- Department of Emergency Medicine, Affiliated Kunshan Hospital of Jiangsu University, Kunshan, China
Intracerebral hemorrhage (ICH) stands as a prevalent and pivotal clinical condition. The potential cooccurrence of acute kidney injury (AKI) among afflicted individuals can profoundly influence their prognosis. In recent times, there has been a growing focus among clinical practitioners on researching the relationship between ICH and AKI. AKI occurring concurrently with ICH predominantly arises from both hemodynamic and non-hemodynamic mechanisms. The latter encompasses neurohumoral regulation, inflammatory response, oxidative stress, and iatrogenic factors such as contrast agents, dehydrating agents, antibiotics, and diuretics. Moreover, advanced age, hypertension, elevated baseline creatinine levels, chronic kidney disease, and larger hematomas predispose patients to AKI. Additionally, the current utilization of biomarkers and the development of predictive models appear promising in identifying patients at risk of AKI after ICH. This article aims to underscore the potential of the aforementioned insights to inspire novel approaches to early clinical intervention.
Background
Intracerebral hemorrhage (ICH) represents a subtype of stroke, comprising 15–30% of all stroke cases (1, 2). The prognosis for individuals affected by ICH is frequently bleak, with many experiencing residual neuropsychiatric symptoms that hinder daily functioning. Studies have documented the mortality rate of ICH as elevated as 50% (3). Acute kidney injury (AKI) is a frequent clinical complication in individuals with ICH. However, due to variations in AKI definitions and differences in study populations, the reported incidence of AKI in ICH patients varies considerably. Studies indicated an incidence ranging from approximately 15–40% (4–6), with the incidence of AKI necessitating dialysis reaching up to 3.5% (7). Patients experiencing AKI following ICH demonstrate elevated mortality rates and a heightened incidence of neurological deterioration compared to those without AKI (8, 9). Therefore, comprehending the pathophysiological mechanisms, risk factors, and current methodologies for AKI diagnosis in individuals with ICH may hold significant importance for patient prognosis.
The pathological mechanisms of AKI in ICH patients
The pathophysiological mechanisms of AKI following ICH are complex, involving both hemodynamic and non-hemodynamic factors that interact and promote each other. Non-hemodynamic mechanisms include neurohumoral mechanisms, inflammation and oxidative stress, and iatrogenic factors such as the use of contrast medium, mannitol, and nephrotoxic drugs. We primarily discuss widely accepted pathophysiological mechanisms (Figure 1). However, the exact mechanisms remain inconclusive, and further experimental research is needed to explore these potential pathways.
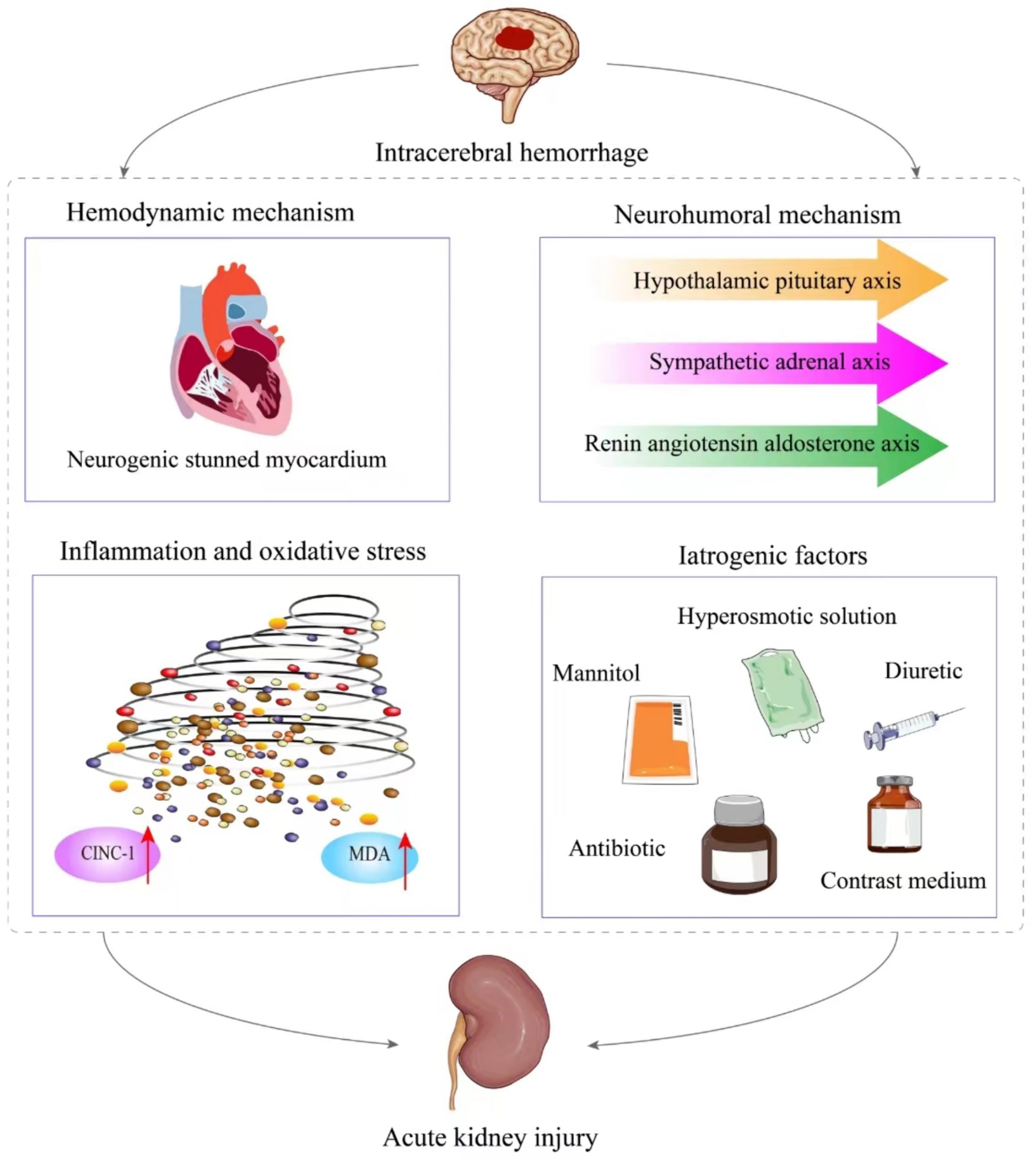
Figure 1. The pathological mechanism of acute kidney injury after intracerebral hemorrhage. It is mainly divided into hemodynamic mechanism and non-hemodynamic mechanism. The latter includes neurohumoral mechanism, inflammation and oxidative stress, as well as iatrogenic factors.
Hemodynamic mechanisms
ICH can induce neurogenic stunned myocardium. Intracranial pressure increases during brain injury, affecting the right insular cortex/Amygdala Hypothalamus, leading to autonomic nervous dysfunction and significant sympathetic system activation. Cardiomyocytes release excessive catecholamines, which open calcium channels and cause contraction band necrosis and myocyte dysfunction (10). This results in neurogenic stunning of the myocardium, leading to kidney injury through two main pathways. Firstly, myocardial cell damage and heart pump function failure lead to a reduction in cardiac output and impaired blood circulation. This compromised circulation fails to meet the normal renal perfusion requirements, resulting in kidney ischemia, hypoxia, and subsequent kidney injury. Moreover, to prioritize blood perfusion to vital organs like the brain and lungs, the renin-angiotensin-aldosterone system is activated. This activation leads to renal artery constriction, exacerbating the decrease in renal perfusion and contributing to the development of AKI (11). Secondly, neurogenic stunned myocardium after intracerebral hemorrhage can lead to congestive heart failure, with or without pulmonary edema (12). Blood stasis in the pulmonary capillary network increases vena cava pressure, blocks venous return, causes renal vein congestion, and decreases the glomerular filtration rate (13).
Non hemodynamic mechanisms
Neurohumoral mechanisms
When patients experience ICH, activation of the hypothalamic–pituitary axis, sympathetic-adrenal axis, and renin-angiotensin-aldosterone axis occur. Animal models have further substantiated this by demonstrating a significant increase in cortisol, adrenaline, and angiotensin II levels in collagenase-induced ICH mice, indicating activation of these three systems during ICH (14, 15). Additionally, vasoconstrictors are present, contributing to the constriction of glomerular arterioles and enhancing renal tubular reabsorption, ultimately leading to a decrease in glomerular filtration rate (GFR) (16).
Inflammatory and oxidative stress
In an experiment inducing ICH in rats with collagenase, notable increases were observed in the levels of cytokine-induced neutrophil chemoattractant-1 (CINC-1) and malondialdehyde (MDA) in urine. They also detected the presence of podocalyxin DNA, indicative of podocyte detachment (17). CINC-1 serves as a neutrophil chemotactic agent, recruiting concentrated granulocytes to trigger an inflammatory cascade response in distant organs such as the kidney (18). MDA represents the primary stable product following oxidative stress and has been extensively implicated in the pathogenesis of kidney injury (19). Hence, these findings suggest the involvement of inflammation and oxidative stress in disrupting podocyte function, consequently contributing to AKI. Additionally, the authors postulated that activation of the NF-κB pathway may also contribute to kidney injury (17). However, further fundamental research is warranted to elucidate the specific mechanism.
Iatrogenic factors
Contrast medium
Etiological identification in ICH patients often necessitates computed tomography angiography (CTA) examination for diagnostic assistance. However, the use of contrast medium poses a significant concern for AKI in such patients. The renal damage caused by contrast medium has been internationally recognized (20), particularly concerning high-osmolarity contrast medium. Close monitoring of renal function and urine output is imperative. The American Radiological Society recommended administering physiological saline hydration therapy before and after contrast medium administration to mitigate the risk of AKI (21). Nonetheless, a study reported that the incidence of AKI in ICH patients after CTA was merely 25%, and correlation analysis indicated no association between AKI and CTA performance (p = 0.187) (22). Another study also found no significant relationship between contrast medium use in CTA and AKI [Odds Ratio (OR) = 1.16, 95%Confidence interval (CI): 0.72–1.95, p = 0.5548] (23). This may be attributed to the widespread use of low-or iso-osmolar contrast medium in clinical practice, as well as the relatively small doses administered during CTA. Nevertheless, clinical vigilance regarding the frequency and dosage of contrast medium administration remains paramount, especially for patients with preexisting chronic kidney disease (CKD).
Mannitol and hypertonic solution
Patients with ICH frequently present with elevated intracranial pressure. Currently, common drugs used in clinical practice include mannitol and hypertonic sodium solutions. A multicenter study involving 9,649 cases of ICH demonstrated that mannitol significantly contributes to AKI (OR = 1.986, 95%CI: 1.426–2.813, p < 0.001) (24). Mannitol initially induces renal vasoconstriction, leading to inadequate renal perfusion. Subsequently, its robust diuretic effect may precipitate volume depletion, alter blood flow distribution, and culminate in renal medullary ischemia and hypoxia (25). Moreover, it can cause swelling of renal tubular cells, resulting in osmotic nephropathy (26). Additionally, hypernatremia and hyperchloremia induced by hypertonic solutions may also correlate with AKI (27). A study on the rapid infusion of a high-sodium solution in dogs demonstrated that despite the decrease in renin secretion, concurrent contraction of renal artery and a reduction in GFR were observed. Authors proposed that this vasoconstriction might be independent of renin and constitutes a “tubular feedback” mechanism of kidneys toward blood sodium (28). Consequently, hypernatremia from hypertonic solutions could induce kidney damage (29). Hyperchloremia is also a significant cause of AKI in ICH patients. Yunos et al. demonstrated that restricting treatment with chlorine-containing drugs significantly reduced creatinine levels compared to the control group (14.8 μmol/L vs. 22.6 μmol/L, p = 0.003). Notably, it also decreased the incidence of AKI (8.4% vs. 14%, p < 0.001) and dialysis rates (10% vs. 6.3%, p = 0.005) (30). Studies have revealed that a variety of vasoactive substances can activate chloride ion channels in vascular smooth muscle cells through calcium ions, inducing chloride ion efflux, cell membrane depolarization, and subsequent calcium ion influx, ultimately leading to vasoconstriction (31). Hence, chloride ions can induce constriction of the afferent arterioles, resulting in diminished renal perfusion and a decline in GFR. Additionally, chloride ions can elevate thromboxane synthesis, further constricting renal blood vessels (32). Furthermore, studies have indicated that hyperchloremia is a significant factor contributing to the delayed resolution of cerebral edema (OR = 5.24, 95%CI: 1.64–16.76) (33). Accordingly, certain scholars have suggested that in cases of ICH, proactive management of blood sodium and chloride levels may help mitigate the incidence of AKI and mortality (5, 34).
Nephrotoxic drugs
Some nephrotoxic drugs commonly used in clinical practice can also induce AKI in patients with ICH. Among these, antibiotics such as vancomycin and diuretics are frequently implicated. She et al. utilized the random forest algorithm and found that vancomycin emerged as a significant predictive factor for AKI in patients with ICH (35). Additionally, another study noted that diuretic escalated the risk of AKI in individuals with acute stroke (OR = 8.5, 95%CI: 3.46–20.86, p < 0.001) (26). This phenomenon could be attributed to the capacity of diuretics to alter renal microcirculation, potentially leading to renal ischemia, hypoxia, and inflammatory reactions, ultimately resulting in AKI. Consequently, it is imperative to judiciously select nephrotoxic drugs in clinical settings to mitigate adverse renal outcomes in ICH patients. Figure 1 shows the relevant mechanisms of acute kidney injury after intracerebral hemorrhage.
Risk factors for AKI in ICH patients
In patients with ICH, the risk of AKI varies. It is essential to understand the clinical characteristics of patients susceptible to AKI, including specific risk factors. This understanding can assist clinical practitioners in identifying high-risk populations for AKI among patients with ICH.
Age
Advanced age is often regarded as one of the risk factors for AKI in patients with ICH. Zhang et al.’s study on ICH patients revealed that age serves as an independent predictor of AKI, irrespective of whether the patient has CKD or not (p = 0.002 and p = 0.008) (36). Another retrospective study similarly discovered that ICH patients aged over 70 years are at higher risk of AKI (OR = 14.652, 95%CI: 1.397–153.634, p = 0.003) (37). This susceptibility in older patients may stem from insufficient residual renal units, rendering them more vulnerable to AKI triggers such as ischemia, hypoxia, inflammatory stimuli, and oxidative stress. Given the advanced age of patients with ICH, some researchers have posited that the association between age and AKI remains uncertain (38). Hence, large-scale clinical studies and fundamental experiments are warranted to provide further confirmation.
Hypertension
Hypertension, as a significant risk factor for AKI in patients with ICH, has been extensively documented. A retrospective study found that the incidence of AKI increased with the level of blood pressure at admission (mild, moderate, and severe: 28.2, 37.1, 56%, p < 0.001), with severe hypertension closely associated with AKI (OR = 2.6, 95%CI: 1.5–4.3, p < 0.001) (39). Furthermore, the magnitude of blood pressure reduction is also linked to AKI. Tanaka’s study demonstrated that the risk of AKI was significantly higher in the group with a high to low systolic blood pressure reduction compared to the group with a medium to low reduction (OR = 3.50, 95%CI: 1.83–6.69, p < 0.01) (40). Another study also observed a correlation between systolic blood pressure reduction and AKI (9). On one hand, hypertension can lead to kidney damage due to endothelial dysfunction in arteries (41). On the other hand, hypertensive patients have a high demand for renal perfusion pressure, which can result in renal ischemia and hypoxia when there is a rapid and significant reduction in blood pressure (42).
Elevated baseline creatinine and CKD
Qureshi’s research indicated that patients with ICH who had a baseline blood creatinine level ≥ 110 μmol/L faced an elevated risk of AKI and renal adverse events, with OR of 2.4 (95%CI, 1.2–4.5) and 3.1 (95%CI, 1.2–8.1), respectively (4). A study involving 1,366 ICH patients similarly highlighted creatinine as a characteristic of AKI (35). However, some studies have found no correlation between renal function and AKI, and the presence of CKD alongside AKI did not correlate with long-term mortality (OR = 3.13, 95%CI: 0.65–15.01, p = 0.154) (43). The author posited that this may be due to the resistance of patients with CKD to kidney damage. However, in reality, the mortality rate in the later stages of CKD significantly increased (41). This underscores the importance for clinicians to remain vigilant in monitoring the renal function of ICH patients. Indeed, certain risk factors for AKI in patients with ICH are commonly observed in ischemic stroke as well, including significant blood pressure fluctuations and chronic kidney disease (44, 45). This indicates that the treatment of ICH should avoid aggravating kidney injury by causing cerebral ischemia.
Large intracranial hematoma
Burgess et al.’s study revealed that compared to non-AKI patients, those with AKI presented with a larger hematoma volume upon admission (15.5 ± 17.8 vs. 12.0 ± 14.0, p = 0.045). Patients with larger hematoma volumes may experience more pronounced activation of the sympathetic nervous system, potentially leading to kidney damage through the neurohumoral regulatory mechanisms mentioned earlier (43). Furthermore, there are reports indicating abnormal coagulation function in ICH patients, which could contribute to hematoma enlargement. Clinicians should therefore closely monitor the patient’s coagulation status and judiciously select medications to mitigate the risk of kidney injury (46).
Early prediction of AKI in ICH patients
Biomarkers
Cystatin C
Cystatin C (CysC) in renal injury has been widely acknowledged (47). Jiang’s study demonstrated that the level of CysC could more accurately predict the occurrence of AKI in patients with acute stroke [Area under the Receiver Operating Characteristic Curve (AUC) = 0.772, 95%CI: 0.726–0.813], surpassing traditional creatinine (AUC = 0.660, 95%CI: 0.610–0.708) (38). Additionally, CysC level were associated with the mortality prognosis of ICH patients (48). Hence, CysC may serve as a more comprehensive biomarker.
β2-microglobulin
β2-microglobulin (β2-MG) is a peptide unaffected by gender or age that serves as a reflection of renal function. A prospective study involving 403 ICH patients revealed that β2-MG exhibited strong predictive efficacy for AKI, with an AUC of 0.712 (95%CI: 0.652–0.772). Additionally, ICH patients with β2-MG levels exceeding 2123.50 mg/L faced a significantly elevated risk of developing AKI (OR = 2.606; 95%CI: 1.315–5.166), which was also associated with in-hospital and 1-year mortality (49). These findings suggest the potential for β2-MG to emerge as a novel predictive indicator.
Procalcitonin
Procalcitonin (PCT) is a widely used indicator in clinical practice to assess infection and inflammation. However, a recent study suggested that PCT could also serve as a risk factor for AKI in non-sepsis patients (OR = 4.430, 95%CI: 1.464–13.399) (50). In studies related to ICH, a PCT value exceeding 0.5 μg/L upon admission was identified as a significant predictor for dialysis in ICH patients (OR = 7.7, 95%CI: 1.4–43.3, p = 0.02) (51), and is independently associated with poor prognosis and 3-month mortality (52). This could be attributed to the activation of immune cells by pro-inflammatory products generated during ICH and kidney injury, leading to an exacerbation of the inflammatory response and subsequent elevation of PCT levels in the patient’s blood (53). Given the widespread use of PCT in clinical practice, understanding its predictive role for AKI in ICH patients is crucial for optimizing medical resource allocation.
Prediction model
Presently, research on predictive models for AKI in ICH patients is highly significant. Tian et al. utilized the least absolute shrinkage and selection operator (LASSO) in conjunction with multivariate logistic regression methods to construct a model comprising 9 clinical and laboratory examination features. Through internal and three external validations, they demonstrated the model’s strong predictive performance, with AUCs of 0.816, 0.776, 0.780, and 0.821, respectively (24). Furthermore, Lin et al.’s acute stroke model exhibited notable predictive efficacy, reaching up to 0.839 (26). In addition, She et al. used six machine learning algorithms—extreme gradient boosting, logistic, light gradient boosting machine, random forest, adaptive boosting, and support vector machine—to develop the model. They found that the random forest algorithm performed the best in both the training and validation sets, achieving an AUC of 1.000 in the training set and 0.698 in the validation set (35). However, these models are constrained by relatively small sample sizes, limiting their credibility. Understanding the timing of AKI occurrence in patients is crucial for facilitating early clinical intervention. Furthermore, the practicality of the model for clinical application should be considered, including the development of simple scoring tables or prediction web platforms. Therefore, further research is imperative to refine and enhance these models.
Conclusion
Patients with ICH are critically ill, and AKI represents a significant clinical complication for them. Those who develop AKI often face a poor long-term prognosis. Currently, there is no specific treatment for AKI following ICH. Therefore, understanding the mechanisms, risk factors, and early diagnosis of this type of AKI is crucial. Further basic and clinical research is needed to improve our understanding of the disease. In the future, we aim to develop better models to identify high-risk patients and create new interventions to improve patient prognosis.
Author contributions
YC: Conceptualization, Formal analysis, Investigation, Methodology, Supervision, Validation, Writing – original draft, Writing – review & editing. GZ: Conceptualization, Funding acquisition, Methodology, Writing – review & editing. XX: Conceptualization, Formal analysis, Funding acquisition, Methodology, Supervision, Writing – review & editing.
Funding
The author(s) declare that no financial support was received for the research, authorship, and/or publication of this article.
Acknowledgments
We extend our heartfelt gratitude to the dedicated doctors and nurses tirelessly serving in the emergency department.
Conflict of interest
The authors declare that the research was conducted in the absence of any commercial or financial relationships that could be construed as a potential conflict of interest.
Publisher’s note
All claims expressed in this article are solely those of the authors and do not necessarily represent those of their affiliated organizations, or those of the publisher, the editors and the reviewers. Any product that may be evaluated in this article, or claim that may be made by its manufacturer, is not guaranteed or endorsed by the publisher.
Abbreviations
ICH, Intracerebral hemorrhage; AKI, Acute kidney injury; GFR, Glomerular filtration rate; CINC-1, Cytokine-induced neutrophil chemoattractant-1; MDA, Malondialdehyde; CTA, Computed tomography angiography; OR, Odds ratio; CI, Confidence interval; CKD, Chronic kidney disease; CysC, Cystatin C; β2-MG, β2-microglobulin; PCT, Procalcitonin; LASSO, Least absolute shrinkage and selection operator; AUC, Area under curve.
References
1. Sahni, R, and Weinberger, J. Management of intracerebral hemorrhage. Vasc Health Risk Manag. (2007) 3:701–9.
2. Rincon, F, and Mayer, SA. Clinical review: critical care management of spontaneous intracerebral hemorrhage. Crit Care. (2008) 12:237. doi: 10.1186/cc7092
3. Caceres, JA, and Goldstein, JN. Intracranial hemorrhage. Emerg Med Clin North Am. (2012) 30:771–94. doi: 10.1016/j.emc.2012.06.003
4. Qureshi, AI, Huang, W, Lobanova, I, Hanley, DF, Hsu, CY, Malhotra, K, et al. Systolic blood pressure reduction and acute kidney injury in intracerebral hemorrhage. Stroke. (2020) 51:3030–8. doi: 10.1161/STROKEAHA.120.030272
5. Riha, HM, Erdman, MJ, Vandigo, JE, Kimmons, LA, Goyal, N, Davidson, KE, et al. Impact of moderate Hyperchloremia on clinical outcomes in intracerebral hemorrhage patients treated with continuous infusion hypertonic saline: a pilot study. Crit Care Med. (2017) 45:e947–53. doi: 10.1097/CCM.0000000000002522
6. Wang, Q, Li, S, Sun, M, Ma, J, Sun, J, and Fan, M. Systemic immune-inflammation index may predict the acute kidney injury and prognosis in patients with spontaneous cerebral hemorrhage undergoing craniotomy: a single-center retrospective study. BMC Nephrol. (2023) 24:73. doi: 10.1186/s12882-023-03124-2
7. Nadkarni, GN, Patel, AA, Konstantinidis, I, Mahajan, A, Agarwal, SK, Kamat, S, et al. Dialysis requiring acute kidney injury in acute cerebrovascular accident hospitalizations. Stroke. (2015) 46:3226–31. doi: 10.1161/STROKEAHA.115.010985
8. Ansaritoroghi, M, Nagaraju, SP, Nair, RP, Kumar, V, Kongwad, LI, Attur, RP, et al. Study on acute kidney injury in patients with spontaneous intracerebral hemorrhage: an overview from a tertiary Care Hospital in South India. World Neurosurg. (2019) 123:e740–6. doi: 10.1016/j.wneu.2018.12.018
9. Qureshi, AI, Palesch, YY, Martin, R, Novitzke, J, Cruz Flores, S, Ehtisham, A, et al. Systolic blood pressure reduction and risk of acute renal injury in patients with intracerebral hemorrhage. Am J Med. (2012) 125:718.e1–6. doi: 10.1016/j.amjmed.2011.09.031
10. Kerro, A, Woods, T, and Chang, JJ. Neurogenic stunned myocardium in subarachnoid hemorrhage. J Crit Care. (2017) 38:27–34. doi: 10.1016/j.jcrc.2016.10.010
11. Zoccali, C, Goldsmith, D, Agarwal, R, Blankestijn, PJ, Fliser, D, Wiecek, A, et al. The complexity of the cardio-renal link: taxonomy, syndromes, and diseases. Kidney Int Suppl. (2011) 1:2–5. doi: 10.1038/kisup.2011.4
12. Crago, EA, Kerr, ME, Kong, Y, Baldisseri, M, Horowitz, M, Yonas, H, et al. The impact of cardiac complications on outcome in the SAH population. Acta Neurol Scand. (2004) 110:248–53. doi: 10.1111/j.1600-0404.2004.00311.x
13. McCoy, IE, Montez-Rath, ME, Chertow, GM, and Chang, TI. Central venous pressure and the risk of diuretic-associated acute kidney injury in patients after cardiac surgery. Am Heart J. (2020) 221:67–73. doi: 10.1016/j.ahj.2019.12.013
14. Zhao, Y, Zeng, H, Liu, B, He, X, and Chen, JX. Endothelial prolyl hydroxylase 2 is necessary for angiotensin II-mediated renal fibrosis and injury. Am J Physiol Renal Physiol. (2020) 319:F345–f357. doi: 10.1152/ajprenal.00032.2020
15. Tsutsui, H, Shimokawa, T, Miura, T, Takama, M, Nishinaka, T, Terada, T, et al. Effect of monoamine oxidase inhibitors on ischaemia/reperfusion-induced acute kidney injury in rats. Eur J Pharmacol. (2018) 818:38–42. doi: 10.1016/j.ejphar.2017.10.021
16. Messerer, DAC, Halbgebauer, R, Nilsson, B, Pavenstädt, H, Radermacher, P, and Huber-Lang, M. Immunopathophysiology of trauma-related acute kidney injury. Nat Rev Nephrol. (2021) 17:91–111. doi: 10.1038/s41581-020-00344-9
17. Chang, CY, Pan, PH, Li, JR, Ou, YC, Liao, SL, Chen, WY, et al. Glycerol improves intracerebral hemorrhagic brain injury and associated kidney dysfunction in rats. Antioxidants (Basel, Switzerland). (2021) 10:623. doi: 10.3390/antiox10040623
18. Campbell, SJ, Hughes, PM, Iredale, JP, Wilcockson, DC, Waters, S, Docagne, F, et al. CINC-1 is an acute-phase protein induced by focal brain injury causing leukocyte mobilization and liver injury. FASEB J. (2003) 17:1168–70. doi: 10.1096/fj.02-0757fje
19. Tsikas, D, Tsikas, SA, Mikuteit, M, and Ückert, S. Circulating and urinary concentrations of malondialdehyde in aging humans in health and disease: review and discussion. Biomedicines. (2023) 11:2744. doi: 10.3390/biomedicines11102744
20. McDonald, JS, McDonald, RJ, Williamson, EE, Kallmes, DF, and Kashani, K. Post-contrast acute kidney injury in intensive care unit patients: a propensity score-adjusted study. Intensive Care Med. (2017) 43:774–84. doi: 10.1007/s00134-017-4699-y
21. Davenport, MS, Perazella, MA, Yee, J, Dillman, JR, Fine, D, McDonald, RJ, et al. Use of intravenous iodinated contrast Media in Patients with kidney disease: consensus statements from the American College of Radiology and the National Kidney Foundation. Radiology. (2020) 294:660–8. doi: 10.1148/radiol.2019192094
22. Shah, N, Koch, S, Hassouneh, Z, Bustillo, A, Simonetto, M, O'Phelan, K, et al. Effect of race-ethnicity and CT angiography on renal injury during blood pressure treatment for intracerebral hemorrhage. Neurocrit Care. (2021) 35:687–92. doi: 10.1007/s12028-021-01206-3
23. Sorimachi, T, Atsumi, H, Yonemochi, T, Hirayama, A, Shigematsu, H, Srivatanakul, K, et al. Benefits and risks of CT angiography immediately after emergency arrival for patients with intracerebral hematoma. Neurol Med Chir. (2020) 60:45–52. doi: 10.2176/nmc.oa.2019-0152
24. Tian, Y, Zhang, Y, He, J, Chen, L, Hao, P, Li, T, et al. Predictive model of acute kidney injury after spontaneous intracerebral hemorrhage: a multicenter retrospective study. Eur Stroke J. (2023) 8:747–55. doi: 10.1177/23969873231184667
25. Better, OS, Rubinstein, I, Winaver, JM, and Knochel, JP. Mannitol therapy revisited (1940-1997). Kidney Int. (1997) 52:886–94. doi: 10.1038/ki.1997.409
26. Lin, SY, Tang, SC, Tsai, LK, Yeh, SJ, Shen, LJ, Wu, FL, et al. Incidence and risk factors for acute kidney injury following mannitol infusion in patients with acute stroke: a retrospective cohort study. Medicine. (2015) 94:e2032. doi: 10.1097/MD.0000000000002032
27. Husain-Syed, F, Takeuchi, T, Neyra, JA, Ramírez-Guerrero, G, Rosner, MH, Ronco, C, et al. Acute kidney injury in neurocritical care. Crit Care. (2023) 27:341. doi: 10.1186/s13054-023-04632-1
28. Gerber, JG, Branch, RA, Nies, AS, Hollifield, JW, and Gerkens, JF. Influence of hypertonic saline on canine renal blood flow and renin release. Am J Phys. (1979) 237:F441–6. doi: 10.1152/ajprenal.1979.237.6.F441
29. Huang, PP, Stucky, FS, Dimick, AR, Treat, RC, Bessey, PQ, and Rue, LW. Hypertonic sodium resuscitation is associated with renal failure and death. Ann Surg. (1995) 221:543–57. discussion: 554–547. doi: 10.1097/00000658-199505000-00012
30. Yunos, NM, Bellomo, R, Hegarty, C, Story, D, Ho, L, and Bailey, M. Association between a chloride-liberal vs chloride-restrictive intravenous fluid administration strategy and kidney injury in critically ill adults. JAMA. (2012) 308:1566–72. doi: 10.1001/jama.2012.13356
31. Hogg, RC, Wang, Q, and Large, WA. Action of niflumic acid on evoked and spontaneous calcium-activated chloride and potassium currents in smooth muscle cells from rabbit portal vein. Br J Pharmacol. (1994) 112:977–84. doi: 10.1111/j.1476-5381.1994.tb13177.x
32. Bullivant, EM, Wilcox, CS, and Welch, WJ. Intrarenal vasoconstriction during hyperchloremia: role of thromboxane. Am J Phys. (1989) 256:F152–7. doi: 10.1152/ajprenal.1989.256.1.F152
33. Rass, V, Ianosi, BA, Wegmann, A, Gaasch, M, Schiefecker, AJ, Kofler, M, et al. Delayed resolution of cerebral edema is associated with poor outcome after nontraumatic subarachnoid hemorrhage. Stroke. (2019) 50:828–36. doi: 10.1161/STROKEAHA.118.024283
34. Wagner, I, Hauer, EM, Staykov, D, Volbers, B, Dörfler, A, Schwab, S, et al. Effects of continuous hypertonic saline infusion on perihemorrhagic edema evolution. Stroke. (2011) 42:1540–5. doi: 10.1161/STROKEAHA.110.609479
35. She, S, Shen, Y, Luo, K, Zhang, X, and Luo, C. Prediction of acute kidney injury in intracerebral hemorrhage patients using machine learning. Neuropsychiatr Dis Treat. (2023) 19:2765–73. doi: 10.2147/NDT.S439549
36. Zhang, C, Xia, J, Ge, H, Zhong, J, Chen, W, Lan, C, et al. Long-term mortality related to acute kidney injury following intracerebral hemorrhage: a 10-year (2010-2019) retrospective study. J Stroke Cerebrovasc Dis. (2021) 30:105688. doi: 10.1016/j.jstrokecerebrovasdis.2021.105688
37. Kim, MY, Park, JH, Kang, NR, Jang, HR, Lee, JE, Huh, W, et al. Increased risk of acute kidney injury associated with higher infusion rate of mannitol in patients with intracranial hemorrhage. J Neurosurg. (2014) 120:1340–8. doi: 10.3171/2013.12.JNS13888
38. Jiang, F, Su, L, Xiang, H, Zhang, X, Xu, D, Zhang, Z, et al. Incidence, risk factors, and biomarkers predicting ischemic or hemorrhagic stroke associated acute kidney injury and outcome: a retrospective study in a general intensive care unit. Blood Purif. (2019) 47:317–26. doi: 10.1159/000499029
39. Hewgley, H, Turner, SC, Vandigo, JE, Marler, J, Snyder, H, Chang, JJ, et al. Impact of admission hypertension on rates of acute kidney injury in intracerebral hemorrhage treated with intensive blood pressure control. Neurocrit Care. (2018) 28:344–52. doi: 10.1007/s12028-017-0488-2
40. Tanaka, K, Koga, M, Fukuda-Doi, M, Qureshi, AI, Yamamoto, H, Miwa, K, et al. Temporal trajectory of systolic blood pressure and outcomes in acute intracerebral hemorrhage: ATACH-2 trial cohort. Stroke. (2022) 53:1854–62. doi: 10.1161/STROKEAHA.121.037186
41. Harvey, A, Montezano, AC, and Touyz, RM. Vascular biology of ageing-implications in hypertension. J Mol Cell Cardiol. (2015) 83:112–21. doi: 10.1016/j.yjmcc.2015.04.011
42. Varon, J, and Marik, PE. Clinical review: the management of hypertensive crises. Crit Care. (2003) 7:374–84. doi: 10.1186/cc2351
43. Burgess, LG, Goyal, N, Jones, GM, Khorchid, Y, Kerro, A, Chapple, K, et al. Evaluation of acute kidney injury and mortality after intensive blood pressure control in patients with intracerebral hemorrhage. J Am Heart Assoc. (2018) 7:e008439. doi: 10.1161/JAHA.117.008439
44. Anderson, CS, Heeley, E, Huang, Y, Wang, J, Stapf, C, Delcourt, C, et al. Rapid blood-pressure lowering in patients with acute intracerebral hemorrhage. N Engl J Med. (2013) 368:2355–65. doi: 10.1056/NEJMoa1214609
45. Kelly, D, and Rothwell, PM. Disentangling the multiple links between renal dysfunction and cerebrovascular disease. J Neurol Neurosurg Psychiatry. (2020) 91:88–97. doi: 10.1136/jnnp-2019-320526
46. Meier, K, Saenz, DM, Torres, GL, Cai, C, Rahbar, MH, McDonald, M, et al. Thrombelastography suggests hypercoagulability in patients with renal dysfunction and intracerebral hemorrhage. J Stroke Cerebrovasc Dis. (2018) 27:1350–6. doi: 10.1016/j.jstrokecerebrovasdis.2017.12.026
47. Onopiuk, A, Tokarzewicz, A, and Gorodkiewicz, E. Cystatin C: a kidney function biomarker. Adv Clin Chem. (2015) 68:57–69. doi: 10.1016/bs.acc.2014.11.007
48. You, S, Shi, L, Zhong, C, Xu, J, Han, Q, Zhang, X, et al. Prognostic significance of estimated glomerular filtration rate and cystatin C in patients with acute intracerebral hemorrhage. Cerebrovasc Dis (Basel, Switzerland). (2016) 42:455–63. doi: 10.1159/000448340
49. Wang, R, Hu, H, Hu, S, He, H, and Shui, H. β2-microglobulin is an independent indicator of acute kidney injury and outcomes in patients with intracerebral hemorrhage. Medicine. (2020) 99:e19212. doi: 10.1097/MD.0000000000019212
50. Jeeha, R, Skinner, DL, De Vasconcellos, K, and Magula, NP. Serum procalcitonin levels predict acute kidney injury in critically ill patients. Nephrology (Carlton). (2018) 23:1090–5. doi: 10.1111/nep.13174
51. Schenk, LM, Schneider, M, Bode, C, Güresir, E, Junghanns, C, Müller, M, et al. Early laboratory predictors for necessity of renal replacement therapy in patients with spontaneous deep-seated intracerebral hemorrhage. Front Neurol. (2021) 12:636711. doi: 10.3389/fneur.2021.636711
52. He, D, Zhang, Y, Zhang, B, Jian, W, Deng, X, Yang, Y, et al. Serum Procalcitonin levels are associated with clinical outcome in intracerebral hemorrhage. Cell Mol Neurobiol. (2018) 38:727–33. doi: 10.1007/s10571-017-0538-5
Keywords: intracerebral hemorrhage, acute kidney injury, pathogenesis, risk factors, diagnostic approaches
Citation: Chen Y, Zhao G and Xia X (2024) Acute kidney injury after intracerebral hemorrhage: a mini review. Front. Med. 11:1422081. doi: 10.3389/fmed.2024.1422081
Edited by:
Jing (Jason) Wu, University of Rochester Medical Center, United StatesReviewed by:
Jiaojiao Xu, Johns Hopkins University, United StatesAshley Pitzer Mutchler, Vanderbilt University Medical Center, United States
Copyright © 2024 Chen, Zhao and Xia. This is an open-access article distributed under the terms of the Creative Commons Attribution License (CC BY). The use, distribution or reproduction in other forums is permitted, provided the original author(s) and the copyright owner(s) are credited and that the original publication in this journal is cited, in accordance with accepted academic practice. No use, distribution or reproduction is permitted which does not comply with these terms.
*Correspondence: Yuyang Chen, Y2hlbnl1eWFuZ25qbXVAMTYzLmNvbQ==; Xiaohua Xia, eHh5ODBfMjAwMUAxNjMuY29t