- Department of Ophthalmology, Tongji Hospital, Tongji Medical College, Huazhong University of Science and Technology, Wuhan, China
In the field of eye health, the profound impact of exercise and physical activity on various ocular diseases has become a focal point of attention. This review summarizes and elucidates the positive effects of exercise and physical activities on common ocular diseases, including dry eye disease (DED), cataracts, myopia, glaucoma, diabetic retinopathy (DR), and age-related macular degeneration (AMD). It also catalogues and offers exercise recommendations based on the varying impacts that different types and intensities of physical activities may have on specific eye conditions. Beyond correlations, this review also compiles potential mechanisms through which exercise and physical activity beneficially affect eye health. From mitigating ocular oxidative stress and inflammatory responses, reducing intraocular pressure, enhancing mitochondrial function, to promoting ocular blood circulation and the release of protective factors, the complex biological effects triggered by exercise and physical activities reveal their substantial potential in preventing and even assisting in the treatment of ocular diseases. This review aims not only to foster awareness and appreciation for how exercise and physical activity can improve eye health but also to serve as a catalyst for further exploration into the specific mechanisms and key targets through which exercise impacts ocular health. Such inquiries are crucial for advancing innovative strategies for the treatment of eye diseases, thereby holding significant implications for the development of new therapeutic approaches.
1 Introduction
Eye health is vital for maintaining physical and mental well-being, which has extensive implications on individual quality of life, economic prosperity of nations, and sustainable development of societies (1). However, global eye health statistics show an alarming picture, with more than 2.2 billion people worldwide suffering from visual impairment, of whom more than 1 billion face moderates to severe visual impairment or even complete blindness (2). Unfortunately, numerous instances of visual impairment within this demographic can be prevented or intervened early, such as cataracts, refractive errors and glaucoma (2). Nevertheless, countless individuals and families endure the repercussions of visual impairment or complete loss of vision due to obstacles in obtaining affordable and high-quality eye care and treatment (2). Recognizing that eye health is a global public priority and repositioning it as a development and health issue, the Global Commission on Eye Health has gained increased attention (1).
In recent years, exercise and physical activity have gained growing attention as cost-effective, feasible and accessible lifestyle interventions for the treatment of various human ailments, notably cardiovascular and other chronic diseases (3). Several studies have indicated that long-term regular exercise and physical activity serve as crucial and modifiable interventions for enhancing the management of diverse medical conditions, including, but not limited to, hypertension, diabetes, obesity, cancer, osteoporosis, osteoarthritis, and depression (4). A cohort study of 110,482 healthy middle-aged adults over an 8 years period revealed that low physical fitness was linked to a higher incidence of mortality from various causes (5). It has been reported that physical inactivity has the highest prevalence among risk factors that can be intervened upon by humans (3). Despite the extensively documented benefits of physical activity in many systemic disorders, exercise is underutilized as an intervention in practice. Astonishingly, only about 26% of men and 19% of women are estimated to meet international physical activity guidelines (6, 7).
The positive impacts of exercise on eye health are increasingly recognized and widely validated by a growing body of research (8–11). This review synthesizes existing studies to describe the potential benefits of exercise and physical activities on common ocular diseases. Based on current epidemiological evidence and exercise guidelines, this review provides exercise recommendations for early to mid-stage patients of specific ocular diseases or high-risk individuals who are physically fit for exercise. It is hoped that this will elevate the awareness and importance of exercise in eye health among various sectors of society. Additionally, this review summarizes and elucidates possible mechanisms through which exercise and physical activity impact common ocular diseases, including direct mechanisms such as lowering intraocular pressure and blood glucose, as well as indirect mechanisms like inhibiting oxidative stress and inflammatory responses, and promoting the release of protective factors. The discussion on mechanisms aims to encourage researchers to delve deeper into unveiling the specific mechanisms and key targets through which exercise positively affects eye health, thereby providing theoretical support for the development of new treatment strategies for ocular diseases.
2 Exercise and physical activity
For sedentary people, the lack of specific knowledge about exercise is one of the main obstacles to the implementation of effective “exercise therapy,” including the differentiation and selection of the type and intensity of exercise (Figure 1).
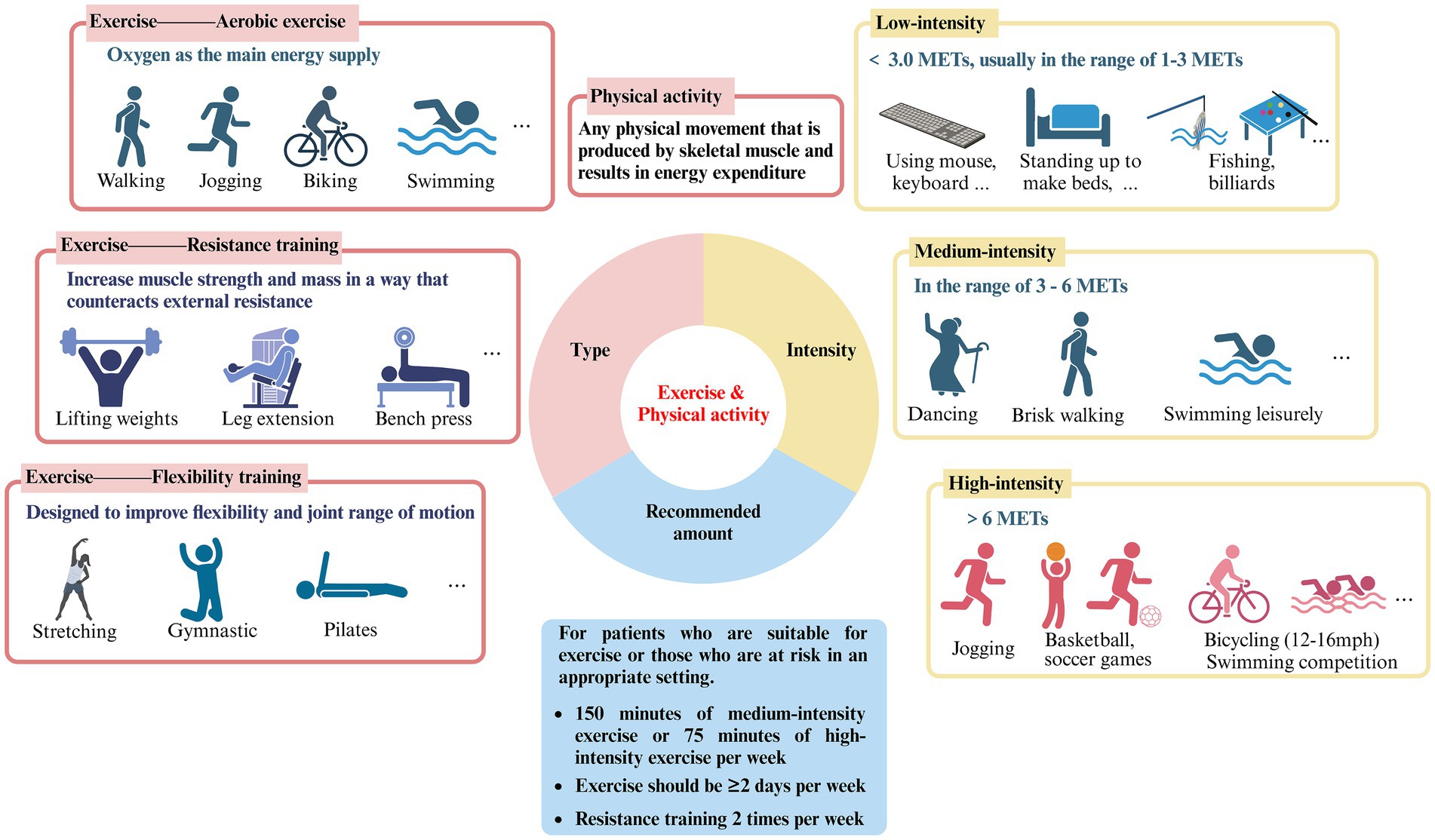
Figure 1. Type, intensity and recommended amount of exercise and physical activity (created with Biorender.com).
2.1 Simple classification
The terms “exercise” and “physical activity” are frequently used interchangeably in literature. Nonetheless, a thorough analysis of these two concepts reveals that they are not precisely the same (12). Exercise refers to organized, planned physical activity that can be categorized into three groups: aerobic exercise, resistance training, and flexibility training. Aerobic exercise uses oxygen as the primary energy supply and includes activities such as running, cycling, swimming, brisk walking, and jumping rope. Resistance training, also known as strength training, involves countering external resistance to increase muscle strength and mass. This can include weight lifting, gymnastics, pull-ups, and the use of devices such as machines or rubber bands. Flexibility training is designed to increase flexibility and joint range of motion and includes activities such as yoga, Pilates, stretching, and gymnastics (13). Physical activity involves a variety of movements generated by skeletal muscles, leading to energy expenditure and encompassing an extensive array of occupational, recreational, and daily activities (12). However, overly detailed requirements and divisions have the potential to confuse and overwhelm individuals in regards to the implementation of “exercise therapy,” leading to a decrease in enthusiasm towards adhering to it. Based on the above explanations, it appears that various exercise modalities have been subsumed into the broader category of physical activity, potentially accounting for why the two terms are often used synonymously in existing literature. Therefore, this review does not make a specific distinction between the two.
2.2 Intensity
Variations in exercise intensity have been strongly associated with effects on ocular disease. The metabolic equivalent of task (MET) is a unit of measurement of the body’s energy expenditure at different levels of activity, with 1 MET representing the energy expenditure of a person at quiet sitting and rest, which is approximately 3.5 mcal/kg/min (14). It allows for the comparison of various forms of exercise and physical activity relative to energy expenditure during periods of rest, thus classifying the intensity of exercise, which is widely recognized and applied (15).
Low-intensity exercise has an energy expenditure of less than 3.0 METs, usually in the range of 1–3 METs, and specifically includes walking slowly (2.0), sitting and using hand tools such as mice and keyboards (1.5), standing for light physical activities such as making beds and washing dishes (1.5–2.5), playing pool (2.5), fishing (2.5), throwing darts (2.5), playing a musical instrument (2.0–2.5), etc.
Moderate-intensity exercise has METs between 3–6, comparable to a brisk walk which significantly accelerates the heart rate. Similar physical activities include washing windows, cars (3.0), sweeping floors (3.0–3.5), carrying & stacking wood (5.5), playing badminton recreationally (4.5), bicycling slowly on flat ground (6.0), dancing (3.0–4.5), playing table tennis (4.0), swimming leisurely (6.0), playing volleyball noncompetitively playing volleyball noncompetitively (3.0–4.0), etc.
High-intensity exercise, with jogging being a typical example, is defined as exercise greater than 6.0 METs, which can cause shortness of breath and a large increase in heart rate. Also included are carrying heavy loads such as bricks (7.5), heavy farming such as bailing hay (8.0), basketball game (8.0), bicycling on flat terrain with moderate (12–14 mph) or fast (14–16 mph) effort (8.0–10.0), cross country skiing (7.0–9.0), playing soccer (7.0–10.0), swimming with moderate to hard effort (8–11), playing competitive volleyball at the gym or beach (8.0), etc.
2.3 Modulation of ocular physiology by exercise and physical activity
The biological effects of exercise and physical activity extend across multiple facets and locations, positively impacting eye health by maintaining the health of normal physiological mechanisms of the eye and offering protection against diseases. Research indicates that exercise can increase tear production, contributing to the stability of the tear film and improvements in DED (16). In terms of corneal health, exercise regulates intraocular pressure, aiding in the maintenance of corneal health and mitigating acute corneal thinning and endothelial cell damage caused by acute high intraocular pressure (17). For the lens, there is a negative correlation between the intensity of physical activity and the degree of lens opacity (18); moderate exercise can reduce levels of inflammation and oxidative stress, slowing down the aging process of the lens (19). Exercise and physical activity also aid in weight control, reduces blood pressure and blood sugar levels, thus diminishing the impact of metabolic factors on the structure and function of the lens (20). The retina, with its intricate structure and complex functions, has a high blood flow and is susceptible to internal and external pathogenic factors. Exercise contributes to improving retinal microvascular blood supply and oxygen return, maintaining the health and functionality of retinal cells (21). Furthermore, exercise enhances the body’s antioxidative capacity and anti-inflammatory responses, protecting retinal cells from oxidative stress and inflammation, thereby reducing the risk of ocular diseases such as AMD and DR (22, 23). By boosting the expression of various neurotrophic factors, such as brain-derived neurotrophic factor (BDNF), exercise potentially offers retinal neuroprotection (24). Additionally, exercise can lower the risk of diabetes, hypertension, and other chronic diseases, preventing associated retinal pathologies such as DR.
2.4 Recommendations
According to the Global Recommendations on Physical Activity for Health and the United States Health and Human Services Physical Activity Guidelines for Adults, it is advised that adults engage in at least 150 min of moderate-intensity exercise or 75 min of vigorous-intensity exercise per week, with the possibility of combining both types based on individual circumstances (7, 25, 26). Exercise sessions should be spread across at least 2 days per week, and additionally, engaging in resistance training twice weekly is also recommended. Pertaining to eye health, the epidemiological evidence cited in this review primarily focuses on the positive impacts of moderate to vigorous-intensity exercise on specific ocular diseases, with the included study subjects being well-suited for physical activity. Consequently, we recommend that early to mid-stage patients of the ocular diseases listed in this review (who are in good physical condition) or high-risk individuals, persistently engage in long-term moderate to vigorous-intensity physical activities, such as running, walking, swimming, and cycling, in a suitable environment to potentially slow the progression of diseases or ameliorate symptoms. It should be noted that our recommendations come with certain prerequisites, as this review does not purport to suggest that exercise can serve as a direct treatment modality for specific ocular conditions. When afflicted with any eye disease, seeking medical assistance always takes precedence. Our aim is for patients to thoroughly assess their own conditions against the backdrop of medical treatment and consider maintaining physical activity and exercise as a supplementary means to assist treatment and improve their condition.
3 Effects of exercise and physical activity on ocular diseases
3.1 DED
3.1.1 Effect of exercise and physical activity on DED
DED is a chronic disease affecting the ocular surface, caused by various factors and thought to be a functional unit disorder of the lacrimal gland, resulting in insufficient quantity and quality of tears to lubricate the eyes (27, 28). DED can cause persistent symptoms of irritation or burning in the eyes and vision impairment that negatively impacts daily life. Epidemiological studies have found that up to 75% of adults over the age of 40 may have DED, and it tends to worsen over time once it develops (29, 30).
In recent years, ample evidence has linked DED with lifestyle, highlighting the impact of exercise, diet, and sleep on its development (31). Two extensive cross-sectional studies based on questionnaires discovered that an increased level of exercise contributes to a reduced prevalence of DED. Individuals experiencing DED had reduced physical activity levels and increased sedentary behavior compared to those without DED (32, 33). Additionally, research indicates that physical activity can positively impact alleviating symptoms of DED. A cross-sectional study conducted among 944 web-based workers in China revealed that individuals who engaged in exercise at a minimum of three times per week experienced significantly reduced discomfort associated with their eyes, including decreased visual clarity (34). Another randomized controlled trial focusing on sedentary young adults in the office setting observed a significant decrease in discomfort symptoms, including eye pain, soreness, and eyestrain, after 3 months of exercise following an exercise guideline (35). These findings imply that exercise and physical activity may contribute to delaying the onset and slowing the progression of DED.
3.1.2 Potential mechanisms
Numerous studies have demonstrated that loss of tear film homeostasis is the central mechanism of DED (31). In addition, tear hyperosmolarity, ocular surface inflammation, oxidative stress, ocular surface injury, and neurosensory abnormalities have all been identified as significant etiological factors in DED (36). Based on pathophysiological differences, DED can be classified into two categories: aqueous-deficient dry eye (ADDE) and evaporative dry eye (EDE) (29). Tear hyperosmolarity caused by decreased tear production (ADDE) or excessive evaporation (EDE) is one of the fundamental mechanisms of DED. Elevated osmolarity triggers apoptosis in epithelial cells and goblet cells, consequently reducing the lubrication ability of the ocular surface. This results in rapid tear film breakup and an additional rise in hyperosmolarity, thereby creating a self-perpetuating cycle. With increasing osmolarity, ocular surface damage increases and oxidative stress intensifies, eventually resulting in tear film homeostasis disruption (37). Studies have shown a temporary surge in tear volume and extended tear film rupture following aerobic exercise, indicating enhanced tear film stability (16). A prospective cross-sectional study involving 43 healthy participants found significant reductions in tear fluid levels of inflammation-related cytokine, including interferon-γ (IFN-γ), tumor necrosis factor-α (TNF-α), interleukin (IL)-1β, IL-4, IL-5, and dozens of others, after a 20 min exercise session in comparison to the baseline levels (38). More excitingly, exercise reduced the tear fluid concentration of the marker of oxidative stress, 8-hydroxy-2′-deoxyguanosine (8-OHdG), suggesting that exercise may play a role in alleviating DED by reducing the oxidative stress present in tears (39) (see Table 1).
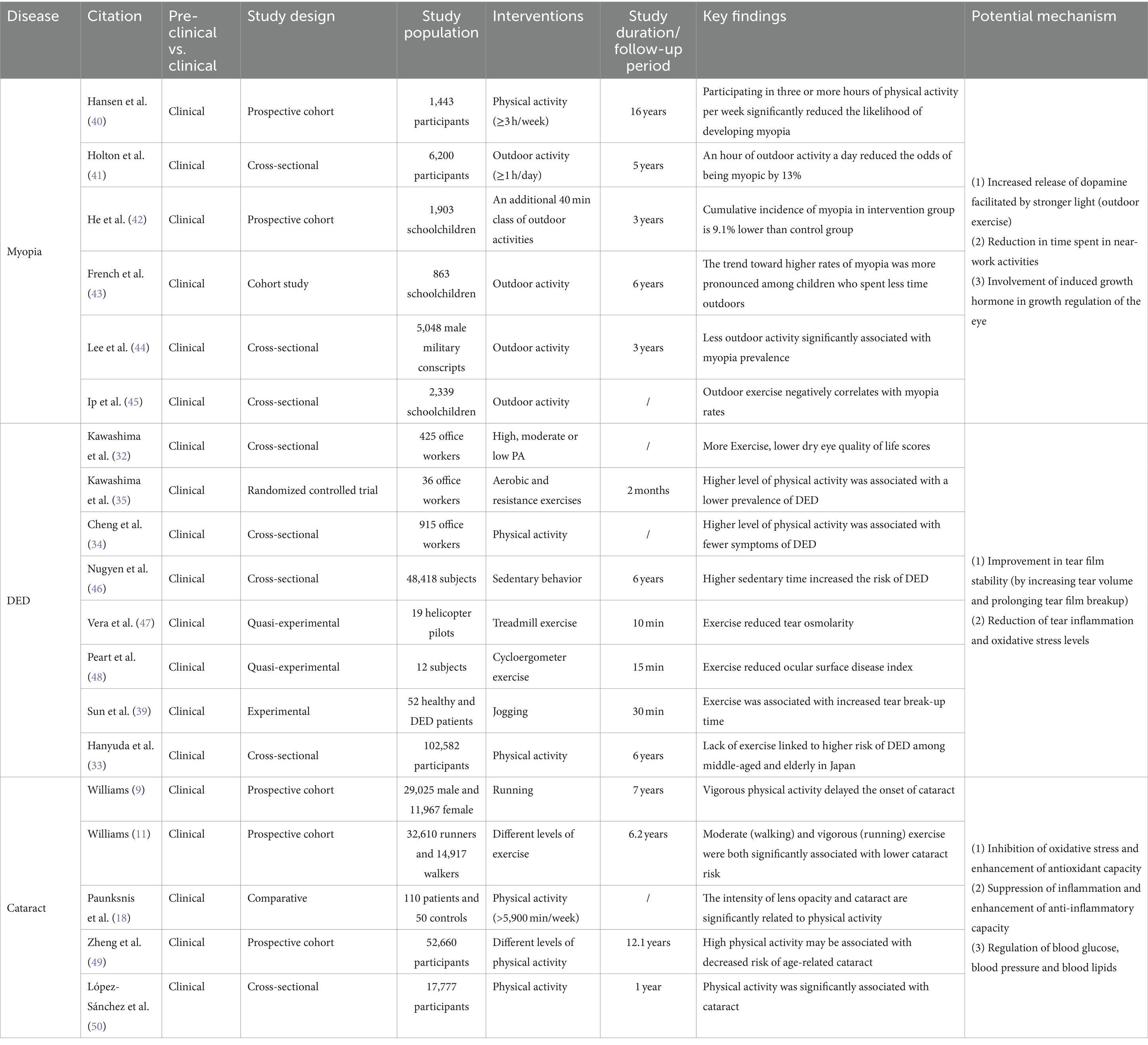
Table 1. Effects of exercise and physical activity on common eye diseases: insights into myopia, cataract and DED with potential mechanisms explored.
3.2 Myopia
3.2.1 Effect of exercise and physical activity on myopia
Myopia, or nearsightedness, is the prevalent refractive error that results in images being focused in front of the retina due to overstretching of the eye’s axis, thus making distant objects appear blurry (51). This condition is most prevalent during childhood and early adulthood (51). Myopia is a widespread ophthalmic disease globally, with an incidence of up to 80%–90% among young populations in certain regions of East and Southeast Asia (52). Its prevalence poses an escalating health issue worldwide. Any degree of myopia increases the likelihood of pathologic changes in ocular tissues, with a corresponding positive co-relation to the severity of myopia (53). High and pathological myopia substantially raise the likelihood of encountering vision-related complications such as glaucoma, retinal detachment, and macular degeneration (54). Therefore, preventing the onset of myopia and delaying its progression have been identified as crucial targets for public health interventions related to myopia (52).
Numerous studies have shown that physical activity positively affects the prevention of myopia and delays its progression (55–58). Those who engage in physical activity for over 3 h a week stand to reduce their chances of developing myopia by approximately 40% (40). One cohort study discovered a critical relevance between higher levels of physical activity and a reduction in the incidence of myopia, and that sedentary behavior increases the risk of myopia (59). Another longitudinal cohort study, based on a questionnaire, found that myopic students spend less time engaging in physical activity per day compared to nonmyopic students (60). Additionally, multiple studies have identified a substantial inverse relationship between time spent outdoors and the onset of myopia, with outdoor time being one of the primary protective factors against myopia (61, 62). A recent meta-analysis presented a notable nonlinear negative correlation between the time spent outside and the prevalence of myopia (63).
3.2.2 Potential mechanisms
The specific mechanisms by which exercise and physical activity prevent and protect against myopia have not yet been clearly established. Some studies have proposed potential mechanisms. Physical activity and exercise can cause the choroidal layer to dilate by enhancing blood flow, potentially hindering eye axis lengthening (64). Studies on animals suggest that eye growth is suppressed when blood flow and choroidal thickness increase (65, 66). Exercise and physical activity may also impact myopia in non-visual ways by influencing vascular function, blood pressure, peripheral and central growth factors, insulin resistance, oxidative stress and inflammation (60). Prior studies have shown that exercise-induced growth hormone and these systemic mechanisms are involved in regulating ocular growth (60). Additionally, studies have shown that engaging in outdoor exercise is more effective in preventing and controlling myopia compared to indoor exercise (62). This is due to the stronger light environment outdoors, which is considered to be a crucial factor in myopia protection (62). Research on animals has demonstrated that myopic susceptibility and dopamine-related genes and proteins can be affected by light intensity and peripheral defocus (67). Among these, dopamine has been found to hinder ocular axis growth, and its protective effects can be counteracted by the presence of its antagonist, spiperone (68). Environmentally influenced peripheral defocus might also contribute to the emergence or progression of myopia (69, 70). When engaging in outdoor sports, objects are typically positioned farther away from the eyes, and refractive conditions are uniform, thus reducing peripheral defocus and decelerating eye axis growth to prevent myopia (53) (see Table 1).
3.3 Cataract
3.3.1 Effect of exercise and physical activity on cataract
The lens is a transparent structure located at the front section of the eye that resembles a convex lens and possesses elastic properties. As one of the refractive media of the eye, the lens has the capacity to alter its shape due to its elasticity, enabling it to refract and focus light rays from varying distances onto the retina, consequently producing a sharp image (71). Cataract refers to the loss of clarity in the lens caused by clouding resulting from various factors (72). Globally, cataracts are a major contributor to visual impairment and blindness. Research indicates that this condition also increases the likelihood of depression and decreases life expectancy (73).
Long-term regular exercise and physical activity have demonstrated a favorable effect on the development and progression of cataract. Two prospective studies indicate that both moderate-intensity (walking) and high-intensity (running) exercise significantly reduce the risk of cataracts in men (9, 11) and women (9). Meanwhile, a case–control study identified an inverse relationship between lens cloudiness and the intensity of physical activity (18). Additionally, various detailed studies were conducted on different types, intensities, and durations of physical activity and exercise. The findings revealed a significant negative correlation between total physical activity and cataract incidence (49). Individuals who participated in rigorous physical labor experienced a 16% reduced risk of developing cataracts compared to those who led a sedentary lifestyle. Similarly, individuals who engaged in walking or biking for over 60 min daily recorded a 12% lowered prevalence of cataracts in comparison to those who rarely partook in such activities (49). Low-intensity physical activities, such as housework, did not seem to be associated with cataract risk (49). The evidence from these studies indicates that engaging in moderate to high intensity exercise and physical activity over a prolonged period can have a more clear-cut and recognized effect on reducing the risk of cataract.
3.3.2 Potential mechanisms
3.3.2.1 Enhancement of antioxidant defense in the lens
It is currently believed that reactive oxygen species (ROS) damage directly contributes to crystal clouding (72). Aging is an important driver of this process. In youth, antioxidants from the aqueous humor are sufficient to scavenge oxygen free radicals to prevent oxidative damage to the lens and, together with nutrients, maintain high metabolic activity of the lens, which is essential for normal clarity and refractive index (71). The levels of antioxidant enzymes, including dismutase (SOD) and glutathione peroxidase (GPX), in both the serum and lens notably decline with age (74). Furthermore, the level of reduced glutathione (GSH), which is the most critical antioxidant factor in the lens, decreases almost linearly and to an even greater extent (75, 76).
Research has indicated that consistent aerobic exercise aids in improving cellular capacity to fight against excessive build-up of ROS (77). Regular moderate-to high-intensity exercise increases the activity of endogenous antioxidant enzymes (SOD, GPX, and CAT), which improves the antioxidant capacity of the lens and slows down the aging process (78). However, this antioxidant effect does not appear to apply to all forms of exercise. For a single session of vigorous aerobic exercise, ROS and reactive nitrogen species are overproduced as a result of rapidly increased metabolism, which may instead induce oxidative stress and related injury (49). Therefore, finding the right form of exercise (regular and periodic exercise of moderate or higher intensity) is also crucial for improving lens turbidity.
3.3.2.2 Suppression of inflammation
Inflammation is an additional crucial risk factor for cataract. Inflammation is intimately related to oxidative stress, and both tend to occur concurrently in multiple pathological conditions, such as cataract (79). An 11 years cohort study found that elevated plasma C-reactive protein (CRP) levels were significantly associated with the risk of developing cataract (80). Aging is a major factor in age-related cataract, and chronic inflammation is one of the hallmarks of aging (81). With aging, circulating levels of pro-inflammatory factors such as IL-6, TNF-α, and CRP generally increase (82). Regular exercise has been found to reduce circulating levels of IL-6 and CRP, which has been associated with exercise-induced upregulation of the anti-inflammatory factors lipocalin and IL-10 (83, 84). In addition, after 10–12 weeks of appropriate exercise in sedentary elderly subjects, circulating levels of TLR4 and other pro-inflammatory biomarkers were found to be reduced to levels similar to those in young adults (19). These findings suggest that chronic inflammation during aging may be ameliorated or even reversed by appropriate exercise, thereby decreasing the likelihood of cataract development or delaying the progression of crystalline opacity.
Analogous to oxidative stress, the modulation of inflammation by exercise and physical activity is strongly connected to the type and intensity of exercise and the physical state of the exerciser. Different studies on resistance and aerobic exercise have found that both have inhibitory effects on chronic inflammation, but aerobic exercise seems to be better suited to modulate the immune system and markers of inflammation in older adults than resistance exercise (85, 86). In contrast, prolonged resistance exercise did not produce positive anti-inflammatory effects in frail older adults.
3.3.2.3 Amelioration of some systemic diseases
Various systemic diseases play a role in the onset and advancement of cataract. Men with hyperlipidemia (≥250 mg/dL) were discovered to have a heightened risk of developing cataract (87). Diabetic patients have a 5-fold increased risk of cataract compared to normal subjects (20). Case-control studies have identified a heightened risk of cataract in populations with hypertension, particularly in the case of posterior subcapsular cataract. Moreover, a linear relationship exists between blood pressure and the risk of cataract (88, 89).
The advantageous impacts of exercise and physical activity on regulating blood glucose, blood pressure, and blood lipid levels are well acknowledged. The American Diabetes Association (ADA) recommends adults with diabetes to participate in at least 150 min of moderate-intensity physical activity per week (90). A systematic review and meta-analysis suggests that a combination of moderate-to high-intensity aerobic exercise and resistance training followed by regular exercise (≥4 weeks) reduces blood pressure by approximately 2–5 mmHg in normotensive adults and 5–7 mmHg in hypertensive adults (91). Exercise and physical activity are now thought to improve hyperlipidemia by modulating plasma lipoprotein levels, and a randomized controlled clinical trial found a significant increase in plasma high density lipoprotein (HDL) (4.4 mg/dL) in the exercise group after 1 year of regular exercise (running at least 8 km per week) (92).
Similarly, the extent to which exercise improves systemic disease is strongly linked to the duration and intensity of exercise. In hyperlipidemia, for example, a significant correlation was found between weekly exercise distance and low-density lipoprotein cholesterol (r = −0.31), plasma HDL cholesterol (r = 0.48) and HDL2 (r = 0.41). In addition, regular exercise for at least up to 9 months was required to observe significant changes in lipid profiles (93, 94) (see Table 1).
3.4 Glaucoma
3.4.1 Effect of exercise and physical activity on glaucoma
Glaucoma is the primary cause of irreversible vision loss globally (95). As a classification of neurodegenerative diseases, is characterized by the gradual degeneration of retinal ganglion cells (RGCs) and axons (96). Degeneration of the optic nerve results in cupping, which is the distinctive appearance of the optic disc and accompanying loss of vision (97). Intraocular pressure (IOP) is the most significant, and the only controllable risk factor for glaucoma, so reducing IOP through medication or surgery is currently the mainstay of glaucoma treatment (98). However, some clinical studies have found that glaucoma patients continue to progress even when IOP is lowered (99).
In recent years, numerous studies have disclosed that modifiable environmental factors, such as exercise, smoking, alcohol consumption, and nutrition, possess a significant impact on the development of glaucoma (100). Long-term, regular exercise and physical activity have been demonstrated to reduce the incidence of glaucoma or slow its progression (8, 101, 102). A prospective study including 9,519 adults for up to 5 years discovered a significantly lower occurrence of glaucoma in those who abided by physical activity guidelines compared to those who did not engage in exercise (2.24% versus 1.14%) (8). A further retrospective analysis examined 24 patients with primary open-angle glaucoma (POAG) or pseudoexfoliation glaucoma and found that those who engaged in exercise habits (≥30 min per week) experienced less glaucomatous visual field deterioration compared to these non-exercising counterparts (10).
A considerable amount of research suggests that exercise and physical activity have the potential to prevent and mitigate various forms of damage to RGCs and optic nerves (103). A cross-sectional study with over 40,000 participants discovered that increased levels of physical activity led to an augmentation of the thickness in the plexiform layer within the retina (104). Experimental studies offer more unambiguous evidence. An experiment investigating age-related optic nerve injury revealed that regular aerobic exercise (swimming for 60 min per day, 5 days per week for 6 weeks) led to similar responses to acute ischemia-reperfusion-induced optic nerve injury in older mice (12 months) as compared to non-exercised young mice (3 months) (105). Additionally, exercise reduced injury-induced retinal neuroinflammation in aged mice. Another study on injury caused by optic nerve severance demonstrated that retinal ganglion cell survival significantly increased following a 4 weeks period of aerobic exercise, specifically running at 9 m/min for 30 min per day (106).
The effect of exercise and physical activity on glaucoma is intricately linked to the type and intensity of exercise undertaking. In a substantial 7.7 years cohort study comprising over 29,854 male runners belonging to running clubs located throughout the United States, an evident dose-dependent correlation was discovered between exercise patterns and glaucoma hazards, with swifter running speeds and longer running distances demonstrating a decreased risk of glaucoma (107). Another retrospective study involving 10,243 South Korean men aged 40 or older uncovered an inverse relationship between the development of glaucoma and engagement in medium-to-high intensity physical activity (108). Furthermore, a longitudinal study observing 141 patients with glaucoma discovered that the rate of visual field loss decreased with increased moderate to high activity levels (according to accelerometer measurements) (109). However, another study revealed that low and high-intensity exercise might elevate the risk of glaucoma in comparison to the moderate-intensity exercise recommended by the American College of Sports Medicine (ACSM) guidelines (110). The study also uncovered that undertaking high-intensity exercise daily was linked to a greater occurrence of glaucoma than exercising three times weekly. It is noteworthy that not all types of exercise and physical activity are advantageous for individuals with glaucoma. The study has demonstrated that engaging in vigorous isometric workouts such as wall push, suspension training, resistance band training, among others, as well as performing exercises in inverted body positions, and playing high-resistance wind instruments such as trumpet, trombone, French horn, and others have been shown to elevate IOP, thereby elevating the risk of developing glaucoma (111, 112).
It should be noted that there are multiple types of glaucoma, and exercise and physical activity may be considered a risk factor for certain types, such as pigmentary glaucoma. Pigmentation of corneal endothelial cells occurs in patients with pigment dispersion syndrome. When pigment particles enter the trabecular meshwork endothelial cells, they can gradually lead to pigment overload and death of the trabecular meshwork cells. This, in turn, causes trabecular collapse, obstruction of atrial aqueous outflow pathways, and increased intraocular pressure. Ultimately, this can lead to pigmentary glaucoma (113). Studies have shown that after aerobic exercise, patients with pigmented dispersion syndrome and pigmentary glaucoma experience a dispersion of pigments in the anterior chamber that is significantly increased (114, 115). A patient with pigment dispersion syndrome presented with typical symptoms of elevated IOP, blurred vision, and halos after strenuous exercise (116). However, after expanding the sample size, it was found that exercise did not significantly increase clinical IOP in patients with pigmentary glaucoma (117). Iris depressions have been suggested as a possible mechanism for pigment release (118). Patients with pigmentary glaucoma experience a significant increase in iris depressions following exercise (119). Research has demonstrated that exercise can increase the IOP pulse and affect atrial fluid dynamics, resulting in enhanced cyclic atrial fluid flow through the pupil. This, combined with reverse pupillary obstruction, can cause intermittent excessive iris depression, leading to an increase in pigment dispersion (119). Although current research does not suggest that all patients with pigmentary dispersion syndrome or pigmentary glaucoma should avoid all forms of exercise, highly stimulating exercise should be avoided as much as possible. It is necessary for these patients to carefully consider their condition and seek evaluation by a healthcare professional prior to exercising at a low or moderate intensity (114).
3.4.2 Potential mechanisms
3.4.2.1 Regulation of IOP
Excessive IOP can compress, deform, and alter the sieve plate on the inner eye wall, leading to mechanical axonal damage and transit disorders that impede the brainstem’s provision of trophic factors to RGCs (120). When IOP exceeds ocular perfusion pressure (OPP), blood supply to the optic nerve is restricted (121). The various factors listed above ultimately lead to the demise of RGCs and initiate the onset of glaucoma. Lowering IOP is a crucial factor in preventing and delaying the progression of glaucoma through exercise and physical activity (122). According to a randomized controlled study, a notable decrease in IOP was observed among healthy adults who engaged in 20 min of aerobic exercise with a return to baseline levels in approximately 1 h (123). Furthermore, post-exercise IOP levels decreased by 2.7 mmHg in a study of resistance training (124). Another prospective, interventional study found that aerobic exercise reduced the baseline level of IOP in subjects with glaucoma by 4.6 ± 0.4 mmHg after 3 months, and this reduction was maintained for 3 weeks (125). Therefore, examining the specific mechanisms by which exercise improves glaucoma requires an examination of how exercise regulates IOP. Maintaining normal intraocular pressure depends on a balanced interplay between the production of aqueous humor by the ciliary body and its drainage through the trabecular meshwork and uveoscleral outflow pathway (96). The present study suggests that acute exercise may alter IOP by, among other things, decreasing blood pH, increasing plasma osmolality, increasing blood lactate, decreasing norepinephrine concentration, increasing the nitric oxide/endothelin ratio (resulting in increased NO production and decreased endothelin content), stimulating β2 receptors, and altering ocular blood flow after exercise (122). Different types of exercise have been found to have varying effects on IOP regulation. Isometric exercise has primarily been associated with hyperventilation and hypocapnia, resulting in a decrease in IOP, while isotonic exercise has been primarily linked to an increase in colloid osmolality (126–128). However, research on the mechanisms by which long-term exercise and physical activity impact baseline levels of IOP is lacking, which suggests an avenue for future research.
3.4.2.2 Inhibition of oxidative stress and inflammation in the trabecular meshwork and retina
Oxidative stress, cellular senescence, and mitochondrial dysfunction increase in the aging retina and are significant risk factors for glaucoma (131). Oxidative stress and lipid peroxidation are principle initial contributors to the onset of para-inflammation in the retina (132). ROS activate retina-resident macrophages and neuroimmune cells, such as microglia and astrocytes, which release proinflammatory mediators and trigger neuroinflammation, ultimately accelerating the death of RGCs (132). Moreover, the trabecular meshwork serves as the “gateway” of aqueous humor outflow in the eye and is a highly vulnerable tissue to oxidative stress. This stress disrupts the normal drainage of aqueous fluids and ultimately leads to increased intraocular pressure and damage to the optic nerve (131). Research has shown that individuals with glaucoma exhibit elevated levels of oxidative stress markers, including 8-OH-dG and malondialdehyde, within their aqueous humor, trabecular meshwork, and serum (133, 134).
Prolonged aerobic exercise has been demonstrated to reduce oxidative stress and enhance antioxidant capacity in elderly individuals (135). An experimental study demonstrated a reduction in levels of oxidative stress within the retinas of mice after 12 weeks of regular bench running exercise (5–12 m/min, 30–60 min/day, 3 days/week) (130). This provides more direct evidence of the benefits of aerobic exercise. Previous studies have revealed that aerobic exercise reduces inflammatory factors in the bloodstream, spinal cord, cortex, and hippocampus, such as IL-1, IL-6, and TNF-α (136). At present, there is no direct evidence indicating that exercise ameliorates retinal inflammation, it has been found that exercise-induced myokine Irisin can inhibit oxygen-induced pathological angiogenesis, inflammation, and apoptosis in the retina in vivo (137).
3.4.2.3 Improvement of mitochondrial function
Mitochondria contribute to oxidative stress in living organisms. Mitochondrial dysfunction is a critical aspect of aging, characterized by changes in biogenesis, protein inhibition, mitochondrial autophagy, and kinetics (138, 139). The retina is among the most metabolically active tissues within the body and contains a substantial quantity of mitochondria. These mitochondria are primarily located in the myelin-free axons of RGCs and the inner segments of photoreceptors, fulfilling a crucial role in normal energy transportation (140). Mitochondrial dysfunction, potentially caused by various mechanisms such as dysregulation of signaling, dysfunction of pathways, genetic variations, and oxidative stress, is significantly associated with a majority of blinding eye conditions, including glaucoma (141, 142). The mitochondria in the RGCs are the first to be affected by glaucoma (143). Furthermore, the expression of OPA 1, a gene that is present in RGCs soma and axons and linked to both spontaneous and hereditary mitochondrial optic neuropathy, exhibits noteworthy down-regulation in patients identified with POAG, providing evidence of a genetic association connecting mitochondrial function and POAG (144). Mitochondrial abnormalities in morphology, reduced mass, decreased oxidative capacity, and abnormal autophagy, among other factors, can all contribute to glaucoma development (142).
Numerous studies indicate that aerobic exercise boosts the mitochondrial biosynthetic pathway and cellular respiratory capacity, leading to improved mitochondrial mass, structure, and function. Exercise improves an organism’s resilience against inhibitors of mitochondrial complex I, which is the initial enzyme-protein complex in the mitochondrial OXPHOS pathway. The downregulation of this complex may result in mitochondrial dysfunction. Physical activity also boosts the expression of mRNAs associated with mitochondrial complex I (145). Experimental studies have demonstrated that aerobic exercise can preserve mitochondrial metabolism by preventing age-related mitochondrial damage in skeletal muscle, achieved through inhibiting mitochondrial schizopyranin expression in a PGC-1α-dependent manner (146). Other studies have indicated that aerobic exercise enhances mitochondrial biogenesis and function by activating Nrf2 (139). Elevated mRNA levels of lysosome-associated membrane protein 2 (LAMP-2), Atg and LC3II in lateral femoral muscles was found in overweight older women after 6 months of weight loss and moderate-intensity exercise. Additionally, this study discovered that lifelong physical activity preserves LC3II and Atg at youthful levels (147). This indicates that physical activity enhances mitochondrial autophagy and consequently attenuates mitochondrial dysfunction. However, further validation is necessary to determine whether these particular mechanisms apply to the optic nerve injury model of glaucoma.
3.4.2.4 Facilitating the release of protective factors
3.4.2.4.1 BDNF
BDNF, a protein from the neurotrophic factor family, is produced by retinal neurons like RGCs, anaplastic synaptic cells, retinal neuroglia (Müller cells), astrocytes, and photoreceptors (148). It has been shown that BDNF protects RGCs’ survival in pathological conditions such as glaucoma and optic nerve injury (149). BDNF can enhance the retina’s resistance to injury by regulating the formation and maintenance of retinal neural circuits. Studies have shown that exercise can effectively prevent complement-mediated synapse loss (150). This helps to resist neurodegenerative changes in the structure, function, and molecules of the inner retina caused by glaucoma (151, 152). Currently, BDNF is widely recognized as a pivotal factor that mediates the impact of exercise on varying forms of retinal damage (153). Aerobic exercise, including long-term endurance training and short-term acute exercise, enhances circulating levels of BDNF in both healthy and chronically ill individuals. However, resistance training did not have such an effect (154). Experimental studies have found that damaged retinas of untrained mice had significantly decreased levels of BDNF, whereas retinal BDNF levels were significantly increased in trained mice (24). The primary mechanism of BDNF action relates to its binding to the TrkB receptor, which triggers the initiation of numerous signaling cascades. Activated TrkB enhances Erk1/2, which are responsible for retinal ganglion cell (RGC) survival, while concurrently inhibiting glycogen synthase kinase 3β (GSK3β) activity through enhancing PI3K/Akt signaling, thereby promoting neuronal axon growth and cell survival (152, 155). Inhibiting TrkB effectively impairs the ameliorative effects of exercise on retinal damage in disease models, including glaucoma and DR (150). In a mouse model of glaucoma, administration of BDNF resulted in increased survival of the RGC and improved retinal function (156).
Furthermore, insulin-like growth factor-1 (IGF-1) and neurotrophic growth factor (NGF) have also been validated for their neuroprotective effects in cases of retinal injury (103). Nonetheless, it is still unclear if they contribute to the protective effects of exercise, necessitating further comprehensive investigation.
3.4.2.4.2 Dopamine
Dopaminergic neurons are considered a crucial component of the motor system, with physical exercise and activity known to stimulate dopamine release (157, 158). Extensive animal studies have demonstrated that regular aerobic activities such as running and swimming lead to significant increases in serum and brain dopamine levels in mice or rats (158, 159). The dopaminergic system’s role in regulating the balance of aqueous humor dynamics, a key pathophysiological process in glaucoma, has been widely studied since the 1980s. Dopamine’s activity is mediated primarily through five G protein-coupled receptors belonging to two subfamilies: the D1-like receptors (D1R and D5R) and the D2-like receptors (D2R, D3R, and D4R) (160). Activation of D2R and D3R helps lower intraocular pressure (161–164). Many classic D2R agonists, including bromocriptine, cabergoline, lisuride, and lergotrile, have been used to reduce intraocular pressure in humans and animals (160). In 2000, a study on the selective D3R agonist 7-OH-DPAT revealed its potential role in reducing rabbit intraocular pressure, marking the first indication of D3R’s involvement in lowering eye pressure (163). Subsequent research on various D3R agonists and D3R−/− mice further confirmed this view (165, 166). The presence of D3R in the sympathetic nerve fibers of the ciliary body suggests that D3R activation could block the inflow of aqueous humor, thereby reducing intraocular pressure (162). A recent study by Reyes-Resina et al. (167) found that D3R expression in the ciliary body slices of glaucoma patients was significantly lower than that in controls, although the sample size of two was too small for statistical analysis, the findings undeniably further elucidate the connection between the dopaminergic system and glaucoma. While D2R and D3R have been detected in the human retina, there is a lack of research on abnormalities in the dopaminergic system within the retina of glaucoma patients. However, a study on a quail model of glaucoma found a reduction in dopaminergic cells and dopamine rhythms in the retina following glaucoma (168). In summary, physical exercise and activity may positively impact glaucoma by promoting dopamine release and its interaction with D2R and D3R. Nonetheless, this hypothesis requires further substantiation through more in-depth and direct evidence (see Table 2).
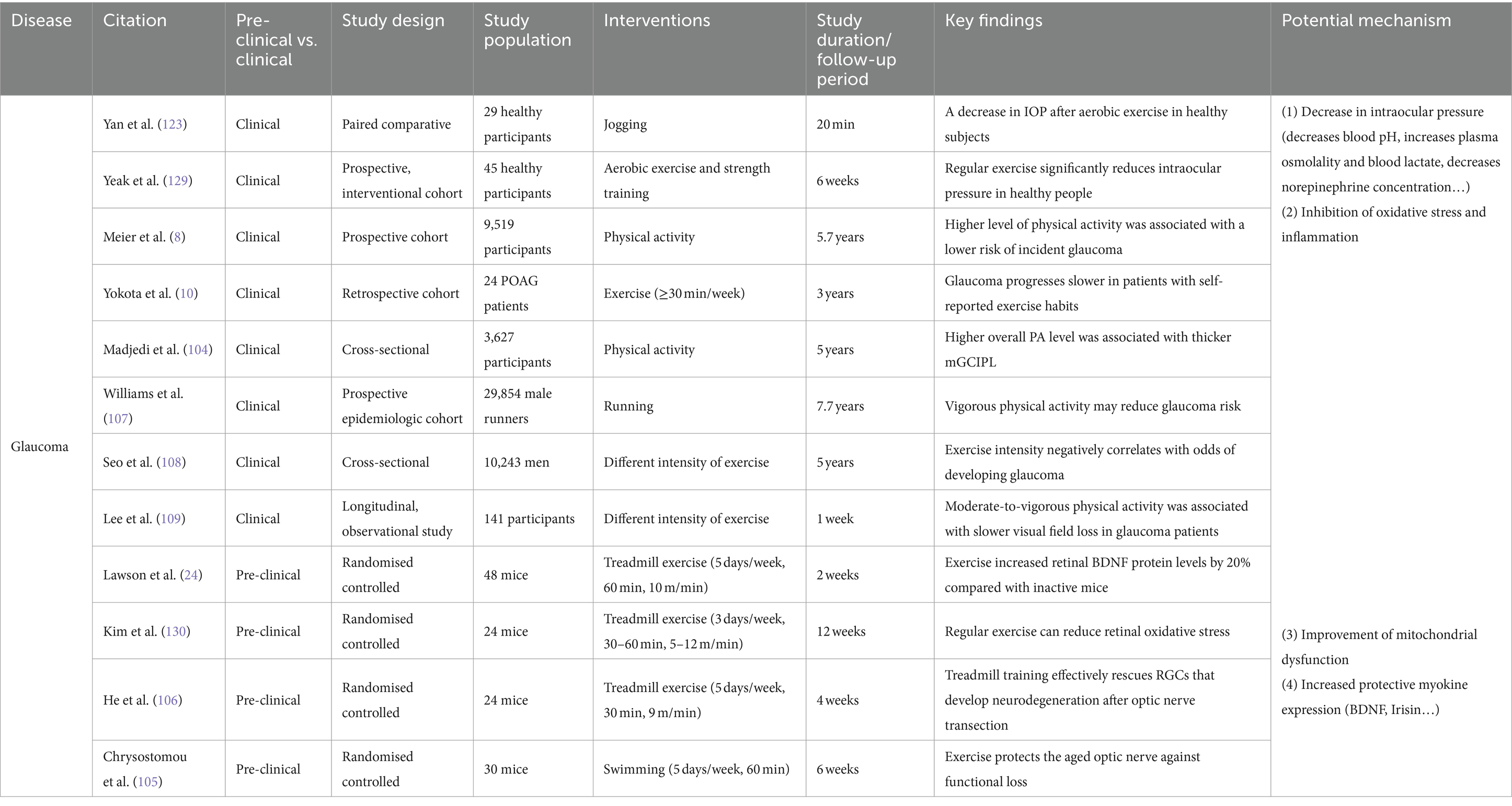
Table 2. Effects of exercise and physical activity on common eye diseases: insights into glaucoma with potential mechanisms explored.
3.5 DR
3.5.1 Effect of exercise and physical activity on DR
DR is the leading retinal vascular ailment that results in visual impairments like floaters, distorted vision, blurry vision, and in more severe cases, retinal detachment that can cause total or partial vision loss (169). DR can also affect the macula and lead to diabetic macular edema (DME). DME is featured by vascular leakage and results in swelling of the macula, which is the primary reason for blindness among diabetic patients (170).
Clinical studies have confirmed that regular exercise or physical activity and maintaining a healthy diet can significantly reduce the risk of developing diabetes and related ocular complications (171). A retrospective study of 3,031 adults demonstrated that individuals who consistently engaged in higher levels of physical activity over an extended period had a lower incidence of DR (172). Conversely, a sedentary lifestyle was found to significantly increase the likelihood of diabetic patients developing DR (173). Another pre-and post-clinical trial conducted on patients with DR indicated significant reductions in fasting blood glucose and central macular thickness in participants who underwent long-term moderate-intensity aerobic exercise regularly (45 min, three times per week for 12 weeks) (174). Basic research has provided further evidence that 8 weeks of treadmill exercise (8 m/min, 30 min per day, 6 days per week) yielded a significant reduction in retinal vascular endothelial growth factor (VEGF) expression and retinal cell apoptosis in diabetic mice models (175).
The intensity of exercise and physical activity is associated with their ameliorative effect on DR. Previous research suggests that the benefits of moderate-intensity aerobic exercise and physical activity for DR are greater than those of low-and high-intensity exercise (176). High-intensity interval training (HIIT) has been found to enhance choriocapillaris perfusion in healthy individuals. Nevertheless, clinical trial studies have not observed any ameliorative effects of HIIT on the microvasculature in patients with type 1 diabetes (T1D) (177, 178). At present, research on the influence of exercise and physical activity on DR primarily concentrates on the advantages of aerobic exercise. Additional studies are necessary to explore and showcase the impacts of other forms of exercise and physical activity on DR.
3.5.2 Potential mechanisms
3.5.2.1 Regulation of blood glucose
Briefly, DR is a microangiopathy with a complex pathophysiology and unclear pathogenesis (179). Various studies have established a correlation between hyperglycemia and retinal microvascular damage, indicating the former as a major and direct cause (180). Hyperglycemia leads to several pathophysiological alterations, such as oxidative stress, accumulation of sorbitol and advanced glycosylation end-products (AGEs), activation of protein kinase C (PKC), chronic neuroinflammation, and others (181). These pathways result in dysfunction of the vascular endothelium of the retina, marked by the loss of pericytes and endothelial cells, thinning of the basement membrane, increased retinal permeability, and the absence of neuroprotective molecules (182, 183). Retinal vascular endothelial dysfunction worsens retinal hypoxia, neuronal dysfunction, and leads to the development of various angiogenic factors such as VEGF, growth hormone-insulin-like growth factor (GH-IGF), and erythropoietin. Ultimately, this results in retinal neoangiogenesis, which is a typical feature of DR and a hallmark of its progressive development (182, 184).
The effectiveness of exercise and physical activity in improving blood glucose levels has been well-established through numerous studies. Different types of physical activity, like aerobic exercise, resistance training, and high-intensity interval exercise (HIIE), have been found to boost blood glucose levels in patients with type 2 diabetes by augmenting insulin sensitivity, promoting mitochondrial function, and adjusting metabolic parameters such as blood pressure and blood lipids (185–191). It has been found that combined exercise produces a more significant effect on blood glucose intervention than any single type of exercise alone (192, 193). A meta-analysis on resistance training demonstrated that high-intensity resistance training is more effective than low or moderate-intensity for reducing insulin levels and overall glycemic management (189). Furthermore, numerous studies indicate that engaging in exercise after a meal is more effective in regulating glycemic levels by reducing sudden spikes in blood glucose. The advantages are particularly evident for exercise sessions lasting 45 min or longer (188, 194).
3.5.2.2 Retinal neuroprotection
A rising number of studies have shown that pure microvascular injury cannot fully elucidate the pathogenesis of DR. Neuroretinal damage is found to be equally indispensable in DR’s development, even before microvascular injury (195). Diabetes mellitus influences the functioning and structure of all retinal cell types, and an increased expression of apoptotic markers of RGCs (caspase-3, Fas, and Bax) was noticed in diabetic patients’ retinas (196). Animal studies show that hyperglycemia speeds up retinal neuron apoptosis in mice, while also promoting glial cell activation and impaired metabolism (195). Additionally, a rat model of diabetes-induced early retinal neuropathy showed reduced BDNF levels, which further increased aberrant autophagy (197). BDNF upregulation has been suggested as a possible therapeutic avenue for DR, as it protects retinal cells by restoring proper autophagic responses in the retina (198).
As previously described, exercise and physical activity enhance the expression of multiple neurotrophic factors, including mainly BDNF, exhibiting greater levels, particularly in the retina and serum (24). BDNF has the potential to mitigate DR by promoting neuroretinal repair and survival caused by ischemia through the activation of TrkB and corresponding downstream pathways (199, 200).
3.5.2.3 Inhibition of hyperglycemia-induced oxidative stress and inflammation in retinal vessels
Oxidative stress is a significant contributor to the development of DR due to hyperglycemia leading to ROS overproduction (201). Oxidative stress causes damage to the cytoarchitecture and mitochondria, leading to apoptosis and depletion of pericytes (one of the major cell types of the retinal microvasculature, which controls endothelial cell proliferation and protects endothelial cells from lipid peroxide-induced damage), ultimately triggering or exacerbating the onset and progression of DR (202–204). Additionally, oxidative stress activates the inflammatory cascade. It has been found that hyperglycemia can lead to abnormal signaling pathways, among them phosphoinositide 3-kinase/Akt/protein kinase B and inducible NOS (iNOS). One specific manifestation is the inhibition of eNO synthase (eNOS) activation and the promotion of up-regulation of inflammatory mediators such as iNOS, IL-6, and TNF-α (205). The paracrine inflammatory response is activated, causing abnormal interactions between leukocytes and endothelial cells, ultimately resulting in retinal microvascular damage (132).
Exercise has been found to provide protection to retinal cells from damage caused by diabetes by inhibiting inflammatory and oxidative stress that are fundamental to the disease (22). For DR, Cheng et al. (175) reported that engaging in long-term regular platform running exercise could suppress vascular inflammation and oxidative stress in diabetic mice by upregulating miR-181b through activation of AMPK, further improving endothelial dysfunction in DR. Another study, based on a survey of 157 patients with retinopathy, found a negative correlation between moderate to high intensity physical activity and systemic inflammation (206).
3.5.2.4 Others
Chronic hyperglycemia-induced retinal microvascular pathological changes and structural abnormalities can result in hemodynamic abnormalities that could facilitate DR progression (183, 207). Studies have shown that exercise may improve retinal hemodynamics by promoting the expression of related adipokines, such as lipocalin, in the plasma and retina. Fundamental research has discovered that lipocalin impedes tube formation in human retinal microvascular endothelial cells. This suggests that lipocalin could be a potential treatment target for angiogenesis in DR (208). In a clinical study involving patients diagnosed with type 2 diabetes, researchers found that an increase in plasma lipocalin levels was positively associated with retinal vessel diameter, retinal blood velocity and flow, while being negatively associated with the total peripheral resistance of retinal arteries (209).
Furthermore, exercise and physical activity may improve DR by influencing 25-hydroxyvitamin D (25 OH-D) levels. A wealth of evidence supports the notion that enhanced physical activity leads to better 25 OH-D status in individuals of all ages, which is linked to microvascular events (210–212). It has been shown that the impact of physical activity on fasting blood sugar levels may be influenced by the genotype of the vitamin D receptor (213) (see Table 3).
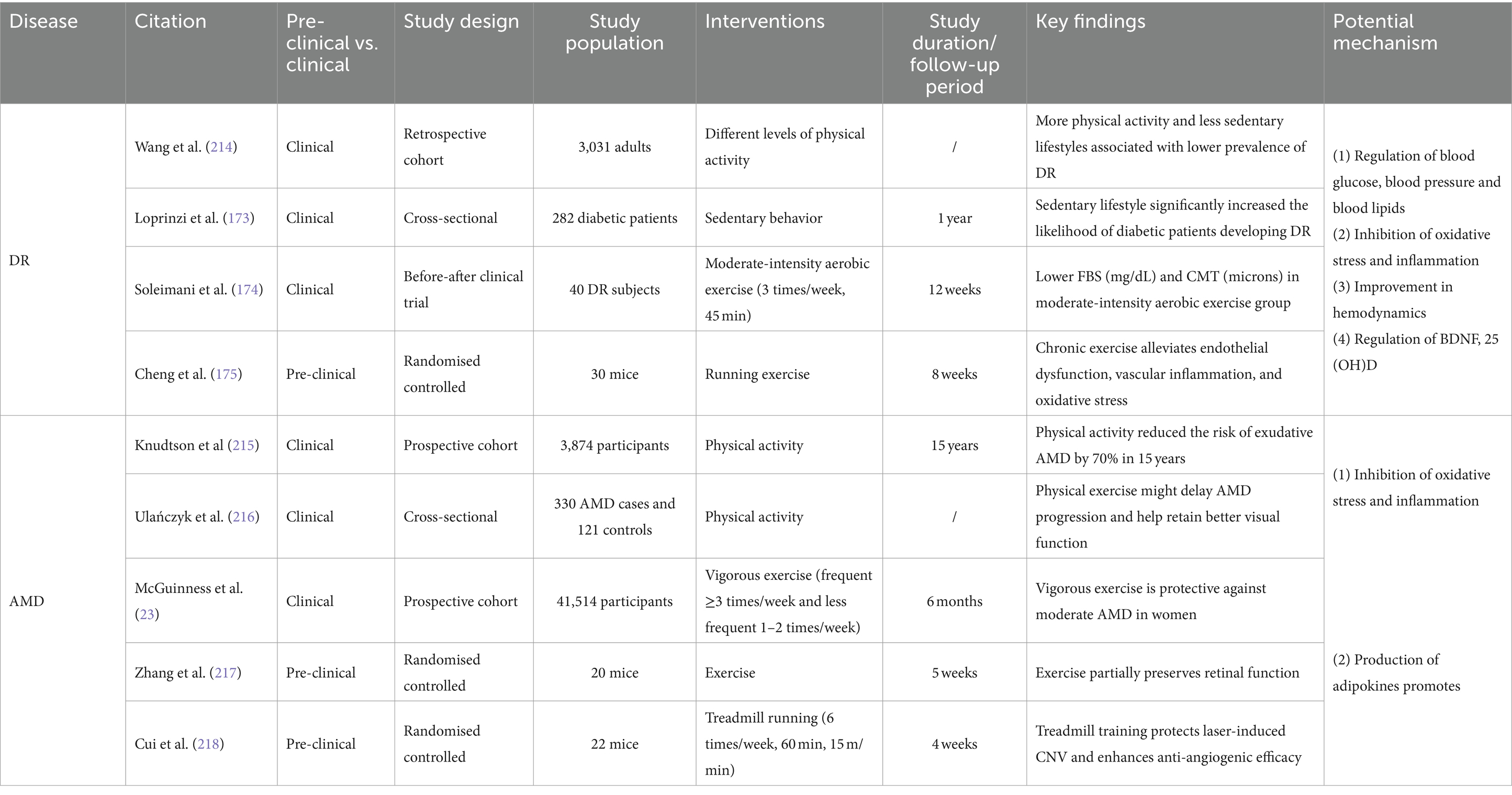
Table 3. Effects of exercise and physical activity on common eye diseases: insights into DR and AMD with potential mechanisms explored.
3.6 AMD
3.6.1 Effect of exercise and physical activity on AMD
The macula is situated in the posterior central portion of the retina, representing the most visually acute part of the retina. AMD is a degenerative condition of the macula caused by the malfunction of retinal pigment epithelium (RPE) and the death of photoreceptor cells (219). AMD is the primary cause of irreversible vision impairment in individuals aged 60 and above. Advanced AMD can drastically diminish visual acuity, impacting overall quality of life (220). Clinically, AMD has two subtypes: non-neovascular and neovascular (219). Between 80 to 85% of patients are diagnosed with non-neovascular AMD, characterized by the loss of photoreceptors and RPE cells, and exposure of the underlying choroidal vessels, generally leading to a better visual prognosis. Conversely, neovascular AMD, marked by the formation of new blood vessels beneath the RPE, within the retina, or under the retina, accounts for less patient numbers but is responsible for nearly 80% of severe vision loss associated with AMD (221, 222).
Age is considered the primary factor in AMD, while smoking is the top modifiable risk factor associated with AMD (219). However, several studies have also confirmed the correlation between exercise and AMD. A study spanning 15 years and including 3,874 adults indicated a marked reduction in the incidence of neovascular AMD among those who maintained an active lifestyle (exercising at least thrice weekly) over an extended period, compared to those who remained inactive. After adjusting for variables, the study discovered that incorporating more daily walks into one’s routine can reduce the risk of developing AMD (215). A sedentary lifestyle can exacerbate AMD progression, whereas patients who engage in regular exercise and physical activity tend to have better vision (216). Additionally, a systematic review and meta-analysis determined that physical activity is linked to a decreased incidence of both early and late-stage AMD (223). Animal studies indicate that aerobic exercise may safeguard photoreceptor cells and RPE from harm and hinder reduction in retinal and photoreceptor layer thickness in a mouse model of retinal degeneration (217).
3.6.2 Potential mechanisms
Various risk factors for AMD, such as age, smoking, diet, sun exposure, and alcohol consumption, can induce or exacerbate a common pathophysiologic process known as oxidative stress at the molecular level (224). The macula ensures high metabolic activity to safeguard the large amount of energy required for light sensing and visual signal processing by the cone cells. This finding suggests that the macula is exposed to high levels of ROS, making it more susceptible to damage from oxidative stress (224). In atrophic AMD, accumulated ROS directly damage the cell membranes, DNA, and proteins of RPE cells and photoreceptor cells, leading to structural and functional impairments. Oxidative damage also results in the accumulation of cellular metabolic byproducts like lipofuscin within the RPE cells, further exacerbating cellular dysfunction and death by disrupting autophagy and affecting normal metabolism (222, 225). VEGF-mediated abnormal vascular generation is a key factor inducing CNV in late-stage neovascular AMD. The NF-κB pathway, activated under oxidative stress, translocates to the nucleus, directly enhancing VEGF gene transcription and stimulating neovascularization (226, 227). Signaling pathways such as MAPK and PI3K/Akt are also involved in the regulation of VEGF by oxidative stress (228, 229). ROS clearance is a popular research topic in AMD treatment. Studies demonstrate that engaging in regular exercise and physical activity can boost the activity of antioxidant enzymes, halt neovascularization (230), and increase resistance to oxidative stress (23).
Inflammation plays a significant role in the pathophysiology of AMD. Research has shown that the AMD pathology is accompanied by the infiltration of various inflammatory cells, primarily microglia and macrophages. Inflammatory responses, mediated by the release of cytokines such as TNF-α and Interleukins, activate signaling pathways including NF-κB and MAPK. This process not only damages RPE and photoreceptor cells but also enhances the transcription and translation of the VEGF gene, inducing retinal neovascularization (231). Laser-induced choroidal neovascularization in CNV mice was restrained by experimental depletion of macrophages (232). Studies indicate that exercise and physical activity play a significant role in inhibiting the inflammatory response through down-regulating the expression of inflammatory factors and adhesion molecules, promoting the release of the anti-inflammatory adipokine lipocalin, decreasing macrophage activation and aggregation, and inhibiting microglial cell expression (218, 233) (see Table 3).
4 Conclusion and perspectives
This review synthesizes the current knowledge on the impact and potential mechanisms of exercise and physical activity on common ocular diseases such as DED, cataracts, myopia, glaucoma, DR, and AMD, as depicted in Figures 2–6. It provides a relatively comprehensive elucidation of the relationship between physical activities and eye health. According to existing research, it can be inferred that for individuals suffering from DED, myopia, cataracts, glaucoma, DR, and AMD, or those at high risk, when physical activity is appropriately tailored to their conditions, long-term, regular exercise of moderate to vigorous intensity can help delay the onset and progression of these diseases or alleviate their symptoms. This offers a promising avenue for the prevention and treatment strategies of a variety of ocular diseases. Aligning with the Global recommendations on physical activity for health and United States Health and Human Services physical activity guidelines for adults (25, 26), combined with the findings of this review, we propose that for early to mid-stage patients or at-risk groups of these ocular diseases, long-term adherence to moderate-intensity physical activities (≥150 min per week) like running, walking, and cycling in suitable environments (considering temperature, humidity, light intensity, etc.) could be instrumental in preventing or delaying the progression of these common eye conditions.
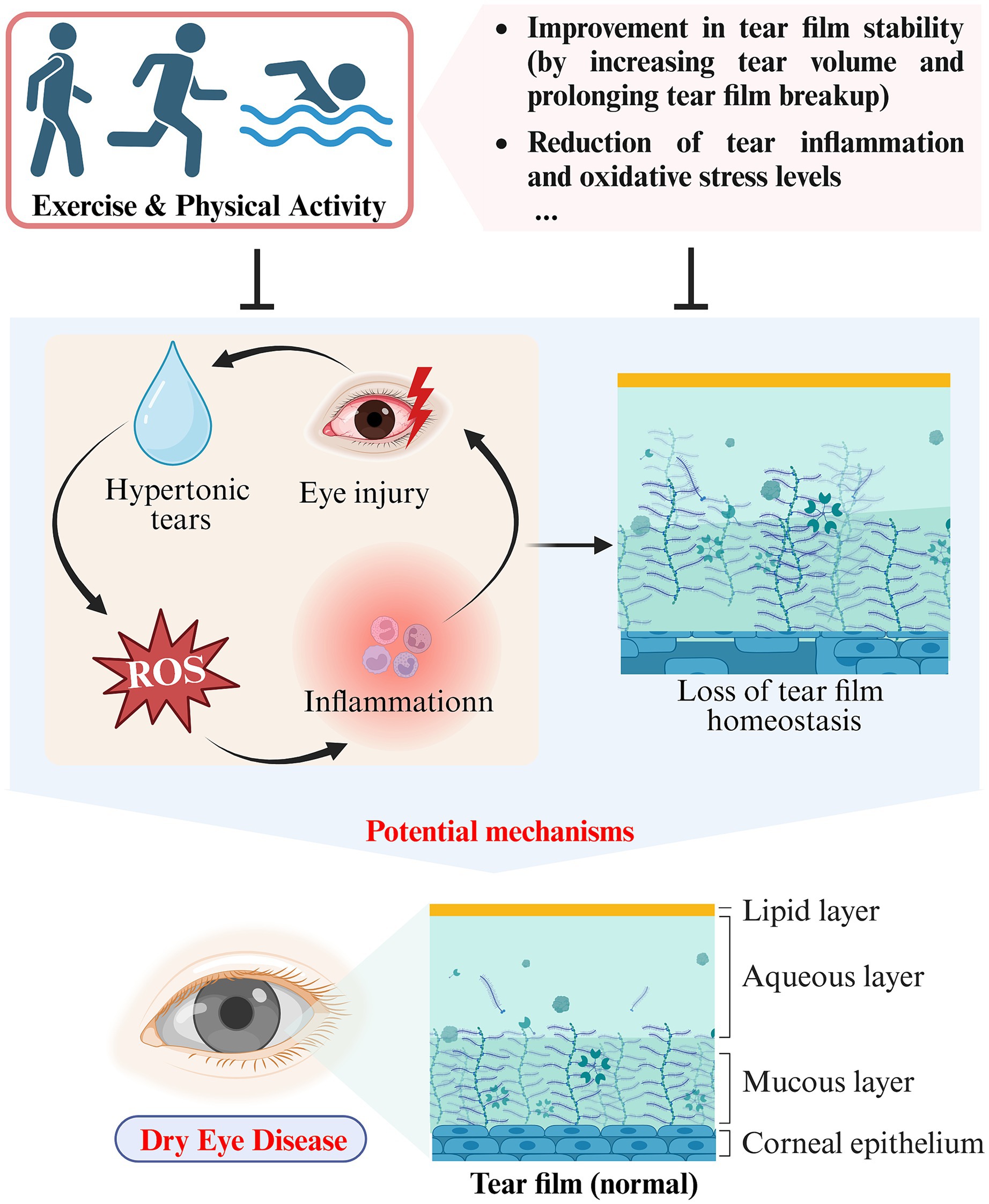
Figure 2. Schematic representation of the impact and potential mechanisms of exercise and physical activity on DED (created with Biorender.com).
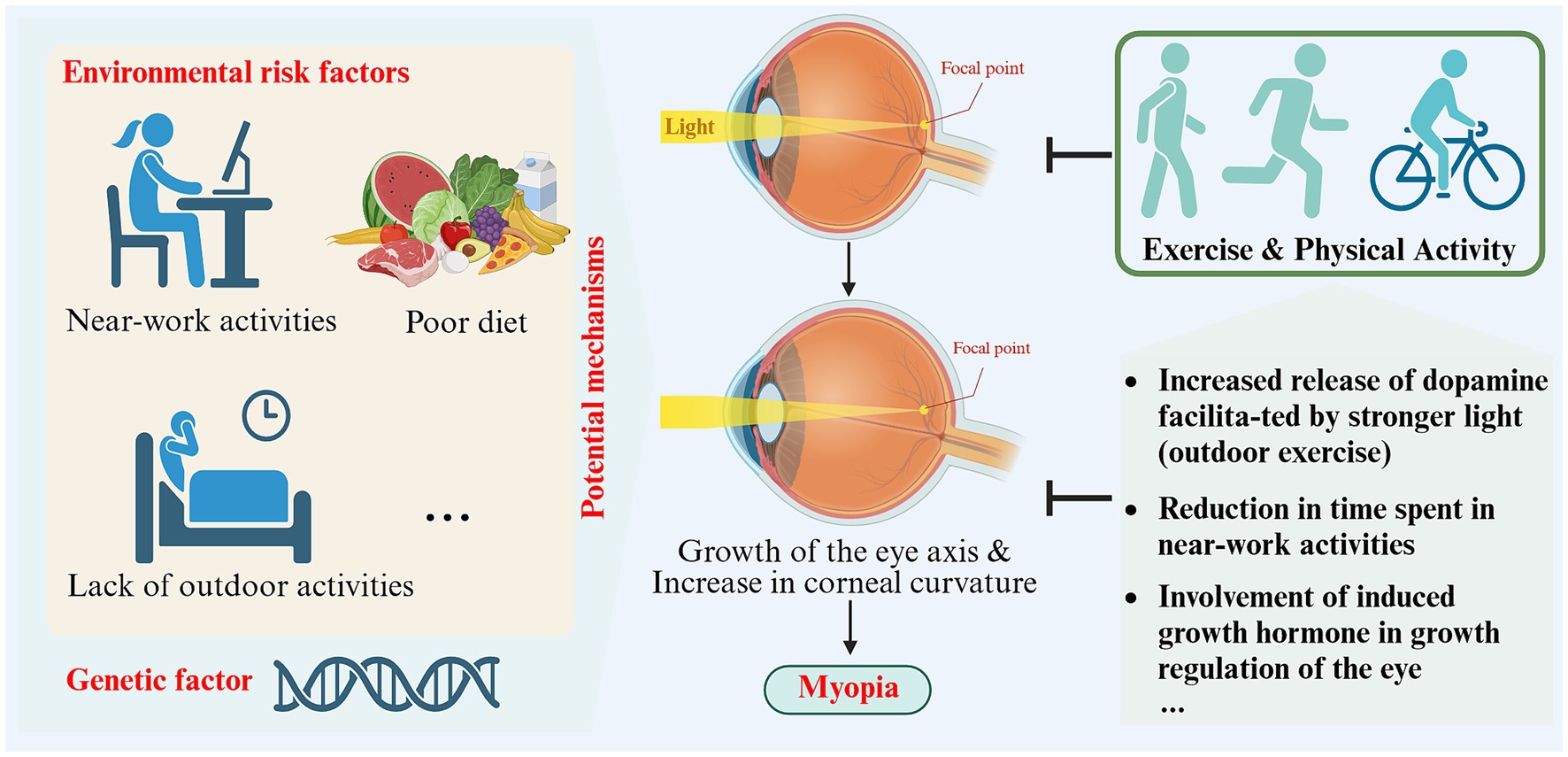
Figure 3. Schematic representation of the impact and potential mechanisms of exercise and physical activity on myopia (created with Biorender.com).
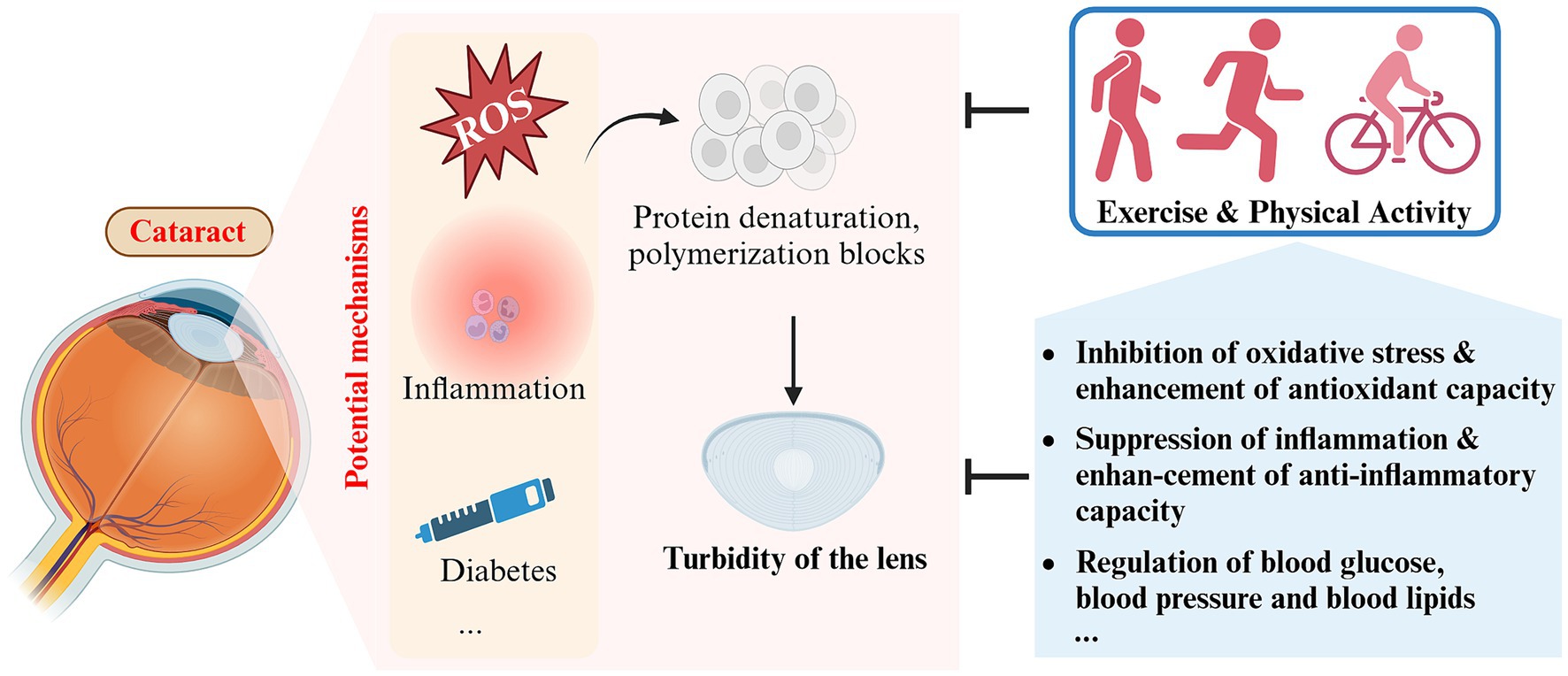
Figure 4. Schematic representation of the impact and potential mechanisms of exercise and physical activity on cataract (created with Biorender.com).
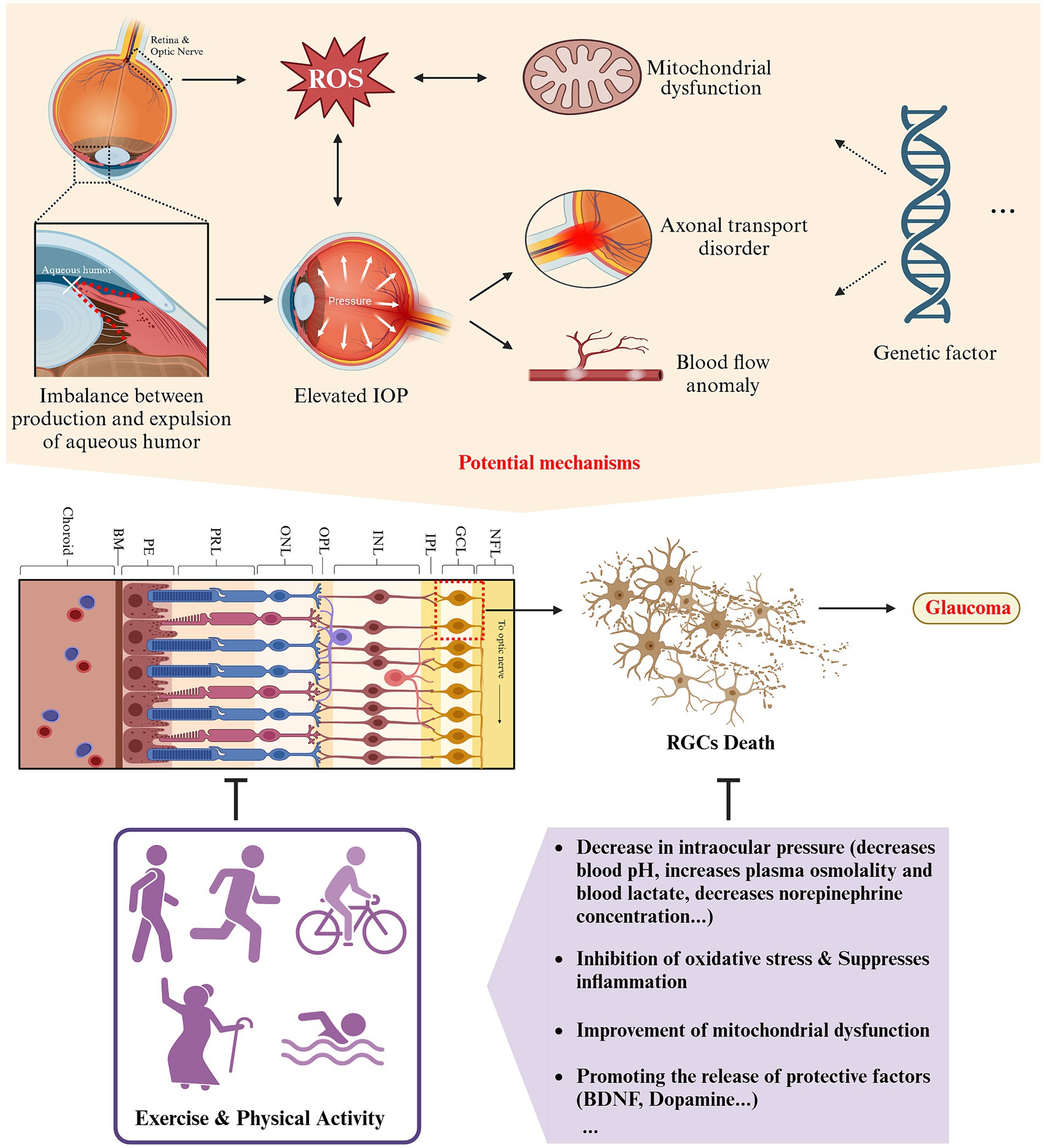
Figure 5. Schematic representation of the impact and potential mechanisms of exercise and physical activity on glaucoma (created with Biorender.com).
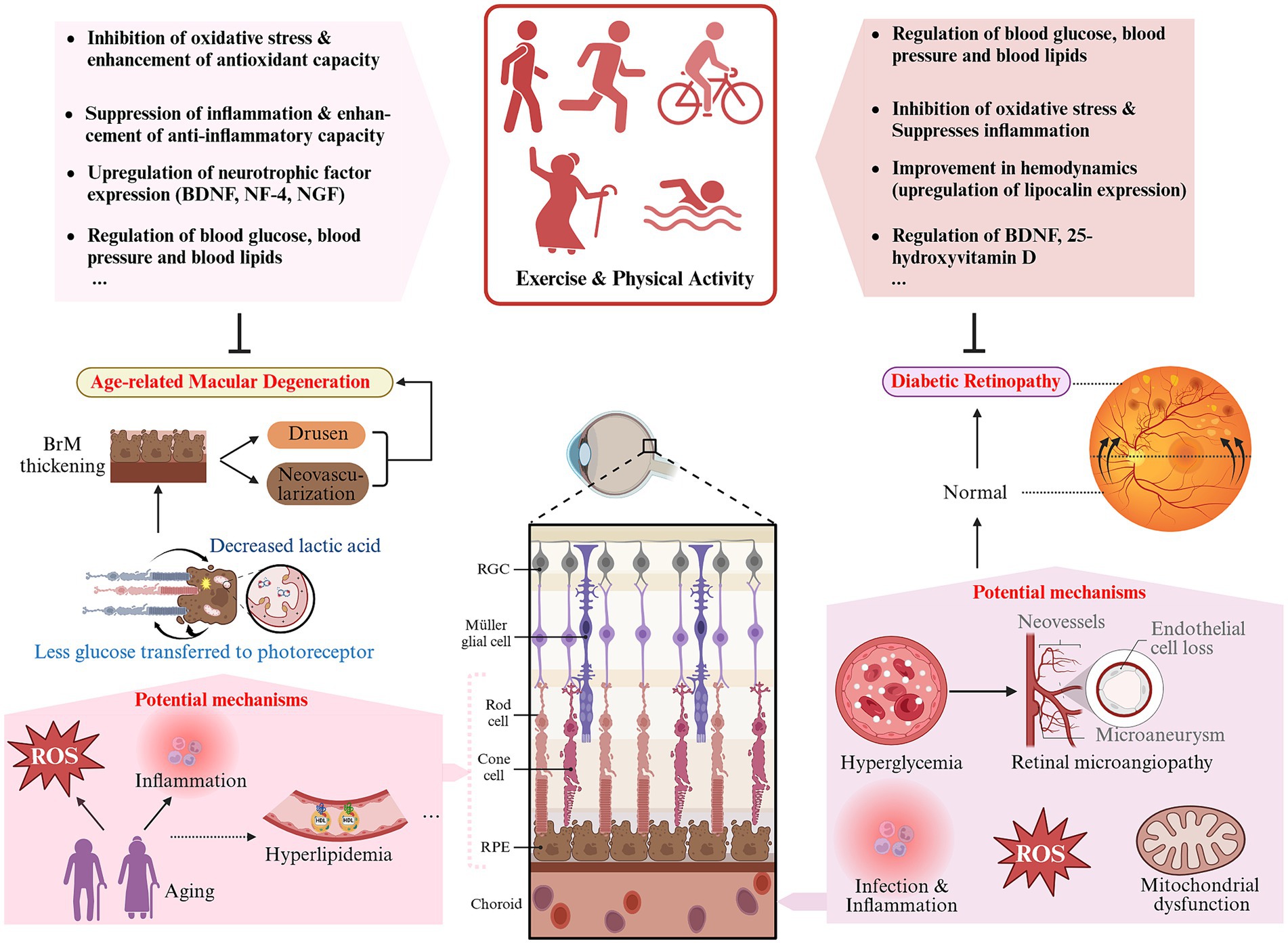
Figure 6. Schematic representation of the impact and potential mechanisms of exercise and physical activity on DR and AMD (created with Biorender.com).
The impact of exercise and physical activity on other eye disorders has also been thoroughly researched. For instance, studies on retinitis pigmentosa (RP) have discovered that patients maintaining higher levels of physical activity exhibit superior overall visual function, color vision, and peripheral vision compared to their less active counterparts, despite the limited sample size and reliance on subjective questionnaire surveys. These findings necessitate further validation in future research (234, 235). Additionally, the benefits of exercise and physical activity on retinal microvasculature, including reductions in blood pressure and glucose levels, decreased plasma viscosity, and enhanced antioxidative capacity of red blood cells (236–239), suggest potential improvements in the development and progression of retinal vein occlusion (RVO), a condition significantly influenced by high blood sugar, hypertension, arteriolar narrowing, or occlusions (240). Moreover, the positive effects of exercise and physical activity on autoimmune diseases, such as rheumatoid arthritis, may extend to uveitis, a common extra-articular manifestation of rheumatoid arthritis (4, 241, 242). In the regulatory mechanisms, a novel myokine, irisin, induced by exercise and secreted by skeletal muscles, has been shown to be downregulated in the aqueous humor of patients with RVO and AMD, as well as in the retinas of mice with oxygen-induced retinopathy, suggesting it may be a marker of retinal damage (137, 243). Current research indicates that irisin can exert anti-inflammatory, antioxidative, and anti-apoptotic neuroprotective effects through several signaling pathways, including Akt and ERK1/2 (244), integrin αVβ5/AMPK (245), and ROS-NLRP3 (246), among others. Further investigation into the impact of this molecule on ocular diseases may enrich the understanding of exercise’s protective mechanisms and potentially offer a new therapeutic strategy for neuroprotection of the visual system.
It’s noteworthy that in epidemiological studies included in this review, the form and intensity of exercise were adapted to the subjects. Under this premise, the positive impact of exercise or physical activity on dry eye syndrome, cataracts, glaucoma, diabetic retinopathy, and other ocular diseases has been validated. However, it is undeniable that in certain specific situations, exercise and physical activity should be reduced or even temporarily prohibited. These include: (1) late stages or acute episodes of vision-impacting ocular diseases, such as cataracts, glaucoma, diabetic retinopathy, where the patient’s vision and/or visual field is severely compromised, making the positive impact of exercise and physical activity almost negligible and potentially exacerbating the condition or increasing the risk of accidental injury (111, 247, 248); (2) the recovery period following ocular surgery (e.g., cataract surgery, vitrectomy, laser treatment), where vigorous exercise should be avoided to ensure proper healing of the wound (249, 250); (3) environments that are dry, windy, or dusty may exacerbate symptoms of dry eye syndrome, and intense sunlight or dim lighting may worsen eye pressure issues in glaucoma patients (251–253); (4) patients with high myopia are at increased risk of retinal detachment and should avoid high-impact and vibration-inducing activities, such as running and lifting heavy objects (254); (5) when specific complications are present, such as diabetic retinopathy patients with concurrent macular edema, short-term high-intensity exercise should be avoided (255).
This review aims to summarize the positive effects of exercise and physical activity on common ocular diseases, but this does not imply that exercise and physical activity can directly serve as a treatment modality. Seeking medical assistance and clinical treatment is necessary and primary for any ocular disease. We hope this review serves as a catalyst to stimulate interest among researchers and medical professionals in further exploring the relationship between exercise and eye health. We look forward to future studies revealing the specific mechanisms and key targets through which exercise benefits ocular health, thereby facilitating the development of innovative strategies for treating eye diseases.
This review also has limitations. Different studies may involve participants of varying ages, genders, races, etc., and employ different forms of exercise and physical activity, leading to variability in type, intensity, frequency, and duration. The classification of exercise intensity in this review utilizes metabolic equivalents (METs), a widely accepted standard. However, it is not the sole method available; other measures such as maximum oxygen uptake (VO2 Max), heart rate, and heart rate reserve also have their advantages. Relying on a single measurement standard could potentially affect our interpretation and summary of the outcomes. Additionally, long-term follow-up studies included in the review may be subject to response bias, such as self-reported exercise and physical activity data potentially being influenced by respondents’ subjective biases. There are also certain limitations and gaps in research on different types of eye diseases. For example, some studies may not have accounted for confounding factors, such as genetic predispositions, environmental factors, and behavioral factors (e.g., near work and screen time usage), making it difficult to ascertain the impact of exercise on dry eye syndrome and myopia. In the case of diseases like AMD, existing research lacks unified recommendations for specific types of exercise, intensity, frequency, and duration, making it challenging to provide optimal exercise guidelines.
Author contributions
QZ: Writing – original draft, Writing – review & editing. YJ: Writing – original draft, Writing – review & editing. CD: Writing – original draft, Writing – review & editing. JW: Writing – original draft, Writing – review & editing.
Funding
The author(s) declare financial support was received for the research, authorship, and/or publication of this article. This research was funded by the grants from National Natural Science Foundation of China grant numbers (82101125, 81974133, and 82271089).
Conflict of interest
The authors declare that the research was conducted in the absence of any commercial or financial relationships that could be construed as a potential conflict of interest.
Publisher’s note
All claims expressed in this article are solely those of the authors and do not necessarily represent those of their affiliated organizations, or those of the publisher, the editors and the reviewers. Any product that may be evaluated in this article, or claim that may be made by its manufacturer, is not guaranteed or endorsed by the publisher.
References
1. Burton, MJ, Ramke, J, Marques, AP, Bourne, RRA, Congdon, N, Jones, I, et al. The Lancet Global Health Commission on global eye health: vision beyond 2020. Lancet Glob Health. (2021) 9:e489–551. doi: 10.1016/s2214-109x(20)30488-5
2. Assi, L, Chamseddine, F, Ibrahim, P, Sabbagh, H, Rosman, L, Congdon, N, et al. A global assessment of eye health and quality of life: a systematic review of systematic reviews. JAMA Ophthalmol. (2021) 139:526–41. doi: 10.1001/jamaophthalmol.2021.0146
3. Piercy, KL, Troiano, RP, Ballard, RM, Carlson, SA, Fulton, JE, Galuska, DA, et al. The physical activity guidelines for Americans. JAMA. (2018) 320:2020–8. doi: 10.1001/jama.2018.14854
4. Pedersen, BK, and Saltin, B. Exercise as medicine—evidence for prescribing exercise as therapy in 26 different chronic diseases. Scand J Med Sci Sports. (2015) 25:1–72. doi: 10.1111/sms.12581
5. Blair, SN, Kohl, HW 3rd, Paffenbarger, RS Jr, Clark, DG, Cooper, KH, and Gibbons, LW. Physical fitness and all-cause mortality. A prospective study of healthy men and women. JAMA. (1989) 262:2395–401. doi: 10.1001/jama.262.17.2395
6. Blair, SN. Physical inactivity: the biggest public health problem of the 21st century. Br J Sports Med. (2009) 43:1–2.
7. Barker, K, and Eickmeyer, S. Therapeutic exercise. Med Clin North Am. (2020) 104:189–98. doi: 10.1016/j.mcna.2019.10.003
8. Meier, NF, Lee, DC, Sui, X, and Blair, SN. Physical activity, cardiorespiratory fitness, and incident glaucoma. Med Sci Sports Exerc. (2018) 50:2253–8. doi: 10.1249/mss.0000000000001692
9. Williams, PT. Prospective epidemiological cohort study of reduced risk for incident cataract with vigorous physical activity and cardiorespiratory fitness during a 7-year follow-up. Invest Ophthalmol Vis Sci. (2009) 50:95–100. doi: 10.1167/iovs.08-1797
10. Yokota, S, Takihara, Y, Kimura, K, Takamura, Y, and Inatani, M. The relationship between self-reported habitual exercise and visual field defect progression: a retrospective cohort study. BMC Ophthalmol. (2016) 16:147. doi: 10.1186/s12886-016-0326-x
11. Williams, PT. Walking and running are associated with similar reductions in cataract risk. Med Sci Sports Exerc. (2013) 45:1089–96. doi: 10.1249/MSS.0b013e31828121d0
12. Mann, S, Beedie, C, and Jimenez, A. Differential effects of aerobic exercise, resistance training and combined exercise modalities on cholesterol and the lipid profile: review, synthesis and recommendations. Sports Med. (2014) 44:211–21. doi: 10.1007/s40279-013-0110-5
13. Zhao, JL, Jiang, WT, Wang, X, Cai, ZD, Liu, ZH, and Liu, GR. Exercise, brain plasticity, and depression. CNS Neurosci Ther. (2020) 26:885–95. doi: 10.1111/cns.13385
14. Ainsworth, BE, Haskell, WL, Herrmann, SD, Meckes, N, Bassett, DR Jr, Tudor-Locke, C, et al. 2011 compendium of physical activities: a second update of codes and MET values. Med Sci Sports Exerc. (2011) 43:1575–81. doi: 10.1249/MSS.0b013e31821ece12
15. Haskell, WL, Lee, IM, Pate, RR, Powell, KE, Blair, SN, Franklin, BA, et al. Physical activity and public health: updated recommendation for adults from the American College of Sports Medicine and the American Heart Association. Med Sci Sports Exerc. (2007) 39:1423–34. doi: 10.1249/mss.0b013e3180616b27
16. Abokyi, S, Mensah, SN, Otchere, H, Akoto, YO, and Ntodie, M. Differential effect of maximal incremental treadmill exercise on tear secretion and tear film stability in athletes and non-athletes. Exp Eye Res. (2022) 214:108865. doi: 10.1016/j.exer.2021.108865
17. Ko, YC, Liu, CJ, Lau, LI, Wu, CW, Chou, JC, and Hsu, WM. Factors related to corneal endothelial damage after phacoemulsification in eyes with occludable angles. J Cataract Refract Surg. (2008) 34:46–51. doi: 10.1016/j.jcrs.2007.07.057
18. Paunksnis, A, Kusleika, S, and Kusleikaite, M. The relationship of the intensity of lens opacity with physical activity. Medicina. (2006) 42:738–43.
19. Stewart, LK, Flynn, MG, Campbell, WW, Craig, BA, Robinson, JP, McFarlin, BK, et al. Influence of exercise training and age on CD14+ cell-surface expression of toll-like receptor 2 and 4. Brain Behav Immun. (2005) 19:389–97. doi: 10.1016/j.bbi.2005.04.003
20. Ang, MJ, and Afshari, NA. Cataract and systemic disease: a review. Clin Experiment Ophthalmol. (2021) 49:118–27. doi: 10.1111/ceo.13892
21. Anuradha, S, Healy, GN, Dunstan, DW, Klein, R, Klein, BE, Cotch, MF, et al. Physical activity, television viewing time, and retinal microvascular caliber: the multi-ethnic study of atherosclerosis. Am J Epidemiol. (2011) 173:518–25. doi: 10.1093/aje/kwq412
22. Dharmastuti, DP, Agni, AN, Widyaputri, F, Pawiroranu, S, Sofro, ZM, Wardhana, FS, et al. Associations of physical activity and sedentary behaviour with vision-threatening diabetic retinopathy in Indonesian population with type 2 diabetes mellitus: Jogjakarta eye diabetic study in the community (JOGED.COM). Ophthalmic Epidemiol. (2018) 25:113–9. doi: 10.1080/09286586.2017.1367410
23. McGuinness, MB, Karahalios, A, Simpson, JA, Guymer, RH, Robman, LD, Hodge, AM, et al. Past physical activity and age-related macular degeneration: the Melbourne collaborative cohort study. Br J Ophthalmol. (2016) 100:1353–8. doi: 10.1136/bjophthalmol-2015-307663
24. Lawson, EC, Han, MK, Sellers, JT, Chrenek, MA, Hanif, A, Gogniat, MA, et al. Aerobic exercise protects retinal function and structure from light-induced retinal degeneration. J Neurosci. (2014) 34:2406–12. doi: 10.1523/jneurosci.2062-13.2014
25. Haskell, WL, Lee, IM, Pate, RR, Powell, KE, Blair, SN, Franklin, BA, et al. Physical activity and public health: updated recommendation for adults from the American College of Sports Medicine and the American Heart Association. Circulation. (2007) 116:1081–93. doi: 10.1161/circulationaha.107.185649
26. WHO Guidelines Approved by the Guidelines Review Committee. Global recommendations on physical activity for health. Geneva: World Health Organization (2010).
27. Rouen, PA, and White, ML. Dry eye disease: prevalence, assessment, and management. Home Healthc Now. (2018) 36:74–83. doi: 10.1097/nhh.0000000000000652
28. Thulasi, P, and Djalilian, AR. Update in current diagnostics and therapeutics of dry eye disease. Ophthalmology. (2017) 124:S27–s33. doi: 10.1016/j.ophtha.2017.07.022
29. Stapleton, F, Alves, M, Bunya, VY, Jalbert, I, Lekhanont, K, Malet, F, et al. TFOS DEWS II epidemiology report. Ocul Surf. (2017) 15:334–65. doi: 10.1016/j.jtos.2017.05.003
30. Ayaki, M, and Negishi, K. Decrease of tear break-up time at Japanese eye clinics during five consecutive years. Sci Rep. (2022) 12:6848. doi: 10.1038/s41598-022-11035-9
31. Kojima, T, Dogru, M, Kawashima, M, Nakamura, S, and Tsubota, K. Advances in the diagnosis and treatment of dry eye. Prog Retin Eye Res. (2020) 78:100842. doi: 10.1016/j.preteyeres.2020.100842
32. Kawashima, M, Uchino, M, Yokoi, N, Uchino, Y, Dogru, M, Komuro, A, et al. The association between dry eye disease and physical activity as well as sedentary behavior: results from the Osaka study. J Ophthalmol. (2014) 2014:943786. doi: 10.1155/2014/943786
33. Hanyuda, A, Sawada, N, Uchino, M, Kawashima, M, Yuki, K, Tsubota, K, et al. Physical inactivity, prolonged sedentary behaviors, and use of visual display terminals as potential risk factors for dry eye disease: JPHC-NEXT study. Ocul Surf. (2020) 18:56–63. doi: 10.1016/j.jtos.2019.09.007
34. Cheng, X, Song, M, Kong, J, Fang, X, Ji, Y, Zhang, M, et al. Influence of prolonged visual display terminal use and exercise on physical and mental conditions of internet staff in Hangzhou, China. Int J Environ Res Public Health. (2019) 16:1829. doi: 10.3390/ijerph16101829
35. Kawashima, M, Sano, K, Takechi, S, and Tsubota, K. Impact of lifestyle intervention on dry eye disease in office workers: a randomized controlled trial. J Occup Health. (2018) 60:281–8. doi: 10.1539/joh.2017-0191-OA
36. Zemanová, M. Dry eye disease. A review. Cesk Slov Oftalmol. (2021) 77:107–19. doi: 10.31348/2020/29
37. Nakamura, S, Shibuya, M, Nakashima, H, Hisamura, R, Masuda, N, Imagawa, T, et al. Involvement of oxidative stress on corneal epithelial alterations in a blink-suppressed dry eye. Invest Ophthalmol Vis Sci. (2007) 48:1552–8. doi: 10.1167/iovs.06-1027
38. Li, H, Li, F, Zhou, R, Gao, K, Liang, L, and Zhang, X. Aerobic exercise increases tear secretion and decreases inflammatory cytokines in healthy subjects. Asia Pac J Ophthalmol. (2020) 9:404–11. doi: 10.1097/apo.0000000000000281
39. Sun, C, Chen, X, Huang, Y, Zou, H, Fan, W, Yang, M, et al. Effects of aerobic exercise on tear secretion and tear film stability in dry eye patients. BMC Ophthalmol. (2022) 22:9. doi: 10.1186/s12886-021-02230-9
40. Hansen, MH, Laigaard, PP, Olsen, EM, Skovgaard, AM, Larsen, M, Kessel, L, et al. Low physical activity and higher use of screen devices are associated with myopia at the age of 16–17 years in the CCC2000 eye study. Acta Ophthalmol. (2020) 98:315–21. doi: 10.1111/aos.14242
41. Holton, V, Hinterlong, JE, Tsai, CY, Tsai, JC, Wu, JS, and Liou, YM. A nationwide study of myopia in Taiwanese school children: family, activity, and school-related factors. J Sch Nurs. (2021) 37:117–27. doi: 10.1177/1059840519850619
42. He, M, Xiang, F, Zeng, Y, Mai, J, Chen, Q, Zhang, J, et al. Effect of time spent outdoors at school on the development of myopia among children in China: a randomized clinical trial. JAMA. (2015) 314:1142–8. doi: 10.1001/jama.2015.10803
43. French, AN, Morgan, IG, Mitchell, P, and Rose, KA. Risk factors for incident myopia in Australian schoolchildren: the Sydney adolescent vascular and eye study. Ophthalmology. (2013) 120:2100–8. doi: 10.1016/j.ophtha.2013.02.035
44. Lee, YY, Lo, CT, Sheu, SJ, and Yin, LT. Risk factors for and progression of myopia in young Taiwanese men. Ophthalmic Epidemiol. (2015) 22:66–73. doi: 10.3109/09286586.2014.988874.
45. Ip, JM, Saw, SM, Rose, KA, Morgan, IG, Kifley, A, Wang, JJ, et al. Role of near work in myopia: findings in a sample of Australian school children. Invest Ophthalmol Vis Sci. (2008) 49:2903–10. doi: 10.1167/iovs.07-0804
46. Nguyen, L, Magno, MS, Utheim, TP, Hammond, CJ, and Vehof, J. The relationship between sedentary behavior and dry eye disease. Ocul Surf. (2023) 28:11–7. doi: 10.1016/j.jtos.2023.01.002
47. Vera, J, Jiménez, R, Madinabeitia, I, Masiulis, N, and Cárdenas, D. A maximal incremental effort alters tear osmolarity depending on the fitness level in military helicopter pilots. Ocul Surf. (2017) 15:795–801. doi: 10.1016/j.jtos.2017.08.001
48. Peart, DJ, Walshe, IH, Sweeney, EL, James, E, Henderson, T, O’Doherty, AF, et al. The effect of acute exercise on environmentally induced symptoms of dry eye. Physiol Rep. (2020) 8:e14262. doi: 10.14814/phy2.14262
49. Zheng Selin, J, Orsini, N, Ejdervik Lindblad, B, and Wolk, A. Long-term physical activity and risk of age-related cataract: a population-based prospective study of male and female cohorts. Ophthalmology. (2015) 122:274–80. doi: 10.1016/j.ophtha.2014.08.023
50. López-Sánchez, GF, Pardhan, S, Trott, M, Sánchez-Castillo, S, Jackson, SE, Tully, M, et al. The association between physical activity and cataracts among 17,777 people aged 15–69 years residing in Spain. Ophthalmic Epidemiol. (2020) 27:272–7. doi: 10.1080/09286586.2020.1730911
51. Dutheil, F, Oueslati, T, Delamarre, L, Castanon, J, Maurin, C, Chiambaretta, F, et al. Myopia and near work: a systematic review and meta-analysis. Int J Environ Res Public Health. (2023) 20:875. doi: 10.3390/ijerph20010875
52. Baird, PN, Saw, SM, Lanca, C, Guggenheim, JA, Smith Iii, EL, Zhou, X, et al. Myopia. Nat Rev Dis Primers. (2020) 6:99. doi: 10.1038/s41572-020-00231-4
53. Flitcroft, DI. The complex interactions of retinal, optical and environmental factors in myopia aetiology. Prog Retin Eye Res. (2012) 31:622–60. doi: 10.1016/j.preteyeres.2012.06.004
54. Bullimore, MA, and Brennan, NA. Myopia control: why each diopter matters. Optom Vis Sci. (2019) 96:463–5. doi: 10.1097/opx.0000000000001367
55. O’Donoghue, L, Kapetanankis, VV, McClelland, JF, Logan, NS, Owen, CG, Saunders, KJ, et al. Risk factors for childhood myopia: findings from the NICER study. Invest Ophthalmol Vis Sci. (2015) 56:1524–30. doi: 10.1167/iovs.14-15549
56. Read, SA, Collins, MJ, and Vincent, SJ. Light exposure and physical activity in myopic and emmetropic children. Optom Vis Sci. (2014) 91:330–41. doi: 10.1097/opx.0000000000000160
57. Khader, YS, Batayha, WQ, Abdul-Aziz, SM, and Al-Shiekh-Khalil, MI. Prevalence and risk indicators of myopia among schoolchildren in Amman, Jordan. East Mediterr Health J. (2006) 12:434–9.
58. Mutti, DO, Mitchell, GL, Moeschberger, ML, Jones, LA, and Zadnik, K. Parental myopia, near work, school achievement, and children’s refractive error. Invest Ophthalmol Vis Sci. (2002) 43:3633–40.
59. Guggenheim, JA, Northstone, K, McMahon, G, Ness, AR, Deere, K, Mattocks, C, et al. Time outdoors and physical activity as predictors of incident myopia in childhood: a prospective cohort study. Invest Ophthalmol Vis Sci. (2012) 53:2856–65. doi: 10.1167/iovs.11-9091
60. Jacobsen, N, Jensen, H, and Goldschmidt, E. Does the level of physical activity in university students influence development and progression of myopia?—a 2-year prospective cohort study. Invest Ophthalmol Vis Sci. (2008) 49:1322–7. doi: 10.1167/iovs.07-1144
61. Guo, Y, Liu, L, Lv, Y, Tang, P, Feng, Y, Wu, M, et al. Outdoor jogging and myopia progression in school children from rural Beijing: the Beijing children eye study. Transl Vis Sci Technol. (2019) 8:2. doi: 10.1167/tvst.8.3.2
62. Zhu, Z, Chen, Y, Tan, Z, Xiong, R, McGuinness, MB, and Müller, A. Interventions recommended for myopia prevention and control among children and adolescents in China: a systematic review. Br J Ophthalmol. (2023) 107:160–6. doi: 10.1136/bjophthalmol-2021-319306
63. Xiong, S, Sankaridurg, P, Naduvilath, T, Zang, J, Zou, H, Zhu, J, et al. Time spent in outdoor activities in relation to myopia prevention and control: a meta-analysis and systematic review. Acta Ophthalmol. (2017) 95:551–66. doi: 10.1111/aos.13403
64. Karthikeyan, SK, Ashwini, DL, Priyanka, M, Nayak, A, and Biswas, S. Physical activity, time spent outdoors, and near work in relation to myopia prevalence, incidence, and progression: An overview of systematic reviews and meta-analyses. Indian J Ophthalmol. (2022) 70:728–39. doi: 10.4103/ijo.IJO_1564_21
65. Fitzgerald, ME, Wildsoet, CF, and Reiner, A. Temporal relationship of choroidal blood flow and thickness changes during recovery from form deprivation myopia in chicks. Exp Eye Res. (2002) 74:561–70. doi: 10.1006/exer.2002.1142.
66. Nickla, DL. Transient increases in choroidal thickness are consistently associated with brief daily visual stimuli that inhibit ocular growth in chicks. Exp Eye Res. (2007) 84:951–9. doi: 10.1016/j.exer.2007.01.017.
67. Landis, EG, Park, HN, Chrenek, M, He, L, Sidhu, C, Chakraborty, R, et al. Ambient light regulates retinal dopamine signaling and myopia susceptibility. Invest Ophthalmol Vis Sci. (2021) 62:28. doi: 10.1167/iovs.62.1.28
68. Ashby, RS, and Schaeffel, F. The effect of bright light on lens compensation in chicks. Invest Ophthalmol Vis Sci. (2010) 51:5247–53. doi: 10.1167/iovs.09-4689.
69. Bowrey, HE, Zeng, G, Tse, DY, Leotta, AJ, Wu, Y, To, CH, et al. The effect of spectacle lenses containing peripheral defocus on refractive error and horizontal eye shape in the Guinea pig. Invest Ophthalmol Vis Sci. (2017) 58:2705–14. doi: 10.1167/iovs.16-20240.
70. Mutti, DO, Sinnott, LT, Mitchell, GL, Jones-Jordan, LA, Moeschberger, ML, Cotter, SA, et al. Relative peripheral refractive error and the risk of onset and progression of myopia in children. Invest Ophthalmol Vis Sci. (2011) 52:199–205. doi: 10.1167/iovs.09-4826
71. Cicinelli, MV, Buchan, JC, Nicholson, M, Varadaraj, V, and Khanna, RC. Cataracts. Lancet. (2023) 401:377–89. doi: 10.1016/s0140-6736(22)01839-6
72. Liu, YC, Wilkins, M, Kim, T, Malyugin, B, and Mehta, JS. Cataracts. Lancet. (2017) 390:600–12. doi: 10.1016/s0140-6736(17)30544-5.
73. Lamoureux, EL, Fenwick, E, Pesudovs, K, and Tan, D. The impact of cataract surgery on quality of life. Curr Opin Ophthalmol. (2011) 22:19–27. doi: 10.1097/ICU.0b013e3283414284
74. Kaur, J, Kukreja, S, Kaur, A, Malhotra, N, and Kaur, R. The oxidative stress in cataract patients. J Clin Diagn Res. (2012) 6:1629–32. doi: 10.7860/jcdr/2012/4856.2626
75. Michael, R, and Bron, AJ. The ageing lens and cataract: a model of normal and pathological ageing. Philos Trans R Soc B. (2011) 366:1278–92. doi: 10.1098/rstb.2010.0300.
76. Chang, D, Zhang, X, Rong, S, Sha, Q, Liu, P, Han, T, et al. Serum antioxidative enzymes levels and oxidative stress products in age-related cataract patients. Oxid Med Cell Longev. (2013) 2013:587826:587826. doi: 10.1155/2013/587826
77. Radak, Z, Zhao, Z, Koltai, E, Ohno, H, and Atalay, M. Oxygen consumption and usage during physical exercise: the balance between oxidative stress and ROS-dependent adaptive signaling. Antioxid Redox Signal. (2013) 18:1208–46. doi: 10.1089/ars.2011.4498
78. He, F, Li, J, Liu, Z, Chuang, CC, Yang, W, and Zuo, L. Redox mechanism of reactive oxygen species in exercise. Front Physiol. (2016) 7:486. doi: 10.3389/fphys.2016.00486
79. Ahmad, A, and Ahsan, H. Biomarkers of inflammation and oxidative stress in ophthalmic disorders. J Immunoassay Immunochem. (2020) 41:257–71. doi: 10.1080/15321819.2020.1726774
80. Schaumberg, DA, Ridker, PM, Glynn, RJ, Christen, WG, Dana, MR, and Hennekens, CH. High levels of plasma C-reactive protein and future risk of age-related cataract. Ann Epidemiol. (1999) 9:166–71. doi: 10.1016/s1047-2797(98)00049-0
81. El Assar, M, Angulo, J, and Rodríguez-Mañas, L. Oxidative stress and vascular inflammation in aging. Free Radic Biol Med. (2013) 65:380–401. doi: 10.1016/j.freeradbiomed.2013.07.003
82. Franceschi, C, and Campisi, J. Chronic inflammation (inflammaging) and its potential contribution to age-associated diseases. J Gerontol A. (2014) 69:S4–9. doi: 10.1093/gerona/glu057.
83. Sallam, N, and Laher, I. Exercise modulates oxidative stress and inflammation in aging and cardiovascular diseases. Oxid Med Cell Longev. (2016) 2016:7239639–2. doi: 10.1155/2016/7239639
84. Monteiro-Junior, RS, de Tarso Maciel-Pinheiro, P, da Matta Mello Portugal, E, da Silva Figueiredo, LF, Terra, R, Carneiro, LSF, et al. Effect of exercise on inflammatory profile of older persons: systematic review and meta-analyses: systematic review and meta-analyses. J Phys Act Health. (2018) 15:64–71. doi: 10.1123/jpah.2016-0735
85. Brooks, N, Layne, JE, Gordon, PL, Roubenoff, R, Nelson, ME, and Castaneda-Sceppa, C. Strength training improves muscle quality and insulin sensitivity in Hispanic older adults with type 2 diabetes. Int J Med Sci. (2006) 4:19–27. doi: 10.7150/ijms.4.19.
86. Abd El-Kader, SM, and Al-Shreef, FM. Inflammatory cytokines and immune system modulation by aerobic versus resisted exercise training for elderly. Afr Health Sci. (2018) 18:120–31. doi: 10.4314/ahs.v18i1.16
87. Hiller, R, Sperduto, RD, Reed, GF, D'Agostino, RB, and Wilson, PW. Serum lipids and age-related lens opacities: a longitudinal investigation: the Framingham studies. Ophthalmology. (2003) 110:578–83. doi: 10.1016/s0161-6420(02)01762-1
88. Klein, BE, Klein, R, Jensen, SC, and Linton, KL. Hypertension and lens opacities from the beaver dam eye study. Am J Ophthalmol. (1995) 119:640–6. doi: 10.1016/s0002-9394(14)70223-5.
89. Yu, X, Lyu, D, Dong, X, He, J, and Yao, K. Hypertension and risk of cataract: a meta-analysis. PLoS One. (2014) 9:e114012. doi: 10.1371/journal.pone.0114012.
90. Esefeld, K, Kress, S, Behrens, M, Zimmer, P, Stumvoll, M, Thurm, U, et al. Diabetes, sports and exercise. Exp Clin Endocrinol Diabetes. (2023) 131:51–60. doi: 10.1055/a-1946-3768
91. Cornelissen, VA, and Smart, NA. Exercise training for blood pressure: a systematic review and meta-analysis. J Am Heart Assoc. (2013) 2:e004473. doi: 10.1161/jaha.112.004473
92. Wood, PD, Haskell, WL, Blair, SN, Williams, PT, Krauss, RM, Lindgren, FT, et al. Increased exercise level and plasma lipoprotein concentrations: a one-year, randomized, controlled study in sedentary, middle-aged men. Metab Clin Exp. (1983) 32:31–9. doi: 10.1016/0026-0495(83)90152-x
93. Figueroa, A, Going, SB, Milliken, LA, Blew, RM, Sharp, S, Teixeira, PJ, et al. Effects of exercise training and hormone replacement therapy on lean and fat mass in postmenopausal women. J Gerontol A. (2003) 58:266–70. doi: 10.1093/gerona/58.3.m266
94. He, N, and Ye, H. Exercise and hyperlipidemia. Adv Exp Med Biol. (2020) 1228:79–90. doi: 10.1007/978-981-15-1792-1_5.
95. Tham, YC, Li, X, Wong, TY, Quigley, HA, Aung, T, and Cheng, CY. Global prevalence of glaucoma and projections of glaucoma burden through 2040: a systematic review and meta-analysis. Ophthalmology. (2014) 121:2081–90. doi: 10.1016/j.ophtha.2014.05.013.
96. Weinreb, RN, Aung, T, and Medeiros, FA. The pathophysiology and treatment of glaucoma: a review. JAMA. (2014) 311:1901–11. doi: 10.1001/jama.2014.3192.
97. Jayaram, H, Kolko, M, Friedman, DS, and Gazzard, G. Glaucoma: now and beyond. Lancet. (2023) 402:1788–801. doi: 10.1016/s0140-6736(23)01289-8
98. Kang, JM, and Tanna, AP. Glaucoma. Med Clin North Am. (2021) 105:493–510. doi: 10.1016/j.mcna.2021.01.004
99. Heijl, A, Leske, MC, Bengtsson, B, Hyman, L, Bengtsson, B, and Hussein, M. Reduction of intraocular pressure and glaucoma progression: results from the early manifest glaucoma trial. Arch Ophthalmol. (2002) 120:1268–79. doi: 10.1001/archopht.120.10.1268
100. Perez, CI, Singh, K, and Lin, S. Relationship of lifestyle, exercise, and nutrition with glaucoma. Curr Opin Ophthalmol. (2019) 30:82–8. doi: 10.1097/icu.0000000000000553.
101. Yuan, Y, Lin, TPH, Gao, K, Zhou, R, Radke, NV, Lam, DSC, et al. Aerobic exercise reduces intraocular pressure and expands Schlemm’s canal dimensions in healthy and primary open-angle glaucoma eyes. Indian J Ophthalmol. (2021) 69:1127–34. doi: 10.4103/ijo.IJO_2858_20
102. Ong, SR, Crowston, JG, Loprinzi, PD, and Ramulu, PY. Physical activity, visual impairment, and eye disease. Eye. (2018) 32:1296–303. doi: 10.1038/s41433-018-0081-8
103. Tribble, JR, Hui, F, Jöe, M, Bell, K, Chrysostomou, V, Crowston, JG, et al. Targeting diet and exercise for neuroprotection and neurorecovery in glaucoma. Cells. (2021) 10:295. doi: 10.3390/cells10020295
104. Madjedi, KM, Stuart, KV, Chua, SYL, Ramulu, PY, Warwick, A, Luben, RN, et al. The association of physical activity with glaucoma and related traits in the UK Biobank. Ophthalmology. (2023) 130:1024–36. doi: 10.1016/j.ophtha.2023.06.009
105. Chrysostomou, V, Kezic, JM, Trounce, IA, and Crowston, JG. Forced exercise protects the aged optic nerve against intraocular pressure injury. Neurobiol Aging. (2014) 35:1722–5. doi: 10.1016/j.neurobiolaging.2014.01.019
106. He, YY, Wang, L, Zhang, T, Weng, SJ, Lu, J, and Zhong, YM. Aerobic exercise delays retinal ganglion cell death after optic nerve injury. Exp Eye Res. (2020) 200:108240. doi: 10.1016/j.exer.2020.108240.
107. Williams, PT. Relationship of incident glaucoma versus physical activity and fitness in male runners. Med Sci Sports Exerc. (2009) 41:1566–72. doi: 10.1249/MSS.0b013e31819e420f.
108. Seo, JH, and Lee, Y. Association of exercise intensity with the prevalence of glaucoma and intraocular pressure in men: a study based on the Korea National Health and Nutrition Examination Survey. J Clin Med. (2022) 11:4725. doi: 10.3390/jcm11164725
109. Lee, MJ, Wang, J, Friedman, DS, Boland, MV, De Moraes, CG, and Ramulu, PY. Greater physical activity is associated with slower visual field loss in glaucoma. Ophthalmology. (2019) 126:958–64. doi: 10.1016/j.ophtha.2018.10.012
110. Lin, SC, Wang, SY, Pasquale, LR, Singh, K, and Lin, SC. The relation between exercise and glaucoma in a South Korean population-based sample. PLoS One. (2017) 12:e0171441. doi: 10.1371/journal.pone.0171441
111. McMonnies, CW. Intraocular pressure and glaucoma: is physical exercise beneficial or a risk? J Opt. (2016) 9:139–47. doi: 10.1016/j.optom.2015.12.001.
112. Schuman, JS, Massicotte, EC, Connolly, S, Hertzmark, E, Mukherji, B, and Kunen, MZ. Increased intraocular pressure and visual field defects in high resistance wind instrument players. Ophthalmology. (2000) 107:127–33. doi: 10.1016/s0161-6420(99)00015-9
113. Okafor, K, Vinod, K, and Gedde, SJ. Update on pigment dispersion syndrome and pigmentary glaucoma. Curr Opin Ophthalmol. (2017) 28:154–60. doi: 10.1097/icu.0000000000000352.
114. Haynes, WL, Johnson, AT, and Alward, WL. Effects of jogging exercise on patients with the pigmentary dispersion syndrome and pigmentary glaucoma. Ophthalmology. (1992) 99:1096–103. doi: 10.1016/s0161-6420(92)31845-7
115. Haynes, WL, Johnson, AT, and Alward, WL. Inhibition of exercise-induced pigment dispersion in a patient with the pigmentary dispersion syndrome. Am J Ophthalmol. (1990) 109:601–2. doi: 10.1016/s0002-9394(14)70696-8
116. Schenker, HI, Luntz, MH, Kels, B, and Podos, SM. Exercise-induced increase of intraocular pressure in the pigmentary dispersion syndrome. Am J Ophthalmol. (1980) 89:598–600. doi: 10.1016/0002-9394(80)90073-2
117. Smith, DL, Kao, SF, Rabbani, R, and Musch, DC. The effects of exercise on intraocular pressure in pigmentary glaucoma patients. Ophthalmic Surg. (1989) 20:561–7.
118. Campbell, DG. Pigmentary dispersion and glaucoma. A new theory. Arch Ophthalmol. (1979) 97:1667–72. doi: 10.1001/archopht.1979.01020020235011
119. Jensen, PK, Nissen, O, and Kessing, SV. Exercise and reversed pupillary block in pigmentary glaucoma. Am J Ophthalmol. (1995) 120:110–2. doi: 10.1016/s0002-9394(14)73767-5
120. Qu, J, Wang, D, and Grosskreutz, CL. Mechanisms of retinal ganglion cell injury and defense in glaucoma. Exp Eye Res. (2010) 91:48–53. doi: 10.1016/j.exer.2010.04.002.
121. Kiyota, N, Shiga, Y, Ichinohasama, K, Yasuda, M, Aizawa, N, Omodaka, K, et al. The impact of intraocular pressure elevation on optic nerve head and choroidal blood flow. Invest Ophthalmol Vis Sci. (2018) 59:3488–96. doi: 10.1167/iovs.18-23872
122. Risner, D, Ehrlich, R, Kheradiya, NS, Siesky, B, McCranor, L, and Harris, A. Effects of exercise on intraocular pressure and ocular blood flow: a review. J Glaucoma. (2009) 18:429–36. doi: 10.1097/IJG.0b013e31818fa5f3.
123. Yan, X, Li, M, Song, Y, Guo, J, Zhao, Y, Chen, W, et al. Influence of exercise on intraocular pressure, Schlemm’s canal, and the trabecular meshwork. Invest Ophthalmol Vis Sci. (2016) 57:4733–9. doi: 10.1167/iovs.16-19475
124. Harris, A, Malinovsky, VE, Cantor, LB, Henderson, PA, and Martin, BJ. Isocapnia blocks exercise-induced reductions in ocular tension. Invest Ophthalmol Vis Sci. (1992) 33:2229–32.
125. Passo, MS, Goldberg, L, Elliot, DL, and Van Buskirk, EM. Exercise training reduces intraocular pressure among subjects suspected of having glaucoma. Arch Ophthalmol. (1991) 109:1096–8. doi: 10.1001/archopht.1991.01080080056027
126. Kiuchi, Y, Mishima, HK, Hotehama, Y, Furumoto, A, Hirota, A, and Onari, K. Exercise intensity determines the magnitude of IOP decrease after running. Jpn J Ophthalmol. (1994) 38:191–5.
127. Qureshi, IA, Xi, XR, Wu, XD, Zhang, J, and Shiarkar, E. The effect of physical fitness on intraocular pressure in Chinese medical students. Zhonghua Yi Xue Za Zhi. (1996) 58:317–22.
128. Harris, A, Malinovsky, V, and Martin, B. Correlates of acute exercise-induced ocular hypotension. Invest Ophthalmol Vis Sci. (1994) 35:3852–7.
129. Yeak Dieu Siang, J, Mohamed, M, Mohd Ramli, NB, and Zahari, MB. Effects of regular exercise on intraocular pressure. Eur J Ophthalmol. (2022) 32:2265–73. doi: 10.1177/11206721211051236
130. Kim, CS, Park, S, Chun, Y, Song, W, Kim, HJ, and Kim, J. Treadmill exercise attenuates retinal oxidative stress in naturally-aged mice: an immunohistochemical study. Int J Mol Sci. (2015) 16:21008–20. doi: 10.3390/ijms160921008
131. Baudouin, C, Kolko, M, Melik-Parsadaniantz, S, and Messmer, EM. Inflammation in Glaucoma: from the back to the front of the eye, and beyond. Prog Retin Eye Res. (2021) 83:100916. doi: 10.1016/j.preteyeres.2020.100916
132. Xu, H, Chen, M, and Forrester, JV. Para-inflammation in the aging retina. Prog Retin Eye Res. (2009) 28:348–68. doi: 10.1016/j.preteyeres.2009.06.001
133. Wang, J, Li, M, Geng, Z, Khattak, S, Ji, X, Wu, D, et al. Role of oxidative stress in retinal disease and the early intervention strategies: a review. Oxid Med Cell Longev. (2022) 2022:7836828. doi: 10.1155/2022/7836828
134. Ferreira, SM, Lerner, SF, Brunzini, R, Evelson, PA, and Llesuy, SF. Oxidative stress markers in aqueous humor of glaucoma patients. Am J Ophthalmol. (2004) 137:62–9. doi: 10.1016/s0002-9394(03)00788-8
135. Bouzid, MA, Filaire, E, McCall, A, and Fabre, C. Radical oxygen species, exercise and aging: an update. Sports Med. (2015) 45:1245–61. doi: 10.1007/s40279-015-0348-1
136. Gleeson, M, Bishop, NC, Stensel, DJ, Lindley, MR, Mastana, SS, and Nimmo, MA. The anti-inflammatory effects of exercise: mechanisms and implications for the prevention and treatment of disease. Nat Rev Immunol. (2011) 11:607–15. doi: 10.1038/nri3041.
137. Zhang, J, Liu, Z, Wu, H, Chen, X, Hu, Q, Li, X, et al. Irisin attenuates pathological neovascularization in oxygen-induced retinopathy mice. Invest Ophthalmol Vis Sci. (2022) 63:21. doi: 10.1167/iovs.63.6.21.
138. Picca, A, Calvani, R, Bossola, M, Allocca, E, Menghi, A, Pesce, V, et al. Update on mitochondria and muscle aging: all wrong roads lead to sarcopenia. Biol Chem. (2018) 399:421–36. doi: 10.1515/hsz-2017-0331.
139. Angulo, J, El Assar, M, Álvarez-Bustos, A, and Rodríguez-Mañas, L. Physical activity and exercise: strategies to manage frailty. Redox Biol. (2020) 35:101513. doi: 10.1016/j.redox.2020.101513
140. Osborne, NN, Núñez-Álvarez, C, Joglar, B, and Del Olmo-Aguado, S. Glaucoma: focus on mitochondria in relation to pathogenesis and neuroprotection. Eur J Pharmacol. (2016) 787:127–33. doi: 10.1016/j.ejphar.2016.04.032
141. Schrier, SA, and Falk, MJ. Mitochondrial disorders and the eye. Curr Opin Ophthalmol. (2011) 22:325–31. doi: 10.1097/ICU.0b013e328349419d.
142. Kuang, G, Halimitabrizi, M, Edziah, AA, Salowe, R, and O'Brien, JM. The potential for mitochondrial therapeutics in the treatment of primary open-angle glaucoma: a review. Front Physiol. (2023) 14:1184060. doi: 10.3389/fphys.2023.1184060
143. Williams, PA, Harder, JM, Foxworth, NE, Cochran, KE, Philip, VM, Porciatti, V, et al. Vitamin B3 modulates mitochondrial vulnerability and prevents glaucoma in aged mice. Science. (2017) 355:756–60. doi: 10.1126/science.aal0092
144. Yang, XJ, Ge, J, and Zhuo, YH. Role of mitochondria in the pathogenesis and treatment of glaucoma. Chin Med J. (2013) 126:4358–65. doi: 10.3760/cma.j.issn.0366-6999.20131956
145. Norat, P, Soldozy, S, Sokolowski, JD, Gorick, CM, Kumar, JS, Chae, Y, et al. Mitochondrial dysfunction in neurological disorders: exploring mitochondrial transplantation. npj Regen Med. (2020) 5:22. doi: 10.1038/s41536-020-00107-x.
146. Leick, L, Lyngby, SS, Wojtaszewski, JF, and Pilegaard, H. PGC-1α is required for training-induced prevention of age-associated decline in mitochondrial enzymes in mouse skeletal muscle. Exp Gerontol. (2010) 45:336–42. doi: 10.1016/j.exger.2010.01.011
147. Wohlgemuth, SE, Lees, HA, Marzetti, E, Manini, TM, Aranda, JM, Daniels, MJ, et al. An exploratory analysis of the effects of a weight loss plus exercise program on cellular quality control mechanisms in older overweight women. Rejuvenation Res. (2011) 14:315–24. doi: 10.1089/rej.2010.1132
148. Fudalej, E, Justyniarska, M, Kasarełło, K, Dziedziak, J, Szaflik, JP, and Cudnoch-Jędrzejewska, A. Neuroprotective factors of the retina and their role in promoting survival of retinal ganglion cells: a review. Ophthalmic Res. (2021) 64:345–55. doi: 10.1159/000514441
149. Mansour-Robaey, S, Clarke, DB, Wang, YC, Bray, GM, and Aguayo, AJ. Effects of ocular injury and administration of brain-derived neurotrophic factor on survival and regrowth of axotomized retinal ganglion cells. Proc Natl Acad Sci USA. (1994) 91:1632–6. doi: 10.1073/pnas.91.5.1632
150. Chrysostomou, V, Galic, S, van Wijngaarden, P, Trounce, IA, Steinberg, GR, and Crowston, JG. Exercise reverses age-related vulnerability of the retina to injury by preventing complement-mediated synapse elimination via a BDNF-dependent pathway. Aging Cell. (2016) 15:1082–91. doi: 10.1111/acel.12512
151. Gupta, V, You, Y, Li, J, Gupta, V, Golzan, M, Klistorner, A, et al. BDNF impairment is associated with age-related changes in the inner retina and exacerbates experimental glaucoma. Biochim Biophys Acta. (2014) 1842:1567–78. doi: 10.1016/j.bbadis.2014.05.026
152. Chitranshi, N, Dheer, Y, Abbasi, M, You, Y, Graham, SL, and Gupta, V. Glaucoma pathogenesis and neurotrophins: focus on the molecular and genetic basis for therapeutic prospects. Curr Neuropharmacol. (2018) 16:1018–35. doi: 10.2174/1570159x16666180419121247
153. Chu-Tan, JA, Kirkby, M, and Natoli, R. Running to save sight: the effects of exercise on retinal health and function. Clin Experiment Ophthalmol. (2022) 50:74–90. doi: 10.1111/ceo.14023
154. Pardue, MT, Chrenek, MA, Schmidt, RH, Nickerson, JM, and Boatright, JH. Potential role of exercise in retinal health. Prog Mol Biol Transl Sci. (2015) 134:491–502. doi: 10.1016/bs.pmbts.2015.06.011.
155. Hu, YS, Long, N, Pigino, G, Brady, ST, and Lazarov, O. Molecular mechanisms of environmental enrichment: impairments in Akt/GSK3β, neurotrophin-3 and CREB signaling. PLoS One. (2013) 8:e64460. doi: 10.1371/journal.pone.0064460
156. Domenici, L, Origlia, N, Falsini, B, Cerri, E, Barloscio, D, Fabiani, C, et al. Rescue of retinal function by BDNF in a mouse model of glaucoma. PLoS One. (2014) 9:e115579. doi: 10.1371/journal.pone.0115579
157. Chen, C, and Nakagawa, S. Physical activity for cognitive health promotion: an overview of the underlying neurobiological mechanisms. Ageing Res Rev. (2023) 86:101868. doi: 10.1016/j.arr.2023.101868
158. Meeusen, R, and De Meirleir, K. Exercise and brain neurotransmission. Sports Med. (1995) 20:160–88. doi: 10.2165/00007256-199520030-00004.
159. Shimojo, G, Joseph, B, Shah, R, Consolim-Colombo, FM, De Angelis, K, and Ulloa, L. Exercise activates vagal induction of dopamine and attenuates systemic inflammation. Brain Behav Immun. (2019) 75:181–91. doi: 10.1016/j.bbi.2018.10.005
160. Bucolo, C, Leggio, GM, Drago, F, and Salomone, S. Dopamine outside the brain: the eye, cardiovascular system and endocrine pancreas. Pharmacol Ther. (2019) 203:107392. doi: 10.1016/j.pharmthera.2019.07.003.
161. Prünte, C, and Flammer, J. The novel dopamine D-1 antagonist and D-2 agonist, SDZ GLC-756, lowers intraocular pressure in healthy human volunteers and in patients with glaucoma. Ophthalmology. (1995) 102:1291–7. doi: 10.1016/s0161-6420(95)30872-x.
162. Leggio, GM, Bucolo, C, Platania, CB, Salomone, S, and Drago, F. Current drug treatments targeting dopamine D3 receptor. Pharmacol Ther. (2016) 165:164–77. doi: 10.1016/j.pharmthera.2016.06.007
163. Chu, E, Chu, TC, and Potter, DE. Mechanisms and sites of ocular action of 7-hydroxy-2-dipropylaminotetralin: a dopamine3 receptor agonist. J Pharmacol Exp Ther. (2000) 293:710–6.
164. Bucolo, C, Leggio, GM, Maltese, A, Castorina, A, D'Agata, V, and Drago, F. Dopamine-₃ receptor modulates intraocular pressure: implications for glaucoma. Biochem Pharmacol. (2012) 83:680–6. doi: 10.1016/j.bcp.2011.11.031
165. Pescosolido, N, Parisi, F, Russo, P, Buomprisco, G, and Nebbioso, M. Role of dopaminergic receptors in glaucomatous disease modulation. Biomed Res Int. (2013) 2013:193048:193048. doi: 10.1155/2013/193048
166. Chu, E, Socci, R, and Chu, TC. PD128,907 induces ocular hypotension in rabbits: involvement of D2/D3 dopamine receptors and brain natriuretic peptide. J Ocul Pharmacol Ther. (2004) 20:15–23. doi: 10.1089/108076804772745428.
167. Reyes-Resina, I, Awad Alkozi, H, Del Ser-Badia, A, Sánchez-Naves, J, Lillo, J, Jiménez, J, et al. Expression of melatonin and dopamine D3 receptor heteromers in eye ciliary body epithelial cells and negative correlation with ocular hypertension. Cells. (2020) 9:152. doi: 10.3390/cells9010152
168. Dkhissi, O, Chanut, E, Versaux-Botteri, C, Minvielle, F, Trouvin, JH, and Nguyen-Legros, J. Changes in retinal dopaminergic cells and dopamine rhythmic metabolism during the development of a glaucoma-like disorder in quails. Invest Ophthalmol Vis Sci. (1996) 37:2335–44.
169. Liu, Y, and Wu, N. Progress of nanotechnology in diabetic retinopathy treatment. Int J Nanomedicine. (2021) 16:1391–403. doi: 10.2147/ijn.S294807.
170. Zhang, J, Zhang, J, Zhang, C, Zhang, J, Gu, L, Luo, D, et al. Diabetic macular edema: current understanding, molecular mechanisms and therapeutic implications. Cells. (2022) 11:3362. doi: 10.3390/cells11213362
171. Testa, R, Bonfigli, AR, Prattichizzo, F, La Sala, L, De Nigris, V, and Ceriello, A. The “metabolic memory” theory and the early treatment of hyperglycemia in prevention of diabetic complications. Nutrients. (2017) 9:437. doi: 10.3390/nu9050437
172. Wang, YX, Wei, WB, Xu, L, and Jonas, JB. Physical activity and eye diseases. The Beijing eye study. Acta Ophthalmol. (2019) 97:325–31. doi: 10.1111/aos.13962
173. Loprinzi, PD. Association of accelerometer-assessed sedentary behavior with diabetic retinopathy in the United States. JAMA Ophthalmol. (2016) 134:1197–8. doi: 10.1001/jamaophthalmol.2016.2400
174. Soleimani, A, Soltani, P, Karimi, H, Mirzaei, M, Esfahanian, F, Yavari, M, et al. The effect of moderate-intensity aerobic exercise on non-proliferative diabetic retinopathy in type II diabetes mellitus patients: a clinical trial. Microvasc Res. (2023) 149:104556. doi: 10.1016/j.mvr.2023.104556
175. Cheng, CK, Shang, W, Liu, J, Cheang, WS, Wang, Y, Xiang, L, et al. Activation of AMPK/miR-181b Axis alleviates endothelial dysfunction and vascular inflammation in diabetic mice. Antioxidants. (2022) 11:1137. doi: 10.3390/antiox11061137
176. Ren, C, Liu, W, Li, J, Cao, Y, Xu, J, and Lu, P. Physical activity and risk of diabetic retinopathy: a systematic review and meta-analysis. Acta Diabetol. (2019) 56:823–37. doi: 10.1007/s00592-019-01319-4.
177. Zinn, S, Nelis, P, Minnebeck, K, Hinder, J, Eter, N, Brand, SM, et al. Effect of high-intensity interval training in patients with type 1 diabetes on physical fitness and retinal microvascular perfusion determined by optical coherence tomography angiography. Microvasc Res. (2020) 132:104057. doi: 10.1016/j.mvr.2020.104057
178. Alten, F, Eter, N, and Schmitz, B. Differential effects of high-intensity interval training (HIIT) on choriocapillaris perfusion in healthy adults and patients with type 1 diabetes mellitus (T1DM). Microvasc Res. (2021) 135:104128. doi: 10.1016/j.mvr.2020.104128
179. Barot, M, Gokulgandhi, MR, Patel, S, and Mitra, AK. Microvascular complications and diabetic retinopathy: recent advances and future implications. Future Med Chem. (2013) 5:301–14. doi: 10.4155/fmc.12.206
180. Lutty, GA. Effects of diabetes on the eye. Invest Ophthalmol Vis Sci. (2013) 54:ORSF81–7. doi: 10.1167/iovs.13-12979
181. Forbes, JM, and Cooper, ME. Mechanisms of diabetic complications. Physiol Rev. (2013) 93:137–88. doi: 10.1152/physrev.00045.2011
182. Tomita, Y, Lee, D, Tsubota, K, Negishi, K, and Kurihara, T. Updates on the current treatments for diabetic retinopathy and possibility of future oral therapy. J Clin Med. (2021) 10:4666. doi: 10.3390/jcm10204666
183. Cheung, N, Mitchell, P, and Wong, TY. Diabetic retinopathy. Lancet. (2010) 376:124–36. doi: 10.1016/s0140-6736(09)62124-3.
184. Watanabe, D, Suzuma, K, Matsui, S, Kurimoto, M, Kiryu, J, Kita, M, et al. Erythropoietin as a retinal angiogenic factor in proliferative diabetic retinopathy. N Engl J Med. (2005) 353:782–92. doi: 10.1056/NEJMoa041773
185. Phielix, E, Meex, R, Moonen-Kornips, E, Hesselink, MK, and Schrauwen, P. Exercise training increases mitochondrial content and ex vivo mitochondrial function similarly in patients with type 2 diabetes and in control individuals. Diabetologia. (2010) 53:1714–21. doi: 10.1007/s00125-010-1764-2.
186. Kadoglou, NP, Iliadis, F, Angelopoulou, N, Perrea, D, Ampatzidis, G, Liapis, CD, et al. The anti-inflammatory effects of exercise training in patients with type 2 diabetes mellitus. Eur J Cardiovasc Prev Rehabil. (2007) 14:837–43. doi: 10.1097/HJR.0b013e3282efaf50.
187. Boulé, NG, Kenny, GP, Haddad, E, Wells, GA, and Sigal, RJ. Meta-analysis of the effect of structured exercise training on cardiorespiratory fitness in type 2 diabetes mellitus. Diabetologia. (2003) 46:1071–81. doi: 10.1007/s00125-003-1160-2.
188. Kanaley, JA, Colberg, SR, Corcoran, MH, Malin, SK, Rodriguez, NR, Crespo, CJ, et al. Exercise/physical activity in individuals with type 2 diabetes: a consensus statement from the American College of Sports Medicine. Med Sci Sports Exerc. (2022) 54:353–68. doi: 10.1249/mss.0000000000002800
189. Liu, Y, Ye, W, Chen, Q, Zhang, Y, Kuo, CH, and Korivi, M. Resistance exercise intensity is correlated with attenuation of HbA1c and insulin in patients with type 2 diabetes: a systematic review and meta-analysis. Int J Environ Res Public Health. (2019) 16:140. doi: 10.3390/ijerph16010140
190. Karstoft, K, Winding, K, Knudsen, SH, Nielsen, JS, Thomsen, C, Pedersen, BK, et al. The effects of free-living interval-walking training on glycemic control, body composition, and physical fitness in type 2 diabetic patients: a randomized, controlled trial. Diabetes Care. (2013) 36:228–36. doi: 10.2337/dc12-0658
191. Nieuwoudt, S, Fealy, CE, Foucher, JA, Scelsi, AR, Malin, SK, Pagadala, M, et al. Functional high-intensity training improves pancreatic β-cell function in adults with type 2 diabetes. Am J Physiol Endocrinol Metab. (2017) 313:E314–e320. doi: 10.1152/ajpendo.00407.2016.
192. Sigal, RJ, Kenny, GP, Boulé, NG, Wells, GA, Prud'homme, D, Fortier, M, et al. Effects of aerobic training, resistance training, or both on glycemic control in type 2 diabetes: a randomized trial. Ann Intern Med. (2007) 147:357–69. doi: 10.7326/0003-4819-147-6-200709180-00005
193. Schwingshackl, L, Missbach, B, Dias, S, König, J, and Hoffmann, G. Impact of different training modalities on glycaemic control and blood lipids in patients with type 2 diabetes: a systematic review and network meta-analysis. Diabetologia. (2014) 57:1789–97. doi: 10.1007/s00125-014-3303-z
194. Giannopoulou, I, Fernhall, B, Carhart, R, Weinstock, RS, Baynard, T, Figueroa, A, et al. Effects of diet and/or exercise on the adipocytokine and inflammatory cytokine levels of postmenopausal women with type 2 diabetes. Metab Clin Exp. (2005) 54:866–75. doi: 10.1016/j.metabol.2005.01.033
195. Antonetti, DA, Barber, AJ, Bronson, SK, Freeman, WM, Gardner, TW, Jefferson, LS, et al. Diabetic retinopathy: seeing beyond glucose-induced microvascular disease. Diabetes. (2006) 55:2401–11. doi: 10.2337/db05-1635
196. Abu-El-Asrar, AM, Dralands, L, Missotten, L, Al-Jadaan, IA, and Geboes, K. Expression of apoptosis markers in the retinas of human subjects with diabetes. Invest Ophthalmol Vis Sci. (2004) 45:2760–6. doi: 10.1167/iovs.03-1392
197. Adornetto, A, Gesualdo, C, Laganà, ML, Trotta, MC, Rossi, S, and Russo, R. Autophagy: a novel pharmacological target in diabetic retinopathy. Front Pharmacol. (2021) 12:695267. doi: 10.3389/fphar.2021.695267
198. Trotta, MC, Gesualdo, C, Herman, H, Gharbia, S, Balta, C, Lepre, CC, et al. Systemic beta-hydroxybutyrate affects BDNF and autophagy into the retina of diabetic mice. Int J Mol Sci. (2022) 23:10184. doi: 10.3390/ijms231710184
199. Liu, Y, Tao, L, Fu, X, Zhao, Y, and Xu, X. BDNF protects retinal neurons from hyperglycemia through the TrkB/ERK/MAPK pathway. Mol Med Rep. (2013) 7:1773–8. doi: 10.3892/mmr.2013.1433
200. Kaviarasan, K, Jithu, M, Arif Mulla, M, Sharma, T, Sivasankar, S, Das, UN, et al. Low blood and vitreal BDNF, LXA4 and altered Th1/Th2 cytokine balance are potential risk factors for diabetic retinopathy. Metab Clin Exp. (2015) 64:958–66. doi: 10.1016/j.metabol.2015.04.005
201. He, M, Long, P, Chen, T, Li, K, Wei, D, Zhang, Y, et al. ALDH2/SIRT1 contributes to type 1 and type 2 diabetes-induced retinopathy through depressing oxidative stress. Oxid Med Cell Longev. (2021) 2021:1641717. doi: 10.1155/2021/1641717
202. Li, C, Miao, X, Li, F, Wang, S, Liu, Q, Wang, Y, et al. Oxidative stress-related mechanisms and antioxidant therapy in diabetic retinopathy. Oxid Med Cell Longev. (2017) 2017:9702820. doi: 10.1155/2017/9702820
203. Kang, Q, and Yang, C. Oxidative stress and diabetic retinopathy: molecular mechanisms, pathogenetic role and therapeutic implications. Redox Biol. (2020) 37:101799. doi: 10.1016/j.redox.2020.101799
204. Elahy, M, Baindur-Hudson, S, Cruzat, VF, Newsholme, P, and Dass, CR. Mechanisms of PEDF-mediated protection against reactive oxygen species damage in diabetic retinopathy and neuropathy. J Endocrinol. (2014) 222:R129–39. doi: 10.1530/joe-14-0065
205. Kowluru, RA. Cross talks between oxidative stress, inflammation and epigenetics in diabetic retinopathy. Cells. (2023) 12:300. doi: 10.3390/cells12020300
206. Frith, E, and Loprinzi, PD. Physical activity, muscle-strengthening activities, and systemic inflammation among retinopathy patients. Diabetes Spectr. (2019) 32:16–20. doi: 10.2337/ds18-0002.
207. Chistiakov, DA. Diabetic retinopathy: pathogenic mechanisms and current treatments. Diabetes Metab Syndr. (2011) 5:165–72. doi: 10.1016/j.dsx.2012.02.025
208. Palanisamy, K, Nareshkumar, RN, Sivagurunathan, S, Raman, R, Sulochana, KN, and Chidambaram, S. Anti-angiogenic effect of adiponectin in human primary microvascular and macrovascular endothelial cells. Microvasc Res. (2019) 122:136–45. doi: 10.1016/j.mvr.2018.08.002.
209. Omae, T, Nagaoka, T, and Yoshida, A. Relationship between retinal blood flow and serum adiponectin concentrations in patients with type 2 diabetes mellitus. Invest Ophthalmol Vis Sci. (2015) 56:4143–9. doi: 10.1167/iovs.15-16447
210. Makanae, Y, Ogasawara, R, Sato, K, Takamura, Y, Matsutani, K, Kido, K, et al. Acute bout of resistance exercise increases vitamin D receptor protein expression in rat skeletal muscle. Exp Physiol. (2015) 100:1168–76. doi: 10.1113/ep085207
211. Klenk, J, Rapp, K, Denkinger, M, Nagel, G, Nikolaus, T, Peter, R, et al. Objectively measured physical activity and vitamin D status in older people from Germany. J Epidemiol Community Health. (2015) 69:388–92. doi: 10.1136/jech-2014-204632
212. Al-Othman, A, Al-Musharaf, S, Al-Daghri, NM, Krishnaswamy, S, Yusuf, DS, Alkharfy, KM, et al. Effect of physical activity and sun exposure on vitamin D status of Saudi children and adolescents. BMC Pediatr. (2012) 12:92. doi: 10.1186/1471-2431-12-92
213. Zakaria, WNA, Mohd Yunus, N, Yaacob, NM, Omar, J, Wan Mohamed, WMI, Sirajudeen, KNS, et al. Association between vitamin D receptor polymorphisms (BsmI and FokI) and glycemic control among patients with type 2 diabetes. Int J Environ Res Public Health. (2021) 18:1595. doi: 10.3390/ijerph18041595
214. Wang, W, and Lo, ACY. Diabetic retinopathy: pathophysiology and treatments. Int J Mol Sci. (2018) 19:1816. doi: 10.3390/ijms19061816
215. Knudtson, MD, Klein, R, and Klein, BE. Physical activity and the 15-year cumulative incidence of age-related macular degeneration: the Beaver Dam Eye Study. Br J Ophthalmol. (2006) 90:1461–3. doi: 10.1136/bjo.2006.103796
216. Ulańczyk, Z, Grabowicz, A, Cecerska-Heryć, E, Śleboda-Taront, D, Krytkowska, E, Mozolewska-Piotrowska, K, et al. Dietary and lifestyle factors modulate the activity of the endogenous antioxidant system in patients with age-related macular degeneration: correlations with disease severity. Antioxidants. (2020) 9:954. doi: 10.3390/antiox9100954
217. Zhang, X, Girardot, PE, Sellers, JT, Li, Y, Wang, J, Chrenek, MA, et al. Wheel running exercise protects against retinal degeneration in the I307N rhodopsin mouse model of inducible autosomal dominant retinitis pigmentosa. Mol Vis. (2019) 25:462–76.
218. Cui, B, Guo, X, Zhou, W, Zhang, X, He, K, Bai, T, et al. Exercise alleviates neovascular age-related macular degeneration by inhibiting AIM2 inflammasome in myeloid cells. Metab Clin Exp. (2023) 144:155584. doi: 10.1016/j.metabol.2023.155584
219. Mitchell, P, Liew, G, Gopinath, B, and Wong, TY. Age-related macular degeneration. Lancet. (2018) 392:1147–59. doi: 10.1016/s0140-6736(18)31550-2
220. Fenwick, EK, Cheung, GCM, Gan, AT, Tan, G, Lee, SY, Wong, D, et al. Change in vision-related quality of life and influencing factors in Asians receiving treatment for neovascular age-related macular degeneration. Br J Ophthalmol. (2018) 102:377–82. doi: 10.1136/bjophthalmol-2017-310532
221. Thomas, CJ, Mirza, RG, and Gill, MK. Age-related macular degeneration. Med Clin North Am. (2021) 105:473–91. doi: 10.1016/j.mcna.2021.01.003.
222. Guymer, RH, and Campbell, TG. Age-related macular degeneration. Lancet. (2023) 401:1459–72. doi: 10.1016/s0140-6736(22)02609-5.
223. Liu, L, Majithia, S, and Tham, YC. Physical activity and age-related macular degeneration: a systematic literature review and meta-analysis. Am J Ophthalmol. (2018) 185:123. doi: 10.1016/j.ajo.2017.10.013
224. Deng, Y, Qiao, L, Du, M, Qu, C, Wan, L, Li, J, et al. Age-related macular degeneration: epidemiology, genetics, pathophysiology, diagnosis, and targeted therapy. Genes Dis. (2022) 9:62–79. doi: 10.1016/j.gendis.2021.02.009
225. Moreno-García, A, Kun, A, Calero, O, Medina, M, and Calero, M. An overview of the role of lipofuscin in age-related neurodegeneration. Front Neurosci. (2018) 12:464. doi: 10.3389/fnins.2018.00464
226. Tang, J, and Kern, TS. Inflammation in diabetic retinopathy. Prog Retin Eye Res. (2011) 30:343–58. doi: 10.1016/j.preteyeres.2011.05.002
227. Li, G, Luna, C, Liton, PB, Navarro, I, Epstein, DL, and Gonzalez, P. Sustained stress response after oxidative stress in trabecular meshwork cells. Mol Vis. (2007) 13:2282–8.
228. Al-Shabrawey, M, Hussein, K, Wang, F, Wan, M, Elmasry, K, Elsherbiny, N, et al. Bone morphogenetic protein-2 induces non-canonical inflammatory and oxidative pathways in human retinal endothelial cells. Front Immunol. (2020) 11:568795. doi: 10.3389/fimmu.2020.568795
229. Paeng, SH, Jung, WK, Park, WS, Lee, DS, Kim, GY, Choi, YH, et al. Caffeic acid phenethyl ester reduces the secretion of vascular endothelial growth factor through the inhibition of the ROS, PI3K and HIF-1α signaling pathways in human retinal pigment epithelial cells under hypoxic conditions. Int J Mol Med. (2015) 35:1419–26. doi: 10.3892/ijmm.2015.2116.
230. Dong, A, Xie, B, Shen, J, Yoshida, T, Yokoi, K, Hackett, SF, et al. Oxidative stress promotes ocular neovascularization. J Cell Physiol. (2009) 219:544–52. doi: 10.1002/jcp.21698
231. McLeod, DS, Bhutto, I, Edwards, MM, Silver, RE, Seddon, JM, and Lutty, GA. Distribution and quantification of choroidal macrophages in human eyes with age-related macular degeneration. Invest Ophthalmol Vis Sci. (2016) 57:5843–55. doi: 10.1167/iovs.16-20049
232. Espinosa-Heidmann, DG, Suner, IJ, Hernandez, EP, Monroy, D, Csaky, KG, and Cousins, SW. Macrophage depletion diminishes lesion size and severity in experimental choroidal neovascularization. Invest Ophthalmol Vis Sci. (2003) 44:3586–92. doi: 10.1167/iovs.03-0038.
233. Siegert, S, Cabuy, E, Scherf, BG, Kohler, H, Panda, S, Le, YZ, et al. Transcriptional code and disease map for adult retinal cell types. Nat Neurosci. (2012) 15:487–95. doi: 10.1038/nn.3032
234. An, AR, Shin, DW, Kim, S, Lee, CH, Park, JH, Park, JH, et al. Health behaviors of people with retinitis pigmentosa in the Republic of Korea. Ophthalmic Epidemiol. (2014) 21:279–86. doi: 10.3109/09286586.2014.926939.
235. Levinson, JD, Joseph, E, Ward, LA, Nocera, JR, Pardue, MT, Bruce, BB, et al. Physical activity and quality of life in retinitis pigmentosa. J Ophthalmol. (2017) 2017:6950642. doi: 10.1155/2017/6950642
236. Huai, P, Xun, H, Reilly, KH, Wang, Y, Ma, W, and Xi, B. Physical activity and risk of hypertension: a meta-analysis of prospective cohort studies. Hypertension. (2013) 62:1021–6. doi: 10.1161/hypertensionaha.113.01965
237. Streese, L, Guerini, C, Bühlmayer, L, Lona, G, Hauser, C, Bade, S, et al. Physical activity and exercise improve retinal microvascular health as a biomarker of cardiovascular risk: a systematic review. Atherosclerosis. (2020) 315:33–42. doi: 10.1016/j.atherosclerosis.2020.09.017
238. Becatti, M, Marcucci, R, Gori, AM, Mannini, L, Grifoni, E, Alessandrello Liotta, A, et al. Erythrocyte oxidative stress is associated with cell deformability in patients with retinal vein occlusion. J Thromb Haemost. (2016) 14:2287–97. doi: 10.1111/jth.13482
239. Tikellis, G, Anuradha, S, Klein, R, and Wong, TY. Association between physical activity and retinal microvascular signs: the atherosclerosis risk in communities (ARIC) study. Microcirculation. (2010) 17:381–93. doi: 10.1111/j.1549-8719.2010.00033.x
240. Scott, IU, Campochiaro, PA, Newman, NJ, and Biousse, V. Retinal vascular occlusions. Lancet. (2020) 396:1927–40. doi: 10.1016/s0140-6736(20)31559-2
241. Baillet, A, Zeboulon, N, Gossec, L, Combescure, C, Bodin, LA, Juvin, R, et al. Efficacy of cardiorespiratory aerobic exercise in rheumatoid arthritis: meta-analysis of randomized controlled trials. Arthritis Care Res. (2010) 62:984–92. doi: 10.1002/acr.20146
242. Lyngberg, K, Danneskiold-Samsøe, B, and Halskov, O. The effect of physical training on patients with rheumatoid arthritis: changes in disease activity, muscle strength and aerobic capacity. A clinically controlled minimized cross-over study. Clin Exp Rheumatol. (1988) 6:253–60.
243. Li, X, Cao, X, Zhao, M, and Bao, Y. The changes of Irisin and inflammatory cytokines in the age-related macular degeneration and retinal vein occlusion. Front Endocrinol. (2022) 13:861757. doi: 10.3389/fendo.2022.861757.
244. Li, DJ, Li, YH, Yuan, HB, Qu, LF, and Wang, P. The novel exercise-induced hormone irisin protects against neuronal injury via activation of the Akt and ERK1/2 signaling pathways and contributes to the neuroprotection of physical exercise in cerebral ischemia. Metab Clin Exp. (2017) 68:31–42. doi: 10.1016/j.metabol.2016.12.003
245. Wang, Y, Tian, M, Tan, J, Pei, X, Lu, C, Xin, Y, et al. Irisin ameliorates neuroinflammation and neuronal apoptosis through integrin αVβ5/AMPK signaling pathway after intracerebral hemorrhage in mice. J Neuroinflammation. (2022) 19:82. doi: 10.1186/s12974-022-02438-6
246. Peng, J, Deng, X, Huang, W, Yu, JH, Wang, JX, Wang, JP, et al. Irisin protects against neuronal injury induced by oxygen-glucose deprivation in part depends on the inhibition of ROS-NLRP3 inflammatory signaling pathway. Mol Immunol. (2017) 91:185–94. doi: 10.1016/j.molimm.2017.09.014.
247. Gillespie, LD, Robertson, MC, Gillespie, WJ, Sherrington, C, Gates, S, Clemson, LM, et al. Interventions for preventing falls in older people living in the community. Cochrane Database Syst Rev. (2012) 2021:CD007146. doi: 10.1002/14651858.CD007146.pub3
248. To, Q, Huynh, VA, Do, D, Do, V, Congdon, N, Meuleners, L, et al. Falls and physical activity among cataract patients in Vietnam. Ophthalmic Epidemiol. (2022) 29:70–7. doi: 10.1080/09286586.2021.1893341
249. Shoss, BL, and Tsai, LM. Postoperative care in cataract surgery. Curr Opin Ophthalmol. (2013) 24:66–73. doi: 10.1097/ICU.0b013e32835b0716.
250. Simó, R, and Hernández, C. What else can we do to prevent diabetic retinopathy? Diabetologia. (2023) 66:1614–21. doi: 10.1007/s00125-023-05940-5
251. Caprioli, J, and Coleman, AL. Blood pressure, perfusion pressure, and glaucoma. Am J Ophthalmol. (2010) 149:704–12. doi: 10.1016/j.ajo.2010.01.018
252. Wu, Z, Ayton, LN, Guymer, RH, and Luu, CD. Low-luminance visual acuity and microperimetry in age-related macular degeneration. Ophthalmology. (2014) 121:1612–9. doi: 10.1016/j.ophtha.2014.02.005
253. Peng, CC, Cerretani, C, Braun, RJ, and Radke, CJ. Evaporation-driven instability of the precorneal tear film. Adv Colloid Interf Sci. (2014) 206:250–64. doi: 10.1016/j.cis.2013.06.001
254. Morgan, I, and Rose, K. How genetic is school myopia? Prog Retin Eye Res. (2005) 24:1–38. doi: 10.1016/j.preteyeres.2004.06.004
Keywords: exercise, physical activity, ocular diseases, oxidative stress, inflammation
Citation: Zhang Q, Jiang Y, Deng C and Wang J (2024) Effects and potential mechanisms of exercise and physical activity on eye health and ocular diseases. Front. Med. 11:1353624. doi: 10.3389/fmed.2024.1353624
Edited by:
Georgios D. Panos, Nottingham University Hospitals NHS Trust, United KingdomReviewed by:
Pan Long, Western Theater General Hospital, ChinaJe Hyun Seo, VHS Medical Center, Republic of Korea
Jeffrey Boatright, Emory University, United States
Yuanbo Liang, Affiliated Eye Hospital of Wenzhou Medical University, China
Copyright © 2024 Zhang, Jiang, Deng and Wang. This is an open-access article distributed under the terms of the Creative Commons Attribution License (CC BY). The use, distribution or reproduction in other forums is permitted, provided the original author(s) and the copyright owner(s) are credited and that the original publication in this journal is cited, in accordance with accepted academic practice. No use, distribution or reproduction is permitted which does not comply with these terms.
*Correspondence: Chaohua Deng, ZGVuZ2NoYW9odWExOTg4QGhvdG1haWwuY29t; Junming Wang, ZXllZHJ3am1AMTYzLmNvbQ==
†These authors have contributed equally to this work