- Department of Respiratory and Critical Medicine, The First Affiliated Hospital of Xi’an Jiaotong University, Xi’an, Shaanxi, China
Objective: Observational studies have reported that chronic obstructive pulmonary disease (COPD) is often accompanied by autoimmune diseases, but the causal relationships between them remain uncertain. In this Mendelian study, we aimed to investigate the potential causal relationship between COPD and four common autoimmune diseases.
Methods: We conducted an analysis of summary data on COPD and autoimmune disease using publicly available genome-wide association studies (GWAS) summary data. We initially employed the inverse- variance weighted method as the primary approach to establish the causal impact of COPD on autoimmune diseases in the sample and conducted additional sensitivity analyses to examine the robustness of the results. Subsequently, we performed reverse Mendelian randomization (MR) analyses for the four autoimmune diseases. Finally, the potential for bidirectional causal relationships was assessed.
Results: Our MR analysis revealed no significant causal relationship between COPD and any of the studied autoimmune diseases. However, reverse MR results indicated a significant association between rheumatoid arthritis (RA), osteoarthritis (OA) and the risk of developing COPD, with respective odds ratios (OR) of 377.313 (95% CI, 6.625–21487.932, P = 0.004) for RA and 11.097 (95% CI, 1.583–77.796, P = 0.015) for OA. Sensitivity analyses confirmed the robustness of the results.
Conclusion: Our findings support a potential causal relationship between autoimmune diseases and COPD, highlighting the importance of considering comorbidities in clinical management of COPD.
1 Introduction
Chronic Obstructive Pulmonary Disease (COPD) is a heterogeneous lung condition characterized by chronic respiratory symptoms (dyspnea, cough, sputum production) due to abnormalities of the airways (bronchitis, bronchiolitis) and/or alveoli (emphysema) that cause persistent, often progressive, airflow obstruction (1). It has evolved into a global health concern, based on the definition of GOLD, the global prevalence rate of COPD among people aged 30–79 in 2019 is 10.3%, about 392 million individuals (2). A 2018 study on adult lung health in China reported an overall COPD prevalence of about 8.6% in the adult population, with a significantly higher rate of 13.7% in adults aged 40 and older (3). The presence of comorbidities, such as autoimmune diseases, significantly exacerbates the course of COPD, yet the management of COPD comorbidities has not received adequate attention in clinical practice. Previous clinical investigations have indicated a reciprocal relationship between autoimmune diseases and the development of COPD, emphasizing the need for a comprehensive understanding of the causal relationship between COPD and autoimmune diseases to improve clinical diagnostics and treatment.
Autoimmune diseases refer to chronic diseases caused by the immune system’s response to self-tissue cells, resulting in cell damage or tissue injury. The pathogenesis of autoimmune diseases is complex. The overall incidence rate accounts for approximately 3–5% of the world’s population, with approximately 40 million people in China suffering from this disease. Female patients outnumber male patients, and autoimmune diseases have a high rate of disability and mortality (4). Although both COPD and autoimmune diseases are common, their co-occurrence has not received sufficient attention. Often, patients with autoimmune diseases who take immunosuppressive drugs to control their condition are more susceptible to infection, which can lead to acute exacerbations of COPD, ultimately imposing a heavy economic and social burden (5–7).
Mendelian randomization (MR) studies are increasingly being used to infer causal relationships between risk factors and disease outcomes. Observational studies are subject to confounding factors and reverse causality, while randomized controlled trials (RCTs) require extensive human resources and follow-up time. MR studies, however, can effectively exclude confounding factors and determine the causal relationship of specific outcomes, as genetic variations are randomly assigned at conception before the onset of disease. Currently, no study has used MR methods to investigate the causal relationship between autoimmune diseases and COPD. This study can provide theoretical support for the development and progression of COPD combined with autoimmune diseases, as well as for the pathogenesis, early detection, and prevention of autoimmune diseases with COPD.
2 Materials and methods
2.1 Data sources and study design
The GWAS data used in this magnetic resonance analysis are summarized statistical data obtained from published studies. The Assumptions and study design flowchart of the study is as Figure 1.
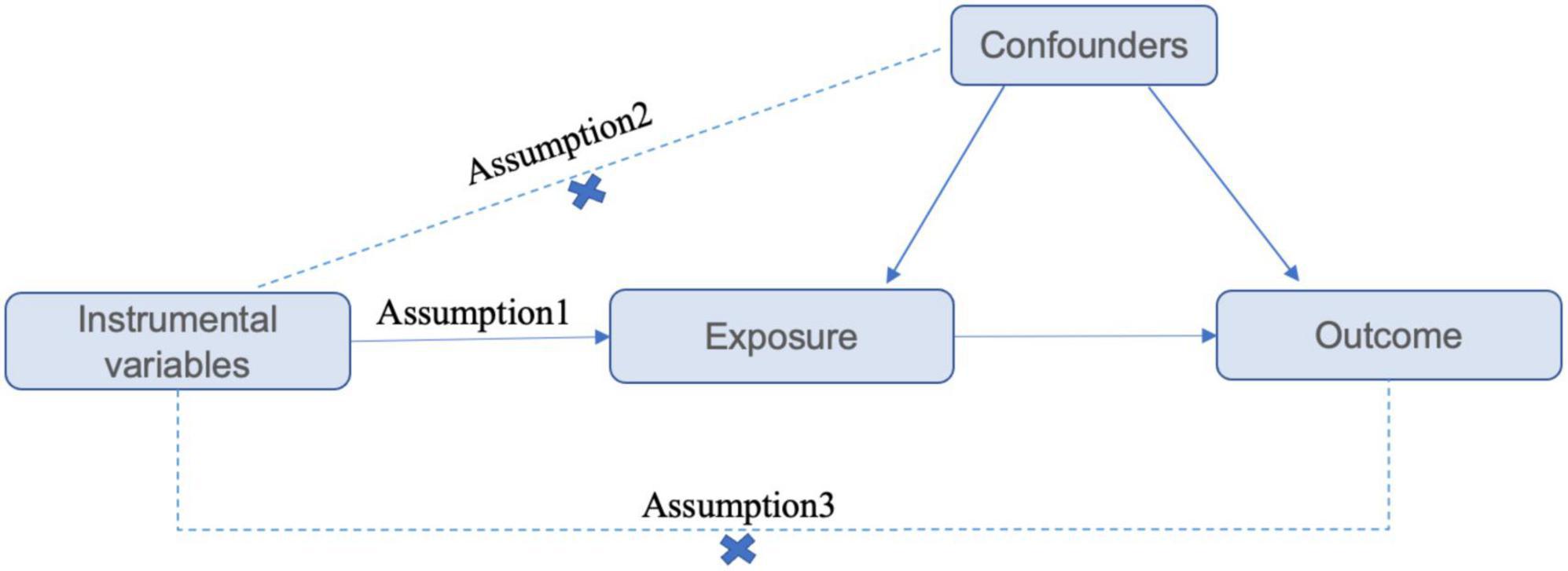
Figure 1. Assumptions and study design flowchart of the MR study. The MR method is based on 3 hypotheses: (1) instrumental variables directly affect the exposure; (2) instrumental variables are independent of any confounding factor; and (3) instrumental variables affect the results only via exposure but not through other pathways.
The FinnGen database1 was used for COPD summary data, including 18,266 cases and 311,286 controls, primarily of European descent.
The IEU database2 was used for autoimmune disease summary data, including 442 cases and 218,254 controls for systemic lupus erythematosus (SLE), 3,730 cases and 333,429 controls for rheumatoid arthritis (RA), 12,882 cases and 21,770 controls for inflammatory bowel disease (IBD), and 28,257 cases and 308,902 controls for osteoarthritis (OA). All samples were from individuals of European descent. Refer to Table 1.
2.2 Instrumental variable selection
To satisfy the first MR assumption, namely that the single nucleotide polymorphism (SNP) must be closely associated with the exposure factor, SNPs significantly associated with the exposure were selected at the genome-wide level (P < 5 × 10^–8, r2 < 0.001, genetic distance = 10,000 kb). If there were too few included SNPs, the criteria could be adjusted to (P < 5 × 10^–6, r2 < 0.001, genetic distance = 10,000 kb).
To ensure that the genetic variation is unrelated to potential confounders, which is the second MR assumption, a query was conducted in the Phenoscanner database to confirm that the included SNPs were unrelated to known confounders. In other words, instrumental variables should not influence the outcome through any other pathway besides exposure.
To meet the third MR assumption, this study employed the MR-Egger intercept test to examine the presence of horizontal pleiotropy between SNPs and the outcome factor (8). When the intercept of the regression line is non-zero, it indicates the presence of horizontal pleiotropy between instrumental variables and the outcome. Conversely, if the intercept is zero, there is no horizontal pleiotropy. Additionally, SNPs with palindromic alleles in the GWAS were excluded in the final calculation. Ultimately, SNPs significantly associated with the exposure factor were obtained as instrumental variables.
The F-statistic for instrumental variables was calculated to assess the strength of the instrumental variables (IVs) chosen in relation to the exposure. R2 represents the variance explained by the genetic instruments and is computed using the formula:
R2 = β2(1−EAF) × 2EAF, where EAF is the allele frequency of the mutant gene. The F-statistic is calculated using the formula:
(8, 9). Here, K is the number of SNP-exposure associations, and N is the sample size of the GWAS SNP-exposure association. SNPs with an F-statistic >10 were defined as reliable and effective IVs, which helps prevent weak instrument bias from affecting MR results (10).
2.3 Statistical analysis
In this study, two-sample MR analyses were primarily conducted using the Inverse Variance Weighted (IVW) method. In the absence of heterogeneity, a fixed-effects model was employed, while a random-effects model was used in the presence of heterogeneity (11). Additionally, MR-Egger regression and the Weighted Median method (WM) were utilized to complement the IVW results. Conventional inverse variance weighting analysis methods may be influenced by weak instrument bias or pleiotropy. Therefore, this study employed sensitivity analysis to assess the validity and robustness of IVW results. As the outcomes were binary variables, effect estimates were further transformed into OR to provide a more intuitive understanding of the relationship between exposure and outcome.
2.4 Sensitivity analysis
Various sensitivity analysis methods were employed in this study to ensure the stability of the results. First, Cochran’s Q-test assessed heterogeneity among individual SNP estimates and provided evidence for selecting the appropriate analysis method. If the p-value was greater than 0.05, indicating no heterogeneity, the fixed-effects IVW method was considered the primary approach; otherwise, a random-effects model was used. Secondly, the MR-Egger intercept method was used to detect horizontal pleiotropy of IVs. In MR-Egger testing, the intercept estimated the overall average horizontal pleiotropic effect of all SNPs. If the p-value was less than 0.05, there might be bias in IVW estimates. Additionally, MR-PRESSO was used to identify the presence of outliers and exclude them from the analysis (12). Finally, we conducted a leave-one-out sensitivity test to evaluate the influence of individual SNPs on causal effects. Furthermore, funnel plots and forest plots were generated to detect the presence of pleiotropy.
Statistical analysis was performed using the “TwoSample MR” and “MR-PRESSO” packages in R 4.2.0 software. All statistical tests were two-tailed, and significance was set at P < 0.05.
3 Result
3.1 Causal impact of COPD on autoimmune diseases
In this study, a total of 13 SNPs strongly associated with COPD were included. After excluding SNPs potentially related to confounding factors and palindromic SNPs, 11, 10, 10, and 10 SNPs were, respectively, selected for Mendelian randomization (MR) analyses concerning Systemic Lupus Erythematosus (SLE), Rheumatoid Arthritis (RA), Inflammatory Bowel Disease (IBD), and Osteoarthritis (OA). The F-statistics for all SNPs exceeded 10, indicating that the estimates were unlikely to be influenced by weak instrumental variable bias refer to Supplementary Table 1 for details.
Results of MR Analysis are Presented in Figure 2 and Supplementary Table 2. The findings indicate that in the IVW analysis, the genetically predicted COPD is not associated with the risk of any of the four autoimmune diseases. The results are consistent in the MR-Egger and WM analyses, and the MR-PRESSO analysis did not detect any outliers, which suggests that the results are robust and reliable (Supplementary Table 2). There was no evidence of heterogeneity among individual SNP estimates in the Cochran’s Q-test (Q = 11.388; P = 0.273), and no pleiotropy was observed in the MR-Egger regression analysis (Intercept = −0.034; P = 0.604; Supplementary Table 2). Furthermore, leave-one-out analysis demonstrates that the causal impact of COPD on the four autoimmune diseases is not driven by any single SNP (Supplementary Figure 1).
3.2 Causal impact of autoimmune diseases on COPD
In the reverse Mendelian randomization (MR) analysis investigating the causal impact of autoimmune diseases on COPD, we opted for 13 SNPs for SLE, 4 SNPs for RA, 49 SNPs for IBD, and 27 SNPs for OA as IVs, following the exclusion of palindromic and uninformative SNPs. All the selected SNPs exhibited F-statistics exceeding 10 to mitigate the potential bias stemming from weak instruments (Supplementary Table 3).
In the IVW analysis, we observed a positive association between the genetic susceptibility of RA and OA with COPD. The odds ratios (OR) for RA and OA were 377.313 (95% CI, 6.625–21487.932; P = 0.004) (Figures 2, 3 and Supplementary Table 4) And 11.097 (95% CI, 1.583–77.796; P = 0.015), respectively. Although the statistical significance was not pronounced in the MR-Egger method for the causal impact of OA on COPD and in the MR-Egger and WM methods for the causal impact of RA on COPD, these methods exhibited similar trends to the primary IVW method. Therefore, we consider these results reliable (Figures 4, 5 and Supplementary Table 4).
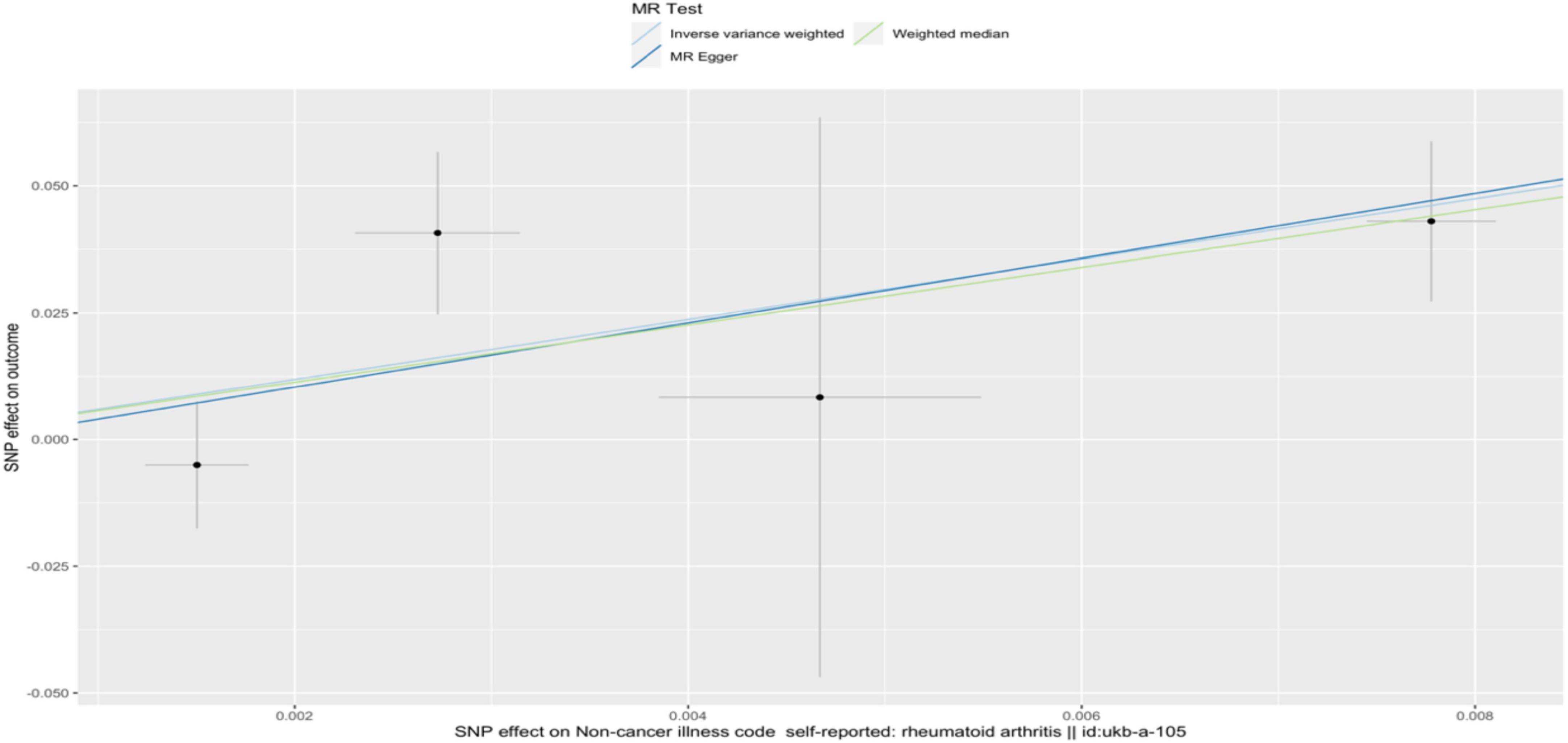
Figure 4. A scatterplot used to analyze the genetic causal relationship between RA and COPD. In the scatterplot, the x-axis represents the effect of individual SNPs on RA, and the y-axis represents the effect of individual SNPs on COPD. The colored diagonal line represents the fitted line for the causal effect estimation of RA on COPD. From the graph, it can be observed that as the risk of RA increases, the risk of COPD incidence also rises.
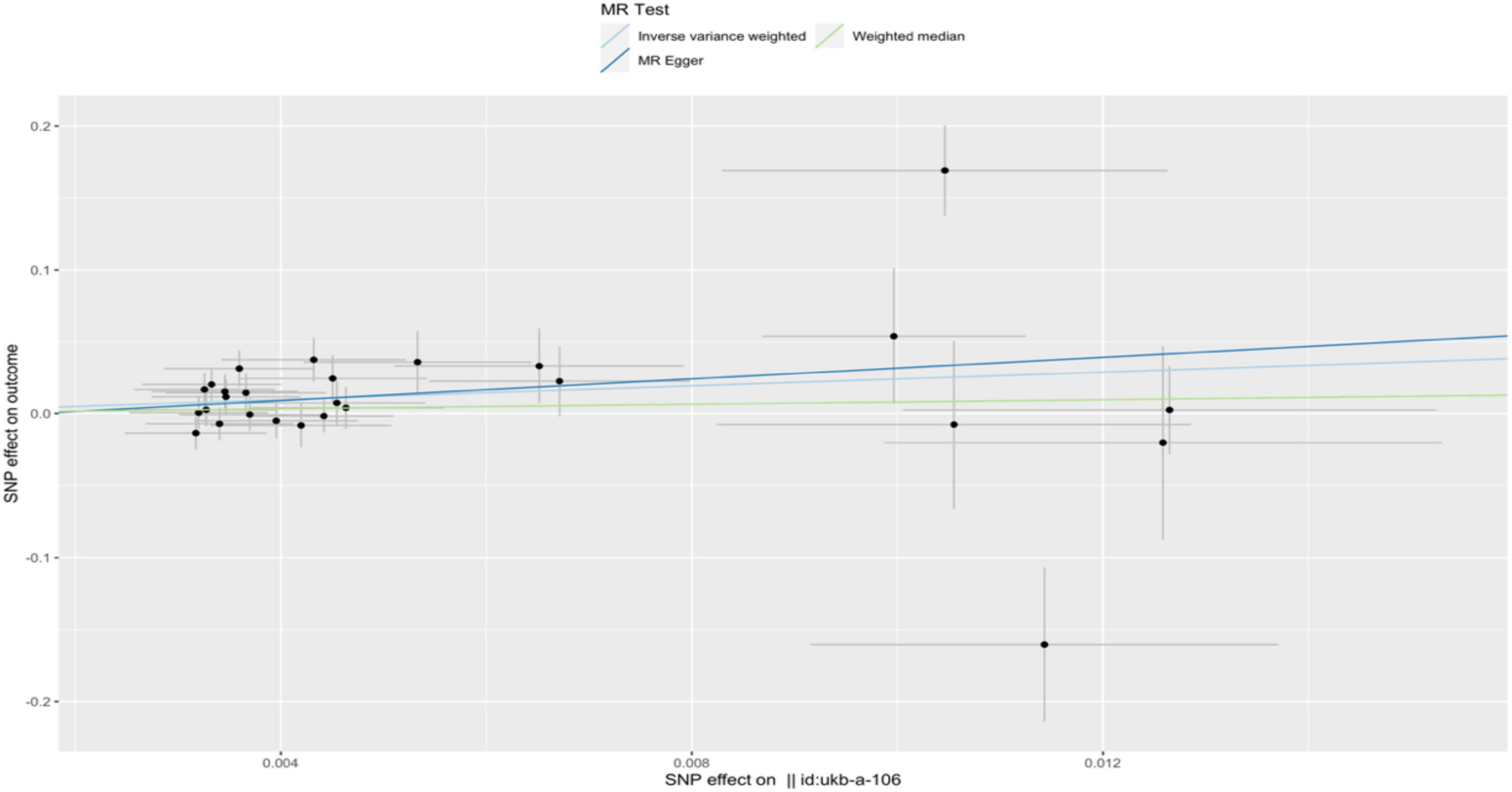
Figure 5. A scatterplot used to analyze the genetic causal relationship between OA and COPD. In the scatterplot, the x-axis represents the effect of individual SNPs on OA, and the y-axis represents the effect of individual SNPs on COPD. The colored diagonal line represents the fitted line for the causal effect estimation of OA on COPD. From the graph, it can be observed that as the risk of OA increases, the risk of COPD incidence also rises.
3.3 Sensitivity analysis
In sensitivity analysis, Cochran’s Q-test revealed significant heterogeneity in IBD and OA (PIBD = 1.62E-11; POA = 0.0003) (Supplementary Table 3). Consequently, the IVW random-effects model was employed for the analysis. MR-PRESSO analysis did not detect any outliers in SLE, RA, IBD, or OA (Supplementary Table 3). Furthermore, leave-one-out analysis indicated that the causal relationship between SLE, RA, IBD, or OA and COPD is not driven by any single SNP. MR funnel plots, leave-one-out analysis, and scatterplots can be found in Supplementary Figure 2.
4 Discussion
In this study, we conducted the first two-sample bidirectional MR analysis to investigate the potential causal relationship between COPD and autoimmune diseases. The results indicate no evidence of an association between COPD and SLE, RA, IBD, or OA. However, reverse MR analysis revealed a significant positive correlation between the genetic predisposition to RA and OA and an increased risk of COPD. Furthermore, the genetic susceptibility of SLE and IBD exhibited a negative association with COPD risk, although it was not statistically significant.
Obstructive pulmonary disease is characterized by persistent respiratory symptoms and airflow limitation, resulting from chronic airway inflammation, tissue destruction, and airflow obstruction. Increasing evidence suggests that autoimmune pathogenesis may play a vital role in the occurrence and development of COPD (13).
Observational studies have found a high correlation between RA and interstitial lung disease, as well as COPD. The incidence of COPD in RA patients has been reported to be significantly high in various studies (14), with a pooled analysis showing a COPD prevalence of 6.2% in RA (15). While smoking is considered a risk factor for both RA and COPD, studies have shown a high prevalence of COPD in RA patients even after adjusting for smoking (16, 17). Observational studies have also demonstrated a significant association between RA and the subsequent development of COPD (18), consistent with our findings of a causal effect of RA on COPD. Currently, there is no concrete evidence to explain the specific interplay between the development of both diseases when they coexist. Some studies suggest that the use of immunosuppressants is not significantly associated with the prognosis of COPD (19). However, other research suggests a correlation with the production of autoantigens and decreased immune tolerance in COPD patients, with a significant increase in serum autoantibody titers related to disease severity (20). There are also studies indicating overlapping pathogenic mechanisms between respiratory inflammation and autoimmune diseases, such as Granulomatosis with Polyangiitis patients showing false-positive COVID-19 antibody tests, possibly due to an excess of autoantibodies against cell antigens in patients with autoimmune diseases (21). Serum from COPD patients has been shown to produce autoantibodies that react with antigens known to be associated with autoimmune diseases, including RA (22). Further research is needed to investigate the potential mechanisms by which RA contributes to COPD.
A pooled analysis has shown a high prevalence of osteoarthritis (OA) in COPD patients, with an estimated overall prevalence of 35.5% for OA in COPD (23). Common risk factors between COPD and OA are believed to increase the likelihood of both diseases occurring simultaneously. The specific mechanisms by which OA functions in COPD may be related to systemic inflammatory mediators, reduced skeletal muscle function, and more (24, 25). Studies suggest that neutrophil elastase, which is associated with tissue destruction in inflammatory joint diseases, also plays a significant role in the pathogenesis of COPD (26).
Research indicates an increased risk of COPD in SLE patients (7). A large population study from Taiwan showed that the overall prevalence of chronic obstructive pulmonary disease was 1.73 times higher in SLE patients than in the control group (7). Population-based studies have found an increased incidence of IBD in COPD patients (27). However, these findings are inconsistent with our study results. The inconsistency may be due to the susceptibility of observational studies to the influence of confounding factors.
In conclusion, this bidirectional MR study investigated the causal relationship between COPD and autoimmune diseases, as well as the causal impact of autoimmune diseases on COPD. Further research is needed to explore and elucidate the biological mechanisms between COPD and autoimmune diseases to better understand and manage these diseases in clinical practice. Considering the potential positive association between autoimmune diseases and the risk of COPD, it is recommended to prioritize early diagnosis and prevention of COPD in populations with autoimmune diseases to provide better prognosis and quality of life for patients.
Our study has several strengths and limitations. Firstly, this is the first study to investigate the bidirectional causal relationship between COPD and autoimmune diseases using a two-sample MR, which is less susceptible to confounding factors and reverse causation compared to observational studies. We also conducted multiple sensitivity tests and IV strength assessments to ensure the robustness and validity of the results. However, the study has some limitations. First, our research is limited to the genetic level and cannot explore more specific phenotypes, such as the severity of COPD or acute exacerbations. Further research, such as randomized controlled trials (RCTs), is needed to help identify patients with specific immune responses to airway epithelial cell damage, combined with biomarkers beneficial for identifying and assessing patient conditions, such as red cell distribution width (RDW) as a prognostic marker for COPD patients (28), to advance toward precision medicine approaches. Secondly, the impact of clinical interventions cannot be determined through Mendelian randomization analysis. Lastly, this study primarily focused on individuals of European ancestry, which limits the generalizability of our findings to other populations. While we explored the genetic aspects of the relationship between COPD and autoimmune diseases, the underlying mechanisms remain unclear and require further investigation.
5 Conclusion
In conclusion, this MR study indicates a potential causal relationship between RA and OA and the risk of COPD. Based on our current research findings, we believe that screening for COPD in patients with RA and OA may be meaningful.
Data availability statement
The original contributions presented in the study are included in the article/Supplementary material, further inquiries can be directed to the corresponding author.
Ethics statement
All data sets provided by GWAS have been approved by the ethics committee related to the original research.
Author contributions
XY: Conceptualization, Methodology, Resources, Visualization, Writing – original draft, Writing – review and editing. XC: Data curation, Formal Analysis, Writing – review and editing. LV: Data curation, Formal Analysis, Writing – review and editing. NW: Software, Validation, Writing – review and editing. ML: Software, Validation, Writing – review and editing. WJ: Software, Validation, Writing – review and editing. TL: Software, Validation, Writing – review and editing. GW: Software, Validation, Writing – review and editing. TH: Supervision, Writing – review and editing. ZS: Funding acquisition, Supervision, Writing – review and editing.
Funding
The author(s) declare that no financial support was received for the research, authorship, and/or publication of this article.
Acknowledgments
We thank all investigators for making available their GWAS data. We also acknowledge the participants and investigators of the FinnGen study.
Conflict of interest
The authors declare that the research was conducted in the absence of any commercial or financial relationships that could be construed as a potential conflict of interest.
Publisher’s note
All claims expressed in this article are solely those of the authors and do not necessarily represent those of their affiliated organizations, or those of the publisher, the editors and the reviewers. Any product that may be evaluated in this article, or claim that may be made by its manufacturer, is not guaranteed or endorsed by the publisher.
Supplementary material
The Supplementary Material for this article can be found online at: https://www.frontiersin.org/articles/10.3389/fmed.2024.1331111/full#supplementary-material
Footnotes
References
1. Global Initiative for Chronic Obstructive Lung Disease. Global Strategy for the Diagnosis, Management, and Prevention of Chronic Obstructive Pulmonary Disease (2023 Report) [EB/OL]. (2023). Available online at: http://goldcopd.org/2023-gold-report-2/
2. Adeloye D, Song P, Zhu Y, Campbell H, Sheikh A, Rudan I, et al. Global, regional, and national prevalence of, and risk factors for, chronic obstructive pulmonary disease (COPD) in 2019: a systematic review and modelling analysis. Lancet Respir Med. (2022) 10:447–58. doi: 10.1016/S2213-2600(21)00511-7
3. Wang C, Xu J, Yang L, Xu Y, Zhang X, Bai C, et al. Prevalence and risk factors of chronic obstructive pulmonary disease in China (the China Pulmonary Health [CPH] study): a national cross-sectional study. Lancet. (2018) 391:1706–17. doi: 10.1016/S0140-6736(18)30841-9
4. Jones TF, McMillian MB, Scallan E, Frenzen PD, Cronquist AB, Thomas S, et al. A population-based estimate of the substantial burden of diarrhoeal disease in the United States; FoodNet, 1996-2003. Epidemiol Infect. (2007) 135:293–301. doi: 10.1017/S0950268806006765
5. Gergianaki I, Tsiligianni I. Chronic obstructive pulmonary disease and rheumatic diseases: a systematic review on a neglected comorbidity. J Comorb. (2019) 9:2235042X18820209. doi: 10.1177/2235042X18820209
6. Han GM, Han XF. Comorbid conditions are associated with emergency department visits, hospitalizations, and medical charges of patients with systemic lupus erythematosus. J Clin Rheumatol. (2017) 23:19–25. doi: 10.1097/RHU.0000000000000437
7. Shen TC, Lin CL, Chen CH, Tu CY, Hsia TC, Shih CM, et al. Increased risk of chronic obstructive pulmonary disease in patients with systemic lupus erythematosus: a population-based cohort study. PLoS One. (2014) 9:e91821. doi: 10.1371/journal.pone.0091821
8. Bowden J, Davey Smith G, Burgess S. Mendelian randomization with invalid instruments: effect estimation and bias detection through egger regression. Int J Epidemiol. (2015) 44:512–25. doi: 10.1093/ije/dyv080
9. Burgess S, Thompson SG, CRP CHD Genetics Collaboration. Avoiding bias from weak instruments in Mendelian randomization studies. Int J Epidemiol. (2011) 40:755–64. doi: 10.1093/ije/dyr036
10. Gao N, Kong M, Li X, Wei D, Zhu X, Hong Z, et al. Systemic lupus erythematosus and cardiovascular disease: a Mendelian randomization study. Front Immunol. (2022) 13:908831. doi: 10.3389/fimmu.2022.908831
11. Lawlor DA, Harbord RM, Sterne JAC, Timpson N, Davey Smith G. Mendelian randomization: using genes as instruments for making causal inferences in epidemiology. Stat Med. (2008) 27:1133–63. doi: 10.1002/sim.3034
12. Verbanck M, Chen CY, Neale B, Do R. Detection of widespread horizontal pleiotropy in causal relationships inferred from Mendelian randomization between complex traits and diseases. Nat Genet. (2018) 50:693–8. doi: 10.1038/s41588-018-0099-7
13. Feghali-Bostwick CA, Gadgil AS, Otterbein LE, Pilewski JM, Stoner MW, Csizmadia E, et al. Autoantibodies in patients with chronic obstructive pulmonary disease. Am J Respir Crit Care Med. (2008) 177:156–63. doi: 10.1164/rccm.200701-014OC
14. Matson SM, Demoruelle MK, Castro M. Airway disease in rheumatoid arthritis. Ann Am Thorac Soc. (2022) 19:343–52. doi: 10.1513/AnnalsATS.202107-876CME
15. Ma Y, Tong H, Zhang X, Wang M, Yang J, Wu M, et al. Chronic obstructive pulmonary disease in rheumatoid arthritis: a systematic review and meta-analysis. Respir Res. (2019) 20:144. doi: 10.1186/s12931-019-1123-x
16. Nannini C, Medina-Velasquez YF, Achenbach SJ, Crowson CS, Ryu JH, Vassallo R, et al. Incidence and mortality of obstructive lung disease in rheumatoid arthritis: a population-based study. Arthritis Care Res (Hoboken). (2013) 65:1243–50. doi: 10.1002/acr.21986
17. Sparks JA, Lin TC, Camargo CA Jr., Barbhaiya M, Tedeschi SK, Costenbader KH, et al. Rheumatoid arthritis and risk of chronic obstructive pulmonary disease or asthma among women: a marginal structural model analysis in the Nurses’ Health Study. Semin Arthritis Rheum. (2018) 47:639–48. doi: 10.1016/j.semarthrit.2017.09.005
18. Sparks JA, Chang SC, Liao KP, Lu B, Fine AR, Solomon DH, et al. Rheumatoid arthritis and mortality among women during 36 years of prospective follow-up: results from the Nurses’ Health Study. Arthritis Care Res (Hoboken). (2016) 68:753–62. doi: 10.1002/acr.22752
19. Hudson M, Dell’Aniello S, Shen S, Simon TA, Ernst P, Suissa S. Comparative safety of biologic versus conventional synthetic DMARDs in rheumatoid arthritis with COPD: a real-world population study. Rheumatology (Oxford). (2020) 59:820–7. doi: 10.1093/rheumatology/kez359
20. Caramori G, Ruggeri P, Di Stefano A, Mumby S, Girbino G, Adcock IM, et al. Autoimmunity and COPD: clinical implications. Chest. (2018) 153:1424–31. doi: 10.1016/j.chest.2017.10.033
21. Tzouvelekis A, Karampitsakos T, Krompa A, Markozannes E, Bouros D. False positive COVID-19 antibody test in a case of granulomatosis with polyangiitis. Front Med (Lausanne). (2020) 7:399. doi: 10.3389/fmed.2020.00399
22. Packard TA, Li QZ, Cosgrove GP, Bowler RP, Cambier JC. COPD is associated with production of autoantibodies to a broad spectrum of self-antigens, correlative with disease phenotype. Immunol Res. (2013) 55:48–57. doi: 10.1007/s12026-012-8347-x
23. Wshah A, Guilcher SJ, Goldstein R, Brooks D. Prevalence of osteoarthritis in individuals with COPD: a systematic review. Int J Chron Obstruct Pulmon Dis. (2018) 13:1207–16. doi: 10.2147/COPD.S158614
24. Schols AM, Buurman WA, Staal van den Brekel AJ, Dentener MA, Wouters EF. Evidence for a relation between metabolic derangements and increased levels of inflammatory mediators in a subgroup of patients with chronic obstructive pulmonary disease. Thorax. (1996) 51:819–24. doi: 10.1136/thx.51.8.819
25. Kim HC, Mofarrahi M, Hussain SN. Skeletal muscle dysfunction in patients with chronic obstructive pulmonary disease. Int J Chron Obstruct Pulmon Dis. (2008) 3:637–58. doi: 10.2147/copd.s4480
26. Wright HL, Moots RJ, Bucknall RC, Edwards SW. Neutrophil function in inflammation and inflammatory diseases. Rheumatology (Oxford). (2010) 49:1618–31. doi: 10.1093/rheumatology/keq045
27. SRaftery AL, Tsantikos E, Harris NL, Hibbs ML. Links between inflammatory bowel disease and chronic obstructive pulmonary disease. Front Immunol. (2020) 11:2144. doi: 10.3389/fimmu.2020.02144
Keywords: COPD, autoimmune diseases, Mendelian randomization, causality, genome-wide association study
Citation: Yu X, Cheng X, Lv L, Wang N, Li M, Ji W, Liu T, Wang G, Hu T and Shi Z (2024) The association between chronic obstructive pulmonary disease and autoimmune diseases: a bidirectional Mendelian randomization study. Front. Med. 11:1331111. doi: 10.3389/fmed.2024.1331111
Received: 01 November 2023; Accepted: 19 February 2024;
Published: 05 March 2024.
Edited by:
Sairam Parthasarathy, The University of Arizona, United StatesReviewed by:
Ourania Papaioannou, General University Hospital of Patras, GreeceTheodoros Karampitsakos, University of South Florida, United States
Copyright © 2024 Yu, Cheng, Lv, Wang, Li, Ji, Liu, Wang, Hu and Shi. This is an open-access article distributed under the terms of the Creative Commons Attribution License (CC BY). The use, distribution or reproduction in other forums is permitted, provided the original author(s) and the copyright owner(s) are credited and that the original publication in this journal is cited, in accordance with accepted academic practice. No use, distribution or reproduction is permitted which does not comply with these terms.
*Correspondence: Zhihong Shi, docszh@163.com