- 1Affiliated Guangdong Hospital of Integrated Traditional Chinese and Western Medicine of Guangzhou University of Chinese Medicine, Guangzhou University of Chinese Medicine, Foshan, Guangdong, China
- 2School of Traditional Chinese Medicine, Jinan University, Guangzhou, Guangdong, China
- 3Department of Scientific Research, Guangdong Provincial Hospital of Integrated Traditional Chinese and Western Medicine, Foshan, Guangdong, China
Metabolic dysfunction-associated steatotic liver disease (MASLD), formerly known as nonalcoholic fatty liver disease, continues to rise with rapid economic development and poses significant challenges to human health. No effective drugs are clinically approved. MASLD is regarded as a multifaceted pathological process encompassing aberrant lipid metabolism, insulin resistance, inflammation, gut microbiota imbalance, apoptosis, fibrosis, and cirrhosis. In recent decades, herbal medicines have gained increasing attention as potential therapeutic agents for the prevention and treatment of MASLD, due to their good tolerance, high efficacy, and low toxicity. In this review, we summarize the pathological mechanisms of MASLD; emphasis is placed on the anti-MASLD mechanisms of Chinese herbal formula (CHF), especially their effects on improving lipid metabolism, inflammation, intestinal flora, and fibrosis. Our goal is to better understand the pharmacological mechanisms of CHF to inform research on the development of new drugs for the treatment of MASLD.
1 Introduction
With the rapid growth of economy and significant changes in lifestyle, metabolic dysfunction-associated steatotic liver disease (MASLD), formerly known as nonalcoholic fatty liver disease (NAFLD), is becoming increasingly prevalent (1). The global prevalence of MASLD is approximately 30% and appears to be increasing (2). MASLD was recently proposed to replace the term “NAFLD” in the Delphi consensus statement (3). MASLD goes beyond the limitations of the previous nomenclature of NAFLD. First, the new nomenclature removes the stigmatizing adjectives “non-alcoholic” and “fatty.” Second, the adjective “metabolic” has been added, which emphasizes the impact of the underlying metabolic pathophysiology of liver disease on cardiac metabolism. Zhou et al. showed that the prevalence and severity of NAFLD and MASLD remain similar (4) and previous relevant literature remains valid (5). MASLD comprises a range of liver damage, from metabolic-dysfunction-associated steatotic liver (MASL—hepatic steatosis without transaminitis) to metabolic-associated steatohepatitis (MASH), liver fibrosis, and ultimately may lead to hepatocellular carcinoma (6). Moreover, the correlation between MASLD and other chronic diseases, such as chronic liver disease, cardiovascular disease, endocrinopathies, and chronic kidney disease, has been reported (7–10). In conclusion, MASLD causes enormous clinical and economic burdens and is one of the leading causes of death.
Currently, there is no officially approved clinical drug for the management of MASLD (11). For one thing, the main treatment methods for MASLD are changing lifestyles, controlling diet, and strengthening exercise to improve liver steatosis and inflammation (12). However, lifestyle modification is hard to achieve weight loss goals and maintain for a long time for most patients. Limitations such as cost, potential side effects, and patient acceptance of invasive bariatric surgery should also be fully considered. For the other thing, numerous drugs have been discarded after unsuccessful clinical trials. A single mechanism has been emphasized in current drug research, while the complex pathophysiology of MASLD has been neglected. Preliminary results of single-drug trials showed that inflammation improved in less than 50% of MASH patients (13). A multitude of novel pharmaceutical agents are undergoing various stages of development but have not yet been formally approved for use, including antidiabetic drugs, peroxisome proliferator-activator receptor modulators, farnesoid X receptor agonists, and fibroblast growth factor analogs (13). Accordingly, the creation of pharmaceuticals for treating MASLD represents a significant unmet medical need. It is necessary and urgent to find economical, safe, and efficient drugs for the treatment of MASLD.
Traditional Chinese medicine has been used for over 2,000 years to treat a wide range of diseases, including those related to the liver. Many traditional Chinese medicines have great potential for preventing and treating MASLD, such as H. erinaceus, which treats a variety of gastrointestinal disorders by modulating intestinal microbiota and improving inflammation (14). CHF contains a wide variety of traditional Chinese medicines, which are a major source of herbal products and natural medicines, and are an important resource for the production of hepatoprotective drugs. CHF contains complex chemical components that can treat diseases through multi-target multi-pathway and multi-level pharmacological activity (15, 16). Therefore, CHF may be a promising candidate for solving the limitations of current single-target drug therapy strategies. In recent years, progress has been made in the development of CHF for MASLD. This review presents an overview of the underlying pathomechanisms of MASLD. Moreover, in light of the latest findings from basic and clinical research, we present a summary of the anti-MASLD mechanisms of CHF, with a particular emphasis on their specific effects on lipid metabolism, intestinal flora, liver inflammation, and fibrosis.
2 The pathogenesis of MASLD
MASLD comprises a range of liver damage, from MASL to MASH, liver fibrosis, and ultimately may lead to hepatocellular carcinoma (6). In order to characterize the pathogenesis of MASLD, the ‘two-hit’ theory and the ‘multiple-hit’ hypothesis have been proposed. The ‘two-hit’ theory proposed that steatosis and oxidative stress play important roles in MASLD progression (17). The ‘multiple-hit’ hypothesis involved richer and more accurate factors, such as abnormal lipid metabolism, insulin resistance (IR), inflammation, gut microbiota imbalance, fibrosis, cirrhosis, and so on (18) (Figure 1).
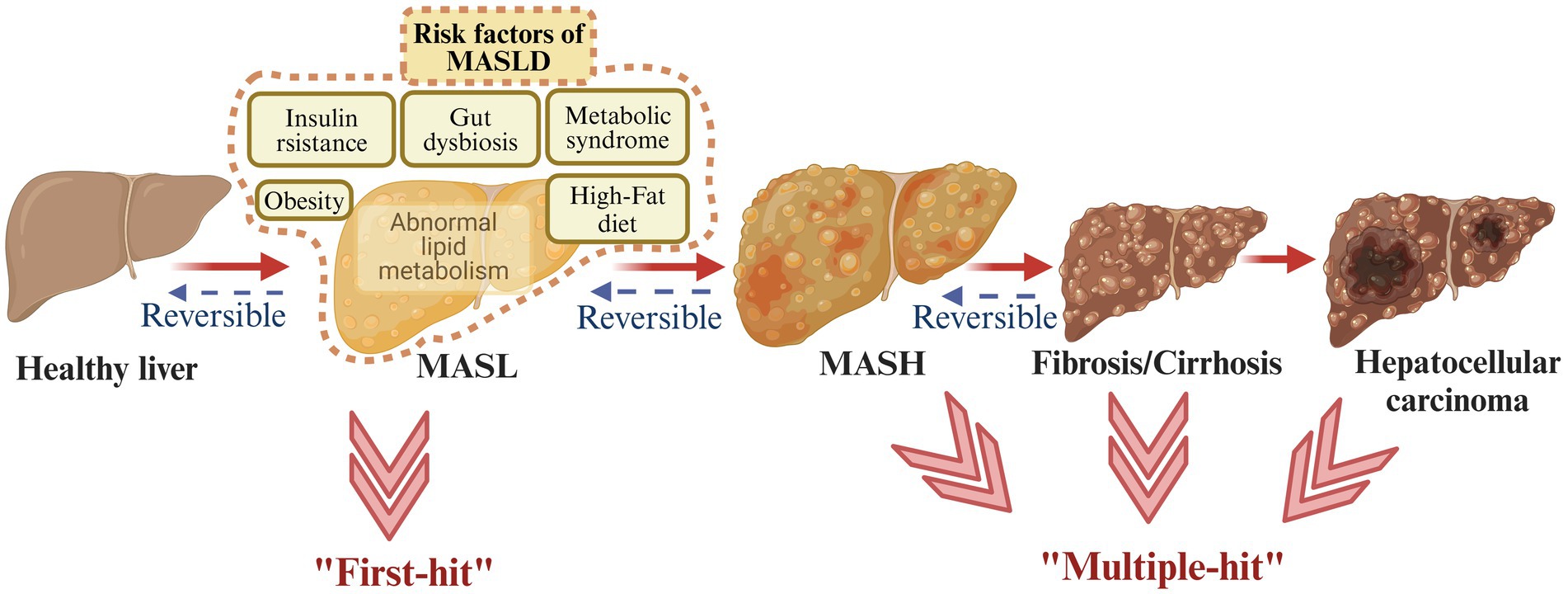
Figure 1. Metabolic dysfunction-associated steatotic liver disease (MASLD) spectrum. MASLD involves four different stages, starting from MASL, the appearance of MASH, the development of fibrosis, and ultimately possibly leading to hepatocellular carcinoma. The ‘two-hit’ theory and the ‘multiple-hit’ hypothesis have been proposed to describe the pathogenesis of MASLD (created with BioRender.com).
2.1 IR and abnormal lipid metabolism
MASLD shares its key risk factor of IR with increasing metabolic problems of type 2 diabetes mellitus, metabolic syndrome, obesity, hypertension, and dyslipidemia (19). On the one hand, IR causes low glucose disposal in adipose and muscle tissue, and further excessive delivery of fatty acids (FAs) to the liver (20). Derived lipogenesis (DNL) represents a metabolic process whereby non-lipids (predominantly carbohydrates) are converted into FAs, which constitute an essential component of lipid metabolism (21). The precise mechanisms underlying DNL in MASLD remain unclear, but sterol regulatory element-binding protein 1c (SREBP-1c) and carbohydrateresponsive element binding protein (ChREBP) have been believed to be coregulators (22). SREBP-1c is activated by insulin and controls enzyme activation in DNL. SREBP1c has been reported to be highly expressed in MASLD patients, which is associated with insulin resistance (23). In hyperglycemic and postprandial states, ChREBP is activated, increasing enzyme activity and gene transcription in the DNL pathway (24). Elevated levels of ChREBP have been demonstrated in the liver biopsies of patients with MASH. Increased ChREBP has beneficial effects on both lipid and glucose metabolism by separating hepatic steatosis from insulin resistance (24). ChREBP also regulates the synthesis of very-low-density lipoprotein (VLDL), which facilitates the transport of triglycerides (TG) in hepatocytes (23). In patients with MASLD, VLDL synthesis and secretion exhibit an increase, yet stabilization occurs when hepatic lipid accumulation exceeds 10% (25). In addition, VLDL-TG molecules in obese individuals are so large that they are unable to penetrate the hepatic vascular sinus and end up in the bloodstream, which is one of the reasons why lipids accumulate in the liver (25). On the other hand, the excessive accumulation in hepatocytes of fat originating from diet leads to abnormal lipid uptake, synthesis, oxidation, and output in the liver (26). With free FAs overloaded, steatosis occurs and lipotoxic mediators comprising diacylglycerols, saturated free FAs, ceramide, and sphingolipids are produced, which further causes endoplasmic reticulum (ER) stress and mitochondrial damage in hepatocytes (27). Meanwhile, the reduction of adiponectin leads to an increase in FAs synthesis and enhancement of mitochondrial β-oxidation (28). Furthermore, IR is always associated with inflammation and lots of immunomodulatory factors, including IL-1, IL-6, TNFα, monocyte chemoattractant protein-1, and the IκB kinase β/nuclear factor-κB (NF-κB) pathway (29, 30). In conclusion, IR is closely associated with steatosis and inflammation which are important factors in the progression of MASLD (Figure 2).
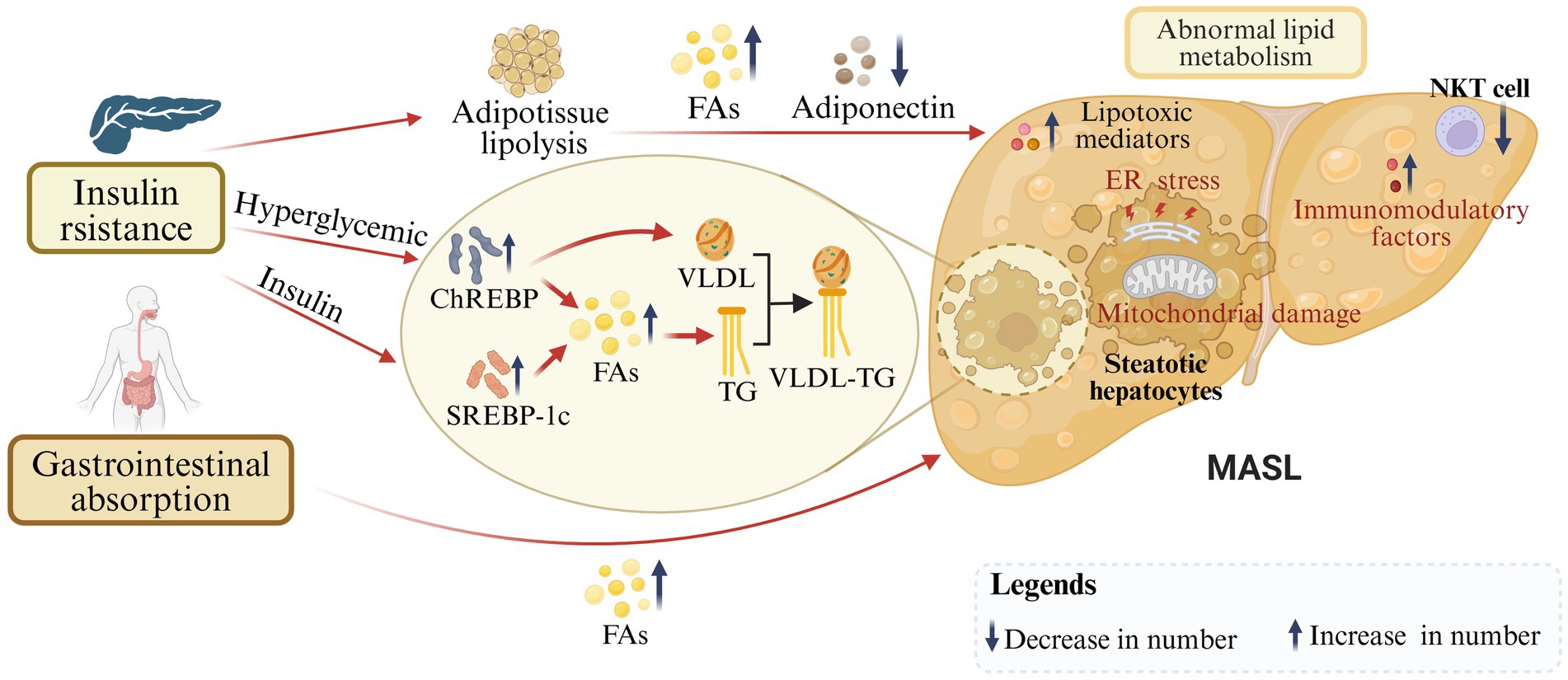
Figure 2. Insulin resistance and abnormal lipid metabolism in the progression of MASLD. On the one hand, under IR conditions, SREBP-1c and ChREBP are activated to further regulate derived adipogenesis and FAs production. On the other hand, dietary-derived FAs are over-accumulated in hepatocytes. In addition, VLDL-TG molecules are oversized, leading to reduced lipid transport. With excessive FAs, production of lipotoxic mediators, and reduction of adiponectin, steatosis, endoplasmic reticulum (ER) stress, mitochondrial damage, and inflammation occur in hepatocytes (created with BioRender.com).
2.2 Immunological mechanisms
Inflammation is a key driver in the progression of MASLD. The immune response is critical for tissue repair, yet excessive immune activation may cause liver tissue damage (Figure 3).
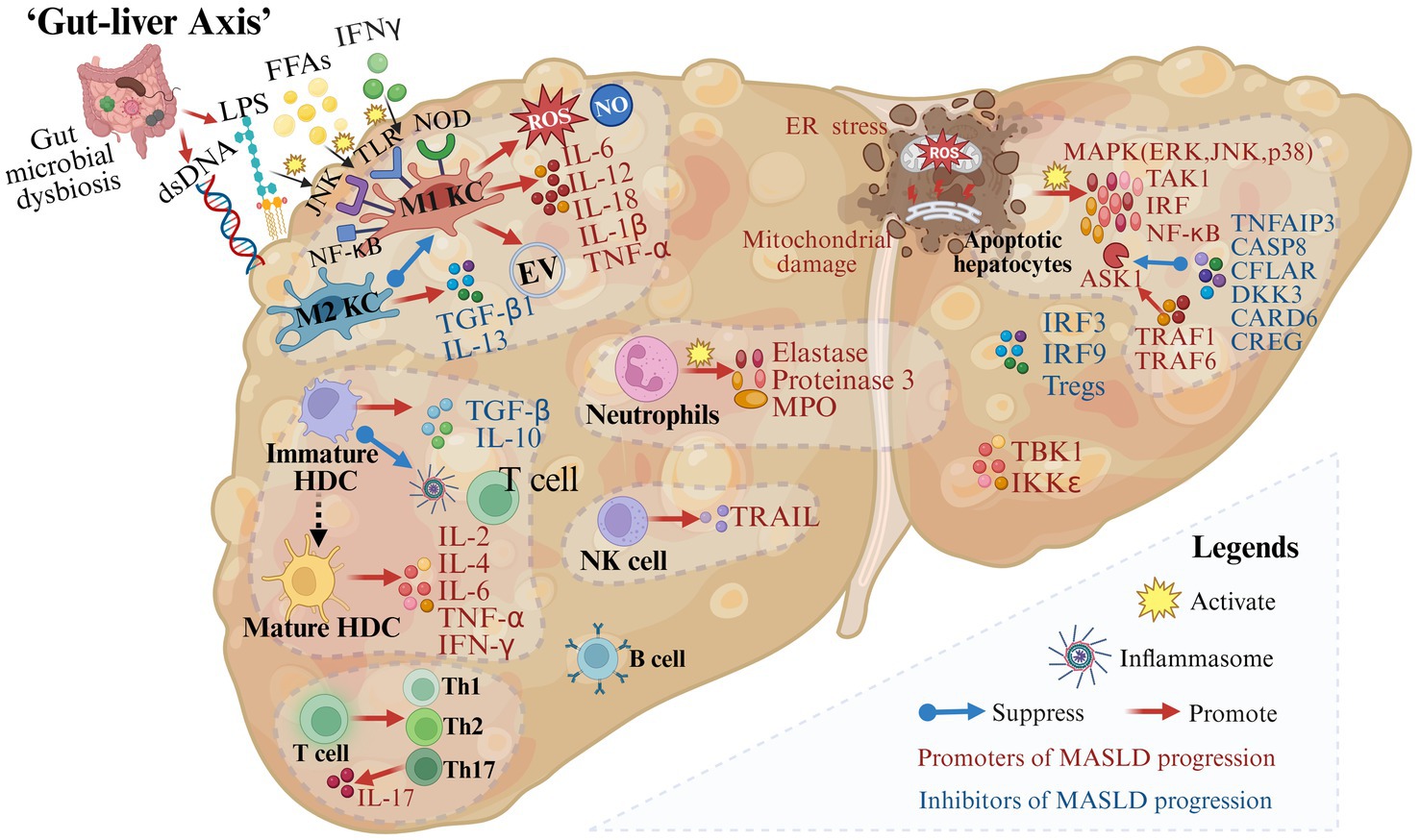
Figure 3. Gut microbial dysbiosis and immune mechanisms in the progression of metabolic dysfunction-associated steatotic liver disease. A variety of immune cells and immune factors are involved in the progression of MASLD. In addition, in the case of impaired intestinal permeability and intestinal microbial disorders, immune cells are activated and produce inflammation-related molecules (created with BioRender.com).
2.2.1 Innate immune system
During MASLD, M1 Kupffer cells (KCs) are induced by mediators such as circulating FFAs, interferon-γ (IFN-γ), and lipopolysaccharide (LPS) through pattern recognition receptors including membrane-bound toll-like receptors (TLRs) and cytoplasmic nucleotide oligomerization domain-like receptors (31–33). Then activated M1 KCs secrete extracellular vesicles and pro-inflammatory cytokines such as IL-6, IL-1β, and TNF-α (34–37). On the contrary, M2 KCs decrease the progression of MASLD by inducing M1 KCs apoptosis and releasing transforming growth factor (TGF)-β1 and IL-13 which can promote liver remodeling and tissue repair (38, 39). Therefore, the balance between M1 KCs and M2 KCs is crucial for liver homeostasis.
Hepatic dendritic cells (HDCs), important immune cells in the liver, play a dual role in MASLD development and progression. In the state of immune tolerance, immature HDCs secrete TGF-β and IL-10, suppress inflammasome activation, restrict T cell expansion, and remove necrotic debris and apoptotic cells to maintain liver homeostasis (40, 41). Conversely, when HDCs are transformed into an active state, their mature forms produce inflammatory cytokines such as IL-2, IL-4, IL-6, TNF-α, and IFN-γ, which further lead to pro-inflammatory responses (41).
Other innate immune cells are also associated with the progression of MASLD. It is reported that neutrophils and factors released from neutrophils including elastase, proteinase 3, and myeloperoxidase are associated with inflammation and fibrosis in MASLD patients (42–44). The number of NKT cells decreases during steatosis and increases in the middle and late stages of the disease. NK cells may promote hepatocyte apoptosis, inflammation, and fibrosis by secreting IFN-γ and TRAIL (45, 46).
In addition, immune factors are indispensable components of the progression of MASLD. Following tissue damage, members of the major mitogen-activated protein kinase (MAPK) families including the extracellular signal-regulated kinase (ERK), Jun N-terminal kinase (JNK), and p38 are activated (47). Besides, upstream and downstream effector kinases of MAPK including TGFβ-activated kinase 1 (TAK1), apoptosis signal-regulating kinase 1 (ASK1), interferon regulatory factors (IRF) and NF-κB are also involved in regulating the inflammatory response in hepatocytes (47). The activation of ASK1, a crucial mechanism in the progression of MASLD, is promoted by tumor necrosis factor receptor-associated factor 1 (TRAF1) (48–50) and TRAF 6 (51), while it is suppressed by deubiquitinase TNFα-induced protein 3 (52), CASP8 and Fadd-like apoptosis regulator (53), dickkopf-3 (54), caspase recruitment domain 6 (55) and cellular repressor of E1A-stimulated genes (56). TANK-binding 1 kinase (57) and IKKε (58) promote hepatocyte steatosis. In contrast, tumor necrosis factor receptor-associated factor 5 has been reported to alleviate hepatic steatosis (20), IRF3 to alleviate IR and reduce hepatic lipid accumulation (59), as well as IRF9 to reduce steatosis and inflammation (60–62).
2.2.2 Adaptive immune system in MASLD
Increased recruitment of CD4+ T cells and CD8+ T cells has been reported in the liver of patients diagnosed with MASH (63–65). Stimulated by inflammation, CD4+ T-cells differentiate into Th1, Th2, and Th17 populations and produce specific cytokines (66). IL-17, one of these cytokines, interferes with the insulin signaling pathway and activates hepatic stellate cells (HSCs), leading to the progression of inflammation and fibrosis (67, 68). Tregs, one of the T-cell subsets, have been reported to have reduced levels in the liver and circulation of MASLD animal models and patients (69). Increasing the number of Tregs reduces liver inflammation and injury in mice (70).
The role of B cells in the pathogenesis of MASLD is not fully understood. IgA has been reported to be associated with hepatocellular inflammation, fibrosis, and carcinoma, and it can predict the progression of advanced liver disease (71–74).
2.3 Gut-liver axis
With impaired intestinal permeability and gut microbial dysbiosis, plentiful gut-derived products (such as LPS and dsDNA) or the microbiota enter the portal circulation and activate immune cells through signaling pathways such as TLR9, TLR4, NF-κB, and JNK (75–77). Activated immune cells promote the secretion of cytokines such as TNF-α, IL-1β, IL-6, IL-12, and IL-18 as well as the production of inflammation-related molecules such as ROS and NO, leading to inflammation in hepatocytes (75–77) (Figure 3). “Fonte Essenziale” water, a bicarbonate-sulfate-calcium–magnesium water, has been shown to produce beneficial effects on the liver-gut axis, modulating gut microbiota and gastrointestinal hormones, and further improving functional gastrointestinal symptoms in patients with MASLD (78, 79).
2.4 Apoptosis and fibrosis
Apoptosis and fibrosis are also important for MASLD progression. Apoptosis occurs throughout the course of MASLD, and it is closely associated with inflammation and fibrosis (80). Hepatocyte damage and death are caused by FFA, lipotoxicity, intestinal microbial products, and inflammasome activation (80). Following hepatocyte injury and apoptosis, major MAPK family members along with their upstream and downstream effector kinases are activated and involved in regulating inflammation (47). Moreover, in MASLD, hepatic stellate cells (HSCs) are the key to the process of fibrogenesis (39). HSCs are activated by LPS through TLR4 which promotes the production and release of cytokines such as TNF-α, IL-6, and IL-8, leading to the activation of signal pathways such as JNK and NF-κB (81). In addition, myeloperoxidase and neutrophils can also lead to the activation of HSCs (44, 82). Activated HSCs are converted to myofibroblasts (83). Unregulated structural remodeling and fibrogenesis may lead to cirrhosis and hepatocellular carcinoma (84) (Figure 4).
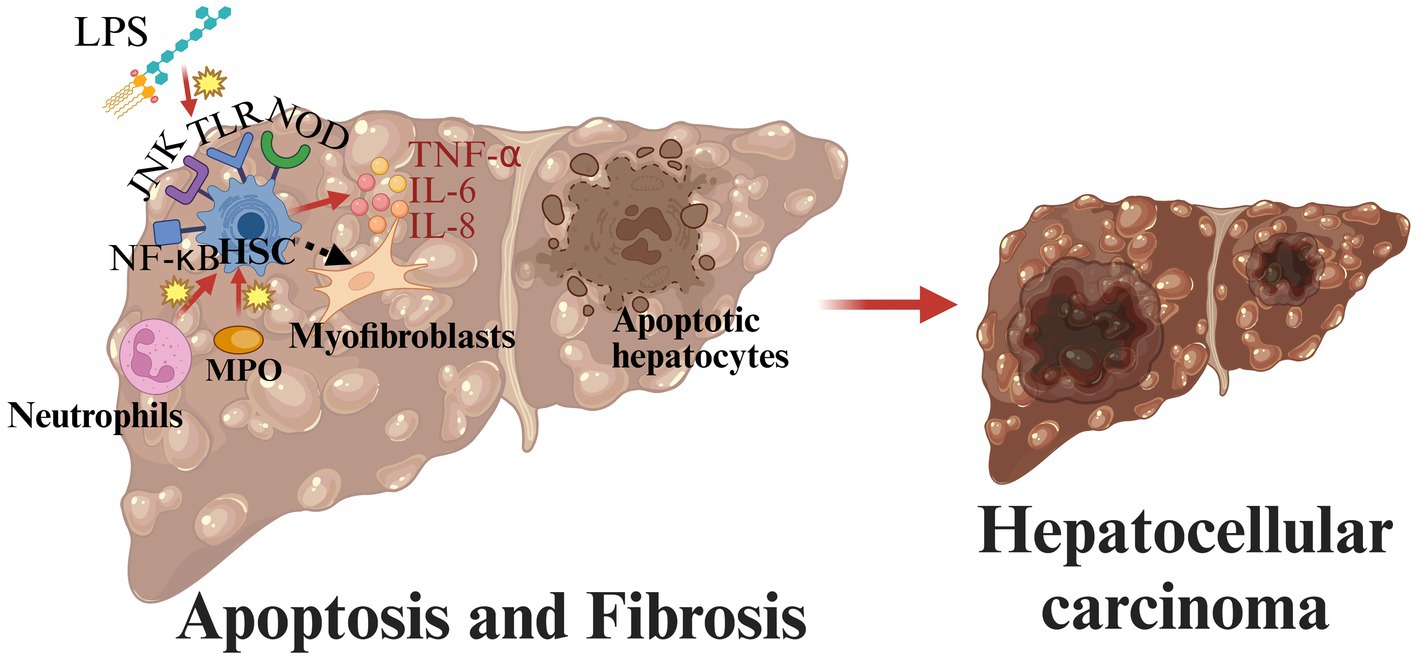
Figure 4. Pathogenesis of MASLD from apoptosis and fibrosis to hepatocellular carcinoma. In MASLD, hepatic stellate cells (HSCs) are the key to the process of fibrogenesis. Activated HSCs are converted to myofibroblasts. Unregulated structural remodeling and fibrogenesis may lead to cirrhosis and hepatocellular carcinoma (created with BioRender.com).
3 The mechanisms of CHF for treating MASLD
CHF has been utilized for the treatment of liver disease for over two millennia, making a significant contribution to the field of healthcare. Although considerable progress has been made in the study of the pathogenesis of MASLD, no effective drugs have been approved for clinical use. Fortunately, CHF may be a potential treasure trove for the treatment of MASLD. To explore the mechanism of the therapeutic effect of CHF on MASLD, many animal experiments have been carried out. We list recent promising CHFs for the treatment of MASLD and their therapeutic mechanisms (Table 1).
3.1 Improving lipid metabolism
The ‘Pandora’s Box’ of MASLD is opened by abnormal lipid metabolism and excessive transportation of FFAs to the liver. Many CHFs can treat MASLD by regulating lipid metabolism. Zexie–Baizhu Decoction (85) activates AMPK and Sirt1 pathway, and then improves lipid metabolism by suppressing gluconeogenesis, inhibiting lipogenesis, activating fatty utilization, promoting FAs oxidation, prompting autophagy, and increasing bile acid metabolism. Si-Ni-San (86) reduces Yes-associated protein 1 in hepatocytes, further reducing lipid droplet deposition. Livsooth authentic herbal formula (87) regulates glucose and lipid metabolism in high fat diet-fed induced mice by promoting β-oxidation, increasing AMPK/ACC activation, and downgrading FAS and SREBP1.
3.2 Ameliorating inflammation
Inflammation within the liver is an important driver of MASLD development. Many CHFs can both improve lipid metabolism and inflammation by acting on different targets. Wang et al. found that compared with the negative control group, the positive therapeutic effect of Danshao Shugan Granule (88) on hepatic steatosis and inflammation in MASLD rats was related to the decrease of NF-κB expression, the reduction of malondialdehyde, and the increase of superoxide dismutase activity. Hedansanqi Tiaozhi Tang (89) exerts lipolysis-promoting and liver-protecting effects by enhancing the nuclear factor-erythroid 2-related factor 2 (Nrf2)/Heme oxygenase 1 antioxidant pathway in hepatocytes and the antioxidant activity of 3T3-L1 adipocytes. Si-Wei-Qing-Gan-Tang (90) ameliorates MASH in rat models by activating autophagy and downregulating NF-κB through p38 MAPK and ERK1/2 signals. Chaihu Shugan powder (91) can reduce FAs synthesis, improve hepatic surface microcirculation disorders, and ultimately reduce transaminase and serum lipid levels by reversing the high expression of 15 miRNAs. Quzhi formula (92) is involved in multi-anti-MASH mechanisms, including inhibition of ER stress, lipid accumulation, and inflammation through Bip/eIF2α Signaling. Yinchenhao Tang (93) has been demonstrated to markedly improve lipid metabolism, reduce body weight, and diminish AST and ALT levels in mice, which is associated with increased NR1H4 and APOA1 expression. The Scutellaria-coptis herb couple (94) alleviates MASH by inhibiting lipotoxicity, inflammation, and oxidative stress via activating the NRF2 and FXR Signaling. The protective effect of Shuangyu Tiaozhi decoction (95) against MASLD is based on the molecular mechanism of the relative mRNA and protein levels of ESR1, p-GSK-3β, mTOR, FASN, HIF-1α, and VEGFA, which ameliorate lipid deposition, inflammation, and IR. The Lingguizhugan decoction (96) alleviates hepatic lipid deposition by suppressing STING-TBK1-NF-κB single in hepatic macrophages.
3.3 Modulating gut microbiota
Dysregulated gut microflora can activate immune cells and promote the progression of MASL to hepatitis and fibrosis. Some CHFs target the ‘liver-gut axis’ to regulate gut microbiota and reduce intestinal mucosal damage to curb the development of MASLD. Jiang Zhi Granule (97) protects intestinal mucosal immune barrier in MASH rats by inhibiting the TLR-4/MyD88 signaling pathway. Si Miao Formula inhibits the expression of inflammatory factors, suppresses the production and transport of FAs (98, 99), and modulates the composition of the gut microbiota, especially increasing the abundance of Akkermansia muciniphila (99). The results also indicate that compared to fenofibrate, Si Miao Formula (99) has a more significant effect on TG reduction. Erchen Decoction (100) and Tianhuang formula (101) are also utilized to treat MASLD by modulating intestinal microbial flora diversity.
3.4 Alleviating fibrosis
The occurrence of fibrosis plays a sufficient role in the progression of MASLD. Chu et al. predicted by transcriptome-based multi-scale network pharmacology logical platform and then proved through animal experiments that Qing-Zhi-Tiao-Gan-Tang (102) inhibits the expression of fibrosis genes (such as TGF-β, Col1a1), reduces the levels of inflammatory factors (such as IL-1β, IL-6, and Tnf-α), and improves the ‘steroid biosynthesis’, ‘bile secretion’ and ‘FAs degradation’ pathways. Qushihuayu formula (103) may exert hepatoprotective effects by enhancing the reprogramming of HSCs, promoting the translocation of p-p65 and PPAR-γ to the nucleus, inhibiting the phosphorylation of MAPK pathway, and further alleviating steatosis and fibrosis. Pien Tze Huang (104) holds a beneficial role in steatosis, inflammation, and fibrosis of MASLD by inhibiting the NF-κB pathway and the degradation of inhibitor of κBα.
In conclusion, it has been demonstrated that CHF, which contains complex chemical components, plays a beneficial role in improving lipid metabolism, ameliorating inflammation, modulating gut microbiota, and inhibiting hepatic fibrosis through multi-target, multi-pathway, and multi-level pharmacological mechanisms. Therefore, CHF may be a promising candidate to overcome the limitations of the current single-target drug treatment strategy.
4 Clinical practices of CHF on MASLD
A number of scholars have conducted clinical trials to explore the clinical effectiveness and safety of CHF in MASLD, and they have confirmed the multiple therapeutic effects and clinical application potential of CHF. Randomized controlled trials (RCTs) comparing CHFs with placebo or other pharmaceutical agents have been explored and the results are encouraging (Table 2).
4.1 Improving imaging findings in patients with MASLD
Several studies have found that ultrasound performed on patients with MASLD who took CHF showed a reduction in hepatic steatosis. In one RCT enrolling 260 patients with MASLD, Wang et al. showed that Danshao Shugan Granule (88) most significantly improved ultrasound finding and reduced the levels of triglyceride, total cholesterol, γ-glutamyl transpeptidase, and aspartate transaminase compared with silibinin and rosiglitazone. Another trial of Qinjiang Baoling Decoction (105) also demonstrated that the CHF had long-lasting efficacy in improving the scores of liver/spleen CT ratio and abdomen B-mode ultrasound.
Some scholars have utilized Fibrotouch to assess fatty liver grading and have found that CHF had superior therapeutic effects in MASLD. The result of one RCT showed better clinical improvement in patients with MASLD taking Lanzhang Granules (106) compared to placebo. Lanzhang Granules can effectively reduce the controlled attenuation parameter (CAP) of patients with a favorable safety profile, and significantly alleviate clinical symptoms such as right hypochondrial pain, fatigue, anorexia, and nausea. Hui et al. reached similar conclusions in their study of spleen-strengthening and liver-draining herbal formula (107), which was additionally found to reduce liver stiffness measurement (LSM) and modulate intestinal flora.
In addition, the favorable therapeutic efficacy of CHF on MASLD has been demonstrated not only by B-ultrasound but also by Fibroscan in several clinical trials. Compared with polyene phosphatidylcholine capsules, Shugan Xiaozhi Decoction (108) can better improve liver stiffness, CAP, fasting blood glucose, BMI, TG, total cholesterol (TC), aspartate aminotransferase (AST), and alanine aminotransferase (ALT). Similar conclusions were reached in the study on Qinghua Decoction by Lu et al. (109).
4.2 Improvement of blood tests in patients with MASLD
During the trial, some CHFs did not affect the degree of hepatic steatosis in patients with MASLD, which may be related to the relatively short intervention time. However, these CHFs improved the lipid profile and hepatic function. A multicenter, double-blind, randomized controlled clinical trial revealed that Qingre Huashi Formula (110) significantly reduced, alkaline phosphatase and body mass index (BMI) levels and improved symptoms such as bitter mouth and incomplete defecation. Long et al. found that a combination of Xiaopi Huatan Granule (111) was more effective in lowering AST, ALT, TG, TC, and BMI in patients with MASLD compared to silymarin capsules. A clinical study on Zhibitai Capsule (112) found similar results. In addition, the study also found that the treatment resulted in significant reductions in enterobacteriaceae, enterococci, staphylococcus, diamine oxidase, serum endotoxin, procalcitonin, TNF-α, IL-6, and HOMA-IR and significant increases in lactobacillus, bifidobacteria, and bacteroidetes. All the indexes of the treatment group (Zibitai capsule combined with polyene phosphatidylcholine capsule) were better than those of the control group (polyene phosphatidylcholine capsule). Besides, Lingguizhugan Decoction (113) improved insulin resistance in overweight/obese patients with MASLD by increasing the levels of DNA N6-methyladenine modification of protein phosphatase 1 regulatory subunit 3A (PPP1R3A) and autophagy related 3 (ATG3).
5 Conclusions and perspectives
In light of the growing prevalence of metabolic diseases, there is a heightened awareness of MASLD and a pressing need for the development of efficacious anti-MASLD drugs. However, currently, no significant therapeutic drugs with a notable curative effect are available. The pathogenesis of MASLD is complex, encompassing a sophisticated interplay of IR, abnormalities in lipid metabolism, inflammation, disturbances in gut flora, apoptosis, and fibrosis. CHFs are rich in natural ingredients and exhibit diverse biological effects in treating many diseases. Importantly, mechanistic studies and clinical trial results suggest that many CHFs have the potential to provide beneficial outcomes in the treatment of MASLD by improving lipid metabolism, inhibiting inflammatory pathways, modulating gut microbiota, and alleviating fibrosis (Figure 5). Mechanically, they primarily reverse the MASLD progression through multi-target regulation of multiple signal pathways and immune factors. In particular, NF-κB, AMPK, FASN, IL-1β, IL-6, Tnf-α, and Nrf2 are identified as key molecular targets for the improvement MASLD through CHF.
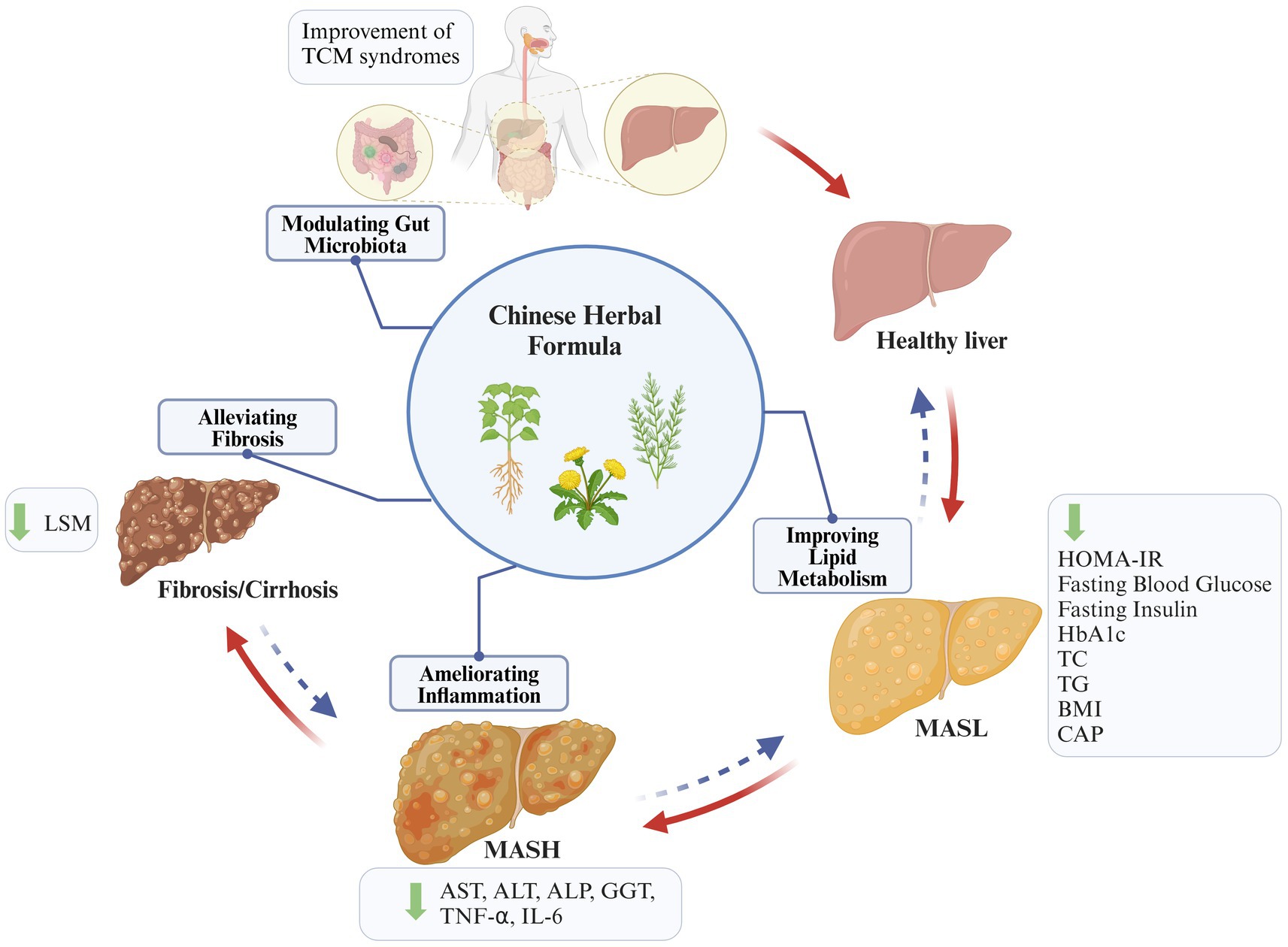
Figure 5. Summary of key mechanisms and events of CHF treating MASLD. CHF plays a beneficial role in inhibiting the progression of MASLD by improving lipid metabolism, ameliorating inflammation, modulating gut microbiota, and inhibiting fibrosis (created with BioRender.com).
The therapeutic efficacy of CHF in MASLD has been widely explored, but toxicological studies are lacking and safety assessment has not been emphasized. To date, the results of clinical trials are limited and tend to show subtle effects compared to cellular and animal models. Furthermore, the relatively modest sample sizes and dearth of liver biopsy data in the majority of clinical studies constrain the scope for deriving robust evidence from clinical trials regarding efficacious strategies for the prevention and treatment of MASLD. The diversity of CHF sources and their chemically active components, as well as the ample anti-MASLD mechanisms, reinforce our confidence and motivation to discover new anti-MASLD drugs. Nevertheless, the screening of CHF and the associated multicenter, more rigorous, and larger-sample RCTs are imminent.
Author contributions
S-HT: Investigation, Resources, Writing – original draft. Y-QL: Writing – review & editing. Y-MT: Writing – review & editing. Y-BY: Writing – review & editing. W-NX: Funding acquisition, Supervision, Writing – review & editing.
Funding
The author(s) declare that financial support was received for the research, authorship, and/or publication of this article. This work was financially supported by the Guangdong Basic and Applied Basic Research Fund Project (No. 2023A1515140125).
Acknowledgments
We are grateful to those who participated in this study. Furthermore, Figures 1–5 were created with BioRender.com (https://www.biorender.com/).
Conflict of interest
The authors declare that the research was conducted in the absence of any commercial or financial relationships that could be construed as a potential conflict of interest.
Publisher’s note
All claims expressed in this article are solely those of the authors and do not necessarily represent those of their affiliated organizations, or those of the publisher, the editors and the reviewers. Any product that may be evaluated in this article, or claim that may be made by its manufacturer, is not guaranteed or endorsed by the publisher.
References
1. Sayiner, M, Koenig, A, Henry, L, and Younossi, ZM. Epidemiology of nonalcoholic fatty liver disease and nonalcoholic steatohepatitis in the United States and the rest of the world. Clin Liver Dis. (2016) 20:205–14. doi: 10.1016/j.cld.2015.10.001
2. Le, MH, Le, DM, Baez, TC, Wu, Y, Ito, T, Lee, EY, et al. Global incidence of non-alcoholic fatty liver disease: a systematic review and meta-analysis of 63 studies and 1,201,807 persons. J Hepatol. (2023) 79:287–95. doi: 10.1016/j.jhep.2023.03.040
3. Lazarus, JV, Newsome, PN, Francque, SM, Kanwal, F, Terrault, NA, and Rinella, ME. Reply: a multi-society Delphi consensus statement on new fatty liver disease nomenclature. Hepatology. (2024) 79:E93–4. doi: 10.1097/hep.0000000000000696
4. Zou, H, Ma, X, Pan, W, and Xie, Y. Comparing similarities and differences between Nafld, Mafld, and Masld in the general U.S. population. Front Nutr. (2024) 11:1411802. doi: 10.3389/fnut.2024.1411802
5. Mary, ER, Graciela, C-N, Aleksander, K, Norah, AT, and Philip, NN. Reply: ‘From Nafld to Masld: promise and pitfalls of a new definition’†. Hepatology. (2023) 29:101179. doi: 10.1097/hep.0000000000000705
6. Sanyal, AJ. Past, present and future perspectives in nonalcoholic fatty liver disease. Nat Rev Gastroenterol Hepatol. (2019) 16:377–86. doi: 10.1038/s41575-019-0144-8
7. Muñoz, M, Sánchez, A, Pilar Martínez, M, Benedito, S, López-Oliva, ME, García-Sacristán, A, et al. Cox-2 is involved in vascular oxidative stress and endothelial dysfunction of renal interlobar arteries from obese Zucker rats. Free Radic Biol Med. (2015) 84:77–90. doi: 10.1016/j.freeradbiomed.2015.03.024
8. Nobili, V, Svegliati-Baroni, G, Alisi, A, Miele, L, Valenti, L, and Vajro, P. A 360-degree overview of paediatric Nafld: recent insights. J Hepatol. (2013) 58:1218–29. doi: 10.1016/j.jhep.2012.12.003
9. Pawlak, M, Lefebvre, P, and Staels, B. Molecular mechanism of Pparα action and its impact on lipid metabolism, inflammation and fibrosis in non-alcoholic fatty liver disease. J Hepatol. (2015) 62:720–33. doi: 10.1016/j.jhep.2014.10.039
10. Popov, VB, and Lim, JK. Treatment of nonalcoholic fatty liver disease: the role of medical, surgical, and endoscopic weight loss. J Clin Transl Hepatol. (2015) 3:230–8. doi: 10.14218/jcth.2015.00019
11. Zheng, X, Zhang, X-G, Liu, Y, Zhu, L-P, Liang, X-S, Jiang, H, et al. Arjunolic acid from Cyclocarya Paliurus ameliorates nonalcoholic fatty liver disease in mice via activating Sirt1/Ampk, triggering autophagy and improving gut barrier function. J Funct Foods. (2021) 86:104686. doi: 10.1016/j.jff.2021.104686
12. Younossi, ZM, Zelber-Sagi, S, Henry, L, and Gerber, LH. Lifestyle interventions in nonalcoholic fatty liver disease. Nat Rev Gastroenterol Hepatol. (2023) 20:708–22. doi: 10.1038/s41575-023-00800-4
13. Sangro, P, de la Torre, AM, Sangro, B, and D'Avola, D. Metabolic dysfunction-associated fatty liver disease (Mafld): an update of the recent advances in pharmacological treatment. J Physiol Biochem. (2023) 79:869–79. doi: 10.1007/s13105-023-00954-4
14. Gravina, AG, Pellegrino, R, Auletta, S, Palladino, G, Brandimarte, G, D'Onofrio, R, et al. Hericium Erinaceus, a medicinal fungus with a centuries-old history: evidence in gastrointestinal diseases. World J Gastroenterol. (2023) 29:3048–65. doi: 10.3748/wjg.v29.i20.3048
15. Deng, X, Zhang, Y, Jiang, F, Chen, R, Peng, P, Wen, B, et al. The Chinese herb-derived Sparstolonin B suppresses Hiv-1 transcription. Virol J. (2015) 12:108. doi: 10.1186/s12985-015-0339-8
16. Li, Y, Wang, X, He, H, Zhang, D, Jiang, Y, Yang, X, et al. Steroidal Saponins from the roots and rhizomes of Tupistra Chinensis. Molecules. (2015) 20:13659–69. doi: 10.3390/molecules200813659
17. Day, CP, and James, OFW. Hepatic steatosis: innocent bystander or guilty party? Hepatology. (1998) 27:1463–6. doi: 10.1002/hep.510270601
18. Tilg, H, and Moschen, AR. Evolution of inflammation in nonalcoholic fatty liver disease: the multiple parallel hits hypothesis. Hepatology. (2010) 52:1836–46. doi: 10.1002/hep.24001
19. Driessen, S, Francque, SM, Anker, SD, Castro Cabezas, M, Grobbee, DE, Tushuizen, ME, et al. Metabolic dysfunction-associated steatotic liver disease and the heart. Hepatology. (2023) 10.1097/hep.0000000000000735. doi: 10.1097/hep.0000000000000735
20. Gao, L, Wang, P-X, Zhang, Y, Yu, C-J, Ji, Y, Wang, X, et al. Tumor necrosis factor receptor-associated factor 5 (Traf5) acts as an essential negative regulator of hepatic steatosis. J Hepatol. (2016) 65:125–36. doi: 10.1016/j.jhep.2016.03.006
21. Softic, S, Cohen, DE, and Kahn, CR. Role of dietary fructose and hepatic De novo lipogenesis in fatty liver disease. Dig Dis Sci. (2016) 61:1282–93. doi: 10.1007/s10620-016-4054-0
22. Smith, GI, Shankaran, M, Yoshino, M, Schweitzer, GG, Chondronikola, M, Beals, JW, et al. Insulin resistance drives hepatic De novo lipogenesis in nonalcoholic fatty liver disease. J Clin Invest. (2020) 130:1453–60. doi: 10.1172/jci134165
23. Rao, G, Peng, X, Li, X, An, K, He, H, Fu, X, et al. Unmasking the enigma of lipid metabolism in metabolic dysfunction-associated steatotic liver disease: from mechanism to the clinic. Front Med. (2023) 10:1294267. doi: 10.3389/fmed.2023.1294267
24. Syed-Abdul, MM. Lipid metabolism in metabolic-associated steatotic liver disease (Masld). Meta. (2023) 14:12. doi: 10.3390/metabo14010012
25. Fabbrini, E, Mohammed, BS, Magkos, F, Korenblat, KM, Patterson, BW, and Klein, S. Alterations in adipose tissue and hepatic lipid kinetics in obese men and women with nonalcoholic fatty liver disease. Gastroenterology. (2008) 134:424–31. doi: 10.1053/j.gastro.2007.11.038
26. Hardy, T, Oakley, F, Anstee, QM, and Day, CP. Nonalcoholic fatty liver disease: pathogenesis and disease Spectrum. Ann Rev Pathol Mech Dis. (2016) 11:451–96. doi: 10.1146/annurev-pathol-012615-044224
27. Farrell, GC, Haczeyni, F, and Chitturi, S. Pathogenesis of Nash: how metabolic complications of overnutrition favour lipotoxicity and pro-inflammatory fatty liver disease. Obesity Fatty Liver Liver Cancer. (2018) 1061:19–44. doi: 10.1007/978-981-10-8684-7_3
28. Khan, RS, Bril, F, Cusi, K, and Newsome, PN. Modulation of insulin resistance in nonalcoholic fatty liver disease. Hepatology. (2019) 70:711–24. doi: 10.1002/hep.30429
29. Glass, CK, and Olefsky, JM. Inflammation and lipid signaling in the etiology of insulin resistance. Cell Metab. (2012) 15:635–45. doi: 10.1016/j.cmet.2012.04.001
30. Baeck, C, Wehr, A, Karlmark, KR, Heymann, F, Vucur, M, Gassler, N, et al. Pharmacological inhibition of the chemokine Ccl2 (Mcp-1) diminishes liver macrophage infiltration and steatohepatitis in chronic hepatic injury. Gut. (2012) 61:416–26. doi: 10.1136/gutjnl-2011-300304
31. Kazankov, K, Jørgensen, SMD, Thomsen, KL, Møller, HJ, Vilstrup, H, George, J, et al. The role of macrophages in nonalcoholic fatty liver disease and nonalcoholic steatohepatitis. Nat Rev Gastroenterol Hepatol. (2019) 16:145–59. doi: 10.1038/s41575-018-0082-x
32. Xu, L, Liu, W, Bai, F, Xu, Y, Liang, X, Ma, C, et al. Hepatic macrophage as a key player in fatty liver disease. Front Immunol. (2021) 12:708978. doi: 10.3389/fimmu.2021.708978
33. Arrese, M, Cabrera, D, Kalergis, AM, and Feldstein, AE. Innate immunity and inflammation in Nafld/Nash. Dig Dis Sci. (2016) 61:1294–303. doi: 10.1007/s10620-016-4049-x
34. Mantovani, A, Biswas, SK, Galdiero, MR, Sica, A, and Locati, M. Macrophage plasticity and polarization in tissue repair and remodelling. J Pathol. (2013) 229:176–85. doi: 10.1002/path.4133
35. Xiao, Z, Liu, M, Yang, F, Liu, G, Liu, J, Zhao, W, et al. Programmed cell death and lipid metabolism of macrophages in Nafld. Front Immunol. (2023) 14:1118449. doi: 10.3389/fimmu.2023.1118449
36. Srinivas, AN, Suresh, D, Santhekadur, PK, Suvarna, D, and Kumar, DP. Extracellular vesicles as inflammatory drivers in Nafld. Front Immunol. (2020) 11:627424. doi: 10.3389/fimmu.2020.627424
37. Dorairaj, V, Sulaiman, SA, Abu, N, and Abdul Murad, NA. Extracellular vesicles in the development of the non-alcoholic fatty liver disease: an update. Biomol Ther. (2020) 10:1494. doi: 10.3390/biom10111494
38. Wan, J, Benkdane, M, Teixeira-Clerc, F, Bonnafous, S, Louvet, A, Lafdil, F, et al. M2 Kupffer cells promote M1 Kupffer cell apoptosis: a protective mechanism against alcoholic and nonalcoholic fatty liver disease. Hepatology. (2014) 59:130–42. doi: 10.1002/hep.26607
39. Schuppan, D, Surabattula, R, and Wang, XY. Determinants of fibrosis progression and regression in Nash. J Hepatol. (2018) 68:238–50. doi: 10.1016/j.jhep.2017.11.012
40. Méndez-Sánchez, N, Córdova-Gallardo, J, Barranco-Fragoso, B, and Eslam, M. Hepatic dendritic cells in the development and progression of metabolic steatohepatitis. Front Immunol. (2021) 12:641240. doi: 10.3389/fimmu.2021.641240
41. Henning, JR, Graffeo, CS, Rehman, A, Fallon, NC, Zambirinis, CP, Ochi, A, et al. Dendritic cells limit fibroinflammatory injury in nonalcoholic steatohepatitis in mice. Hepatology. (2013) 58:589–602. doi: 10.1002/hep.26267
42. Mirea, A-M, Toonen, EJM, van den Munckhof, I, Munsterman, ID, Tjwa, ETTL, Jaeger, M, et al. Increased proteinase 3 and neutrophil elastase plasma concentrations are associated with non-alcoholic fatty liver disease (Nafld) and type 2 diabetes. Mol Med. (2019) 25:1–10. doi: 10.1186/s10020-019-0084-3
43. Alkhouri, N, Li, L, Hanouneh, I, Feldstein, A, and Hazen, S. Plasma myeloperoxidase levels correlate with the presence of fibrosis in patients with nonalcoholic fatty liver disease: 433. Off J Am Coll Gastroenterol ACG. (2012) 107:S181. doi: 10.14309/00000434-201210001-00433
44. Pulli, B, Ali, M, Iwamoto, Y, Zeller, MWG, Schob, S, Linnoila, JJ, et al. Myeloperoxidase–hepatocyte–stellate cell cross talk promotes hepatocyte injury and fibrosis in experimental nonalcoholic steatohepatitis. Antioxid Redox Signal. (2015) 23:1255–69. doi: 10.1089/ars.2014.6108
45. Moayedfard, Z, Sani, F, Alizadeh, A, Bagheri Lankarani, K, Zarei, M, and Azarpira, N. The role of the immune system in the pathogenesis of Nafld and potential therapeutic impacts of mesenchymal stem cell-derived extracellular vesicles. Stem Cell Res Ther. (2022) 13:242. doi: 10.1186/s13287-022-02929-6
46. Kahraman, A, Fingas, CD, Syn, WK, Gerken, G, and Canbay, A. Role of stress-induced Nkg2d ligands in liver diseases. Liver Int. (2012) 32:370–82. doi: 10.1111/j.1478-3231.2011.02608.x
47. Arthur, JSC, and Ley, SC. Mitogen-activated protein kinases in innate immunity. Nat Rev Immunol. (2013) 13:679–92. doi: 10.1038/nri3495
48. Lu, Y-Y, Li, Z-Z, Jiang, D-S, Wang, L, Zhang, Y, Chen, K, et al. TRAF1 is a critical regulator of cerebral ischaemia–reperfusion injury and neuronal death. Nat Commun. (2013) 4:2852. doi: 10.1038/ncomms3852
49. Xiang, M, Wang, P-X, Wang, A-B, Zhang, X-J, Zhang, Y, Zhang, P, et al. Targeting hepatic Traf1-Ask1 signaling to improve inflammation, insulin resistance, and hepatic steatosis. J Hepatol. (2016) 64:1365–77. doi: 10.1016/j.jhep.2016.02.002
50. Zhang, XF, Zhang, R, Huang, L, Wang, PX, Zhang, Y, Jiang, DS, et al. Traf1 is a key mediator for hepatic ischemia/reperfusion injury. Cell Death Dis. (2014) 5:e1467. doi: 10.1038/cddis.2014.411
51. Ji, Y-X, Zhang, P, Zhang, X-J, Zhao, Y-C, Deng, K-Q, Jiang, X, et al. The ubiquitin E3 ligase Traf6 exacerbates pathological cardiac hypertrophy via Tak1-dependent Signalling. Nat Commun. (2016) 7:11267. doi: 10.1038/ncomms11267
52. Zhang, P, Wang, P-X, Zhao, L-P, Zhang, X, Ji, Y-X, Zhang, X-J, et al. The deubiquitinating enzyme Tnfaip3 mediates inactivation of hepatic Ask1 and ameliorates nonalcoholic steatohepatitis. Nat Med. (2018) 24:84–94. doi: 10.1038/nm.4453
53. Wang, P-X, Ji, Y-X, Zhang, X-J, Zhao, L-P, Yan, Z-Z, Zhang, P, et al. Targeting Casp8 and Fadd-like apoptosis regulator ameliorates nonalcoholic steatohepatitis in mice and nonhuman primates. Nat Med. (2017) 23:439–49. doi: 10.1038/nm.4290
54. Xie, L, Wang, P-X, Zhang, P, Zhang, X-J, Zhao, G-N, Wang, A, et al. Dkk3 expression in hepatocytes defines susceptibility to liver steatosis and obesity. J Hepatol. (2016) 65:113–24. doi: 10.1016/j.jhep.2016.03.008
55. Qin, J-J, Mao, W, Wang, X, Sun, P, Cheng, D, Tian, S, et al. Caspase recruitment domain 6 protects against hepatic ischemia/reperfusion injury by suppressing Ask1. J Hepatol. (2018) 69:1110–22. doi: 10.1016/j.jhep.2018.06.014
56. Zhang, QY, Zhao, LP, Tian, XX, Yan, CH, Li, Y, Liu, YX, et al. The novel intracellular protein Creg inhibits hepatic steatosis, obesity, and insulin resistance. Hepatology. (2017) 66:834–54. doi: 10.1002/hep.29257
57. Zhao, P, Sun, X, Reilly, SM, Uhm, M, Liao, Z, Skorobogatko, Y, et al. Tbk1 at the crossroads of inflammation and energy homeostasis in adipose tissue. Cell. (2018) 172:731–743.e12. doi: 10.1016/j.cell.2018.01.007
58. Deng, K-Q, Wang, A, Ji, Y-X, Zhang, X-J, Fang, J, Zhang, Y, et al. Suppressor of Ikkɛ is an essential negative regulator of pathological cardiac hypertrophy. Nat Commun. (2016) 7:11432. doi: 10.1038/ncomms11432
59. Wang, X-A, Zhang, R, She, Z-G, Zhang, X-F, Jiang, D-S, Wang, T, et al. Interferon regulatory factor 3 constrains Ikkβ/Nf-Κb signaling to alleviate hepatic steatosis and insulin resistance. Hepatology. (2014) 59:870–85. doi: 10.1002/hep.26751
60. Wang, XA, Zhang, R, Jiang, D, Deng, W, Zhang, S, Deng, S, et al. Interferon regulatory factor 9 protects against hepatic insulin resistance and steatosis in male mice. Hepatology. (2013) 58:603–16. doi: 10.1002/hep.26368
61. Zhang, S-M, Zhu, L-H, Chen, H-Z, Zhang, R, Zhang, P, Jiang, D-S, et al. Interferon regulatory factor 9 is critical for Neointima formation following vascular injury. Nat Commun. (2014) 5:5160. doi: 10.1038/ncomms6160
62. Wang, P-X, Zhang, R, Huang, L, Zhu, L-H, Jiang, D-S, Chen, H-Z, et al. Interferon regulatory factor 9 is a key mediator of hepatic ischemia/reperfusion injury. J Hepatol. (2015) 62:111–20. doi: 10.1016/j.jhep.2014.08.022
63. Sutti, S, Jindal, A, Locatelli, I, Vacchiano, M, Gigliotti, L, Bozzola, C, et al. Adaptive immune responses triggered by oxidative stress contribute to hepatic inflammation in Nash. Hepatology. (2014) 59:886–97. doi: 10.1002/hep.26749
64. Wolf, MJ, Adili, A, Piotrowitz, K, Abdullah, Z, Boege, Y, Stemmer, K, et al. Metabolic activation of intrahepatic Cd8+ T cells and Nkt cells causes nonalcoholic steatohepatitis and liver Cancer via cross-talk with hepatocytes. Cancer Cell. (2014) 26:549–64. doi: 10.1016/j.ccell.2014.09.003
65. Inzaugarat, ME, Ferreyra Solari, NE, Billordo, LA, Abecasis, R, Gadano, AC, and Cherñavsky, AC. Altered phenotype and functionality of circulating immune cells characterize adult patients with nonalcoholic steatohepatitis. J Clin Immunol. (2011) 31:1120–30. doi: 10.1007/s10875-011-9571-1
66. Sutti, S, and Albano, E. Adaptive immunity: an emerging player in the progression of Nafld. Nat Rev Gastroenterol Hepatol. (2020) 17:81–92. Epub 2019/10/13. doi: 10.1038/s41575-019-0210-2
67. Tang, Y, Bian, Z, Zhao, L, Liu, Y, Liang, S, Wang, Q, et al. Interleukin-17 exacerbates hepatic steatosis and inflammation in non-alcoholic fatty liver disease. Clin Exp Immunol. (2011) 166:281–90. doi: 10.1111/j.1365-2249.2011.04471.x
68. Meng, F, Wang, K, Aoyama, T, Grivennikov, SI, Paik, Y, Scholten, D, et al. Interleukin-17 signaling in inflammatory, Kupffer cells, and hepatic stellate cells exacerbates liver fibrosis in mice. Gastroenterology. (2012) 143:765–76.e3. doi: 10.1053/j.gastro.2012.05.049
69. Van Herck, MA, Weyler, J, Kwanten, WJ, Dirinck, EL, De winter, BY, Francque, SM, et al. The differential roles of T cells in non-alcoholic fatty liver disease and obesity. Front Immunol. (2019) 10:82. doi: 10.3389/fimmu.2019.00082
70. Rau, M, Schilling, AK, Meertens, J, Hering, I, Weiss, J, Jurowich, C, et al. Progression from nonalcoholic fatty liver to nonalcoholic steatohepatitis is marked by a higher frequency of Th17 cells in the liver and an increased Th17/resting regulatory T cell ratio in peripheral blood and in the liver. J Immunol (Baltimore, Md: 1950). (2016) 196:97–105. doi: 10.4049/jimmunol.1501175
71. McPherson, S, Henderson, E, Burt, AD, Day, CP, and Anstee, QM. Serum immunoglobulin levels predict fibrosis in patients with non-alcoholic fatty liver disease. J Hepatol. (2014) 60:1055–62. doi: 10.1016/j.jhep.2014.01.010
72. Shalapour, S, Lin, X-J, Bastian, IN, Brain, J, Burt, AD, Aksenov, AA, et al. Inflammation-induced Iga+ cells dismantle anti-liver cancer immunity. Nature. (2017) 551:340–5. doi: 10.1038/nature24302
73. Gregory, SN, Perati, SR, and Brown, ZJ. Alteration in immune function in patients with fatty liver disease. Hepatoma Res. (2022) 8:31. doi: 10.20517/2394-5079.2022.34
74. Sung, PS, Park, DJ, Roh, PR, Do Mun, K, Cho, SW, Lee, GW, et al. Intrahepatic inflammatory Iga+ Pd-L1high monocytes in hepatocellular carcinoma development and immunotherapy. J Immunother Cancer. (2022) 10:e003618. doi: 10.1136/jitc-2021-003618
75. Bibbò, S, Ianiro, G, Dore, MP, Simonelli, C, Newton, EE, and Cammarota, G. Gut microbiota as a driver of inflammation in nonalcoholic fatty liver disease. Mediat Inflamm. (2018) 2018:1–7. doi: 10.1155/2018/9321643
76. Marra, F, and Svegliati-Baroni, G. Lipotoxicity and the gut-liver Axis in Nash pathogenesis. J Hepatol. (2018) 68:280–95. doi: 10.1016/j.jhep.2017.11.014
77. Schroeder, BO, and Bäckhed, F. Signals from the gut microbiota to distant organs in physiology and disease. Nat Med. (2016) 22:1079–89. doi: 10.1038/nm.4185
78. Gravina, AG, Romeo, M, Pellegrino, R, Tuccillo, C, Federico, A, and Loguercio, C. Just drink a glass of water? Effects of bicarbonate-sulfate-calcium-magnesium water on the gut-liver axis. Front Pharmacol. (2022) 13:869446. doi: 10.3389/fphar.2022.869446
79. Gravina, AG, Pellegrino, R, Romeo, M, Ventriglia, L, Scognamiglio, F, Tuccillo, C, et al. The use of bicarbonate-sulphate-calcium-magnesium and sodium-low drinkable water improves functional gastrointestinal symptoms in patients with non-alcoholic fatty liver disease: a prospective study. Clin Nutr ESPEN. (2023) 57:281–7. doi: 10.1016/j.clnesp.2023.07.008
80. Wree, A, Broderick, L, Canbay, A, Hoffman, HM, and Feldstein, AE. From Nafld to Nash to cirrhosis—new insights into disease mechanisms. Nat Rev Gastroenterol Hepatol. (2013) 10:627–36. doi: 10.1038/nrgastro.2013.149
81. Abu-Shanab, A, and Quigley, EMM. The role of the gut microbiota in nonalcoholic fatty liver disease. Nat Rev Gastroenterol Hepatol. (2010) 7:691–701. doi: 10.1038/nrgastro.2010.172
82. Rensen, SS, Slaats, Y, Nijhuis, J, Jans, A, Bieghs, V, Driessen, A, et al. Increased hepatic myeloperoxidase activity in obese subjects with nonalcoholic steatohepatitis. Am J Pathol. (2009) 175:1473–82. doi: 10.2353/ajpath.2009.080999
83. Heyens, LJM, Busschots, D, Koek, GH, Robaeys, G, and Francque, S. Liver fibrosis in non-alcoholic fatty liver disease: from liver biopsy to non-invasive biomarkers in diagnosis and treatment. Front Med. (2021) 8:615978. doi: 10.3389/fmed.2021.615978
84. Petagine, L, Zariwala, MG, and Patel, VB. Non-alcoholic fatty liver disease: immunological mechanisms and current treatments. World J Gastroenterol. (2023) 29:4831–50. Epub 2023/09/13. doi: 10.3748/wjg.v29.i32.4831
85. Cao, Y, Shi, J, Song, L, Xu, J, Lu, H, Sun, J, et al. Multi-omics integration analysis identifies lipid disorder of a non-alcoholic fatty liver disease (Nafld) mouse model improved by Zexie-Baizhu decoction. Front Pharmacol. (2022) 13:858795. doi: 10.3389/fphar.2022.858795
86. Zheng, K, Zhou, W, Ji, J, Xue, Y, Liu, Y, Li, C, et al. Si-Ni-san reduces lipid droplet deposition associated with decreased Yap1 in metabolic dysfunction-associated fatty liver disease. J Ethnopharmacol. (2023) 305:116081. doi: 10.1016/j.jep.2022.116081
87. Ning, DS, Chen, YJ, Lin, CJ, Wang, CC, Zhao, HW, Wang, KT, et al. Hepatoprotective effect of botanical drug formula on high-fat diet-induced non-alcoholic fatty liver disease by inhibiting lipogenesis and promoting anti-oxidation. Front Pharmacol. (2022) 13:1026912. doi: 10.3389/fphar.2022.1026912
88. Wang, H, Xu, Z, Wang, Q, and Shu, S. Danshao Shugan granule therapy for non-alcoholic fatty liver disease. Lipids Health Dis. (2022) 21:76. doi: 10.1186/s12944-022-01689-9
89. Qiu, M, Xiao, F, Wang, T, Piao, S, Zhao, W, Shao, S, et al. Protective effect of Hedansanqi Tiaozhi Tang against non-alcoholic fatty liver disease in vitro and in vivo through activating Nrf2/ho-1 antioxidant signaling pathway. Phytomedicine. (2020) 67:153140. doi: 10.1016/j.phymed.2019.153140
90. Xiao, L, Liang, S, Ge, L, Qiu, S, Wan, H, Wu, S, et al. Si-Wei-Qing-Gan-Tang improves non-alcoholic steatohepatitis by modulating the nuclear factor-Κb signal pathway and autophagy in methionine and choline deficient diet-fed rats. Front Pharmacol. (2020) 11:530. doi: 10.3389/fphar.2020.00530
91. Zheng, C, Nie, H, Pan, M, Fan, W, Pi, D, Liang, Z, et al. Chaihu Shugan powder influences nonalcoholic fatty liver disease in rats in remodeling micrornaome and decreasing fatty acid synthesis. J Ethnopharmacol. (2024) 318:116967. doi: 10.1016/j.jep.2023.116967
92. Wu, YL, Wu, JX, Shen, TT, Chai, HS, Chen, HF, and Zhang, Q. Quzhi formula alleviates nonalcoholic steatohepatitis by impairing hepatocyte lipid accumulation and inflammation via Bip/Eif2α signaling. J Clin Transl Hepatol. (2022) 10:1050–8. doi: 10.14218/jcth.2021.00458
93. Xu, L, and Cui, H. Yinchenhao Tang alleviates high fat diet induced Nafld by increasing Nr1h4 and Apoa1 expression. J Tradit Complement Med. (2023) 13:325–36. doi: 10.1016/j.jtcme.2023.02.010
94. Xue, Y, Wei, Y, Cao, L, Shi, M, Sheng, J, Xiao, Q, et al. Protective effects of Scutellaria-Coptis herb couple against non-alcoholic steatohepatitis via activating Nrf2 and Fxr pathways in vivo and in vitro. J Ethnopharmacol. (2024) 318:116933. doi: 10.1016/j.jep.2023.116933
95. Yin, G, Liang, H, Sun, W, Zhang, S, Feng, Y, Liang, P, et al. Shuangyu Tiaozhi decoction alleviates non-alcoholic fatty liver disease by improving lipid deposition, insulin resistance, and inflammation in vitro and in vivo. Front Pharmacol. (2022) 13:1016745. doi: 10.3389/fphar.2022.1016745
96. Cao, L, Xu, E, Zheng, R, Zhangchen, Z, Zhong, R, Huang, F, et al. Traditional Chinese medicine Lingguizhugan decoction ameliorate Hfd-induced hepatic-lipid deposition in mice by inhibiting Sting-mediated inflammation in macrophages. Chin Med. (2022) 17:7. doi: 10.1186/s13020-021-00559-3
97. Yu, X, Zhang, H, Pan, J, Zou, L, Tang, L, Miao, H, et al. Jiang Zhi granule protects immunological barrier of intestinal mucosa in rats with non-alcoholic steatohepatitis. Pharm Biol. (2021) 59:1359–68. doi: 10.1080/13880209.2021.1979594
98. Li, Y, Zheng, N, Gao, X, Huang, W, Wang, H, Bao, Y, et al. The identification of material basis of Si Miao formula effective for attenuating non-alcoholic fatty liver disease. J Ethnopharmacol. (2024) 318:116988. doi: 10.1016/j.jep.2023.116988
99. Han, R, Qiu, H, Zhong, J, Zheng, N, Li, B, Hong, Y, et al. Si Miao formula attenuates non-alcoholic fatty liver disease by modulating hepatic lipid metabolism and gut microbiota. Phytomedicine. (2021) 85:153544. doi: 10.1016/j.phymed.2021.153544
100. Zhang, L, Chen, N, Zhan, L, Bi, T, Zhou, W, Zhang, L, et al. Erchen decoction alleviates obesity-related hepatic steatosis via modulating gut microbiota-drived butyric acid contents and promoting fatty acid Β-oxidation. J Ethnopharmacol. (2023) 317:116811. doi: 10.1016/j.jep.2023.116811
101. Luo, D, Yang, L, Pang, H, Zhao, Y, Li, K, Rong, X, et al. Tianhuang formula reduces the oxidative stress response of Nafld by regulating the gut microbiome in mice. Front Microbiol. (2022) 13:984019. doi: 10.3389/fmicb.2022.984019
102. Chu, H, Zhang, W, Tan, Y, Diao, Z, Li, P, Wu, Y, et al. Qing-Zhi-Tiao-Gan-Tang (Qztgt) prevents nonalcoholic steatohepatitis (Nash) by expression pattern correction. J Ethnopharmacol. (2023) 317:116665. doi: 10.1016/j.jep.2023.116665
103. Lan, Q, Ren, Z, Chen, Y, Cui, G, Choi, IC, Ung, COL, et al. Hepatoprotective effect of Qushihuayu formula on non-alcoholic steatohepatitis induced by mcd diet in rat. Chin Med. (2021) 16:27. doi: 10.1186/s13020-021-00434-1
104. Lian, B, Cai, L, Zhang, Z, Lin, F, Li, Z, Zhang, XK, et al. The anti-inflammatory effect of Pien Tze Huang in non-alcoholic fatty liver disease. Biomed Pharmacother. (2022) 151:113076. doi: 10.1016/j.biopha.2022.113076
105. Minxin, Y, Qingxiang, H, and Yi, C. Clinical study on treatment of non-alcoholic fatty liver disease of phlegm-blood stasis type with Qinjiang Baoling decoction. Shanghai J Tradit Chin Med. (2019) 53:56–60. (in Chinese). doi: 10.16305/j.1007-1334.2019.02.015
106. Nan, S, Peiyong, Z, Yucheng, F, Hongyu, M, Jielu, P, Ruiqing, W, et al. Treatment of non-alcoholic fatty liver disease with spleen deficiency and binding of phlegm and stasis syndrome by Lanzhang granules: a randomized controlled study. J Tradit Chin Med. (2023) 64:1448–54. (in Chinese). doi: 10.13288/j.11-2166/r.2023.14.008
107. Hui, D, Liu, L, Azami, NLB, Song, J, Huang, Y, Xu, W, et al. The spleen-strengthening and liver-draining herbal formula treatment of non-alcoholic fatty liver disease by regulation of intestinal Flora in clinical trial. Front Endocrinol. (2022) 13:1107071. doi: 10.3389/fendo.2022.1107071
108. Haihong, T, Chunshan, W, Yingjun, Z, Mei, Q, Yufeng, X, and Guangdong, T. Evaluating the clinical effect of modified Shugan Xiaozhi decoction in treating nonalcoholic fatly liver disease based on Fibroscan technique. J Tradit Chin Med. (2018) 59:594–8. (in Chinese). doi: 10.13288/j.11-2166/r.2018.07.014
109. Shuang, L, Shengxia, L, Yang, C, Tianbai, S, and Ying, L. Clinical study on phlegm-obstruction type of Nafld patients with the treatment of Qinghua decoction. Moder Trad Chinese Med Mater Med World Sci Technol. (2019) 21:1759–65. (in Chinese). doi: 10.11842/wst.20180922007
110. Chaoqun, Z, Hong, C, Gaofeng, C, Long, C, Huiming, Z, Chengbao, W, et al. The effect of Qingre Huashi formula on chronic hepatitis B or nonalcoholic fatty liver disease with damp-heat syndrome: a multicenter randomized double-blind placebo-controlled trial. J Tradit Chin Med. (2022) 63:745–53. (in Chinese). doi: 10.13288/j.11-2166/r.2022.08.010
111. Shuangshuang, L, Wei, J, Yanan, D, Wenqian, W, and Rundong, L. Clinical observation of self-proposed Xiaopi Huatan granules combined with Silibinin capsules in the treatment of non-alcoholic steatohepatitis with phlegm-damp internal resistance syndrome. Guid J Trad Chinese Med Pharm. (2022) 28:66–70. (in Chinese). doi: 10.13862/j.cnki.cn43-1446/r.2022.02.036
112. Zhe, P, Wenfu, Z, Jianlin, L, Wei, G, Lili, Y, Liping, H, et al. Clinical observation of Zhibitai capsule on nonalcoholic fatty liver disease. Chin Arch Tradit Chin Med. (2020) 38:78–80. (in Chinese). doi: 10.13193/j.issn.1673-7717.2020.11.021
113. Dai, L, Xu, J, Liu, B, Dang, Y, Wang, R, Zhuang, L, et al. Lingguizhugan decoction, a Chinese herbal formula, improves insulin resistance in overweight/obese subjects with non-alcoholic fatty liver disease: a translational approach. Front Med. (2022) 16:745–59. doi: 10.1007/s11684-021-0880-3
Glossary
Keywords: metabolic dysfunction-associated steatotic liver disease, Chinese herbal formula, traditional Chinese medicine, mechanisms, review
Citation: Tao S-H, Lei Y-Q, Tan Y-M, Yang Y-B and Xie W-N (2024) Chinese herbal formula in the treatment of metabolic dysfunction-associated steatotic liver disease: current evidence and practice. Front. Med. 11:1476419. doi: 10.3389/fmed.2024.1476419
Edited by:
Ana Sandoval-Rodriguez, University of Guadalajara, MexicoReviewed by:
Belinda Vargas Guerrero, University of Guadalajara, MexicoRaffaele Pellegrino, University of Campania Luigi Vanvitelli, Italy
Copyright © 2024 Tao, Lei, Tan, Yang and Xie. This is an open-access article distributed under the terms of the Creative Commons Attribution License (CC BY). The use, distribution or reproduction in other forums is permitted, provided the original author(s) and the copyright owner(s) are credited and that the original publication in this journal is cited, in accordance with accepted academic practice. No use, distribution or reproduction is permitted which does not comply with these terms.
*Correspondence: Wei-Ning Xie, eHduMTIxOUBxcS5jb20=