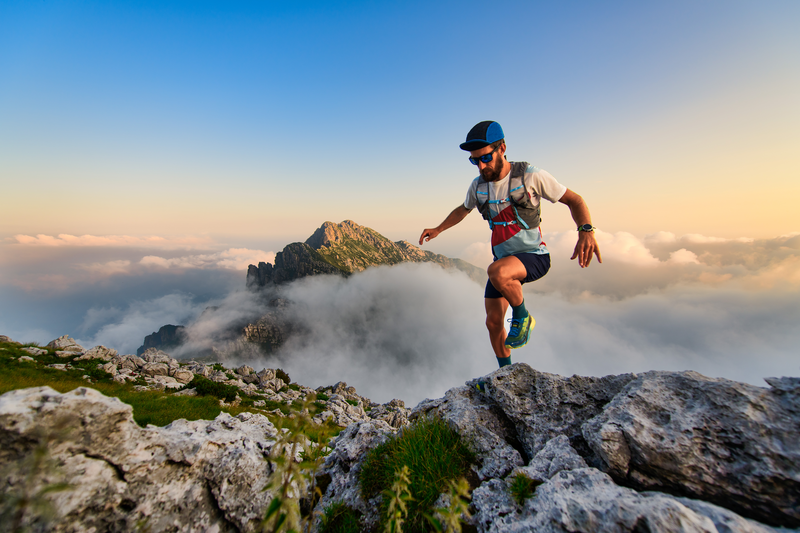
94% of researchers rate our articles as excellent or good
Learn more about the work of our research integrity team to safeguard the quality of each article we publish.
Find out more
REVIEW article
Front. Med. , 04 April 2024
Sec. Ophthalmology
Volume 11 - 2024 | https://doi.org/10.3389/fmed.2024.1384500
This article is part of the Research Topic Updates in Ocular Therapeutics and Surgery, volume III View all 12 articles
The repair mechanism for corneal epithelial cell injuries encompasses migration, proliferation, and differentiation of corneal epithelial cells, and extracellular matrix remodeling of the stromal structural integrity. Furthermore, it involves the consequential impact of corneal limbal stem cells (LSCs). In recent years, as our comprehension of the mediating mechanisms underlying corneal epithelial injury repair has advanced, it has become increasingly apparent that growth factors play a pivotal role in this intricate process. These growth factors actively contribute to the restoration of corneal epithelial injuries by orchestrating responses and facilitating specific interactions at targeted sites. This article systematically summarizes the role of growth factors in corneal epithelial cell injury repair by searching relevant literature in recent years, and explores the limitations of current literature search, providing a certain scientific basis for subsequent basic research and clinical applications.
The cornea, in direct contact with the external environment, stands out as one of the body’s tissues with the most dense innervation (1). Its structure is crucial for preserving the health and functionality of the ocular surface (Figure 1 shows the anatomical structure of the cornea). Damage to the corneal epithelium can result in corneal infections, ulcers, scar formation and vision loss ultimately. In recent years, factors such as surgical trauma, drug use, and infection have contributed to a rising number of patients with corneal epithelial injuries. As a result, the repair of corneal epithelial injuries has emerged not only as a prominent topic in basic scientific research but also as an urgent clinical problem that requires attention.
Figure 1. Histology of cornea. The cornea is structurally divided into five layers. The anterior epithelial layer comprises 5–7 layers of renewable epithelial cells. Behind the epithelial basement membrane lies the Bowman membrane, consisting of collagen fibers. The thickest layer, the stroma, primarily consists of keratocytes and collagen fibers, crucial for maintaining corneal transparency. Descemet, generated by the endothelium, is a transparent, elastic thin film with no distinct structure but possesses strong resistance. The endothelium is formed by a layer of hexagonal endothelial cells, incapable of regeneration.
The corneal epithelium plays a crucial role in safeguarding the eye’s barrier, stabilizing the tear film and maintaining the microenvironment of the ocular surface (2). Currently, the model governing corneal epithelial homeostasis relies on the XYZ hypothesis. According to this framework, the migration of limbal stem cells (LSCs) towards the central region of the cornea (X), combined with the vertical proliferation and differentiation of basal cells (Y), balances with the shedding of squamous cells (Z) from the epithelial surface. Healthy eyes are continuously bathed in tears containing growth factors, essential substances for maintaining the normal function of ocular surface tissue (3). Due to the unique anatomical location of the cornea, it is particularly susceptible to various injuries (4). When damaged, there is an upregulation of growth factors in tears, which target the cornea through relevant signaling pathways, thereby promoting corneal epithelial repair (5) and maintaining corneal epithelial homeostasis. Therefore, growth factors play a pivotal role in repairing corneal epithelial injuries and maintaining the normal microenvironment of the corneal epithelium.
In consideration of the aforementioned, this review aims to elucidate the roles played by various growth factors in the repair of corneal epithelial cells and comprehensively analyze their respective mechanisms of action. The overarching objective is to establish a robust scientific foundation that can serve as a springboard for subsequent basic research endeavors and clinical applications. The ensuing discussion will delve into the intricate interplay of growth factors within the context of repair mechanisms.
Epidermal growth factor (EGF) stands as one of the earliest identified single-chain peptides recognized for its ability to stimulate cell growth, playing a pivotal role in wound healing and maintaining tissue homeostasis by regulating cell survival, growth, motility and differentiation (6). In instances of corneal epithelial cell damage resulting from trauma, surgery or infection, EGF facilitates the migration and proliferation of corneal epithelial cells through the activation of its receptor EGFR/ErbB and subsequent binding. Consequently, this process promotes the effective repair of corneal epithelial injuries (7, 8). During the initial phases of corneal epithelial damage healing, EGFR1/ErbB1 tyrosine kinase instigates cellular signaling, activating downstream effectors such as the type III phosphoinositide 3-kinase (PI3K)—protein kinase B (Akt) axis and extracellular signal-regulated kinase (ERK). This orchestrated activation contributes significantly to the overall repair mechanism of corneal epithelial injuries (9). Furthermore, substance P also emerges as a noteworthy contributor to corneal epithelial injury repair, operating through the activation of EGFR and downstream signaling molecules, such as Akt (10). Abnormal activation of the EGFR-PI3K-AKT and ERK signaling pathways may result in increased cell apoptosis, decreased cell proliferation and delayed wound closure (11). The EGFR signaling pathway can further activate nuclear factor kappa-B (NF-κB) and histone deacetylase 6 (HDAC6). NF-κB, in turn, activates the transcription inhibitor CCCTC binding factor (CTCF) while downregulating the paired box gene 6 (PAX6), mediating the migration and proliferation of corneal epithelial cells. Simultaneously, HDAC6 promotes the migration of corneal epithelial cells and contributes to injury repair (12, 13).
There are four EGF receptors, with EGFR1 showing relatively high expression in corneal epithelial cells and demonstrating a reparative effect on the cornea during epithelial injury (14). EGFR2/ErbB2 and EGFR3/ErbB3 have also been confirmed to be expressed in the corneal epithelium, sharing a distribution pattern similar to EGFR1. Among them, the EGFR2/ErbB2 receptor enhances the corneal epithelial wound healing process by activating the ERK and PI3K signaling pathways (15). While the role of EGFR3/ErbB3 has not been fully elucidated, the existence of specific antibody inhibitors for EGFR3/ErbB3 has been confirmed. Utilizing these inhibitors and genetic techniques, studies have demonstrated that EGFR3/ErbB3 signaling can assist in the migration of corneal epithelial cells (16, 17). It’s worth noting that EGFR4/ErbB4 is not expressed in the corneal epithelium (18).
Presently, seven EGFR ligands have been identified. In addition to EGF, six other endogenous ligands capable of binding to EGFR have been recognized, including heparin-binding EGF-like growth factor (HB-EGF), transforming growth factor-α (TGF-α), betacellulin (BTC), epiregulin, amphiregulin, and epigen. HB-EGF, integral in promoting growth and development, plays a crucial role, as evidenced by the fact that knockout mice perish shortly after birth (19). Functioning as a soluble transmembrane protein, HB-EGF binds to an additional domain of negatively charged polysaccharides, thereby enhancing in vitro cell adhesion and promoting corneal epithelial injury repair (20). A notable discovery in the study indicates that HB-EGF exhibits prolonged cell attachment compared to EGF, resulting in a sustained impact on wound healing following brief therapy (21). TGF-α, a member of the epidermal growth factor family, is produced by both epidermal cells and macrophages. It plays a crucial role in the repair of corneal epithelial injuries by initiating multiple signaling cascade reactions upon binding with the EGFR (18, 22). The bidirectional interaction facilitated by TGF-α between corneal epithelial cells and mesenchymal cells assumes a pivotal morphological role in both corneal development and tissue repair. Any disruption in this intricate interplay can result in ocular lesions. Notably, TGF-α knockout mice exhibit significant ocular abnormalities, characterized by corneal epithelial thinning, inflammation, and edema (23, 24). TGF-α also promotes the proliferation of corneal epithelial and stromal cells (25). Additionally, TGF-α stimulates EGFR, facilitating the internalization and recycling of ligand-receptor complexes (22). Conversely, overexpression of TGF-α has been observed to induce corneal damage by activating EGFR in both corneal epithelium and stroma. This pathological manifestation is evident through a reduction in the number of corneal epithelial cell layers, corneal epithelial degeneration, conjunctivalization of the cornea, inhibition of the expression of the corneal pigment protein Kera, and a marked decrease in fibrocollagen types I and V collagen. Simultaneously, TGF-α overexpression can lead to corneal opacity by upregulating α-SMA and Wnt5a, while downregulating Col1a1, Col1a2, and Col5a1 (25–28). Some in vitro analysis of BTC indicates that BTC can expedite corneal epithelial injury repair and may even possess advantages over EGF in promoting corneal epithelial injury repair (18). LSCs, primarily located at the corneal-scleral junction, possess lifelong self-renewal capabilities and can produce transient amplifying cells (TACs). During corneal epithelial injury repair, TACs migrate towards the corneal center, proliferate, and differentiate into corneal epithelial cells, thereby promoting the healing of corneal epithelial wounds (29). Research has shown that treating injured mouse eyes with BTC results in significant increases in the expression of putative stem cell markers, such as DNp63α, ABCB5 and CK14. This suggests that BTC accelerates corneal LSCs proliferation and enhances mouse corneal epithelial repair by phosphorylating erk1/2 (30, 31). Despite the efficacy of various EGFR ligands in in vitro settings, in vivo wound healing is uniquely facilitated by EGF among the seven mentioned ligands. EGF also stands out as the sole ligand in human tears with an EGFR concentration closely aligned with the ligand Kd (18). Furthermore, EGFR can be reactivated through various effectors, such as phospholipase D (PLD) and extracellular ATP, to foster the migration and proliferation of cells during the wound healing process (32). Transient receptor potential (TRP) non-selective cation channels constitute a superfamily, which contains 28 different genes, and widely distributed in corneal epithelial cells and endothelial cells, its expression in the corneal epithelial layer contributes to the maintenance of corneal transparency and barrier function of the corneal epithelium. Research has shown that TRPV1 stimulation also induces increases in the proliferation and migration of corneal epithelial cells and the release of IL-6 and IL-8, and reduces the formation and of corneal neovascularization (CNV) and scar through transactivation of the EGFR. Meanwhile, TRPC4 stimulates corneal epithelial proliferation and migration by transactivation of the EGFR. TRPV in corneal epithelium can also promote homeostasis under thermal stimulation (33, 34).
While EGF holds the potential to stimulate the migration and proliferation of corneal epithelial cells, caution is warranted, as excessively increasing the intensity and duration of EGF may not yield positive effects. An experiment assessing EGF’s effectiveness has revealed potential harm from continuous daily injections in rats (35). Furthermore, injecting recombinant EGF into the cornea post-corneal epithelial cell injury can lead to CNV (36). Elevated tear EGF levels are also associated with meibomian duct hypertrophy, contributing to meibomian gland hyperplasia (37). These findings underscore the need for caution when employing exogenous EGF in treating corneal injuries. Additionally, EGFR activity is a critical determinant in maintaining corneal epithelial homeostasis and plays a pivotal role in restoring damaged corneal epithelial cells. Despite the inherent regulatory mechanisms preventing sustained EGFR signal transduction, a deeper understanding of the molecular mechanisms governing EGFR signaling holds promise for developing new methods to overcome these regulatory barriers and enhance the efficacy of EGF (Figure 2 shows the EGF signaling pathway).
Figure 2. The signaling pathway of EGF. This figure illustrates the signal transduction mechanism of EGF in corneal epithelial injury repair. EGF binding activates EGFR, stimulating various signaling pathways like PLCγ, Ras-GAP, Grb2, and Shc. These pathways collectively contribute to the reparative effects on corneal epithelial injuries.
Hepatocyte growth factor (HGF) is a growth factor originating from fibroblasts, predominantly produced by mesenchymal cells, and expressed in various cell types, including corneal epithelial cells, keratocytes and endothelial cells. Its mode of action is paracrine, exerting its effects on adjacent cells (38). Structurally, HGF consists of α- and β-chains and serves as a mitogen and motility factor. In the cornea, HGF plays a significant role by binding to its receptor c-met and primarily participating in the proliferation, mitosis, and morphogenesis of corneal epithelial cells (39–41).
When the corneal epithelium undergoes damage, the expression of HGF in corneal epithelial cells and keratocytes is upregulated. This upregulation activates the signal mediators phosphatidylinositol of PI3K/Akt, phosphoprotein 70 ribosomal protein S6 kinase (p70s6K), and ERK. Consequently, it controls the cell cycle, promoting cell division and proliferation of corneal epithelial cells by triggering the activity of NF-κB. Simultaneously, it reverses the anti-proliferative effect of pro-inflammatory cytokines interleukin-1β (IL-1β) and TNF-α on these cells in the inflammatory environment, mediating corneal epithelial injury repair (42–46). HGF also exhibits wound repair effects by inhibiting the inflammatory response of corneal epithelial cells. Studies have demonstrated that HGF can inhibit the activation of immune cells and the expression of inflammatory factors. It further suppresses the expression of TNF-α, monocyte chemotactic protein-1 (MCP-1), and IL-6 in the macrophage system in vitro. Additionally, it promotes the production of the anti-inflammatory cytokine IL-10 in bone marrow-derived macrophages and dendritic cells stimulated by lipopolysaccharide (LPS) (43, 47–49). Evidence supports that HGF significantly inhibits cell apoptosis, temporarily downregulates the expression of cell cycle inhibitors in corneal epithelial cells, and upregulates cyclin and cyclin-dependent kinases. It also influences tumor suppressor proteins Rb and p53, which regulate cell cycle and apoptosis. Through these mechanisms, HGF actively participates in corneal epithelial injury repair (50, 51). HGF also has the capability to penetrate through LPS-induced corneal opacity, promoting recovery, diminishing corneal fibrosis, restoring normal corneal tissue structure, and reestablishing immune quiescence after keratitis (52). In cases of diabetes-related corneal epithelial damage, HGF exhibits a reparative effect by restoring the level of c-met in the cornea of diabetic patients through downstream activation of p38 mitogen-activated protein kinase (MAPK) and the production of several putative stem cell markers. Importantly, this positive effect is observed in cultured corneas, regardless of whether gene therapy is applied to the entire corneal epithelial cells or only to the corneal edge area containing stem cells (53). Research indicates that silencing the HGF gene inhibits corneal epithelial proliferation and UVR-induced CNV. Additionally, HGF contributes to the upregulation of vascular endothelial growth factor (VEGF) and plays a role in angiogenesis regulation. These findings open up new avenues for exploring treatment strategies for CNV (54, 55).
Insulin-like growth factor (IGF) belongs to the multifunctional cell proliferation regulatory factor, representing a group of peptide substances capable of promoting growth. Its secretory cells are widely distributed in various tissues, including the liver, kidneys, heart and eyes of the human body. The IGF family comprises two peptide ligands (IGF-1 and IGF-2), three receptors, and six binding proteins, collectively maintaining tissue homeostasis by regulating metabolism and/or mitotic pathways at the level of all corneal cells (56).
IGF-1, a multifunctional cytokine with broad biological activity, holds considerable promise for applications in corneal epithelial injury repair. By binding to the insulin-like growth factor 1 receptor (IGF-1R), IGF-1 actively maintains and regulates corneal epithelial cell growth, proliferation, differentiation, maturation, migration, regeneration, and energy metabolism. It promotes corneal epithelial cell proliferation through the activation of the hybrid of IGF-1R and insulin receptor (INSR), leading to subsequent Akt phosphorylation. Additionally, IGF-1 mediates corneal epithelial cell migration through the PI3K/AKT pathway. Furthermore, IGF-1 promotes the expression of IGF receptors in corneal limbal cells, stimulating LSCs to differentiate into corneal epithelial cells (57, 58). Beyond its role in cell proliferation and migration, IGF-1 serves as a crucial neurotrophic factor facilitating the regeneration and restoration of nerves following peripheral nerve damage in the cornea (59). In combination with substance P, IGF-1 demonstrates a synergistic effect in promoting corneal epithelial injury repair. The co-application accelerates the ex vivo migration of corneal epithelial cells in the injured corneal stroma. Mediated by the interaction between substance P and tachykinin receptors, it enhances the adhesion of corneal epithelium to fibronectin (FN) and type IV collagen, thereby augmenting the protective role of the corneal epithelium through the stimulation of wound healing (60–64). The low levels of IGF-1 in tears, particularly the reduced proportion of IGF-1 and IGF binding protein 3 (IGFBP-3) in tears of diabetic patients, have been associated with decreased proliferation of corneal epithelial cells and delayed wound repair. This change inhibits the capacity of IGF-1 to induce IGF-1R or hybrid R phosphorylation (65, 66). Research has demonstrated that mRNA of adipose-derived stem cells (ADSCs) modified with IGF-1 exhibits stronger cell proliferation and migration abilities, promoting wound repair, morphological and functional recovery, corneal nerve regeneration, and maintenance of corneal homeostasis after acute alkali burns. Importantly, it can prevent the generation of CNV and corneal lymphatic vessels, highlighting the crucial role of IGF-1 in the repair of corneal epithelial injury (60). However, the application of IGF-1 protein to the cornea in the form of eye drops faces limitations, including a limited duration of effect, elevated attrition rates, and the need for repeated administration. Further research is expected to explore new carrier forms that can overcome these shortcomings and enhance the effectiveness of IGF-1 in corneal injury repair. The expression of IGF-2 and its receptors significantly increases after corneal epithelial cell injury, promoting the transformation of LSCs in the basal layer of the cornea into corneal epithelial cells and subsequently supporting corneal epithelial cell repair (67). Additionally, both IGF-1 and IGF-2 play roles in promoting the proliferation of keratocytes and collagen synthesis (68).
IGFBP primarily exists in the aqueous humor and vitreous body, exerting unique, cell- and tissue-dependent effects through interactions with the IGF family via binding (69–71). The primary function of IGFBP is to bind to IGF-1, extending its half-life in circulation and preventing IGF-1R activation induced by IGF-1 (72, 73). IGFBP-2 and IGFBP-3 play pivotal roles in corneal tissue homeostasis, particularly in regulating the growth of corneal epithelial cells and the localization of intracellular receptors (74, 75). The mutual regulation between IGFBP-3 and IGF-1R maintains corneal epithelial homeostasis. Previous studies have shown that IGFBP-3 is essential for inducing the transport of IGF-1R, and the absence of IGF-1R will downregulate IGFBP-3 in turn (76). During conditions such as hypoxia and hyperglycemia, the secretion of IGFBP-3 increases. For instance, the level of IGFBP-3 in the tears of diabetic patients rises, suggesting its potential role in regulating eye homeostasis in diabetic patients and indicating therapeutic potential in ocular surface diseases associated with diabetes (66).
Moreover, IGF and insulin share a close relationship, with the former mediating the action of insulin to promote the growth of corneal epithelial cells. This suggests a potential collaborative repair effect between the two, offering a promising avenue for future research exploration (77, 78).
Neurogenic growth factor (NGF) belongs to the family of neurotrophic factors, exhibiting dual biological functions of neuronal nourishment and promoting synaptic growth (79). In the context of the cornea, signals mediated by NGF propagate through the high-affinity receptor tropomyosin receptor kinase A (TrkA) and the low-affinity non-selective transmembrane glycoprotein receptor p75NTR. When combined with NGF, TrkA activates Ras MAPK, ERK, phospholipase C-γ (PLC-γ), and PI3K. This activation includes stimulating D-type cell cycle regulatory proteins through PI3K/Akt and MAPK/ERK, subsequently promoting corneal epithelial cell cycle progression. Simultaneously, p75NTR activates the c-Jun kinase and NF-kB signaling pathway, exhibiting a protective effect on corneal epithelial cells by inhibiting the inflammatory signaling pathway of NF-kB (80–82).
Research has demonstrated that NGF participates in the repair process of corneal epithelial and stromal damage by upregulating matrix metalloproteinase-9 (MMP-9) and cleaving integrins β4 to stimulate the migration of corneal epithelial cells, promotes the differentiation of keratocytes into myofibroblasts, and reduces the formation of corneal haze (83–85). Moreover, NGF induces the differentiation of goblet cells and the production of mucin through receptors expressed in the lacrimal gland and neural reflexes, thereby contributing to the maintenance of corneal epithelial function (86). In addition to its role in cellular functions, NGF regulates immune function through Toll-like receptors (TLRs) in corneal physiology and pathology, playing a crucial role in maintaining corneal homeostasis both in vivo and in vitro settings (87). NGF has also been identified as a key promoter for the proliferation of LSCs, the formation of colonies in LSCs, and the maintenance of the LSC phenotype (79). For patients with corneal ulcers, local application of NGF eye drops has been shown to improve the speed of corneal epithelial repair and the sensitivity of the cornea. In cases of herpes simplex keratitis (HSK), endogenous NGF, akin to acyclovir, significantly improves the condition and inhibits recurrence. Clinical studies indicate that eye drops containing NGF can induce complete healing in HSK patients resistant to acyclovir (88, 89). Treatment with recombinant human NGF (rhNGF) has proven effective in enhancing corneal perception in patients with neurotrophic keratitis (NK) by increasing the density and number of nerve fibers in the basal layer of the corneal epithelium. It also promotes the healing of persistent corneal epithelial defects and ulcers. Furthermore, rhNGF provides lubrication and natural protection against pathogen damage to the corneal epithelium by promoting tear secretion from the lacrimal gland. RhNGF has received approval as a primary therapeutic drug for NK (87, 88). Additionally, NGF exhibits the ability to inhibit oxidative damage caused by hyperosmotic stress or high glucose levels. This finding suggests its potential therapeutic effect on conditions such as dry eye syndrome and diabetic keratopathy (DK) (90, 91).
TGF is a protein composed of amino acids in the cytoplasm, belonging to the family of peptide growth factors. It includes two main types: TGF-α and TGF-β (92). TGF-α has been described in the EGF section. TGF-β is a multifunctional growth factor, further divided into three subtypes: TGF-β1, TGF-β2 and TGF-β3. All three subtypes and their receptors are expressed in corneal epithelium and keratocytes (93). TGF-β assumes a pivotal role in orchestrating and coordinating the response to corneal injury repair, exerting influence over various facets such as the proliferation, motility, and differentiation of corneal epithelial cells. Moreover, TGF-β modulates the activity and apoptosis of keratocytes, as well as the development of myofibroblasts (94). By stimulating the migration of corneal epithelial cells through integrin β1, TGF-β enhances the fluidity of these cells (95). Notably, the conditional ablation of its type II receptor has been found to impede the repair of corneal epithelial wounds and the activation of p38 MAPK, thereby hindering the migration of corneal epithelial cells (96). Furthermore, TGF-β2 has been substantiated to expedite the repair of corneal epithelial wounds in rabbits, augmenting barrier integrity by promoting cell adhesion to substrates and enhancing the functionality of corneal endothelial cells (CECs) (97). Tgfbr-2 also plays a crucial role in maintaining corneal stromal homeostasis, as studies have demonstrated that Tgfbr-2 knockout mice display significant corneal thinning and a potential for corneal ectasia (98). TGF-β3 exhibits the capability to mitigate interstitial scars induced by the activity of TGF-β1 and TGF-β2. Moreover, it demonstrates potential therapeutic effects in addressing corneal and skin wounds in diabetic patients, acting through the PI3K-Akt and SMAD signaling pathways, along with their target genes (99). Additionally, if the Bowman layer is damaged, corneal cells are highly susceptible to exposure to TGF-β. In such cases, TGF-β promotes damage repair through various mechanisms (100). Despite its essential role in corneal epithelial injury repair, TGF-β also has negative effects on the cornea. For instance, it can promote the aging of corneal epithelial cells through the NF-κB signaling pathway. This aging process can be alleviated by inhibiting the NF-κB signaling pathway (101). TGF-β is implicated in the pathogenesis of various eye diseases, including pterygium, vernal keratoconjunctivitis (VKC), atopic keratoconjunctivitis (AKC), and graft-versus-host disease (GVHD). Elevated levels of TGF-β are observed in the corneas of individuals with these diseases (102). Additionally, TGF-β regulates the transformation of corneal epithelial cells and corneal fibroblasts into myofibroblasts, and the high expression of α-SMA and F-actin in myofibroblasts can lead to the loss of corneal transparency and corresponding corneal haze (103). Moreover, TGF-β1 and TGF-β2 can prevent corneal epithelial cells from proliferating in vitro (104).
Platelet-derived growth factor (PDGF), secreted by epithelial cells, endothelial cells and inflammatory cells, serves as a potent mitogenic factor, existing in diverse isoforms, namely PDGF-AA, PDGF-BB, PDGF-CC, PDGF-DD, and PDGF-AB (105). Featuring both α and β types of receptors, PDGF exerts its cellular effects by inducing the complex formation of α-tyrosine kinase receptors and β-tyrosine kinase receptors. This induction, in turn, triggers processes such as cell growth, chemotaxis, actin recombination and protection against apoptosis. Analogous to TGF-β, PDGF assumes a pivotal role in regulating and coordinating the response to corneal wound repair. It influences the proliferation, motility and differentiation of corneal epithelial cells, while also modulating the activity and apoptosis of keratocytes and contributing to the development of myofibroblasts (94). Corneal epithelial cells express PDGF AA, PDGF BB and PDGF AB, which regulate the migration and proliferation of keratocytes. In the presence of FN, these isoforms can enhance the migration of corneal epithelial cells (106–108). Research indicates that PDGF-AB and PDGF-BB promote the migration of corneal fibroblasts in vitro, leading to an increase in the concentration of cytosolic free Ca2+. PDGF-BB also significantly stimulates DNA synthesis in bovine corneal endothelial cells (BCEC) and human corneal fibroblasts (HCF) in a dose-dependent manner (108–110). Moreover, under high fibroblast density, PDGF isomers act as mitogens for interstitial fibroblasts during wound healing, conversely, at low cell density, PDGF-AA and PDGF-AB can prevent cell loss during the corneal homeostasis process (111). The secretion of PDGF is activated during corneal trauma, infection, or inflammation, providing significant stimulation for tissue repair. However, hyperstimulation can have negative effects. For instance, PDGF-α hyperstimulation can promote the proliferation and migration of lens epithelial cells, leading to epithelial-mesenchymal transition (EMT) (112, 113).
Fibroblast growth factor (FGF) is secreted by the hypothalamus and pituitary gland, serving as a broad-spectrum mitogen, currently, at least 23 FGF families have been identified, stimulating or maintaining specific cellular functions required for tissue metabolism, homeostasis and development through signaling axes mediated by their receptors (114). Corneal epithelial changes, accompanied by decreased vision and dry eye symptoms, have been observed after treatment with inhibitors of the FGF receptor (FGFR), indirectly indicating FGF’s involvement in corneal epithelial homeostasis (115). Basic FGF (b-FGF/FGF2), approved for the treatment of corneal damage, accelerates the repair of corneal epithelial cell damage and reduces keratitis by promoting the proliferation, differentiation and migration of corneal epithelial cells (116, 117). In experiments involving FGFR2 knockout mice, observations reveal localized central corneal thinning, along with the loss of collagen fibers and apoptosis of keratocytes (118). In addition, the signal transduction of FGFR2b promotes corneal epithelial injury repair, studies have found that FGFR2b knockout mice exhibit reduced proliferation of corneal epithelial cells, as well as loss of lacrimal gland and meibomian gland. FGFR2b is also necessary for the development of submandibular glands (119). FGF-10 plays a crucial role in the development of the cornea, morphogenesis and growth of the lens and induction and branching of the lacrimal gland and meibomian gland. Research has shown that FGF-10 is essential for the development of lacrimal glands in humans and mice (120, 121). FGF-10 can upregulate the expression of mucin in conjunctival epithelial cells, protecting the ocular surface in a dry eye model of rabbits and controlling the migration of epithelial cells during the process of embryonic eyelid closure (122, 123). Additionally, FGF-10 is associated with adult tissue homeostasis and the function of stem cells (124). In an experimental study of DK, it has been found that rhFGF-21 can improve the vitality and migration of human corneal epithelial cells, promote the healing of corneal wounds and the production of tears, and improve corneal edema. RhFGF-21 significantly reduces the expression of pro-inflammatory cytokines such as TNF-α and MMPs in corneal epithelial cells, while increasing the level of anti-inflammatory molecules IL-10 and SOD-1. RhFGF-21 also inhibits excessive production of reactive oxygen species (ROS) and alleviates oxidative stress induced by hyperglycemia in corneal epithelial cells. Therefore, the application of drugs containing FGF-21 may be a potential treatment method for DK (125, 126).
Keratinocyte growth factor (KGF) belongs to the FGF family, officially known as FGF-7. It is produced by mesenchymal cells and acts on adjacent cells in a paracrine manner. KGF promotes the repair of corneal epithelial wounds through the signaling cascade of MAPK and PI3K/p70 S6 in corneal epithelial cells. It also inhibits the destruction of the barrier function caused by hypoxia in corneal epithelial cells by activating ERK (46, 127, 128). In vitro experiments have shown that KGF can protect cells from apoptosis for an extended period, with the final percentage of apoptosis in cells treated with KGF being only 10% (129). Similar to HGF, KGF has the capacity to inhibit UVR-induced corneal epithelial proliferation. Through gene silencing, it downregulates the expression of VEGF and its receptors, consequently mitigating CNV (48, 49). Additionally, KGF-2 exhibits certain effects in re-epithelialization, accelerating migration, reducing scar formation and edema. It is considered superior to b-FGF and holds potential as a new drug for treating corneal injuries (116). KGF also can promote the migration of LSCs, thereby promoting the repair of corneal epithelial damage (36).
Opioid growth factor (OGF) is an endogenous peptide found together with its receptor OGFr in or on the basal layer of many species’ corneas, when combined, OGF regulates DNA synthesis in corneal epithelial cells and influences cell migration (130). Experimental evidence suggests that OGF inhibits cell overgrowth by upregulating cyclin-dependent inhibitory kinases p16 and p21, contributing to the maintenance of corneal epithelial homeostasis (131). Studies conducted on patients and rats with diabetes have indicated elevated levels of OGF and OGFr in serum and corneal epithelium. This elevation has been associated with ocular surface complications, including dry eyes, abnormal sensitivity of the corneal surface, and delayed corneal epithelial repair. In rats, an OGF-OGFr axis is present in the corneal limbus, and its dysregulation in hyperglycemia impacts the morphology of the corneal limbus, exacerbating diabetes-related complications on the corneal surface. Local application of the opioid antagonist naltrexone (NTX) has demonstrated improvement in this situation (132, 133). NTX disrupts the OGF-OGFr interaction, resulting in increased DNA synthesis in epithelial cells of the peripheral cornea and limbus corneae, as well as the proliferation of fibroblast cells. The latter plays a crucial role in corneal wound healing (134, 135). Additionally, the application of NTX significantly promotes corneal re-epithelialization and increases tear production (136–138). In summary, the use of eye drops containing opioid antagonists, such as NTX, holds promise as a novel therapy for treating wound repair disorders of the corneal epithelium.
VEGF, recognized as a highly specific mitogen promoting endothelial cell growth, is also referred to as vascular permeability factor (VPF) due to its ability to significantly enhance vascular permeability (139). The VEGF family comprises seven subtypes: VEGF-A, VEGF-B, VEGF-C, VEGF-D, VEGF-E, VEGF-F, and placental growth factor (PLGF). Additionally, there are three receptors, VEGFR-1, VEGFR-2, and VEGFR-3. Among these, VEGF-A stands out for its profound ability to promote angiogenesis and is the most prevalent subtype in the eyes (140). The VEGF family and its receptors are expressed in the corneal epithelium (4). Under normal circumstances, a delicate balance is maintained between ocular angiogenic factors and anti-angiogenic factors to prevent pathological CNV production. However, factors such as wearing contact lenses, inflammation, and infection can disrupt this balance and lead to CNV (141). Research has highlighted the crucial role of VEGF in the pathogenesis of CNV, making the use of anti-VEGF drugs a feasible therapeutic approach (142, 143). Additionally, VEGF is implicated in non-angiogenic functions, such as neuroprotection and serving as a nutritional factor for corneal nerves (144). VEGF also plays a role in wound repair; studies have shown that VEGF accelerates corneal epithelial wound healing by stimulating corneal nerve regeneration (145). VEGF may be linked to the pathogenesis of pterygium, with higher expression detected in pterygium compared to normal tissue (146). Pigment epithelial-derived factors (PEDFs) are closely related to VEGF, sharing anti-angiogenic functions and a protective role for corneal nerves (147). Both factors exhibit synergistic therapeutic effects in certain diseases. However, in pterygium, a decrease in PEDF expression has been observed (146). Moreover, PEDF has been found to promote the self-renewal and migration of LSCs, thereby facilitating corneal epithelial repair (148). (The expression of growth factors in the corneal epithelium is depicted in Figure 3, and the biological effects of corneal epithelial growth factor receptors are summarized in Table 1).
Eye injuries often involve damage to the corneal epithelial layer, leading to symptoms such as eye pain, bleeding, ulcers, and vision loss, significantly impacting quality of life (151, 152). The repair of corneal epithelial injuries has emerged as a prominent research focus, with growing recognition of the crucial role played by growth factors in this process. These growth factors contribute to the wound healing of corneal epithelium through intricate mechanisms.
Despite the significant role of growth factors in corneal epithelial injury repair, there are existing limitations. The current understanding of the signal transduction pathways of various growth factors is not yet comprehensive, and their full potential as a treatment method for corneal epithelial injury remains to be realized. Some growth factors, like TGF, have stringent usage and dosage guidelines in corneal epithelium treatment—only within a safe usage range can they effectively repair the corneal epithelium. This presents a crucial and challenging aspect in utilizing growth factors for corneal epithelium treatment. For targeted repair effects of growth factors, many studies focus on single targets or signal pathways. The comprehensive repair mechanisms involving different growth factors still require further exploration. Additionally, some growth factors are primarily limited to basic research, and their potential for improving corneal epithelial cells in clinical practice needs further validation. Growth factors can be categorized into endogenous and exogenous types, with exogenous growth factors often utilized in experimental studies involving mice. However, when it comes to the role of growth factors in corneal epithelial injury repair, there is a noticeable gap in research and discussion regarding whether the mechanisms differ between the two types. Furthermore, in the case of the growth factor VEGF, exploring more suitable drug carriers could potentially enhance its therapeutic efficacy. This avenue of research could lead to the development of more effective delivery systems for VEGF, optimizing its impact on corneal epithelial repair.
Future research endeavors should leverage current multi-omics techniques to explore and study the signaling mechanisms and synergistic effects of different growth factors, aiming to enhance their roles in corneal epithelial injury repair. Simultaneously, through in-depth basic and clinical research, optimizing the dosage and understanding side effects of growth factors can contribute to revealing their basic mechanisms and optimal usage methods. This foundational work will pave the way for the development of new treatment methods involving growth factors. Further research and exploration are essential to determine potential differences in the roles of endogenous and exogenous growth factors in corneal epithelial repair, a facet not addressed in the current study. Additionally, investigating ways to enhance exogenous growth factors and identifying more suitable drug carriers are critical for optimizing the use of growth factors in the future.
JG: Writing – original draft. GD: Writing – review & editing. ZH: Writing – review & editing. YL: Writing – review & editing. AD: Writing – review & editing. CZ: Writing – review & editing.
The author(s) declare that financial support was received for the research, authorship, and/or publication of this article. This study was supported by Jinan Clinical Medical Technology Innovation Project (No. 202225058) and Shandong Province Medical and Health Technology Project (No. 202307021389).
The authors declare that the research was conducted in the absence of any commercial or financial relationships that could be construed as a potential conflict of interest.
All claims expressed in this article are solely those of the authors and do not necessarily represent those of their affiliated organizations, or those of the publisher, the editors and the reviewers. Any product that may be evaluated in this article, or claim that may be made by its manufacturer, is not guaranteed or endorsed by the publisher.
1. Ludwig, PE, Lopez, MJ, and Sevensma, KE. Anatomy, head and neck, eye cornea In: StatPearls. Treasure Island, FL: StatPearls Publishing (2023).
2. Downie, LE, Bandlitz, S, Bergmanson, JPG, Craig, JP, Dutta, D, Maldonado-Codina, C, et al. CLEAR—anatomy and physiology of the anterior eye. Cont Lens Anterior Eye. (2021) 44:132–56. doi: 10.1016/j.clae.2021.02.009
3. Koh, S, Rao, SK, Srinivas, SP, Tong, L, and Young, AL. Evaluation of ocular surface and tear function—a review of current approaches for dry eye. Indian J Ophthalmol. (2022) 70:1883–91. doi: 10.4103/ijo.IJO_1804_21
4. Tarvestad-Laise, KE, and Ceresa, BP. Modulating growth factor receptor signaling to promote corneal epithelial homeostasis. Cells. (2023) 12:2730. doi: 10.3390/cells12232730
5. Klenkler, B, Sheardown, H, and Jones, L. Growth factors in the tear film: role in tissue maintenance, wound healing, and ocular pathology. Ocul Surf. (2007) 5:228–39. doi: 10.1016/s1542-0124(12)70613-4
6. Shin, SH, Koh, YG, Lee, WG, Seok, J, and Park, KY. The use of epidermal growth factor in dermatological practice. Int Wound J. (2023) 20:2414–23. doi: 10.1111/iwj.14075
7. Zieske, JD, Takahashi, H, Hutcheon, AE, and Dalbone, AC. Activation of epidermal growth factor receptor during corneal epithelial migration. Invest Ophthalmol Vis Sci. (2000) 41:1346–55.
8. Wilson, SE . Corneal wound healing. Exp Eye Res. (2020) 197:108089. doi: 10.1016/j.exer.2020.108089
9. Zhang, Y, and Akhtar, RA. Epidermal growth factor stimulation of phosphatidylinositol 3-kinase during wound closure in rabbit corneal epithelial cells. Invest Ophthalmol Vis Sci. (1997) 38:1139–48.
10. Yang, L, Di, G, Qi, X, Qu, M, Wang, Y, Duan, H, et al. Substance P promotes diabetic corneal epithelial wound healing through molecular mechanisms mediated via the neurokinin-1 receptor. Diabetes. (2014) 63:4262–74. doi: 10.2337/db14-0163
11. Xu, K, and Yu, FS. Impaired epithelial wound healing and EGFR signaling pathways in the corneas of diabetic rats. Invest Ophthalmol Vis Sci. (2011) 52:3301–8. doi: 10.1167/iovs.10-5670
12. Li, T, and Lu, L. Epidermal growth factor-induced proliferation requires down-regulation of Pax 6 in corneal epithelial cells. J Biol Chem. (2005) 280:12988–95. doi: 10.1074/jbc.M412458200
13. Imanishi, J, Kamiyama, K, Iguchi, I, Kita, M, Sotozono, C, and Kinoshita, S. Growth factors: importance in wound healing and maintenance of transparency of the cornea. Prog Retin Eye Res. (2000) 19:113–29. doi: 10.1016/s1350-9462(99)00007-5
14. Liu, Z, Carvajal, M, Carraway, CA, Carraway, K, and Pflugfelder, SC. Expression of the receptor tyrosine kinases, epidermal growth factor receptor, ErbB2, and ErbB3, in human ocular surface epithelia. Cornea. (2001) 20:81–5. doi: 10.1097/00003226-200101000-00016
15. Xu, KP, Riggs, A, Ding, Y, and Yu, FS. Role of ErbB2 in corneal epithelial wound healing. Invest Ophthalmol Vis Sci. (2004) 45:4277–83. doi: 10.1167/iovs.04-0119
16. Huang, J, Wang, S, Lyu, H, Cai, B, Yang, XH, Wang, J, et al. The anti-ErbB3 antibody MM-121/SAR256212 in combination with trastuzumab exerts potent antitumor activity against trastuzumab-resistant breast cancer cells. Mol Cancer. (2013) 12:134. doi: 10.1186/1476-4598-12-134
17. Schoeberl, B, Faber, AC, Li, D, Liang, MC, Crosby, K, Onsum, M, et al. An ErbB3 antibody, MM-121, is active in cancers with ligand-dependent activation. Cancer Res. (2010) 70:2485–94. doi: 10.1158/0008-5472.CAN-09-3145
18. Peterson, JL, Phelps, ED, Doll, MA, Schaal, S, and Ceresa, BP. The role of endogenous epidermal growth factor receptor ligands in mediating corneal epithelial homeostasis. Invest Ophthalmol Vis Sci. (2014) 55:2870–80. doi: 10.1167/iovs.13-12943
19. Iwamoto, R, Yamazaki, S, Asakura, M, Takashima, S, Hasuwa, H, Miyado, K, et al. Heparin-binding EGF-like growth factor and ErbB signaling is essential for heart function. Proc Natl Acad Sci USA. (2003) 100:3221–6. doi: 10.1073/pnas.0537588100
20. Block, ER, Matela, AR, Sundar Raj, N, Iszkula, ER, and Klarlund, JK. Wounding induces motility in sheets of corneal epithelial cells through loss of spatial constraints: role of heparin-binding epidermal growth factor-like growth factor signaling. J Biol Chem. (2004) 279:24307–12. doi: 10.1074/jbc.M401058200
21. Tolino, MA, Block, ER, and Klarlund, JK. Brief treatment with heparin-binding EGF-like growth factor, but not with EGF, is sufficient to accelerate epithelial wound healing. Biochim Biophys Acta. (2011) 1810:875–8. doi: 10.1016/j.bbagen.2011.05.011
22. Roepstorff, K, Grandal, MV, Henriksen, L, Knudsen, SLJ, Lerdrup, M, Grøvdal, L, et al. Differential effects of EGFR ligands on endocytic sorting of the receptor. Traffic. (2009) 10:1115–27. doi: 10.1111/j.1600-0854.2009.00943.x
23. Singh, B, and Coffey, RJ. From wavy hair to naked proteins: the role of transforming growth factor alpha in health and disease. Semin Cell Dev Biol. (2014) 28:12–21. doi: 10.1016/j.semcdb.2014.03.003
24. Luetteke, NC, Qiu, TH, Peiffer, RL, Oliver, P, Smithies, O, and Lee, DC. TGF alpha deficiency results in hair follicle and eye abnormalities in targeted and waved-1 mice. Cell. (1993) 73:263–78. doi: 10.1016/0092-8674(93)90228-i
25. Zhang, L, Yuan, Y, Yeh, LK, Dong, F, Zhang, J, Okada, Y, et al. Excess transforming growth factor-α changed the cell properties of corneal epithelium and stroma. Invest Ophthalmol Vis Sci. (2020) 61:20. doi: 10.1167/iovs.61.8.20
26. Reneker, LW, Silversides, DW, Xu, L, and Overbeek, PA. Formation of corneal endothelium is essential for anterior segment development—a transgenic mouse model of anterior segment dysgenesis. Development. (2000) 127:533–42. doi: 10.1242/dev.127.3.533
27. Liu, CY, Shiraishi, A, Kao, CW, Converse, RL, Funderburgh, JL, Corpuz, LM, et al. The cloning of mouse keratocan cDNA and genomic DNA and the characterization of its expression during eye development. J Biol Chem. (1998) 273:22584–8. doi: 10.1074/jbc.273.35.22584
28. Chen, S, Mienaltowski, MJ, and Birk, DE. Regulation of corneal stroma extracellular matrix assembly. Exp Eye Res. (2015) 133:69–80. doi: 10.1016/j.exer.2014.08.001
29. Lehrer, MS, Sun, TT, and Lavker, RM. Strategies of epithelial repair: modulation of stem cell and transit amplifying cell proliferation. J Cell Sci. (1998) 111:2867–75. doi: 10.1242/jcs.111.19.2867
30. Jeong, WY, Yoo, HY, and Kim, CW. β-cellulin promotes the proliferation of corneal epithelial stem cells through the phosphorylation of erk1/2. Biochem Biophys Res Commun. (2018) 496:359–66. doi: 10.1016/j.bbrc.2018.01.054
31. Seyed-Safi, AG, and Daniels, JT. The limbus: structure and function. Exp Eye Res. (2020) 197:108074. doi: 10.1016/j.exer.2020.108074
32. Zhang, F, Yang, H, Pan, Z, Wang, Z, Wolosin, JM, Gjorstrup, P, et al. Dependence of resolvin-induced increases in corneal epithelial cell migration on EGF receptor transactivation. Invest Ophthalmol Vis Sci. (2010) 51:5601–9. doi: 10.1167/iovs.09-4468
33. Pan, Z, Yang, H, and Reinach, PS. Transient receptor potential (TRP) gene superfamily encoding cation channels. Hum Genomics. (2011) 5:108–16. doi: 10.1186/1479-7364-5-2-108
34. Yang, Y, Yang, H, Wang, Z, Okada, Y, Saika, S, and Reinach, PS. Wakayama symposium: dependence of corneal epithelial homeostasis on transient receptor potential function. Ocul Surf. (2013) 11:8–11. doi: 10.1016/j.jtos.2012.09.001
35. Hennessey, PJ, Nirgiotis, JG, Shinn, MN, and Andrassy, RJ. Continuous EGF application impairs long-term collagen accumulation during wound healing in rats. J Pediatr Surg. (1991) 26:362–6. doi: 10.1016/0022-3468(91)90980-8
36. Nezu, E, Ohashi, Y, Kinoshita, S, and Manabe, R. Recombinant human epidermal growth factor and corneal neovascularization. Jpn J Ophthalmol. (1992) 36:401–6.
37. Rao, K, Farley, WJ, and Pflugfelder, SC. Association between high tear epidermal growth factor levels and corneal subepithelial fibrosis in dry eye conditions. Invest Ophthalmol Vis Sci. (2010) 51:844–9. doi: 10.1167/iovs.09-3875
38. Bottaro, DP, Rubin, JS, Faletto, DL, Chan, AML, Kmiecik, TE, Vande Woude, GF, et al. Identification of the hepatocyte growth factor receptor as the c-met proto-oncogene product. Science. (1991) 251:802–4. doi: 10.1126/science.1846706
39. Pai, P, and Kittur, SK. Hepatocyte growth factor: a novel tumor marker for breast cancer. J Cancer Res Ther. (2023) 19:S0. doi: 10.4103/jcrt.JCRT_1084_16
40. Nakamura, T, Nishizawa, T, Hagiya, M, Seki, T, Shimonishi, M, Sugimura, A, et al. Molecular cloning and expression of human hepatocyte growth factor. Nature. (1989) 342:440–3. doi: 10.1038/342440a0
41. Wright, JW, Church, KJ, and Harding, JW. Hepatocyte growth factor and macrophage-stimulating protein “hinge” analogs to treat pancreatic cancer. Curr Cancer Drug Targets. (2019) 19:782–95. doi: 10.2174/1568009619666190326130008
42. Chandrasekher, G, Kakazu, AH, and Bazan, HE. HGF- and KGF-induced activation of PI-3K/p 70 s6 kinase pathway in corneal epithelial cells: its relevance in wound healing. Exp Eye Res. (2001) 73:191–202. doi: 10.1006/exer.2001.1026
43. Omoto, M, Suri, K, Amouzegar, A, Li, M, Katikireddy, KR, Mittal, SK, et al. Hepatocyte growth factor suppresses inflammation and promotes epithelium repair in corneal injury. Mol Ther. (2017) 25:1881–8. doi: 10.1016/j.ymthe.2017.04.020
44. Okunishi, K, Dohi, M, Fujio, K, Nakagome, K, Tabata, Y, Okasora, T, et al. Hepatocyte growth factor significantly suppresses collagen-induced arthritis in mice. J Immunol. (2007) 179:5504–13. doi: 10.4049/jimmunol.179.8.5504
45. Benkhoucha, M, Santiago-Raber, ML, Schneiter, G, Chofflon, M, Funakoshi, H, Nakamura, T, et al. Hepatocyte growth factor inhibits CNS autoimmunity by inducing tolerogenic dendritic cells and CD25+ Foxp3+ regulatory T cells. Proc Natl Acad Sci USA. (2010) 107:6424–9. doi: 10.1073/pnas.0912437107
46. Giannopoulou, M, Dai, C, Tan, X, Wen, X, Michalopoulos, GK, and Liu, Y. Hepatocyte growth factor exerts its anti-inflammatory action by disrupting nuclear factor-kappa B signaling. Am J Pathol. (2008) 173:30–41. doi: 10.2353/ajpath.2008.070583
47. Kusunoki, H, Taniyama, Y, Otsu, R, Rakugi, H, and Morishita, R. Anti-inflammatory effects of hepatocyte growth factor on the vicious cycle of macrophages and adipocytes. Hypertens Res. (2014) 37:500–6. doi: 10.1038/hr.2014.41
48. Coudriet, GM, He, J, Trucco, M, Mars, WM, and Piganelli, JD. Hepatocyte growth factor modulates interleukin-6 production in bone marrow derived macrophages: implications for inflammatory mediated diseases. PLoS One. (2010) 5:e15384. doi: 10.1371/journal.pone.0015384
49. Rutella, S, Bonanno, G, Procoli, A, Mariotti, A, de Ritis, DG, Curti, A, et al. Hepatocyte growth factor favors monocyte differentiation into regulatory interleukin (IL)-10++IL-12low/neg accessory cells with dendritic-cell features. Blood. (2006) 108:218–27. doi: 10.1182/blood-2005-08-3141
50. Chandrasekher, G, Pothula, S, Maharaj, G, and Bazan, HE. Differential effects of hepatocyte growth factor and keratinocyte growth factor on corneal epithelial cell cycle protein expression, cell survival, and growth. Mol Vis. (2014) 20:24–37.
51. Sherr, CJ, and McCormick, F. The RB and p 53 pathways in cancer. Cancer Cell. (2002) 2:103–12. doi: 10.1016/s1535-6108(02)00102-2
52. Elbasiony, E, Cho, W, Mittal, SK, and Chauhan, SK. Suppression of lipopolysaccharide-induced corneal opacity by hepatocyte growth factor. Sci Rep. (2022) 12:494. doi: 10.1038/s41598-021-04418-x
53. Sharma, GD, He, J, and Bazan, HE. p38 and ERK1/2 coordinate cellular migration and proliferation in epithelial wound healing: evidence of cross-talk activation between MAP kinase cascades. J Biol Chem. (2003) 278:21989–97. doi: 10.1074/jbc.M302650200
54. He, M, Han, T, Wang, Y, Wu, YH, Qin, WS, du, LZ, et al. Effects of HGF and KGF gene silencing on vascular endothelial growth factor and its receptors in rat ultraviolet radiation-induced corneal neovascularization. Int J Mol Med. (2019) 43:1888–99. doi: 10.3892/ijmm.2019.4114
55. Matsumura, A, Kubota, T, Taiyoh, H, Fujiwara, H, Okamoto, K, Ichikawa, D, et al. HGF regulates VEGF expression via the c-met receptor downstream pathways, PI3K/Akt, MAPK and STAT3, in CT26 murine cells. Int J Oncol. (2013) 42:535–42. doi: 10.3892/ijo.2012.1728
56. Baxter, RC . Signaling pathways of the insulin-like growth factor binding proteins. Endocr Rev. (2023) 44:753–78. doi: 10.1210/endrev/bnad008
57. Stuard, WL, Titone, R, and Robertson, DM. The IGF/insulin-IGFBP axis in corneal development, wound healing, and disease. Front Endocrinol. (2020) 11:24. doi: 10.3389/fendo.2020.00024
58. Trosan, P, Svobodova, E, Chudickova, M, Krulova, M, Zajicova, A, and Holan, V. The key role of insulin-like growth factor I in limbal stem cell differentiation and the corneal wound-healing process. Stem Cells Dev. (2012) 21:3341–50. doi: 10.1089/scd.2012.0180
59. Slavin, BR, Sarhane, KA, von Guionneau, N, Hanwright, PJ, Qiu, C, Mao, HQ, et al. Insulin-like growth factor-1: a promising therapeutic target for peripheral nerve injury. Front Bioeng Biotechnol. (2021) 9:695850. doi: 10.3389/fbioe.2021.695850
60. Yu, F, Gong, D, Yan, D, Wang, H, Witman, N, Lu, Y, et al. Enhanced adipose-derived stem cells with IGF-1-modified mRNA promote wound healing following corneal injury. Mol Ther. (2023) 31:2454–71. doi: 10.1016/j.ymthe.2023.05.002
61. Nakamura, M, Chikama, TI, and Nishida, T. Characterization of insulin-like growth factor-1 receptors in rabbit corneal epithelial cells. Exp Eye Res. (2000) 70:199–204. doi: 10.1006/exer.1999.0775
62. Todorović, V, Peško, P, Micev, M, Bjelović, M, Budeč, M, Mićić, M, et al. Insulin-like growth factor-I in wound healing of rat skin. Regul Pept. (2008) 150:7–13. doi: 10.1016/j.regpep.2008.05.006
63. Ghiasi, Z, Gray, T, Tran, P, Dubielzig, R, Murphy, C, McCartney, D, et al. The effect of topical substance-P plus insulin-like growth factor-1 (IGF-1) on epithelial healing after photorefractive keratectomy in rabbits. Transl Vis Sci Technol. (2018) 7:12. doi: 10.1167/tvst.7.1.12
64. Nagano, T, Nakamura, M, Nakata, K, Yamaguchi, T, Takase, K, Okahara, A, et al. Effects of substance P and IGF-1 in corneal epithelial barrier function and wound healing in a rat model of neurotrophic keratopathy. Invest Ophthalmol Vis Sci. (2003) 44:3810–5. doi: 10.1167/iovs.03-0189
65. Patel, R, Zhu, M, and Robertson, DM. Shifting the IGF-axis: an age-related decline in human tear IGF-1 correlates with clinical signs of dry eye. Growth Hormon IGF Res. (2018) 40:69–73. doi: 10.1016/j.ghir.2018.02.001
66. Wu, YC, Buckner, BR, Zhu, M, Cavanagh, HD, and Robertson, DM. Elevated IGFBP3 levels in diabetic tears: a negative regulator of IGF-1 signaling in the corneal epithelium. Ocul Surf. (2012) 10:100–7. doi: 10.1016/j.jtos.2012.01.004
67. Jiang, Y, Ju, Z, Zhang, J, Liu, X, Tian, J, and Mu, G. Effects of insulin-like growth factor 2 and its receptor expressions on corneal repair. Int J Clin Exp Pathol. (2015) 8:10185–91.
68. Hassell, JR, and Birk, DE. The molecular basis of corneal transparency. Exp Eye Res. (2010) 91:326–35. doi: 10.1016/j.exer.2010.06.021
69. Ferry, RJ Jr, Katz, LE, Grimberg, A, Cohen, P, and Weinzimer, SA. Cellular actions of insulin-like growth factor binding proteins. Horm Metab Res. (1999) 31:192–202. doi: 10.1055/s-2007-978719
70. Arnold, DR, Moshayedi, P, Schoen, TJ, Jones, BE, Chader, GJ, and Waldbillig, RJ. Distribution of IGF-I and -II, IGF binding proteins (IGFBPs) and IGFBP mRNA in ocular fluids and tissues: potential sites of synthesis of IGFBPs in aqueous and vitreous. Exp Eye Res. (1993) 56:555–65. doi: 10.1006/exer.1993.1069
71. Titone, R, Zhu, M, and Robertson, DM. Insulin mediates de novo nuclear accumulation of the IGF-1/insulin hybrid receptor in corneal epithelial cells. Sci Rep. (2018) 8:4378. doi: 10.1038/s41598-018-21031-7
72. Conover, CA, and Oxvig, C. PAPP-A: a promising therapeutic target for healthy longevity. Aging Cell. (2017) 16:205–9. doi: 10.1111/acel.12564
73. Argente, J, Chowen, JA, Pérez-Jurado, LA, Frystyk, J, and Oxvig, C. One level up: abnormal proteolytic regulation of IGF activity plays a role in human pathophysiology. EMBO Mol Med. (2017) 9:1338–45. doi: 10.15252/emmm.201707950
74. Park, SH, Kim, KW, and Kim, JC. The role of insulin-like growth factor binding protein 2 (IGFBP2) in the regulation of corneal fibroblast differentiation. Invest Ophthalmol Vis Sci. (2015) 56:7293–302. doi: 10.1167/iovs.15-16616
75. Rao, P, Suvas, PK, Jerome, AD, Steinle, JJ, and Suvas, S. Role of insulin-like growth factor binding protein-3 in the pathogenesis of herpes stromal keratitis. Invest Ophthalmol Vis Sci. (2020) 61:46. doi: 10.1167/iovs.61.2.46
76. Titone, R, Zhu, M, and Robertson, DM. Mutual regulation between IGF-1R and IGFBP-3 in human corneal epithelial cells. J Cell Physiol. (2019) 234:1426–41. doi: 10.1002/jcp.26948
77. Lima, MH, Caricilli, AM, de Abreu, LL, Araújo, EP, Pelegrinelli, FF, Thirone, ACP, et al. Topical insulin accelerates wound healing in diabetes by enhancing the AKT and ERK pathways: a double-blind placebo-controlled clinical trial. PLoS One. (2012) 7:e36974. doi: 10.1371/journal.pone.0036974
78. Wirostko, B, Rafii, M, Sullivan, DA, Morelli, J, and Ding, J. Novel therapy to treat corneal epithelial defects: a hypothesis with growth hormone. Ocul Surf. (2015) 13:204–212.e1. doi: 10.1016/j.jtos.2014.12.005
79. Kolli, S, Bojic, S, Ghareeb, AE, Kurzawa-Akanbi, M, Figueiredo, FC, and Lako, M. The role of nerve growth factor in maintaining proliferative capacity, colony-forming efficiency, and the limbal stem cell phenotype. Stem Cells. (2019) 37:139–49. doi: 10.1002/stem.2921
80. Huang, EJ, and Reichardt, LF. Trk receptors: roles in neuronal signal transduction. Annu Rev Biochem. (2003) 72:609–42. doi: 10.1146/annurev.biochem.72.121801.161629
81. Lambiase, A, Manni, L, Bonini, S, Rama, P, Micera, A, and Aloe, L. Nerve growth factor promotes corneal healing: structural, biochemical, and molecular analyses of rat and human corneas. Invest Ophthalmol Vis Sci. (2000) 41:1063–9.
82. Chen, H, Zhang, J, Dai, Y, and Xu, J. Nerve growth factor inhibits TLR3-induced inflammatory cascades in human corneal epithelial cells. J Inflamm. (2019) 16:27. doi: 10.1186/s12950-019-0232-0
83. Micera, A, Lambiase, A, Puxeddu, I, Aloe, L, Stampachiacchiere, B, Levi-Schaffer, F, et al. Nerve growth factor effect on human primary fibroblastic-keratocytes: possible mechanism during corneal healing. Exp Eye Res. (2006) 83:747–57. doi: 10.1016/j.exer.2006.03.010
84. Blanco-Mezquita, T, Martinez-Garcia, C, Proença, R, Zieske, JD, Bonini, S, Lambiase, A, et al. Nerve growth factor promotes corneal epithelial migration by enhancing expression of matrix metalloprotease-9. Invest Ophthalmol Vis Sci. (2013) 54:3880–90. doi: 10.1167/iovs.12-10816
85. Anitua, E, Muruzabal, F, Alcalde, I, Merayo-Lloves, J, and Orive, G. Plasma rich in growth factors (PRGF-endoret) stimulates corneal wound healing and reduces haze formation after PRK surgery. Exp Eye Res. (2013) 115:153–61. doi: 10.1016/j.exer.2013.07.007
86. Muzi, S, Colafrancesco, V, Sornelli, F, Mantelli, F, Lambiase, A, and Aloe, L. Nerve growth factor in the developing and adult lacrimal glands of rat with and without inherited retinitis pigmentosa. Cornea. (2010) 29:1163–8. doi: 10.1097/ICO.0b013e3181d3d3f9
87. Aloe, L, Tirassa, P, and Lambiase, A. The topical application of nerve growth factor as a pharmacological tool for human corneal and skin ulcers. Pharmacol Res. (2008) 57:253–8. doi: 10.1016/j.phrs.2008.01.010
88. Aloe, L, Rocco, ML, Balzamino, BO, and Micera, A. Nerve growth factor: a focus on neuroscience and therapy. Curr Neuropharmacol. (2015) 13:294–303. doi: 10.2174/1570159x13666150403231920
89. Wu, D, Qian, T, Hong, J, Li, G, Shi, W, and Xu, J. Micro RNA-494 inhibits nerve growth factor-induced cell proliferation by targeting cyclin D1 in human corneal epithelial cells. Mol Med Rep. (2017) 16:4133–42. doi: 10.3892/mmr.2017.7083
90. Sun, Z, Hu, W, Yin, S, Lu, X, Zuo, W, Ge, S, et al. NGF protects against oxygen and glucose deprivation-induced oxidative stress and apoptosis by up-regulation of HO-1 through MEK/ERK pathway. Neurosci Lett. (2017) 641:8–14. doi: 10.1016/j.neulet.2017.01.046
91. Park, JH, Kang, SS, Kim, JY, and Tchah, H. Nerve growth factor attenuates apoptosis and inflammation in the diabetic cornea. Invest Ophthalmol Vis Sci. (2016) 57:6767–75. doi: 10.1167/iovs.16-19747
92. Ding, Z, Jiang, M, Qian, J, Gu, D, Bai, H, Cai, M, et al. Role of transforming growth factor-β in peripheral nerve regeneration. Neural Regen Res. (2024) 19:380–6. doi: 10.4103/1673-5374.377588
93. Nishida, K, Kinoshita, S, Yokoi, N, Kaneda, M, Hashimoto, K, and Yamamoto, S. Immunohistochemical localization of transforming growth factor-beta 1, -beta 2, and -beta 3 latency-associated peptide in human cornea. Invest Ophthalmol Vis Sci. (1994) 35:3289–94.
94. Singh, V, Jaini, R, Torricelli, AA, Santhiago, MR, Singh, N, Ambati, BK, et al. TGFβ and PDGF-B signaling blockade inhibits myofibroblast development from both bone marrow-derived and keratocyte-derived precursor cells in vivo. Exp Eye Res. (2014) 121:35–40. doi: 10.1016/j.exer.2014.02.013
95. Bhowmick, NA, Zent, R, Ghiassi, M, McDonnell, M, and Moses, HL. Integrin beta 1 signaling is necessary for transforming growth factor-beta activation of p38MAPK and epithelial plasticity. J Biol Chem. (2001) 276:46707–13. doi: 10.1074/jbc.M106176200
96. Terai, K, Call, MK, Liu, H, Saika, S, Liu, CY, Hayashi, Y, et al. Crosstalk between TGF-beta and MAPK signaling during corneal wound healing. Invest Ophthalmol Vis Sci. (2011) 52:8208–15. doi: 10.1167/iovs.11-8017
97. Er, H, and Uzmez, E. Effects of transforming growth factor-beta 2, interleukin 6 and fibronectin on corneal epithelial wound healing. Eur J Ophthalmol. (1998) 8:224–9. doi: 10.1177/112067219800800404
98. Wang, YC, Zolnik, OB, Yasuda, S, Yeh, LK, Yuan, Y, Kao, W, et al. Transforming growth factor beta receptor 2 (Tgfbr 2) deficiency in keratocytes results in corneal ectasia. Ocul Surf. (2023) 29:557–65. doi: 10.1016/j.jtos.2023.06.014
99. Bettahi, I, Sun, H, Gao, N, Wang, F, Mi, X, Chen, W, et al. Genome-wide transcriptional analysis of differentially expressed genes in diabetic, healing corneal epithelial cells: hyperglycemia-suppressed TGFβ3 expression contributes to the delay of epithelial wound healing in diabetic corneas. Diabetes. (2014) 63:715–27. doi: 10.2337/db13-1260
100. Rocher, M, Robert, PY, and Desmoulière, A. The myofibroblast, biological activities and roles in eye repair and fibrosis. A focus on healing mechanisms in avascular cornea. Eye. (2020) 34:232–40. doi: 10.1038/s41433-019-0684-8
101. Li, ZY, Chen, ZL, Zhang, T, Wei, C, and Shi, WY. TGF-β and NF-κB signaling pathway crosstalk potentiates corneal epithelial senescence through an RNA stress response. Aging. (2016) 8:2337–54. doi: 10.18632/aging.101050
102. Benito, MJ, Calder, V, Corrales, RM, García-Vázquez, C, Narayanan, S, Herreras, JM, et al. Effect of TGF-β on ocular surface epithelial cells. Exp Eye Res. (2013) 107:88–100. doi: 10.1016/j.exer.2012.11.017
103. Fink, MK, Giuliano, EA, Tandon, A, and Mohan, RR. Therapeutic potential of pirfenidone for treating equine corneal scarring. Vet Ophthalmol. (2015) 18:242–50. doi: 10.1111/vop.12194
104. Saikia, P, Thangavadivel, S, Medeiros, CS, Lassance, L, de Oliveira, RC, and Wilson, SE. IL-1 and TGF-β modulation of epithelial basement membrane components perlecan and nidogen production by corneal stromal cells. Invest Ophthalmol Vis Sci. (2018) 59:5589–98. doi: 10.1167/iovs.18-25202
105. Heldin, CH, and Westermark, B. Platelet-derived growth factor: mechanism of action and possible in vivo function. Cell Regul. (1990) 1:555–66. doi: 10.1091/mbc.1.8.555
106. Daniels, JT, and Khaw, PT. Temporal stimulation of corneal fibroblast wound healing activity by differentiating epithelium in vitro. Invest Ophthalmol Vis Sci. (2000) 41:3754–62.
107. Nishida, T . Translational research in corneal epithelial wound healing. Eye Contact Lens. (2010) 36:300–4. doi: 10.1097/ICL.0b013e3181f016d0
108. Kamiyama, K, Iguchi, I, Wang, X, and Imanishi, J. Effects of PDGF on the migration of rabbit corneal fibroblasts and epithelial cells. Cornea. (1998) 17:315–25. doi: 10.1097/00003226-199805000-00013
109. Hoppenreijs, VP, Pels, E, Vrensen, GF, Felten, PC, and Treffers, WF. Platelet-derived growth factor: receptor expression in corneas and effects on corneal cells. Invest Ophthalmol Vis Sci. (1993) 34:637–49.
110. Williamson, JR, and Monck, JR. Hormone effects on cellular Ca2+ fluxes. Annu Rev Physiol. (1989) 51:107–24. doi: 10.1146/annurev.ph.51.030189.000543
111. Denk, PO, and Knorr, M. The in vitro effect of platelet-derived growth factor isoforms on the proliferation of bovine corneal stromal fibroblasts depends on cell density. Graefes Arch Clin Exp Ophthalmol. (1997) 235:530–4. doi: 10.1007/BF00947012
112. Schultz, T, Conrad-Hengerer, I, Hengerer, FH, and Dick, HB. Intraocular pressure variation during femtosecond laser-assisted cataract surgery using a fluid-filled interface. J Cataract Refract Surg. (2013) 39:22–7. doi: 10.1016/j.jcrs.2012.10.038
113. Wei, J, Tang, H, Xu, ZQ, Li, B, Xie, LQ, and Xu, GX. Expression and function of PDGF-α in columnar epithelial cells of age-related cataracts patients. Genet Mol Res. (2015) 14:13320–7. doi: 10.4238/2015.October.26.28
114. Jin, L, Yang, R, Geng, L, and Xu, A. Fibroblast growth factor-based pharmacotherapies for the treatment of obesity-related metabolic complications. Annu Rev Pharmacol Toxicol. (2023) 63:359–82. doi: 10.1146/annurev-pharmtox-032322-093904
115. Shin, E, Lim, DH, Han, J, Nam, DH, Park, K, Ahn, MJ, et al. Markedly increased ocular side effect causing severe vision deterioration after chemotherapy using new or investigational epidermal or fibroblast growth factor receptor inhibitors. BMC Ophthalmol. (2020) 20:19. doi: 10.1186/s12886-019-1285-9
116. Cai, J, Zhou, Q, Wang, Z, Guo, R, Yang, R, Yang, X, et al. Comparative analysis of KGF-2 and bFGF in prevention of excessive wound healing and scar formation in a corneal alkali burn model. Cornea. (2019) 38:1430–7. doi: 10.1097/ICO.0000000000002134
117. Reneker, LW, Irlmeier, RT, Shui, YB, Liu, Y, and Huang, AJW. Histopathology and selective biomarker expression in human meibomian glands. Br J Ophthalmol. (2020) 104:999–1004. doi: 10.1136/bjophthalmol-2019-314466
118. Zhang, J, Upadhya, D, Lu, L, and Reneker, LW. Fibroblast growth factor receptor 2 (FGFR2) is required for corneal epithelial cell proliferation and differentiation during embryonic development. PLoS One. (2015) 10:e0117089. doi: 10.1371/journal.pone.0117089
119. Joseph, R, Boateng, A, Srivastava, OP, and Pfister, RR. Role of fibroblast growth factor receptor 2 (FGFR2) in corneal stromal thinning. Invest Ophthalmol Vis Sci. (2023) 64:40. doi: 10.1167/iovs.64.12.40
120. Qu, X, Carbe, C, Tao, C, Powers, A, Lawrence, R, van Kuppevelt, TH, et al. Lacrimal gland development and Fgf 10-Fgfr2b signaling are controlled by 2-O- and 6-O-sulfated heparan sulfate. J Biol Chem. (2011) 286:14435–44. doi: 10.1074/jbc.M111.225003
121. Tsau, C, Ito, M, Gromova, A, Hoffman, MP, Meech, R, and Makarenkova, HP. Barx 2 and Fgf 10 regulate ocular glands branching morphogenesis by controlling extracellular matrix remodeling. Development. (2011) 138:3307–17. doi: 10.1242/dev.066241
122. Ma, M, Zhang, Z, Niu, W, Zheng, W, Kelimu, J, and Ke, B. Fibroblast growth factor 10 upregulates the expression of mucins in rat conjunctival epithelial cells. Mol Vis. (2011) 17:2789–97.
123. Tao, H, Shimizu, M, Kusumoto, R, Ono, K, Noji, S, and Ohuchi, H. A dual role of FGF10 in proliferation and coordinated migration of epithelial leading edge cells during mouse eyelid development. Development. (2005) 132:3217–30. doi: 10.1242/dev.01892
124. Thotakura, S, Basova, L, and Makarenkova, HP. FGF gradient controls boundary position between proliferating and differentiating cells and regulates lacrimal gland growth dynamics. Front Genet. (2019) 10:362. doi: 10.3389/fgene.2019.00362
125. Li, J, Zhang, R, Wang, C, Wang, X, Xu, M, Ma, J, et al. Activation of the small GTPase rap 1 inhibits choroidal neovascularization by regulating cell junctions and ROS generation in rats. Curr Eye Res. (2018) 43:934–40. doi: 10.1080/02713683.2018.1454477
126. Li, L, Wang, H, Pang, S, Wang, L, Fan, Z, Ma, C, et al. Rh FGF-21 accelerates corneal epithelial wound healing through the attenuation of oxidative stress and inflammatory mediators in diabetic mice. J Biol Chem. (2023) 299:105127. doi: 10.1016/j.jbc.2023.105127
127. Wilson, SE, Li, Q, Mohan, RR, Tervo, T, Vesaluoma, M, Bennett, GL, et al. Lacrimal gland growth factors and receptors: lacrimal fibroblastic cells are a source of tear HGF. Adv Exp Med Biol. (1998) 438:625–8. doi: 10.1007/978-1-4615-5359-5_88
128. Teranishi, S, Kimura, K, Kawamoto, K, and Nishida, T. Protection of human corneal epithelial cells from hypoxia-induced disruption of barrier function by keratinocyte growth factor. Invest Ophthalmol Vis Sci. (2008) 49:2432–7. doi: 10.1167/iovs.07-1464
129. Wilson, SE, Walker, JW, Chwang, EL, and He, YG. Hepatocyte growth factor, keratinocyte growth factor, their receptors, fibroblast growth factor receptor-2, and the cells of the cornea. Invest Ophthalmol Vis Sci. (1993) 34:2544–61.
130. Kareem, ZY, McLaughlin, PJ, and Kumari, R. Opioid growth factor receptor: anatomical distribution and receptor colocalization in neurons of the adult mouse brain. Neuropeptides. (2023) 99:102325. doi: 10.1016/j.npep.2023.102325
131. Cheng, F, McLaughlin, PJ, Verderame, MF, and Zagon, IS. The OGF-OGFr axis utilizes the p16INK4a and p21WAF1/CIP1 pathways to restrict normal cell proliferation. Mol Biol Cell. (2009) 20:319–27. doi: 10.1091/mbc.e08-07-0681
132. McLaughlin, PJ, Sassani, JW, Titunick, MB, and Zagon, IS. Efficacy and safety of a novel naltrexone treatment for dry eye in type 1 diabetes. BMC Ophthalmol. (2019) 19:35. doi: 10.1186/s12886-019-1044-y
133. McLaughlin, PJ, Sassani, JW, Diaz, D, and Zagon, IS. Elevated opioid growth factor alters the limbus in type 1 diabetic rats. J Diabetes Clin Res. (2023) 5:1–10. doi: 10.33696/diabetes.4.054
134. Zagon, IS, Sassani, JW, Purushothaman, I, and McLaughlin, PJ. Blockade of OGFr delays the onset and reduces the severity of diabetic ocular surface complications. Exp Biol Med. (2021) 246:629–36. doi: 10.1177/1535370220972060
135. Immonen, JA, Zagon, IS, and McLaughlin, PJ. Selective blockade of the OGF-OGFr pathway by naltrexone accelerates fibroblast proliferation and wound healing. Exp Biol Med. (2014) 239:1300–9. doi: 10.1177/1535370214543061
136. Zagon, IS, Sassani, JW, Purushothaman, I, and McLaughlin, PJ. Dysregulation of the OGF-OGFr pathway correlates with elevated serum OGF and ocular surface complications in the diabetic rat. Exp Biol Med. (2020) 245:1414–21. doi: 10.1177/1535370220940273
137. Zagon, IS, Klocek, MS, Sassani, JW, and McLaughlin, PJ. Dry eye reversal and corneal sensation restoration with topical naltrexone in diabetes mellitus. Arch Ophthalmol. (2009) 127:1468–73. doi: 10.1001/archophthalmol.2009.270
138. Klocek, MS, Sassani, JW, McLaughlin, PJ, and Zagon, IS. Naltrexone and insulin are independently effective but not additive in accelerating corneal epithelial healing in type I diabetic rats. Exp Eye Res. (2009) 89:686–92. doi: 10.1016/j.exer.2009.06.010
139. Bussolino, F, di Renzo, MF, Ziche, M, Bocchietto, E, Olivero, M, Naldini, L, et al. Hepatocyte growth factor is a potent angiogenic factor which stimulates endothelial cell motility and growth. J Cell Biol. (1992) 119:629–41. doi: 10.1083/jcb.119.3.629
140. Beheshtizadeh, N, Gharibshahian, M, Bayati, M, Maleki, R, Strachan, H, Doughty, S, et al. Vascular endothelial growth factor (VEGF) delivery approaches in regenerative medicine. Biomed Pharmacother. (2023) 166:115301. doi: 10.1016/j.biopha.2023.115301
141. Giannaccare, G, Pellegrini, M, Bernabei, F, Scorcia, V, and Campos, E. Ocular surface system alterations in ocular graft-versus-host disease: all the pieces of the complex puzzle. Graefes Arch Clin Exp Ophthalmol. (2019) 257:1341–51. doi: 10.1007/s00417-019-04301-6
142. Amano, S, Rohan, R, Kuroki, M, Tolentino, M, and Adamis, AP. Requirement for vascular endothelial growth factor in wound- and inflammation-related corneal neovascularization. Invest Ophthalmol Vis Sci. (1998) 39:18–22.
143. Giannaccare, G, Pellegrini, M, Bovone, C, Spena, R, Senni, C, Scorcia, V, et al. Anti-VEGF treatment in corneal diseases. Curr Drug Targets. (2020) 21:1159–80. doi: 10.2174/1389450121666200319111710
144. Goldhardt, R, Batawi, HIM, Rosenblatt, M, Lollett, IV, Park, JJ, and Galor, A. Effect of anti-vascular endothelial growth factor therapy on corneal nerves. Cornea. (2019) 38:559–64. doi: 10.1097/ICO.0000000000001871
145. Pan, Z, Fukuoka, S, Karagianni, N, Guaiquil, VH, and Rosenblatt, MI. Vascular endothelial growth factor promotes anatomical and functional recovery of injured peripheral nerves in the avascular cornea. FASEB J. (2013) 27:2756–67. doi: 10.1096/fj.12-225185
146. Jin, J, Guan, M, Sima, J, Gao, G, Zhang, M, Liu, Z, et al. Decreased pigment epithelium-derived factor and increased vascular endothelial growth factor levels in pterygia. Cornea. (2003) 22:473–7. doi: 10.1097/00003226-200307000-00015
147. Yeh, SI, Yu, SH, Chu, HS, Huang, CT, Tsao, YP, Cheng, CM, et al. Pigment epithelium-derived factor peptide promotes corneal nerve regeneration: an in vivo and in vitro study. Invest Ophthalmol Vis Sci. (2021) 62:23. doi: 10.1167/iovs.62.1.23
148. Ho, TC, Chen, SL, Wu, JY, Ho, MY, Chen, LJ, Hsieh, JW, et al. PEDF promotes self-renewal of limbal stem cell and accelerates corneal epithelial wound healing. Stem Cells. (2013) 31:1775–84. doi: 10.1002/stem.1393
149. Versura, P, Giannaccare, G, Pellegrini, M, Sebastiani, S, and Campos, EC. Neurotrophic keratitis: current challenges and future prospects. Eye Brain. (2018) 10:37–45. doi: 10.2147/EB.S117261
150. Hao, M, Cheng, Y, Wu, J, Cheng, Y, and Wang, J. Clinical observation of recombinant human nerve growth factor in the treatment of neurotrophic keratitis. Int J Ophthalmol. (2023) 16:60–6. doi: 10.18240/ijo.2023.01.09
151. Sridhar, MS . Anatomy of cornea and ocular surface. Indian J Ophthalmol. (2018) 66:190–4. doi: 10.4103/ijo.IJO_646_17
152. Sun, X, Song, W, Teng, L, Huang, Y, Liu, J, Peng, Y, et al. MiRNA 24-3p-rich exosomes functionalized DEGMA-modified hyaluronic acid hydrogels for corneal epithelial healing. Bioact Mater. (2022) 25:640–56. doi: 10.1016/j.bioactmat.2022.07.011
Keywords: cornea, corneal epithelial cells, growth factors, growth factor receptor, repair mechanism
Citation: Gong J, Ding G, Hao Z, Li Y, Deng A and Zhang C (2024) Elucidating the mechanism of corneal epithelial cell repair: unraveling the impact of growth factors. Front. Med. 11:1384500. doi: 10.3389/fmed.2024.1384500
Received: 09 February 2024; Accepted: 21 March 2024;
Published: 04 April 2024.
Edited by:
Georgios D. Panos, Nottingham University Hospitals NHS Trust, United KingdomReviewed by:
Ruchi Shah, Cedars Sinai Medical Center, United StatesCopyright © 2024 Gong, Ding, Hao, Li, Deng and Zhang. This is an open-access article distributed under the terms of the Creative Commons Attribution License (CC BY). The use, distribution or reproduction in other forums is permitted, provided the original author(s) and the copyright owner(s) are credited and that the original publication in this journal is cited, in accordance with accepted academic practice. No use, distribution or reproduction is permitted which does not comply with these terms.
*Correspondence: Chenming Zhang, Y2hlbm1pbmctemhhbmdAMTYzLmNvbQ==; Aijun Deng, ZGVuZ2FpanVuQGhvdG1haWwuY29t
Disclaimer: All claims expressed in this article are solely those of the authors and do not necessarily represent those of their affiliated organizations, or those of the publisher, the editors and the reviewers. Any product that may be evaluated in this article or claim that may be made by its manufacturer is not guaranteed or endorsed by the publisher.
Research integrity at Frontiers
Learn more about the work of our research integrity team to safeguard the quality of each article we publish.