, Boonyaratanakornkit V. Sex steroid hormones and DNA repair regulation: implications on cancer treatment responses. J Steroid Biochem Mol Biol. (2022) 227:106230. doi: 10.1016/j.jsbmb.2022.106230
166. Generali D, Bates G, Berruti A, Brizzi MP, Campo L, Bonardi S, et al. Immunomodulation of FOXP3+ regulatory T cells by the aromatase inhibitor letrozole in breast cancer patients. Clin Cancer Res. (2009) 15(3):1046–51. doi: 10.1158/1078-0432.CCR-08-1507
167. Siemens DR, Klotz L, Heidenreich A, Chowdhury S, Villers A, Baron B, et al. Efficacy and safety of enzalutamide vs bicalutamide in younger and older patients with metastatic castration resistant prostate cancer in the TERRAIN trial. J Urol. (2018) 199(1):147–54. doi: 10.1016/j.juro.2017.08.080
168. Lee M, Piao J, Jeon MJ. Risk factors associated with endometrial pathology in premenopausal breast cancer patients treated with tamoxifen. Yonsei Med J. (2020) 61(4):317. doi: 10.3349/ymj.2020.61.4.317
169. Raberi VS, Shariati A, Abbasnezhad M, Aslanabadi N, Abbasnezhad A, Bahmani A. Drug resistance and cardiovascular safety of second-generation anti-androgens in patients with advanced prostate cancer. Galen Medical Journal. (2022) 11:e2727–e2727. doi: 10.31661/gmj.v11i.2727
170. Irelli A, Sirufo MM, D'Ugo C, Ginaldi L, De Martinis M. Sex and gender influences on cancer immunotherapy response. Biomedicines. (2020) 8(7):232. doi: 10.3390/biomedicines8070232
171. Conforti F, Pala L, Pagan E, Corti C, Bagnardi V, Queirolo P, et al. Sex-based differences in response to anti-PD-1 or PD-L1 treatment in patients with non-small-cell lung cancer expressing high PD-L1 levels. A systematic review and meta-analysis of randomized clinical trials. ESMO Open. (2021) 6(5):100251. doi: 10.1016/j.esmoop.2021.100251
172. Conforti F, Pala L, Bagnardi V, Viale G, De Pas T, Pagan E, et al. Sex-based heterogeneity in response to lung cancer immunotherapy: a systematic review and meta-analysis. J Nat Cancer Inst. (2019) 111(8):772–81. doi: 10.1093/jnci/djz094
173. Rothenberger NJ, Somasundaram A, Stabile LP. The role of the estrogen pathway in the tumor microenvironment. Int J Mol Sci. (2018) 19(2):611. doi: 10.3390/ijms19020611
174. Langer R, Hodis H, Lobo R, Allison M. Hormone replacement therapy–where are we now? Climacteric. (2021) 24(1):3–10. doi: 10.1080/13697137.2020.1851183
175. Cagnacci A, Venier M. The controversial history of hormone replacement therapy. Medicina (B Aires). (2019) 55(9):602. doi: 10.3390/medicina55090602
Keywords: sex hormone, sex-specific oncology, cancer therapy, personalized medicine, sex-specific medicine
Citation: Bakhshi P, Ho JQ and Zanganeh S (2024) Sex-specific outcomes in cancer therapy: the central role of hormones. Front. Med. Technol. 6:1320690. doi: 10.3389/fmedt.2024.1320690
Received: 12 October 2023; Accepted: 8 January 2024;
Published: 1 February 2024.
Edited by:
Ghanbar Mahmoodi Chalbatani, Mayo Clinic Arizona, United StatesReviewed by:
Seyedvahid Hosseini, Tabriz University of Medical Sciences, Iran© 2024 Bakhshi, Ho and Zanganeh. This is an open-access article distributed under the terms of the Creative Commons Attribution License (CC BY). The use, distribution or reproduction in other forums is permitted, provided the original author(s) and the copyright owner(s) are credited and that the original publication in this journal is cited, in accordance with accepted academic practice. No use, distribution or reproduction is permitted which does not comply with these terms.
*Correspondence: Steven Zanganeh c3RldmVuLnphbmdhbmVoQHVjb25uLmVkdQ==
Disclaimer: All claims expressed in this article are solely those of the authors and do not necessarily represent those of their affiliated organizations, or those of the publisher, the editors and the reviewers. Any product that may be evaluated in this article or claim that may be made by its manufacturer is not guaranteed or endorsed by the publisher.
Research integrity at Frontiers
Learn more about the work of our research integrity team to safeguard the quality of each article we publish.