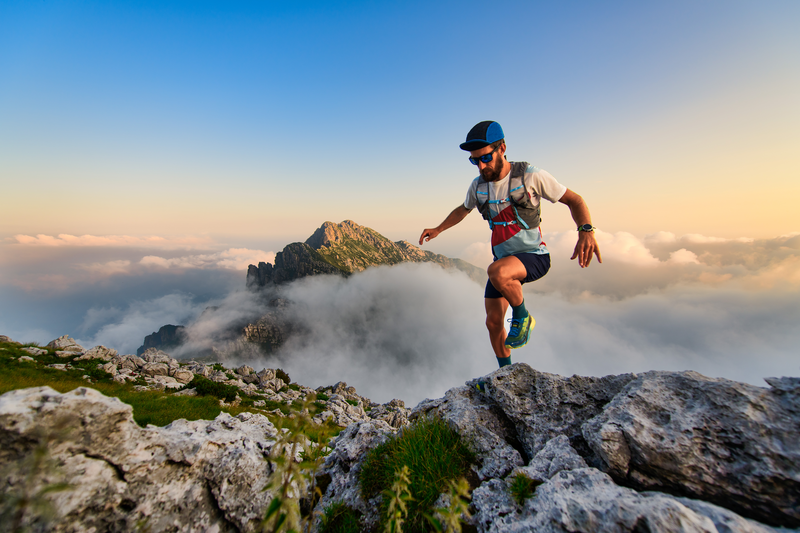
95% of researchers rate our articles as excellent or good
Learn more about the work of our research integrity team to safeguard the quality of each article we publish.
Find out more
ORIGINAL RESEARCH article
Front. Med. , 16 September 2024
Sec. Gastroenterology
Volume 11 - 2024 | https://doi.org/10.3389/fmed.2024.1462122
This article is part of the Research Topic Diagnosis and Management of Acute, Chronic, and Autoimmune Pancreatitis View all 10 articles
Background: Development of pancreatic necroses or pseudocysts are typical complications of pancreatitis and may require endoscopic drainage therapy using metal or plastic stents. Microbial infection of these lesions poses a major challenge. So far, the composition and significance of the microbial colonization on drainage stents are largely unknown although it may impact outcomes during endoscopic drainage therapy.
Methods: A total of 26 stents used for drainage of pancreatic lesions were retrieved and the stent microbiome was determined by 16S rRNA gene sequencing. Additional analysis included comparison of the stent microbiome to the intracavitary necrosis microbiome as well as scanning electron microscopy (SEM) and micro-computed tomography (μCT) imaging of selected metal or plastic stents.
Results: The stent microbiome comprises a large proportion of opportunistic enteric pathogens such as Enterococcus (14.4%) or Escherichia (6.1%) as well as oral bacteria like Streptococcus (13.1%). Increased levels of opportunistic enteric pathogens were associated with a prolonged hospital stay (r = 0.77, p = 3e−06) and the occurrence of adverse events during drainage therapy (p = 0.011). Higher levels of oral bacteria were associated (r = −0.62, p = 8e-04) with shorter durations of inpatient treatment. SEM and μCT investigations revealed complex biofilm networks on the stent surface.
Conclusion: The composition of the stent microbiome is associated with prolonged hospital stays and adverse events during endoscopic drainage therapy, highlighting the need for effective infection control to improve patient outcomes. In addition to systemic antibiotic therapy, antimicrobial stent coatings could be a conceivable option to influence the stent microbiome and possibly enhance control of the necrotic microflora.
Acute and chronic pancreatitis are common reasons for hospital admissions to gastroenterological wards (1), with abdominal pain being the primary symptom. The most frequent causes are excessive alcohol consumption or biliary obstruction, the latter only for acute pancreatitis. The development of pancreatic necrosis or pseudocysts are a feared complication in patients with pancreatitis, which is associated with significant morbidity and mortality (2). The revised Atlanta classification (3) categorizes necrosis and fluid collections of the pancreas into four different categories. Areas of pancreatic necrosis are called acute necrotic collection for up to 4 weeks, until they develop a thickened wall and are then named walled-off necrosis (WON). Liquid lesions without solid contents are called peripancreatic fluid collections within the first 4 weeks after the initial pancreatitis episode. When these lesions mature, they develop a well-defined wall and are called pseudocysts. Pancreatic necrosis or pseudocysts may become superinfected or cause complications such as biliary or gastric outlet obstruction or analgesics-resistant pain. In such cases, drainage may be indicated which is mostly being performed endoscopically via transgastric or transduodenal drainage, as these methods are associated with lower morbidity or mortality compared to (open) surgical approaches (2, 4–6). These procedures involve creating an opening through the gastric or duodenal wall to connect the necrosis or fluid collection with the gastrointestinal tract, thus allowing its drainage. Lumen-apposing metal stents (LAMS) or multiple pigtail plastic stents are then placed into the opening to avoid its closure or blockage. In cases of necrosis, this opening can then be used to perform repeated endoscopic necrosectomies as needed. One of the most important factors influencing the success of endoscopic drainage therapy and its complication rate is the presence of bacteria within the pancreatic collections (7). When a superinfection is present, rates of stent dislocations or obstructions, as well as residual lesions requiring repeated interventions are more common. This highlights the need of adequately controlling superinfections during endoscopic drainage therapy. Presently, there is some knowledge about the composition of microbial communities within pancreatic necrosis or pseudocysts but very little about the microbiome colonizing the stents used to drain them. We have recently shown that the necrosis microbiome usually consists of a multitude of different bacteria with a strong presence of gram-negative opportunistic enteric pathogens like Escherichia, Klebsiella, or Citrobacter and gram-positive opportunistic pathogens like Enterococcus as well as anaerobic bacteria like Bacteroides (8). These opportunistic enteric pathogens are part of the natural gut microbiome and usually do not cause disease. However, they can become pathogenic when the gut barrier is impaired, especially when they translocate to areas with compromised immune control, such as encapsulated cystic or necrotic lesions. To date, the composition and significance of the microbial colonization on stents used for drainage of pancreatic necrosis or pseudocysts are largely unknown. Investigations of the biofilm on drainage stents in the hepatopancreaticobiliary tract have so far been limited to those used to treat biliary obstruction, where diverse microbial communities have been identified (9). In the present study, we characterized the microbiome detectable on the stents used for endoscopic drainage therapy of pancreatic necroses or pseudocysts. We investigated the clinical significance of the stent microbiome, and analyzed possible differences to the necrosis microbiome. Moreover, we performed scanning electron microscopy and micro-computed tomography (CT) analyses of explanted drainage stents to determine microbial growth and stent degradation patterns.
Patients who underwent endoscopic drainage therapy of pancreatic necrosis or pseudocysts were prospectively recruited at the University Medicine Greifswald (Germany) in the period of March 2019 to June 2021. All participants provided written informed consent, and the study was approved by the ethics committee of the University Medicine Greifswald (III UV 91/03b). All methods were carried out in accordance with the relevant guidelines and regulations (Declaration of Helsinki).
Endoscopic ultrasound-guided transluminal drainage of pancreatic necroses or pseudocysts was performed in all 26 independent cases. The inclusion in this observational study had no impact on the modality of endoscopic drainage therapy. LAMS (HotAxios, Boston Scientific, Marlborough, MA, United States or SPAXUS, TaeWoong Medical, Ilsan, Korea) were used for the initial drainage in 23 cases and double-pigtail plastic stents in further three cases, according to the endoscopists choice. The stents were collected after a median time of 18.5 (10.8–45.5, 1st–3rd quartile) days. In case of WONs, additional tissue from the necrotic cavity was collected shortly before the removal of the stents. In four WON cases, however, no residual necrotic debris was left to collect before stent extraction.
Debris was collected from the inner stent surface and DNA was isolated using the PureLink Quick Plasmid Miniprep Kit (Invitrogen, Thermo Fisher Scientific, Waltham, MA, United States). 16S rRNA gene sequencing was then performed as previously described (10). In brief, amplification of the V1 and V2 regions of bacterial 16S rRNA genes was performed using the primer pair 27F and 338R and samples subsequently sequenced on a MiSeq platform (Illumina, San Diego, United States) using a dual-indexing approach. The open-source software package DADA2 (v.1.10) (11) was used for amplicon-data processing following the authors’ recommended procedure for large datasets1 as described before (12). This approach allows for single-nucleotide resolution of amplicons (amplicon sequence variants, ASVs). Data processing was adapted to the V1-V2 amplicon. Five bases were truncated from the 5′ end of the sequence on both reads. Forward and reverse reads were truncated to a length of 200 and 150 bp, respectively. A shorter resulting read length after truncation was possible if the sequence quality dropped below five. Read-pairs were discarded if they contained ambiguous bases, expected errors higher than 2 and when originating from PhiX spike-in. Error profiles were inferred using 1 million reads of the respective sequencing run, followed by dereplication, error correction and merging of forward and reverse reads. After creation of ASV abundance tables of all samples, chimeric amplicon sequences were identified and removed using the removeBimeraDenovo() function in consensus mode. For taxonomic annotation, a Bayesian classifier and the Ribosomal Database Project (RDP) training set version 16 were used. The resulting median read count of the stent microbiome samples was 10,531 (6,004-17,942; first-third quartile).
After extraction, stents were cut into two pieces along the longitudinal axis. One half of the stent was used for microbiological analysis as described above. The other half was prepared for scanning electron microscopy described before in detail (13). This included rinsing in 0.1 M sodium phosphate buffer (pH 7.4) and fixation with 2.5% glutaraldehyde/2% formaladehyde over night at 4°C. After fixation, the samples were rinsed in phosphate buffer before dehydration in ascending ethanol series (70–80% to 96–100%). Afterward, all samples were chemically dried using hexamethyldisiliazane. Scanning electron microscopy was performed on a Quanta FEG 250 (FEI Company, Germany). Prior to scanning, samples were sputter coated with gold.
The analysis of the distribution of necrotic debris on drainage stents was performed using a Skyscan 1273 (Bruker, United States) microCT system. To enhance contrast between the necrotic debris and the metal stent parts in the microCT, each stent was transferred to and kept overnight in Lugol’s solution (Carl Roth, Germany) at room temperature. Afterwards, excess solution was removed by placing the stent on tissue paper followed by airdrying overnight under the fume hood. For scanning, stents were placed on a specimen holder using double sided sticky tape. Stents were scanned using voltages between 55 and 100 kV and currents between 40 and 272 μA with an exposure time of 200 ms. Achieved resolutions were between 13 and 17 μm voxel size. For visualization of the acquired datasets either ImageJ (NIH, United States) or CTVox (Bruker, United States) were used.
Body mass index (BMI) was calculated by dividing the body weight in kilogram by the square of the body height. Patients were considered as smokers if they consumed at least one cigarette daily. The diameter of the necrotic or fluid lesions before drainage was measured using available imaging data (computed tomography or magnetic resonance imaging). Antibiotic treatment states if any course of antibiotics was taken during initial drainage therapy, not including single shot antibiotic periinterventional prophylaxis. The initial duration of hospital stay indicates the days of the first hospital stay, whereas the total duration of hospital stay also includes follow-up inpatient treatments that were directly linked to the endoscopic drainage therapy. Laboratory values for white blood cells, hemoglobin, platelet count, estimated glomerular filtration rate (eGFR), urea, and bilirubin were obtained on admission. In one pseudocyst drainage case, the bilirubin level was not available. For C-reactive protein we documented the highest value within the first 48 h.
All statistical analyses were performed using the statistical language “R” (v.4.3.2, https://www.R-project.org/). For microbiome related analyses, the bacterial read counts resulting from 16S rRNA gene sequencing were transformed into relative abundance data. The index “Bray–Curtis dissimilarity” was computed prior to ordination using the R package “vegan” (function “vegdist”) (14). Principal coordinate analysis (PCoA) was performed using the “vegan” function “cmdscale.” The “vegan” function “adonis” was used to perform permutational analysis of variance (PERMANOVA, 1,000 permutations) based on a Bray–Curtis dissimilarity. The two-sided Mann–Whitney test (MW, “stats” function “wilcox.test”) was applied for assessment of statistical significance in case of unpaired microbiome data, whereas the Wilcoxon signed-rank test was employed for paired data (WSR, “stats” function “wilcox.test,” paired = true). Spearman correlations between continuous phenotypes and microbial taxa were calculated using the “cor.test” function (“stats” package). p values <0.05 were considered significant.
The study cohort comprised 26 patients who underwent endoscopic drainage of pancreatic necroses or pseudocysts using LAMS or plastic stents. Table 1 shows the characteristics of these drainage cases of which 16 were performed to treat pancreatic necroses and 10 for pancreatic pseudocysts. Suspicion of infection was the most common indication (73.1%) for drainage. The median age was 58.0 years and 73.1% of patients were males. Alcohol abuse was the most common etiology for development of the underlying acute or chronic pancreatitis in 50.0% of cases. A total of 30.8% of patients were treated in an intensive care unit at some point during treatment. The mortality rate was 7.7%.
A total of 23 LAMS and three plastic stents were collected after being removed during pancreatic necrosis or pseudocyst drainage therapy and the stent microbiome was determined using 16S rRNA gene sequencing. Figure 1 shows that the most frequently occurring bacteria were Enterococcus, Streptococcus, Prevotella, Lactobacillus, and Escherichia together accounting for 52.2% of the total abundance (Supplementary Table S1). Taken together, typical gut microbial opportunistic enteric pathogens such as Enterococcus as well as gram-negative Escherichia, Citrobacter, Klebsiella, and Enterobacter comprised 24.8% of the bacteria. Microbes that are found in high abundance in the oral cavity (15) such as Streptococcus, Veillonella, Fusobacterium, Lactobacillus, Prevotella, and Haemophilus made up 42.4% of the stent microbiome. Permutational ANOVA revealed some differences between the stent microbiome of necrosis and pseudocyst stents (r2 = 7.9%, p = 0.024). Specifically, stents used for necrosis drainage showed higher abundance of Enterococcus (mean 20.2% vs. 5.1%, p = 0.007) and opportunistic enteric pathogens in general (mean 33.8% vs. 10.3%, p = 0.016) as compared to pseudocyst stents. Oral microbes were less abundant in necrosis stents with a mean abundance of 35.6% vs. 53.3% in pseudocyst stents, however, this was not significant (p = 0.109).
Figure 1. Stent microbiome composition. (A) Stacked bar plots show the average composition of the stent microbiome in all stents (left), necrosis stents (middle), and pseudocyst stents (right). (B) Boxplot shows the distribution of the 10 most abundant bacteria within the stent microbiome (all stents). The y-axis limits were set from 0 to 0.5 (50% abundance) for better display of the lesser abundant taxa.
The stent microbiome composition was associated with the initial (r2 = 14.2%, p < 0.001) and total length of hospital stay (r2 = 13.3%, p < 0.001). The associations with the initial and total length of hospital stay were also replicated in the subgroups of necrosis (r2 = 12.1%, p = 0.042 and r2 = 12.4%, p = 0.035, respectively) and pseudocyst drainage cases (r2 = 25.9%, p < 0.001 and r2 = 23.3, p = 0.008, respectively). More specifically, the 10 most abundant stent microbiome bacteria, as well as the groups of opportunistic enteric pathogens and typical oral microbes, were correlated with the length of hospital stay (Figure 2A; Supplementary Table S2). The analysis revealed a strong positive correlation between the presence of Enterococcus (rho = 0.72, p < 0.001 and rho = 0.69, p < 0.001) or in general opportunistic enteric pathogens (rho = 0.80, p < 0.001 and rho = 0.78, p < 0.001) with the initial and total length of hospital stay. The presence of oral bacteria (rho = −0.62, p < 0.001 and rho = −0.62, p < 0.001) was associated with shorter hospital stay durations. Specific bacteria from the oral microbiome such as Veillonella, Prevotella, or Streptococcus exhibited the same inverse associations with the initial or total length of hospital stay in the overall group or the subgroups of necrosis or pseudocyst drainage stents (Figure 2A; Supplementary Table S2).
Figure 2. Association of the stent microbiome with length of hospital stay and occurrence of adverse events during endoscopic drainage therapy. (A) Heatmap depicts the strength of the Spearman correlation (rho) between bacteria from the stent microbiome in all stents (left), necrosis stents (middle), and pseudocyst stents (right) and the length of the initial and total hospital stay. (B) Boxplots show the distribution of opportunistic enteric pathogens or oral bacteria of the stent microbiomes of cases without adverse events as compared to cases where adverse events occurred during endoscopic drainage therapy. *Indicates significant result (p < 0.05).
Adverse events such as instant or delayed bleeding, stent dislocation, buried LAMS, or residual lesions requiring a surgical intervention occurred in seven out of 26 cases undergoing endoscopic drainage therapy. These adverse events cases exhibited a different stent microbiome composition than the other uncomplicated cases (r2 = 8.0%, p = 0.022). Specifically, we found a higher abundance of opportunistic enteric pathogens (mean 53.3% vs. 14.3%, p = 0.011) and a lower abundance of oral bacteria (mean 25.0% vs. 48.7%, p = 0.026) in cases where adverse events occurred (Figure 2B).
A direct comparison of the stent microbiome with the corresponding necrotic cavity microbiome was performed in 12 patients from whom paired stent and necrosis sample could be obtained. Figure 3 shows a high similarity between the stent and the necrosis microbiome of these patients. PCoA indicated that the microbiomes of paired necrosis stent and necrotic cavity samples were positioned in closer proximity to each other as compared to unrelated samples. According to PERMANOVA, there was no major difference between the stent and the necrosis microbiome (r2 = 2.1%, p = 0.119). The stent microbiome merely yielded a higher abundance of Lactobacillus (mean 7.4% vs. 3.4%, p = 0.029) or typical oral microbes (mean 28.9% vs. 18.0%, p = 0.029) as compared to the necrosis microbiome (Supplementary Table S3).
Figure 3. Microbiome comparison of necrosis drainage stents and paired necrotic cavity samples. (A) Stacked bar plots show the composition of the microbiome in the necrotic cavity (left) and necrosis stents (right). (B) Principal coordinate analysis (PCoA) depicts necrotic cavity and necrosis drainage stents microbiomes. Paired samples are connected via a thin line and share the same color. They are located more closely to each other than unrelated samples.
To investigate whether the stent microbiome differs between the outer and inner surface, the microbiomes of both stent sides were determined in a subsample of six stents. There was no significant difference in the microbial community composition in terms of the most abundant microbes of the stent microbiome when comparing both sides as shown in Figure 4 and Supplementary Table S4.
Figure 4. Comparison of the inner and outer surface stent microbiome. (A) Stacked bar plots show the composition of the microbiome of the inner (left bars) and outer (right bars) stent surface of six necrosis or pseudocyst drainage stents. (B) Principal coordinate analysis (PCoA) displays the microbiomes of the inner and outer surface of different drainage stents. Paired samples are connected via a thin line and share the same color. They are located very closely to each other, indicating high similarity, as compared to unrelated samples.
To investigate the distribution of necrotic material and the bacterial biofilm on the stent surface, micro-computed tomography (μCT) analyses were performed on six extracted LAMS used for pancreatic necrosis drainage. Figure 5 shows a scattered distribution of the necrotic debris along the inner surfaces of the stents with the largest amount of necrotic content at both flanges.
Figure 5. Micro-computed tomography (μCT) analysis of six extracted lumen-apposing metal stents (LAMS). The stents were used for drainage of pancreatic necrotic collections. Necrotic debris (orange-red color) is mostly located at both flanges.
To further elucidate a possible link between the stent microbiome and the intensity of the degradation of the stent cover, scanning electron microscopy (SEM) of different LAMS (n = 10) and plastic stents (n = 3) was performed. As shown in Supplementary Figures S1, S2, degradation of the stent cover is present in all explanted LAMS as well as plastic stents. There was no apparent difference in the visual intensity of degradation in relation to the microbiome of the stent. Most stents showed dense bacterial populations. The dominating bacterial taxa, however, varied widely in the investigated stent explants, as demonstrated by the visually detectable different shapes and growth patterns of the stent microbial flora. Figure 6 shows exemplary SEM images of one double-pigtail plastic stent and three LAMS stents.
Figure 6. Examples of scanning electron microscopy investigations of stent surfaces. Shown are exemplary scanning electron microscopy images of three lumen-apposing metal stents (LAMS) and one double-pigtail plastic stent. All stents show distinct signs of surface degradation as well as bacterial overgrowth resulting in biofilms with complex meshworks.
We analyzed the bacterial composition on stents used for endoscopic drainage therapy of pancreatic necroses or pseudocysts to determine the stent-attached microbiome. The analyses showed that the stent microbiome mainly consists of two groups of bacteria: The first one consists of those that are highly abundant in the oral cavity (15) such as Streptococcus, Veillonella, Prevotella, Lactobacillus, or Fusobacterium whereas the second group comprises opportunistic enteric pathogens such as Enterococcus or Escherichia. We suggest that the group of opportunistic enteric pathogens colonized the stent surfaces secondarily from the necrotic cavity or fluid collection. Translocation of bacteria into areas of pancreatic necrosis or pseudocysts may occur via translocation of luminal intestinal bacteria, via bloodstream or ascending through the lymphatic system. Patients with acute or chronic pancreatitis are particularly vulnerable to translocation events because their dysbiotic gut microbiome is enriched with opportunistic enteric pathogens, such as Enterococcus and other gram-negative bacteria like Escherichia (16–20). This can partly be explained by the prominent role of the exocrine pancreas in regulating the gut microbiome composition (10, 12). Moreover, during the course of acute pancreatitis local und systemic immunosuppression and a disturbed gut barrier function promote translocation of gut bacteria into areas of necrosis (8).
We have recently shown that a higher proportion of Enterococcus in the pancreatic necrosis microbiome correlates with longer hospital stays of patients with necrotizing pancreatitis (8). Also, the rate of adverse events during endoscopic drainage therapy is associated with infected pancreatic necrosis (based on necrosis culture) (7). In the present analysis, patients harboring a stent microbiome with high abundance of opportunistic enteric pathogens had longer hospital stays and were more likely to experience adverse events such as instant or delayed bleeding, stent dislocation, buried LAMS, or residual lesions during endoscopic drainage therapy. The presence of opportunistic enteric pathogens in areas of pancreatic necrosis or pseudocyst can have multiple detrimental effects. First, the local infection drives systemic inflammation and may trigger recurring septicemia. This is complicated by the fact that the host’s immune system is severely compromised in these encapsulated lesions, which is why endoscopic drainage may be required. Another challenge posed by these bacteria, even after drainage, is their ability for agglutination and biofilm formation. Lipopolysaccharides (LPS), which are an integral part of the outer membrane of gram-negative Enterobacteria such as Escherichia, play an important role in the initiation of biofilm formation (21, 22), which in turn may explain the increased rates of stent obstruction and residual lesions associated with infected pancreatic necrosis during endoscopic drainage therapy (7). Likewise the gram-positive bacterium Enterococcus faecalis induces local inflammation, protects itself from immune clearance via the multiple peptide resistance factor and delays wound healing (23). Taken together, these mechanisms may also delay contraction of the necrotic cavity or pseudocyst and possibly promote events of stent dislocation.
While a greater abundance of opportunistic enteric pathogens correlated with longer disease duration, we found that stent microbiomes enriched with bacteria that are frequently found in the oral microbiome, such as Streptococcus, Veillonella, Fusobacterium, Lactobacillus, Prevotella, and Haemophilus (15), were associated with shorter hospital stays and the absence of adverse events during endoscopic drainage therapy. These bacteria also account for the largest proportion of bacteria found in the gastric juice (24). Therefore, we hypothesize that oral bacteria secondarily colonize the stent surfaces, and to some extent the necrotic or pseudocyst cavity, after a connection to the gastrointestinal tract is being established by transgastric or transduodenal stent placement. Correspondingly, the stent microbiome contained a higher abundance of oral bacteria as compared to matched pancreatic necrotic cavity samples, the probable reason being the luminal proximity of the stent. Whether the presence of oral microbes among the stent microbiome is of pathophysiological relevance is currently unclear. On the one hand, it would be conceivable that these oral microbes suppress the growth of opportunistic enteric pathogens if present in abundance, promote successful endoscopic drainage therapy and lead to shorter hospital stays. On the other hand, a strong presence of oral bacteria in the stent microbiome could result from the absence of competing opportunistic enteric pathogens and have now pathophysiological relevance.
Tackling infected pancreatic necrosis or fluid collections has always been a therapeutic challenge and various approaches have tried to alleviate the disease burden. Attempts to avoid infection of pancreatic necrosis in the first place using antibiotic prophylaxis did not result in a significant reduction of infected pancreatic necrosis or mortality as shown in a recent meta-analysis (25). However, there is no debate that broad-spectrum antibiotics should be administered when there is suspicion of infected pancreatic necrosis or septicemia (26). Yet, in case of infected pancreatic collections, antibiotics alone are often insufficient and additional endoscopic drainage is required (26). LAMS have become the first choice in drainage of pancreatic necrosis containing large amounts of necrotic debris, whereas multiple plastic stents are still used for lesions with little solid content (27). The obvious advantage of LAMS is its larger diameter that may facilitate clearance of larger pieces of necrotic debris and also enables the endoscopist to perform repeated necrosectomies without having to exchange stents. Yet, even with an optimal antibiotic and endoscopic drainage therapy, morbidity and mortality in infected pancreatic necrosis remain significant (28). One possible concept to improve the clinical results of endoscopic drainage therapy is the utilization of antimicrobial stent coatings which have already been proposed for biliary stents e.g., to avoid cholangitis after endoscopic retrograde cholangiography (ERC) (29, 30). Similarly, stents for endoscopic drainage therapy of pancreatic necrosis or fluid collections could be coated with antimicrobial agents, potentially improving control of infection within the necrotic cavity, thus reducing the rate of drainage therapy associated complications. For such an approach, it is important to determine where on the stent the coating should be applied and to define the bacterial spectrum that needs to be effectively covered. Our SEM micrographs showed complex network-like biofilm formation on almost all stent explants. Further μCT analyses showed that adhesive necrotic debris can be found on various spots along all of the stent surface on the inside as well as on the outside. Comparative analysis of the composition of the stent microbiome on the inner and outer surface showed no major differences. Therefore, a homogenous antimicrobial coating that covers all stent surfaces could be conceived. Its antimicrobial activity would primarily need to cover gram-negative Enterobacteriaceae like Escherichia or Citrobacter as well as Enterococcus as these were the most abundant opportunistic enteric pathogens present. To this end, an antimicrobial coating e.g., with gentamicin, which has already been preclinically tested for use with plastic biliary stents (29), could be an option. Gentamicin possesses antimicrobial bactericidal activity against the aforementioned bacteria (31) and it works synergistically with other antimicrobial compounds (e.g., ß-lactam antibiotics) that may be used for systemic therapy (32, 33). A localized gentamicin application would also allow to achieve higher local concentrations than would be tolerable if administered systemically. However, before this approach could be implemented in clinical practice, an antimicrobial coating system for drainage stents would have to be developed that ensures a uniform release of the antimicrobial agent into the stent environment. Further preclinical trials will need to investigate the duration of the antimicrobial effect to determine the appropriate replacement intervals for the stents. Last, it would have to be shown in vivo that stents with an antimicrobial coating can in fact influence the stent microbiome and possibly also the microbiome of the necrotic cavity before investigating its effect on clinical outcomes in a randomized-controlled trial.
In the present study, we investigated the composition of the stent microbiome, elucidated differences between the microbiome of the stent and that of the pancreatic necrotic cavity and performed SEM and μCT imaging of stent explants to examine the distribution patterns of pancreatic debris and biofilm formation. However, despite these thorough analyses, this study also has some limitations: First, this is a single-center study, which limits the sample size and reduces the statistical power to detect small differences or weaker clinical associations. Secondly, the composition of the stent microbiome prior to the intervention remains unknown, as the stents are usually retrieved several weeks after initial placement. However, since the microbiome of pancreatic necrosis is relatively stable over time, as we have shown before (8), the same could be assumed for the microbiome of the stent.
In summary, we have determined the microbiome composition on stents used for drainage therapy of pancreatic necrosis or pseudocysts. The resulting microbiome consisted predominantly of opportunistic enteric pathogens as well as oral bacteria. The presence of opportunistic enteric pathogens was associated with prolonged hospitalization whereas stent microbiomes dominated by oral bacteria indicated uncomplicated disease. Our data not only highlight the need for infection control in patients undergoing pancreatic endoscopic drainage therapy, but also suggests the possibility of applying antimicrobial coatings to drainage stents to improve local control of infection.
The datasets presented in this study can be found in online repositories. The names of the repository/repositories and accession number(s) can be found in the article/Supplementary material.
The studies involving humans were approved by Ethics committee of the University Medicine Greifswald. The studies were conducted in accordance with the local legislation and institutional requirements. The participants provided their written informed consent to participate in this study.
FF: Writing – review & editing, Writing – original draft, Visualization, Validation, Supervision, Resources, Project administration, Methodology, Investigation, Funding acquisition, Formal analysis, Data curation, Conceptualization. VK: Writing – review & editing, Writing – original draft, Methodology, Formal analysis, Data curation. VS: Writing – review & editing, Writing – original draft, Methodology, Formal analysis, Data curation. SW: Writing – review & editing, Writing – original draft, Visualization, Methodology, Investigation, Formal analysis. BK-F: Writing – review & editing, Writing – original draft, Methodology, Investigation, Formal analysis. MR: Writing – review & editing, Writing – original draft, Methodology, Investigation, Data curation. CBa: Writing – review & editing, Writing – original draft, Project administration, Methodology, Investigation. AF: Writing – review & editing, Writing – original draft, Supervision, Resources, Project administration, Funding acquisition. TP: Writing – review & editing, Writing – original draft, Supervision, Investigation. CBu: Writing – review & editing, Writing – original draft, Investigation. AA: Writing – review & editing, Writing – original draft, Investigation. SS: Writing – review & editing, Writing – original draft, Investigation, Formal analysis, Data curation. FW: Writing – review & editing, Writing – original draft, Supervision, Resources, Project administration, Methodology. NG: Writing – review & editing, Writing – original draft, Supervision, Resources, Project administration, Funding acquisition. ML: Writing – review & editing, Writing – original draft, Supervision, Resources, Project administration, Funding acquisition, Conceptualization. MS: Writing – review & editing, Writing – original draft, Supervision, Resources, Project administration, Methodology, Investigation, Formal analysis, Conceptualization.
The author(s) declare that financial support was received for the research, authorship, and/or publication of this article. This work was supported by the RESPONSE project (BMBF grant numbers 03ZZ0921A, 03ZZ0921B, and 03ZZ0921E, and 03ZZ0931A, 03ZZ0931D, and 03ZZ0931F).
We thank Katja Hahn, Babette Hummel, Jenny Radel, and Susanne Wiche for expert technical assistance.
The authors declare that the research was conducted in the absence of any commercial or financial relationships that could be construed as a potential conflict of interest.
All claims expressed in this article are solely those of the authors and do not necessarily represent those of their affiliated organizations, or those of the publisher, the editors and the reviewers. Any product that may be evaluated in this article, or claim that may be made by its manufacturer, is not guaranteed or endorsed by the publisher.
The Supplementary material for this article can be found online at: https://www.frontiersin.org/articles/10.3389/fmed.2024.1462122/full#supplementary-material
1. Peery, AF, Crockett, SD, Murphy, CC, Jensen, ET, Kim, HP, Egberg, MD, et al. Burden and cost of gastrointestinal, liver, and pancreatic diseases in the United States: update 2021. Gastroenterology. (2022) 162:621–44. doi: 10.1053/j.gastro.2021.10.017
2. van Santvoort, HC, Bakker, OJ, Bollen, TL, Besselink, MG, Ahmed Ali, U, Schrijver, AM, et al. A conservative and minimally invasive approach to necrotizing pancreatitis improves outcome. Gastroenterology. (2011) 141:1254–63. doi: 10.1053/j.gastro.2011.06.073
3. Banks, PA, Bollen, TL, Dervenis, C, Gooszen, HG, Johnson, CD, Sarr, MG, et al. Classification of acute pancreatitis--2012: revision of the Atlanta classification and definitions by international consensus. Gut. (2013) 62:102–11. doi: 10.1136/gutjnl-2012-302779
4. van Brunschot, S, Hollemans, RA, Bakker, OJ, Besselink, MG, Baron, TH, Beger, HG, et al. Minimally invasive and endoscopic versus open necrosectomy for necrotising pancreatitis: a pooled analysis of individual data for 1980 patients. Gut. (2018) 67:gutjnl-2016-313341–706. doi: 10.1136/gutjnl-2016-313341
5. van Brunschot, S, van Grinsven, J, van Santvoort, HC, Bakker, OJ, Besselink, MG, Boermeester, MA, et al. Endoscopic or surgical step-up approach for infected necrotising pancreatitis: a multicentre randomised trial. Lancet. (2018) 391:51–8. doi: 10.1016/S0140-6736(17)32404-2
6. Bang, JY, Arnoletti, JP, Holt, BA, Sutton, B, Hasan, MK, Navaneethan, U, et al. An endoscopic transluminal approach, compared with minimally invasive surgery, reduces complications and costs for patients with necrotizing pancreatitis. Gastroenterology. (2019) 156:1027–1040.e3. doi: 10.1053/j.gastro.2018.11.031
7. Frost, F, Schlesinger, L, Wiese, ML, Urban, S, Von Rheinbaben, S, Tran, QT, et al. Infection of (Peri-)pancreatic necrosis is associated with increased rates of adverse events during endoscopic drainage: a retrospective study. J Clin Med. (2022) 11:5851. doi: 10.3390/jcm11195851
8. Glaubitz, J, Wilden, A, Frost, F, Ameling, S, Homuth, G, Mazloum, H, et al. Activated regulatory T-cells promote duodenal bacterial translocation into necrotic areas in severe acute pancreatitis. Gut. (2023) 72:1355–69. doi: 10.1136/gutjnl-2022-327448
9. Blanco-Míguez, A, Carloni, S, Cardenas, C, Dioguardi, CC, Lambroia, L, Capretti, G, et al. Microbial composition associated with biliary stents in patients undergoing pancreatic resection for cancer. NPJ Biofilms Microbiomes. (2024) 10:35. doi: 10.1038/s41522-024-00506-8
10. Frost, F, Kacprowski, T, Rühlemann, M, Bülow, R, Kühn, J-P, Franke, A, et al. Impaired exocrine pancreatic function associates with changes in intestinal microbiota composition and diversity. Gastroenterology. (2019) 156:1010–5. doi: 10.1053/j.gastro.2018.10.047
11. Callahan, BJ, McMurdie, PJ, Rosen, MJ, Han, AW, Johnson, AJA, and Holmes, SP. DADA2: high-resolution sample inference from Illumina amplicon data. Nat Methods. (2016) 13:581–3. doi: 10.1038/nmeth.3869
12. Frost, F, Kacprowski, T, Rühlemann, M, Pietzner, M, Bang, C, Franke, A, et al. Long-term instability of the intestinal microbiome is associated with metabolic liver disease, low microbiota diversity, diabetes mellitus and impaired exocrine pancreatic function. Gut. (2021) 70:522–30. doi: 10.1136/gutjnl-2020-322753
13. Khaimov, V, Frost, F, Lerch, MM, Senz, V, Grabow, N, and Schmitz, K-P. Systematic microscopic analysis of retrieved stents from a patient with pancreatic necrosis. Curr Direct Biomed Eng. (2020) 6:450–3. doi: 10.1515/cdbme-2020-3116
14. Oksanen, FJ, Blanchet, G, Friendly, M, Kindt, R, Legendre, P, and McGlinn, PD (2020). vegan: Community Ecology Package. R package version 2.5-7. Available online at: https://CRAN.R-project.org/package=vegan
15. Human Microbiome Project Consortium. Structure, function and diversity of the healthy human microbiome. Nature. (2012) 486:207–14. doi: 10.1038/nature11234
16. Frost, F, Weiss, FU, Sendler, M, Kacprowski, T, Rühlemann, M, Bang, C, et al. The gut microbiome in patients with chronic pancreatitis is characterized by significant Dysbiosis and overgrowth by opportunistic pathogens. Clin Transl Gastroenterol. (2020) 11:e00232. doi: 10.14309/ctg.0000000000000232
17. Zhu, Y, He, C, Li, X, Cai, Y, Hu, J, Liao, Y, et al. Gut microbiota dysbiosis worsens the severity of acute pancreatitis in patients and mice. J Gastroenterol. (2019) 54:347–58. doi: 10.1007/s00535-018-1529-0
18. Zhou, C-H, Meng, Y-T, Xu, J-J, Fang, X, Zhao, J-L, Zhou, W, et al. Altered diversity and composition of gut microbiota in Chinese patients with chronic pancreatitis. Pancreatology. (2020) 20:16–24. doi: 10.1016/j.pan.2019.11.013
19. Jandhyala, SM, Madhulika, A, Deepika, G, Rao, GV, Reddy, DN, Subramanyam, C, et al. Altered intestinal microbiota in patients with chronic pancreatitis: implications in diabetes and metabolic abnormalities. Sci Rep. (2017) 7:43640. doi: 10.1038/srep43640
20. Yu, S, Xiong, Y, Fu, Y, Chen, G, Zhu, H, Mo, X, et al. Shotgun metagenomics reveals significant gut microbiome features in different grades of acute pancreatitis. Microb Pathog. (2021) 154:104849. doi: 10.1016/j.micpath.2021.104849
21. Ruhal, R, and Kataria, R. Biofilm patterns in gram-positive and gram-negative bacteria. Microbiol Res. (2021) 251:126829. doi: 10.1016/j.micres.2021.126829
22. Nakao, R, Ramstedt, M, Wai, SN, and Uhlin, BE. Enhanced biofilm formation by Escherichia coli LPS mutants defective in Hep biosynthesis. PLoS One. (2012) 7:e51241. doi: 10.1371/journal.pone.0051241
23. Chong, KKL, Tay, WH, Janela, B, Yong, AMH, Liew, TH, Madden, L, et al. Enterococcus faecalis modulates immune activation and slows healing during wound infection. J Infect Dis. (2017) 216:1644–54. doi: 10.1093/infdis/jix541
24. Schulz, C, Schütte, K, Koch, N, Vilchez-Vargas, R, Wos-Oxley, ML, Oxley, APA, et al. The active bacterial assemblages of the upper GI tract in individuals with and without Helicobacter infection. Gut. (2018) 67:216–25. doi: 10.1136/gutjnl-2016-312904
25. Ding, N, Sun, Y-H, Wen, L-M, Wang, J-H, Yang, J-H, Cheng, K, et al. Assessment of prophylactic antibiotics administration for acute pancreatitis: a meta-analysis of randomized controlled trials. Chin Med J. (2020) 133:212–20. doi: 10.1097/CM9.0000000000000603
26. Baron, TH, DiMaio, CJ, Wang, AY, and Morgan, KA. American Gastroenterological Association clinical practice update: management of pancreatic necrosis. Gastroenterology. (2020) 158:67–75.e1. doi: 10.1053/j.gastro.2019.07.064
27. Angadi, S, Mahapatra, SJ, Sethia, R, Elhence, A, Krishna, A, Gunjan, D, et al. Endoscopic transmural drainage tailored to quantity of necrotic debris versus laparoscopic transmural internal drainage for walled-off necrosis in acute pancreatitis: a randomized controlled trial. Pancreatology. (2021) 21:1291–8. doi: 10.1016/j.pan.2021.06.006
28. Yasuda, I, and Takahashi, K. Endoscopic management of walled-off pancreatic necrosis. Dig Endosc. (2021) 33:335–41. doi: 10.1111/den.13699
29. Obermeier, A, Würstle, S, Tübel, J, Stolte, P, Feihl, S, Lipovcic, N, et al. Novel antimicrobial coatings based on polylactide for plastic biliary stents to prevent post-endoscopic retrograde cholangiography cholangitis. J Antimicrob Chemother. (2019) 74:1911–20. doi: 10.1093/jac/dkz128
30. Yamabe, A, Irisawa, A, Wada, I, Shibukawa, G, Fujisawa, M, Sato, A, et al. Application of a silver coating on plastic biliary stents to prevent biofilm formation: an experimental study using electron microscopy. Endosc Int Open. (2016) 4:E1090–5. doi: 10.1055/s-0042-115173
31. Lefort, A, Arthur, M, Garry, L, Carbon, C, Courvalin, P, and Fantin, B. Bactericidal activity of gentamicin against Enterococcus faecalis in vitro and in vivo. Antimicrob Agents Chemother. (2000) 44:2077–80. doi: 10.1128/AAC.44.8.2077-2080.2000
32. Dressel, DC, Tornatore-Reuscher, MA, Boschman, CR, Stosor, V, Zembower, T, Postelnick, MJ, et al. Synergistic effect of gentamicin plus ampicillin on enterococci with differing sensitivity to gentamicin: a phenotypic assessment of NCCLS guidelines. Diagn Microbiol Infect Dis. (1999) 35:219–25. doi: 10.1016/s0732-8893(99)00088-7
Keywords: acute pancreatitis, bacteria, pancreatic necrosis, necrosis microbiome, microbiota, LAMS, WON, WOPN
Citation: Frost F, Khaimov V, Senz V, Weiss S, Klußmann-Fricke B, Rühlemann M, Bang C, Franke A, Pickartz T, Budde C, Aghdassi AA, Siewert S, Weiss FU, Grabow N, Lerch MM and Sendler M (2024) The composition of the stent microbiome is associated with morbidity and adverse events during endoscopic drainage therapy of pancreatic necroses and pseudocysts. Front. Med. 11:1462122. doi: 10.3389/fmed.2024.1462122
Received: 09 July 2024; Accepted: 02 September 2024;
Published: 16 September 2024.
Edited by:
Ravi Kumar Sharma, Chandigarh University, IndiaReviewed by:
Mateusz Jagielski, Nicolaus Copernicus University in Toruń, PolandCopyright © 2024 Frost, Khaimov, Senz, Weiss, Klußmann-Fricke, Rühlemann, Bang, Franke, Pickartz, Budde, Aghdassi, Siewert, Weiss, Grabow, Lerch and Sendler. This is an open-access article distributed under the terms of the Creative Commons Attribution License (CC BY). The use, distribution or reproduction in other forums is permitted, provided the original author(s) and the copyright owner(s) are credited and that the original publication in this journal is cited, in accordance with accepted academic practice. No use, distribution or reproduction is permitted which does not comply with these terms.
*Correspondence: Fabian Frost, ZmFiaWFuLmZyb3N0QG1lZC51bmktZ3JlaWZzd2FsZC5kZQ==
†These authors have contributed equally to this work and share senior authorship
Disclaimer: All claims expressed in this article are solely those of the authors and do not necessarily represent those of their affiliated organizations, or those of the publisher, the editors and the reviewers. Any product that may be evaluated in this article or claim that may be made by its manufacturer is not guaranteed or endorsed by the publisher.
Research integrity at Frontiers
Learn more about the work of our research integrity team to safeguard the quality of each article we publish.