- Department of Pharmacy and Biotechnology, University of Bologna, Bologna, Italy
The spread of biofilm-related diseases in developed countries has led to increased mortality rates and high health care costs. A biofilm is a community of microorganisms that is irreversibly attached to a surface, behaving very differently from planktonic cells and providing resistance to antimicrobials and immune response. Oral diseases are an excellent example of infection associated with the formation of highly pathogenic biofilms. It is generally accepted that, when the oral homeostasis is broken, the overgrowth of pathogens is facilitated. Among them, Porphyromonas gingivalis and Aggregatibacter actinomycetemcomitans are the main etiological agents of periodontitis, while Streptococcus mutans is strongly associated with the onset of dental caries. Other microorganisms, such as the fungus Candida albicans, may also be present and contribute to the severity of infections. Since the common antibiotic therapies usually fail to completely eradicate biofilm-related oral diseases, alternative approaches are highly required. In this regard, the topical administration of probiotics has recently gained interest in treating oral diseases. Thus, the present mini-review focuses on the possibility of using Lactobacillus spp. as probiotics to counteract biofilm-mediated oral infections. Many evidence highlight that Lactobacillus living cells can impede the biofilm formation and eradicate mature biofilms of different oral pathogens, by acting through different mechanisms. Even more interestingly, lactobacilli derivatives, namely postbiotics (soluble secreted products) and paraprobiotics (cell structural components) are able to trigger anti-biofilm effects too, suggesting that they can represent a novel and safer alternative to the use of viable cells in the management of biofilm-related oral diseases.
Introduction
Among virulence factors for pathogens, biofilms are associated with a broad array of topical infections, including periodontal diseases, chronic wounds, and vaginosis (1). The burden of biofilm-related disease contributes to patient morbidity and increased mortality rates, thus representing a major public health issue in developed countries, which adds over $1 billion to US hospitalization costs annually (2, 3). Biofilm is a structured community of microorganisms that irreversibly adhere to an abiotic surface or biological tissue and represents a phenotypically distinct state from the planktonic counterpart, particularly in terms of growth rate and gene expression (1, 4).
Biofilms are morphologically distinguishable by the presence of a self-produced extracellular polymeric matrix that encloses microbial cells, offering great protection against chemical disinfectants, antimicrobials, and human immune response. The host immune system is not only ineffective in fully eradicating mature biofilm, but the presence of antibodies in the surrounding environment can contribute to tissue damage (5). Moreover, sessile microbial biofilm cells are up to 1,000-fold more resistant to antibiotics than planktonic cells, mainly due to the difficulty of high-molecular-weight antibiotics to penetrate the viscous matrix and to reach the deeper layers of biofilm (6, 7). The antimicrobial tolerance can be also ascribed to the presence in the biofilm core of a subpopulation of persistent cells, which in virtue of their dormant nonproliferative phenotype are not susceptible to mechanisms of action of common antibiotics (6, 8). Other factors, such as the slow growth rate, the presence of multidrug efflux pumps, and stress response regulation, and the peculiar biofilm microenvironment characterized by differences in pH, pCO2, and pO2, contribute to reducing the susceptibility of biofilm to antimicrobials (9). Furthermore, the higher cell density in biofilms favors the horizontal gene transfer, which in turn, increases the probability of emergence of strains with resistance or altered virulence profiles (10, 11). Although antimicrobial treatment can alleviate the symptoms of infection by killing the free-floating microorganisms disseminated from the adherent population (12), it usually fails to completely eradicate pathogens in the biofilm. As a result, when antibiotic therapy stops, the persistent cells can revert to their phenotype (13), causing a relapse of the condition and potentially leading to recurrent or chronic infections (5). Given the importance of clearance of mature biofilm for the successful resolution of such infections, alternative strategies to the use of antibiotics are highly desirable. These may include the use of plant-derived compounds, antimicrobial peptides from various sources and probiotics (14). Traditionally, probiotic microorganisms, mainly lactic acid bacteria (LAB) and bifidobacteria, have been orally administered to treat or prevent several gastrointestinal disorders, including diarrhea, gastritis, Crohn's disease, colitis, allergies, food intolerances, and obesity (15). In the last years, there has been an increased interest in the topical administration of probiotic cells to overcome the biofilm-related problem of antibiotic resistance. Probiotics are defined as “Live microorganisms which, when administered in adequate amounts, confer a health benefit to the host” (16). Among LAB bacteria, Lactobacillus is the largest genus and comprises the most widely used probiotic species (i.e., L. rhamnosus, L. acidophilus, L. casei, L. plantarum, L. reuteri, L. delbrueckii). Lactobacilli are gram-positive, nonsporing, nonrespiring rods, and they are considered as Generally Recognized As Safe (GRAS) (17). In the present mini-review, we explored the possibility of using lactobacilli, intended both as living cells and as derivatives, in the treatment of biofilm-mediated oral infections.
Biofilm-Mediated Oral Diseases
Oral diseases (i.e., periodontitis, gingivitis, and dental caries) are an excellent example of infection associated with the formation of a highly pathogenic biofilm (18, 19). Periodontitis is one of the most prevalent diseases worldwide and is caused by gram-negative bacteria, such as Porphyromonas gingivalis and Treponema denticola (20), able to form polymicrobial dental plaque biofilms and to produce virulence factors responsible for an exacerbated inflammatory response, which leads to the destruction of the supporting periodontal tissue and eventually teeth loss (19). Severe forms of periodontitis are also associated with the colonization of subgingival sites by Aggregatibacter actinomycetemcomitans and the concomitant reduction of commensal bacteria (21).
The treatment of periodontitis includes hygiene practices, mechanical debridement, and eventually the use of antimicrobials. However, these interventions are not enough to achieve long-term stability, especially in more severe cases, and regular maintenance care based on biofilm control is essential to preserve the equilibrium of the oral microbiome (22). This aspect is also pivotal in the management of dental caries. Members of the Streptococcus genus, such as S. gordonii, S. oralis, S. sanguinis, and S. salivarius, promote the initial colonization on dental pellicle and thus allow for subsequent binding of S. mutans (23). S. mutans is the main etiological agent of dental caries owing to its ability to produce exopolysaccharides (EPS) such as glucan and to rapidly form a mature biofilm on the tooth surface (22). Besides bacteria, the fungal pathogen C. albicans can be present in mixed-species biofilms in dental plaque together with S. mutans (24) and is a frequent cause of stomatitis in children, elderly, and immunocompromised patients (25). In oral candidiasis, the switch of Candida from budding yeast to filamentous hyphae allows for covalent attachment to the oral mucosal surface, followed by biofilm formation, invasion, and tissue damage.
Lactobacilli: Probiotics to Counteract Oral Pathogenic Biofilms
The use of probiotics to face up to biofilm-mediated oral diseases has been studied during the last 20 years (26). Examples of lactobacilli exerting anti-biofilm activity against pathogens causing oral diseases are reported in Table 1. The oral cavity is densely colonized by approximately 1,000 bacterial species. The current understanding is that the overgrowth of cariogenic microorganisms in dental caries is driven by a shift in the oral homeostasis toward less diversity (37), favored by acidic production from sugars that create optimal microenvironmental conditions to support the proliferation and biofilm formation of acid-tolerant S. mutans. Although in the oral cavity, certain strains of lactobacilli can be cariogenic due to their acidogenic and aciduric features, several studies have reported the therapeutic potential of other beneficial Lactobacillus strains (38–40). It has been proposed that lactobacilli may hamper oral pathogens in different ways, even if the exact mechanisms are still poorly understood and could vary among different strains (41). However, lactobacilli can interfere with harmful bacteria and fungi through competition for nutrients, co-aggregation, production of antimicrobials (i.e., bacteriocin, hydrogen peroxide, and organic acids), and modulation of the immune system (42). Moreover, there are few studies showing that lactobacilli are able to integrate into the target biofilm and transiently colonize the oral cavity, thus, competing with pathogens for adhesion sites (43). As an example, Romani et al. demonstrated that L. reuteri PTA5289 can be temporarily incorporated in oral microbiota during a 6-weeks intervention, causing a delay in the regrowth of S. mutants after full-mouth disinfection with chlorhexidine (44). Some authors reported that commercially available Lactobacillus strains (L. rhamnosus GG, L. plantarum 299v, L. reuteri PTA5289 and SD2112, L. paracasei DSM16671) reduced biofilm formation of S. mutans clinical isolates on both smooth glass surface (27) and saliva-coated hydroxyapatite (28). The persistent presence of a Lactobacillus strain potentiates its interference with pathogens. For this reason, Wu et al. evaluated the anti-biofilm activity of Lactobacillus salivarius, a species frequently found in human saliva and tooth surface of healthy subjects (45). They observed that the co-cultivation of S. mutans with a panel of 64 strains of L. salivarius resulted in a reduction of biofilm formation up to 69%, which was higher than that obtained with the reference strain L. rhamnosus GG. Moreover, L. salivarius reduced the expression of genes (gtfB, gtfC, and gtfD) encoding for three glucosyltransferase (Gtfs) involved in the synthesis of exopolysaccharide matrix, and consequently crucial for S. mutans biofilm formation (29). Similarly, Lee at al. demonstrated that L. rhamnosus GG exerted an anti-biofilm activity by decreasing the expression of gtfs in S. mutans but it was not able to integrate into the oral biofilm model. Contrariwise, L. acidophilus ATCC4356 and L. reuteri ATCC334 integrated into S. mutants biofilm without affecting glucan production (30). S. mutans produces proteins anchored in the cell walls to facilitate binding to C. albicans, which in turn, supports streptococcal colonization and caries progression of the formed biofilm (46, 47). Krzyściak et al. showed that L. salivarius (HM6 Paradens) not only reduced the biomass of mono-species biofilms of S. mutans and C. albicans clinical strains, but also the multispecies biofilm. Interestingly, SEM images revealed that the addiction to viable probiotics inhibited the formation of hyphae and germ tubes in C. albicans, consequently hindering the fungal pathogenesis (32). Other authors supported this observation. In particular, three Lactobacillus strains isolated from the saliva of caries-free subjects were able to reduce biofilm development when co-incubated with two oral strains of C. albicans (35). The anti-biofilm activity seemed to be related to the downregulation of Candida biofilm-specific genes, including HWP1 (a glycoprotein located in the hyphal surface), ALS3 (a protein similar to alpha-agglutinin that is essential for the adhesion of Candida), and CPH1 (a regulator of morphogenesis implicated in the maintenance of cell wall organization, pseudohyphal formation in response to oxidative stress, and biofilm development) (39, 40). Viela et al. demonstrated that L. acidophilus ATCC4356, a strain widely employed as food supplements, inhibited the formation of C. albicans ATCC18804 biofilm (57%) and filamentation in vitro, with the best results achieved after 24 h of incubation; the reduction in the number of fungal hyphae produced after probiotic treatment was also observed in Galleria mellonella infection model (48). James et al. have found that a multistrain probiotic combination (Lactobacillus helveticus CBS N116411, L. plantarum SD57870, S. salivarius DSM14685) was effective at both preventing the formation of (>67%) and removing preformed (>63%) C. albicans biofilms (36). Moreover, the combination of two lactobacilli supernatant significantly reduced the expression of several C. albicans genes involved in the yeast–hyphae transition, such as ALS3, EFG1 (hyphae-specific gene activator), SAP5 (secreted protease), and HWP1, in agreement with the results of Rossoni et al. (35).
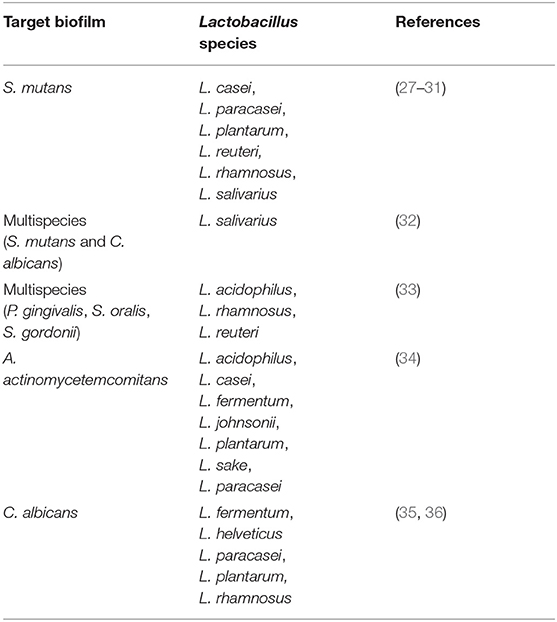
Table 1. Probiotic Lactobacillus spp. exhibiting in vitro anti-biofilm activity against pathogens involved in oral biofilm-related diseases.
Regarding periodontal disease therapy, in a study carried out by Jaffar et al., different species of lactobacilli were sought for their ability to eradicate preformed biofilm of three strains of A. actinomycetemcomitans, with promising results. In particular, biofilms of A. actinomycetemcomitans Y4 (serotype b) and A. actinomycetemcomitans OMZ 534 (serotype e) were effectively dispersed after the addiction of lactobacilli, with reduction rates up to 90% and, interestingly, the authors identified three probiotic enzymes, namely protease, lipase, and amylase, that may be responsible for the anti-biofilm activity (34).
Beyond Probiotics: Lactobacillus Derivatives
Beneficial outcomes of lactobacilli can be obtained not only by living cells, but their byproducts (cell components or metabolites) may be also able to trigger probiotic effects (49). The terms “postbiotics” and “paraprobiotics” have emerged recently and have gained increasing interest since they can exert a wide range of positive effects on the host, such as immunomodulatory, antitumor, and antimicrobial activities, as well as preservation of intestinal barrier (50). Postbiotics are defined as soluble products or metabolites secreted by probiotics capable of providing physiological benefits through direct or indirect mechanisms. They include metabolic byproducts of live probiotic cells, such as cell-free supernatant (CFS), secreted proteins, bacteriocins, organic acids, and secreted biosurfactants (BS) (51). The term paraprobiotics is used to indicate nonviable probiotics (inactivated or dead intact cells), or their cell structural components that can be recovered after cell rupture. The latter comprise peptidoglycans, teichoic acid, cell wall polysaccharides, surface proteins, and cell wall-bound BS (52). Concerns about the administration of living probiotics have been described in experimental models, clinical trials, and case reports (50, 53). Postbiotics/paraprobiotics display prolonged shelf life and good stability (54) and, in this regard, can be potentially employed in oral therapy as a safer alternative to the use of viable cells. Lactobacilli postbiotics/paraprobiotics with anti-biofilm properties toward oral pathogens are listed in Table 2 and briefly described in the following sections.
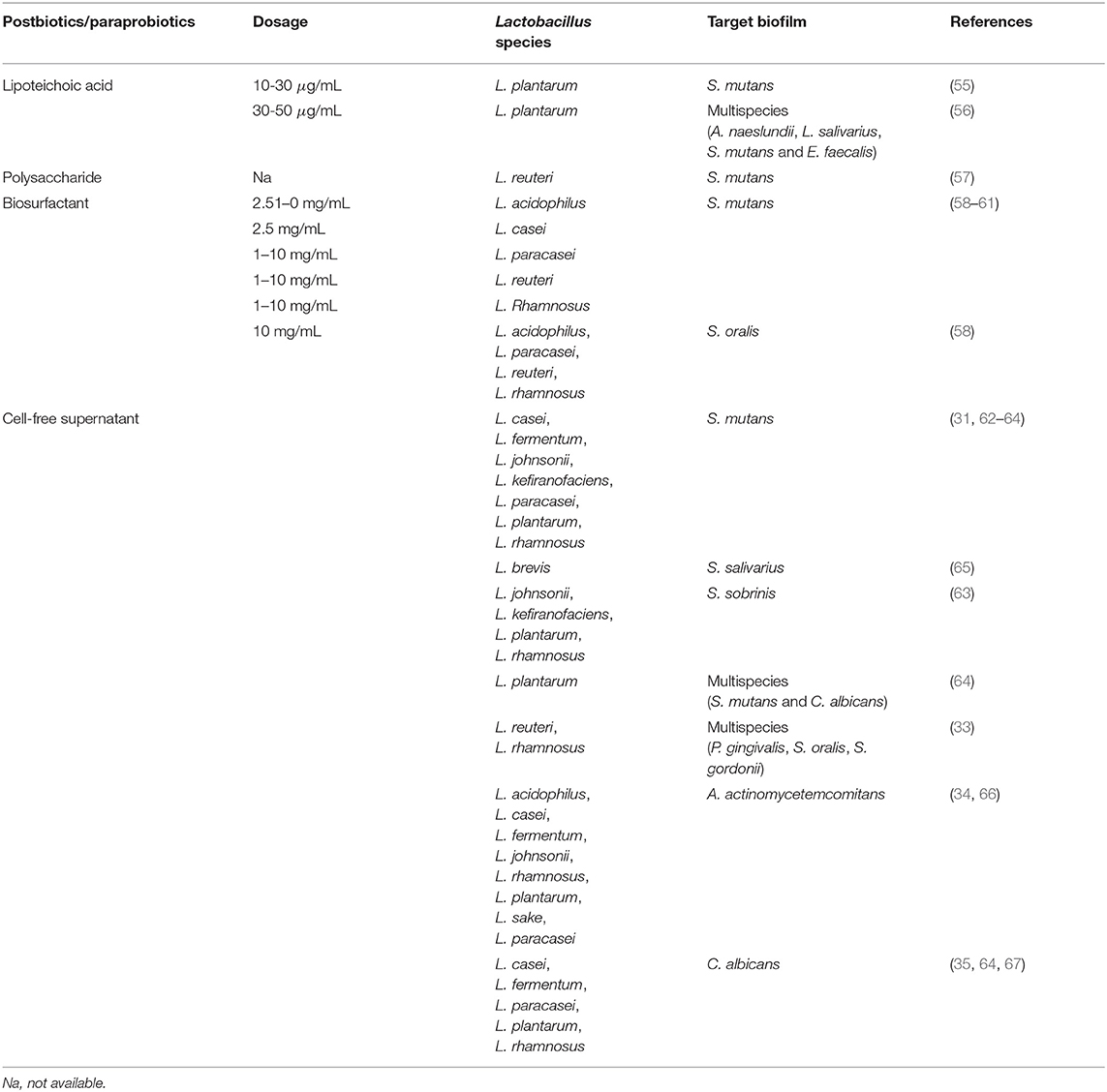
Table 2. Postbiotics/paraprobiotics from Lactobacillus spp. exhibiting in vitro anti-biofilm activity against pathogens involved in oral biofilm-related diseases.
Lipoteichoic Acid
Teichoic acids are the second main constituent of cell walls of lactobacilli and can be covalently linked to peptidoglycan as wall teichoic acid or anchored to the cytoplasmic membrane as lipoteichoic acid (LTA) (68). L. plantarum LTA is known to possess anti-biofilm activity against Staphylococcus aureus (69) and Enterococcus faecalis (70). Ahn et al. demonstrated that it was also able to prevent the biofilm formation of S. mutans on polystyrene plates, hydroxyapatite disk, and dentine slices without affecting streptococcal growth, but interfering with sucrose decomposition essential for the production of EPS (55). Furthermore, L. plantarum LTA disrupted preformed oral multispecies biofilm (Actinomyces naeslundii ATCC12104, L. salivarius ATCC11741, S. mutans KCTC3065, and E. faecalis ATCC29212) in a dose-dependent manner and potentiated the effectiveness of common intracanal medicaments (i.e., calcium hydroxide and chlorhexidine digluconate) (56).
Polysaccharides
The most studied polysaccharides are EPS. Since their production is highly strain-specific and depends on several variables (i.e., medium, pH, age of culture), EPS greatly varies in the degree of branching and monosaccharide composition. EPS mediates the interactions between bacteria and the environment and protects against hostile conditions and immune response. On the other hand, Lactobacillus-derived EPS revealed antimicrobial and anti-biofilm activities toward a broad range of pathogens, including E. faecalis, S. aureus, Escherichia coli, and Pseudomonas aeruginosa (71). Moreover, L. reuteri BM53-1 was found to produce a short extracellular polysaccharide with a molecular weight of about 30 kDa that impeded the production of sticky β-glucans by S. mutans and consequently the biofilm formation. In particular, the authors suggested that it acts by lowering the expression of gtfD that is necessary to give sickness to insoluble glucans produced in large amounts during the initial attachment of S. mutans on the surface (57).
Biosurfactants
Biosurfactants are secondary metabolites consisting of complex polymeric mixtures (i.e., glycolipids, lipopeptides) that can be secreted extracellularly or bounded to the cell wall. In virtue of their amphiphilic nature, BS exhibits emulsification properties and may assist in the dispersal of preformed biofilms or preventing the onset of pathogenic biofilms (52). Some studies highlighted that BS may be used as an alternative to antibiotics to decrease the chance of dental caries by acting as anti-biofilm agents toward S. mutans. For instance, the anti-adhesive activity of L. paracasei BS against several bacteria, including S. mutans and S. oralis, was reported by Gudiña et al. (72). Furthermore, excreted BS purified from cultures of L. reuteri DSM17938, L. acidophilus DDS-1, L. rhamnosus ATCC53103, and L. paracasei B21060 reduced the adhesion and biofilm formation on the titanium surface of S. mutans and S. oralis in a dose-dependent manner (58). Evidence shows that the anti-biofilm behavior of both excreted and cell-bound Lactobacillus BS can be ascribed to the downregulation of Gtfs genes, mainly gtfB and gtfC, as previously described for living probiotic cells (59–61). In addition, the expression of ftf , a proadhesive gene encoding for S. mutans fructosyltransferase, resulted significantly reduced in the presence of BS produced by L. acidophilus DSM20079 (59), L. casei ATCC39392 (60), and L. rhamnosus ATCC7469 (61).
Cell-Free Supernatant
Cell-free supernatant of lactobacilli is a consortium of low (i.e., organic acids, hydrogen peroxide, and reuterin) and high molecular weight (i.e., bacteriocins and bacteriocin-like polypeptides) metabolites (52). Several findings suggested that Lactobacillus-derived CFS acts as bio-liquid-detergent reducing the adhesion and biofilm formation of pathogens to abiotic and biotic surfaces. The formation of biofilms of multidrug resistant superbugs, namely P. aeruginosa and S. aureus, were successfully mitigated by CFS recovered from different Lactobacillus species, including L. casei, L. fermentum, L. gasseri, L. plantarum, and L. salivarius (73–77). Several authors investigated the effects of CFS deriving from Lactobacillus spp. commonly used as dietary products on the development of dental caries. As an example, Lactobacillus brevis FF2, isolated from fermented oil, exerted anti-biofilm activity against S. salivarius. Clinically isolates belonging to L. paracasei and L. fermentum species significantly reduced the number of cariogenic S. mutans UA159 cells in biofilm grown on hydroxyapatite (62), while Lin et al. found that CFS from five lactobacilli inhibited the biofilm formation of the same pathogen on the glass surface. The acidic environment produced by CFS seemed to be important to elicit the anti-biofilm effects since the activity was lost for three strains out of five after adjusting the pH of CFS to 6.5 (31). Moreover, Jeong et al. observed that Lactobacillus-derived CFS caused a variation in streptococcal expression of three classes of biofilm-formation associated genes, namely those involved in carbohydrate metabolism, adhesion, and biofilm structure (63). Srivastava et al. reported that L. plantarum 108 CFS inhibited the formation of S. mutans and C. albicans mixed biofilm by 85%, and also reduced preformed biofilm by 33%. As observed for living cells, probiotic CFS downregulated the expression of S. mutans genes associated with Gtfs activity (gtfB, gtfC, and gtfD) and C. albicans genes involved in adhesion (ALS3 and ALS1) and hyphae formation (HWP1) (64). Furthermore, Lactobacillus-derived CFS altered biofilm formation and the transcription of virulence-associated genes of periodontal pathogens. The anti-biofilm activity exerted by L. rhamnosus (Lr32 and HN001) and L. acidophilus (LA5 and NCFM) CFS was associated with a diminished expression of two exotoxins of A. actinomycetemcomitans (leukotoxin and CDT) and with the downregulation of katA, encoding a catalase, which promote Aggregatibacter survival under oxidative stress (66). L. acidophilus LA5 CFS also reduced the abundance of P. gingivalis in multispecies biofilm along with S. oralis and S. gordonii. In this case, a panel of P. gingivalis genes resulted downregulated, including fimA (encoding the main fimbriae), MFA1 (involved in auto-aggregation), kgp and rgp (involved in EPS accumulation), and Lux6 (pivotal in quorum sensing) (33).
Conclusions
Therapies for periodontal diseases are costly and may not be sufficiently effective. Findings explored in the present mini-review highlighted that lactobacilli can behave as anti-biofilm agents against a variety of microorganisms responsible for oral diseases, although the activity strongly varies among different Lactobacillus strains. Notably, not only living cells but also Lactobacillus derivatives may exert antipathogenic effects. Thus, the employment of postbiotics, paraprobiotics, or a mixture of both can represent a novel bio-therapeutic approach to face biofilm-related oral diseases, favoring the formulation stability and safety. It is also a step forward in the search for alternative solutions to the use of antibiotics, in the perspective of containing the spread of antibiotic resistance.
Author Contributions
All authors listed have made a substantial, direct and intellectual contribution to the work, and approved it for publication.
Funding
This study was supported by the Italian Ministry for University and Research.
Conflict of Interest
The authors declare that the research was conducted in the absence of any commercial or financial relationships that could be construed as a potential conflict of interest.
Publisher's Note
All claims expressed in this article are solely those of the authors and do not necessarily represent those of their affiliated organizations, or those of the publisher, the editors and the reviewers. Any product that may be evaluated in this article, or claim that may be made by its manufacturer, is not guaranteed or endorsed by the publisher.
References
1. Del Pozo JL. Biofilm-related disease. Expert Rev Anti Infect Ther. (2018) 16:51–65. doi: 10.1080/14787210.2018.1417036
2. Hall-Stoodley L, Costerton JW, Stoodley P. Bacterial biofilms: from the natural environment to infectious diseases. Nat Rev Microbiol. (2004) 2:95–108. doi: 10.1038/nrmicro821
3. Darouiche RO. Treatment of infections associated with surgical implants. N Engl J Med. (2004) 350:1422–9. doi: 10.1056/NEJMra035415
4. Donlan RM. Biofilm formation: a clinically relevant microbiological process. Clin Infect Dis an Off Publ Infect Dis Soc Am. (2001) 33:1387–92. doi: 10.1086/322972
5. Hardy L, Cerca N, Jespers V, Vaneechoutte M, Crucitti T. Bacterial biofilms in the vagina. Res Microbiol. (2017) 168:865–74. doi: 10.1016/j.resmic.2017.02.001
6. Lewis K. Riddle of biofilm resistance. Antimicrob Agents Chemother. (2001) 45:999–1007. doi: 10.1128/AAC.45.4.999-1007.2001
7. Cerca N, Martins S, Cerca F, Jefferson KK, Pier GB, Oliveira R, et al. Comparative assessment of antibiotic susceptibility of coagulase-negative staphylococci in biofilm versus planktonic culture as assessed by bacterial enumeration or rapid XTT colorimetry. J Antimicrob Chemother. (2005) 56:331–6. doi: 10.1093/jac/dki217
8. Prax M, Bertram R. Metabolic aspects of bacterial persisters. Front Cell Infect Microbiol. (2014) 4:148. doi: 10.3389/fcimb.2014.00148
9. Gupta P, Sarkar S, Das B, Bhattacharjee S, Tribedi P. Biofilm, pathogenesis and prevention–a journey to break the wall: a review. Arch Microbiol. (2016) 198:1–15. doi: 10.1007/s00203-015-1148-6
10. Teitzel GM, Parsek MR. Heavy metal resistance of biofilm and planktonic Pseudomonas aeruginosa. Appl Environ Microbiol. (2003) 69:2313–20. doi: 10.1128/AEM.69.4.2313-2320.2003
11. Walters MC. 3rd, Roe F, Bugnicourt A, Franklin MJ, Stewart PS. Contributions of antibiotic penetration, oxygen limitation, and low metabolic activity to tolerance of Pseudomonas aeruginosa biofilms to ciprofloxacin and tobramycin. Antimicrob Agents Chemother. (2003) 47:317–23. doi: 10.1128/AAC.47.1.317-323.2003
12. Del Pozo JL, Patel R. Are antibiotics and surgery sufficient to treat biofilm-associated infections? Enferm Infecc Microbiol Clin. (2013) 31:641–2. doi: 10.1016/j.eimc.2013.10.001
13. Lewis K. Persister cells. Annu Rev Microbiol. (2010) 64:357–72. doi: 10.1146/annurev.micro.112408.134306
14. Cotter PD, Ross RP, Hill C. Bacteriocins - a viable alternative to antibiotics? Nat Rev Microbiol. (2013) 11:95–105. doi: 10.1038/nrmicro2937
15. Whelan K, Quigley EMM. Probiotics in the management of irritable bowel syndrome and inflammatory bowel disease. Curr Opin Gastroenterol. (2013) 29:184–9. doi: 10.1097/MOG.0b013e32835d7bba
16. FAO/WHO. Probiotics in food. Health and nutritional properties and guidelines for evaluation. FAO Food Nutr. (2006) 85:ISBN 92-5-105513-0.
17. Parvez S, Malik KA, Ah Kang S, Kim H-Y. Probiotics and their fermented food products are beneficial for health. J Appl Microbiol. (2006) 100:1171–85. doi: 10.1111/j.1365-2672.2006.02963.x
18. Marsh PD, Zaura E. Dental biofilm: ecological interactions in health and disease. J Clin Periodontol. (2017) 44:S12–22. doi: 10.1111/jcpe.12679
19. Lafaurie GI, Sabogal MA, Castillo DM, Rincón MV, Gómez LA, Lesmes YA, et al. Microbiome and microbial biofilm profiles of peri-implantitis: a systematic review. J Periodontol. (2017) 88:1066–89. doi: 10.1902/jop.2017.170123
20. How KY, Song KP, Chan KG. Porphyromonas gingivalis: An overview of periodontopathic pathogen below the gum line. Front Microbiol. (2016) 7:53. doi: 10.3389/fmicb.2016.00053
21. Amado PPP, Kawamoto D, Albuquerque-Souza E, Franco DC, Saraiva L, Casarin RCV, et al. Oral and fecal microbiome in molar-incisor pattern periodontitis. Front Cell Infect Microbiol. (2020) 10:583761. doi: 10.3389/fcimb.2020.583761
22. Teughels W, Dhondt R, Dekeyser C, Quirynen M. Treatment of aggressive periodontitis. Periodontol 2000. (2014) 65:107–133. doi: 10.1111/prd.12020
23. Socransky SS, Haffajee AD. Dental biofilms: difficult therapeutic targets. Periodontol 2000. (2002) 28:12–55. doi: 10.1034/j.1600-0757.2002.280102.x
24. de Carvalho FG, Silva DS, Hebling J, Spolidorio LC, Spolidorio DMP. Presence of mutans streptococci and Candida spp. in dental plaque/dentine of carious teeth and early childhood caries. Arch Oral Biol. (2006) 51:1024–8. doi: 10.1016/j.archoralbio.2006.06.001
25. Radović K, Ilić J, Roganović J, Stojić D, Brković B, Pudar G. Denture stomatitis and salivary vascular endothelial growth factor in immediate complete denture wearers with type 2 diabetes. J Prosthet Dent. (2014) 111:373–9. doi: 10.1016/j.prosdent.2013.07.019
26. Hasslöf P, Stecksén-Blicks C. Chapter 10: Probiotic bacteria and dental caries. Monogr Oral Sci. (2020) 28:99–107. doi: 10.1159/000455377
27. Söderling EM, Marttinen AM, Haukioja AL. Probiotic lactobacilli interfere with Streptococcus mutans biofilm formation in vitro. Curr Microbiol. (2011) 62:618–22. doi: 10.1007/s00284-010-9752-9
28. Marttinen AM, Haukioja AL, Keskin M, Söderling EM. Effects of Lactobacillus reuteri PTA 5289 and L. paracasei DSMZ16671 on the adhesion and biofilm formation of Streptococcus mutans. Curr Microbiol. (2013) 67:193–199. doi: 10.1007/s00284-013-0352-3
29. Wu CC, Lin CT, Wu CY, Peng WS, Lee MJ, Tsai YC. Inhibitory effect of Lactobacillus salivarius on Streptococcus mutans biofilm formation. Mol Oral Microbiol. (2015) 30:16–26. doi: 10.1111/omi.12063
30. Lee SH, Kim YJ A. comparative study of the effect of probiotics on cariogenic biofilm model for preventing dental caries. Arch Microbiol. (2014) 196:601–9. doi: 10.1007/s00203-014-0998-7
31. Lin X, Chen X, Chen Y, Jiang W, Chen H. The effect of five probiotic lactobacilli strains on the growth and biofilm formation of Streptococcus mutans. Oral Dis. (2015) 21:e128–34. doi: 10.1111/odi.12257
32. Krzyściak W, Kościelniak D, Papiez M, Vyhouskaya P, Zagórska-Swiezy K, Kołodziej I, Bystrowska B, Jurczak A. Effect of a lactobacillus salivarius probiotic on a double-species streptococcus mutans and candida albicans caries biofilm. Nutrients. (2017) 9:1242. doi: 10.3390/nu9111242
33. Ishikawa KH, Mita D, Kawamoto D, Nicoli JR, Albuquerque-Souza E, Lorenzetti Simionato MR, et al. Probiotics alter biofilm formation and the transcription of Porphyromonas gingivalis virulence-associated genes. J Oral Microbiol. (2020) 12:1805553. doi: 10.1080/20002297.2020.1805553
34. Jaffar N, Ishikawa Y, Mizuno K, Okinaga T, Maeda T. Mature Biofilm Degradation by Potential Probiotics: Aggregatibacter actinomycetemcomitans versus Lactobacillus spp. PLoS ONE. (2016) 11:e0159466. doi: 10.1371/journal.pone.0159466
35. Rossoni RD, de Barros PP, de Alvarenga JA, Ribeiro F de C, Velloso MDS, Fuchs BB, et al. Antifungal activity of clinical Lactobacillus strains against Candida albicans biofilms: identification of potential probiotic candidates to prevent oral candidiasis. Biofouling. (2018) 34:212–25. doi: 10.1080/08927014.2018.1425402
36. James KM, MacDonald KW, Chanyi RM, Cadieux PA, Burton JP. Inhibition of Candida albicans biofilm formation and modulation of gene expression by probiotic cells and supernatant. J Med Microbiol. (2016) 65:328–36. doi: 10.1099/jmm.0.000226
37. Takahashi N, Nyvad B. The role of bacteria in the caries process: ecological perspectives. J Dent Res. (2011) 90:294–303. doi: 10.1177/0022034510379602
38. Twetman S, Keller MK. Probiotics for caries prevention and control. Adv Dent Res. (2012) 24:98–102. doi: 10.1177/0022034512449465
39. Fan Y, He H, Dong Y, Pan H. Hyphae-specific genes HGC1, ALS3, HWP1, and ECE1 and relevant signaling pathways in Candida albicans. Mycopathologia. (2013) 176:329–35. doi: 10.1007/s11046-013-9684-6
40. Maiti P, Ghorai P, Ghosh S, Kamthan M, Tyagi RK, Datta A. Mapping of functional domains and characterization of the transcription factor Cph1 that mediate morphogenesis in Candida albicans. Fungal Genet Biol. (2015) 83:45–57. doi: 10.1016/j.fgb.2015.08.004
41. Ezendam J, van Loveren H. Probiotics: immunomodulation and evaluation of safety and efficacy. Nutr Rev. (2006) 64:1–14. doi: 10.1111/j.1753-4887.2006.tb00168.x
42. Yanine N, Araya I, Brignardello-Petersen R, Carrasco-Labra A, González A, Preciado A, et al. Effects of probiotics in periodontal diseases: a systematic review. Clin Oral Investig. (2013) 17:1627–34. doi: 10.1007/s00784-013-0990-7
43. Twetman S, Stecksén-Blicks C. Probiotics and oral health effects in children. Int J Paediatr Dent. (2008) 18:3–10. doi: 10.1111/j.1365-263X.2007.00885.x
44. Romani Vestman N, Hasslöf P, Keller MK, Granström E, Roos S, Twetman S, et al. Lactobacillus reuteri influences regrowth of mutans streptococci after full-mouth disinfection: a double-blind, randomised controlled trial. Caries Res. (2013) 47:338–45. doi: 10.1159/000347233
45. Colloca ME, Ahumada MC, López ME, Nader-Macías ME. Surface properties of lactobacilli isolated from healthy subjects. Oral Dis. (2000) 6:227–33. doi: 10.1111/j.1601-0825.2000.tb00118.x
46. Matsuda Y, Cho O, Sugita T, Ogishima D, Takeda S. Culture Supernatants of Lactobacillus gasseri and L. crispatus Inhibit Candida albicans Biofilm Formation and Adhesion to HeLa Cells. Mycopathologia. (2018) 183:691–700. doi: 10.1007/s11046-018-0259-4
47. Bamford C V. d'Mello A, Nobbs AH, Dutton LC, Vickerman MM, Jenkinson HF. Streptococcus gordonii modulates Candida albicans biofilm formation through intergeneric communication. Infect Immun. (2009) 77:3696–704. doi: 10.1128/IAI.00438-09
48. Vilela SFG, Barbosa JO, Rossoni RD, Santos JD, Prata MCA, Anbinder AL, et al. Lactobacillus acidophilus ATCC 4356 inhibits biofilm formation by C. albicans and attenuates the experimental candidiasis in Galleria mellonella. Virulence. (2015) 6:29–39. doi: 10.4161/21505594.2014.981486
49. Cicenia A, Scirocco A, Carabotti M, Pallotta L, Marignani M, Severi C. Postbiotic activities of lactobacilli-derived factors. J Clin Gastroenterol. (2014) 48 Suppl 1:S18–22. doi: 10.1097/MCG.0000000000000231
50. Teame T, Wang A, Xie M, Zhang Z, Yang Y, Ding Q, et al. Paraprobiotics and postbiotics of probiotic lactobacilli, their positive effects on the host and action mechanisms: a review. Front Nutr. (2020) 7:570344. doi: 10.3389/fnut.2020.570344
51. Aguilar-Toalá JE, Garcia-Varela R, Garcia HS, Mata-Haro V, González-Córdova AF, Vallejo-Cordoba B, et al. Postbiotics: An evolving term within the functional foods field. Trends Food Sci Technol. (2018) 75:105–14. doi: 10.1016/j.tifs.2018.03.009
52. Nataraj BH, Ali SA, Behare P V, Yadav H. Postbiotics-parabiotics: the new horizons in microbial biotherapy and functional foods. Microb Cell Fact. (2020) 19:168. doi: 10.1186/s12934-020-01426-w
53. Doron S, Snydman DR. Risk and safety of probiotics. Clin Infect Dis. (2015) 60 Suppl 2:S129–34. doi: 10.1093/cid/civ085
54. Koohestani M, Moradi M, Tajik H, Badali A. Effects of cell-free supernatant of Lactobacillus acidophilus LA5 and Lactobacillus casei 431 against planktonic form and biofilm of Staphylococcus aureus. Vet Res forum. (2018) 9:301–6. doi: 10.30466/vrf.2018.33086
55. Ahn KB, Baik JE, Park OJ, Yun CH, Han SH. Lactobacillus plantarum lipoteichoic acid inhibits biofilm formation of Streptococcus mutans. PLoS ONE. (2018) 13:e0192694. doi: 10.1371/journal.pone.0192694
56. Kim AR, Ahn KB, Yun CH, Park OJ, Perinpanayagam H, Yoo Y-J, et al. Lactobacillus plantarum Lipoteichoic Acid Inhibits Oral Multispecies Biofilm. J Endod. (2019) 45:310–5. doi: 10.1016/j.joen.2018.12.007
57. Noda M, Sugihara N, Sugimoto Y, Hayashi I, Sugimoto S, Danshiitsoodol N, Sugiyama M. Lactobacillus reuteri BM53-1 Produces a Compound That Inhibits Sticky Glucan Synthesis by Streptococcus mutans. Microorganisms. (2021) 9:1390. doi: 10.3390/microorganisms9071390
58. Ciandrini E, Campana R, Casettari L, Perinelli DR, Fagioli L, Manti A, et al. Characterization of biosurfactants produced by Lactobacillus spp. and their activity against oral streptococci biofilm. Appl Microbiol Biotechnol. (2016) 100:6767–77. doi: 10.1007/s00253-016-7531-7
59. Tahmourespour A, Salehi R, Kasra Kermanshahi R. Lactobacillus acidophilus-derived bosurfactant effect on GTFB and GTFC expression level in Streptococcus mutans biofilm Cells. Brazilian J Microbiol. (2011) 42:330–9. doi: 10.1590/S1517-83822011000100042
60. Savabi O, Kazemi M, Kamali S, Salehi AR, Eslami G, Tahmourespour A, et al. Effects of biosurfactant produced by Lactobacillus casei on gtfB, gtfC, and ftf gene expression level in S. mutans by real-time RT-PCR. Adv Biomed Res. (2014) 3:231. doi: 10.4103/2277-9175.145729
61. Tahmourespour A, Kasra-Kermanshahi R, Salehi R. Lactobacillus rhamnosus biosurfactant inhibits biofilm formation and gene expression of caries-inducing Streptococcus mutans. Dent Res J. (2019) 16:87–94.
62. Rossoni RD, Velloso MDS, de Barros PP, de Alvarenga JA, Santos JD. Dos, Santos Prado ACC Dos, et al. Inhibitory effect of probiotic Lactobacillus supernatants from the oral cavity on Streptococcus mutans biofilms. Microb Pathog. (2018) 123:361–7. doi: 10.1016/j.micpath.2018.07.032
63. Jeong D, Kim DH, Song KY, Seo KH. Antimicrobial and anti-biofilm activities of Lactobacillus kefiranofaciens DD2 against oral pathogens. J Oral Microbiol. (2018) 10:1472985. doi: 10.1080/20002297.2018.1472985
64. Srivastava N, Ellepola K, Venkiteswaran N, Chai LYA, Ohshima T, Seneviratne CJ. Lactobacillus Plantarum 108 Inhibits Streptococcus mutans and Candida albicans Mixed-Species Biofilm Formation. Antibiotics. (2020) 9:1–20. doi: 10.3390/antibiotics9080478
65. Ben Taheur F, Kouidhi B, Fdhila K, Elabed H, Ben Slama R, Mahdouani K, et al. Anti-bacterial and anti-biofilm activity of probiotic bacteria against oral pathogens. Microb Pathog. (2016) 97:213–20. doi: 10.1016/j.micpath.2016.06.018
66. Ishikawa KH, Bueno MR, Kawamoto D, Simionato MRL, Mayer MPA. Lactobacilli postbiotics reduce biofilm formation and alter transcription of virulence genes of Aggregatibacter actinomycetemcomitans. Mol Oral Microbiol. (2021) 36:92–102. doi: 10.1111/omi.12330
67. Song YG, Lee SH. Inhibitory effects of Lactobacillus rhamnosus and Lactobacillus casei on Candida biofilm of denture surface. Arch Oral Biol. (2017) 76:1–6. doi: 10.1016/j.archoralbio.2016.12.014
68. Weidenmaier C, Peschel A. Teichoic acids and related cell-wall glycopolymers in Gram-positive physiology and host interactions. Nat Rev Microbiol. (2008) 6:276–87. doi: 10.1038/nrmicro1861
69. Ahn KB, Baik JE, Yun CH, Han SH. Lipoteichoic acid inhibits staphylococcus aureus biofilm formation. Front Microbiol. (2018) 9:327. doi: 10.3389/fmicb.2018.00327
70. Jung S, Park OJ, Kim AR, Ahn KB, Lee D, Kum KY, et al. Lipoteichoic acids of lactobacilli inhibit Enterococcus faecalis biofilm formation and disrupt the preformed biofilm. J Microbiol. (2019) 57:310–5. doi: 10.1007/s12275-019-8538-4
71. Abdalla AK, Ayyash MM, Olaimat AN, Osaili TM, Al-Nabulsi AA, Shah NP, et al. Exopolysaccharides as Antimicrobial Agents: Mechanism and Spectrum of Activity. Front Microbiol. (2021) 12:664395. doi: 10.3389/fmicb.2021.664395
72. Gudiña EJ, Teixeira JA, Rodrigues LR. Isolation and functional characterization of a biosurfactant produced by Lactobacillus paracasei. Colloids Surf B Biointerfaces. (2010) 76:298–304. doi: 10.1016/j.colsurfb.2009.11.008
73. Lenzmeier TD, Mudaliar NS, Stanbro JA, Watters C, Ahmad A, Simons MP, et al. Application of Lactobacillus gasseri 63 AM supernatant to Pseudomonas aeruginosa-infected wounds prevents sepsis in murine models of thermal injury and dorsal excision. J Med Microbiol. (2019) 68:1560–72. doi: 10.1099/jmm.0.001066
74. Abootaleb M, Mohammadi Bandari N, Arbab Soleimani N. Interference of Lactobacillus casei with Pseudomonas aeruginosa in the treatment of infected burns in Wistar rats. Iran J Basic Med Sci. (2021) 24:143–9. doi: 10.22038/IJBMS.2020.47447.10920
75. Díaz MA, González SN, Alberto MR, Arena ME. Human probiotic bacteria attenuate Pseudomonas aeruginosa biofilm and virulence by quorum-sensing inhibition. Biofouling. (2020) 36:597–609. doi: 10.1080/08927014.2020.1783253
76. Kang MS, Lim HS, Oh JS, Lim YJ, Wuertz-Kozak K, Harro JM, Shirtliff ME, Achermann Y. Antimicrobial activity of Lactobacillus salivarius and Lactobacillus fermentum against Staphylococcus aureus. Pathog Dis. (2017) 75: ftx009. doi: 10.1093/femspd/ftx009
Keywords: lactobacilli, probiotics, postbiotics, paraprobiotics, biofilm, oral diseases
Citation: Giordani B, Parolin C and Vitali B (2021) Lactobacilli as Anti-biofilm Strategy in Oral Infectious Diseases: A Mini-Review. Front. Med. Technol. 3:769172. doi: 10.3389/fmedt.2021.769172
Received: 01 September 2021; Accepted: 20 September 2021;
Published: 20 October 2021.
Edited by:
Zeljka Vanic, University of Zagreb, CroatiaReviewed by:
Maja Šegvić Klarić, University of Zagreb, CroatiaDaniela Jakšić, University of Zagreb, Croatia
Copyright © 2021 Giordani, Parolin and Vitali. This is an open-access article distributed under the terms of the Creative Commons Attribution License (CC BY). The use, distribution or reproduction in other forums is permitted, provided the original author(s) and the copyright owner(s) are credited and that the original publication in this journal is cited, in accordance with accepted academic practice. No use, distribution or reproduction is permitted which does not comply with these terms.
*Correspondence: Barbara Giordani, barbara.giordani4@unibo.it