- 1Service d’Anesthésie Réanimation, Hôpital Universitaire Louis Pradel, Hospices Civils de Lyon, Lyon, France
- 2Laboratoire CarMeN, Inserm UMR 1060, Université Claude Bernard Lyon 1, Lyon, France
Cardiac surgery with cardiopulmonary bypass results in global myocardial ischemia–reperfusion injury, leading to significant postoperative morbidity and mortality. Although cardioplegia is the cornerstone of intraoperative cardioprotection, a number of additional strategies have been identified. The concept of preconditioning and postconditioning, despite its limited direct clinical application, provided an essential contribution to the understanding of myocardial injury and organ protection. Therefore, physicians can use different tools to limit perioperative myocardial injury. These include the choice of anesthetic agents, remote ischemic preconditioning, tight glycemic control, optimization of respiratory parameters during the aortic unclamping phase to limit reperfusion injury, appropriate choice of monitoring to optimize hemodynamic parameters and limit perioperative use of catecholamines, and early reintroduction of cardioprotective agents in the postoperative period. Appropriate management before, during, and after cardiopulmonary bypass will help to decrease myocardial damage. This review aimed to highlight the current advancements in cardioprotection and their potential applications during cardiac surgery.
1 Introduction
Cardiac surgery with cardiopulmonary bypass (CPB) includes an expected ischemia–reperfusion (I/R) sequence, leading to myocardial injury and significant postoperative morbidity and mortality. The unavoidable and sustained elevation of postoperative serum troponin is multifactorial because of direct surgical trauma, systemic inflammation, and reversible ischemia or irreversible necrosis. Its short- and long-term prognosis value has been validated for years (1–3). Irrespective of the surgical procedure itself, the early or late elevation of peak serum troponin I has probably a distinct meaning, with a pejorative value in cases of elevation beyond the 24th postoperative hour (4, 5). A large prospective cohort study recently demonstrated that threshold values of hypersensitive troponin I above 5,670 ng/L on postoperative day 1 after coronary artery bypass grafting (CABG) or aortic valve surgery and above 12,981 ng/L following other cardiac surgeries were associated with increased mortality at day 30 (6). A whole literature on myocardial protection techniques during cardiac surgery has therefore emerged. While cardioplegia remains the cornerstone of intraoperative cardioprotection, the lack of consensus on its practice is striking. The concept of cardioplegia is based on the administration of a hyperkalemic solution that induces a rapid diastolic arrest by depolarizing cardiomyocytes. Different solutions and components (crystalloids, warm or cold blood cardioplegia, anterograde, retrograde, or combined delivery approaches) are used by surgical teams and make synthesis difficult (7). It is beyond the scope of this review to provide a detailed overview of the different cardioplegia solutions and practices. In this update, we will focus on other cardioprotection approaches administered during cardiac surgery that have been developed in addition to cardioplegia techniques.
2 The concept of preconditioning and postconditioning
In 1986, Murry et al. (8) published an experimental study that had a major impact and proved to be a turning point in the field of cardioprotection. Using an in vivo animal model, they demonstrated that four brief sequences of 5 min I/R, applied just before a lethal 40-min ischemia, reduced the size of myocardial infarction by more than 75% (Figure 1). The ischemic preconditioning concept was born. Then, we learned that patients presenting with an inaugural myocardial infarction have greater myocardial damage than those whose infarction is preceded by a period of angina (9). Shortly after, Ishihara et al. (10) demonstrated that prodromal angina occurring before the onset of infarction had a beneficial effect on long-term prognosis. The human myocardium is therefore just as receptive as the animal myocardium to the preconditioning signal. It is possible to modify the phenotype of the myocardium before an ischemic process so that it increases its tolerance to oxygen deprivation. By shifting the time-dependent necrosis curve to the right, preconditioning reduces the lesion for a given time.
Myocardial reperfusion, while essential to limit the infarct size, can itself induce injury, thereby reducing its expected beneficial effects (11). It is described as a double-edged sword. Several abrupt metabolic and biochemical changes occur within minutes of reperfusion, including the generation of reactive oxygen species (ROS), intracellular calcium overload, and rapid restoration of normal intracellular pH. In 2003, Zhao et al. (12) demonstrated that the application of brief I/R sequences in the early stages of reperfusion limited myocardial injury. By analogy with the preconditioning phenomenon, this strategy applied following ischemia is known as ischemic postconditioning (Figure 1) and has been described in humans. Indeed, in patients with ongoing acute myocardial infarction, necrosis size has been significantly reduced by 36% using sequential re-inflation of the intracoronary balloon upstream of the stenting zone (13). Moreover, postconditioning by angioplasty has shown better long-term benefits in patients with acute myocardial infarction, including a reduction in infarct size at 6 months and an improvement in myocardial contractile function at 1 year (14).
Preconditioning and postconditioning are ubiquitous processes that protect organs against I/R injury. Many organs other than the myocardium (such as the brain, lung, kidney, digestive tract, and skeletal muscle) respond to conditioning. We also know that the various protective signals are transmitted remotely within the body via neuronal and humoral pathways, a process known as remote ischemic preconditioning (RIPC, Figure 2) (15). Finally, preconditioning can protect an organ for up to 24–72 h before an ischemic episode. This phenomenon, known as the late phase of preconditioning, follows the activation of protein neo-syntheses such as nitric oxide (NO) synthases and cyclooxygenase-2 (COX-2) in response to the administration of a signal-inducing protection (16). A vast body of literature has been devoted to deciphering the cellular mechanisms of conditioning (17, 18). Experimental studies carried out on both in vivo and in vitro models (isolated perfused heart, cell culture preparation, etc.) clarified the more intimate mechanisms (Figure 3). In brief, the protective signal travels to the cellular level, involving various surface receptors (notably those coupled with inhibitory G proteins), leading to the production of diacylglycerol, the activation of different protein kinases (such as protein kinase C), and the opening of various channels, such as ATP-gated potassium channels (KATP). This information will then trigger different protective cascades via the reperfusion injury salvage kinases (RISK) and survivor activating factor enhancement (SAFE) pathways to lead to intracellular mitochondrial effectors. In the event of I/R, those organelles are the site of membrane permeabilization, known as the mitochondrial permeability transition pore (mPTP) opening (19). Permeabilization induces mitochondrial swelling, which causes them to lose their capacity to produce ATP at the level of the respiratory chain, ultimately dislocating within the cytoplasm and releasing pro-apoptotic substances such as cytochrome C. Conditioning works through several mechanisms: (i) delaying the mPTP opening, in particular through its action on a matrix protein (cyclophilin D); (ii) modifying the ROS production at the level of the mitochondrial respiratory chain (inhibition of complex I); and (iii) acting at the mitochondria-associated membrane, junction zones between the mitochondria, and the sarcoplasmic reticulum (20–23).
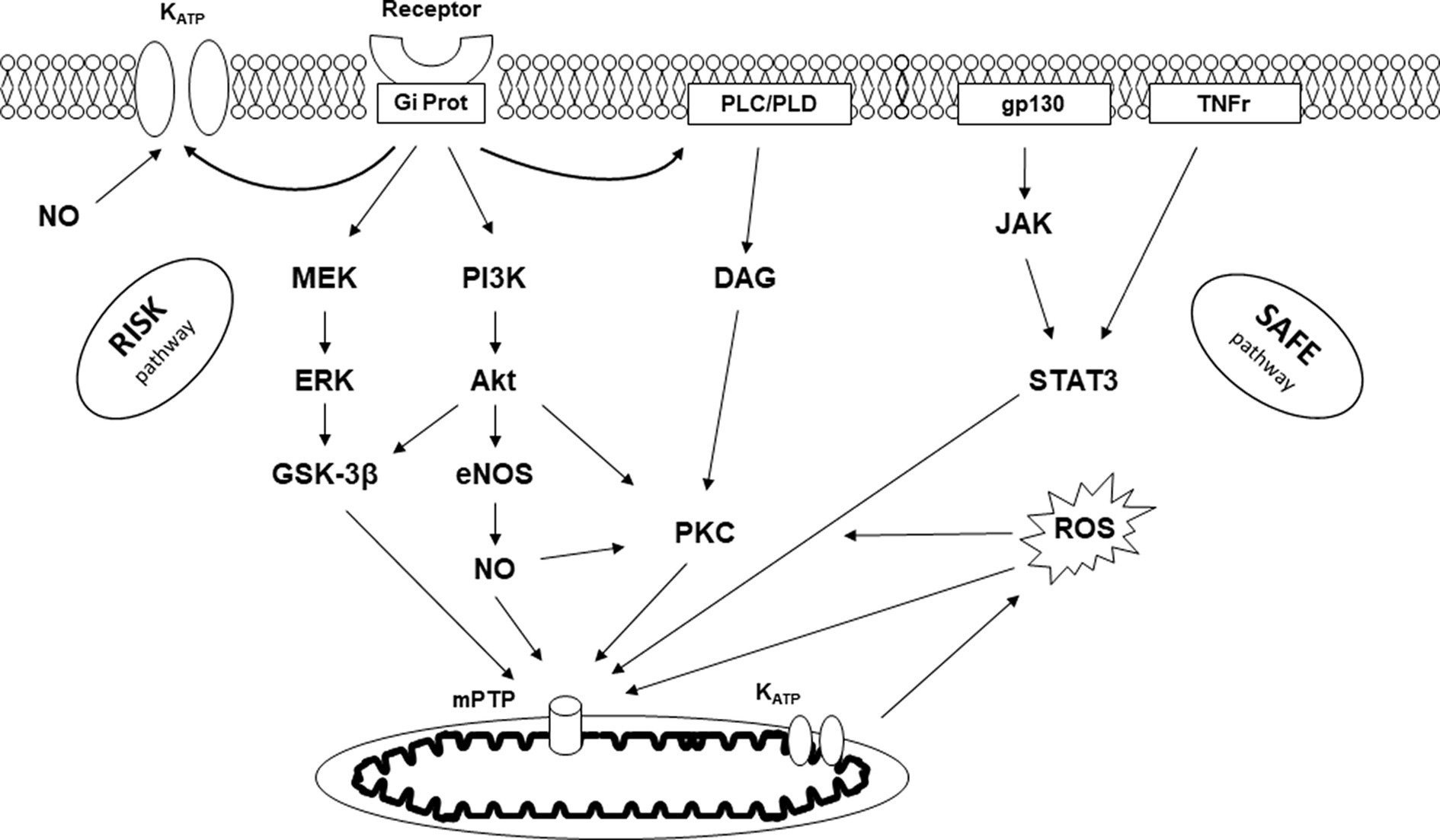
Figure 3. Signaling pathway of conditioning. KATP, ATP-dependent potassium channel; Gi Prot, inhibitory G protein; PLC/PLD, phospholipase C/D; gp130, glycoprotein 130; TNFr, tumor necrosis factor receptor; RISK, reperfusion injury salvage kinase; SAFE, survivor activating factor enhancement; MEK, mitogen-activated protein kinase; ERK, extracellular signal-regulated kinase; GSK-3β, glycogen synthase kinase-3β; PI3K, phosphatidylinositol-3-kinase; Akt, protein kinase B; NO, nitric oxide; eNOS, endothelial NO synthase; DAG, diacylglycerol; PKC, protein kinase C; JAK, Janus kinase; STAT3, signal transducer and activator of transcription 3; ROS, radical oxygen species; mPTP, mitochondrial permeability transition pore.
3 Volatile halogenated agents-induced myocardial protection
3.1 Experimental approach to myocardial conditioning with volatile halogenated agents
Ischemic conditioning has little direct clinical application in cardiac surgery. Numerous experimental studies have therefore focused on identifying the mechanisms of conditioning to decrypt, mimic, or amplify them. Volatile halogenated agents (VHAs) are the typical pharmacological application of the concept of conditioning in the operating room (Figure 1). Following several studies published in the late 80s, three independent teams demonstrated in 1997 the preconditioning effect of VHA (24–26). Comparing total intravenous anesthesia to VHA on a rabbit model, they reported a significant cardioprotective effect of VHA depending on both adenosine receptors and protein kinase C (24) and found that temporary administration of isoflurane reduced infarct size in dogs (25). The sulfonylurea glyburide abolished VHA-induced cardioprotection, highlighting the role of KATP channels in this mechanism (25). Since then, numerous studies have shown that VHA, administered before ischemia, reduced the size of myocardial infarction (27). This protective effect persists for several hours, a phenomenon called VHA-induced late preconditioning or second window of preconditioning (16). Meanwhile, Tanaka et al. (28) showed that animals anesthetized for 120 min with isoflurane had more than a 40% reduction in myocardial infarct size. This process results from the neosynthesis of proteins such as COX-2 (28) and endothelial NO synthases (eNOS) in response to VHA administration (29). In the late 1990s, several studies demonstrating the postconditioning effect of VHA were published (30–32). On isolated perfused hearts or in vivo models, halothane, enflurane, isoflurane, sevoflurane, and desflurane reduced myocardial injury when applied only during reperfusion. Sevoflurane-induced protection was observed at one minimal alveolar concentration (MAC), with no additional benefit at a higher dose (32). Even after cardioplegia, sevoflurane and desflurane provided additional protection when administered at the early phase of reperfusion (33). Intriguingly, 2 min of administration during reperfusion provided maximum protection, whereas less protective effects were observed with longer administration (34). The administration of one MAC isoflurane started 3 min before coronary reperfusion and maintained for only the first 2 min of reperfusion reduced myocardial infarct size by 50%, a phenomenon mediated by the activation of phosphatidylinositol-3-kinase (PI3K) signal transduction (35). VHA administration at this point could have a preferential impact at the mitochondrial level, resulting in a temporary inhibition of respiration, depolarization, and mitochondrial pH, subsequently slowing the opening of mPTP during the first time of reperfusion (36). Thus, VHAs are able to induce both valuable preconditioning and postconditioning (27, 37). Importantly, any postconditioning treatment must be applied at the initial phase of reperfusion before irreversible damage occurs. If the intervention is delayed for even 10 min, the protection is lost (38). In other words, VHA administration must be started a few minutes before reperfusion (before aortic unclamping) and continued immediately afterward in order to have a full protective effect.
3.2 Clinical studies of volatile halogenated agents in cardiac surgery
As early as 1999, Belhomme et al. (39) demonstrated that the administration of 5 min of isoflurane before aortic clamping during CABG surgery induced the activation of protein kinase C (a decisive step in the protective signaling pathway) as well as a decrease in postoperative troponin I. Subsequently, several studies by de Hert et al. (40–42) found marked cardioprotective effects of sevoflurane compared to propofol, responsible for the improvement in postoperative myocardial function, lower postoperative troponin I release, and shorter stay in the intensive care unit and hospital. Sevoflurane (43) and desflurane (44) significantly reduced postoperative troponin release in off-pump CABG surgery. However, those initial encouraging results have not been confirmed by other teams (45–48). More recently, the large MYRIAD trial (49), prospectively including over 5,000 patients in 36 centers, failed to demonstrate a benefit of VHA on 1-year mortality compared to intravenous anesthesia: 2.8% versus 3.0% (RR 0.94 [95% CI: 0.69–1.29], p = 0.71).
Several points need to be discussed. Anesthetic preconditioning seems to be more effective following repeated administration spaced by washout periods than continuous administration before ischemia. Two administrations of sevoflurane at one MAC spaced for at least 10 min significantly reduced serum troponin T release compared to a single continuous administration in patients undergoing CABG (50). Similarly, better cardioprotection following the administration of two sequences of one MAC sevoflurane for 5 min interspersed by a 5-min washout was found when compared with a single administration before CPB (51). The VHA administration protocol during surgery plays certainly a key role in the success of perioperative conditioning. Thus, the administration of VHA throughout surgery, including CPB, provided better protection than administration only before or after CPB (52). This specific efficiency of VHA, when administered during CPB, could be related to their immunomodulatory effects (53, 54). Finally, those cardioprotective effects of VHA must also be linked to their potential beneficial renal effects. It was reported in patients scheduled for CABG surgery that 10 min administration of two MAC sevoflurane during CPB and before aortic clamping significantly decreased postoperative release of brain natriuretic peptide and plasma cystatin C, biochemical markers of myocardial contractile, and renal dysfunction (55). The 1-year follow-up of this last cohort showed a reduced incidence of late cardiac events after surgery (56). Among the many meta-analyses published on the topic, Uhlig et al. (57) reviewed 45 cardiac surgery clinical studies involving 4,840 patients and found a reduction in overall mortality with VHA (OR 0.55; 95% CI: 0.35–0.85), as well as a reduction in perioperative complications. Similar results have been reported when VHAs were used throughout the surgical procedure (OR 0.66 [95% CI: 0.49–0.89]) (58). Those meta-analyses included small and sometimes single-center studies (59). International guidelines are in favor of the use of VHA during cardiac surgery (60, 61).
4 Remote ischemic preconditioning
The heart and other organs can be protected against I/R by applying brief periods of non-lethal I/R sequences to remote tissues, a phenomenon called RIPC (15). The simplest way to perform RIPC is to repeatedly inflate above systolic blood pressure, a blood pressure cuff placed at the root of the upper and/or lower limb (Figure 2). Numerous experimental studies demonstrated that the protective signal was transmitted to other organs, including the heart, via humoral and neuronal pathways. In a proof-to-concept study, Hausenloy et al. (62) demonstrated that a RIPC protocol consisting of three 5-min cycles of upper limb ischemia significantly reduced the postoperative troponin T release in patients undergoing CABG surgery. Other studies subsequently confirmed these initial encouraging results (63, 64). Meanwhile, Zarbock et al. (65) investigated the benefit of RIPC on renal protection during CPB and found that RIPC reduced acute kidney injury by 15% in high-risk patients. In a follow-up study of the same cohort, they showed persistent renal protection at 90 days with an 18% absolute risk reduction of acute kidney injury (66). However, those promising results have not been confirmed by others. A cycle of 3 × 5-min cuff inflations to 200 mmHg separated by 5-min periods of cuff deflation did not reduce troponin release or other organ protection during cardiac surgery (67). More recently, two multicenter, prospective, randomized trials involving a large number of patients were unable to demonstrate any benefit of RIPC. The RIPHeart study (68) found that a RIPC protocol did not modify the rate of a composite endpoint (postoperative myocardial infarction, stroke, renal failure, and death within 90 days) in 1,385 patients undergoing cardiac surgery. Similarly, the ERICCA trial (69) included 1,612 patients and, using a similar design and endpoint, found no benefit of RIPC. Moreover, a protocol combining RIPC and postconditioning (four cycles of 5-min ischemia/5-min reperfusion applied before and after CPB) did not improve the outcome in 1,280 patients scheduled for cardiac surgery (70). Finally, meta-analyses confirmed that RIPC could reduce postoperative troponin release without clinical benefit to overall outcomes (71, 72).
Several factors are expected to interfere with the clinical effectiveness of RIPC. The algorithm is probably of major importance in terms of the number of cycles, the duration of each ischemic sequence, and the RIPC application site (arm and/or thigh) (73, 74). In an ex vivo mice Langendorff model, Johnsen et al. (73) found that four, six, or eight cycles were effective, while two were not. Ischemic cycles lasting 2 min or 5 min reduced infarct size, but 10 min abolished cardioprotection. In clinical studies, the majority of medical staff use a 5-min ischemia protocol. There may be a dose dependence on the RIPC protocol, as previously suggested (74). Increasing the power of the stimulus could also be more effective, as demonstrated in patients undergoing cardiac surgery and having received a RIPC protocol by simultaneous inflation of a balloon on both the arm and the thigh (75). Drugs administered during cardiac surgery may also interfere with the effectiveness of a RIPC protocol. Thus, nitrates (despite their intrinsic cardioprotective properties) can inhibit the beneficial effect of RIPC (75, 76). Nitrates could inhibit RIPC-induced cardioprotection by NO inhibiting afferent nerve conduction in the limb. In the ERIC-GTN study (76), intravenous infusion of nitrates during surgery abrogated RIPC protection. Anesthetic agents could also affect the cardioprotective effectiveness of RIPC. Kottenberg et al. (77) reported that RIPC reduced the postoperative troponin release after CABG surgery in patients receiving isoflurane but not propofol. This latest study can be interpreted in two ways. Either the RIPC protocol was insufficiently powerful to protect the myocardium under clinical conditions and required synergistic protection by isoflurane or propofol by itself negated the protective effect of RIPC. Indeed, experimental studies have shown that propofol abolishes desflurane-induced preconditioning and RIPC (78, 79). In a recent meta-analysis focusing on the renal protective effects of RIPC, the authors emphasized that RIPC’s beneficial effects were mainly found during anesthesia with VHA (80).
5 Glycemic balance
Hyperglycemia is both a common phenomenon observed during cardiac surgery and a well-known independent risk factor of mortality (81, 82). In a large cohort including more than 8,000 patients undergoing cardiac surgery, Ascione et al. (83) found that 15% of them had blood sugar levels above 200 mg/dL, more than half of perioperative hyperglycemia occurring in non-diabetic patients. Hyperglycemia was associated with postoperative myocardial infarction (OR: 2.73 [95% CI, 1.74–4.26]). Among various processes, acute hyperglycemia increases ROS production, leading to endothelial dysfunction and worsening of myocardial I/R injury (84–86). Experimentally, myocardial infarct size was linearly related to blood glucose concentration (87). Several studies have also demonstrated that cardioprotective strategies such as VHA-induced preconditioning and postconditioning were abolished in hyperglycemic conditions (88–90). In addition, oral antidiabetic drugs such as glyburide could also inhibit preconditioning (25). Insulin is therefore a first-line therapy during cardiac surgery. In addition to lowering blood glucose levels, insulin has cardioprotective properties by activating the RISK pathway, especially when administered during reperfusion (91–93). It is important to emphasize the benefit of continuous infusion of insulin rather than boluses (94, 95). Beyond hyperglycemia, the variability of blood glucose concentration is harmful (96). If hyperglycemia must be undoubtedly treated (97), the current objective is to determine the optimal blood glucose threshold to be reached during cardiac surgery. Several studies reported an increased incidence of stroke (98) and delirium (99) during intraoperative tight glucose control. In a retrospective study of 4,000 patients treated at the Cleveland Clinic, Duncan et al. (100) reported that maintaining intraoperative blood glucose levels below 140 mg/dL increased morbidity and mortality. However, the same team conducted a prospective study in which a treated group received a fixed high-dose insulin and concomitant variable glucose infusion during cardiac surgery (101). In more than 1,400 randomized patients, they observed a 38% reduction of 30-day morbimortality in the treated group, demonstrating once again the intraoperative protective effect of insulin. The current consensus is, therefore, to treat with continuous insulin infusion when intraoperative blood glucose values are 180 mg/dL (10 mM) or higher; the target range is between 140 and 180 mg/dL (7.7–10 mM) (102–106). Finally, glycemic management appears to be an integral part of the cardioprotection strategy during cardiac surgery (Figure 4).
6 The challenge of aortic unclamping
6.1 Oxygenation control
Uncoupling of the mitochondrial respiratory chain during ischemia induces ROS overproduction in the event of excessive oxygenation at reperfusion (107). In vitro and in vivo experiments demonstrated that hyperoxic reperfusion increased inflammatory response and apoptosis and altered hemodynamic performances (108). Additionally, hyperoxia causes a significant reduction in coronary blood flow, which can further exacerbate reperfusion injury (109). Several clinical studies emphasized the potentially harmful effects of high-concentration oxygen therapy for the treatment of heart disorders in the medical setting (110, 111). The AVOID trial (112) showed, in non-hypoxemic patients suffering from ST-segment elevation myocardial infarction, that high-flow O2 therapy at reperfusion increased the peak of creatine kinase and the rate of recurrent myocardial infarction. Similarly, a recent meta-analysis (113) did not find evidence to support the use of oxygen therapy in normoxemic patients with acute myocardial infarction. Although debated, those questions arise in the context of cardiac surgery with CPB (114–116). In pediatric heart surgery, controlled reoxygenation during CPB decreased the markers of inflammation and organ damage (117). In patients scheduled for CABG surgery, hyperoxia (PaO2 = 400 mmHg) compared to normoxia (PaO2 = 140 mmHg) during CPB increased oxidative myocardial damage (118). Inoue et al. (119) explored the effects of reducing hyperoxia (PaO2 = 450–550 mmHg versus 200–250 mmHg) during reperfusion in cardiac surgery. They found that lowering the reperfused PaO2 after aortic unclamping significantly reduced oxidative damage and myocardial enzyme release. Conversely, McGuinness et al. (120) failed to demonstrate any difference in myocardial ischemia damage when comparing modest hyperoxia (178 mmHg) with normoxia. Interestingly, there was significant pre- and post-CPB hyperoxia (approximately 200 mmHg) in both groups, precisely during high-risk periods of hyperoxia-induced cellular damage. It is therefore quite likely that hyperoxia at the time of aortic unclamping may worsen myocardial lesions. Moreover, intraoperative oxidative damage is associated with postoperative delirium and neuronal injury (121). To sum up, it seems reasonable to advise that excessive use of supplemental oxygen in normoxic patients, particularly at the time of aortic unclamping, could potentially lead to exacerbated myocardial injury (Figure 4).
6.2 Impact of acid–base balance
Using a pH electrode on a rabbit heart model, Cobbe et al. (122) demonstrated that tissue pH fell continuously during ischemia because of progressive H+ ion accumulation, with a rapid recovery of pH during the first minutes of reperfusion. However, it has been shown that part of reperfusion injury comes from the rapidity of the pH rise. In rat livers, a slow increase in pH over 15 min after reperfusion dramatically reduced LDH release, a phenomenon known as pH paradox (123). Some years later, this result was confirmed on a cardiomyocyte model (124). It is well established that sudden reperfusion generates ROS production and induces calcium overload (125). Rapid recovery of cellular acidosis during the first moment of reperfusion ultimately leads to hypercontraction of the myocardium. At this point, ROS production at the mitochondrial level induces mPTP opening and subsequent myocyte death, a phenomenon that can be prevented by initial acidotic reperfusion (126). Several techniques, such as hypercapnic acidosis and Na+/H+ exchange inhibition, used during reperfusion attenuate lethal damage, whereas an alkaline solution (pH = 7.6) aggravated it (127). How long this temporary acidosis should be maintained after reperfusion is probably a pivotal question. Using a Langendorff-isolated rat heart model, Ohashi et al. (128) found that reperfusion for less than 3 min with an acid solution provided better recovery. Experimentally, acid reperfusion for 3 min delays the normalization of myocardial tissue pH and enhances myocardial salvage (129). Several studies suggest that the protective effect of postconditioning could be mediated by prolonged transient acidosis (130–133). Experimentally, infusion of NaHCO3 during a postconditioning protocol abolished cardioprotection and blunted the activation of RISK pathways (130). It is postulated that postconditioning prevents mPTP opening by maintaining temporary acidosis during the first minutes of reperfusion (131). VHA could also induce postconditioning through inhibition of respiration, depolarization, and ultimately mitochondrial acidification upon reperfusion (36). A complementary approach to maintaining a temporary acidosis during reperfusion is to inhibit Na+/H+ exchange. Administration of cariporide reduced Na+ overload and contributed to H+ extrusion during reperfusion (134, 135). Interestingly, this process also leads to a drop in Ca++ level during reperfusion (136). This proof of concept was demonstrated in the prospective, multicenter EXPEDITION study (137), which included 5,761 patients undergoing high-risk CABG surgery. The administration of cariporide before, during, and after surgery significantly reduced the incidence of myocardial infarction from 18.9% in the placebo group to 14.4% in the treatment group. However, because of an increase in cerebrovascular events in the treated group, the clinical use of cariporide was halted. Surprisingly, the EXPEDITION study design required cariporide administration to be continued for 48 h after surgery, i.e., a potentially too long way from the time of myocardial reperfusion. To summarize, the myocardial tissue is subject to significant pH variations at the time of aortic unclamping. At the very least, the clinician should avoid any alkalosis just before and just after myocardial reperfusion, which is very likely to be deleterious at this stage (Figure 4).
6.3 Controlling reperfusion pressure
Reperfusion injury may also be the result of excess pressure in the first moments following aortic unclamping. Okamoto et al. (138) demonstrated that early gentle reperfusion (50 mmHg versus 80 mmHg during the first 20 min of reperfusion) limited the post-ischemic damage in animals subjected to 4 h of ischemia. Similarly, a staged reperfusion protocol in which the coronary perfusion pressure was maintained at 40% of control for 0–3 min after the onset of reperfusion, 60% of control for 4–6 min, and 80% of control for 7–10 min has been suggested (139). This graduated reperfusion could mitigate myocardial stunning via transient acidosis during early reperfusion. Controlled reperfusion decreases calcium deposition and increases both mitochondrial oxidative phosphorylation rate and myocardial ATP content (140). Furthermore, low-pressure reperfusion limited myocardial necrosis by inhibiting mPTP opening on an isolated Langendorff heart model (141). Low-pressure reperfusion appears to offer similar protection to that provided by postconditioning, both techniques involving the activation of the PI3K-mPTP pathway (142). Testing this concept of gradual reperfusion on a population of patients undergoing CABG surgery, other authors (143) found a significant regression of interstitial edema at 60 min reperfusion. Although it is well established that excessive pressure worsens myocardial damage during reperfusion, there is currently no precise scheme for gentle reperfusion that can be clinically applied. Consequently, the practical implementation of this concept varies widely from one center to another (144).
7 The postoperative period
During the postoperative period, several factors will also influence myocardial tolerance to cardiac surgery with CPB. Maintaining the right balance between myocardial oxygen supply and demand remains a key issue. A transfusion threshold adapted to the needs of the myocardium and resumption of treatments such as beta-blockers at an early stage is traditionally part of good practices. In addition, two other specific points are worth mentioning here. During cardiac surgery, catecholamines are widely used to prevent or treat low-cardiac output syndrome, depending on preoperative patients’ status, the complexity of the surgical procedure, and, above all, the physician’s decision (145, 146). However, those drugs should be used only as needed to maintain adequate organ perfusion (147). Because of their positive inotropic and/or chronotropic effects, the overuse of catecholamines can lead to cardiac arrhythmias and myocyte death. Studying a large cohort of patients undergoing conventional cardiac surgery, we found (148) that perioperative use of dobutamine, simply based on the clinical judgment of the anesthesiologist, increased postoperative major cardiac morbidity. Exploring the data of a national cohort of 6,005 consecutive cardiac surgery patients, Nielsen et al. (149) demonstrated that inotropic therapy was independently associated with short- and long-term postoperative myocardial infarction and death. It seems essential to carefully monitor the patients’ macrohemodynamic parameters to correctly assess volemia and cardiovascular function so that catecholamines can be judiciously used and discontinued as soon as possible. In addition, the pleiotropic beneficial effects of statins have been widely described, hence their widespread use in cardiac surgery patients (150, 151). Although the administration of statins in the preoperative phase of cardiac surgery has recently been shown to be ineffective, physicians must be aware that these drugs can cause a rebound effect (152–154). Postoperative statin withdrawal was an independent predictor of postoperative myocardial infarction after major vascular surgery (155). Initiation of statin treatment results in endothelial eNOS upregulation due to the inactivation of a Rho protein, which usually inhibits eNOS. In the case of statin chronic therapy discontinuation, there is an overshoot translocation and activation of Rho, causing downregulation of eNOS production below baseline levels (156).
8 Modulating factors
During the intraoperative period, due to the multiplicity of patient-related factors (comorbidities and treatments) as well as operative techniques (anesthetic agents, CPB, and cardioplegia), the efficacy of cardioprotective techniques is questionable. First, the phenomenon of cardiac conditioning is influenced by age, with drop-in effectiveness in elderly patients (157). Senescent myocardium is particularly sensitive to ischemia, probably due to metabolic degradation and impaired mitochondrial function (158, 159). A progressive loss of response to preconditioning was demonstrated by comparing three cohorts of 3-, 12-, and 20-month-old rats subjected to 1 or 3 cycles of ischemic preconditioning (160). It has also been shown that the protective effect of sevoflurane gradually disappeared with age (161). Second, the cardioprotective effects of estrogens and their possible interference with preconditioning have been regularly reported. Estrogens are thought to be protective via several mechanisms: activation of KATP channels, reduced leukocyte adhesion, ROS, and NO production, and reduced calcium influx during ischemia (162–166). In an experimental study, the injection of 17b-estradiol was shown to induce cardioprotection mediated by mitochondrial KATP channels, identical to that of ischemic preconditioning (162). It was also found under similar conditions that female mice were already protected and that ischemic preconditioning did not provide any additional protection (167). Myocardial infarct size was significantly smaller in female rabbits compared to male rabbits, and isoflurane did not provide any additional benefit (166). Third, many pharmacological agents used during surgery could interfere with perioperative cardioprotection (Table 1). Several reviews have focused on the cardioprotective effects of opioids (168–170). These agents act via activation of κ-, δ-, and/or even μ-opioid receptors, leading to protein kinase C activation and potentiation of KATP channels opening (171, 172). Propofol is known to abolish myocardial conditioning, possibly due to its ROS scavenger effects (79, 173). Ketamine inhibits ischemic preconditioning through its action on KATP channels (174). This pharmacological effect appears to be linked to the stereoselectivity of ketamine since the S(+)-form is neutral on both early and late preconditioning (175, 176). Lidocaine could also interfere with either anti-apoptotic cardioprotective or antagonist effects (177–179). A large prospective, comparative, randomized, multicenter study demonstrated that xenon, known to induce preconditioning and postconditioning (180, 181), significantly reduced postoperative troponin release in patients undergoing CABG surgery when compared to total intravenous anesthesia (182). Cyclosporine, in addition to its immunosuppressive properties, is a potent inhibitor of mPTP opening by preventing the calcium-induced interaction of cyclophilin D with a pore component (183). A single intravenous bolus of cyclosporine administered before CPB—as preconditioning (184)—or 10 min before aortic cross-unclamping—as postconditioning (185)—reduced the extent of myocardial injury in patients undergoing CABG or aortic valve surgery. However, clinical trials about the use of cyclosporine in cardiac surgery were interrupted following the publication of the large-scale multicenter CIRCUS study (186), which failed to demonstrate any improvement in patients with ST-segment elevation myocardial infarction.
9 Synergistic approaches
Experimental studies have suggested that a bundle of care could reinforce myocardial conditioning (187, 188). The interaction between several interventions to mitigate cardiac reperfusion injury has been shown between VHA and a postconditioning intervention (35). These results further suggest that the administration of 0.5 MAC isoflurane at reperfusion, a concentration that does not provide cardioprotection alone, reduces the threshold of ischemic postconditioning. Huhn et al. (89) showed that hyperglycemia abolished sevoflurane-induced postconditioning and that cyclosporine A reversed this loss of protection. The combination of RIPC with local ischemic postconditioning has previously been tested in ST-elevation myocardial infarction (189, 190). However, the efficacy of those multimodal interventions on surrogate markers of reperfusion injury, such as serum creatine kinase-MB isoenzyme for the RIPOST-MI study (189) or the salvage index by cardiac magnetic resonance imaging for the LIPSIA CONDITIONING study (190), is controversial. The ProCCard study (191) evaluated the relevance of a cardioprotective bundle of care during cardiac surgery with CPB. A total of 210 patients were randomized into a standard-of-care group and a treatment group simultaneously combining five modes of cardioprotection: sevoflurane administration, RIPC, tight intraoperative blood glucose control, induction of a moderate respiratory acidosis to prevent the pH paradox phenomenon, and gentle reperfusion to limit myocardial reperfusion injury (Figure 4). Unfortunately, the primary endpoint (the postoperative 72-h area under the curve of high-sensitivity cardiac troponin I) was not significantly modified: the mean ratio between control and treatment groups was 0.92; 95%CI: 0.71–1.21; p = 0.55. However, VHA administration modalities during CPB could be a crucial point in that study (192). These various factors indicate that the notion of synergy in cardioprotection remains a point that needs to be further explored.
10 Conclusion
After many years of translational research in the field of perioperative cardioprotection with inconsistent results, many questions remain. The specificity of the pathophysiology of I/R during cardiac surgery with CPB makes the equation complex. Notably, the myocardium is subjected to global ischemia on a non-beating heart, to which are added the effects of cardioplegia as well as those of CPB. This review highlighted different perioperative strategies to limit perioperative myocardial injury in patients undergoing cardiac surgery with CPB. Myocardial preconditioning and postconditioning, despite their limited clinical applications, have highlighted the understanding of the underlying mechanisms of intraoperative I/R myocardial injury. It is now up to the healthcare providers to integrate these different elements and maintain a comprehensive approach pre-, intra-, and post-CPB to limit intraoperative myocardial injury as effectively as possible during cardiac surgery.
Author contributions
PC: Conceptualization, Validation, Writing – original draft. J-LF: Conceptualization, Supervision, Validation, Visualization, Writing – review & editing.
Funding
The author(s) declare that no financial support was received for the research, authorship, and/or publication of this article.
Conflict of interest
The authors declare that the research was conducted in the absence of any commercial or financial relationships that could be construed as a potential conflict of interest.
The handling editor EB declared a past co-authorship with one of the author J-LF.
Publisher’s note
All claims expressed in this article are solely those of the authors and do not necessarily represent those of their affiliated organizations, or those of the publisher, the editors and the reviewers. Any product that may be evaluated in this article, or claim that may be made by its manufacturer, is not guaranteed or endorsed by the publisher.
Abbreviations
CPB, Cardiopulmonary bypass; I/R, Ischemia/reperfusion; CABG, Coronary artery bypass graft; ROS, Radical oxygen species; RIPC, Remote ischemic preconditioning; NO, Nitric oxide; COX-2, Cyclooxygenase-2; KATP, ATP-gated potassium; RISK, Reperfusion injury salvage kinases; SAFE, Survivor activating factor enhancement; mPTP, Mitochondrial permeability transition pore; VHA, Volatile halogenated agents; eNOS, Endothelial NO synthase; MAC, Minimal alveolar concentration; PI3K, Phosphatidylinositol-3-kinase
References
1. Fellahi, JL, Gue, X, Richomme, X, Monier, E, Guillou, L, and Riou, B. Short- and long-term prognostic value of postoperative cardiac troponin I concentration in patients undergoing coronary artery bypass grafting. Anesthesiology. (2003) 99:270–4. doi: 10.1097/00000542-200308000-00007
2. Croal, BL, Hillis, GS, Gibson, PH, Fazal, MT, El-Shafei, H, Gibson, G, et al. Relationship between postoperative cardiac troponin I levels and outcome of cardiac surgery. Circulation. (2006) 114:1468–75. doi: 10.1161/CIRCULATIONAHA.105.602370
3. Lurati Buse, GA, Koller, MT, Grapow, M, Bolliger, D, Seeberger, M, and Filipovic, M. The prognostic value of troponin release after adult cardiac surgery - a meta-analysis. Eur J Cardiothorac Surg. (2010) 37:399–406. doi: 10.1016/j.ejcts.2009.05.054
4. Carrier, M, Pellerin, M, Perrault, LP, Solymoss, BC, and Pelletier, LC. Troponin levels in patients with myocardial infarction after coronary artery bypass grafting. Ann Thorac Surg. (2000) 69:435–40. doi: 10.1016/s0003-4975(99)01294-1
5. Fellahi, J-L, Hanouz, J-L, Gué, X, Monier, E, Guillou, L, and Riou, B. Kinetic analysis of cardiac troponin I release is no more accurate than a single 24-h measurement in predicting in-hospital outcome after cardiac surgery. Eur J Anaesthesiol. (2008) 25:490–7. doi: 10.1017/S0265021508003827
6. Devereaux, PJ, Lamy, A, Chan, MTV, Allard, RV, Lomivorotov, VV, Landoni, G, et al. VISION cardiac surgery Investigators. High-sensitivity troponin I after cardiac surgery and 30-day mortality. N Engl J Med. (2022) 386:827–36. doi: 10.1056/NEJMoa2000803
7. Chambers, DJ, and Fallouh, HB. Cardioplegia and cardiac surgery: pharmacological arrest and cardioprotection during global ischemia and reperfusion. Pharmacol Ther. (2010) 127:41–52. doi: 10.1016/j.pharmthera.2010.04.001
8. Murry, CE, Jennings, RB, and Reimer, KA. Preconditioning with ischemia: a delay of lethal cell injury in ischemic myocardium. Circulation. (1986) 74:1124–36. doi: 10.1161/01.cir.74.5.1124
9. Ottani, F, Galvani, M, Ferrini, D, Sorbello, F, Limonetti, P, Pantoli, D, et al. Prodromal angina limits infarct size. A role for ischemic preconditioning. Circulation. (1995) 91:291–7. doi: 10.1161/01.cir.91.2.291
10. Ishihara, M, Sato, H, Tateishi, H, Kawagoe, T, Shimatani, Y, Kurisu, S, et al. Implications of prodromal angina pectoris in anterior wall acute myocardial infarction: acute angiographic findings and long-term prognosis. J Am Coll Cardiol. (1997) 30:970–5. doi: 10.1016/s0735-1097(97)00238-6
11. Yellon, DM, and Hausenloy, DJ. Myocardial reperfusion injury. N Engl J Med. (2007) 357:1121–35. doi: 10.1056/NEJMra071667
12. Zhao, ZQ, Corvera, JS, Halkos, ME, Kerendi, F, Wang, NP, Guyton, RA, et al. Inhibition of myocardial injury by ischemic postconditioning during reperfusion: comparison with ischemic preconditioning. Am J Physiol Heart Circ Physiol. (2003) 285:H579–88. doi: 10.1152/ajpheart.01064.2002
13. Staat, P, Rioufol, G, Piot, C, Cottin, Y, Cung, TT, L’Huillier, I, et al. Postconditioning the human heart. Circulation. (2005) 112:2143–8. doi: 10.1161/CIRCULATIONAHA.105.558122
14. Thibault, H, Piot, C, Staat, P, Bontemps, L, Sportouch, C, Rioufol, G, et al. Long-term benefit of postconditioning. Circulation. (2008) 117:1037–44. doi: 10.1161/CIRCULATIONAHA.107.729780
15. Heusch, G, Botker, HE, Przyklenk, K, Redington, A, and Yellon, D. Remote ischemic conditioning. J Am Coll Cardiol. (2015) 65:177–95. doi: 10.1016/j.jacc.2014.10.031
16. Bolli, R, Li, QH, Tang, XL, Guo, Y, Xuan, YT, Rokosh, G, et al. The late phase of preconditioning and its natural clinical application--gene therapy. Heart Fail Rev. (2007) 12:189–99. doi: 10.1007/s10741-007-9031-4
17. Cohen, MV, and Downey, JM. Signalling pathways and mechanisms of protection in pre- and postconditioning: historical perspective and lessons for the future. Br J Pharmacol. (2015) 172:1913–32. doi: 10.1111/bph.12903
18. Torregroza, C, Raupach, A, Feige, K, Weber, NC, Hollmann, MW, and Huhn, R. Perioperative Cardioprotection: general mechanisms and pharmacological approaches. Anesth Analg. (2020) 131:1765–80. doi: 10.1213/ANE.0000000000005243
19. Ong, SB, Dongworth, RK, Cabrera-Fuentes, HA, and Hausenloy, DJ. Role of the MPTP in conditioning the heart - translatability and mechanism. Br J Pharmacol. (2015) 172:2074–84. doi: 10.1111/bph.13013
20. Li, B, Chauvin, C, De Paulis, D, De Oliveira, F, Gharib, A, Vial, G, et al. Inhibition of complex I regulates the mitochondrial permeability transition through a phosphate-sensitive inhibitory site masked by cyclophilin D. Biochim Biophys Acta. (2012) 1817:1628–34. doi: 10.1016/j.bbabio.2012.05.011
21. Teixeira, G, Abrial, M, Portier, K, Chiari, P, Couture-Lepetit, E, Tourneur, Y, et al. Synergistic protective effect of cyclosporin a and rotenone against hypoxia-reoxygenation in cardiomyocytes. J Mol Cell Cardiol. (2013) 56:55–62. doi: 10.1016/j.yjmcc.2012.11.023
22. Giorgi, C, De Stefani, D, Bononi, A, Rizzuto, R, and Pinton, P. Structural and functional link between the mitochondrial network and the endoplasmic reticulum. Int J Biochem Cell Biol. (2009) 41:1817–27. doi: 10.1016/j.biocel.2009.04.010
23. Paillard, M, Tubbs, E, Thiebaut, PA, Gomez, L, Fauconnier, J, Da Silva, CC, et al. Depressing mitochondria-reticulum interactions protects cardiomyocytes from lethal hypoxia-reoxygenation injury. Circulation. (2013) 128:1555–65. doi: 10.1161/CIRCULATIONAHA.113.001225
24. Cope, DK, Impastato, WK, Cohen, MV, and Downey, JM. Volatile anesthetics protect the ischemic rabbit myocardium from infarction. Anesthesiology. (1997) 86:699–709. doi: 10.1097/00000542-199703000-00023
25. Kersten, JR, Schmeling, TJ, Pagel, PS, Gross, GJ, and Warltier, DC. Isoflurane mimics ischemic preconditioning via activation of K(ATP) channels: reduction of myocardial infarct size with an acute memory phase. Anesthesiology. (1997) 87:361–70. doi: 10.1097/00000542-199708000-00024
26. Cason, BA, Gamperl, AK, Slocum, RE, and Hickey, RF. Anesthetic-induced preconditioning: previous administration of isoflurane decreases myocardial infarct size in rabbits. Anesthesiology. (1997) 87:1182–90. doi: 10.1097/00000542-199711000-00023
27. Pagel, PS, and Crystal, GJ. The discovery of myocardial preconditioning using volatile anesthetics: a history and contemporary clinical perspective. J Cardiothorac Vasc Anesth. (2018) 32:1112–34. doi: 10.1053/j.jvca.2017.12.029
28. Tanaka, K, Ludwig, LM, Krolikowski, JG, Alcindor, D, Pratt, PF, Kersten, JR, et al. Isoflurane produces delayed preconditioning against myocardial ischemia and reperfusion injury: role of cyclooxygenase-2. Anesthesiology. (2004) 100:525–31. doi: 10.1097/00000542-200403000-00010
29. Chiari, PC, Bienengraeber, MW, Weihrauch, D, Krolikowski, JG, Kersten, JR, Warltier, DC, et al. Role of endothelial nitric oxide synthase as a trigger and mediator of isoflurane-induced delayed preconditioning in rabbit myocardium. Anesthesiology. (2005) 103:74–83. doi: 10.1097/00000542-200507000-00014
30. Schlack, W, Preckel, B, Stunneck, D, and Thämer, V. Effects of halothane, enflurane, isoflurane, sevoflurane and desflurane on myocardial reperfusion injury in the isolated rat heart. Br J Anaesth. (1998) 81:913–9. doi: 10.1093/bja/81.6.913
31. Preckel, B, Schlack, W, Comfère, T, Obal, D, Barthel, H, and Thämer, V. Effects of enflurane, isoflurane, sevoflurane and desflurane on reperfusion injury after regional myocardial ischaemia in the rabbit heart in vivo. Br J Anaesth. (1998) 81:905–12. doi: 10.1093/bja/81.6.905
32. Obal, D, Preckel, B, Scharbatke, H, Müllenheim, J, Höterkes, F, Thämer, V, et al. One MAC of sevoflurane provides protection against reperfusion injury in the rat heart in vivo. Br J Anaesth. (2001) 87:905–11. doi: 10.1093/bja/87.6.905
33. Preckel, B, Thämer, V, and Schlack, W. Beneficial effects of sevoflurane and desflurane against myocardial reperfusion injury after cardioplegic arrest. Can J Anaesth. (1999) 46:1076–81. doi: 10.1007/BF03013206
34. Obal, D, Scharbatke, H, Barthel, H, Preckel, B, Müllenheim, J, and Schlack, W. Cardioprotection against reperfusion injury is maximal with only two minutes of sevoflurane administration in rats. Can J Anaesth. (2003) 50:940–5. doi: 10.1007/BF03018744
35. Chiari, PC, Bienengraeber, MW, Pagel, PS, Krolikowski, JG, Kersten, JR, and Warltier, DC. Isoflurane protects against myocardial infarction during early reperfusion by activation of phosphatidylinositol-3-kinase signal transduction: evidence for anesthetic-induced postconditioning in rabbits. Anesthesiology. (2005) 102:102–9. doi: 10.1097/00000542-200501000-00018
36. Pravdic, D, Mio, Y, Sedlic, F, Pratt, PF, Warltier, DC, Bosnjak, ZJ, et al. Isoflurane protects cardiomyocytes and mitochondria by immediate and cytosol-independent action at reperfusion. Br J Pharmacol. (2010) 160:220–32. doi: 10.1111/j.1476-5381.2010.00698.x
37. Lemoine, S, Tritapepe, L, Hanouz, JL, and Puddu, PE. The mechanisms of cardio-protective effects of desflurane and sevoflurane at the time of reperfusion: anaesthetic post-conditioning potentially translatable to humans? Br J Anaesth. (2016) 116:456–75. doi: 10.1093/bja/aev451
38. Yang, XM, Proctor, JB, Cui, L, Krieg, T, Downey, JM, and Cohen, MV. Multiple, brief coronary occlusions during early reperfusion protect rabbit hearts by targeting cell signaling pathways. J Am Coll Cardiol. (2004) 44:1103–10. doi: 10.1016/j.jacc.2004.05.060
39. Belhomme, D, Peynet, J, Louzy, M, Launay, JM, Kitakaze, M, and Menasché, P. Evidence for preconditioning by isoflurane in coronary artery bypass graft surgery. Circulation. (1999) 100:II340–4. doi: 10.1161/circ.100.suppl_2.Ii-340
40. De Hert, SG, ten Broecke, PW, Mertens, E, Van Sommeren, EW, De Blier, IG, Stockman, BA, et al. Sevoflurane but not propofol preserves myocardial function in coronary surgery patients. Anesthesiology. (2002) 97:42–9. doi: 10.1097/00000542-200207000-00007
41. De Hert, SG, Cromheecke, S, ten Broecke, PW, Mertens, E, De Blier, IG, Stockman, BA, et al. Effects of propofol, desflurane, and sevoflurane on recovery of myocardial function after coronary surgery in elderly high-risk patients. Anesthesiology. (2003) 99:314–23. doi: 10.1097/00000542-200308000-00013
42. De Hert, SG, Van der Linden, PJ, Cromheecke, S, Meeus, R, ten Broecke, PW, De Blier, IG, et al. Choice of primary anesthetic regimen can influence intensive care unit length of stay after coronary surgery with cardiopulmonary bypass. Anesthesiology. (2004) 101:9–20. doi: 10.1097/00000542-200407000-00005
43. Conzen, PF, Fischer, S, Detter, C, and Peter, K. Sevoflurane provides greater protection of the myocardium than propofol in patients undergoing off-pump coronary artery bypass surgery. Anesthesiology. (2003) 99:826–33. doi: 10.1097/00000542-200310000-00013
44. Guarracino, F, Landoni, G, Tritapepe, L, Pompei, F, Leoni, A, Aletti, G, et al. Myocardial damage prevented by volatile anesthetics: a multicenter randomized controlled study. J Cardiothorac Vasc Anesth. (2006) 20:477–83. doi: 10.1053/j.jvca.2006.05.012
45. Fellahi, JL, Gue, X, Philippe, E, Riou, B, and Gerard, JL. Isoflurane may not influence postoperative cardiac troponin I release and clinical outcome in adult cardiac surgery. Eur J Anaesthesiol. (2004) 21:688–93. doi: 10.1017/s0265021504009044
46. Piriou, V, Mantz, J, Goldfarb, G, Kitakaze, M, Chiari, P, Paquin, S, et al. Sevoflurane preconditioning at 1 MAC only provides limited protection in patients undergoing coronary artery bypass surgery: a randomized bi-Centre trial. Br J Anaesth. (2007) 99:624–31. doi: 10.1093/bja/aem264
47. De Hert, SG, Vlasselaers, D, Barbe, R, Ory, JP, Dekegel, D, Donnadonni, R, et al. A comparison of volatile and non volatile agents for cardioprotection during on-pump coronary surgery. Anaesthesia. (2009) 64:953–60. doi: 10.1111/j.1365-2044.2009.06008.x
48. Flier, S, Post, J, Concepcion, AN, Kappen, TH, Kalkman, CJ, and Buhre, WF. Influence of propofol-opioid vs isoflurane-opioid anaesthesia on postoperative troponin release in patients undergoing coronary artery bypass grafting. Br J Anaesth. (2010) 105:122–30. doi: 10.1093/bja/aeq111
49. Landoni, G, Lomivorotov, VV, Nigro Neto, C, Monaco, F, Pasyuga, VV, Bradic, N, et al. Volatile anesthetics versus Total intravenous anesthesia for cardiac surgery. N Engl J Med. (2019) 380:1214–25. doi: 10.1056/NEJMoa1816476
50. Bein, B, Renner, J, Caliebe, D, Hanss, R, Bauer, M, Fraund, S, et al. The effects of interrupted or continuous administration of sevoflurane on preconditioning before cardio-pulmonary bypass in coronary artery surgery: comparison with continuous propofol. Anaesthesia. (2008) 63:1046–55. doi: 10.1111/j.1365-2044.2008.05563.x
51. Frässdorf, J, Borowski, A, Ebel, D, Feindt, P, Hermes, M, Meemann, T, et al. Impact of preconditioning protocol on anesthetic-induced cardioprotection in patients having coronary artery bypass surgery. J Thorac Cardiovasc Surg. (2009) 137:1436–1442.e2. doi: 10.1016/j.jtcvs.2008.04.034
52. De Hert, SG, Van der Linden, PJ, Cromheecke, S, Meeus, R, Nelis, A, Van Reeth, V, et al. Cardioprotective properties of sevoflurane in patients undergoing coronary surgery with cardiopulmonary bypass are related to the modalities of its administration. Anesthesiology. (2004) 101:299–310. doi: 10.1097/00000542-200408000-00009
53. Stollings, LM, Jia, LJ, Tang, P, Dou, H, Lu, B, and Xu, Y. Immune modulation by volatile anesthetics. Anesthesiology. (2016) 125:399–411. doi: 10.1097/ALN.0000000000001195
54. Yuki, K, and Eckenhoff, RG. Mechanisms of the immunological effects of volatile anesthetics: a review. Anesth Analg. (2016) 123:326–35. doi: 10.1213/ANE.0000000000001403
55. Julier, K, da Silva, R, Garcia, C, Bestmann, L, Frascarolo, P, Zollinger, A, et al. Preconditioning by sevoflurane decreases biochemical markers for myocardial and renal dysfunction in coronary artery bypass graft surgery: a double-blinded, placebo-controlled, multicenter study. Anesthesiology. (2003) 98:1315–27. doi: 10.1097/00000542-200306000-00004
56. Garcia, C, Julier, K, Bestmann, L, Zollinger, A, von Segesser, LK, Pasch, T, et al. Preconditioning with sevoflurane decreases PECAM-1 expression and improves one-year cardiovascular outcome in coronary artery bypass graft surgery. Br J Anaesth. (2005) 94:159–65. doi: 10.1093/bja/aei026
57. Uhlig, C, Bluth, T, Schwarz, K, Deckert, S, Heinrich, L, De Hert, S, et al. Effects of volatile anesthetics on mortality and postoperative pulmonary and other complications in patients undergoing surgery: a systematic review and Meta-analysis. Anesthesiology. (2016) 124:1230–45. doi: 10.1097/ALN.0000000000001120
58. Bonanni, A, Signori, A, Alicino, C, Mannucci, I, Grasso, MA, Martinelli, L, et al. Volatile anesthetics versus Propofol for cardiac surgery with cardiopulmonary bypass: Meta-analysis of randomized trials. Anesthesiology. (2020) 132:1429–46. doi: 10.1097/ALN.0000000000003236
59. Ștefan, M, Predoi, C, Goicea, R, and Filipescu, D. Volatile Anaesthesia versus Total intravenous Anaesthesia for cardiac surgery-a narrative review. J Clin Med. (2022) 11:6031. doi: 10.3390/jcm11206031
60. Hillis, LD, Smith, PK, Anderson, JL, Bittl, JA, Bridges, CR, Byrne, JG, et al. 2011 ACCF/AHA guideline for coronary artery bypass graft surgery: a report of the American College of Cardiology Foundation/American Heart Association task force on practice guidelines. Circulation. (2011) 124:e652–735. doi: 10.1161/CIR.0b013e31823c074e
61. Wahba, A, Milojevic, M, Boer, C, De Somer, F, Gudbjartsson, T, van den Goor, J, et al. 2019 EACTS/EACTA/EBCP guidelines on cardiopulmonary bypass in adult cardiac surgery. Eur J Cardiothorac Surg. (2020) 57:210–51. doi: 10.1093/ejcts/ezz267
62. Hausenloy, DJ, Mwamure, PK, Venugopal, V, Harris, J, Barnard, M, Grundy, E, et al. Effect of remote ischaemic preconditioning on myocardial injury in patients undergoing coronary artery bypass graft surgery: a randomised controlled trial. Lancet. (2007) 370:575–9. doi: 10.1016/S0140-6736(07)61296-3
63. Venugopal, V, Hausenloy, DJ, Ludman, A, Di Salvo, C, Kolvekar, S, Yap, J, et al. Remote ischaemic preconditioning reduces myocardial injury in patients undergoing cardiac surgery with cold-blood cardioplegia: a randomised controlled trial. Heart. (2009) 95:1567–71. doi: 10.1136/hrt.2008.155770
64. Thielmann, M, Kottenberg, E, Boengler, K, Raffelsieper, C, Neuhaeuser, M, Peters, J, et al. Remote ischemic preconditioning reduces myocardial injury after coronary artery bypass surgery with crystalloid cardioplegic arrest. Basic Res Cardiol. (2010) 105:657–64. doi: 10.1007/s00395-010-0104-5
65. Zarbock, A, Schmidt, C, Van Aken, H, Wempe, C, Martens, S, Zahn, PK, et al. For the RenalRIPC Investigators. Effect of remote ischemic preconditioning on kidney injury among high-risk patients undergoing cardiac surgery. A randomized clinical trial. JAMA. (2015) 313:2133–41. doi: 10.1001/jama.2015.4189
66. Zarbock, A, Kellum, JA, Van Aken, H, Schmidt, C, Küllmar, M, Rosenberger, P, et al. Long-term effects of remote ischemic preconditioning on kidney function in high-risk cardiac surgery patients: follow-up results from the RenalRIP trial. Anesthesiology. (2017) 126:787–98. doi: 10.1097/ALN.0000000000001598
67. Rahman, IA, Mascaro, JG, Steeds, RP, Frenneaux, MP, Nightingale, P, Gosling, P, et al. Remote ischemic preconditioning in human coronary artery bypass surgery: from promise to disappointment? Circulation. (2010) 122:S53–9. doi: 10.1161/CIRCULATIONAHA.109.926667
68. Meybohm, P, Bein, B, Brosteanu, O, Cremer, J, Gruenewald, M, Stoppe, C, et al. For the RIPHeart study collaborators. A multicenter trial of remote ischemic preconditioning for heart surgery. N Engl J Med. (2015) 373:1397–407. doi: 10.1056/NEJMoa1413579
69. Hausenloy, DJ, Candilio, L, Evans, R, Ariti, C, Jenkins, DP, Kolvekar, S, et al. For the ERICCA trial Investigators. Remote ischemic preconditioning and outcomes of cardiac surgery. N Engl J Med. (2015) 373:1408–17. doi: 10.1056/NEJMoa1413534
70. Hong, DM, Lee, EH, Kim, HJ, Min, JJ, Chin, JH, Choi, DK, et al. Does remote ischaemic preconditioning with postconditioning improve clinical outcomes of patients undergoing cardiac surgery? Remote Ischaemic preconditioning with Postconditioning outcome trial. Eur Heart J. (2014) 35:176–83. doi: 10.1093/eurheartj/eht346
71. Benstoem, C, Stoppe, C, Liakopoulos, OJ, Ney, J, Hasenclever, D, Meybohm, P, et al. Remote ischaemic preconditioning for coronary artery bypass grafting (with or without valve surgery). Cochrane Database Syst Rev. (2017) 2017:CD011719. doi: 10.1002/14651858.CD011719.pub3
72. Pierce, B, Bole, I, Patel, V, and Brown, DL. Clinical outcomes of remote ischemic preconditioning prior to cardiac surgery: a Meta-analysis of randomized controlled trials. J Am Heart Assoc. (2017) 6:e004666. doi: 10.1161/JAHA.116.004666
73. Johnsen, J, Pryds, K, Salman, R, Løfgren, B, Kristiansen, SB, and Bøtker, HE. The remote ischemic preconditioning algorithm: effect of number of cycles, cycle duration and effector organ mass on efficacy of protection. Basic Res Cardiol. (2016) 111:10. doi: 10.1007/s00395-016-0529-6
74. Meersch, M, Küllmar, M, Pavenstädt, H, Rossaint, J, Kellum, JA, Martens, S, et al. Effects of different doses of remote ischemic preconditioning on kidney damage among patients undergoing cardiac surgery: a single-center mechanistic randomized controlled trial. Crit Care Med. (2020) 48:e690–7. doi: 10.1097/CCM.0000000000004415
75. Candilio, L, Malik, A, Ariti, C, Barnard, M, Di Salvo, C, Lawrence, D, et al. Effect of remote ischaemic preconditioning on clinical outcomes in patients undergoing cardiac bypass surgery: a randomised controlled clinical trial. Heart. (2015) 101:185–92. doi: 10.1136/heartjnl-2014-306178
76. Hamarneh, A, Ho, AFW, Bulluck, H, Sivaraman, V, Ricciardi, F, Nicholas, J, et al. Negative interaction between nitrates and remote ischemic preconditioning in patients undergoing cardiac surgery: the ERIC-GTN and ERICCA studies. Basic Res Cardiol. (2022) 117:31. doi: 10.1007/s00395-022-00938-3
77. Kottenberg, E, Thielmann, M, Bergmann, L, Heine, T, Jakob, H, Heusch, G, et al. Protection by remote ischemic preconditioning during coronary artery bypass graft surgery with isoflurane but not propofol - a clinical trial. Acta Anaesthesiol Scand. (2012) 56:30–8. doi: 10.1111/j.1399-6576.2011.02585.x
78. Smul, TM, Stumpner, J, Blomeyer, C, Lotz, C, Redel, A, Lange, M, et al. Propofol inhibits desflurane-induced preconditioning in rabbits. J Cardiothorac Vasc Anesth. (2011) 25:276–81. doi: 10.1053/j.jvca.2010.07.018
79. Behmenburg, F, van Caster, P, Bunte, S, Brandenburger, T, Heinen, A, Hollmann, MW, et al. Impact of anesthetic regimen on remote ischemic preconditioning in the rat heart in vivo. Anesth Analg. (2018) 126:1377–80. doi: 10.1213/ANE.0000000000002563
80. Long, YQ, Feng, XM, Shan, XS, Chen, QC, Xia, Z, Ji, FH, et al. Remote ischemic preconditioning reduces acute kidney injury after cardiac surgery: a systematic review and Meta-analysis of randomized controlled trials. Anesth Analg. (2022) 134:592–605. doi: 10.1213/ANE.0000000000005804
81. Doenst, T, Wijeysundera, D, Karkouti, K, Zechner, C, Maganti, M, Rao, V, et al. Hyperglycemia during cardiopulmonary bypass is an independent risk factor for mortality in patients undergoing cardiac surgery. J Thorac Cardiovasc Surg. (2005) 130:1144.e1–8. doi: 10.1016/j.jtcvs.2005.05.049
82. Ouattara, A, Lecomte, P, Le Manach, Y, Landi, M, Jacqueminet, S, Platonov, I, et al. Poor intraoperative blood glucose control is associated with a worsened hospital outcome after cardiac surgery in diabetic patients. Anesthesiology. (2005) 103:687–94. doi: 10.1097/00000542-200510000-00006
83. Ascione, R, Rogers, CA, Rajakaruna, C, and Angelini, GD. Inadequate blood glucose control is associated with in-hospital mortality and morbidity in diabetic and nondiabetic patients undergoing cardiac surgery. Circulation. (2008) 118:113–23. doi: 10.1161/CIRCULATIONAHA.107.706416
84. Gross, ER, LaDisa, JF Jr, Weihrauch, D, Olson, LE, Kress, TT, Hettrick, DA, et al. Reactive oxygen species modulate coronary wall shear stress and endothelial function during hyperglycemia. Am J Physiol Heart Circ Physiol. (2003) 284:H1552–9. doi: 10.1152/ajpheart.01013.2002
85. Amour, J, Brzezinska, AK, Jager, Z, Sullivan, C, Weihrauch, D, Du, J, et al. Hyperglycemia adversely modulates endothelial nitric oxide synthase during anesthetic preconditioning through tetrahydrobiopterin- and heat shock protein 90-mediated mechanisms. Anesthesiology. (2010) 112:576–85. doi: 10.1097/ALN.0b013e3181cded1f
86. Mapanga, RF, and Essop, MF. Damaging effects of hyperglycemia on cardiovascular function: spotlight on glucose metabolic pathways. Am J Physiol Heart Circ Physiol. (2016) 310:H153–73. doi: 10.1152/ajpheart.00206.2015
87. Kersten, JR, Toller, WG, Gross, ER, Pagel, PS, and Warltier, DC. Diabetes abolishes ischemic preconditioning: role of glucose, insulin, and osmolality. Am J Physiol Heart Circ Physiol. (2000) 278:H1218–24. doi: 10.1152/ajpheart.2000.278.4.H1218
88. Kehl, F, Krolikowski, JG, Mraovic, B, Pagel, PS, Warltier, DC, and Kersten, JR. Hyperglycemia prevents isoflurane-induced preconditioning against myocardial infarction. Anesthesiology. (2002) 96:183–8. doi: 10.1097/00000542-200201000-00032
89. Huhn, R, Heinen, A, Weber, NC, Hollmann, MW, Schlack, W, and Preckel, B. Hyperglycaemia blocks sevoflurane-induced postconditioning in the rat heart in vivo: cardioprotection can be restored by blocking the mitochondrial permeability transition pore. Br J Anaesth. (2008) 100:465–71. doi: 10.1093/bja/aen022
90. Canfield, SG, Zaja, I, Godshaw, B, Twaroski, D, Bai, X, and Bosnjak, ZJ. High glucose attenuates anesthetic Cardioprotection in stem-cell-derived cardiomyocytes: the role of reactive oxygen species and mitochondrial fission. Anesth Analg. (2016) 122:1269–79. doi: 10.1213/ANE.0000000000001254
91. Jonassen, AK, Sack, MN, Mjos, OD, and Yellon, DM. Myocardial protection by insulin at reperfusion requires early administration and is mediated via Akt and p70s6 kinase cell-survival signaling. Circ Res. (2001) 89:1191–8. doi: 10.1161/hh2401.101385
92. Sack, MN, and Yellon, DM. Insulin therapy as an adjunct to reperfusion after acute coronary ischemia: a proposed direct myocardial cell survival effect independent of metabolic modulation. J Am Coll Cardiol. (2003) 41:1404–7. doi: 10.1016/s0735-1097(03)00164-5
93. Ng, KW, Allen, ML, Desai, A, Macrae, D, and Pathan, N. Cardioprotective effects of insulin: how intensive insulin therapy may benefit cardiac surgery patients. Circulation. (2012) 125:721–8. doi: 10.1161/CIRCULATIONAHA.111.063784
94. Furnary, AP, Gao, G, Grunkemeier, GL, Wu, Y, Zerr, KJ, Bookin, SO, et al. Continuous insulin infusion reduces mortality in patients with diabetes undergoing coronary artery bypass grafting. J Thorac Cardiovasc Surg. (2003) 125:1007–21. doi: 10.1067/mtc.2003.181
95. Subramaniam, B, Panzica, PJ, Novack, V, Mahmood, F, and Matyal, R. Continuous perioperative insulin infusion decreases major cardiovascular events in patients undergoing vascular surgery: a prospective, randomized trial. Anesthesiology. (2009) 110:970–7. doi: 10.1097/ALN.0b013e3181a1005b
96. Egi, M, Bellomo, R, Stachowski, E, French, CJ, and Hart, G. Variability of blood glucose concentration and short-term mortality in critically ill patients. Anesthesiology. (2006) 105:244–52. doi: 10.1097/00000542-200608000-00006
97. van den Berghe, G, Wouters, P, Weekers, F, Verwaest, C, Bruyninckx, F, Schetz, M, et al. Intensive insulin therapy in critically ill patients. N Engl J Med. (2001) 345:1359–67. doi: 10.1056/NEJMoa011300
98. Gandhi, GY, Nuttall, GA, Abel, MD, Mullany, CJ, Schaff, HV, O'Brien, PC, et al. Intensive intraoperative insulin therapy versus conventional glucose management during cardiac surgery: a randomized trial. Ann Intern Med. (2007) 146:233–43. doi: 10.7326/0003-4819-146-4-200702200-00002
99. Saager, L, Duncan, AE, Yared, JP, Hesler, BD, You, J, Deogaonkar, A, et al. Intraoperative tight glucose control using hyperinsulinemic normoglycemia increases delirium after cardiac surgery. Anesthesiology. (2015) 122:1214–23. doi: 10.1097/ALN.0000000000000669
100. Duncan, AE, Abd-Elsayed, A, Maheshwari, A, Xu, M, Soltesz, E, and Koch, CG. Role of intraoperative and postoperative blood glucose concentrations in predicting outcomes after cardiac surgery. Anesthesiology. (2010) 112:860–71. doi: 10.1097/ALN.0b013e3181d3d4b4
101. Duncan, AE, Sessler, DI, Sato, H, Sato, T, Nakazawa, K, Carvalho, G, et al. Hyperinsulinemic normoglycemia during cardiac surgery reduces a composite of 30-day mortality and serious in-hospital complications: a randomized clinical trial. Anesthesiology. (2018) 128:1125–39. doi: 10.1097/ALN.0000000000002156
102. Lazar, HL, McDonnell, M, Chipkin, SR, Furnary, AP, Engelman, RM, Sadhu, AR, et al. Society of Thoracic Surgeons blood glucose guideline task force. The Society of Thoracic Surgeons practice guideline series: blood glucose management during adult cardiac surgery. Ann Thorac Surg. (2009) 87:663–9. doi: 10.1016/j.athoracsur.2008.11.011
103. Sebranek, JJ, Lugli, AK, and Coursin, DB. Glycaemic control in the perioperative period. Br J Anaesth. (2013) 111:i18–34. doi: 10.1093/bja/aet381
104. Reddy, P, Duggar, B, and Butterworth, J. Blood glucose management in the patient undergoing cardiac surgery: a review. World J Cardiol. (2014) 6:1209–17. doi: 10.4330/wjc.v6.i11.1209
105. Duggan, EW, Carlson, K, and Umpierrez, GE. Perioperative hyperglycemia management: an update. Anesthesiology. (2017) 126:547–60. doi: 10.1097/ALN.0000000000001515
106. Galindo, RJ, Fayfman, M, and Umpierrez, GE. Perioperative Management of Hyperglycemia and Diabetes in cardiac surgery patients. Endocrinol Metab Clin N Am. (2018) 47:203–22. doi: 10.1016/j.ecl.2017.10.005
107. Zweier, JL, and Talukder, MA. The role of oxidants and free radicals in reperfusion injury. Cardiovasc Res. (2006) 70:181–90. doi: 10.1016/j.cardiores.2006.02.025
108. Peng, YW, Mohammed, A, Deatrick, KB, Major, T, Cheng, D, Charpie, I, et al. Differential effects of normoxic and Hyperoxic reperfusion on global myocardial ischemia-reperfusion injury. Semin Thorac Cardiovasc Surg. (2019) 31:188–98. doi: 10.1053/j.semtcvs.2018.09.018
109. Moradkhan, R, and Sinoway, LI. Revisiting the role of oxygen therapy in cardiac patients. J Am Coll Cardiol. (2010) 56:1013–6. doi: 10.1016/j.jacc.2010.04.052
110. Farquhar, H, Weatherall, M, Wijesinghe, M, Perrin, K, Ranchord, A, Simmonds, M, et al. Systematic review of studies of the effect of hyperoxia on coronary blood flow. Am Heart J. (2009) 158:371–7. doi: 10.1016/j.ahj.2009.05.037
111. Hofmann, R, James, SK, Jernberg, T, Lindahl, B, Erlinge, D, and Witt, N. Et al; DETO2X–SWEDEHEART Investigators. Oxygen therapy in suspected acute myocardial infarction. N Engl J Med. (2017) 377:1240–9. doi: 10.1056/NEJMoa1706222
112. Stub, D, Smith, K, Bernard, S, Nehme, Z, Stephenson, M, Bray, JE, et al. Air versus oxygen in ST-segment-elevation myocardial infarction. Circulation. (2015) 131:2143–50. doi: 10.1161/CIRCULATIONAHA.114.014494
113. Sepehrvand, N, James, SK, Stub, D, Khoshnood, A, Ezekowitz, JA, and Hofmann, R. Effects of supplemental oxygen therapy in patients with suspected acute myocardial infarction: a meta-analysis of randomised clinical trials. Heart. (2018) 104:1691–8. doi: 10.1136/heartjnl-2018-313089
114. Ferrando, C, Soro, M, and Belda, FJ. Protection strategies during cardiopulmonary bypass: ventilation, anesthetics and oxygen. Curr Opin Anaesthesiol. (2015) 28:73–80. doi: 10.1097/ACO.0000000000000143
115. Heinrichs, J, and Grocott, HP. Pro: Hyperoxia should be used during cardiac surgery. J Cardiothorac Vasc Anesth. (2019) 33:2070–4. doi: 10.1053/j.jvca.2018.02.015
116. Roberts, SM, and Cios, TJ. Con: Hyperoxia should not be used routinely in the Management of Cardiopulmonary Bypass. J Cardiothorac Vasc Anesth. (2019) 33:2075–8. doi: 10.1053/j.jvca.2019.02.027
117. Caputo, M, Mokhtari, A, Miceli, A, Ghorbel, MT, Angelini, GD, Parry, AJ, et al. Controlled reoxygenation during cardiopulmonary bypass decreases markers of organ damage, inflammation, and oxidative stress in single-ventricle patients undergoing pediatric heart surgery. J Thorac Cardiovasc Surg. (2014) 148:792–801.e8. doi: 10.1016/j.jtcvs.2014.06.001
118. Ihnken, K, Winkler, A, Schlensak, C, Sarai, K, Neidhart, G, Unkelbach, U, et al. Normoxic cardiopulmonary bypass reduces oxidative myocardial damage and nitric oxide during cardiac operations in the adult. J Thorac Cardiovasc Surg. (1998) 116:327–34. doi: 10.1016/s0022-5223(98)70134-5
119. Inoue, T, Ku, K, Kaneda, T, Zang, Z, Otaki, M, and Oku, H. Cardioprotective effects of lowering oxygen tension after aortic unclamping on cardiopulmonary bypass during coronary artery bypass grafting. Circ J. (2002) 66:718–22. doi: 10.1253/circj.66.718
120. McGuinness, SP, Parke, RL, Drummond, K, Willcox, T, Bailey, M, Kruger, C, et al. SO-COOL investigators. A multicenter, randomized, controlled phase IIb trial of avoidance of Hyperoxemia during cardiopulmonary bypass. Anesthesiology. (2016) 125:465–73. doi: 10.1097/ALN.0000000000001226
121. Lopez, MG, Hughes, CG, DeMatteo, A, O'Neal, JB, McNeil, JB, Shotwell, MS, et al. Intraoperative oxidative damage and delirium after cardiac surgery. Anesthesiology. (2020) 132:551–61. doi: 10.1097/ALN.0000000000003016
122. Cobbe, SM, and Poole-Wilson, PA. The time of onset and severity of acidosis in myocardial ischaemia. J Mol Cell Cardiol. (1980) 12:745–60. doi: 10.1016/0022-2828(80)90077-2
123. Currin, RT, Gores, GJ, Thurman, RG, and Lemasters, JJ. Protection by acidotic pH against anoxic cell killing in perfused rat liver: evidence for a pH paradox. FASEB J. (1991) 5:207–10. doi: 10.1096/fasebj.5.2.2004664
124. Bond, JM, Harper, IS, Chacon, E, Reece, JM, Herman, B, and Lemasters, JJ. The pH paradox in the pathophysiology of reperfusion injury to rat neonatal cardiac myocytes. Ann N Y Acad Sci. (1994) 723:25–37. doi: 10.1111/j.1749-6632.1994.tb36714.x
125. Kitakaze, M, Takashima, S, Funaya, H, Minamino, T, Node, K, Shinozaki, Y, et al. Temporary acidosis during reperfusion limits myocardial infarct size in dogs. Am J Phys. (1997) 272:H2071–8. doi: 10.1152/ajpheart.1997.272.5.H2071
126. Kim, JS, Jin, Y, and Lemasters, JJ. Reactive oxygen species, but not Ca2+ overloading, trigger pH- and mitochondrial permeability transition-dependent death of adult rat myocytes after ischemia-reperfusion. Am J Physiol Heart Circ Physiol. (2006) 290:H2024–34. doi: 10.1152/ajpheart.00683.2005
127. Kaplan, SH, Yang, H, Gilliam, DE, Shen, J, Lemasters, JJ, and Cascio, WE. Hypercapnic acidosis and dimethyl amiloride reduce reperfusion induced cell death in ischaemic ventricular myocardium. Cardiovasc Res. (1995) 29:231–8. doi: 10.1016/S0008-6363(96)88575-0
128. Ohashi, T, Yamamoto, F, Yamamoto, H, Ichikawa, H, Shibata, T, and Kawashima, Y. Transient reperfusion with acidic solution affects postischemic functional recovery: studies in the isolated working rat heart. J Thorac Cardiovasc Surg. (1996) 111:613–20. doi: 10.1016/s0022-5223(96)70313-6
129. Inserte, J, Barba, I, Hernando, V, Abellán, A, Ruiz-Meana, M, Rodríguez-Sinovas, A, et al. Effect of acidic reperfusion on prolongation of intracellular acidosis and myocardial salvage. Cardiovasc Res. (2008) 77:782–90. doi: 10.1093/cvr/cvm082
130. Fujita, M, Asanuma, H, Hirata, A, Wakeno, M, Takahama, H, Sasaki, H, et al. Prolonged transient acidosis during early reperfusion contributes to the cardioprotective effects of postconditioning. Am J Physiol Heart Circ Physiol. (2007) 292:H2004–8. doi: 10.1152/ajpheart.01051.2006
131. Cohen, MV, Yang, XM, and Downey, JM. The pH hypothesis of postconditioning: staccato reperfusion reintroduces oxygen and perpetuates myocardial acidosis. Circulation. (2007) 115:1895–903. doi: 10.1161/CIRCULATIONAHA.106.675710
132. Inserte, J, Barba, I, Hernando, V, and Garcia-Dorado, D. Delayed recovery of intracellular acidosis during reperfusion prevents calpain activation and determines protection in postconditioned myocardium. Cardiovasc Res. (2009) 81:116–22. doi: 10.1093/cvr/cvn260
133. Inserte, J, Ruiz-Meana, M, Rodríguez-Sinovas, A, Barba, I, and Garcia-Dorado, D. Contribution of delayed intracellular pH recovery to ischemic postconditioning protection. Antioxid Redox Signal. (2011) 14:923–39. doi: 10.1089/ars.2010.3312
134. Hartmann, M, and Decking, UK. Blocking Na(+)-H+ exchange by cariporide reduces Na(+)-overload in ischemia and is cardioprotective. J Mol Cell Cardiol. (1999) 31:1985–95. doi: 10.1006/jmcc.1999.1029
135. Avkiran, M, and Marber, MS. Na(+)/H(+) exchange inhibitors for cardioprotective therapy: progress, problems and prospects. J Am Coll Cardiol. (2002) 39:747–53. doi: 10.1016/s0735-1097(02)01693-5
136. Strömer, H, de Groot, MC, Horn, M, Faul, C, Leupold, A, Morgan, JP, et al. Na(+)/H(+) exchange inhibition with HOE642 improves postischemic recovery due to attenuation of ca(2+) overload and prolonged acidosis on reperfusion. Circulation. (2000) 101:2749–55. doi: 10.1161/01.cir.101.23.2749
137. Mentzer, RM Jr, Bartels, C, Bolli, R, Boyce, S, Buckberg, GD, Chaitman, B, et al. EXPEDITION study Investigators. Sodium-hydrogen exchange inhibition by cariporide to reduce the risk of ischemic cardiac events in patients undergoing coronary artery bypass grafting: results of the EXPEDITION study. Ann Thorac Surg. (2008) 85:1261–70. doi: 10.1016/j.athoracsur.2007.10.054
138. Okamoto, F, Allen, BS, Buckberg, GD, Bugyi, H, and Leaf, J. Reperfusion conditions: importance of ensuring gentle versus sudden reperfusion during relief of coronary occlusion. J Thorac Cardiovasc Surg. (1986) 92:613–20. doi: 10.1016/S0022-5223(19)36512-2
139. Hori, M, Kitakaze, M, Sato, H, Takashima, S, Iwakura, K, Inoue, M, et al. Staged reperfusion attenuates myocardial stunning in dogs. Role of transient acidosis during early reperfusion. Circulation. (1991) 84:2135–45. doi: 10.1161/01.cir.84.5.2135
140. Peng, CF, Murphy, ML, Colwell, K, and Straub, KD. Controlled versus hyperemic flow during reperfusion of jeopardized ischemic myocardium. Am Heart J. (1989) 117:515–22. doi: 10.1016/0002-8703(89)90723-0
141. Bopassa, JC, Michel, P, Gateau-Roesch, O, Ovize, M, and Ferrera, R. Low-pressure reperfusion alters mitochondrial permeability transition. Am J Physiol Heart Circ Physiol. (2005) 288:H2750–5. doi: 10.1152/ajpheart.01081.2004
142. Bopassa, JC, Ferrera, R, Gateau-Roesch, O, Couture-Lepetit, E, and Ovize, M. PI 3-kinase regulates the mitochondrial transition pore in controlled reperfusion and postconditioning. Cardiovasc Res. (2006) 69:178–85. doi: 10.1016/j.cardiores.2005.07.014
143. Lindal, S, Gunnes, S, Lund, I, Straume, BK, Jørgensen, L, and Sørlie, D. Myocardial and microvascular injury following coronary surgery and its attenuation by mode of reperfusion. Eur J Cardiothorac Surg. (1995) 9:83–9. doi: 10.1016/s1010-7940(05)80024-7
144. Beyersdorf, F. The use of controlled reperfusion strategies in cardiac surgery to minimize ischaemia/reperfusion damage. Cardiovasc Res. (2009) 83:262–8. doi: 10.1093/cvr/cvp110
145. Williams, JB, Hernandez, AF, Li, S, Dokholyan, RS, O'Brien, SM, Smith, PK, et al. Postoperative inotrope and vasopressor use following CABG: outcome data from the CAPS-care study. J Card Surg. (2011) 26:572–8. doi: 10.1111/j.1540-8191.2011.01301.x
146. Belletti, A, Castro, ML, Silvetti, S, Greco, T, Biondi-Zoccai, G, Pasin, L, et al. The effect of inotropes and vasopressors on mortality: a meta-analysis of randomized clinical trials. Br J Anaesth. (2015) 115:656–75. doi: 10.1093/bja/aev284
147. Fellahi, JL, Fischer, MO, Daccache, G, Gerard, JL, and Hanouz, JL. Positive inotropic agents in myocardial ischemia-reperfusion injury: a benefit/risk analysis. Anesthesiology. (2013) 118:1460–5. doi: 10.1097/ALN.0b013e31828f4fc3
148. Fellahi, JL, Parienti, JJ, Hanouz, JL, Plaud, B, Riou, B, and Ouattara, A. Perioperative use of dobutamine in cardiac surgery and adverse cardiac outcome: propensity-adjusted analyses. Anesthesiology. (2008) 108:979–87. doi: 10.1097/ALN.0b013e318173026f
149. Nielsen, DV, Hansen, MK, Johnsen, SP, Hansen, M, Hindsholm, K, and Jakobsen, CJ. Health outcomes with and without use of inotropic therapy in cardiac surgery: results of a propensity score-matched analysis. Anesthesiology. (2014) 120:1098–108. doi: 10.1097/ALN.0000000000000224
150. Ray, KK, and Cannon, CP. The potential relevance of the multiple lipid-independent (pleiotropic) effects of statins in the management of acute coronary syndromes. J Am Coll Cardiol. (2005) 46:1425–33. doi: 10.1016/j.jacc.2005.05.086
151. Ouattara, A, Benhaoua, H, Le Manach, Y, Mabrouk-Zerguini, N, Itani, O, Osman, A, et al. Perioperative statin therapy is associated with a significant and dose-dependent reduction of adverse cardiovascular outcomes after coronary artery bypass graft surgery. J Cardiothorac Vasc Anesth. (2009) 23:633–8. doi: 10.1053/j.jvca.2009.02.008
152. Ludman, AJ, Hausenloy, DJ, Babu, G, Hasleton, J, Venugopal, V, Boston-Griffiths, E, et al. Failure to recapture cardioprotection with high-dose atorvastatin in coronary artery bypass surgery: a randomised controlled trial. Basic Res Cardiol. (2011) 106:1387–95. doi: 10.1007/s00395-011-0209-5
153. Allah, EA, Kamel, EZ, Osman, HM, Abd-Elshafy, SK, Nabil, F, Elmelegy, TTH, et al. Could short-term perioperative high-dose atorvastatin offer antiarrhythmic and cardio-protective effects in rheumatic valve replacement surgery? J Cardiothorac Vasc Anesth. (2019) 33:3340–7. doi: 10.1053/j.jvca.2019.05.013
154. Liakopoulos, OJ, Kuhn, EW, Hellmich, M, Schlömicher, M, Strauch, J, Reents, W, et al. StaRT-CABG Investigators. Statin loading before coronary artery bypass grafting: a randomized trial. Eur Heart J. (2023) 44:2322–31. doi: 10.1093/eurheartj/ehad238
155. Le Manach, Y, Godet, G, Coriat, P, Martinon, C, Bertrand, M, Fléron, MH, et al. The impact of postoperative discontinuation or continuation of chronic statin therapy on cardiac outcome after major vascular surgery. Anesth Analg. (2007) 104:1326–33. doi: 10.1213/01.ane.0000263029.72643.10
156. Le Manach, Y, Coriat, P, Collard, CD, and Riedel, B. Statin therapy within the perioperative period. Anesthesiology. (2008) 108:1141–6. doi: 10.1097/ALN.0b013e318173ef8e
157. Boengler, K, Schulz, R, and Heusch, G. Loss of cardioprotection with ageing. Cardiovasc Res. (2009) 83:247–61. doi: 10.1093/cvr/cvp033
158. Lesnefsky, EJ, Moghaddas, S, Tandler, B, Kerner, J, and Hoppel, CL. Mitochondrial dysfunction in cardiac disease: ischemia--reperfusion, aging, and heart failure. J Mol Cell Cardiol. (2001) 33:1065–89. doi: 10.1006/jmcc.2001.1378
159. Taylor, RP, and Starnes, JW. Age, cell signalling and cardioprotection. Acta Physiol Scand. (2003) 178:107–16. doi: 10.1046/j.1365-201X.2003.01132.x
160. Schulman, D, Latchman, DS, and Yellon, DM. Effect of aging on the ability of preconditioning to protect rat hearts from ischemia-reperfusion injury. Am J Physiol Heart Circ Physiol. (2001) 281:H1630–6. doi: 10.1152/ajpheart.2001.281.4.H1630
161. Sniecinski, R, and Liu, H. Reduced efficacy of volatile anesthetic preconditioning with advanced age in isolated rat myocardium. Anesthesiology. (2004) 100:589–97. doi: 10.1097/00000542-200403000-00019
162. Lee, TM, Su, SF, Tsai, CC, Lee, YT, and Tsai, CH. Cardioprotective effects of 17 beta-estradiol produced by activation of mitochondrial ATP-sensitive K(+)channels in canine hearts. J Mol Cell Cardiol. (2000) 32:1147–58. doi: 10.1006/jmcc.2000.1167
163. Kim, YD, Chen, B, Beauregard, J, Kouretas, P, Thomas, G, Farhat, MY, et al. 17 beta-estradiol prevents dysfunction of canine coronary endothelium and myocardium and reperfusion arrhythmias after brief ischemia/reperfusion. Circulation. (1996) 94:2901–8. doi: 10.1161/01.cir.94.11.2901
164. Miyawaki, H, Wang, Y, and Ashraf, M. Oxidant stress with hydrogen peroxide attenuates calcium paradox injury: role of protein kinase C and ATP-sensitive potassium channel. Cardiovasc Res. (1998) 37:691–9. doi: 10.1016/s0008-6363(97)00249-6
165. Node, K, Kitakaze, M, Kosaka, H, Minamino, T, Funaya, H, and Hori, M. Amelioration of ischemia- and reperfusion-induced myocardial injury by 17beta-estradiol: role of nitric oxide and calcium-activated potassium channels. Circulation. (1997) 96:1953–63. doi: 10.1161/01.cir.96.6.1953
166. Wang, C, Chiari, PC, Weihrauch, D, Krolikowski, JG, Warltier, DC, Kersten, JR, et al. Gender-specificity of delayed preconditioning by isoflurane in rabbits: potential role of endothelial nitric oxide synthase. Anesth Analg. (2006) 103:274–80. doi: 10.1213/01.ANE.0000230389.76351.0C
167. Song, X, Li, G, Vaage, J, and Valen, G. Effects of sex, gonadectomy, and oestrogen substitution on ischaemic preconditioning and ischaemia-reperfusion injury in mice. Acta Physiol Scand. (2003) 177:459–66. doi: 10.1046/j.1365-201X.2003.01068.x
168. Tanaka, K, Kersten, JR, and Riess, ML. Opioid-induced cardioprotection. Curr Pharm Des. (2014) 20:5696–705. doi: 10.2174/1381612820666140204120311
169. Headrick, JP, See Hoe, LE, Du Toit, EF, and Peart, JN. Opioid receptors and cardioprotection - 'opioidergic conditioning' of the heart. Br J Pharmacol. (2015) 172:2026–50. doi: 10.1111/bph.13042
170. Irwin, MG, and Wong, GT. Remifentanil and opioid-induced cardioprotection. J Cardiothorac Vasc Anesth. (2015) 29:S23–6. doi: 10.1053/j.jvca.2015.01.021
171. Patel, HH, Ludwig, LM, Fryer, RM, Hsu, AK, Warltier, DC, and Gross, GJ. Delta opioid agonists and volatile anesthetics facilitate cardioprotection via potentiation of K(ATP) channel opening. FASEB J. (2002) 16:1468–70. doi: 10.1096/fj.02-0170fje
172. Ludwig, LM, Patel, HH, Gross, GJ, Kersten, JR, Pagel, PS, and Warltier, DC. Morphine enhances pharmacological preconditioning by isoflurane: role of mitochondrial K(ATP) channels and opioid receptors. Anesthesiology. (2003) 98:705–11. doi: 10.1097/00000542-200303000-00019
173. Lucchinetti, E, Lou, PH, Gandhi, M, Clanachan, AS, and Zaugg, M. Differential effects of anesthetics and opioid receptor activation on Cardioprotection elicited by reactive oxygen species-mediated Postconditioning in Sprague-Dawley rat hearts. Anesth Analg. (2018) 126:1739–46. doi: 10.1213/ANE.0000000000002676
174. Han, J, Kim, N, Joo, H, and Kim, E. Ketamine abolishes ischemic preconditioning through inhibition of K(ATP) channels in rabbit hearts. Am J Physiol Heart Circ Physiol. (2002) 283:H13–21. doi: 10.1152/ajpheart.01064.2001
175. Müllenheim, J, Frässdorf, J, Preckel, B, Thämer, V, and Schlack, W. Ketamine, but not S(+)-ketamine, blocks ischemic preconditioning in rabbit hearts in vivo. Anesthesiology. (2001) 94:630–6. doi: 10.1097/00000542-200104000-00017
176. Müllenheim, J, Rulands, R, Wietschorke, T, Frässdorf, J, Preckel, B, and Schlack, W. Late preconditioning is blocked by racemic ketamine, but not by S(+)-ketamine. Anesth Analg. (2001) 93:265–70. doi: 10.1097/00000539-200108000-00005
177. Barthel, H, Ebel, D, Müllenheim, J, Obal, D, Preckel, B, and Schlack, W. Effect of lidocaine on ischaemic preconditioning in isolated rat heart. Br J Anaesth. (2004) 93:698–704. doi: 10.1093/bja/aeh262
178. Kaczmarek, DJ, Herzog, C, Larmann, J, Gillmann, HJ, Hildebrand, R, Schmitz, M, et al. Lidocaine protects from myocardial damage due to ischemia and reperfusion in mice by its antiapoptotic effects. Anesthesiology. (2009) 110:1041–9. doi: 10.1097/ALN.0b013e31819dabda
179. Lee, EH, Lee, HM, Chung, CH, Chin, JH, Choi, DK, Chung, HJ, et al. Impact of intravenous lidocaine on myocardial injury after off-pump coronary artery surgery. Br J Anaesth. (2011) 106:487–93. doi: 10.1093/bja/aeq416
180. Roehl, AB, Funcke, S, Becker, MM, Goetzenich, A, Bleilevens, C, Rossaint, R, et al. Xenon and isoflurane reduce left ventricular remodeling after myocardial infarction in the rat. Anesthesiology. (2013) 118:1385–94. doi: 10.1097/ALN.0b013e31828744c0
181. Schwiebert, C, Huhn, R, Heinen, A, Weber, NC, Hollmann, MW, Schlack, W, et al. Postconditioning by xenon and hypothermia in the rat heart in vivo. Eur J Anaesthesiol. (2010) 27:734–9. doi: 10.1097/EJA.0b013e328335fc4c
182. Hofland, J, Ouattara, A, Fellahi, JL, Gruenewald, M, Hazebroucq, J, Ecoffey, C, et al. Effect of xenon anesthesia compared to sevoflurane and Total intravenous anesthesia for coronary artery bypass graft surgery on postoperative cardiac troponin release: an international, multicenter, phase 3, single-blinded, randomized noninferiority trial. Anesthesiology. (2017) 127:918–33. doi: 10.1097/ALN.0000000000001873
183. Javadov, S, and Karmazyn, M. Mitochondrial permeability transition pore opening as an endpoint to initiate cell death and as a putative target for cardioprotection. Cell Physiol Biochem. (2007) 20:1–22. doi: 10.1159/000103747
184. Dj, H, Kunst, G, Boston-Griffiths, E, Kolvekar, S, Chaubey, S, John, L, et al. The effect of cyclosporin-a on peri-operative myocardial injury in adult patients undergoing coronary artery bypass graft surgery: a randomised controlled clinical trial. Heart. (2014) 100:544–9. doi: 10.1136/heartjnl-2013-304845
185. Chiari, P, Angoulvant, D, Mewton, N, Desebbe, O, Obadia, JF, Robin, J, et al. Cyclosporine protects the heart during aortic valve surgery. Anesthesiology. (2014) 121:232–8. doi: 10.1097/ALN.0000000000000331
186. Cung, TT, Morel, O, Cayla, G, Rioufol, G, Garcia-Dorado, D, Angoulvant, D, et al. Cyclosporine before PCI in patients with acute myocardial infarction. N Engl J Med. (2015) 373:1021–31. doi: 10.1056/NEJMoa1505489
187. Fan, Y, Yang, S, Zhang, X, Cao, Y, and Huang, Y. Comparison of cardioprotective efficacy resulting from a combination of atorvastatin and ischaemic post-conditioning in diabetic and non-diabetic rats. Clin Exp Pharmacol Physiol. (2012) 39:938–43. doi: 10.1111/1440-1681.12014
188. Yang, XM, Cui, L, Alhammouri, A, Downey, JM, and Cohen, MV. Triple therapy greatly increases myocardial salvage during ischemia/reperfusion in the in situ rat heart. Cardiovasc Drugs Ther. (2013) 27:403–12. doi: 10.1007/s10557-013-6474-9
189. Prunier, F, Angoulvant, D, Saint Etienne, C, Vermes, E, Gilard, M, Piot, C, et al. The RIPOST-MI study, assessing remote ischemic perconditioning alone or in combination with local ischemic postconditioning in ST-segment elevation myocardial infarction. Basic Res Cardiol. (2014) 109:400. doi: 10.1007/s00395-013-0400-y
190. Eitel, I, Stiermaier, T, Rommel, KP, Fuernau, G, Sandri, M, Mangner, N, et al. Cardioprotection by combined intrahospital remote ischaemic perconditioning and postconditioning in ST-elevation myocardial infarction: the randomized LIPSIA CONDITIONING trial. Eur Heart J. (2015) 36:3049–57. doi: 10.1093/eurheartj/ehv463
191. Chiari, P, Mewton, N, Maucort-Boulch, D, Desebbe, O, Durand, M, Fischer, MO, et al. Multimodal strategy for myocardial protection during cardiac surgery: the ProCCard study. J Am Coll Cardiol. (2021) 77:827–9. doi: 10.1016/j.jacc.2020.12.020
Keywords: cardiac surgery, ischemia–reperfusion injury, myocardial protection, preconditioning, postconditioning
Citation: Chiari P and Fellahi J-L (2024) Myocardial protection in cardiac surgery: a comprehensive review of current therapies and future cardioprotective strategies. Front. Med. 11:1424188. doi: 10.3389/fmed.2024.1424188
Edited by:
Emmanuel Besnier, Centre Hospitalier Universitaire (CHU) de Rouen, FranceReviewed by:
Yong tao Sun, The First Affiliated Hospital of Shandong First Medical University and Shandong Provincial Qianfoshan Hospital, ChinaAly Elezaby, Stanford Healthcare, United States
Copyright © 2024 Chiari and Fellahi. This is an open-access article distributed under the terms of the Creative Commons Attribution License (CC BY). The use, distribution or reproduction in other forums is permitted, provided the original author(s) and the copyright owner(s) are credited and that the original publication in this journal is cited, in accordance with accepted academic practice. No use, distribution or reproduction is permitted which does not comply with these terms.
*Correspondence: Jean-Luc Fellahi, jean-luc.fellahi@chu-lyon.fr