- 1Department of Internal Medicine, Yale School of Medicine, New Haven, CT, United States
- 2Specialty Pharmacy, Yale New Haven Hospital, New Haven, CT, United States
- 3Department of Pediatrics, Yale School of Medicine, New Haven, CT, United States
Introduction: Gaucher disease (GD), a lysosomal storage disorder, results from the accumulation of glycosphingolipids due to deficient lysosomal glucocerebrosidase activity. This pathological accumulation triggers immune activation, which paradoxically induces UDPglucose ceramide glucosyltransferase (UGCG), further exacerbating the metabolic defect. Eliglustat, a highly specific inhibitor of UGCG, functions as a substrate reduction therapy (SRT) and has demonstrated efficacy in reversing GD manifestations in clinical trials and real-world settings. Despite its established safety profile, preclinical studies have shown that supratherapeutic concentrations of eliglustat can inhibit ion channels involved in cardiac electrophysiology. However, pharmacogenomic-guided dosing ensures therapeutic efficacy while maintaining a wide safety margin, minimizing such risks. Nevertheless, lingering concerns regarding cardiac safety have persisted, particularly in patients with preexisting cardiac comorbidities.
Methods: We report a single-center experience of eliglustat use in 13 patients with type 1 Gaucher disease (GD1) and concurrent cardiac comorbidities. Patients underwent standard cardiac evaluations, including electrocardiogram (EKG) with QTc interval assessment and echocardiogram. Eliglustat dosing was guided by CYP2D6 metabolizer status, and potential drug–drug interactions (DDIs) were carefully monitored.
Results: Cardiac comorbidities included prior myocardial infarction (n = 2), aortic stenosis (n = 2), atrial fibrillation (n = 2), Wolff-Parkinson-White syndrome (n = 1), pericarditis (n = 1), premature ventricular complexes (n = 2), severe pulmonary arterial hypertension with right heart strain (n = 1), mitral annular calcification with diastolic dysfunction (n = 1), and mildly prolonged QTc interval (n = 1). No patients experienced arrhythmia, QTc prolongation, or arrhythmia-related symptoms. Treatment discontinuation was not required. All patients achieved expected therapeutic outcomes, as evidenced by serial reductions in glucosylsphingosine (GlcSph) levels and other disease indicators.
Conclusion: This study represents the first real-world clinical evidence evaluating Eliglustat’s cardiac safety in a high-risk GD1 population. Unlike prior theoretical concerns derived from in vitro ion channel studies, our findings demonstrate that Eliglustat does not induce clinically significant cardiac events when administered according to pharmacogenomic guidelines. The misinformation regarding Eliglustat’s cardiotoxicity, largely driven by speculative interpretations rather than clinical data, is effectively countered by our findings, which show no significant QT prolongation or arrhythmias over a median treatment duration of 8 years.
Introduction
Gaucher disease (GD) arises from biallelic mutations in the GBA1 gene, resulting in defective acid β-glucosidase activity and the lysosomal accumulation of glucosylceramide (GlcCer) and glucosylsphingosine (GlcSph) (1, 2). The accumulation of these lipids triggers immune activation and paradoxically upregulates UDP-glucose ceramide glucosyltransferase (UGCG), further exacerbating the metabolic defect (3, 4). Accordingly, eliglustat, a selective UGCG inhibitor, has shown excellent efficacy in type 1 Gaucher disease (GD1) in both clinical trials and real-world settings (5–9). Pharmacogenomic dosing based on CYP2D6 genotype ensures therapeutic levels are achieved while maintaining a wide safety margin (10).
Preclinical studies of eliglustat demonstrated in vitro inhibition of several cardiac ion channels, including the human Ether-à-go-go Related Gene (hERG) channel (IC50 730 nM), Nav1.5 (IC50 11,000 nM), and Cav1.2 (IC50 25,000 nM), as illustrated in Figure 1 (7). However, Clinical Evidence from real-world applications strongly suggests that pharmacogenomic-based dosing ensures plasma levels remain well below proarrhythmic thresholds. Despite this safety margin, concerns have persisted about potential cardiac risks, including arrhythmias such as polymorphic ventricular tachycardia, particularly in patients with preexisting cardiac conditions or long QT syndrome (11, 12). Notably, neither EMA nor FDA has revised cardiac safety labeling since regulatory approval, reflecting the absence of real-world safety concerns.
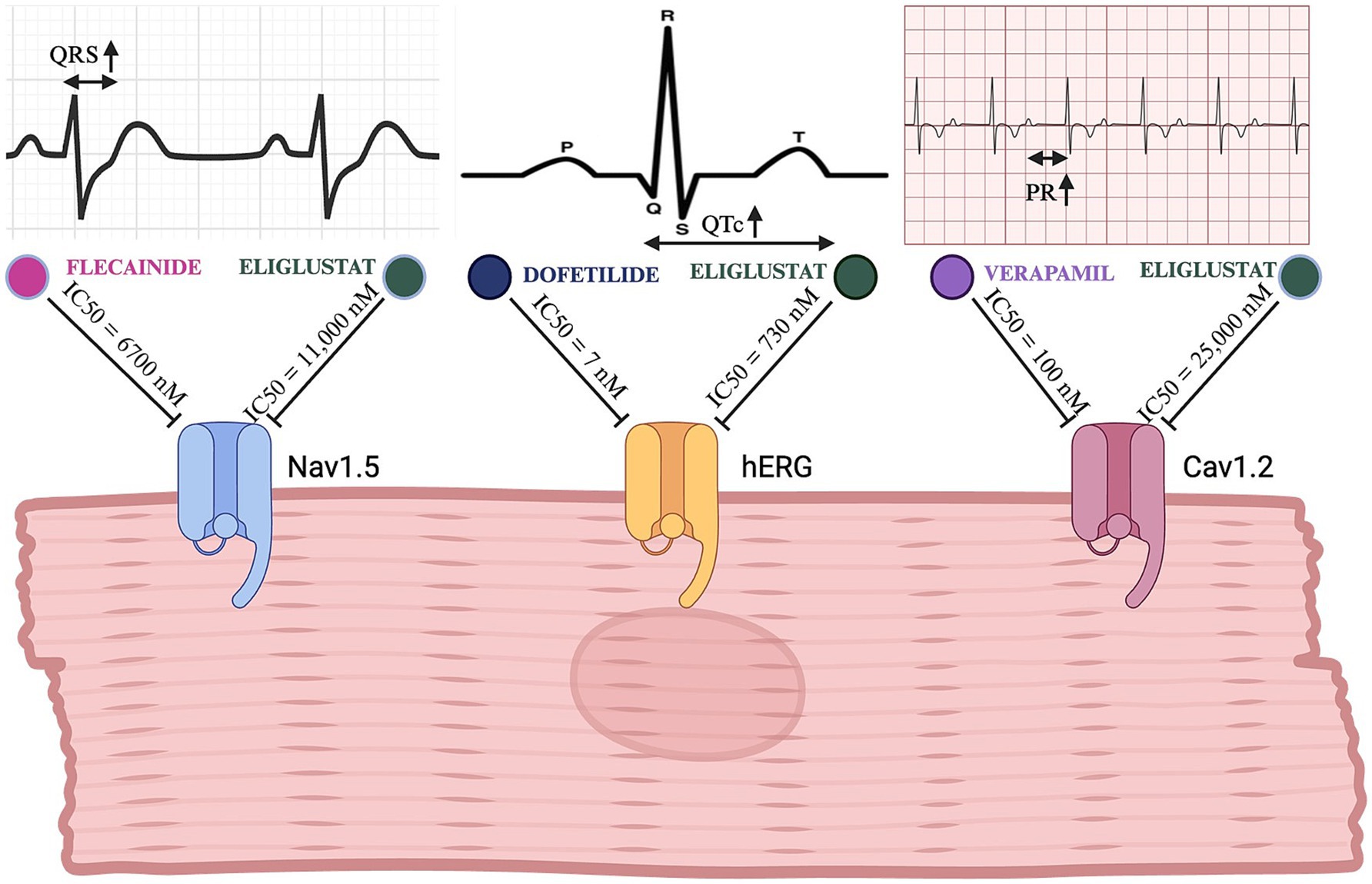
Figure 1. Comparative electrophysiological effects of eliglustat and clinically used channel-specific inhibitors. This figure illustrates the inhibitory effects of eliglustat on the Nav1.5, hERG, and Cav1.2 cardiac channels in comparison to specific channel inhibitors (flecainide, dofetilide, and verapamil, respectively). The top panels show EKG tracings highlighting the specific intervals/durations affected by each channel’s inhibition (QRS for Nav1.5, QTc for hERG, and PR for Cav1.2). Created in BioRender. (23) BioRender.com/u76q381.
To date, there remains a paucity of real-world clinical data evaluating the safety and efficacy of eliglustat substrate reduction therapy (SRT) in GD1 patients with significant cardiac comorbidities. To address this gap, we present a single-center experience involving 13 GD1 patients with concurrent cardiac conditions treated at a large tertiary referral center in the United States (Figure 2).
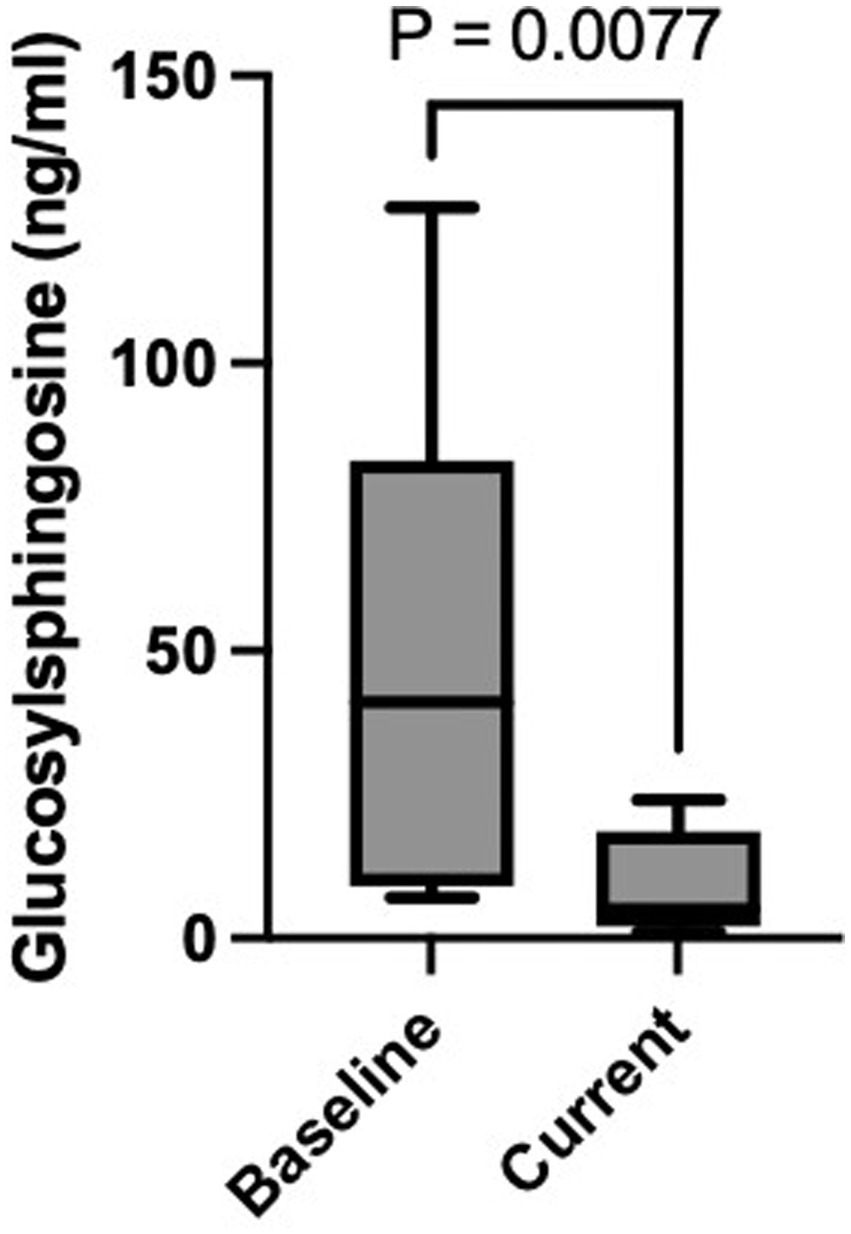
Figure 2. Baseline and current plasma GlcSph level. Figure highlights the statistically significant difference in plasma GlcSph level between baseline and after treatment with eliglustat SRT (p = 0.007).
Methods
Study setting
The study was conducted at a tertiary referral center managing a large cohort of Gaucher disease (GD) patients. This specialized setting allowed for a robust evaluation of substrate reduction therapy (SRT) in a subset of GD1 patients with cardiac comorbidities.
Study population
The study included 13 patients diagnosed with GD1 and concurrent cardiac comorbidities. GD diagnosis was confirmed by reduced leukocyte acid β-glucosidase activity and/or GBA1 mutation analysis. Baseline characteristics and demographics are summarized in Table 1. Cardiac comorbidities included myocardial infarction (n = 2), atrial fibrillation (n = 2), aortic stenosis (n = 2), Wolff-Parkinson-White syndrome (n = 1), pericarditis (n = 1), severe pulmonary arterial hypertension with right bundle branch block (RBBB) (n = 1), premature ventricular contractions (PVCs) (n = 2), acquired long QT syndrome (n = 1), and diastolic dysfunction (n = 1). These patients were selected to evaluate eliglustat therapy outcomes in the context of cardiac comorbidities.
Demonstrative case of pulmonary arterial hypertension, RBBB, LAFB, and SRT transition
This case exemplifies the significance of evaluating the safety and efficacy of eliglustat in patients with Gaucher disease and concurrent cardiac conditions. An adult patient, who underwent splenectomy for hypersplenism at age 14, developed severe pulmonary hypertension as a consequence of untreated Gaucher disease (13). Despite 20 years of intensive enzyme replacement therapy (ERT), which even required weekly dosing to achieve a modest response and significantly disrupted the patient’s life, residual disease persisted. The patient exhibited markedly elevated serum glucosylsphingosine (GlcSph) levels, >155-fold above the normal range, suggesting ongoing metabolic burden. Since the patient had achieved remission of visceral disease (normal liver volumes and liver function tests; the patient was asplenic), hematologic disease (normal blood counts and no marrow infiltration on MRI), and bone disease (stable bone density, no new avascular necrosis, and no active bone infiltration), the persistently elevated biomarker suggested a primary contribution from the lungs and pulmonary vascular disease. Studies of ERT targeting in vivo have shown that>95% of infused enzyme molecules are taken up the liver, spleen, and bone marrow; hence, pulmonary disease is a sanctuary site for Gaucher cells (14, 15).
Cardiac evaluation revealed right bundle branch block (RBBB) and left anterior fascicular block (LAFB) on electrocardiogram (EKG). Given the patient’s CYP2D6 metabolizer status, eliglustat was introduced as an alternative therapy. The transition to eliglustat led to marked clinical improvement, with the patient reporting significant reductions in dyspnea, fatigue, and palpitations. Follow-up assessments demonstrated stabilization of cardiac conduction intervals on EKG and an impressive 91% reduction in GlcSph levels, from 155 ng/mL to 14 ng/mL after just 1 year of SRT (Figures 3A,B). These findings reinforce the potential therapeutic advantage of eliglustat in managing Gaucher disease patients with pulmonary and cardiac involvement, particularly in cases where ERT may be suboptimal.
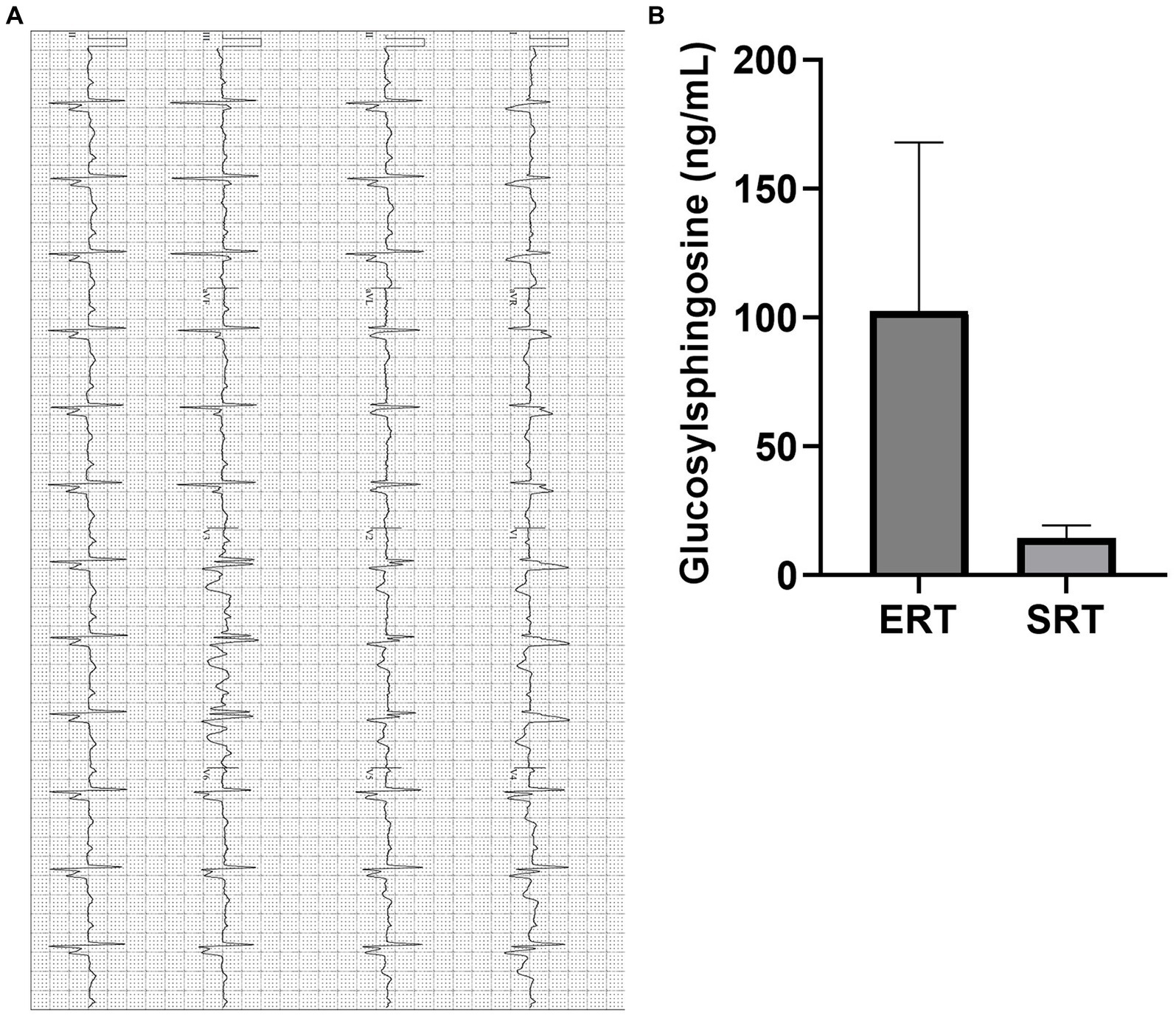
Figure 3. (A) EKG of a patient with PAH (Illustrative case). Electrocardiogram showing evidence of right bundle branch block (RBBB) with left axis bifascicular block (LAF) and mild atrial enlargement suggested by widening of the QRS complex, typical RSR’ pattern and left axis deviation. The QTc interval has remained within normal range on SRT, and symptomatically improved. PR: 214 ms, QTc: 460 ms, QRS: 159 ms. EKG, Electrocardiogram; PAH, Pulmonary Arterial Hypertension. (B) Plasma GlcSph levels on ERT and SRT of a patient with PAH (Illustrative case). Graph illustrates the reduction in plasma GlcSph level on more than 20 years of treatment with ERT vs. treatment with SRT for 1 year. There is 91% reduction in plasma GlcSph level. Error bars represent SD. GlcSph, Glucosylsphingosine, ERT, Enzyme replacement therapy; SRT, Substrate reduction therapy; PAH, Pulmonary Arterial Hypertension; SD, Standard Deviation.
Baseline evaluations
Comprehensive baseline evaluations included complete blood count (CBC), comprehensive metabolic profile (CMP), GD-specific biomarkers (chitotriosidase and GlcSph), ferritin, iron saturation, lipid profile, immunofixation electrophoresis, EKG (QTc interval, QRS, and PR intervals, arrhythmias), and echocardiography to assess cardiac function. Standard imaging testing, MRI of the abdomen lower extremities, and DXA scans were also performed.
Drug dosing
Pharmacogenomics-guided dosing of eliglustat was implemented based on each patient’s CYP2D6 metabolizer status, determined via genotyping.
DNA analysis for CYP2D6 genotyping is conducted using allele-specific real-time polymerase chain reaction (RT-PCR) to identify single-nucleotide polymorphisms (SNPs), insertions or deletions (indels), hybrid alleles, hybrid tandem variants, and copy number variants (CNVs) within the CYP2D6 gene. This assay enables the detection and classification of variant CYP2D6 alleles, including *2, *3, *4, *5 (deletion), *6, *7, *8, *9, *11, *12, *13 (hybrid), *13, *15, *17, *29, *31, *35, *36 (hybrid), *36 + *10 (hybrid tandem), *40, *41, *42, *49, *53, *59, and *68 (hybrid), as well as gene duplications (DUPs) with copy number (CN) designation. The *1 allele represents the reference (wild-type) sequence detected at the assessed loci (16).
Patients were classified as poor (PM), intermediate (IM), extensive (EM), or ultra-rapid metabolizers (UM). Poor metabolizers, who have significantly reduced CYP2D6 enzyme function, received 84 mg once daily to minimize drug accumulation. Intermediate metabolizers, with moderately reduced enzyme activity, were prescribed 84 mg twice daily, while extensive metabolizers, who exhibit normal CYP2D6 activity, followed the standard recommended dose of 84 mg twice daily.
Drug–drug interactions
In addition to pharmacogenomic stratification, potential drug–drug interactions (DDIs) were assessed. Medications that inhibit CYP2D6 (e.g., fluoxetine, paroxetine) or CYP3A4 (e.g., ketoconazole) were reviewed, and additional monitoring was implemented where necessary. We adhered to the recommended procedures for patients on concurrent QT-prolonging medications, conducting serial electrocardiographic assessments to ensure cardiac safety (Supplementary Table 1). In our study, no drug–drug interactions (DDIs) necessitated dose adjustments for any patient.
Outcome measures
Primary outcomes assessed safety and efficacy of eliglustat in GD1 patients with cardiac comorbidities. Hematological responses, visceral organ volumes, and GD-specific biomarkers (GlcSph and chitotriosidase) were measured. Serial EKGs monitored QTc prolongation, QRS and PR interval changes, and arrhythmias. Concomitant medications were reviewed to mitigate drug–drug interaction risks.
Disease-specific parameters
Efficacy was evaluated by monitoring GD-specific biomarkers (GlcSph and chitotriosidase) alongside hematological and visceral parameters. Biochemical responses and their impact on disease progression were tracked.
Plasma GlcSph was measured using LC–MS/MS: For sample preparation, 25 pg. of internal standard (N,N-Dimethylsphingosine; Matreya, Pleasant Gap, PA) was added to a 2.0 mL Eppendorf tube and evaporated to dryness under nitrogen gas. Plasma samples (20 μL) were mixed with 1,000 μL of chloroform:methanol (2:3, v/v), vortexed for 5 min, and centrifuged at 16,000 × g for 3 min. The supernatant was transferred to a fresh tube, followed by the addition of 200 μL chloroform and 520 μL water for liquid–liquid extraction. The solution was vortexed for 3 min, centrifuged at 16,000 × g for 3 min, and the lower organic phase was collected into an autosampler vial. The aqueous phase was re-extracted with chloroform, and the pooled lower phases were combined in the autosampler vial. The sample was then evaporated under nitrogen gas and reconstituted in 100 μL methanol:water (9:1, v/v) for liquid chromatography–tandem mass spectrometry (LC–MS/MS) analysis. A calibration curve was generated using lyso-GL1 standards (Matreya, Pleasant Gap, PA) processed identically to the plasma samples.
Reconstituted samples were analyzed using an LC–MS/MS system consisting of a Waters Acquity UPLC coupled to an API-5000 triple-quadrupole mass spectrometer. Separation of lyso-GL1 (glucosylsphingosine, lyso-Gb1) and other matrix components was achieved on an Acquity BEH C18 column (2.1 × 50 mm, 1.7 μm) under gradient elution conditions, with mobile phase A comprising 0.1% formic acid in water and mobile phase B consisting of 0.1% formic acid in acetonitrile. Mass spectrometry was performed in selected ion monitoring (SIM) mode with the following transitions: m/z 462.5 → 282.4 for lyso-GL1 and m/z 490.3 → 292.4 for N,N-Dimethylsphingosine (17).
Statistical analysis
Statistical analyses were conducted using R Studio and IBM SPSS Statistics. Paired t-tests assessed normally distributed variables (GlcSph, QTc, PR intervals) as determined by the Shapiro–Wilk test. Non-normally distributed variables (QRS intervals) were analyzed using the Wilcoxon rank-sum test. Missing data were excluded from the analysis.
Ethical considerations
Informed consent was obtained from all participants, allowing data collection from their standard care management for this longitudinal study.
Results
Patient characteristics
Baseline characteristics and demographics are summarized in Table 1. Among the 13 patients with GD1 and cardiac comorbidities, 8 (61%) were homozygous for the p.Asn409Ser/p.Asn409Ser mutation. The remaining 5 patients (39%) were heteroallelic, with the following genotypes: p.Asn409Ser and 84GinsertionG (n = 2; 15%), IVS2 + 1 (n = 1; 8%), p.Leu483Leu (n = 1; 8%), and a complex allele (n = 1).
Duration of therapy
The median duration of eliglustat therapy was 8 years (range: 1–13 years). Eleven patients (85%) remained on therapy throughout the study. One patient discontinued therapy due to dry skin and scaling, a known adverse effect of eliglustat. Another patient died at the age of 87 following complications from Parkinson’s disease and aspiration pneumonia.
Cardiac response
Despite a wide spectrum of pre-existing cardiac comorbidities, no new cardiac events were observed during the study. Specific responses included:
• A patient with recurrent pericarditis for over a decade requiring innumerable hospital admissions despite pericardiectomy achieved complete remission on eliglustat.
• A patient with severe pulmonary arterial hypertension (PAH) and right heart strain showed significant clinical improvement after transitioning from enzyme replacement therapy (ERT) to eliglustat monotherapy. This improvement correlated with a marked reduction in GlcSph levels.
• One patient was newly diagnosed with Wolff-Parkinson-White (WPW) syndrome during routine EKG monitoring and underwent successful accessory bundle ablation. Post-procedure, the patient tolerated eliglustat without complications.
No significant changes were observed in EKG parameters (PR, QRS, QTc intervals) during the study period. Furthermore, no patient experienced syncope, palpitations, or Torsade de Pointes on follow-up EKGs.
Biomarker response
Patients transitioning from ERT to eliglustat demonstrated a marked reduction in plasma GlcSph levels. The mean plasma GlcSph level decreased from 50.8 ng/mL pre-treatment to 9.5 ng/mL (normal <1.0 ng/mL), representing an 81% reduction (p = 0.007) (Table 2).
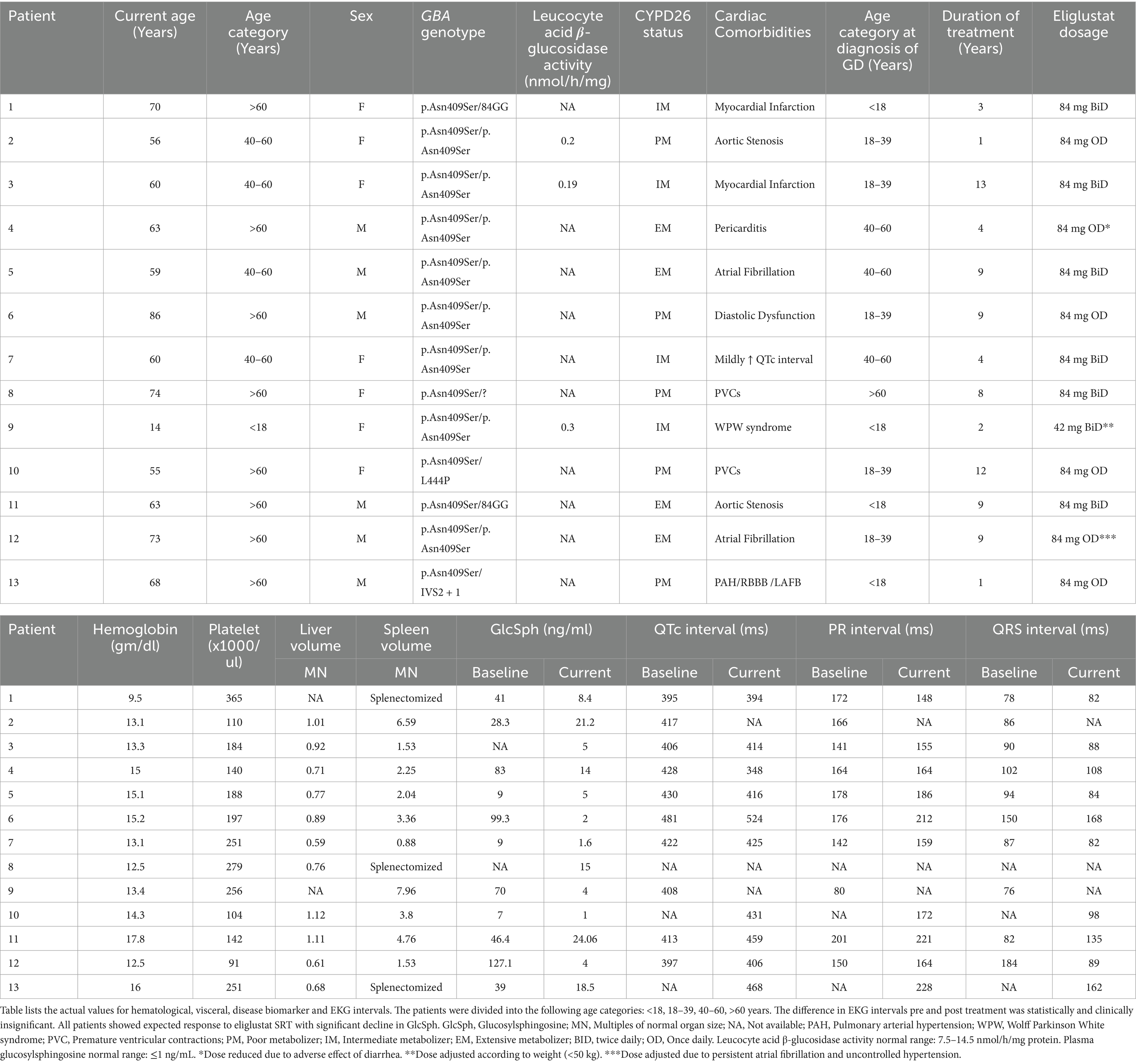
Table 2. Hematological, visceral, and EKG interval values at baseline and post treatment with eliglustat.
Adverse effects
Eliglustat was well tolerated across the cohort. Importantly, no significant EKG changes, including QTc interval prolongation, were observed. No syncopal episodes or adverse cardiac events occurred during the treatment period, supporting the favorable long-term safety profile of eliglustat in patients with cardiac comorbidities.
Discussion
This study provides valuable real-world evidence on the safety and efficacy of eliglustat as a substrate reduction therapy (SRT) in patients with type 1 Gaucher disease (GD1) and concurrent cardiac comorbidities. By implementing pharmacogenomic-guided dosing based on CYP2D6 metabolizer status, our findings demonstrate that eliglustat can be safely administered in this high-risk population without significant adverse cardiac events. This is particularly noteworthy given previous concerns regarding eliglustat’s potential to induce cardiac arrhythmias, primarily based on in vitro studies showing inhibition of key ion channels, including hERG, Nav1.5, and Cav1.2 (7). In our cohort, there were no significant changes in QTc, PR, or QRS durations, and no incidences of torsades de pointes, atrioventricular (AV) block, or life-threatening arrhythmias following the initiation of eliglustat therapy. These results align with existing clinical trial data and pharmacokinetic/pharmacodynamic (PK/PD) modeling. A Phase I study by Ruskin et al. (7) found no clinically significant electrocardiographic (EKG) changes at a mean maximum plasma concentration (Cmax) of 236.8 ng/mL. Furthermore, PK/PD modeling predicts that even at an eliglustat Cmax of 500 ng/mL—a level only achievable with concomitant CYP3A4 and CYP2D6 inhibitors—EKG intervals would increase only modestly (QTc by 13 ms, QRS by 7 ms, and PR by 22 ms). However, pharmacogenomic-guided dosing ensures that actual Cmax levels remain significantly lower, with typical values of 12.1–25 ng/mL in extensive metabolizers, 44.6 ng/mL in intermediate metabolizers, and 75 ng/mL in poor metabolizers—all well below the threshold associated with clinically meaningful EKG changes (7).
From a regulatory perspective, both the EMA and FDA have maintained Eliglustat’s cardiovascular safety labeling without revisions, indicating that no significant safety concerns have emerged from post-marketing surveillance or adverse event reporting. This further supports the conclusion that Eliglustat does not present an increased cardiac risk when dosed appropriately. The use of eliglustat in patients with pre-existing cardiac conditions has not been extensively evaluated in clinical trials. However, a pooled analysis of four completed eliglustat trials identified only two cases of serious cardiac arrhythmias—one involving ventricular tachycardia and another atrioventricular (AV) block, which necessitated withdrawal from the trial in one patient. Notably, the AV block was successfully managed with dose adjustment, indicating that appropriate dosing strategies can mitigate such risks. Additionally, 2.8% of participants reported palpitations as an adverse event, though these were transient, self-limiting, and did not require treatment discontinuation (18).
Only a single case of severe bradycardia and hemodynamic compromise has been reported in the literature, attributed to an overdose of 94 capsules of eliglustat—a dose far exceeding the therapeutic range (19). This underscores that, when administered within recommended guidelines, eliglustat maintains a strong cardiac safety profile. Furthermore, careful monitoring for potential drug–drug interactions (DDIs) remains an essential component of safe prescribing, ensuring optimal patient outcomes even in those with underlying cardiac disease. While in vitro studies have demonstrated eliglustat’s inhibitory effects on hERG, Nav1.5, and Cav1.2 channels, these findings must be interpreted in the context of clinically relevant concentrations. Eliglustat’s IC50 values for these channels (hERG: 730 nM; Nav1.5: 11,000 nM; Cav1.2: 25,000 nM) are orders of magnitude higher than those of clinically used channel specific inhibitors like dofetilide (hERG: 7 nM), verapamil (Cav1.2: 100 nM), and flecainide (Nav1.5: 6,700 nM) (20). This suggests that the concentrations required to significantly affect cardiac electrophysiology far exceed those achieved in clinical use.
Moreover, the simultaneous inhibition of multiple ion channels by eliglustat may exert a protective electrophysiological effect rather than a proarrhythmic one. For instance, the combined inhibition of calcium (Cav1.2) and potassium (hERG) currents may create a balancing effect that stabilizes the cardiac action potential plateau, reducing the likelihood of arrhythmias (7). Similarly, inhibition of the late Nav1.5 current could help prevent cellular calcium overload and early afterdepolarizations, both of which are key triggers for torsades de pointes (21). These mechanistic insights reinforce that eliglustat, when used at therapeutic doses, is unlikely to pose a clinically significant proarrhythmic risk.
All patients in our cohort exhibited significant reductions in glucosylsphingosine (GlcSph) levels, accompanied by clinical stabilization or improvement. The notable clinical benefits observed in patients with complex cardiac conditions, including the remission of recurrent pericarditis and improvement in right heart strain associated with severe pulmonary arterial hypertension (PAH), highlight eliglustat’s efficacy in managing GD1 patients with cardiac comorbidities. Certain cardiac complications represent rare but direct manifestations of GD, and their poor response to enzyme replacement therapy (ERT) underscores the limitations of ERT due to inefficient enzyme delivery beyond visceral, hematologic, and skeletal compartments (13, 22). These findings align with previous studies demonstrating eliglustat’s effectiveness in reducing GlcSph levels and improving hematological and visceral parameters in GD1 patients. Furthermore, our results expand the therapeutic scope of eliglustat, showing greater efficacy compared to ERT monotherapy in managing disease burden within sanctuary sites such as the lungs and pericardium, where ERT penetration is limited (14).
Conclusion
Our findings suggest that eliglustat SRT, when dosed according to pharmacogenomic profiling and monitored for drug–drug interactions (DDIs), is both safe and effective in GD1 patients with cardiac comorbidities. This study highlights the critical role of personalized medicine in optimizing outcomes for GD patients with significant cardiac risks. Future prospective studies with larger cohorts are needed to confirm these findings and explore the long-term impact of eliglustat on cardiac health in this population.
Limitations
While this study offers valuable insights, certain limitations must be acknowledged. The small sample size and single-center design restrict the generalizability of the findings to broader GD1 populations. Additionally, the retrospective nature of the study introduces the potential for selection and recall bias, and the absence of a control group limits direct comparative analyses. Despite these constraints, the long follow-up duration (mean 6.4 years) and consistent cardiac monitoring strengthen the reliability and clinical relevance of our observations.
Data availability statement
The original contributions presented in the study are included in the article/Supplementary material, further inquiries can be directed to the corresponding author.
Ethics statement
The studies involving humans were approved by Yale University Institutional Review Board. The studies were conducted in accordance with the local legislation and institutional requirements. The participants provided their written informed consent to participate in this study.
Author contributions
NA: Formal analysis, Methodology, Writing – original draft, Visualization. AS: Formal analysis, Visualization, Writing – original draft, Methodology. AR: Writing – review & editing. RY: Data curation, Writing – review & editing, Resources. AB: Writing – review & editing. PM: Project administration, Supervision, Writing – review & editing, Conceptualization.
Funding
The author(s) declare that financial support was received for the research and/or publication of this article. PKM receives research funding from Sanofi. Noor Ul Ain and Armaan Saith are the recipients of Rare Lysosomal Diseases Fellowship awarded by Sanofi. Sanofi had no role in the study design, data collection, analysis, or interpretation, nor in the writing of this manuscript or the decision to submit it for publication.
Conflict of interest
The authors declare that the research was conducted in the absence of any commercial or financial relationships that could be construed as a potential conflict of interest.
Generative AI statement
The author(s) declare that no Gen AI was used in the creation of this manuscript.
Publisher’s note
All claims expressed in this article are solely those of the authors and do not necessarily represent those of their affiliated organizations, or those of the publisher, the editors and the reviewers. Any product that may be evaluated in this article, or claim that may be made by its manufacturer, is not guaranteed or endorsed by the publisher.
Supplementary material
The Supplementary material for this article can be found online at: https://www.frontiersin.org/articles/10.3389/fmed.2025.1535099/full#supplementary-material
References
1. Baris, HN, Cohen, IJ, and Mistry, PK. Gaucher disease: the metabolic defect, pathophysiology, phenotypes and natural history. Pediatr Endocrinol Rev. (2014) 12:72–81.
2. Stirnemann, J, Belmatoug, N, Camou, F, Serratrice, C, Froissart, R, Caillaud, C, et al. A review of Gaucher disease pathophysiology, clinical presentation and treatments. Int J Mol Sci. (2017) 18:441. doi: 10.3390/ijms18020441
3. Pandey, MK, Burrow, TA, Rani, R, Martin, LJ, Witte, D, Setchell, KD, et al. Complement drives glucosylceramide accumulation and tissue inflammation in Gaucher disease. Nature. (2017) 543:108–12. doi: 10.1038/nature21368
4. Boddupalli, CS, Nair, S, Belinsky, G, Gans, J, Teeple, E, Nguyen, TH, et al. Neuroinflammation in neuronopathic Gaucher disease: role of microglia and NK cells, biomarkers, and response to substrate reduction therapy. eLife. (2022) 11:79830. doi: 10.7554/eLife.79830
5. Cox, TM, Drelichman, G, Cravo, R, Balwani, M, Burrow, TA, Martins, AM, et al. Eliglustat compared with imiglucerase in patients with Gaucher’s disease type 1 stabilised on enzyme replacement therapy: a phase 3, randomised, open-label, non-inferiority trial. Lancet. (2015) 385:2355–62. doi: 10.1016/S0140-6736(14)61841-9
6. Cox, TM, Drelichman, G, Cravo, R, Balwani, M, Burrow, TA, Martins, AM, et al. Eliglustat maintains long-term clinical stability in patients with Gaucher disease type 1 stabilized on enzyme therapy. Blood. (2017) 129:2375–83. doi: 10.1182/blood-2016-12-758409
7. Ruskin, JN, Ortemann-Renon, C, Msihid, J, Ross, L, Puga, AC, Peterschmitt, MJ, et al. How a concentration-effect analysis of data from the eliglustat thorough electrocardiographic study was used to support dosing recommendations. Mol Genet Metab. (2020) 131:211–8. doi: 10.1016/j.ymgme.2020.09.003
8. Mistry, PK, Balwani, M, Charrow, J, Kishnani, P, Niederau, C, and Underhill, LH. Real-world effectiveness of eliglustat in treatment-naïve and switch patients enrolled in the international collaborative Gaucher group Gaucher registry. Am J Hematol. (2020) 95:1038–46. doi: 10.1002/ajh.25875
9. Mistry, PK, Lukina, E, Ben Turkia, H, Amato, D, Baris, H, Dasouki, M, et al. Effect of Oral eliglustat on splenomegaly in patients with Gaucher disease type 1. JAMA. (2015) 313:695–706. doi: 10.1001/jama.2015.459
10. Balwani, M, Burrow, TA, Charrow, J, Goker-Alpan, O, Kaplan, P, Kishnani, PS, et al. Recommendations for the use of eliglustat in the treatment of adults with Gaucher disease type 1 in the United States. Mol Genet Metab. (2016) 117:95–103. doi: 10.1016/j.ymgme.2015.09.002
11. Mistry, PK, Kishnani, PS, Balwani, M, Charrow, JM, Hull, J, Weinreb, NJ, et al. The two substrate reduction therapies for type 1 Gaucher disease are not equivalent. Comment on Hughes et al. switching between enzyme replacement therapies and substrate reduction therapies in patients with Gaucher disease: data from the Gaucher outcome survey (GOS). J Clin Med. (2022) 11:5158. doi: 10.3390/jcm12093269
12. Hughes, DA, Deegan, P, Giraldo, P, Göker-Alpan, Ö, Lau, H, Lukina, E, et al. Switching between enzyme replacement therapies and substrate reduction therapies in patients with Gaucher disease: data from the Gaucher outcome survey (GOS). J Clin Med. (2022) 11:5158. doi: 10.3390/jcm11175158
13. Lo, SM, Liu, J, Chen, F, Pastores, GM, Knowles, J, Boxer, M, et al. Pulmonary vascular disease in Gaucher disease: clinical spectrum, determinants of phenotype and long-term outcomes of therapy. J Inherit Metab Dis. (2011) 34:643–50. doi: 10.1007/s10545-011-9313-9
14. Mistry, PK, Wraight, EP, and Cox, TM. Therapeutic delivery of proteins to macrophages: implications for treatment of Gaucher’s disease. Lancet. (1996) 348:1555–9. doi: 10.1016/S0140-6736(96)04451-0
15. Hughes, D, Mikosch, P, Belmatoug, N, Carubbi, F, Cox, TM, Goker-Alpan, O, et al. Gaucher disease in bone: from pathophysiology to practice. J Bone Miner Res. (2019) 34:996–1013. doi: 10.1002/jbmr.3734
16. Pratt, VM, Cavallari, LH, Del Tredici, AL, Gaedigk, A, Hachad, H, Ji, Y, et al. Recommendations for clinical CYP2D6 genotyping allele selection: a joint consensus recommendation of the Association for Molecular Pathology, College of American Pathologists, Dutch pharmacogenetics working Group of the Royal Dutch Pharmacists Association, and the European Society for Pharmacogenomics and Personalized Therapy. J Mol Diag. (2021) 23:1047–64. doi: 10.1016/j.jmoldx.2021.05.013
17. Murugesan, V, Chuang, W, Liu, J, Lischuk, A, Kacena, K, Lin, H, et al. Glucosylsphingosine is a key biomarker of Gaucher disease. Am J Hematol. (2016) 91:1082–9. doi: 10.1002/ajh.24491
18. Peterschmitt, MJ, Freisens, S, Underhill, LH, Foster, MC, Lewis, G, and Gaemers, SJM. Longterm adverse event profile from four completed trials of oral eliglustat in adults with Gaucher disease type 1. Orphanet J Rare Dis. (2019) 14:128. doi: 10.1186/s13023-019-1085-6
19. Nadler, J, Hermanns-Clausen, M, and Dilger, K. Suicidal attempt with eliglustat overdose. JIMD Rep. (2023) 64:23–6. doi: 10.1002/jmd2.12341
20. Crumb, WJ, Vicente, J, Johannesen, L, and Strauss, DG. An evaluation of 30 clinical drugs against the comprehensive in vitro proarrhythmia assay (CiPA) proposed ion channel panel. J Pharmacol Toxicol Methods. (2016) 81:251–62. doi: 10.1016/j.vascn.2016.03.009
21. Antoons, G, Oros, A, Beekman, JDM, Engelen, MA, Houtman, MJC, Belardinelli, L, et al. Late Na+current inhibition by Ranolazine reduces Torsades de pointes in the chronic atrioventricular block dog model. J Am Coll Cardiol. (2010) 55:801–9. doi: 10.1016/j.jacc.2009.10.033
22. Roberts, WC, and Fredrickson, DS. Gaucher’s disease of the lung causing severe pulmonary hypertension with associated acute recurrent pericarditis. Circulation. (1967) 35:783–9. doi: 10.1161/01.CIR.35.4.783
23. Saith, S. Comparative electrophysiological effects of eliglustat and clinically used channel-specific inhibitors [Figure]. 2024. Created in BioRender. Available from: BioRender.com/u76q381
Keywords: eliglustat, precision medicine, pharmacogenomcis, Gaucher disease, cardiac comorbidities, drug safety
Citation: Ain NU, Saith A, Ruan A, Yang R, Burton A and Mistry PK (2025) Eliglustat and cardiac comorbidities in Gaucher disease: a pharmacogenomic approach to safety and efficacy. Front. Med. 12:1535099. doi: 10.3389/fmed.2025.1535099
Edited by:
Patryk Lipiński, Maria Sklodowska-Curie Medical Academy, PolandReviewed by:
Segundo Mariz, European Medicines Agency, NetherlandsYing Sun, Cincinnati Children’s Hospital Medical Center, United States
Copyright © 2025 Ain, Saith, Ruan, Yang, Burton and Mistry. This is an open-access article distributed under the terms of the Creative Commons Attribution License (CC BY). The use, distribution or reproduction in other forums is permitted, provided the original author(s) and the copyright owner(s) are credited and that the original publication in this journal is cited, in accordance with accepted academic practice. No use, distribution or reproduction is permitted which does not comply with these terms.
*Correspondence: Pramod K. Mistry, cHJhbW9kLm1pc3RyeUB5YWxlLmVkdQ==
†These authors share first authorship