- Department of Anesthesiology, Affiliated Hospital of North Sichuan Medical College, Nanchong, China
Study objective: Recent studies have shown that dexmedetomidine can be safely used in peripheral nerve blocks and spinal anesthesia. Epidural administration of dexmedetomidine produces analgesia and sedation, prolongs motor and sensory block time, extends postoperative analgesia, and reduces the need for rescue analgesia. This investigation seeks to identify the median effective concentration (EC50) of ropivacaine for epidural motor blockade, and assess how incorporating varying doses of dexmedetomidine impacts this EC50 value.
Design: Prospective, double-blind, up-down sequential allocation study.
Setting: Operating room, post-anesthesia care unit, and general ward.
Interventions: One hundred and fifty patients were allocated into five groups in a randomized, double-blinded manner as follows: NR (normal saline combined with ropivacaine) group, RD0.25 (0.25 μg/kg dexmedetomidine combined with ropivacaine) group, RD0.5 (0.5 μg/kg dexmedetomidine combined with ropivacaine) group, RD0.75 (0.75 μg/kg dexmedetomidine combined with ropivacaine) group, RD1.0 (1.0 μg/kg dexmedetomidine combined with ropivacaine) group. The concentration of epidural ropivacaine for the first patient in each group was 0.5%. Following administration, the patients were immediately placed in a supine position for observation, and the lower limb motor block was assessed every 5 min using the modified Bromage score within 30 min after drug administration. According to the sequential method, the concentration of ropivacaine in the next patient was adjusted according to the reaction of the previous patient: effective motor block was defined as the modified Bromage score > 0 within 30 min after epidural administration. If the modified Bromage score of the previous patient was >0 within 30 min after drug administration, the concentration of ropivacaine in the next patient was decreased by 1 gradient. Conversely, if the score did not exceed 0, the concentration of ropivacaine in the next patient was increased by 1 gradient. The up-down sequential allocation method and probit regression were used to calculate the EC50 of epidural ropivacaine.
Measurements: Adverse events, hemodynamic changes, demographic data and clinical characteristics.
Main results: The EC50 of epidural ropivacaine required to achieve motor block was 0.677% (95% CI, 0.622–0.743%) in the NR group, 0.624% (95% CI, 0.550–0.728%) in the RD0.25 group, 0.549% (95% CI, 0.456–0.660%) in the RD0.5 group, 0.463% (95% CI, 0.408–0.527%) in the RD0.75 group, and 0.435% (95% CI, 0.390–0.447%) in the RD1.0 group. The EC50 of the NR group and the RD0.25 group were significantly higher than that of the RD0.75 and the RD1.0 groups, and the EC50 of the RD0.5 group was significantly higher than that of the RD1.0 group.
Conclusion: The EC50 of epidural ropivacaine required to achieve motor block was 0.677% in the NR group, 0.624% in the RD0.25 group, 0.549% in the RD0.5 group, 0.463% in the RD0.75 group, and 0.435% in the RD1.0 group. Dexmedetomidine as an adjuvant for ropivacaine dose-dependently reduce the EC50 of epidural ropivacaine for motor block and shorten the onset time of epidural ropivacaine block. The optimal dose of dexmedetomidine combined with ropivacaine for epidural anesthesia was 0.5 μg/kg.
1 Background
Epidural anesthesia is extensively applied across various surgical domains, including urology, lower abdominal and lower limb orthopedic procedures (1, 2). Epidural anesthesia is administered to provide intraoperative surgical anesthesia and postoperative analgesia (3). It not only diminishes the incidence of complications by mitigating the perioperative stress response but also facilitates early mobilization through alleviation of postoperative pain, thereby preventing the development of lower limb venous thrombosis (4–7). However, epidural anesthesia frequently results in inadequate or superficial blockade (8). In order to overcome these weaknesses, we usually enhance the effect of epidural anesthesia by adding opioids (9, 10). Opioids like fentanyl and sufentanil, as adjuvants for epidural administration, provide good analgesic effects (11), but can cause adverse effects such as itching, nausea, vomiting, and respiratory depression (12, 13).
Dexmedetomidine is a highly selective α2 adrenergic receptor agonist (14). Clinical studies have shown that dexmedetomidine can be safely used for peripheral nerve blockade and spinal anesthesia (15–19). Recent investigations have demonstrated that the administration of dexmedetomidine via epidural route not only induces analgesia and sedation (20) but also intensifies the efficacy of motor and sensory blockades (21, 22). Nonetheless, as the dosage of epidural dexmedetomidine escalates, there is a corresponding increase in the occurrence of adverse effects (23). Consequently, investigating the optimal dosage of dexmedetomidine in combination with ropivacaine for epidural administration is imperative.
This study is designed to identify the EC50 of ropivacaine for epidural motor blockade, as well as to assess the impact of various doses of dexmedetomidine on this EC50 value. Concurrently, it aims to offer guidance on the optimal dosage of dexmedetomidine when used as an adjunct to ropivacaine in epidural anesthesia.
2 Methods
2.1 Study design
We conducted a prospective, double-blind, up-down sequential allocation study to determine the EC50 of ropivacaine for motor blockade in epidural anesthesia when combined with different does of dexmedetomidine. The study received approval from the Ethics Committee of the Affiliated Hospital of North Sichuan Medical College (2022ER083-1) and was registered at the Chinese Clinical Trial Registry (registration number: ChiCTR2200065955, registration date: November 19, 2022). All participants in the clinical trial provided written informed consent and were treated at the Affiliated Hospital of North Sichuan Medical College. The enrollment of the first patient took place on November 25, 2022.
Study subjects who met the following inclusion criteria were considered: all patients scheduled for elective urological surgery, aged from 18 to 65 years, with body mass index (BMI) more than or equal to 18.5 kg/m2 and less than 30 kg/m2, American Society of Anesthesiologists (ASA) physical status grade I or II, were enrolled from November 2022 to March 2023. Exclusion criteria included patients with a history of drug addiction, those whose patients or their families refusing to participate, those with anticipated difficulty in regional anesthesia, allergies to the study drug, and those with bradycardia. Also excluded were individuals with severe cardiovascular or pulmonary diseases, liver or kidney dysfunction, spinal deformity, and coagulation disorders. Further exclusions were patients with infection at the site of epidural puncture, cognitive dysfunction, hearing impairment, lower limb motor disorders, or sensory abnormalities.
All patients were divided into five groups using computer-generated random numbers. These random numbers were marked on cards and placed in sealed envelopes within an opaque box. Upon the patient’s arrival in the operating room, the anesthesia nurse randomly drew an envelope and administered the test drug according to the group in the envelope, which used sealed envelopes indicating the allocation: normal saline combined with ropivacaine (Ropivacaine hydrochloride injection, 10 mL:100 mg, AstraZeneca AB, Sweden) (NR) group, 0.25 μg/kg dexmedetomidine combined with ropivacaine (RD0.25) group, 0.50 μg/kg dexmedetomidine combined with ropivacaine (RD0.5) group, 0.75 μg/kg dexmedetomidine combined with ropivacaine (RD0.75) group, 1.0 μg/kg dexmedetomidine combined with ropivacaine (RD1.0) group. The anesthesia nurse completed the drug preparation and gave it to the anesthesiologist in this study. After the experiment, the anesthesiologist showed the data back to the statistician. Study participants and the investigators who performed outcome assessments were blinded to the grouping during the study period.
2.2 Anesthesia
Prior to surgery, all patients underwent an 8-h fasting period for solid food and a 2-h fasting period for clear liquids. Additionally, they did not receive any preoperative medication. Upon arrival in the operating room, a secure intravenous access was established. Simultaneously, patients were preloaded with 10 mL/kg of lactated Ringer’s solution and continuously monitored with electrocardiogram (ECG), oxygen saturation, and noninvasive blood pressure (NIBP). A face mask was used to administer a supplemental oxygen flow rate of 6 L/min to all patients. The study drug was prepared by an anesthesia nurse and then handed to another anesthesiologist who was unaware of its composition. Epidural anesthesia was performed with patients positioned in the left lateral decubitus position in the L2-L3 intervertebral space. The epidural space was confirmed using the loss of resistance technique. An epidural catheter was inserted 4–5 cm into the epidural space and securely fixed. To rule out the possibility of intrathecal injection, 3 mL of 2% lidocaine was administered as a test dose after negative aspiration for blood and cerebrospinal fluid, no adrenaline was added to the test dose. The NR group received a 15 mL mixture of normal saline and ropivacaine, whereas the RD0.25, RD0.5, RD0.75 and RD1.0 groups were administered a 15 mL combination of dexmedetomidine and ropivacaine, the dosages of dexmedetomidine for these groups were 0.25 μg/kg, 0.5 μg/kg, 0.75 μg/kg, and 1.0 μg/kg, respectively. The mixed solution was then continuously infused into epidural space at a rate of 1 mL/s in a single administration. The concentration of epidural ropivacaine for the first patient in each group was 0.5% (24). Following administration, the patients were immediately placed in a supine position for observation, and the lower limb motor block was assessed every 5 min using the modified Bromage score (Bromage 0 = fully able to flex knees and feet; Bromage 1 = just able to move knees; Bromage 2 = unable to move knees, able to move feet only; Bromage 3 = unable to move knees and feet) (25) within 30 min after drug administration. According to the sequential method (26), the concentration of ropivacaine in the next patient was adjusted according to the reaction of the previous patient: effective motor block was defined as the modified Bromage score > 0 within 30 min after epidural administration. If the modified Bromage score of the previous patient was >0 within 30 min after drug administration, the concentration of ropivacaine in the next patient was decreased by 1 gradient (division of the current patient’s concentration by 1.2); if the modified Bromage score of the previous patient was = 0 within 30 min after drug administration, the concentration of ropivacaine in the next patient was increased by 1 gradient (multiplication of the current patient’s concentration by 1.2). Those patients who reported ineffective analgesia at 30 min after administration of the study solution received a 5 mL bolus of 2% lidocaine administered via epidural catheter to supplement the analgesia. If the patient still reported ineffective analgesia, the patient’s data were excluded from the study analysis, and general anesthesia was used instead (21). Hypotension, defined as systolic blood pressure (SBP) < 20% of baseline value or < 90 mmHg (27), was treated with an intravenous injection of ephedrine 6 mg to restore blood pressure. Bradycardia, defined as a heart rate of less than 50 beats per minute, was treated with intravenous atropine 0.5 mg. When nausea and vomiting occurred, ondansetron 4 mg was injected intravenously.
2.3 Measurements
The primary outcome of this study was to ascertain the effective concentration of ropivacaine, when combined with varying doses of dexmedetomidine, for achieving motor blockade.
2.3.1 Secondary outcome
General patient information was recorded. Sedation was assessed using the Ramsay sedation scale (Grade 1 = patient anxious, or agitated, or both; Grade 2 = patient cooperative, oriented, and tranquil; Grade 3 = patient responds to commands only; Grade 4 = a brisk response to a light glabellar tap; Grade 5 = a sluggish response to light glabellar tap; Grade 6 = no response) (23) at the time of the patients arriving at the operating room (T0), 5 min (T1), 15 min (T2), 30 min (T3), 1 h (T4), and 2 h (T5) after epidural administration. Systolic blood pressure (SBP), diastolic blood pressure (DBP), and heart rate (HR) were also measured and recorded at T0, T1, T2, T3, and T4. The level of sensory block was assessed bilaterally along the midclavicular line using the pinprick test. The onset time of sensory block was defined as the time between epidural injection and a T10 sensory block level being achieved, and the highest level of sensory block was also recorded. The adverse effects and complications during and 24 h after surgery, including hypotension, bradycardia, dry mouth, dizziness, nausea, and vomiting, were observed. Other indicators were recorded, such as surgery time, intraoperative fluid infusion volume and blood loss, intraoperative and postoperative use of vasoactive drugs.
2.4 Statistical analysis
According to published research (28), the up-down allocation method requires 20–40 study subjects to estimate the EC50. It is considered sufficient to obtain six pairs of reversals of sequence for a valid sample size. Based on the pre-experimental results, we calculated the sample size. When compared with the NR group, the EC50 values of the RD0.25 group showed an 8% decrease, the EC50 of the RD0.5 group a 15% decrease, the EC50 of the RD0.75 group a 33% decrease, and the EC50 of the RD1.0 group a 36% decrease. In the equation below, n symbolizes the total sample size, while Pmin and Pmax indicate the minimum and maximum rates, respectively. Therefore, a total sample size of 118 achieves 90% power to detect an effect size (W) of 0.3615 using a 4 degrees of freedom Chi-Square Test with a significance level (alpha) of 0.05. To account for 20% dropout rate, we thus planned to enroll 150 patients in our study. Therefore, we determined that a sample size of 30 patients for each group would be necessary to observe more than six pairs of reversals of sequence. As a backup and sensitivity analysis, probit regression analysis was conducted by analyzing the tallied numbers of “effective” and “ineffective” responses for each concentration category in each group.
Statistical analysis utilized SPSS 26.0 statistical software. Continuous variables adhering to a normal distribution were presented as mean ± standard deviation ( ± s). For comparison among groups, one-way analysis of variation (ANOVA) was utilized, complemented by Bonferroni correction for post hoc pairwise comparison. In cases where continuous variables were measured at multiple time points within each group, two-way repeated measures ANOVA was employed, accompanied by simple effects analysis to examine any interaction effects between group and time. Variables exhibiting a skewed distribution were characterized by the median (M) and interquartile range (IQR), with the Kruskal-Wallis H test being applied for group comparisons and Bonferroni correction for ensuing pairwise comparisons. Categorical data were reported as percentiles and subjected to analysis by Pearson’s X2 test or Fisher’s exact test. For comparing ordinal variables across groups, the Kruskal-Wallis H test was employed, and the Friedman test was used for within-group comparisons, with Bonferroni correction facilitated for subsequent post hoc pairwise analyses. A p-value <0.05 was deemed statistically significant.
3 Results
We recruited 168 patients for our study, 13 of them did not meet inclusion criteria, 3 of them refused participation, and 2 of them underwent a failed epidural anesthesia. Later, 150 subjects were enrolled in this study (Figure 1).
3.1 Demographic data and clinical characteristics
There were no significant differences in age, gender, ASA grade, weight, height, duration of surgery, fluid infusion volume, blood loss, and maximum sensory block among the five groups (p > 0.05). Although the overall difference in the number of people using vasoactive drugs was statistically significant (p < 0.05), there was no statistically significant difference in pairwise comparison among the five groups. The onset time of sensory block in the RD0.25, RD0.5, RD0.75, and RD1.0 groups was significantly shorter (p < 0.05), as shown in Table 1.
3.2 Median effective concentration
As shown in Figure 2, the EC50 of epidural ropivacaine required to achieve motor block was 0.677% (95% CI, 0.622–0.743%) in the NR group, 0.624% (95% CI, 0.550–0.728%) in the RD0.25 group, 0.549% (95% CI, 0.456–0.660%) in the RD0.5 group, 0.463% (95% CI, 0.408–0.527%) in the RD0.75 group, and 0.435% (95% CI, 0.390–0.447%) in the RD1.0 group. The EC50 of the NR group and the RD0.25 group were significantly higher than that of the RD0.75 and the RD1.0 groups (p < 0.05), and the EC50 of the RD0.5 group was significantly higher than that of the RD1.0 group (p < 0.05).
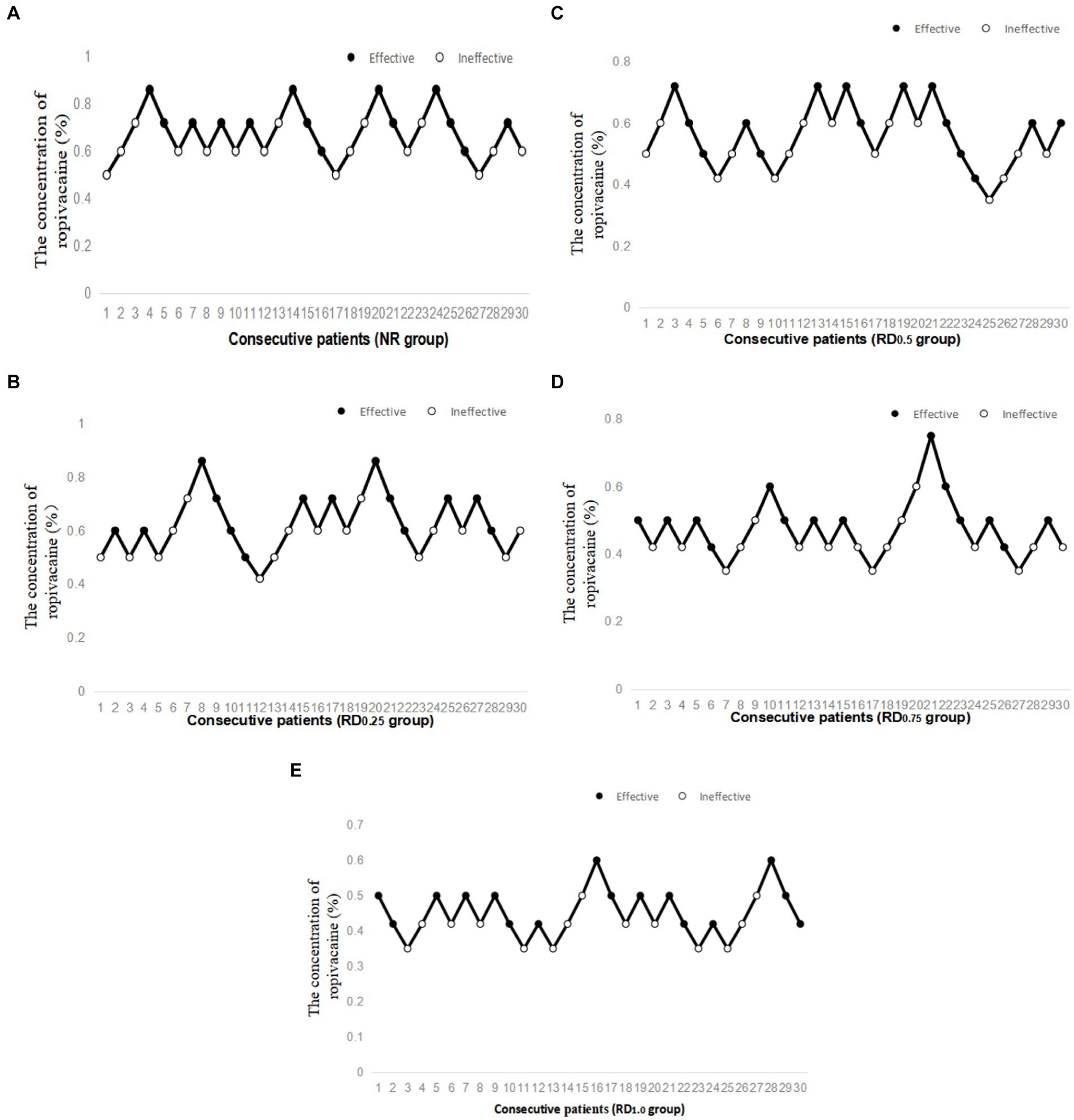
Figure 2. Individual response to epidural ropivacaine at corresponding concentrations (%). NR: normal saline; RD0.25: ropivacaine combined with 0.25 μg/kg dexmedetomidine; RD0.5: ropivacaine combined with 0.50 μg/kg dexmedetomidine; RD0.75: ropivacaine combined with 0.75 μg/kg dexmedetomidine; RD1.0: ropivacaine combined with 1.0 μg/kg dexmedetomidine. (A) Individual response to epidural ropivacaine at corresponding concentrations (%) in the NR group, (B) Individual response to epidural ropivacaine at corresponding concentrations (%) in the RD0.25 group, (C) Individual response to epidural ropivacaine at corresponding concentrations (%) in the RD0.5 group, (D) Individual response to epidural ropivacaine at corresponding concentrations (%) in the RD0.75 group and (E) Individual response to epidural ropivacaine at corresponding concentrations (%) in the RD1.0 group.
3.3 Hemodynamics
In the five groups, SBP, DBP, and HR significantly decreased at T2-4 compared to T0. Additionally, these parameters saw a marked reduction at T3-4 compared with T2, but exhibited a significant increase at T0-2 when contrasted with T3 (p < 0.05). However, in the RD0.5, RD0.75, and RD1.0 groups, the SBP at T4 was notably lower than at T3. Furthermore, the HR at T3 and T4 in the RD0.75 and RD1.0 groups was significantly lower compared to the NR, RD0.25, RD0.5 groups (p < 0.05), as depicted in Figure 3.
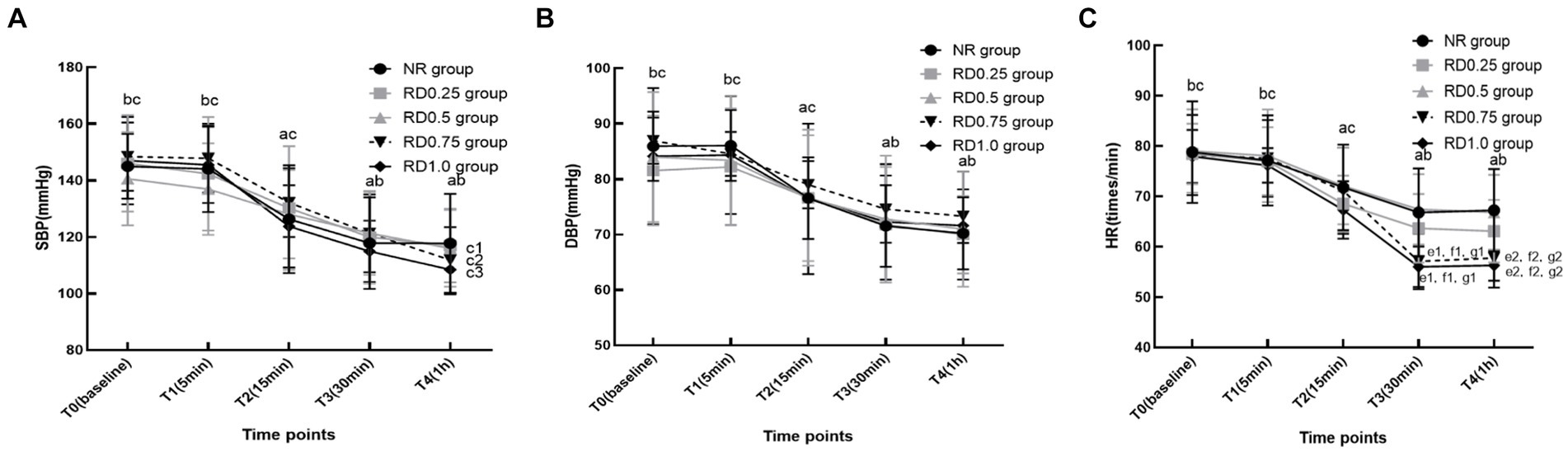
Figure 3. The hemodynamic changes in five groups at different time points. SBP: systolic blood pressure; DBP: diastolic blood pressure; HR: heart rate; NR: normal saline; RD0.25: ropivacaine combined with 0.25 μg/kg dexmedetomidine; RD0.5: ropivacaine combined with 0.50 μg/kg dexmedetomidine; RD0.75: ropivacaine combined with 0.75 μg/kg dexmedetomidine; RD1.0: ropivacaine combined with 1.0 μg/kg dexmedetomidine. aP < 0.05 vs. the five groups at T0, bP < 0.05 vs. the five groups at T2, cP < 0.05 vs. the five groups at T3, c1P < 0.05 vs. the RD0.5 group at T3, c2P < 0.05 vs. the RD0.75 group at T3, c3P < 0.05 vs. the RD1.0 group at T3, d1P < 0.05 vs. the NR group at T3, d2P < 0.05 vs. the NR group at T4, e1P < 0.05 vs. the RD0.25 group at T3, e2P < 0.05 vs. the RD0.25 group at T4, f1P < 0.05 vs. the RD0.5 group at T3, f2P < 0.05 vs. the RD0.5 group at T4. (A) SBP, (B) DBP and (C) HR.
3.4 Ramsay sedation score
Compared with the NR group, Ramsay sedation score in the RD0.25 group was significantly higher at T3 (p < 0.05), Ramsay sedation score in the RD0.5 group was significantly higher at T3-5 (p < 0.05), and Ramsay sedation score in the RD0.75 and RD1.0 groups was significantly higher at T2-5 (p < 0.05). In the RD0.25 group, Ramsay Sedation score increased at T3-4 compared with T0, in the RD0.5 and RD0.75 groups, Ramsay score increased significantly at T3-5 compared with T0, and in the RD1.0 group, Ramsay score increased at T2-5 compared with T0 (p < 0.05), as shown in Table 2.
3.5 Side effects
The overall differences in hypotension, bradycardia, and thirst were statistically significant (p < 0.05), but there was no statistically significant difference in pairwise comparison among the five groups, as shown in Table 3.
4 Discussion
This study found that dexmedetomidine as an adjuvant dose-dependently reduced the EC50 of epidural ropivacaine for motor block and shortened the onset time of epidural anesthesia block. 0.75 μg/kg and 1.0 μg/kg, of dexmedetomidine combined with ropivacaine resulted in a significant reduction in heart rate. However, when patients were administered with 0.5 μg/kg of dexmedetomidine combined with ropivacaine, the heart rate remained largely consistent with that of the control group. These findings suggest that the optimal dose of dexmedetomidine combined with ropivacaine for epidural anesthesia is 0.5 μg/kg.
When used as an adjuvant, dexmedetomidine combined with ropivacaine not only enhances the local anesthetic effect of ropivacaine but also reduces the EC50 of ropivacaine for labor analgesia (17, 22). Kazim et al. (29) found that when dexmedetomidine was added to epidural ropivacaine, patients undergoing percutaneous renal lithotripsy experienced an earlier onset of motor and sensory blockade and longer postoperative analgesia. These findings are similar to the results of our study. The EC50 value of ropivacaine, when combined with either 0.75 μg/kg or 1.0 μg/kg of dexmedetomidine, was significantly reduced compared to ropivacaine used alone. Furthermore, the EC50 value of ropivacaine with 1.0 μg/kg dexmedetomidine was distinctly lower than when combined with 0.5 μg/kg dexmedetomidine. Our findings indicated that the epidural infusion of dexmedetomidine could reduce the EC50 of ropivacaine for motor blockade. Furthermore, as the dosage of epidural dexmedetomidine increases, the EC50 for ropivacaine-induced motor blockade gradually decreases. This effect is attributed to several mechanisms: Firstly, dexmedetomidine acts as a highly selective α2 adrenoceptor agonist. Its epidural infusion affects both presynaptic and postsynaptic α2 receptors on dorsal horn neurons, leading to neuronal cell membrane hyperpolarization, which in turn produces analgesic and motor block effects (30–33). Secondly, the epidural infusion of dexmedetomidine opens K+ channels on the nerve cell membrane and strengthens the local anesthetics’ inhibitory action on Na+ channels, thereby enhancing the anesthetic effects (34). Lastly, it reduces the secretion of norepinephrine in the spinal cord, stimulates cholinergic nerves, and generates an anti-nociceptive effect (35).
Kiran et al. (30) found that compared with 0.5% ropivacaine alone, the map and heart rate of 10 μg dexmedetomidine combined with 0.5% ropivacaine decreased significantly 10 min after epidural administration. Our findings align with these results, as we observed a significant decrease in blood pressure and heart rate among the five groups of patients 15 min after epidural administration. These results indicated that dexmedetomidine could inhibit hemodynamics to a certain extent. But we also discovered that, compared with ropivacaine combined with 0.25 μg/kg and 0.5 μg/kg dexmedetomidine, the heart rate significantly decreased at 30 min and 1 h after administration when ropivacaine was used in conjunction with 0.75 μg/kg and 1.0 μg/kg of dexmedetomidine. This finding suggests that as the dosage of dexmedetomidine increases, its inhibitory effect on the heart rate intensifies, indicating a dose-dependent relationship. This is mainly because epidural infusion of dexmedetomidine can act on the α2 receptor of the spinal cord’s presynaptic and postsynaptic nerve endings, which causes neuronal cell membrane hyperpolarization, inhibits neuronal firing and sympathetic outflow, thereby inhibiting norepinephrine release, causing a drop in blood pressure and a slowing heart rate (36, 37).
Epidural anesthesia is a kind of awake anesthesia. The sedative effect of dexmedetomidine can not only relieve patients’ tension and anxiety, but also provide patients with good comfort (38). Kaur et al. (21) found that compared with 0.75% ropivacaine alone, the sedation score of 0.75% ropivacaine combined with 1.0 μg/kg dexmedetomidine significantly increased 30 min after epidural administration. This is similar to the results of this study, compared with ropivacaine alone, the sedation score of ropivacaine combined with 0.25 μg/kg dexmedetomidine significantly increased 30 min after epidural administration, the sedation score of ropivacaine combined with 0.5 μg/kg dexmedetomidine significantly increased at 30 min, 1 h and 2 h after epidural administration, the sedation score of ropivacaine combined with 0.75 μg/kg and 1.0 μg/kg dexmedetomidine significantly increased at 15 min, 30 min, 1 h and 2 h after epidural administration. This indicates that epidural dexmedetomidine could produce sedation. Furthermore, our study findings also reveal that with an increasing dose of epidural dexmedetomidine, the time required to achieve the same level of sedation is shorter, and the duration of the sedative effect is longer. This may be due to the fact that dexmedetomidine with high fat solubility is easily absorbed into the blood through epidural adipose tissue and acts in the brain stem α2 receptor, which produces sedation (39).
Soni et al. (40) found that compared with 0.75% ropivacaine alone, the anesthesia onset time of 0.75% ropivacaine combined with 1.5 μg/kg dexmedetomidine was shorter. This study also discovered that the onset time of anesthesia was shorter when dexmedetomidine was combined with ropivacaine compared to ropivacaine alone. This finding suggests that epidural dexmedetomidine has the potential to expedite the onset time of anesthesia. In our study, we found that the rates of using vasoactive drugs in the five groups were 3.3, 6.7, 6.7, 30, 23.3%, the incidences of hypotension were 6.7, 13.3, 10, 33.3, 30%, the incidences of bradycardia were 13.3, 10, 13.3, 36.7, 40%, and the incidences of thirst were 0, 0, 6.7, 10, 16.7%, respectively. The overall difference in the five groups was statistically significant (p < 0.05), but there was no statistically significant difference in pairwise comparison among the five groups. This may be due to our small sample size, and more randomized controlled trials are needed for further research in the future.
There are several limitations in our study. First, we can calculate the EC50 value by the Dixon sequential method, but the sequential method is only suitable for the calculation of half-effective measurement in studies with a small number of cases. The EC95 calculation, which is widely used in clinics, requires a large number of samples. Although we can calculate the EC95 through the EC50, the sequential method is the main method in this study. Therefore, it is necessary for us to explore the EC95 of ropivacaine for motor block in the future. Second, in this study, the onset time of anesthesia was observed, but the duration of anesthesia was not observed, which needs further study in the future. Third, we observed that the incidence of hypotension, bradycardia, and thirst significantly increased when ropivacaine was combined with 0.75 μg/kg and 1.0 μg/kg dexmedetomidine. However, the pairwise comparisons among the five groups did not reach statistical significance, likely attributable to the small sample size. To confirm these findings, a larger sample from randomized controlled trials is required.
5 Conclusion
Dexmedetomidine as an adjuvant for ropivacaine can dose-dependently reduce the EC50 of epidural ropivacaine for motor block and shorten the onset time of epidural ropivacaine block. 0.75 μg/kg and 1.0 μg/kg of dexmedetomidine combined with ropivacaine resulted in a significant reduction in heart rate. However, when patients were administered with 0.5 μg/kg of dexmedetomidine combined with ropivacaine, the heart rate remained largely consistent with that of the control group. Therefore, the optimal dose of dexmedetomidine combined with ropivacaine for epidural anesthesia was 0.5 μg/kg.
Data availability statement
The datasets presented in this study can be found in online repositories. The names of the repository/repositories and accession number(s) can be found at: http://www.chictr.org.cn/listbycreater.aspx.
Ethics statement
The studies involving humans were approved by the Ethics Committee of the Affiliated Hospital of North Sichuan Medical College. The studies were conducted in accordance with the local legislation and institutional requirements. The participants provided their written informed consent to participate in this study.
Author contributions
J-XW: Conceptualization, Data curation, Formal analysis, Funding acquisition, Investigation, Methodology, Project administration, Resources, Validation, Visualization, Writing – original draft, Writing – review & editing, Software, Supervision. CL: Conceptualization, Data curation, Formal analysis, Funding acquisition, Methodology, Validation, Visualization, Writing – original draft. Z-QW: Conceptualization, Data curation, Investigation, Methodology, Project administration, Resources, Visualization, Writing – original draft. DF: Conceptualization, Data curation, Formal analysis, Investigation, Methodology, Project administration, Resources, Writing – original draft. YW: Conceptualization, Formal analysis, Funding acquisition, Investigation, Methodology, Project administration, Resources, Writing – original draft. F-JW: Conceptualization, Data curation, Formal analysis, Funding acquisition, Investigation, Methodology, Project administration, Resources, Software, Supervision, Validation, Visualization, Writing – original draft, Writing – review & editing.
Funding
The author(s) declare that no financial support was received for the research, authorship, and/or publication of this article.
Conflict of interest
The authors declare that the research was conducted in the absence of any commercial or financial relationships that could be construed as a potential conflict of interest.
Publisher’s note
All claims expressed in this article are solely those of the authors and do not necessarily represent those of their affiliated organizations, or those of the publisher, the editors and the reviewers. Any product that may be evaluated in this article, or claim that may be made by its manufacturer, is not guaranteed or endorsed by the publisher.
References
1. Simon, MJ, Veering, BT, Stienstra, R, van Kleef, JW, and Burm, AG. The effects of age on neural blockade and hemodynamic changes after epidural anesthesia with ropivacaine. Anesth Analg. (2002) 94:1325–30. doi: 10.1097/00000539-200205000-00052
2. Schultz, AM, Werba, A, Ulbing, S, Gollmann, G, and Lehofer, F. Peri-operative thoracic epidural analgesia for thoracotomy. Eur J Anaesthesiol. (1997) 14:600–3. doi: 10.1097/00003643-199711000-00007
3. Sirvinskas, E, and Laurinaitis, R. Magnio sulfato panaudojimas anesteziologijai [Use of magnesium sulfate in anesthesiology]. Medicina. (2002) 38:695–8.
4. Park, WY, Thompson, JS, and Lee, KK. Effect of epidural anesthesia and analgesia on perioperative outcome: a randomized, controlled veterans affairs cooperative study. Ann Surg. (2001) 234:560–71. doi: 10.1097/00000658-200110000-00015
5. Holte, K, and Kehlet, H. Epidural anaesthesia and analgesia - effects on surgical stress responses and implications for postoperative nutrition. Clin Nutr. (2002) 21:199–206. doi: 10.1054/clnu.2001.0514
6. Rigg, JR, Jamrozik, K, Myles, PS, Silbert, BS, Peyton, PJ, Parsons, RW, et al. Epidural anaesthesia and analgesia and outcome of major surgery: a randomised trial. Lancet. (2002) 359:1276–82. doi: 10.1016/S0140-6736(02)08266-1
7. Moraca, RJ, Sheldon, DG, and Thirlby, RC. The role of epidural anesthesia and analgesia in surgical practice. Ann Surg. (2003) 238:663–73. doi: 10.1097/01.sla.0000094300.36689.ad
8. Choi, DH, Kim, JA, and Chung, IS. Comparison of combined spinal epidural anesthesia and epidural anesthesia for cesarean section. Acta Anaesthesiol Scand. (2000) 44:214–9. doi: 10.1034/j.1399-6576.2000.440214.x
9. Carrie, LE. Extradural, spinal or combined block for obstetric surgical anaesthesia. Br J Anaesth. (1990) 65:225–33. doi: 10.1093/bja/65.2.225
10. Brownridge, P. Epidural and subarachnoid analgesia for elective caesarean section. Anaesthesia. (1981) 36:70. doi: 10.1111/j.1365-2044.1981.tb08614.x
11. Polley, LS, Columb, MO, Wagner, DS, and Naughton, NN. Dose-dependent reduction of the minimum local analgesic concentration of bupivacaine by sufentanil for epidural analgesia in labor. Anesthesiology. (1998) 89:626–32. doi: 10.1097/00000542-199809000-00011
12. Chen, XR, Gao, T, Zhang, Y, and Peng, MQ. Addition of low-dose sufentanil to ropivacaine for reducing shivering and visceral traction pain during cesarean section. J Int Med Res. (2021) 49:3000605211017000. doi: 10.1177/03000605211017000
13. Lorenzini, C, Moreira, LB, and Ferreira, MB. Efficacy of ropivacaine compared with ropivacaine plus sufentanil for postoperative analgesia after major knee surgery. Anaesthesia. (2002) 57:424–8. doi: 10.1046/j.0003-2409.2001.02393.x
14. Wang, F, Yang, Z, Zeng, S, Gao, L, Li, J, and Wang, N. Effects of etomidate combined with dexmedetomidine on adrenocortical function in elderly patients: a double-blind randomized controlled trial. Sci Rep. (2022) 12:12296. doi: 10.1038/s41598-022-16679-1
15. Yoshitomi, T, Kohjitani, A, Maeda, S, Higuchi, H, Shimada, M, and Miyawaki, T. Dexmedetomidine enhances the local anesthetic action of lidocaine via an alpha-2A adrenoceptor. Anesth Analg. (2008) 107:96–101. doi: 10.1213/ane.0b013e318176be73
16. El-Hennawy, AM, Abd-Elwahab, AM, Abd-Elmaksoud, AM, El-Ozairy, HS, and Boulis, SR. Addition of clonidine or dexmedetomidine to bupivacaine prolongs caudal analgesia in children. Br J Anaesth. (2009) 103:268–74. doi: 10.1093/bja/aep159
17. Zhang, W, and Li, C. EC50 of epidural Ropivacaine combined with Dexmedetomidine for labor analgesia. Clin J Pain. (2018) 34:950–3. doi: 10.1097/AJP.0000000000000613
18. Solanki, SL, and Goyal, VK. Neuraxial dexmedetomidine: wonder drug or simply harmful. Anesth. Pain Med. (2015) 5:e22651. Published 2015 Mar 30. doi: 10.5812/aapm.22651
19. Abdallah, FW, and Brull, R. Facilitatory effects of perineural dexmedetomidine on neuraxial and peripheral nerve block: a systematic review and meta-analysis. Br J Anaesth. (2013) 110:915–25. doi: 10.1093/bja/aet066
20. Kamibayashi, T, and Maze, M. Clinical uses of alpha2 -adrenergic agonists. Anesthesiology. (2000) 93:1345–9. doi: 10.1097/00000542-200011000-00030
21. Kaur, S, Attri, JP, Kaur, G, and Singh, TP. Comparative evaluation of ropivacaine versus dexmedetomidine and ropivacaine in epidural anesthesia in lower limb orthopedic surgeries. Saudi J Anaesth. (2014) 8:463–9. doi: 10.4103/1658-354X.140838
22. Liu, L, Drzymalski, D, Xu, W, Zhang, W, Wang, L, and Xiao, F. Dose dependent reduction in median effective concentration (EC50) of ropivacaine with adjuvant dexmedetomidine in labor epidural analgesia: an up-down sequential allocation study. J Clin Anesth. (2021) 68:110115. doi: 10.1016/j.jclinane.2020.110115
23. Wangping, Z, and Ming, R. Optimal dose of epidural Dexmedetomidine added to Ropivacaine for epidural labor analgesia: a pilot study. Evid Based Complement Alternat Med. (2017) 2017:7924148–4. doi: 10.1155/2017/7924148
24. Xie, K, Wang, YL, Teng, WB, He, R, Li, YH, and Huang, SQ. The median effective concentration (EC50) of epidural Ropivacaine with different doses of oxycodone during limb surgery in elderly patients. Front Med. (2022) 8:808850. doi: 10.3389/fmed.2021.808850
25. Camorcia, M, Capogna, G, Berritta, C, and Columb, MO. The relative potencies for motor block after intrathecal ropivacaine, levobupivacaine, and bupivacaine. Anesth Analg. (2007) 104:904–7. doi: 10.1213/01.ane.0000256912.54023.79
26. Dixon, WJ. Staircase bioassay: the up-and-down method. Neurosci Biobehav Rev. (1991) 15:47–50. doi: 10.1016/s0149-7634(05)80090-9
27. Sessler, DI, Bloomstone, JA, Aronson, S, Berry, C, Gan, TJ, Kellum, JA, et al. Perioperative quality initiative consensus statement on intraoperative blood pressure, risk and outcomes for elective surgery. Br J Anaesth. (2019) 122:563–74. doi: 10.1016/j.bja.2019.01.013
28. Pace, NL, and Stylianou, MP. Advances in and limitations of up-and-down methodology: a précis of clinical use, study design, and dose estimation in anesthesia research. Anesthesiology. (2007) 107:144–52. doi: 10.1097/01.anes.0000267514.42592.2a
29. Kazim, M, Kothari, V, Priyadarshi, S, and Mistry, T. A comparative study to assess the effect of dexmedetomidine and fentanyl as an adjuvant to ropivacaine for epidural anesthesia in percutaneous nephrolithotomy. Anaesth Pain Intens Care. (2019) 2:59–64.
30. Kiran, S, Jinjil, K, Tandon, U, and Kar, S. Evaluation of dexmedetomidine and fentanyl as additives to ropivacaine for epidural anesthesia and postoperative analgesia. J Anaesthesiol Clin Pharmacol. (2018) 34:41–5. doi: 10.4103/joacp.JOACP_205_16
31. Lawhead, RG, Blaxall, HS, and Bylund, DB. Alpha-2A is the predominant alpha-2 adrenergic receptor subtype in human spinal cord. Anesthesiology. (1992) 77:983–91. doi: 10.1097/00000542-199211000-00022
32. Joy, R, Pujari, VS, Chadalawada, MV, Cheruvathoor, AV, Bevinguddaiah, Y, and Sheshagiri, N. Epidural ropivacaine with dexmedetomidine reduces propofol requirement based on bispectral index in patients undergoing lower extremity and abdominal surgeries. Anesth Essays Res. (2016) 10:45–9. doi: 10.4103/0259-1162.164676
33. Kanazi, GE, Aouad, MT, Jabbour-Khoury, SI, al Jazzar, MD, Alameddine, MM, al-Yaman, R, et al. Effect of low-dose dexmedetomidine or clonidine on the characteristics of bupivacaine spinal block. Acta Anaesthesiol Scand. (2006) 50:222–7. doi: 10.1111/j.1399-6576.2006.00919.x
34. Brown, EN, Pavone, KJ, and Naranjo, M. Multimodal general anesthesia: theory and practice. Anesth Analg. (2018) 127:1246–58. doi: 10.1213/ANE.0000000000003668
35. Weerink, MAS, Struys, MMRF, Hannivoort, LN, Barends, CRM, Absalom, AR, and Colin, P. Clinical pharmacokinetics and pharmacodynamics of Dexmedetomidine. Clin Pharmacokinet. (2017) 56:893–913. doi: 10.1007/s40262-017-0507-7
36. Ng, KT, Shubash, CJ, and Chong, JS. The effect of dexmedetomidine on delirium and agitation in patients in intensive care: systematic review and meta-analysis with trial sequential analysis. Anaesthesia. (2019) 74:380–92. doi: 10.1111/anae.14472
37. Salim, B, Rashid, S, Ali, MA, Raza, A, and Khan, FA. Effect of pharmacological agents administered for attenuating the Extubation response on the quality of Extubation: a systematic review. Cureus. (2019) 11:e6427. doi: 10.7759/cureus.6427
38. Wang, J, Wang, Z, Liu, J, and Wang, N. Intravenous dexmedetomidine versus tramadol for treatment of shivering after spinal anesthesia: a meta-analysis of randomized controlled trials. BMC Anesthesiol. (2020) 20. Published 2020 May 4:104. doi: 10.1186/s12871-020-01020-y
39. Sottas, CE, and Anderson, BJ. Dexmedetomidine: the new all-in-one drug in paediatric anaesthesia? Curr Opin Anaesthesiol. (2017) 30:441–51. doi: 10.1097/ACO.0000000000000488
Keywords: dexmedetomidine, epidural, median effective concentration, motor blockade, ropivacaine
Citation: Wan J-X, Lin C, Wu Z-Q, Feng D, Wang Y and Wang F-J (2024) The median effective concentration of epidural ropivacaine with different doses of dexmedetomidine for motor blockade: an up-down sequential allocation study. Front. Med. 11:1413191. doi: 10.3389/fmed.2024.1413191
Edited by:
Abhijit Nair, Ministry of Health, OmanReviewed by:
Minal Harde, Topiwala National Medical College and BYL Nair Charitable Hospital, IndiaAanchal Bharuka, Basavatarakam Indo American Cancer Hospital and Research Institute, India
Copyright © 2024 Wan, Lin, Wu, Feng, Wang and Wang. This is an open-access article distributed under the terms of the Creative Commons Attribution License (CC BY). The use, distribution or reproduction in other forums is permitted, provided the original author(s) and the copyright owner(s) are credited and that the original publication in this journal is cited, in accordance with accepted academic practice. No use, distribution or reproduction is permitted which does not comply with these terms.
*Correspondence: Fang-Jun Wang, d2ZqbHh5MDA2QG5zbWMuZWR1LmNu