- 1Centre for Neuroimaging Sciences, Institute of Psychiatry, Psychology and Neuroscience, King’s College London, London, United Kingdom
- 2Groover Consulting, Rotterdam, Netherlands
- 3Department of Psychosis Studies, Institute of Psychiatry, Psychology and Neuroscience, King’s College London, London, United Kingdom
- 4Department of Computer Science, Centre for Medical Image Computing, University College London, London, United Kingdom
- 5Dementia Research Centre, Institute of Neurology, University College London, London, United Kingdom
Background: Rheumatoid arthritis (RA) and ulcerative colitis (UC) are two autoimmune diseases where patients report high levels of fatigue, pain, and depression. The effect of systemic inflammation from these diseases is likely affecting the brain, however, it is unknown whether there are measurable neuroanatomical changes and whether these are a contributing factor to these central symptoms.
Methods: We included 258 RA patients with 774 age and sex matched controls and 249 UC patients with 747 age and sex matched controls in a case control study utilizing the UK Biobank dataset. We used imaging derived phenotypes (IDPs) to determine whether there were differences in (1) hippocampal volume and (2) additional subcortical brain volumes between patients compared to controls and if there were common regions affected between these two diseases.
Results: Patients with UC had moderately smaller hippocampi compared to age and sex matched controls (difference: 134.15 mm3, SD ± 64.76, p = 0.035). This result was not seen in RA patients. RA patients had a significantly smaller amygdala volume than age and sex matched controls (difference: 91.27 mm3, SD ± 30.85, p = 0.0021, adjusted p = 0.012). This result was not seen in UC patients. All other subcortical structures analyzed were comparable between the patients and control groups.
Conclusion: These results indicate there are subcortical brain differences between UC, RA and controls but different regions of the limbic system are preferentially affected by UC and RA. This study may provide evidence for different neurodegenerative mechanisms in distinct autoimmune diseases.
1 Introduction
Rheumatoid arthritis (RA) and ulcerative colitis (UC) are two of the most prevalent autoimmune diseases and are both projected to have increasing incidence and prevalence rates globally (1, 2). Autoimmune diseases represent a large and heterogeneous group of disorders that afflict specific target organs (3). However, there are common links between these different diseases. This includes the presence of both individual and familial polyautoimmunity, which is defined as the presence of more than one autoimmune disease in a single patient or within a familial line (4).
There is also significant overlap in the treatment of these two diseases both in the acute, flare stage and long-term disease management (5–7). The common genetic markers and antigen patterns observed may provide some indication of shared disease pathogenesis specifically in genes, including CCL3 and CXCL10, that are related to T-cell activation and leukocyte migration (8–12).
Traditionally, both in clinical drug development and clinical practice of autoimmune diseases, the primary focus has been in the management of disease burden in the periphery (13–16). However, the presence of central symptoms, such as pain and fatigue, present a distinct challenge in effectively treating patients with these diseases (17). Patients with RA and UC have increased risk for psychiatric disorders including anxiety and depression (18–21). One study reported that 23% of UC patients suffer from depression and 33% suffer from anxiety (22). This has been reported to be even higher in RA with one study showing 55% of patients reporting depressive symptoms (22, 23). This is considerably higher than the base rate of anxiety and depression in the UK population at the time this data was acquired which was 4.65 and 4.12%, respectively (24, 25). In addition, RA patients with depression report increased autoimmune disease activity and lower response to treatment (6, 19, 26).
A previous study in RA has shown smaller hippocampal volume in patients is associated with more severe functional disability and higher pain perception both on a visual analog scale and in functional pain response to pressure stimulus (27). There have also been previous studies which have looked at volumetric differences in gray matter in both UC and RA that suggest subcortical differences in both diseases that may involve the pain processing pathways (28, 29). These central manifestations combined with increased interest in improving our understanding of the neuro-immune axis, is motivating research into whether systemic inflammation from these autoimmune pathways has a central effect. Following on from that, this raises the question whether there is a common or discrete central effect caused by different autoimmune diseases.
The aims of our study were to determine if there were structural brain differences between patients with RA compared to matched controls and patients with UC compared to matched controls. The primary analysis focused on the total hippocampal volume differences between groups with a secondary analysis looking at further subcortical regions. The hippocampus was chosen as the region of interest for the primary analysis due to previous research linking the hippocampus to systemic inflammation and autoimmune diseases (30–32). Additionally, we looked to see if there was overlap in the subcortical regions affected between these two diseases.
2 Methods
2.1 Study population
This is a nested case control study utilizing the UK Biobank data. The UK Biobank is a large, prospective observational study of 500,000 participants providing extensive biological information (33). The imaging substudy is planned to scan 100,000 of those participants with a standardized scanning protocol including MRI of the brain.
The UK Biobank has approval from the North West Multi-center Research Ethics Committee (MREC) as a Research Tissue Bank (RTB) approval. This research was done under the RTB approval and separate ethical clearance was not required. The UK Biobank Ethics and Governance Council (EGC) was established as an independent guardian of the UK Biobank Ethics and Governance Framework (EGF). All participant materials, including the informed consent form, have been developed and are monitored under this framework. All data received from the UK Biobank is anonymized and additional consent for this research was not required. All data was accessed under UK Biobank application number 40933.
At the time of this investigation, brain MRI were available from 40,681 participants. For the purposes of the present study, we selected 2,028 individuals including patients with RA, UC and healthy controls. Due to the difference in age and sex distribution between RA and UC patient populations, separate controls groups were matched to each patient population in a 1:3 patient: control ratio. Using the matchit algorithm in R, an exact matching strategy was employed for sex and a nearest neighbor matching strategy was utilized for age matching and selection of the control groups. This method was chosen given the large number of potential control subjects to choose from and resulted in an exact match by patient for sex and a nearly identical age distribution between patients and controls. A matching ratio of 1:3 was determined to be optimal as it allowed for the highest matching ratio while utilizing the matching strategy outlined above.
Data from 258 individuals with a primary or secondary diagnosis of RA identified using International Clarification of Disease (ICD)-10 codes M05 or M06 were included in the RA patient group (mean age ± SD in RA = 65.41 ± 7.06, 71% female) with 774 age and sex matched controls (mean age ± SD in RA control group = 65.41 ± 7.05, 71% female). Data from 249 individuals with a primary or secondary diagnosis of UC identified using ICD-10 code K51 were included in the UC patient group (mean age ± SD in UC = 64.06 ± 7.05, 50% female) with 747 age and sex matched controls (mean age ± SD in UC control group = 64.06 ± 7.06, 50% female). There were four patients who had both an ICD-10 code of K51 and either M05 or M06. These 4 patients are included in both patient groups.
2.2 Data acquisition and processing
Full details on the UK Biobank neuroimaging data are provided here: https://biobank.ctsu.ox.ac.uk/crystal/crystal/docs/brain_mri.pdf. In Short: multi-modal MR images were acquired on a Siemens 3 T scanner. The T1-weighted MRI used an MPRAGE sequence with 1-mm isotropic resolution. From the T1w data, volumetric imaging derived phenotypes (IDPs) are generated by the UK Biobank using an established image-processing pipeline (34). The subcortical volumetric measurements specifically utilize the FMRIB Integrated Registration and Segmentation Tool (FIRST) (35). FSL FIRST is a model-based segmentation tool trained on manually segmented images that provides automated subcortical volumetric measurements.
2.3 Statistical analysis
All statistical analyses were carried out using R version 4.1.1.
The primary analysis for this study compared total hippocampal volume in each patient group to their respective matched control groups. Model one consisted of a linear model regression with sex, age and total intracranial volume (ICV) as covariates.
Hypertension is a known risk factor for brain atrophy and is highly associated with atrophy in the hippocampus (36). Given this association and the increased prevalence of hypertension in both the UC and RA patient populations, as reported in the demographics table below, a second model was run analyzing hippocampal volume with hypertension as an additional covariate to gender, age and ICV.
A secondary analysis utilized the same linear model regression with sex, age and ICV as covariates for the remaining subcortical volume measures from the FSL FIRST pipeline. This included total volume of the following structures: nucleus accumbens, amygdala, caudate, pallidum, putamen and thalamus. Multiple testing corrections for these separate measures were conducted using a Bonferroni correction across all 6 IDPs.
A tertiary analysis was completed where the individual left and right volumes were analyzed in those structures where a statistically significant difference was measured in the total structure to look for potential unilateral effect. This consisted of a linear model regression of the left and right volumes for the hippocampus analyzed separately with sex, age and ICV as covariates.
To calculate an effect-size we used Cohen’s d. All p-values <0.05 were considered statistically significant.
3 Results
3.1 Demographics
Detailed demographic information can be found in Table 1. The control groups were matched directly on age and sex. Both control groups were generally comparable to their matched patient population except for rates of hypertension and hypercholesterolemia. Hypertension specifically is nearly twice as prevalent in both patient populations as compared to their control groups. As this discrepancy was anticipated, we examine the role of hypertension in these diseases using a second model with hypertension as a covariate.
3.2 Hippocampal volume in RA and UC
A significantly lower total hippocampal volume was observed in UC patients compared to the control group (p = 0.035). In contrast RA patients did not show a significant reduction in total hippocampal volume (p = 0.42). A tertiary analysis was completed to investigate right and left hippocampal volumes separately to ascertain whether there was a bilateral effect. While the p value was not statistically significant for the left hippocampal volume in UC patients, the raw volume measurements combined with the calculated p value in the right (p = 0.043, Cohen’s d = −0.15, 95% CI −0.3, −0.01) versus left (p = 0.096, Cohen’s d = −0.13, 95% CI −0.27, 0.02) hippocampal volume differences are however similar enough not to suggest lateralization of the difference observed. The results for total hippocampal volume in UC can be found in Figure 1. Full results of the primary and tertiary analysis in hippocampal volumes for both UC and RA can be found in Table 2.
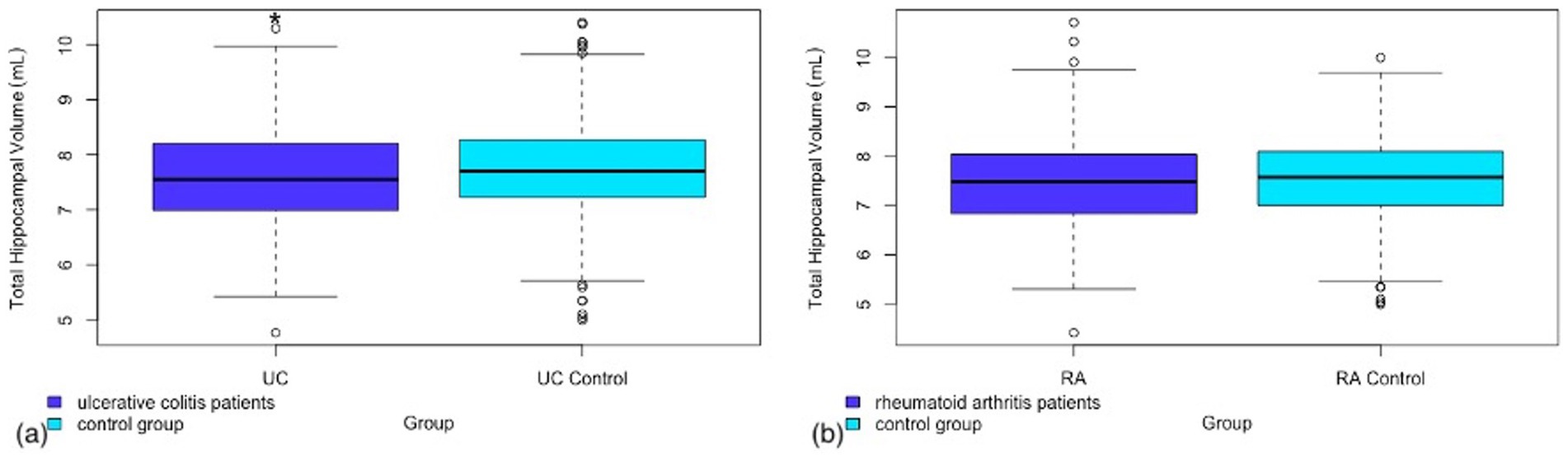
Figure 1. Box and whisker plot representing the total hippocampal volume in (a) patients with ulcerative colitis as compared to a matched control group (b) patients with rheumatoid arthritis as compared to a matched control group.
As mentioned in the methods section, a second model was run including hypertension as a covariate. This was performed to account for any potential signal being attributable to the most prevalent cerebrovascular risk factor in these patient populations. This did account for some of the signal with a p-value of 0.08 in model 2 for total hippocampal volume in UC versus controls compared to 0.035 in model 1 without hypertension as a covariate. Full results can be found in Supplementary Table S1.
3.3 Additional subcortical regions analysis
A secondary analysis was performed in both RA and UC patients looking at total volume of additional subcortical regions provided by the FSL FIRST pipeline. Of the additional regions analyzed there was a significant difference in amygdala volume between RA patients and controls (Figure 2). No other sub-cortical regions showed significant volume differences between RA patients and controls (see Table 3).
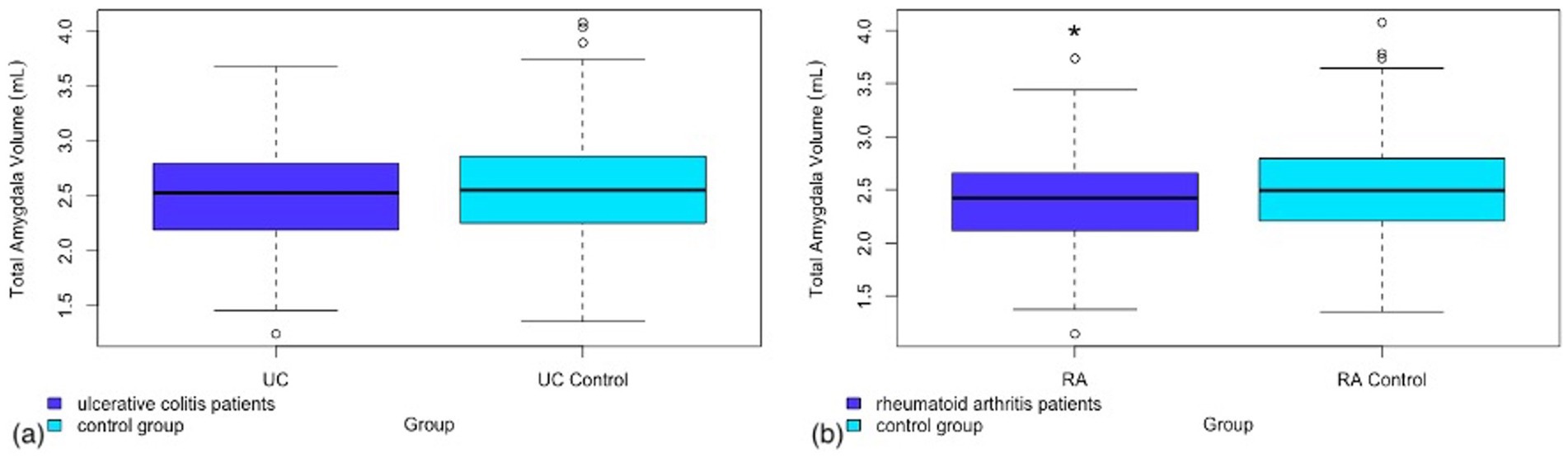
Figure 2. Box and whisker plot representing the total amygdala volume in (a) patients with ulcerative colitis as compared to a matched control group (b) patients with rheumatoid arthritis as compared to a matched control group.
This same result was not found in the UC population. There was no significant difference seen between UC patients and controls in any of the additional subcortical regions analyzed. Full results from this analysis can be found in Table 4.
4 Discussion
In patients with UC compared with controls, we found a moderately smaller total hippocampal volume. Additional analyses were conducted looking at the right and left hippocampus separately and the resulting p-values did not indicate a strong unilateral affect. No similar, significant reduction in hippocampal volume was observed in the RA population. In the secondary analysis, we found a significantly lower amygdala volume in patients with RA compared to controls. This same result was not seen in the UC population.
These findings may suggest there are different areas of the brain effected in these two diseases. At the same time, the close relationship between the amygdala and hippocampus may also suggest a common pathway affecting the brain in these diseases. This is particularly interesting as both the amygdala and the hippocampus are associated with chronic pain and depression (37, 38).
The fact that in this work both regions were not affected in the same way in these two diseases suggests potential differences in the specific disease processes that may preferentially affect one region over another and may result in varying degrees of these central symptoms. The significant difference in amygdala volumes in RA may be linked to the increased rates of anxiety in RA versus UC patients. Previous rs-fMRI research has suggested that the cognitive impairments and reported “brain fog” in UC may be predominantly linked to the limbic system which would be consistent with these findings (39).
Increased rates of cardiovascular risk factors are a known complication of autoimmune diseases (40, 41). The prevalence of hypertension seen in our RA population is consistent with previously published literature (42). In the case of UC, it is more complicated with some of the literature reporting higher rates of hypertension and some reporting similar rates of hypertension to the general population, but higher rates of other cardiovascular risk factors (43). In both diseases there is extensive literature pointing to a host of higher cardiovascular risk factors with unknown etiology (44). It has been proposed that this could be a result of medication usage, lack of physical activity due to disease burden and/or a function of the disease process directly (45, 46). Irrespective of the origin of these risk factors there is clear evidence outlined here in this study, of localized, lower subcortical volumes in both diseases.
This is the largest dataset, to date, investigating subcortical brain volume measures in both RA and UC. Previously published work has looked at total gray matter volume differences in IBD, an umbrella term including both Crohn’s disease and UC, and explored volumetric differences correlated to systemic inflammatory markers and clinical disease status (n = 18 in both studies) (47, 48). Similar work has been published in RA linking increased peripheral inflammation with lower GM volumes and larger ventricle-to-brain ratios to disease duration (n = 33 and n = 54) (49, 50). There is some precedent for looking at subcortical volumes in RA (n = 31 and n = 54) (28, 51). A meta-analysis and literature review by these authors provides a more comprehensive outline of previously published volumetric work in both RA and UC (30).
A limitation to this study is the lack of information on disease duration and severity. Both UC and RA are relapsing–remitting diseases and can vary widely in their individual presentation. In a recent paper Zhang et al. sub-categorized UC patients into active stage versus remission and reported fewer regions of decreased neuroanatomical volume in those currently in remission (52). There is also previously published data that suggests that the age of onset can significantly affect disease severity (53). The lack of this information prevents us from stratifying this patient population based on their clinical disease activity and management. How well controlled an individual’s disease is and on what medications may have an important impact on these central effects which we are unable to quantify with this data set.
One ongoing point of debate with regards to the UK Biobank data set is the lack of heterogeneity and the question of whether it is truly representative of the wider population. Participants tend to be healthier than the general population reporting lower rates of cancer and overall all-cause mortality in addition to being more health conscious. This is a well-established effect seen in volunteer-based cohort studies (54). While this is important to acknowledge, the breadth of lifestyle, genetic and demographic information allows us to appropriately contextualize our patient and control populations. The UK Biobank is continually adding lifestyle, wellness and cognitive information on subjects and could be a valuable additional next step to expanding this research. As a resource this allowed us access to neuroimaging data in these two diseases that is substantially larger than anything published to date.
A potential next step for this work would be to look at volumetric changes in these populations over time. There is an ongoing project to acquire follow up scans in a subset of up to 10,000 UK Biobank Imaging cohort participants. This may make it possible to analyze atrophy over time in these patient populations. This would provide additional insight into how the presence of these systemic autoimmune diseases may affect atrophy and aging in these populations compared to their peers.
These findings play a potentially important role in further understanding brain volume differences in preferential areas of the brain in RA and UC. With increased focus on understanding and mitigating risk factors for neurodegenerative diseases this provides the potential foundation for future work in exploring the link between these autoimmune diseases and the development of future neurodegenerative diseases in these populations. There is a known link between UC and Parkinson’s Disease (PD) and between RA and Alzheimer’s Disease (AD) (55–58). There is also documented research suggesting accelerated atrophy in PD in the hippocampus, as seen in our results for UC, and in the amygdala in AD, as seen here in our RA results (59, 60).
The results of this study highlight the central effect of rheumatoid arthritis and ulcerative colitis and the importance of continued monitoring and treatment of central symptoms for brain health long term in people with chronic autoimmune conditions.
Data availability statement
The raw data supporting the conclusions of this article will be made available by the authors, without undue reservation.
Ethics statement
The studies involving humans were approved by North West Multi-Centre Research Ethics Committee (MREC). The studies were conducted in accordance with the local legislation and institutional requirements. The participants provided their written informed consent to participate in this study.
Author contributions
JGC: Data curation, Formal analysis, Writing – original draft. MG: Writing – review & editing. MK: Writing – review & editing. SW: Writing – review & editing. JHC: Writing – review & editing.
Funding
The author(s) declare that financial support was received for the research, authorship, and/or publication of this article. The authors acknowledged financial support from the Wellcome Trust and the Engineering and Physical Sciences Research Council for the King’s Medical Engineering Centre and the National Institute for Health Research (NIHR) Biomedical Research Centre at South London and Maudsley NHS Foundation Trust and King’s College London.
Acknowledgments
The authors are thankful to the Department of Neuroimaging and the Department of Psychosis Studies, Institute of Psychiatry, Psychology, and Neuroscience, King’s College London.
Conflict of interest
Jennifer Cox is an industry funded PhD student funded by GlaxoSmithKline and an employee of Johnson & Johnson. GSK and Johnson & Johnson had no role in the design or conduct of the study. Marius de Groot is a former employee of, and holds shares in GlaxoSmithKline (GSK). GSK had no role in the design or conduct of the study. Kempton was funded by an MRC Career Development Fellowship (grant MR/J008915/1). Williams has received research funding from Bionomics, Eli Lilly, the Engineering and Physical Sciences Research Council, GlaxoSmithKline, Johnson & Johnson, Lundbeck, the National Institute of Health Research, Pfizer, Takeda, and Wellcome Trust. Marius de Groot was employed by the Groover Consulting.
The remaining author declares that the research was conducted in the absence of any commercial or financial relationships that could be construed as a potential conflict of interest.
Publisher’s note
All claims expressed in this article are solely those of the authors and do not necessarily represent those of their affiliated organizations, or those of the publisher, the editors and the reviewers. Any product that may be evaluated in this article, or claim that may be made by its manufacturer, is not guaranteed or endorsed by the publisher.
Author disclaimer
The views expressed here are those of the authors and not necessarily those of the NHS, the NIHR, or the Department of Health.
Supplementary material
The Supplementary material for this article can be found online at: https://www.frontiersin.org/articles/10.3389/fmed.2024.1468910/full#supplementary-material
References
1. Richardson, J, Nelson, L, Curry, A, and Ralston, S. The increasing global incidence of ulcerative colitis; implications for the economic burden of ulcerative colitis. Value Health. (2018) 21:S82. doi: 10.1016/j.jval.2018.04.556
2. Safiri, S, Kolahi, AA, Hoy, D, Smith, E, Bettampadi, D, Mansournia, MA, et al. Global, regional and national burden of rheumatoid arthritis 1990-2017: a systematic analysis of the global burden of Disease study 2017. Ann Rheum Dis. (2019) 78:1463–71. doi: 10.1136/annrheumdis-2019-215920
4. Matusiewicz, A, Strozynska-Byrska, J, and Olesinska, M. Polyautoimmunity in rheumatological conditions. Int J Rheum Dis. (2019) 22:386–91. doi: 10.1111/1756-185X.13454
5. Bruscoli, S, Febo, M, Riccardi, C, and Migliorati, G. Glucocorticoid therapy in inflammatory bowel Disease: mechanisms and clinical practice. Front Immunol. (2021) 12:691480. doi: 10.3389/fimmu.2021.691480
6. Smolen, JS, Landewé, RBM, Bijlsma, JWJ, Burmester, GR, Dougados, M, Kerschbaumer, A, et al. EULAR recommendations for the management of rheumatoid arthritis with synthetic and biological disease-modifying antirheumatic drugs: 2019 update. Ann Rheum Dis. (2020) 79:685–99. doi: 10.1136/annrheumdis-2019-216655
7. Rubin, DT, Ananthakrishnan, AN, Siegel, CA, Sauer, BG, and Long, MD. ACG clinical guideline: ulcerative colitis in adults. Am J Gastroenterol. (2019) 114:384–413. doi: 10.14309/ajg.0000000000000152
8. Chen, Y, Li, H, Lai, L, Feng, Q, and Shen, J. Identification of common differentially expressed genes and potential therapeutic targets in ulcerative colitis and rheumatoid arthritis. Front Genet. (2020) 11:572194. doi: 10.3389/fgene.2020.572194
9. Attalla, MG, Singh, SB, Khalid, R, Umair, M, and Epenge, E. Relationship between ulcerative colitis and rheumatoid arthritis: A review. Cureus. (2019) 11:e5695. doi: 10.7759/cureus.5695
10. Min, S-H, Wang, Y, Gonsiorek, W, Anilkumar, G, Kozlowski, J, Lundell, D, et al. Pharmacological targeting reveals distinct roles for CXCR2/CXCR1 and CCR2 in a mouse model of arthritis. Biochem Biophys Res Commun. (2010) 391:1080–6. doi: 10.1016/j.bbrc.2009.12.025
11. Pan, Z, Lin, H, Fu, Y, Zeng, F, Gu, F, Niu, G, et al. Identification of gene signatures associated with ulcerative colitis and the association with immune infiltrates in colon cancer. Front Immunol. (2023) 14:1086898. doi: 10.3389/fimmu.2023.1086898
12. Guan, Y, Zhang, Y, Zhu, Y, and Wang, Y. CXCL10 as a shared specific marker in rheumatoid arthritis and inflammatory bowel disease and a clue involved in the mechanism of intestinal flora in rheumatoid arthritis. Sci Rep. (2023) 13:9754. doi: 10.1038/s41598-023-36833-7
13. Gaujoux-Viala, C, Mouterde, G, Baillet, A, Claudepierre, P, Fautrel, B, le Loët, X, et al. Evaluating disease activity in rheumatoid arthritis: which composite index is best? A systematic literature analysis of studies comparing the psychometric properties of the DAS, DAS28, SDAI and CDAI. Joint Bone Spine. (2012) 79:149–55. doi: 10.1016/j.jbspin.2011.04.008
14. Walsh, AJ, Ghosh, A, Brain, AO, Buchel, O, Burger, D, Thomas, S, et al. Comparing disease activity indices in ulcerative colitis. J Crohn's Colitis. (2014) 8:318–25. doi: 10.1016/j.crohns.2013.09.010
15. Pabla, BS, and Schwartz, DA. Assessing severity of disease in patients with ulcerative colitis. Gastroenterol Clin N Am. (2020) 49:671–88. doi: 10.1016/j.gtc.2020.08.003
16. Rodriguez-García, SC, Montes, N, Ivorra-Cortes, J, Triguero-Martinez, A, Rodriguez-Rodriguez, L, Castrejón, I, et al. Disease activity indices in rheumatoid arthritis: comparative performance to detect changes in function, IL-6 levels, and radiographic progression. Front Med. (2021) 8:8. doi: 10.3389/fmed.2021.669688
17. Choy, EH, and Dures, E. Fatigue in rheumatoid arthritis. Rheumatology. (2019) 58:v1–2. doi: 10.1093/rheumatology/kez314
18. Zhang, B, Wang, HHE, Bai, YM, Tsai, SJ, Su, TP, Chen, TJ, et al. Bidirectional association between inflammatory bowel disease and depression among patients and their unaffected siblings. J Gastroenterol Hepatol. (2022) 37:1307–15. doi: 10.1111/jgh.15855
19. Matcham, F, Rayner, L, Steer, S, and Hotopf, M. The prevalence of depression in rheumatoid arthritis: a systematic review and meta-analysis. Rheumatology. (2013) 52:2136–48. doi: 10.1093/rheumatology/ket169
20. Eugenicos, MP, and Ferreira, NB. Psychological factors associated with inflammatory bowel disease. Br Med Bull. (2021) 138:16–28. doi: 10.1093/bmb/ldab010
21. Margaretten, M, Julian, L, Katz, P, and Yelin, E. Depression in patients with rheumatoid arthritis: description, causes and mechanisms. Int J Clin Rheumtol. (2011) 6:617–23. doi: 10.2217/ijr.11.62
22. Yuan, X, Chen, B, Duan, Z, Xia, Z, Ding, Y, Chen, T, et al. Depression and anxiety in patients with active ulcerative colitis: crosstalk of gut microbiota, metabolomics and proteomics. Gut Microbes. (2021) 13:1987779. doi: 10.1080/19490976.2021.1987779
23. Englbrecht, M, Alten, R, Aringer, M, Baerwald, CG, Burkhardt, H, Eby, N, et al. New insights into the prevalence of depressive symptoms and depression in rheumatoid arthritis - implications from the prospective multicenter VADERA II study. PLoS One. (2019) 14:e0217412. doi: 10.1371/journal.pone.0217412
24. Dettmann, LM, Adams, S, and Taylor, G. Investigating the prevalence of anxiety and depression during the first COVID-19 lockdown in the United Kingdom: systematic review and meta-analyses. Br J Clin Psychol. (2022) 61:757–80. doi: 10.1111/bjc.12360
25. Disease, GBD, Injury, I, and Prevalence, C. Global, regional, and national incidence, prevalence, and years lived with disability for 354 diseases and injuries for 195 countries and territories, 1990-2017: a systematic analysis for the global burden of Disease study 2017. Lancet. (2018) 392:1789–858. doi: 10.1016/S0140-6736(18)32279-7
26. Pezzato, S, Bonetto, C, Caimmi, C, Tomassi, S, Montanari, I, Gnatta, MG, et al. Depression is associated with increased disease activity and higher disability in a large Italian cohort of patients with rheumatoid arthritis. Adv Rheumatol. (2021) 61:57. doi: 10.1186/s42358-021-00214-3
27. Andersson, KME, Wasén, C, Juzokaite, L, Leifsdottir, L, Erlandsson, MC, Silfverswärd, ST, et al. Inflammation in the hippocampus affects IGF1 receptor signaling and contributes to neurological sequelae in rheumatoid arthritis. Proc Natl Acad Sci USA. (2018) 115:E12063–72. doi: 10.1073/pnas.1810553115
28. Wartolowska, K, Hough, MG, Jenkinson, M, Andersson, J, Wordsworth, BP, and Tracey, I. Structural changes of the brain in rheumatoid arthritis. Arthritis Rheum. (2012) 64:371–9. doi: 10.1002/art.33326
29. Zikou, AK, Kosmidou, M, Astrakas, LG, Tzarouchi, LC, Tsianos, E, and Argyropoulou, MI. Brain involvement in patients with inflammatory bowel disease: a voxel-based morphometry and diffusion tensor imaging study. Eur Radiol. (2014) 24:2499–506. doi: 10.1007/s00330-014-3242-6
30. Cox, JG, de Groot, M, Cole, JH, Williams, SCR, and Kempton, MJ. A meta-analysis of structural MRI studies of the brain in systemic lupus erythematosus (SLE). Clin Rheumatol. (2022) 42:319–26. doi: 10.1007/s10067-022-06482-8
31. Monje, ML, Toda, H, and Palmer, TD. Inflammatory blockade restores adult hippocampal neurogenesis. Science. (2003) 302:1760–5. doi: 10.1126/science.1088417
32. Kornelsen, J, Witges, K, Labus, J, Mayer, EA, and Bernstein, CN. Brain structure and function changes in ulcerative colitis. Neuroimage. (2021) 1:100064. doi: 10.1016/j.ynirp.2021.100064
33. Miller, KL, Alfaro-Almagro, F, Bangerter, NK, Thomas, DL, Yacoub, E, Xu, J, et al. Multimodal population brain imaging in the UK biobank prospective epidemiological study. Nat Neurosci. (2016) 19:1523–36. doi: 10.1038/nn.4393
34. Alfaro-Almagro, F, Jenkinson, M, Bangerter, NK, Andersson, JLR, Griffanti, L, Douaud, G, et al. Image processing and quality control for the first 10,000 brain imaging datasets from UK biobank. Neuroimage. (2018) 166:400–24. doi: 10.1016/j.neuroimage.2017.10.034
35. Patenaude, B, Smith, SM, Kennedy, DN, and Jenkinson, M. A Bayesian model of shape and appearance for subcortical brain segmentation. Neuroimage. (2011) 56:907–22. doi: 10.1016/j.neuroimage.2011.02.046
36. Canavan, M, and O'Donnell, MJ. Hypertension and cognitive impairment: A review of mechanisms and key concepts. Front Neurol. (2022) 13:821135. doi: 10.3389/fneur.2022.821135
37. Seno, MDJ, Assis, DV, Gouveia, F, Antunes, GF, Kuroki, M, Oliveira, CC, et al. The critical role of amygdala subnuclei in nociceptive and depressive-like behaviors in peripheral neuropathy. Sci Rep. (2018) 8:13608. doi: 10.1038/s41598-018-31962-w
38. Mutso, AA, Radzicki, D, Baliki, MN, Huang, L, Banisadr, G, Centeno, MV, et al. Abnormalities in hippocampal functioning with persistent pain. J Neurosci. (2012) 32:5747–56. doi: 10.1523/JNEUROSCI.0587-12.2012
39. Fan, W, Zhang, S, Hu, J, Liu, B, Wen, L, Gong, M, et al. Aberrant Brain function in active-stage ulcerative colitis patients: A resting-state functional MRI study. Front Hum Neurosci. (2019) 13:107. doi: 10.3389/fnhum.2019.00107
40. Conrad, N, Verbeke, G, Molenberghs, G, Goetschalckx, L, Callender, T, Cambridge, G, et al. Autoimmune diseases and cardiovascular risk: a population-based study on 19 autoimmune diseases and 12 cardiovascular diseases in 22 million individuals in the UK. Lancet. (2022) 400:733–43. doi: 10.1016/S0140-6736(22)01349-6
41. Cafaro, G, Perricone, C, Gerli, R, and Bartoloni, E. Cardiovascular risk in systemic autoimmune diseases. Lancet. (2023) 401:21–2. doi: 10.1016/S0140-6736(22)02477-1
42. Panoulas, VF, Metsios, GS, Pace, AV, John, H, Treharne, GJ, Banks, MJ, et al. Hypertension in rheumatoid arthritis. Rheumatology. (2008) 47:1286–98. doi: 10.1093/rheumatology/ken159
43. Yarur, AJ, Deshpande, AR, Pechman, DM, Tamariz, L, Abreu, MT, and Sussman, DA. Inflammatory bowel disease is associated with an increased incidence of cardiovascular events. Am J Gastroenterol. (2011) 106:741–7. doi: 10.1038/ajg.2011.63
44. Czubkowski, P, Osiecki, M, Szymańska, E, and Kierkuś, J. The risk of cardiovascular complications in inflammatory bowel disease. Clin Exp Med. (2020) 20:481–91. doi: 10.1007/s10238-020-00639-y
45. Manavathongchai, S, Bian, A, Rho, YH, Oeser, A, Solus, JF, Gebretsadik, T, et al. Inflammation and hypertension in rheumatoid arthritis. J Rheumatol. (2013) 40:1806–11. doi: 10.3899/jrheum.130394
46. Xu, X, Ye, D, Liu, B, Yang, Y, Chen, Y, Qian, Y, et al. Assessing the impact of blood pressure in the development of inflammatory bowel disease. J Clin Hypertens. (2022) 24:566–72. doi: 10.1111/jch.14477
47. Agostini, A, Benuzzi, F, Filippini, N, Bertani, A, Scarcelli, A, Farinelli, V, et al. New insights into the brain involvement in patients with Crohn's disease: a voxel-based morphometry study. Neurogastroenterol Motil. (2013) 25:147–e82. doi: 10.1111/nmo.12017
48. Agostini, A, Campieri, M, Bertani, A, Scarcelli, A, Ballotta, D, Calabrese, C, et al. Absence of change in the gray matter volume of patients with ulcerative colitis in remission: a voxel based morphometry study. Biopsychosoc Med. (2015) 9:1. doi: 10.1186/s13030-014-0028-7
49. Bekkelund, SIPJC, Husby, G, and Mellgren, SI. Quantitative cerebral MR in rheumatoid arthritis. Am J Neuroradiol. (1995) 16:767–72.
50. Schrepf, A, Kaplan, CM, Ichesco, E, Larkin, T, Harte, SE, Harris, RE, et al. A multi-modal MRI study of the central response to inflammation in rheumatoid arthritis. Nat Commun. (2018) 9:2243. doi: 10.1038/s41467-018-04648-0
51. Basu, N, Kaplan, CM, Ichesco, E, Larkin, T, Schrepf, A, Murray, AD, et al. Functional and structural magnetic resonance imaging correlates of fatigue in patients with rheumatoid arthritis. Rheumatology. (2019) 58:1822–30. doi: 10.1093/rheumatology/kez132
52. Zhang, S, Chen, F, Wu, J, Liu, C, Yang, G, Piao, R, et al. Regional gray matter volume changes in brains of patients with ulcerative colitis. Inflamm Bowel Dis. (2022) 28:599–610. doi: 10.1093/ibd/izab252
53. Quezada, SM, and Cross, RK. Association of age at diagnosis and ulcerative colitis phenotype. Dig Dis Sci. (2012) 57:2402–7. doi: 10.1007/s10620-012-2081-z
54. Fry, A, Littlejohns, TJ, Sudlow, C, Doherty, N, Adamska, L, Sprosen, T, et al. Comparison of sociodemographic and health-related characteristics of UK biobank participants with those of the general population. Am J Epidemiol. (2017) 186:1026–34. doi: 10.1093/aje/kwx246
55. Zhu, Y, Yuan, M, Liu, Y, Yang, F, Chen, WZ, Xu, ZZ, et al. Association between inflammatory bowel diseases and Parkinson's disease: systematic review and meta-analysis. Neural Regen Res. (2022) 17:344–53. doi: 10.4103/1673-5374.317981
56. Kang, X, Ploner, A, Wang, Y, Ludvigsson, JF, Williams, DM, Pedersen, NL, et al. Genetic overlap between Parkinson’s disease and inflammatory bowel disease. Brain Commun. (2023) 5:fcad002. doi: 10.1093/braincomms/fcad002
57. Sangha, PS, Thakur, M, Akhtar, Z, Ramani, S, and Gyamfi, RS. The link between rheumatoid arthritis and dementia: a review. Cureus. (2020) 12:e7855. doi: 10.7759/cureus.7855
58. Trzeciak, P, Herbet, M, and Dudka, J. Common factors of Alzheimer’s disease and rheumatoid arthritis—pathomechanism and treatment. Molecules. (2021) 26:6038. doi: 10.3390/molecules26196038
59. Laakso, MP, Partanen, K, Riekkinen, P Jr, Lehtovirta, M, Helkala, EL, Hallikainen, M, et al. Hippocampal volumes in Alzheimer's disease, Parkinson's disease with and without dementia, and in vascular dementia: an MRI study. Neurology. (1996) 46:678–81. doi: 10.1212/WNL.46.3.678
Keywords: autoimmune diseases, brain volumetry, ulcerative colitis, rheumatoid arthritis, systemic inflammation, magnetic resonance imaging
Citation: Cox JG, de Groot M, Kempton MJ, Williams SCR and Cole JH (2024) Comparison of volumetric brain analysis in subjects with rheumatoid arthritis and ulcerative colitis. Front. Med. 11:1468910. doi: 10.3389/fmed.2024.1468910
Edited by:
Pankaj Gaur, Georgetown University, United StatesReviewed by:
Amar Kumar, University of Illinois Chicago, United StatesPavan Kumar, University of Illinois Chicago, United States
Copyright © 2024 Cox, de Groot, Kempton, Williams and Cole. This is an open-access article distributed under the terms of the Creative Commons Attribution License (CC BY). The use, distribution or reproduction in other forums is permitted, provided the original author(s) and the copyright owner(s) are credited and that the original publication in this journal is cited, in accordance with accepted academic practice. No use, distribution or reproduction is permitted which does not comply with these terms.
*Correspondence: Jennifer G. Cox, amVubmlmZXIuZy5jb3hAa2NsLmFjLnVr