- 1Department of Rehabilitation Medicine, The General Hospital of Western Theater Command, Sichuan, Chengdu, China
- 2Sichuan Clinical Medical Research Center for Traditional Chinese Medicine Orthopedics and Sports Medicine Rehabilitation, Sichuan, Chengdu, China
- 3Department of Ophthalmology, The General Hospital of Western Theater Command, Sichuan, Chengdu, China
Objective: Sarcopenia is a significant healthcare challenge in the aging population. Oxidative stress (OS) is acknowledged to play a pivotal role in the pathological progression of sarcopenia. Numerous studies have demonstrated that mitigating or eliminating OS can ameliorate the pathological manifestations associated with sarcopenia. However, current clinical antioxidant therapies often fall short of anticipated outcomes. This bibliometric analysis aims to delineate prevailing research trends, thematic emphases, focal points, and developmental trajectories within the domain of OS in sarcopenia, while also endeavoring to explore prospective anti-oxidative stress strategies for future clinical interventions.
Methods: Relevant publications were retrieved from the Web of Science (WOS) Core Collection database for the period 2000-2024. Citespace was employed for retrieving and analyzing trends and emerging topics.
Results: In the field of OS in sarcopenia, the number of publications has significantly increased from 2000 to 2024. The United States and China are the primary contributors to global publication output. The most productive research institution is INRAE. The most prolific author is Holly Van Remmen from the United States, while the most frequently cited author is Cruz-Jentoft AJ from Spain. Experimental Gerontology is the journal with the highest volume of published articles, whereas the Journal of Gerontology Series A: Biological Sciences and Medical Sciences holds the record for the highest number of citations. The research keywords in this field can be categorized into eight domains: “Physiology and anatomy”, “Physiological mechanisms”, “Pathology associations”, “Experimental studies”, “Nutrition and metabolism”, “Sports and physical activities”, “Age” and “Oxidation and antioxidation”. Moreover, recent years have seen the emergence of “TNF-α,” “insulin resistance”, “mitochondrial autophagy”, “signal pathways”, and “mechanisms” as focal points in the realm of OS in sarcopenia, encompassing related fundamental research and clinical translation.
Conclusion: This bibliometric and visualization provides a comprehensive analysis of the global research landscape in the field of OS in sarcopenia, identifies priorities, summarizes the current research status and suggests possible future research priorities. In addition, in order to benefit more sarcopenia patients, strengthening cooperation and communication between institutions and research teams is the key to the future development of this field. Given the expectation that research on OS in sarcopenia will remain a prominent area of interest in the future, this article could serve as a valuable resource for scholars seeking to shape future studies through an understanding of influential scholarly contributions and key research findings.
Systematic review registration: https://www.crd.york.ac.uk, identifier CRD42024528628.
1 Introduction
Sarcopenia, marked by the rapid decline in muscle mass and function, is an advancing and prevalent skeletal muscle condition (1). In clinical practice, it often manifests insidiously, significantly increasing elderly patients’ susceptibility to falls, fractures, functional decline, and mortality (1–3). Data released by the Asian Working Group for Sarcopenia (AWGS) indicate that sarcopenia’s prevalence among elderly individuals across Asia ranges from 5.5 to 25.7% (2). The incidence of sarcopenia increases with age. Research indicates that sarcopenia’s prevalence can reach up to 15% among individuals aged 65 years and above, and up to 50% among those aged 80 years and above (4). A 2019 study conducted by the University of Oxford in the United Kingdom and the Abbott-Nutrition Division in the United States revealed that patients hospitalized due to sarcopenia incurred average annual medical costs as high as $2,376 and £2,707 (5, 6). The acceleration of global population aging is anticipated to lead to a projected increase in worldwide sarcopenia cases to 500 million by 2050, consequently resulting in a substantial rise in both family economic and social medical burdens (7).
Reactive oxygen species (ROS), which include molecules such as hydrogen peroxide, superoxide anion, and hydroxyl radical are known for their high reactivity and oxygen content (8). These highly reactive molecules exhibit strong oxidizing abilities and play important roles in biological systems. Excessive production of ROS can overwhelm the body’s natural antioxidant defenses, resulting in oxidative stress (OS) closely associated with the pathological advancement of sarcopenia (9). OS can disrupt the equilibrium of skeletal muscle hypertrophy and regeneration through mechanisms such as disturbances in protein synthesis and catabolism, impairment of neuromuscular junction function, and inhibition of satellite cell activation, proliferation, and myoblast differentiation (10–12). Furthermore, inflammation and mitochondrial dysfunction intensify OS, creating a vicious cycle that promotes muscle cell death and progressively reduces muscle fiber number and size (13).
Owing to the scarcity of bibliometric studies on research trends related to OS in sarcopenia, a combination of bibliometric analysis and comprehensive review is employed in this paper to furnish researchers with a foundational knowledge base and outline potential directions for future research. Consequently, an extensive search for articles pertaining to OS and sarcopenia since the 21st century was conducted, significant articles in this field were explored (Figure 1; Supplementary Table S1), and the current research status and cutting-edge trends were analyzed. The objectives were: (1) to identify the predominant academic groups in the domain of OS research in sarcopenia; (2) to conduct a comprehensive analysis of the research foundation, present highlights, and future research directions; (3) based on literature metrology study results, to review key areas and discuss the specific role of crosstalk between oxidative stress and complex pathological mechanisms in muscle diseases.
2 Materials and methods
2.1 Inclusion and exclusion criteria
The Web of Science (WOS) core collection database is widely acknowledged as a comprehensive, systematic, and authoritative repository of influential academic journals, extensively utilized for bibliometric analysis (14, 15). Relevant literature was consulted, and search strategies pertaining to OS and sarcopenia were formulated (Supplementary Table S1) (16, 17). The inclusion and exclusion criteria, as depicted in Figure 1, were: (i) the study period from January 1, 2000, to June 23, 2024; (ii) inclusion of articles and reviews only; and (iii) restriction to publications in English. A total of 720 articles were yielded by the search. Each publication included titles, keywords, publication dates, journal names, author information, countries of origin, affiliated institutions, and citation counts. The literature search and evaluation were independently conducted by two researchers, who resolved any discrepancies through discussion with a third party.
2.2 Tools and data analysis
To conduct further analysis, the plain text export of the acquired articles as “download XXX.txt” was imported into CiteSpace 6.3.1, along with their complete data and references. During the process of mapping and visualizing knowledge figures, a set of primary steps including temporal segmentation, thresholding, modeling, pruning, merging, and mapping was strictly adhered to. CiteSpace encompasses several fundamental concepts such as burst detection, betweenness centrality, and heterogeneous networks, which contribute to the timely visualization of research status updates and the identification of prominent areas and frontiers.
Nodes in different maps show authors, institutions, countries, or keywords, with links between nodes describing co-occurrence or co-citation. Node size represents the frequency of occurrence or citation, while node color denotes the respective years of occurrence or citation. A thicker link denotes more frequent coexistence or cooperation between the nodes. It is noteworthy that betweenness centrality is a tool used to assess the significance of each element in the visualization network. Typically, elements with a betweenness centrality of less than 0.1 will have a purple outer circle, signifying that the node is a hub with significant interactive importance. A modularity value (Q-value) >0.3, combined with an average contour value (S-value) >0.7, suggests that clustering is both statistically significant and reasonable (18). Furthermore, burst detection, pioneered by Kleinberg, is a potent analytical tool. Its strength is measured by the growth rate of keyword citations over time. Therefore, it can be used to investigate research hotspots or frontiers over a specific period, illustrating their evolution (19).
3 Results
3.1 The publication outputs trend
The annual publication production has steadily increased from January 1, 2000, to December 31, 2023, as evidenced by the data (Figure 2). Additionally, based on the fitting curve from 2000 to 2023 (R2 = 0.9405), the projected trend indicates a future increase. This suggests that OS in sarcopenia is a hot topic in the field, attracting more researchers to contribute. Significant progress is projected in understanding the molecular mechanisms related to OS in sarcopenia, potentially providing new ideas for treatment and interventions.
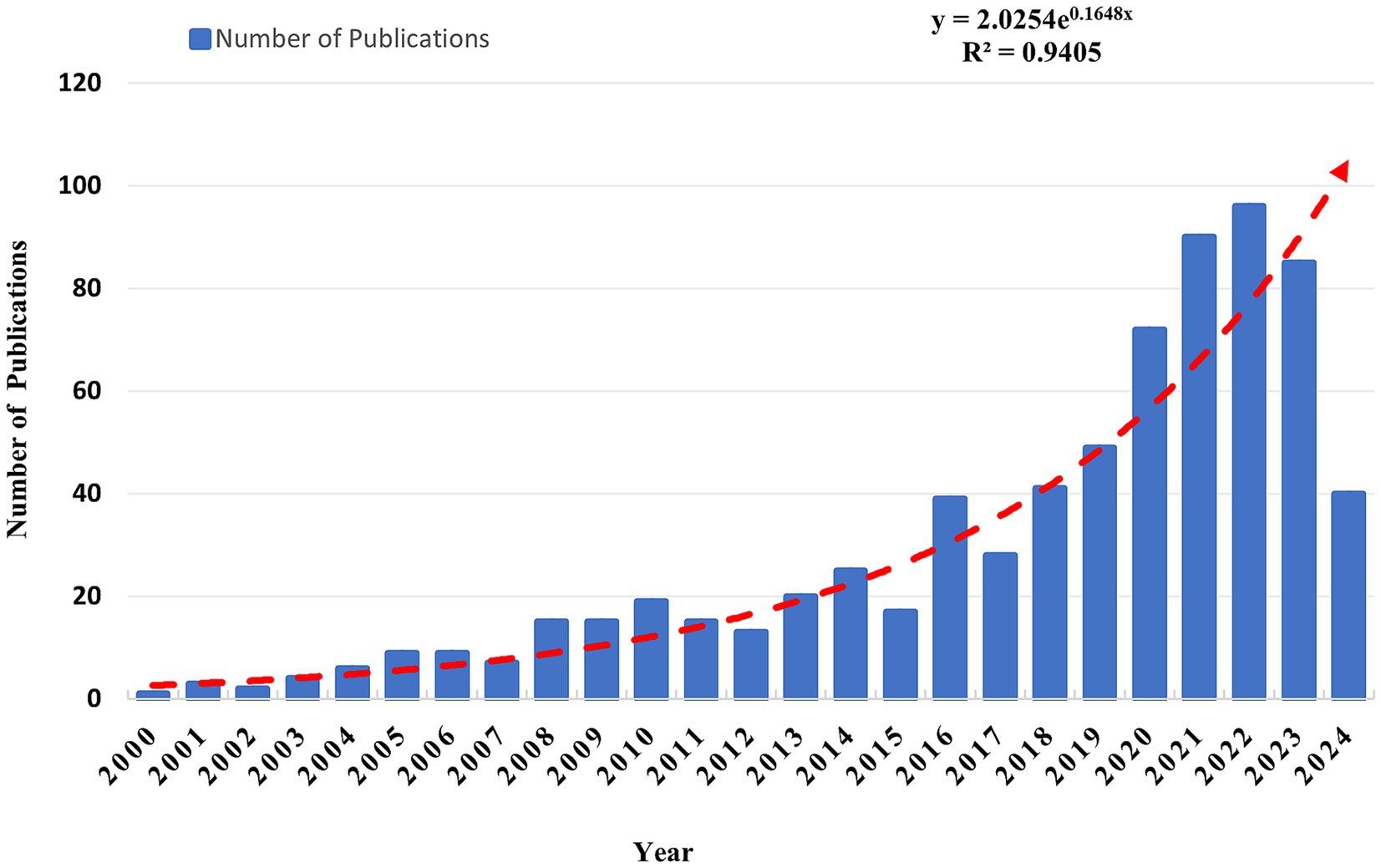
Figure 2. Trends of publications on OS in sarcopenia (the formulae only utilize publications from 2000 to 2023).
3.2 Distribution of countries/regions and institutions
By statistically analyzing the number of papers published by countries, regions, institutions, and authors, the key countries, research institutions, and authors with strong influence in the research field of OS in sarcopenia can be identified, and the cooperation relationships between them can be clarified.
To identify the leading countries/regions in this field, a bibliometric analysis of publications from various countries/regions was conducted using CiteSpace. Publications on OS in sarcopenia originate from a total of 57 nations/regions. There are 57 nodes and 165 links representing the collaborative relationships between nations and regions (Figure 3). This indicates that there is a certain basis of cooperation between countries/regions. The world map generated by Python 3.8, shown in Figure 4, was utilized to visualize the number of publications contributed by each country/region, with each country/region represented by a distinct color. Publications and centrality were used to rank the top 10 nations in Table 1. The United States, China, and Italy were the most prolific, accounting for 26.11, 17.36, and 13.47% of all publications, respectively. Japan, France, South Korea, the United Kingdom, Spain, Canada, and Brazil followed in the rankings. It is worth noting that among the top 10 countries in the number of publications, eight countries, including the United States, Italy, and France, are also among the top 10 in intermediary centrality.
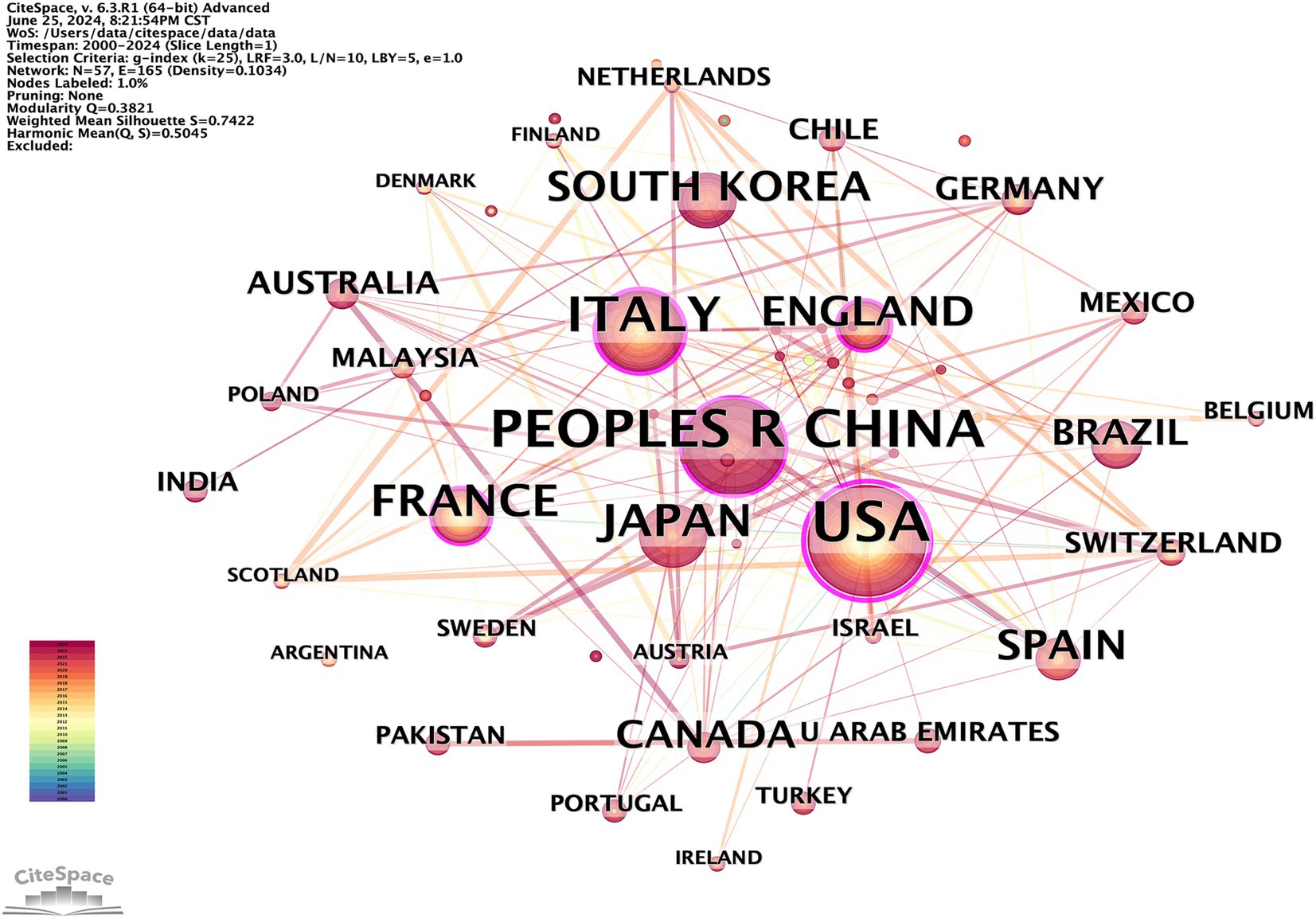
Figure 3. CiteSpace visualization map of publications on OS in sarcopenia from different countries/regions.
The CiteSpace tool was used to construct a visual mapping, aiming to enhance the clarity of institutions involved in cooperation within the field. A total of 410 institutions have published works in this field (Figure 5). These publications are concentrated in universities worldwide and reflect close cooperative relationships. According to their publications and centrality, the top 10 institutions are presented in Table 2. INRAE (26), Oklahoma Medical Research Foundation (21), University of Oklahoma System (21), University of Oklahoma Health Sciences Center (21), and University of Texas System (20) are the top five institutions contributing the most to this discipline. State University System of Florida (0.1), University of Florida (0.1), Aalborg University (0.09), University of Texas System (0.08), and Consiglio Nazionale delle Ricerche (0.08) round out the top five institutions for centrality. Consistent with the main research countries, the leading research institutions in this field are primarily in European and American countries. This information can be utilized by experts in this field to inform research, study, and further cooperation.
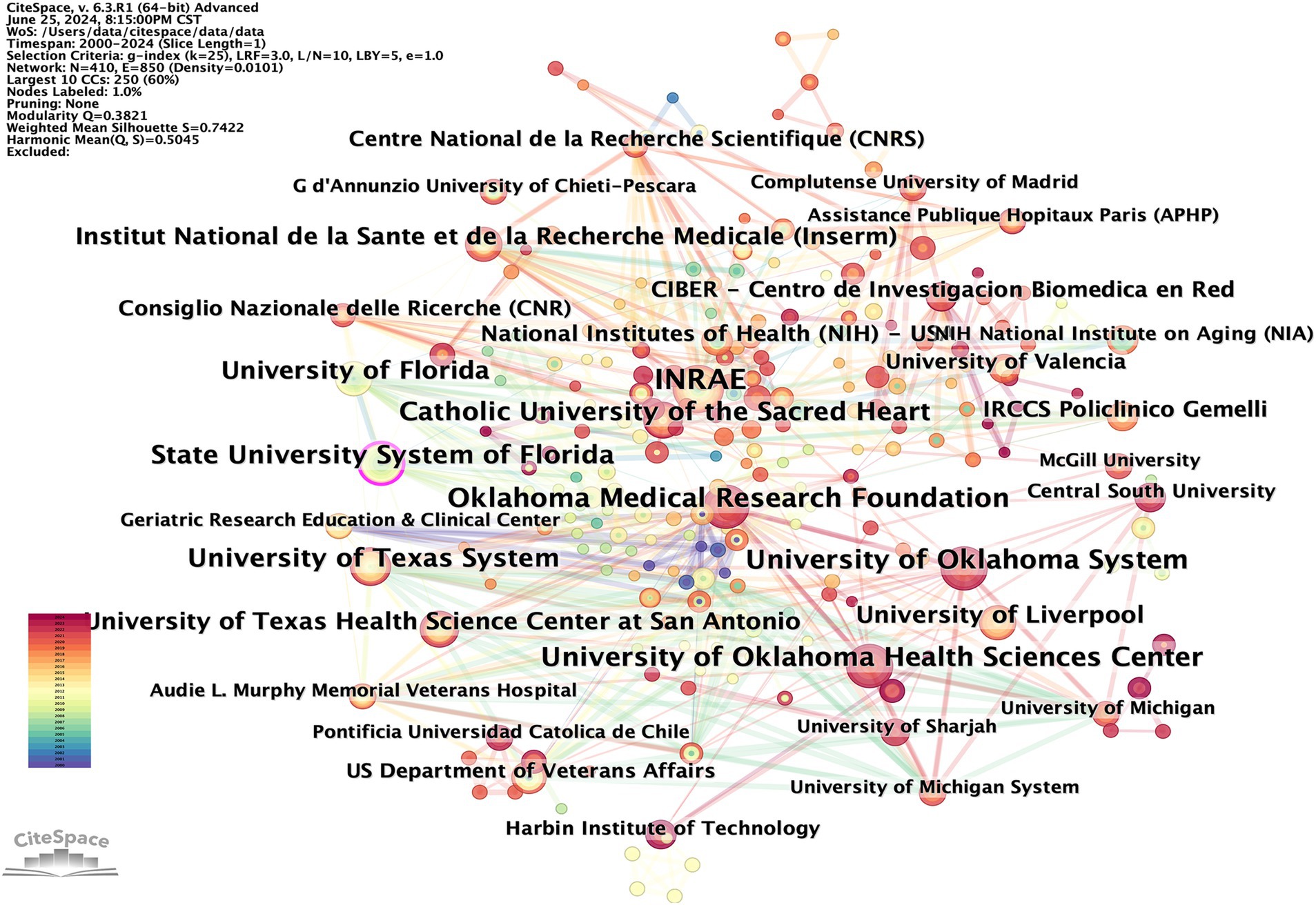
Figure 5. CiteSpace visualization map of publications on OS in sarcopenia from different institutions.
3.3 Authors and co-cited authors
Research typically requires collaboration among multiple researchers. By analyzing the characteristics of the author co-occurrence network, the central figures in a given research field can be identified based on their level of cooperation with other authors. The author co-occurrence network depicted in Figure 6 consists of 616 nodes and 1,125 links, with a density of 0.0059. This indicates a high level of collaboration among scholars in the field, with some potentially establishing formal research groups. A total of 616 authors have conducted relevant research. The most prolific author is Holly Van Remmen from the United States, followed by Rizwan Qaisar from the United Arab Emirates and Malcolm J. Jackson from the UK. Co-citation occurs when two authors’ works are cited together by a third author’s work. This indicates a close academic relationship and suggests their proximity within the scholarly domain (20). The author co-citation network depicted in Figure 7 consists of 853 nodes and 5,349 links, with a density of 0.0147. The top three most frequently co-cited authors are Cruz-Jentoft AJ from Spain, Marzetti E from Italy, and Powers SK from the United States. Table 3 lists the top 10 high-yield authors and the top 10 most cited authors.
3.4 Journals and cited journals
Publications on oxidative stress (OS) in sarcopenia were distributed across 596 academic journals (Figure 8). Among these, 219 articles, comprising 30.41% of the total publications, appeared in the top 10 most active journals (Table 4).
According to Brookes’ Bradford Law, the top 10 journals with the highest publication frequency are acknowledged as pivotal journals in their specific fields (21). These journals serve as the primary options for researchers to submit and refer to articles on OS in sarcopenia. The journals with the highest number of publications were Experimental Gerontology (33) and International Journal of Molecular Sciences (33), followed by Antioxidants (30). The most cited journals were Journals of Gerontology Series A: Biological Sciences and Medical Sciences (422), followed by PLoS One (416) and Free Radical Biology and Medicine (407).
3.5 Co-cited references and research clusters
Highly cited papers are authoritative and influential within a specific field, offering researchers a distinctive methodological or theoretical foundation. Reference co-citation analysis is an effective method for evaluating progress and tracing the development of any research field (22). These co-cited papers likely share common characteristics, such as elucidating and supplementing existing theories or methodologies, thereby establishing the groundwork for a specific field. Networks are consequently utilized to delineate research foundations and forecast forthcoming advancements in areas of interest, capturing the dynamic evolution within the knowledge graph. A co-citation network comprising 992 nodes and 3,916 edges was subsequently established (Figure 9). Although there are two nodes in Figure 9 that are both Cruz-Jentoft AJ (2019), we find that their sizes are different. In the cited map, the size of the node represents the cited frequency of the literature. The larger the node, the more frequently the literature is cited. In combination with Table 5, the large Cruz-Jentoft AJ (2019) node and the small Cruz-Jentoft AJ (2019) node are two papers published by Professor Cruz-Jentoft AJ’s team, respectively, in Age and Ageing and Lancet in 2019. They were also the two most frequently cited articles.
The first and third most frequently cited papers were the expert consensus on diagnosis and treatment of sarcopenia formed by European and Asian experts, respectively. The content addresses European and Asian experts’ consensus on the definition, diagnosis, and prevention of muscle diseases, considering different geographical features (2, 3). The second most-cited paper, “Sarcopenia, “was published in The Lancet in 2019. It summarizes the definition, screening, diagnosis, differential diagnosis, epidemiology, and pathophysiological mechanisms of sarcopenia treatment methods, including drug and non-drug therapies (23). Figure 10 displays the top 25 most cited references exhibiting the highest citation bursts, which initially emerged in 2004, with most occurring between 2012 and 2022, including 17 of the 25 peaks since the turn of the century. This indicates that the research in this field has been further developed and improved since 2012. In addition, due to the lag of literature citation, we can speculate that this field still receives continuous attention to this day.
The subsequent step involved cluster analysis, as depicted in Figure 11. A knowledge graph was constructed from all co-cited references, comprising 19 clusters with distinct labels, providing an intuitive depiction of the temporal evolution of the oxidative stress (OS) in the sarcopenia research knowledge base (Table 6). It is noteworthy that the modularity value (Q-value) and mean silhouette value (S-value) are two key metrics used to evaluate the significance of community structure., with clustering being deemed significant when Q > 0.3 and S > 0.7 (24). Different clusters were obtained using the LLR clustering method. The Q-value (0.7749) and weighted mean silhouette (0.8839) indicated that the network was reasonable, suggesting that the co-cited references in each cluster were highly consistent. Based on the results of the clustering analysis, “mitochondrial homeostasis” (Cluster #0) emerged as the predominant cluster in this field, with an average publication year of 2018. The average year of co-cited reference literature for the most recent cluster was 2021, featuring mitochondrial dysregulation (Cluster #13), oxidative balance score (Cluster #14), and antioxidant activity (Cluster #15).
3.6 Keyword co-occurrence and research clusters
Keywords provide a summary of the abstract, and those with high frequency and centrality often indicate current hot topics in the field of research (25). Hot topics in the study can be revealed by clustering analysis of co-occurring keywords using CiteSpace. The merged co-occurring keywords network, depicted in Figure 12, consists of 552 nodes and 3,761 links. Based on frequency, the two most common keywords are “oxidative stress” and “skeletal muscle,” the themes of this study, pursued by “sarcopenia,” “exercise,” “expression,” “age,” “inflammation,” “body composition,” “older adults,” and “insulin resistance” (Table 7).
The 86 popular keywords appearing more than 10 times were divided into eight themes to determine the research focus (Table 8): Physiology and Anatomy Physiological Mechanism Pathology Associated Experimental Study Nutrition and Metabolism Sport and Physical Activity Age and Oxidation and Antioxidation
Keyword clustering is a method of organizing and categorizing the internal knowledge structure of a particular research field, as illustrated in Figure 13 (26). Seven different clusters were obtained using the LLR clustering method. The Q-value (0.3821) and weighted mean silhouette (0.7422) indicated that the network was reasonable, suggesting that the keywords in each cluster were highly consistent. The average year of the keyword cluster corresponds to the mean publication year of the literature that encompasses these keywords (Table 9). The terms “obesity,” “mitochondrial dysfunction,” and “oxidative balance score” have emerged as prominent research areas in recent years. This indicates the research direction of the current stage.
3.7 Analysis of burst keywords
Figure 14 displays the top 25 keywords exhibiting the most pronounced bursts. Blue bars denote the time interval, while red bars indicate the duration of each burst. It is evident that hot topics have shifted significantly. Since 2019, thirteen keywords, including “mortality,” “mitochondrial biogenesis,” “diabetes mellitus,” “vitamin D,” “cells,” “TNF-α,” “muscle mass,” “mechanisms,” “injury,” “insulin resistance,” “resistance,” “autophagy,” and “protein,” have begun to emerge prominently. Currently, seven terms continue to feature prominently in research: cells, muscle mass, mechanisms, insulin resistance, resistance, autophagy, and protein. This suggests that research on sarcopenia is at the forefront of OS, exploring novel complex cellular and molecular mechanisms of insulin resistance and muscle-related diseases.
4 Discussion
This study employed bibliometrics and visual analysis techniques to investigate the research hotspots and trends pertaining to OS in sarcopenia since the 21st century. The use of bibliometrics and visual analysis to discover international focal points and trends in this research. Domain provides valuable insights into evolving research patterns, helping researchers select future areas of investigation and appropriate journals.
4.1 Knowledge base
Based on the analysis, the quantity of published papers in this area has exhibited an upward trend since 2000. The number of published papers in 2023 was 4.5 times greater than in 2013 and 21.25 times greater than in 2003. The significant increase in publications indicates that OS in sarcopenia is gaining sustained attention from scholars worldwide as an emerging research focus.
Cooperative partnerships among nations, organizations, and scholars. The most impactful countries, institutions, and authors in the field of muscle symptoms were visually analyzed. Among the countries/regions with the greatest number of articles, North America and Eastern Asia have made significant contributions, while African countries have made the least. Articles from the United States, China, and Italy accounted for 56.94% of the total. The United States leads in terms of publications and intermediate centrality. Additionally, six European and North American countries—Italy, France, the United Kingdom, Spain, Canada, and Switzerland—are also in the top 10 for number of publications and intermediary centrality. In Asia, only China, South Korea, and the United Arab Emirates ranked among the top 10 in publication volume and intermediary centrality. South Korea (8) and the United Arab Emirates (10) ranked relatively low in intermediary centrality. This may be due to higher levels of scientific and technological advancement, earlier development, and greater recognition in Western countries. The institution with the greatest publication count in the institutional cooperation network is INRAE in France, while the State University System of Florida in the United States has the highest intermediary centrality. This indicates its pivotal role in linking institutions.
The findings of this study indicate that articles published in age-related and fundamental research journals tend to have higher impact factors, suggesting a greater level of quality. Additionally, Experimental Gerontology had the largest number of publications, and the Journals of Gerontology had the highest citation ranking. This shows that research in elderly diseases is taken seriously and that there is significant attention to basic research in this field. Holly Van Remmen from the Oklahoma Medical Research Foundation is a prominent scholar who has made significant contributions to the field. His extensive research focuses on investigating the mechanisms of OS in sarcopenia, including both upstream and downstream effects, as well as potential treatment interventions (27–29). In general, authors who are frequently cited are regarded as having greater influence than those who are cited less frequently, and co-cited authors often concentrate on related research areas. The scholar with the most citations is Cruz-Jentoft AJ from the Hospital Universitario Ramon y Cajal (IRYCIS) in Madrid, Spain. His primary research focus revolves around clinical aspects, specifically the conduction of clinical trials to investigate the causes and impacts on mortality of muscle diseases. Furthermore, he collaborates with other industry experts to publish expert consensus statements, which exert a significant influence in the field (30–32).
Among the top 10 cited documents, four are review articles, four original research articles, and two expert consensus statements. Interest in these articles has focused on sarcopenia screening and pathophysiological mechanisms, including inflammation, mitochondrial dysfunction, the coupling between increased OS and the disruption of cellular excitatory contraction mechanisms, as well as molecular reciprocity between muscle protein metabolism and therapeutic targets. Additionally, four original articles are dedicated to exploring and elucidating the intrinsic correlation between oxidative stress and sarcopenia. These articles explore various facets, encompassing physiological mechanism of muscle weakness caused by oxidative stress-induced excitation-contraction coupling disorder, the based part of mitochondrial metabolism alterations in elderly sarcopenia, and the involvement of Nrf2 in sarcopenia development. While citation analysis may not be as prompt as keyword analysis in identifying emerging frontiers, it is well-suited for elucidating the evolution of research fields. Therefore, recent clustering effectively showcases current trending topics and future directions (33). The average year of co-cited reference literature for the most recent cluster is 2021, highlighting mitochondrial dysregulation (cluster #13), oxidative balance score (cluster #14), and antioxidant activity (cluster #15). These areas warrant attention from researchers in future studies.
The term “keyword burst” refers to keywords extensively referenced in academic publications, serving as valuable indicators for identifying emerging trends and research hotspots within a specific field. Visualizing these keywords in Figure 14 helps us better understand the research hotspots and frontiers. The most frequent keywords are “oxidative stress” and “skeletal muscle,” which are the themes of this study. The analysis of 86 prominent keywords revealed that “Physiological mechanism” and “Pathology associated” emerged as the most prevalent terms. In addition to oxidative stress (357), sarcopenia (103), and muscle atrophy (39), which are the subjects of this study, it is noteworthy that inflammation (58), insulin resistance (53), and autophagy (31) are the three keywords with the highest frequency. Additionally, “TNF-α (inflammation), ““insulin resistance, “and “autophagy” were identified in the analysis of burst keywords, with chronologically ordered bursts, particularly emphasizing the persistence of the latter two keywords until the present time.
4.2 Research hotspots and frontiers
4.2.1 Inflammation
A growing body of research has shown the crucial role of chronic inflammation in the development and advancement of sarcopenia. Inflammatory factors, particularly TNF-α, are believed to have a negative impact on muscle catabolism directly and indirectly. It has been noted that the release of TNF-α may adversely impact muscle strength and mass as sarcopenia progresses in elderly individuals. For instance, Li’s research indicates that elevated levels of TNF-α (>11.15 pg./mL) are associated with a 7.6-fold increase in the risk of sarcopenia compared to the control group (nonsarcopenia) (34). Consistent with the murine model, 24-month-old wild-type mice showed increased TNF-α levels and reduced muscle mass in the gastrocnemius, soleus, and quadriceps muscles compared to their 6-month-old counterparts (35).
Exposure to TNF-α leads to a significant loss of muscle mass and strength, closely connected with increased ROS production (36). Myostatin (Mstn), a negative regulator of muscle growth, influences the proliferation and differentiation of muscle cells and contributes to sarcopenia. Mstn acts as an oxidant by activating NF-κB and NADPH oxidase, which generates ROS in skeletal muscle cells, thereby inducing OS. TNF-α and hydrogen peroxide (H2O2) are potent inducers of Mstn expression, requiring NF-κB signal transduction for activation. A positive feedback loop exists between Mstn and TNF-α: Mstn triggers ROS production through TNF-α and NADPH oxidase, while elevated TNF-α levels stimulate Mstn expression. Elevated Mstn levels subsequently lead to muscle atrophy by promoting proteasome-mediated intracellular protein degradation (37).
Moreover, the study revealed that aging in mice resulted in a reduction of intestinal barrier thickness, leading to increased bacterial permeability and heightened release of damage-associated molecular patterns (DAMPs) (38, 39). Pattern recognition receptors recognize these DAMPs and trigger immune responses, resulting in elevated inflammation and the production of inflammatory cytokines such as TNF-α and IL-6 (40). Consequently, it is unsurprising that the gut microbiome can impact muscle function. TNF-α enhances the degradation of muscle proteins by activating the ubiquitin-proteasome pathway and upregulating atrogin1/MAFbx, a pivotal ubiquitin ligase involved in muscle breakdown (41). The role of TNF-α in muscle wastage has been confirmed through its complex signaling pathways, including cell death signal transduction. For instance, studies have indicated that targeting the Caspase-8/Caspase-3/GSDME signal-mediated cell pyroptosis pathway may hold promise as a therapeutic strategy for muscle-wasting disorders (42).
Recent investigations have initiated the exploration of TNF-α as a potential biomarker for sarcopenia. The assessment of TNF-α levels in blood or muscle tissue holds promise for the early prediction and diagnosis of sarcopenia (43–45). Therapeutic interventions targeting TNF-α, such as the administration of TNF-α inhibitors (e.g., etanercept and adalimumab), have demonstrated efficacy in various inflammatory conditions (46, 47). Eriodictyol, which naturally occurs in various fruits and vegetables, can activate the PI3K/AKT signaling pathway to alleviate oxidative stress associated with acute liver injury, but it has not been reported in sarcopenia related studies (48). Ongoing research is investigating the potential utility of these agents in mitigating or delaying the progression of sarcopenia. Etanercept has the potential to attenuate fibrotic transformation, reduce muscle wasting, enhance muscle function, and consequently extend the lifespan of aging mice (49, 50). However, in light of the current state of research, further refinement is required for the clinical application of anti-inflammatory cytokine therapy in sarcopenia. This includes addressing late-stage disease treatment and determining the optimal dosage to effectively inhibit inflammatory processes, irrespective of concurrent drug usage.
4.2.2 Insulin resistance
Insulin resistance (IR) is characterized by decreased sensitivity of the body to insulin, resulting in abnormal glucose metabolism. It is highly prevalent in clinical settings and is considered a common underlying factor for chronic metabolic disorders and the pathophysiological basis for certain rare diseases. According to the National Health and Nutrition Examination Survey in the United States, approximately 40% of adults aged 18 to 44 exhibit IR (51). Age-related insulin resistance is related to impaired muscle glucose metabolism, which results in compromised cellular energy production and weakened muscle contraction. Furthermore, a detrimental feedback loop exists between insulin function and age-related decline, worsening insulin resistance (52, 53). The association between muscle characteristics and insulin resistance is strongest in terms of relative muscle mass (54).
With aging, the muscle cell composition shifts from type II fast fibers to type I slow fibers, which have reduced aerobic respiration capacity and a diminished ability to perform rapid, forceful movements. Additionally, muscle quality declines due to adipose tissue infiltration within the muscles, including both intramuscular and intermuscular fat. This infiltration correlates significantly with insulin resistance and is an independent predictor of decreased physical function and increased risk of falls (3). On one hand, insulin resistance can impede the PI3K/protein kinase B signaling pathway, thereby suppressing protein synthesis and reducing muscle growth. On the other hand, insulin resistance may hinder the translocation of glucose transporter 4, thereby reducing muscle glycogen synthesis and resulting in an inadequate energy supply for muscles and decreased muscular strength. Furthermore, insulin resistance has the potential to induce cellular autophagy and mitochondrial dysfunction, which consequently impacts muscular strength (55).
The mammalian target of rapamycin (mTOR) pathway is crucial for muscle synthesis, whereas muscle breakdown is primarily regulated by the ubiquitin-proteasome pathway. IR leads to an imbalance between these processes (56, 57). Meanwhile, elevated levels of TNF-α have been demonstrated to inhibit the AKT/mTOR pathway, governing skeletal muscle hypertrophy, thereby enhancing muscle breakdown (58). However, Sasako et al. showed that interfering with the insulin/IGF-1 signaling pathway by suppressing Akt activity in mouse skeletal muscle can accelerate sarcopenia and reduce lifespan, a phenomenon reversed by deactivating FoxOs rather than activating mTOR (59). Moreover, inflammatory factors (such as TNF-α, IL-1β, and IFN-γ) can further exacerbate IR, resulting in increased inflammation and OS. This establishes a detrimental cycle of muscle wasting, IR, inflammation, and OS, contributing to a decline in muscle mass and a rise in fatness (60, 61). In conclusion, decreased skeletal muscle mass may hinder insulin-driven glucose disposal due to its role as the primary tissue governing insulin-regulated glucose metabolism. This, in conjunction with increased insulin resistance during skeletal muscle aging, contributes to the cascades driving disease progression (62, 63).
The management strategies for IR encompass two key components: lifestyle interventions and pharmacotherapy. Lifestyle interventions include adherence to a nutritious diet, increased physical activity, stress reduction, smoking cessation, alcohol moderation, regular sleep patterns, and supplementation with essential minerals and trace elements. Notably, Harvie et al. observed that the 5: 2 dietary pattern, compared to mere calorie control, demonstrates greater efficacy in ameliorating insulin resistance in the short term (64). Although exercise can enhance insulin sensitivity, the superiority of anaerobic exercise over aerobic exercise in improving insulin resistance remains inconclusive (65). Chobanyan-Jurgens et al. reported that hypoxic endurance training does not confer a superior improvement in insulin resistance compared to normoxic training (66). Conversely, Mai et al. demonstrated that hypoxic exercise results in greater enhancement of insulin resistance compared to normoxic exercise (67). In summary, both moderate aerobic exercise and anaerobic exercise are beneficial for individuals with sarcopenia. At present, there are no drugs sanctioned by regulatory authorities treating muscle wasting related to insulin resistance (68). Presently, therapeutic indications for addressing insulin resistance primarily pertain to type 2 diabetes mellitus, making drug therapy generally appropriate for individuals with both conditions. In cases where insulin resistance coexists with sarcopenia in non-diabetic patients, viable symptomatic treatment alternatives are lacking when lifestyle interventions fail to adequately manage both conditions. Significant advancements are anticipated in this domain.
4.2.3 Autophagy
Autophagy is a highly conserved, lysosome-dependent degradative metabolic process wherein cellular components, including damaged organelles, protein aggregates, and lipid droplets, undergo degradation and subsequent recycling (69). The classical autophagy process encompasses five stages: phagophore formation, nucleation, elongation, autophagosome-lysosome fusion, and degradation (70). Autophagy is facilitated in the elimination of damaged mitochondria and proteins from muscle cells, thereby preserving their health and functionality. Specifically, moderate autophagy can mitigate the accumulation of detrimental substances, diminish OS and inflammation within cells, consequently upholding muscle quality (71, 72). However, exorbitant autophagy may cause the heightened degradation of muscle proteins, thereby exacerbating muscle atrophy and loss of function. In certain pathological conditions, the dysregulation of autophagy, such as overactivation or insufficiency, may serve as a pivotal driving force in sarcopenia (73, 74).
Research has demonstrated that impaired autophagy disrupts cellular homeostasis, impairs mitochondrial function, intensifies OS, and accelerates cellular senescence, thereby compromising satellite cell (75–77). Satellite cells reside between the plasma membrane and the basal lamina of muscle fibers. They have demonstrated effectiveness and autonomy in muscle regeneration, capable of proliferating and differentiating into various types of muscle fibers in response to mechanical damage in the extracellular environment (78, 79). A decrease in satellite cells, or a decline in their function, can compromise muscle regeneration, ultimately resulting in sarcopenia (80, 81). Researchers discovered that activation of the autophagy-AMPK/p27(Kip1) pathway enhances the proliferation efficiency of aging satellite cells, mitigating cell apoptosis and senescence, and ultimately improving muscle regeneration (82). SIRT1 may activate autophagy via the FoXO3a way to satisfy the bioenergetic demands of stimulating satellite cells (83, 84). Disruption of autophagy in satellite cells through ATG7 knockout may impede their regeneration by downregulating the levels of growth hormone receptor (GHR) and insulin-like growth factor-1 (IGF-1) (85). IGF-1 is crucial in different anabolic metabolic pathways within skeletal muscle. Studies suggest that the IGF-1Ea and IGF-1Eb subtypes can induce autophagy/lysosome system activation, which is generally modified in the aging process. Furthermore, these subtypes promote the upregulation of PGC1-α expression, thus affecting mitochondrial function, enhancing ROS detoxification, and adjusting the inflammatory situation in elderly participants (86).
Autophagy plays a crucial role in clearing excessive ROS generated during OS. Studies have shown that compromised autophagic function leads to ineffective regulation of pathological OS within cells (75, 87, 88). Autophagy competes with p62 for binding to Nrf2, inhibiting its degradation and mitigating OS responses (89). Nrf2 depletion enhances the OS response and increases ROS levels by modulating the expression of antioxidant genes (90, 91). Additionally, autophagy can inhibit OS and degrade NLRP3 to prevent the generation of the inflammatory factor IL-1β (92). When NLRP3 is activated, impaired autophagy results in elevated IL-1β expression, which triggers the NF-κB signaling pathway, exacerbating the onset of sarcopenia (93, 94).
Exercise-induced autophagy is promoted through the Akt/mTORC or FoxO3a pathways, potentially representing one of the mechanisms by which aerobic and resistance exercises mitigate muscle loss (95, 96). When long-term supplementation with spermidine, an autophagy inducer, activates autophagy, satellite cell proliferation is increased (97). While the role of spermine in enhancing mitophagy to mitigate oxidative stress-induced cardiac damage is well-established, there is a lack of research exploring its potential beneficial effects on sarcopenia (98, 99). Melatonin, an antioxidant, can promote mitophagy via the cGAS-STING-TBK1 pathway, thereby ameliorating oxidative stress and inflammatory responses. However, the clinical efficacy of melatonin in the treatment of sarcopenia remains uncertain (100, 101).
4.3 Strengths and limitations
The study is constrained by the limited availability of data sources and analytical tools. The primary data source used was the WOS Core Collection database, which may result in the omission of relevant literature. In addition, The WOS database has known biases toward English-language publications and certain geographic regions, potentially underrepresenting valuable research from non-English speaking countries. While CiteSpace software is beneficial for bibliometric analysis, its algorithms and visualization outcomes can be affected by the quantity and quality of the literature analyzed. Additionally, the criteria for selecting literature and the methods for data processing may introduce bias, as no retrieval strategy is flawless. To achieve the most reliable and relevant results, we focused on gathering high-quality literature. What is more, the analysis does not account for negative citations or self-citations, which may inflate impact metrics. On top of that, the real-time updating of the online database introduces a time-sensitive element to bibliometric analysis. Despite these challenges, this study has encompassed the majority of published research on OS in sarcopenia since the 21st century, effectively capturing the prevailing research focal points, developmental trajectory, and emerging trends in this domain. Future studies should consider integrating multiple databases and employing diverse analytical tools for cross-validation.
5 Conclusion
This study marks the pioneering application of bibliometric and visualization analysis in the field of OS in sarcopenia. In this field it has also carried on the comprehensive analysis of the pattern of global research, identified the key focus, summarized the research status and put forward the possible future research priorities. Since the early 21st century, there has been a steady rise in the number of publications, with great contributions from the United States, China, and Italy. Holly Van Remmen is the most prolific author in this field. Experimental Gerontology has the highest volume of published articles, whereas the Journal of Gerontology Series A: Biological Sciences and Medical Sciences holds the record for the highest number of citations. Future research is supposed to pay attention to “TNF-α,” “insulin resistance,” “mitochondrial autophagy,” “signaling pathways,” and “mechanisms” as pivotal areas in the study of oxidative stress in sarcopenia. The current literature review suggests that more high-quality basic research and clinical translational studies are necessary to determine the most effective strategies for reducing oxidative stress in sarcopenic patients, potentially improving their quality of life. Therefore, to benefit more patients with sarcopenia, enhanced collaboration and communication among institutions and research teams are essential for future advancements in this field.
Data availability statement
The raw data supporting the conclusions of this article will be made available by the authors, without undue reservation.
Author contributions
LW: Data curation, Software, Writing – original draft, Visualization. DG: Conceptualization, Formal analysis, Investigation, Writing – original draft. YH: Conceptualization, Formal analysis, Investigation, Writing – original draft. PL: Project administration, Writing – original draft. XZ: Project administration, Supervision, Writing – original draft. LB: Project administration, Resources, Supervision, Visualization, Writing – review & editing. JL: Conceptualization, Formal analysis, Investigation, Writing – review & editing. XH: Conceptualization, Formal analysis, Investigation, Writing – review & editing. RP: Project administration, Supervision, Writing – original draft. XG: Project administration, Resources, Supervision, Visualization, Writing – review & editing.
Funding
The author(s) declare that financial support was received for the research, authorship, and/or publication of this article. This study was supported by the Sichuan Administration of Traditional Chinese Medicine Fund (2021MS257) and the Basic Research Project of the Western Theater General Hospital of the People’s Liberation Army of China (2024YGJCB08).
Conflict of interest
The authors declare that the research was conducted in the absence of any commercial or financial relationships that could be construed as a potential conflict of interest.
Publisher’s note
All claims expressed in this article are solely those of the authors and do not necessarily represent those of their affiliated organizations, or those of the publisher, the editors and the reviewers. Any product that may be evaluated in this article, or claim that may be made by its manufacturer, is not guaranteed or endorsed by the publisher.
Supplementary material
The Supplementary material for this article can be found online at: https://www.frontiersin.org/articles/10.3389/fmed.2024.1472413/full#supplementary-material
References
1. Cruz-Jentoft, AJ, and Sayer, AA. Sarcopenia. Lancet. (2019) 393:2636–46. doi: 10.1016/S0140-6736(19)31138-9
2. Chen, LK, Woo, J, Assantachai, P, Auyeung, TW, Chou, MY, Iijima, K, et al. Asian working Group for Sarcopenia: 2019 consensus update on sarcopenia diagnosis and treatment. J Am Med Dir Assoc. (2020) 21:300–307.e2. doi: 10.1016/j.jamda.2019.12.012
3. Cruz-Jentoft, AJ, Bahat, G, Bauer, J, Boirie, Y, Bruyere, O, Cederholm, T, et al. Sarcopenia: revised European consensus on definition and diagnosis. Age Ageing. (2019) 48:16–31. doi: 10.1093/ageing/afy169
4. Cohen, S, Nathan, JA, and Goldberg, AL. Muscle wasting in disease: molecular mechanisms and promising therapies. Nat Rev Drug Discov. (2015) 14:58–74. doi: 10.1038/nrd4467
5. Pinedo-Villanueva, R, Westbury, LD, Syddall, HE, Sanchez-Santos, MT, Dennison, EM, Robinson, SM, et al. Health care costs associated with muscle weakness: a UK population-based estimate. Calcif Tissue Int. (2019) 104:137–44. doi: 10.1007/s00223-018-0478-1
6. Goates, S, Du, K, Arensberg, MB, Gaillard, T, Guralnik, J, and Pereira, SL. Economic impact of hospitalizations in US adults with sarcopenia. J Frailty Aging. (2019) 8:93–9. doi: 10.14283/jfa.2019.10
7. Antunes, AC, Araujo, DA, Verissimo, MT, and Amaral, TF. Sarcopenia and hospitalisation costs in older adults: a cross-sectional study. Nutr Diet. (2017) 74:46–50. doi: 10.1111/1747-0080.12287
8. Mann, V, Sundaresan, A, and Shishodia, S. Overnutrition and lipotoxicity: impaired Efferocytosis and chronic inflammation as precursors to multifaceted disease pathogenesis. Biology. (2024) 13:13. doi: 10.3390/biology13040241
9. Zhang, H, Qi, G, Wang, K, Yang, J, Shen, Y, Yang, X, et al. Oxidative stress: roles in skeletal muscle atrophy. Biochem Pharmacol. (2023) 214:115664. doi: 10.1016/j.bcp.2023.115664
10. Khodabukus, A, Madden, L, Prabhu, NK, Koves, TR, Jackman, CP, Muoio, DM, et al. Electrical stimulation increases hypertrophy and metabolic flux in tissue-engineered human skeletal muscle. Biomaterials. (2019) 198:259–69. doi: 10.1016/j.biomaterials.2018.08.058
11. Malta, F, and Goncalves, DC. A triple-masked, two-center, randomized parallel clinical trial to assess the superiority of eight weeks of grape seed flour supplementation against placebo for weight loss attenuation during perioperative period in patients with cachexia associated with colorectal cancer: a study protocol. Front Endocrinol. (2023) 14:1146479. doi: 10.3389/fendo.2023.1146479
12. Li, J, Lu, M, Ahn, Y, Cao, K, Pinkus, CA, Stansfield, JC, et al. CHAC1 inactivation is effective to preserve muscle glutathione but is insufficient to protect against muscle wasting in cachexia. PLoS One. (2023) 18:e0283806. doi: 10.1371/journal.pone.0283806
13. Rezzani, R, Favero, G, Ferroni, M, Lonati, C, and Moghadasian, MH. A carnosine analog with therapeutic potentials in the treatment of disorders related to oxidative stress. PLoS One. (2019) 14:e0215170. doi: 10.1371/journal.pone.0215170
14. Wen, P, Luo, P, Zhang, B, and Zhang, Y. Mapping knowledge structure and global research trends in gout: a bibliometric analysis from 2001 to 2021. Front Public Health. (2022) 10:924676. doi: 10.3389/fpubh.2022.924676
15. An, N, An, J, Zeng, T, Wang, S, Li, P, Hu, X, et al. Research progress of mitochondria in chronic obstructive pulmonary disease: a bibliometric analysis based on the web of science Core collection. J Thorac Dis. (2024) 16:215–30. doi: 10.21037/jtd-23-777
16. Jiang, N, Yang, T, Han, H, Shui, J, Hou, M, Wei, W, et al. Exploring Research Trend and hotspots on oxidative stress in ischemic stroke (2001-2022): insights from bibliometric. Mol Neurobiol. (2024) 61:6200–16. doi: 10.1007/s12035-023-03909-4
17. Zeng, Y, He, X, Peng, X, Zhao, L, Yin, C, and Mao, S. Combined nutrition with exercise: fueling the fight against sarcopenia through a bibliometric analysis and review. Int J Gen Med. (2024) 17:1861–76. doi: 10.2147/IJGM.S462594
18. Wu, H, Cheng, K, Guo, Q, Yang, W, Tong, L, Wang, Y, et al. Mapping knowledge structure and themes trends of osteoporosis in rheumatoid arthritis: a bibliometric analysis. Front Med. (2021) 8:787228. doi: 10.3389/fmed.2021.787228
19. Xu, Y, Zhang, Z, He, J, and Chen, Z. Immune effects of macrophages in rheumatoid arthritis: a bibliometric analysis from 2000 to 2021. Front Immunol. (2022) 13:903771. doi: 10.3389/fimmu.2022.903771
20. Pang, XM, Peng, ZY, Zheng, X, Shi, JJ, and Zhou, BC. Analysis of research hotspots in COVID-19 genomics based on CiteSpace software: bibliometric analysis. Front Cell Infect Microbiol. (2022) 12:1060031. doi: 10.3389/fcimb.2022.1060031
21. Brookes, BC . Bradford's law and the bibliography of science. Nature. (1969) 224:953–6. doi: 10.1038/224953a0
22. Cai, H, Du, R, Yang, K, Li, W, and Wang, Z. Association between electroconvulsive therapy and depressive disorder from 2012 to 2021: bibliometric analysis and global trends. Front Hum Neurosci. (2022) 16:1044917. doi: 10.3389/fnhum.2022.1044917
23. Shamseer, L, Moher, D, Clarke, M, Ghersi, D, Liberati, A, Petticrew, M, et al. Preferred reporting items for systematic review and meta-analysis protocols (PRISMA-P) 2015: elaboration and explanation. BMJ. (2015) 349:g7647. doi: 10.1136/bmj.g7647
24. Zhang, G, Song, J, Feng, Z, Zhao, W, Huang, P, Liu, L, et al. Artificial intelligence applicated in gastric cancer: a bibliometric and visual analysis via CiteSpace. Front Oncol. (2022) 12:1075974. doi: 10.3389/fonc.2022.1075974
25. Chen, C . Searching for intellectual turning points: progressive knowledge domain visualization. Proc Natl Acad Sci USA. (2004) 101:5303–10. doi: 10.1073/pnas.0307513100
26. Qin, Y, Zhang, Q, and Liu, Y. Analysis of knowledge bases and research focuses of cerebral ischemia-reperfusion from the perspective of mapping knowledge domain. Brain Res Bull. (2020) 156:15–24. doi: 10.1016/j.brainresbull.2019.12.004
27. Brown, JL, Peelor, FR, Georgescu, C, Wren, JD, Kinter, M, Tyrrell, VJ, et al. Lipid hydroperoxides and oxylipins are mediators of denervation induced muscle atrophy. Redox Biol. (2022) 57:102518. doi: 10.1016/j.redox.2022.102518
28. Xu, H, Ahn, B, and Van Remmen, H. Impact of aging and oxidative stress on specific components of excitation contraction coupling in regulating force generation. Sci Adv. (2022) 8:eadd7377. doi: 10.1126/sciadv.add7377
29. Ahn, B, Ranjit, R, Kneis, P, Xu, H, Piekarz, KM, Freeman, WM, et al. Scavenging mitochondrial hydrogen peroxide by peroxiredoxin 3 overexpression attenuates contractile dysfunction and muscle atrophy in a murine model of accelerated sarcopenia. Aging Cell. (2022) 21:e13569. doi: 10.1111/acel.13569
30. Benz, E, Pinel, A, Guillet, C, Capel, F, Pereira, B, De Antonio, M, et al. Sarcopenia and Sarcopenic obesity and mortality among older people. JAMA Netw Open. (2024) 7:e243604. doi: 10.1001/jamanetworkopen.2024.3604
31. Kirk, B, Cawthon, PM, and Cruz-Jentoft, AJ. Global consensus for sarcopenia. Aging (Albany NY). (2024) 16:9306–8. doi: 10.18632/aging.205919
32. Kirk, B, Cawthon, PM, Arai, H, Avila-Funes, JA, Barazzoni, R, Bhasin, S, et al. The conceptual definition of sarcopenia: Delphi consensus from the global leadership initiative in sarcopenia (GLIS). Age Ageing. (2024) 53:afae052. doi: 10.1093/ageing/afae052
33. Feng, Z, Si, M, Fan, H, Zhang, Y, Yuan, R, and Hao, Z. Evolution, current status, and future trends of maxillary skeletal expansion: a bibliometric analysis. Clin Oral Investig. (2023) 28:14. doi: 10.1007/s00784-023-05430-3
34. Li, CW, Yu, K, Shyh-Chang, N, Li, GX, Jiang, LJ, Yu, SL, et al. Circulating factors associated with sarcopenia during ageing and after intensive lifestyle intervention. J Cachexia Sarcopenia Muscle. (2019) 10:586–600. doi: 10.1002/jcsm.12417
35. Wang, Y, Welc, SS, Wehling-Henricks, M, and Tidball, JG. Myeloid cell-derived tumor necrosis factor-alpha promotes sarcopenia and regulates muscle cell fusion with aging muscle fibers. Aging Cell. (2018) 17:e12828. doi: 10.1111/acel.12828
36. Thoma, A, and Lightfoot, AP. NF-kB and inflammatory cytokine Signalling: role in skeletal muscle atrophy. Adv Exp Med Biol. (2018) 1088:267–79. doi: 10.1007/978-981-13-1435-3_12
37. Sriram, S, Subramanian, S, Sathiakumar, D, Venkatesh, R, Salerno, MS, McFarlane, CD, et al. Modulation of reactive oxygen species in skeletal muscle by myostatin is mediated through NF-kappaB. Aging Cell. (2011) 10:931–48. doi: 10.1111/j.1474-9726.2011.00734.x
38. Liang, Z, Zhang, T, Liu, H, Li, Z, Peng, L, Wang, C, et al. Inflammaging: the ground for sarcopenia? Exp Gerontol. (2022) 168:111931. doi: 10.1016/j.exger.2022.111931
39. Wilson, QN, Wells, M, Davis, AT, Sherrill, C, Tsilimigras, M, Jones, RB, et al. Greater microbial translocation and vulnerability to metabolic disease in healthy aged female monkeys. Sci Rep. (2018) 8:11373. doi: 10.1038/s41598-018-29473-9
40. Thevaranjan, N, Puchta, A, Schulz, C, Naidoo, A, Szamosi, JC, Verschoor, CP, et al. Age-associated microbial Dysbiosis promotes intestinal permeability, systemic inflammation, and macrophage dysfunction. Cell Host Microbe. (2018) 23:570. doi: 10.1016/j.chom.2018.03.006
41. Ryu, H, Jeong, HH, Kim, MJ, Lee, S, Jung, WK, and Lee, B. Modulation of macrophage transcript and secretion profiles by Sargassum Serratifolium extract is associated with the suppression of muscle atrophy. Sci Rep. (2024) 14:13282. doi: 10.1038/s41598-024-63146-0
42. Wu, J, Lin, S, Chen, W, Lian, G, Wu, W, Chen, A, et al. TNF-alpha contributes to sarcopenia through caspase-8/caspase-3/GSDME-mediated pyroptosis. Cell Death Discov. (2023) 9:76. doi: 10.1038/s41420-023-01365-6
43. Demir, L, and Oflazoglu, U. The relationship between sarcopenia and serum irisin and TNF-alpha levels in newly diagnosed cancer patients. Support Care Cancer. (2023) 31:586. doi: 10.1007/s00520-023-08041-6
44. Byrne, T, Cooke, J, Bambrick, P, McNeela, E, and Harrison, M. Circulating inflammatory biomarker responses in intervention trials in frail and sarcopenic older adults: a systematic review and meta-analysis. Exp Gerontol. (2023) 177:112199. doi: 10.1016/j.exger.2023.112199
45. Xuekelati, S, Maimaitiwusiman, Z, Bai, X, Xiang, H, Li, Y, and Wang, H. Sarcopenia is associated with hypomethylation of TWEAK and increased plasma levels of TWEAK and its downstream inflammatory factor TNF-alpha in older adults: a case-control study. Exp Gerontol. (2024) 188:112390. doi: 10.1016/j.exger.2024.112390
46. Fouad, MA, Tadros, MG, and Michel, HE. Etanercept ameliorates chronic mild stress-induced depressive-like behavior in rats: crosstalk between MAPK and STAT3 pathways and norepinephrine and serotonin transporters. Eur J Pharmacol. (2024) 978:176801. doi: 10.1016/j.ejphar.2024.176801
47. Morozova, N, Avramovic, MZ, Markelj, G, Toplak, N, and Avcin, T. Dynamics of serum levels of TNF-alpha in a longitudinal follow-up study in 98 patients with juvenile idiopathic arthritis treated with anti-TNF-alpha biological drugs. Clin Rheumatol. (2024) 43:2287–93. doi: 10.1007/s10067-024-07012-4
48. Zheng, X, Wu, X, Wen, Q, Tang, H, Zhao, L, Shi, F, et al. Eriodictyol alleviated LPS/D-GalN-induced acute liver injury by inhibiting oxidative stress and cell apoptosis via PI3K/AKT signaling pathway. Nutrients. (2023) 15:4349. doi: 10.3390/nu15204349
49. Sciorati, C, Gamberale, R, Monno, A, Citterio, L, Lanzani, C, De Lorenzo, R, et al. Pharmacological blockade of TNFalpha prevents sarcopenia and prolongs survival in aging mice. Aging. (2020) 12:23497–508. doi: 10.18632/aging.202200
50. Dionyssiotis, Y . Sarcopenia in the elderly. Eur Endocrinol. (2019) 15:13–4. doi: 10.17925/EE.2019.15.1.13
51. Parcha, V, Heindl, B, Kalra, R, Li, P, Gower, B, Arora, G, et al. Insulin resistance and Cardiometabolic risk profile among nondiabetic American young adults: insights from NHANES. J Clin Endocrinol Metab. (2022) 107:e25–37. doi: 10.1210/clinem/dgab645
52. Gungor, O, Ulu, S, Hasbal, NB, Anker, SD, and Kalantar-Zadeh, K. Effects of hormonal changes on sarcopenia in chronic kidney disease: where are we now and what can we do? J Cachexia Sarcopenia Muscle. (2021) 12:1380–92. doi: 10.1002/jcsm.12839
53. Abbatecola, AM, Paolisso, G, Fattoretti, P, Evans, WJ, Fiore, V, Dicioccio, L, et al. Discovering pathways of sarcopenia in older adults: a role for insulin resistance on mitochondria dysfunction. J Nutr Health Aging. (2011) 15:890–5. doi: 10.1007/s12603-011-0366-0
54. Bijlsma, AY, Meskers, CG, van Heemst, D, Westendorp, RG, de Craen, AJ, and Maier, AB. Diagnostic criteria for sarcopenia relate differently to insulin resistance. Age (Dordr). (2013) 35:2367–75. doi: 10.1007/s11357-013-9516-0
55. Tack, W, De Cock, AM, Dirinck, EL, Bastijns, S, Arien, F, and Perkisas, S. Pathophysiological interactions between sarcopenia and type 2 diabetes: a two-way street influencing diagnosis and therapeutic options. Diabetes Obes Metab. (2024) 26:407–16. doi: 10.1111/dom.15321
56. Zhang, B, Yang, Q, Liu, N, Zhong, Q, and Sun, Z. The effects of glutamine supplementation on liver inflammatory response and protein metabolism in muscle of lipopolysaccharide-challenged broilers. Animals. (2024) 14:14. doi: 10.3390/ani14030480
57. Yang, X, Wang, L, Zhang, L, Zhai, X, Sheng, X, Quan, H, et al. Exercise mitigates Dapagliflozin-induced skeletal muscle atrophy in STZ-induced diabetic rats. Diabetol Metab Syndr. (2023) 15:154. doi: 10.1186/s13098-023-01130-w
58. Sabbah, HN . Barth syndrome cardiomyopathy: targeting the mitochondria with elamipretide. Heart Fail Rev. (2021) 26:237–53. doi: 10.1007/s10741-020-10031-3
59. Sasako, T, Umehara, T, Soeda, K, Kaneko, K, Suzuki, M, Kobayashi, N, et al. Deletion of skeletal muscle Akt1/2 causes osteosarcopenia and reduces lifespan in mice. Nat Commun. (2022) 13:5655. doi: 10.1038/s41467-022-33008-2
60. Wannamethee, SG, and Atkins, JL. Muscle loss and obesity: the health implications of sarcopenia and sarcopenic obesity. Proc Nutr Soc. (2015) 74:405–12. doi: 10.1017/S002966511500169X
61. Li, CW, Yu, K, Shyh-Chang, N, Jiang, Z, Liu, T, Ma, S, et al. Pathogenesis of sarcopenia and the relationship with fat mass: descriptive review. J Cachexia Sarcopenia Muscle. (2022) 13:781–94. doi: 10.1002/jcsm.12901
62. Kalyani, RR, Corriere, M, and Ferrucci, L. Age-related and disease-related muscle loss: the effect of diabetes, obesity, and other diseases. Lancet Diabetes Endocrinol. (2014) 2:819–29. doi: 10.1016/S2213-8587(14)70034-8
63. DeFronzo, RA, and Tripathy, D. Skeletal muscle insulin resistance is the primary defect in type 2 diabetes. Diabetes Care. (2009) 32:S157–63. doi: 10.2337/dc09-S302
64. Harvie, M, Wright, C, Pegington, M, McMullan, D, Mitchell, E, Martin, B, et al. The effect of intermittent energy and carbohydrate restriction v. daily energy restriction on weight loss and metabolic disease risk markers in overweight women. Br J Nutr. (2013) 110:1534–47. doi: 10.1017/S0007114513000792
65. Kanaley, JA, Colberg, SR, Corcoran, MH, Malin, SK, Rodriguez, NR, Crespo, CJ, et al. Exercise/physical activity in individuals with type 2 diabetes: a consensus statement from the American College of Sports Medicine. Med Sci Sports Exerc. (2022) 54:353–68. doi: 10.1249/MSS.0000000000002800
66. Chobanyan-Jurgens, K, Scheibe, RJ, Potthast, AB, Hein, M, Smith, A, Freund, R, et al. Influences of hypoxia exercise on whole-body insulin sensitivity and oxidative metabolism in older individuals. J Clin Endocrinol Metab. (2019) 104:5238–48. doi: 10.1210/jc.2019-00411
67. Mai, K, Klug, L, Rakova, N, Piper, SK, Mahler, A, Bobbert, T, et al. Hypoxia and exercise interactions on skeletal muscle insulin sensitivity in obese subjects with metabolic syndrome: results of a randomized controlled trial. Int J Obes. (2020) 44:1119–28. doi: 10.1038/s41366-019-0504-z
68. Nishikawa, H, Asai, A, Fukunishi, S, Nishiguchi, S, and Higuchi, K. Metabolic syndrome and sarcopenia. Nutrients. (2021) 13:13. doi: 10.3390/nu13103519
69. Kitada, M, and Koya, D. Autophagy in metabolic disease and ageing. Nat Rev Endocrinol. (2021) 17:647–61. doi: 10.1038/s41574-021-00551-9
70. Galluzzi, L, and Green, DR. Autophagy-independent functions of the autophagy machinery. Cell. (2019) 177:1682–99. doi: 10.1016/j.cell.2019.05.026
71. Jia, M, Yue, X, Sun, W, Zhou, Q, Chang, C, Gong, W, et al. ULK1-mediated metabolic reprogramming regulates Vps34 lipid kinase activity by its lactylation. Sci Adv. (2023) 9:eadg4993. doi: 10.1126/sciadv.adg4993
72. Villalon-Garcia, I, Povea-Cabello, S, Alvarez-Cordoba, M, Talaveron-Rey, M, Suarez-Rivero, JM, Suarez-Carrillo, A, et al. Vicious cycle of lipid peroxidation and iron accumulation in neurodegeneration. Neural Regen Res. (2023) 18:1196–202. doi: 10.4103/1673-5374.358614
73. Liu, Z, Guo, Y, and Zheng, C. Type 2 diabetes mellitus related sarcopenia: a type of muscle loss distinct from sarcopenia and disuse muscle atrophy. Front Endocrinol. (2024) 15:1375610. doi: 10.3389/fendo.2024.1375610
74. Mohd, SN, and Makpol, S. Suppression of Inflamm-aging by Moringa oleifera and Zingiber officinale roscoe in the prevention of degenerative diseases: a review of current evidence. Molecules. (2023) 28:28. doi: 10.3390/molecules28155867
75. Xie, G, Jin, H, Mikhail, H, Pavel, V, Yang, G, Ji, B, et al. Autophagy in sarcopenia: possible mechanisms and novel therapies. Biomed Pharmacother. (2023) 165:115147. doi: 10.1016/j.biopha.2023.115147
76. Garcia-Prat, L, Martinez-Vicente, M, Perdiguero, E, Ortet, L, Rodriguez-Ubreva, J, Rebollo, E, et al. Autophagy maintains stemness by preventing senescence. Nature. (2016) 529:37–42. doi: 10.1038/nature16187
77. Garcia-Prat, L, Munoz-Canoves, P, and Martinez-Vicente, M. Monitoring autophagy in muscle stem cells. Methods Mol Biol. (2017) 1556:255–80. doi: 10.1007/978-1-4939-6771-1_14
78. Tsuchiya, Y, Kitajima, Y, Masumoto, H, and Ono, Y. Damaged Myofiber-derived metabolic enzymes act as activators of muscle satellite cells. Stem Cell Rep. (2020) 15:926–40. doi: 10.1016/j.stemcr.2020.08.002
79. Collins, CA, Olsen, I, Zammit, PS, Heslop, L, Petrie, A, Partridge, TA, et al. Stem cell function, self-renewal, and behavioral heterogeneity of cells from the adult muscle satellite cell niche. Cell. (2005) 122:289–301. doi: 10.1016/j.cell.2005.05.010
80. Ding, H, Chen, S, Pan, X, Dai, X, Pan, G, Li, Z, et al. Transferrin receptor 1 ablation in satellite cells impedes skeletal muscle regeneration through activation of ferroptosis. J Cachexia Sarcopenia Muscle. (2021) 12:746–68. doi: 10.1002/jcsm.12700
81. Wang, Y, Wehling-Henricks, M, Welc, SS, Fisher, AL, Zuo, Q, and Tidball, JG. Aging of the immune system causes reductions in muscle stem cell populations, promotes their shift to a fibrogenic phenotype, and modulates sarcopenia. FASEB J. (2019) 33:1415–27. doi: 10.1096/fj.201800973R
82. White, JP, Billin, AN, Campbell, ME, Russell, AJ, Huffman, KM, and Kraus, WE. The AMPK/p27(Kip1) Axis regulates autophagy/apoptosis decisions in aged skeletal muscle stem cells. Stem Cell Rep. (2018) 11:425–39. doi: 10.1016/j.stemcr.2018.06.014
83. Zheng, Y, Shi, B, Ma, M, Wu, X, and Lin, X. The novel relationship between Sirt3 and autophagy in myocardial ischemia-reperfusion. J Cell Physiol. (2019) 234:5488–95. doi: 10.1002/jcp.27329
84. Tang, AH, and Rando, TA. Induction of autophagy supports the bioenergetic demands of quiescent muscle stem cell activation. EMBO J. (2014) 33:2782–97. doi: 10.15252/embj.201488278
85. Zecchini, S, Giovarelli, M, Perrotta, C, Morisi, F, Touvier, T, Di Renzo, I, et al. Autophagy controls neonatal myogenesis by regulating the GH-IGF1 system through a NFE2L2- and DDIT3-mediated mechanism. Autophagy. (2019) 15:58–77. doi: 10.1080/15548627.2018.1507439
86. Ascenzi, F, Barberi, L, Dobrowolny, G, Villa, NBA, Nicoletti, C, Rizzuto, E, et al. Effects of IGF-1 isoforms on muscle growth and sarcopenia. Aging Cell. (2019) 18:e12954. doi: 10.1111/acel.12954
87. Ianniciello, A, Zarou, MM, Rattigan, KM, Scott, M, Dawson, A, Dunn, K, et al. ULK1 inhibition promotes oxidative stress-induced differentiation and sensitizes leukemic stem cells to targeted therapy. Sci Transl Med. (2021) 13:eabd5016. doi: 10.1126/scitranslmed.abd5016
88. Ruart, M, Chavarria, L, Camprecios, G, Suarez-Herrera, N, Montironi, C, Guixe-Muntet, S, et al. Impaired endothelial autophagy promotes liver fibrosis by aggravating the oxidative stress response during acute liver injury. J Hepatol. (2019) 70:458–69. doi: 10.1016/j.jhep.2018.10.015
89. Tang, Z, Hu, B, Zang, F, Wang, J, Zhang, X, and Chen, H. Nrf2 drives oxidative stress-induced autophagy in nucleus pulposus cells via a Keap1/Nrf2/p62 feedback loop to protect intervertebral disc from degeneration. Cell Death Dis. (2019) 10:510. doi: 10.1038/s41419-019-1701-3
90. Kitaoka, Y, Tamura, Y, Takahashi, K, Takeda, K, Takemasa, T, and Hatta, H. Effects of Nrf2 deficiency on mitochondrial oxidative stress in aged skeletal muscle. Physiol Rep. (2019) 7:e13998. doi: 10.14814/phy2.13998
91. Huang, DD, Yan, XL, Fan, SD, Chen, XY, Yan, JY, Dong, QT, et al. Nrf2 deficiency promotes the increasing trend of autophagy during aging in skeletal muscle: a potential mechanism for the development of sarcopenia. Aging. (2020) 12:5977–91. doi: 10.18632/aging.102990
92. Lin, JQ, Tian, H, Zhao, XG, Lin, S, Li, DY, Liu, YY, et al. Zinc provides neuroprotection by regulating NLRP3 inflammasome through autophagy and ubiquitination in a spinal contusion injury model. CNS Neurosci Ther. (2021) 27:413–25. doi: 10.1111/cns.13460
93. Liu, T, Wang, L, Liang, P, Wang, X, Liu, Y, Cai, J, et al. USP19 suppresses inflammation and promotes M2-like macrophage polarization by manipulating NLRP3 function via autophagy. Cell Mol Immunol. (2021) 18:2431–42. doi: 10.1038/s41423-020-00567-7
94. Mehto, S, Jena, KK, Nath, P, Chauhan, S, Kolapalli, SP, Das, SK, et al. The Crohn's disease risk factor IRGM limits NLRP3 Inflammasome activation by impeding its assembly and by mediating its selective autophagy. Mol Cell. (2019) 73:429–445.e7. doi: 10.1016/j.molcel.2018.11.018
95. Colleluori, G, Aguirre, L, Phadnis, U, Fowler, K, Armamento-Villareal, R, Sun, Z, et al. Aerobic plus resistance exercise in obese older adults improves muscle protein synthesis and preserves Myocellular quality despite weight loss. Cell Metab. (2019) 30:261–273.e6. doi: 10.1016/j.cmet.2019.06.008
96. Valero-Breton, M, Warnier, G, Castro-Sepulveda, M, Deldicque, L, and Zbinden-Foncea, H. Acute and chronic effects of high frequency electric pulse stimulation on the Akt/mTOR pathway in human primary Myotubes. Front Bioeng Biotechnol. (2020) 8:565679. doi: 10.3389/fbioe.2020.565679
97. Madeo, F, Eisenberg, T, Pietrocola, F, and Kroemer, G. Spermidine in health and disease. Science. (2018) 359:359. doi: 10.1126/science.aan2788
98. Srivastava, V, Zelmanovich, V, Shukla, V, Abergel, R, Cohen, I, Ben-Sasson, SA, et al. Distinct designer diamines promote mitophagy, and thereby enhance healthspan in C. Elegans and protect human cells against oxidative damage. Autophagy. (2023) 19:474–504. doi: 10.1080/15548627.2022.2078069
99. Chen, JC, Wang, R, and Wei, CC. Anti-aging effects of dietary phytochemicals: from Caenorhabditis elegans, Drosophila melanogaster, rodents to clinical studies. Crit Rev Food Sci Nutr. (2024) 64:5958–83. doi: 10.1080/10408398.2022.2160961
100. Wang, S, Wang, L, Qin, X, Turdi, S, Sun, D, Culver, B, et al. ALDH2 contributes to melatonin-induced protection against APP/PS1 mutation-prompted cardiac anomalies through cGAS-STING-TBK1-mediated regulation of mitophagy. Signal Transduct Target Ther. (2020) 5:119. doi: 10.1038/s41392-020-0171-5
Keywords: bibliometric analysis, bibliometric, CiteSpace, oxidative stress, sarcopenia
Citation: Wang L, Guo D, Huang Y, Long P, Zhang X, Bai L, Liu J, Hu X, Pang R and Gou X (2024) Scientific landscape of oxidative stress in sarcopenia: from bibliometric analysis to hotspots review. Front. Med. 11:1472413. doi: 10.3389/fmed.2024.1472413
Edited by:
Gang Ye, Sichuan Agricultural University, ChinaCopyright © 2024 Wang, Guo, Huang, Long, Zhang, Bai, Liu, Hu, Pang and Gou. This is an open-access article distributed under the terms of the Creative Commons Attribution License (CC BY). The use, distribution or reproduction in other forums is permitted, provided the original author(s) and the copyright owner(s) are credited and that the original publication in this journal is cited, in accordance with accepted academic practice. No use, distribution or reproduction is permitted which does not comply with these terms.
*Correspondence: Rizhao Pang, cHJ6cHJ6MTdAMTI2LmNvbQ==; Xiang Gou, NDI1MTcwMDg5QHFxLmNvbQ==
†These authors share first authorship