- 1Department of Anesthesiology, West Virginia University, Morgantown, WV, United States
- 2Division of Health, Science, and Technology, Howard Community College, Columbia, MD, United States
- 3University of Texas at Southwestern, Dallas, TX, United States
- 4Department of Anesthesiology & Critical Care Medicine, Johns Hopkins School of Medicine, Baltimore, MD, United States
- 5Howard University College of Medicine, Washington, DC, United States
- 6Advocate Aurora Health, Downers Grove, IL, United States
- 7Johns Hopkins University School of Medicine, Baltimore, MD, United States
- 8University of Maryland School of Medicine, Baltimore, MD, United States
Introduction: Intravenous (IV) therapy is a crucial aspect of care for the critically ill patient. Barriers to IV infusion pumps in low-resource settings include high costs, lack of access to electricity, and insufficient technical support. Inaccuracy of traditional drop-counting practices places patients at risk. By conducting a comparative assessment of IV infusion methods, we analyzed the efficacy of different devices and identified one that most effectively bridges the gap between accuracy, cost, and electricity reliance in low-resource environments.
Methods: In this prospective mixed methods study, nurses, residents, and medical students used drop counting, a manual flow regulator, an infusion pump, a DripAssist, and a DripAssist with manual flow regulator to collect normal saline at goal rates of 240, 120, and 60 mL/h. Participants’ station setup time was recorded, and the amount of fluid collected in 10 min was recorded (in milliliters). Participants then filled out a post-trial survey to rate each method (on a scale of 1 to 5) in terms of understandability, time consumption, and operability. Cost-effectiveness for use in low-resource settings was also evaluated.
Results: The manual flow regulator had the fastest setup time, was the most cost effective, and was rated as the least time consuming to use and the easiest to understand and operate. In contrast, the combination of the DripAssist and manual flow regulator was the most time consuming to use and the hardest to understand and operate.
Conclusion: The manual flow regulator alone was the least time consuming and easiest to operate. The DripAssist/Manual flow regulator combination increases accuracy, but this combination was the most difficult to operate. In addition, the manual flow regulator was the most cost-effective. Healthcare providers can adapt these devices to their practice environments and improve the safety of rate-sensitive IV medications without significant strain on electricity, time, or personnel resources.
1 Introduction
Intravenous (IV) therapy is used to achieve homeostatic balance and is crucial to the care of critically ill patients. Intravenous fluids are required to maintain or increase cardiac output vital for tissue perfusion and for the sedation necessary to promote healing during admission to an intensive care unit (ICU) (1). Yet, excessive administration or under-delivery of fluids or medications can result in complications that contribute to morbidity and mortality (2). Furthermore, accuracy, cost, and the perspectives of healthcare workers with respect to the range of IV therapies available are still minimally explored in the low-resource setting.
In critically ill patients with hemodynamic instability, such as with distributive shock, appropriate fluid balance is crucial. Aggressive fluid administration can result in volume overload and worsen the patient’s condition (3–6). Similarly, sedation overdosage and underdosage can negatively affect health measures such as the depth of sedation for ventilator use or successful pain management to reduce hospital stay. Accurate infusion methods can improve recovery outcomes in the ICU and reduce hospital stay (7). Although different IV delivery methods may have different levels of accuracy, additional factors must be considered for low-resource environments.
Accuracy in delivering IV fluids and other medications is achieved by using infusion pumps and other volumetric devices. Many of these devices are expensive and require electricity. Most available infusion pumps range in price from $1,200 to $4,000 each (8) and may be prohibitively expensive in low-resource settings. To overcome these financial barriers, other devices may be considered.
Health providers in low-resource settings usually resort to drop-counting, which relies on raising the IV bag above a patient to increase the hydrostatic pressure to overcome the vein’s peripheral pressure, and observing the flow rate by counting the number of drops per minute (9). Inaccuracy of traditional drop-counting practices places patients at risk for complications that may increase morbidity and mortality (9).
The manual flow regulator is a circular device that allows the healthcare provider to manually set the flow rate in milliliters per hour (10). This device is advantageous because it facilitates a consistent infusion rate, does not require electricity or batteries, and costs $4.00 U.S. dollars (USD). A disadvantage, however, is that the accuracy may not approach that of the IV infusion pump.
A portable device known as the DripAssist has been shown in 2 studies to be accurate and can be used in low-resource settings such as prehospital and military medicine (11, 12). The DripAssist was developed to administer IV fluid infusions in low-resource areas at a low cost with no electricity requirements (11, 12). Each DripAssist device is priced at $400 USD and requires one AA battery. Though the DripAssist provides a low-cost option for achieving accuracy in low-resource settings, it is very sensitive to any movement.
According to the current literature, accuracy, precision, and setup time are crucial considerations when comparing medical devices (5). In low-resource environments, such as India and Senegal, both overall costs and running considerations, such as electricity reliance, should be considered (13–17). The perceptions of healthcare workers are additionally important when it comes to the use of devices (18, 19). In this prospective mixed methods comparative study, we aimed to compare the different methods available for IV infusions that require close volume and rate control. To this end, we (1) compared the accuracy, precision, and setup time over 3 drip goals of 5 IV infusion methods, (2) evaluated healthcare worker perception of these options, and finally (3) conducted a cost-effectiveness analysis of the devices. The ultimate goal of this analysis is to identify a device that bridges the gap between accuracy, cost, and electricity reliance for IV infusion methods in low-resource settings.
2 Methods
This study was a mixed-methods prospective trial and a cost-effectiveness analysis to compare IV infusion devices in terms of their use in 2 countries, Senegal and India. It was conducted at the Johns Hopkins University School of Medicine and Howard Community College and was approved by the Institutional Review Boards at both institutions (IRB00254064 and HCC − 2021-08-25, respectively). This approval included special permission to conduct research with institutional employees and special permission from the nursing department leadership to recruit nurses. Participants were informed about the study through a detailed consent form, which was signed prior to participation.
2.1 Participant recruitment
The study included a set of local health nurse, resident, and medical student volunteers. Inclusion criteria for participants included age ≥ 18 years, training or practicing with a minimum of a registered nurse (RN) license for nurses, a medical degree for resident trainees in the workforce, or enrollment in a U.S. medical school for medical students. Sample size determination was based on sufficient numbers needed for usability studies (at least 30 for quantitative analysis) (20). No patients were involved in the study. For this study, recruitment was targeted at local nurses who could participate at either the Johns Hopkin Hospital site or the Howard Community College site (both in Maryland) because nurses are typically responsible for administering the proper IV infusion rate prescribed by the doctor. Medical students and residents were also recruited to the study because residents are also part of the workforce in low-resource settings and because we wanted to investigate whether medical students with no experience could easily learn the IV infusion setup.
Exclusion criteria included nurses with less than an RN level of education, and undergraduate (pre-health) students.
Electronic flyers were created to advertise the study and to recruit participants. Participants were scheduled in advance or recruited onsite on the day of participation. Prior to the experiment, participants had the option of watching a demonstration of each IV infusion method through a series of videos made by the researchers. They also had the option of observing a 5-min, live, in-person didactic and demonstration session of each IV infusion at the time of registration. Incentives for participation included a free designer Risen Regalia facemask, a chance to win an Amazon or Visa gift card in a raffle, or a hospital cafeteria gift card.
2.2 Study design
Healthcare nurses and trainee volunteers were asked to operate 5 different IV infusion methods (Figure 1). Each method was performed at one of 5 stations: manually counting drops (station 1), using an IV manual flow regulator (station 2), using an Alaris IV infusion pump: BD Alaris™ Pump Module (station 3), using the DripAssist device: DripAssist Infusion Rate Monitor from Shiftlabs (station 4), and using the DripAssist device and the IV manual flow regulator together (station 5). Each station had 1 to 3 IV bags, an IV pole, an infusion set, timers, and 3 graduated cylinders. The IV bags contained saline solution, and all the parameters, including IV fluid bag, fluid type, and fluid viscosity, were kept the same to avoid confounding bias. Study team members were aware that 1 mL in different solutions could lead to different drop count measurements; thus, IV saline was the only IV fluid used in the study.
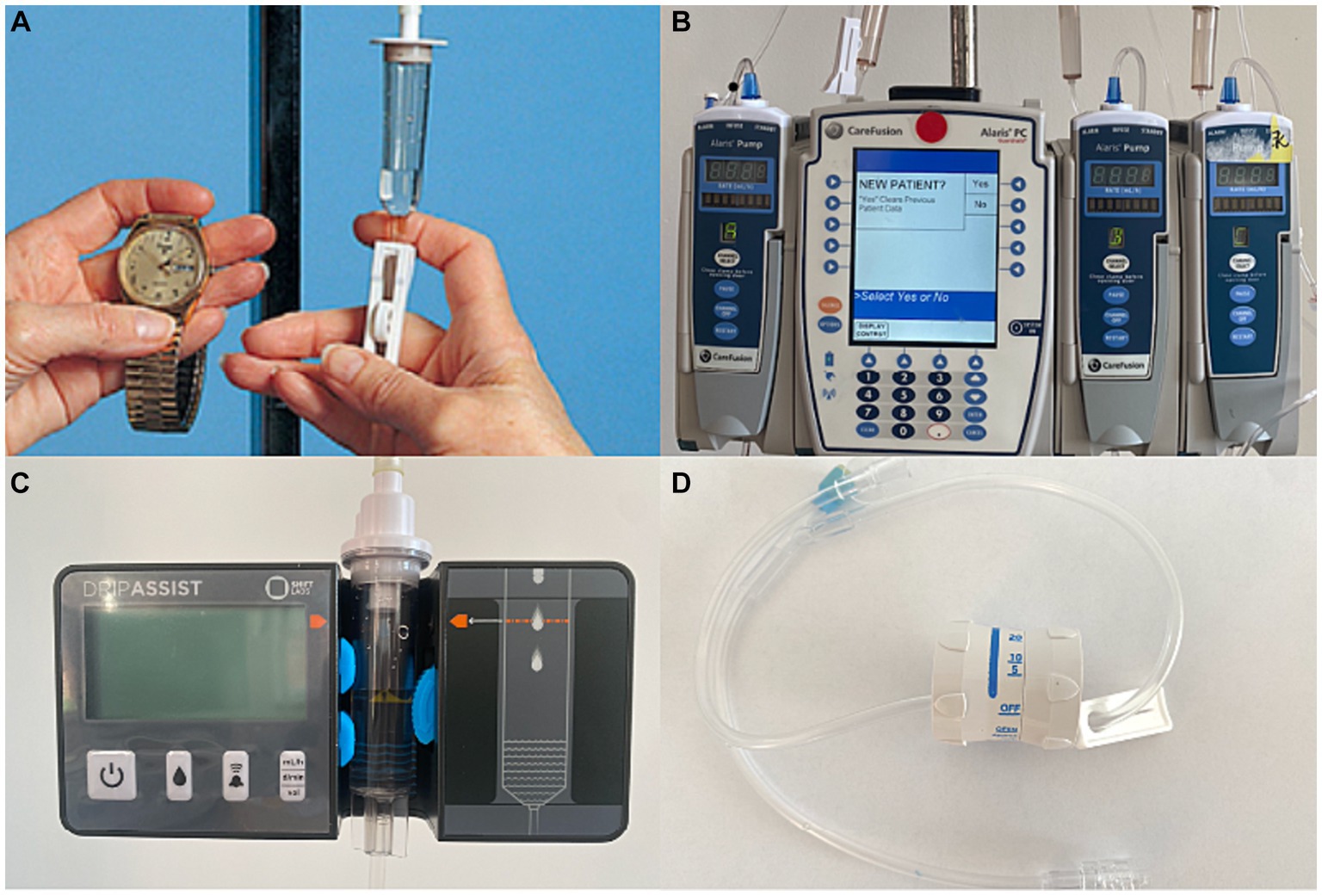
Figure 1. Intravenous (IV) infusion methods. (A) Drop counting. This method is the most traditional way of delivering infusions in low-resource settings. Gravity is used as the driving force to administer the IV fluid through tubing with a drip chamber. (B) IV infusion pump. The Alaris IV infusion pump is a relatively expensive device that administers fluid in controlled amounts using a built-in software interface. (C) The DripAssist is a small, lightweight device that operates with one AA battery. The rate of fluid infused is automatically monitored by the device itself. The DripAssist device also uses an alarm technology that alerts the user of a significant change in drip rate. It provides a display of the rate as milliliters/h, drops/min, or total milliliters. (D) The manual flow regulator is a simple cylindrical device that is attached to the IV tubing and allows the user to set the flow to a constant rate. This device may make the infusion process easier, although the drip rate must be monitored occasionally.
For accurate volume measurements, the fluid was collected in a 250 mL graduated cylinder clamped to a stand.
2.3 Data collection
Each data collector was trained on how to operate equipment at each station and how to demonstrate each station to study participants. We employed beta-testing with 10 participants to refine our recruitment, enrollment, equipment use, and data collection methods. These participants were not reflected in the participant demographics or data analysis. Outcomes included station setup time, drip rate accuracy, drip rate precision, and cost of using these devices in an acute care setting. Participants completed a questionnaire after the trial in which they were asked to rate the operability, understandability, and perceived time consumption of each method on a 5-point Likert scale. Participants also had the option to leave qualitative comments on the survey, which we separately analyzed for common themes.
The accuracy and precision of each device was measured by comparing drip rates. The participants were asked to set each device to a specific drip rate: 240, 120, and 60 mL/h. After 10 min, the researcher measured the total volume collected in the cylinder and calculated the drip rate with the following formula:
Study team members were aware that 1 mL in different solutions could indicate a different drop count; thus, IV saline was the only IV fluid used in the study.
The accuracy of the setup was independently determined by 2 researchers, who measured the volume of the fluid collected in the cylinder after 10 min. The difference between expected and calculated drip rate determined the accuracy level. The range of the standard deviation determined the precision, with a wider standard deviation demonstrating lower precision and a narrower standard deviation showing higher precision.
Setup time was recorded with a stopwatch and included the time to determine the given flow rate until the time that the participant was satisfied with the setup and informed the researcher. Setup time did not include the time to set up the bag (which was measured separately). We averaged and reported the bag setup times for those who set up the bag before setting up the infusion rate.
To accurately represent cost of care in an ICU setting, 2 investigators independently collected data about cost using public health resources in Senegal and India (21–25).
2.4 Cost-effectiveness modeling in Senegal and India
To determine the cost-effectiveness of the infusion methods in low-resources settings, we created a model of a hypothetical ICU in India and Senegal in which the methods would be deployed. The model assumed that a patient would enter the ICU and receive intravenous sedation therapy for 3 days. The cost measures were the costs of materials for each infusion method, time/hly wages for nurses and physicians, hospital stay, days on ventilator, and costs of sedatives (midazolam and fentanyl) at a rate of 10 mL/h (Table 1). We also made the assumption that the accuracy of the infusion method would be reflected in a patient’s sedation score. A traditional Richmond-Agitation Sedation Scale (RASS) (26) ranges from +4 to −5. To make mathematical calculations and comparisons between the infusion methods possible, corresponding numerical equivalents (ranging from 1 to 10) were substituted for each RASS score (Table 2).
Cost-effectiveness of the infusion methods was compared by using the incremental cost-effectiveness ratio (ICER). ICER is calculated by dividing the difference in total cost (incremental cost) by the difference in the chosen measure of health outcome or effect (incremental effect) to provide a ratio of “extra cost per extra unit of health effect” for the more expensive therapy versus the alternative. Suboptimal sedation in our model would be associated with lower quality-adjusted life years (QALY) (27). Therefore, we used QALY as our health effect in the calculation of ICER.
2.5 Data analysis
Quantitative variables were compared by a 2-way ANOVA, testing with replication for all different devices. ANOVA testing was completed with WINKS WDA 7.0.9 (Texasoft, Dallas TX). The confidence level for hypothesis testing is 95%, and the α level is 0.05. For each dataset, the F-distribution and p value were determined. ANOVA was used for station setup time.
Kruskal Wallis tests were used to assess differences between types of practitioners and for setup time, flow rate accuracy, and participant ratings of each station. The Newman–Keuls multiple comparison test was used to assess statistical significance between stations for setup time and participant ratings. The statistical software used was SAS version 9.4 (SAS institute, Cary, NC).
For the qualitative, open-ended responses in the survey, data were analyzed by noting themes using content analysis as determined by 2 independent researchers.
3 Results
The study included 54 nurse, resident, and medical student volunteers (Table 3; Figure 2). Most participants were nurses, and the average number of years in practice was less than 10 but varied by vocation. The study included health professionals with diverse backgrounds, including previous experiences in low-resource settings around the world.
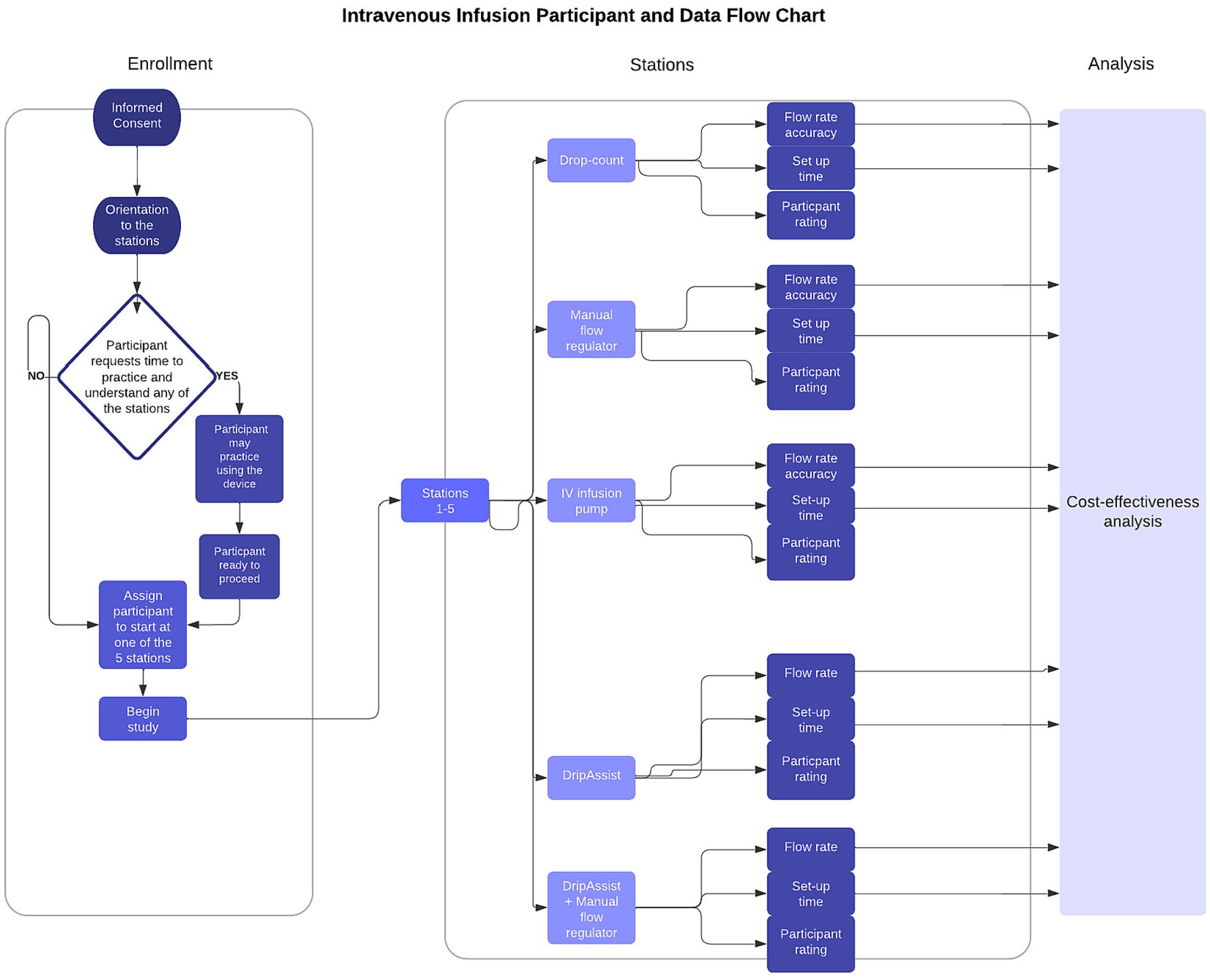
Figure 2. Study participant and data flow chart. Fifty-four participants (n = 54) enrolled and completed the study at all 5 stations and filled out a survey to rate each station. Data collected from participant flow rate accuracy and participant set-up time were used for cost-effectiveness analysis.
3.1 Accuracy and precision of the infusion methods
A comparison of the results from all 5 stations showed no statistically significant differences in accuracy at various flow rates of 240 mL/h (p = 0.878), 120 mL/hour (p = 0.093), and 60 mL/h (p = 0.105; Table 4). At the highest infusion rate, the infusion pump had the greatest accuracy of flow, with a mean (SD) of 236 (21.7) mL/h, but this value was not statistically significant from that at other stations. The infusion pump and the manual flow regulator (226 [49.0] mL/h) appeared to have the highest precision.
When we analyzed the results based on residents and nurses alone, we found that at 240 mL/h, the differences in stations were significant (p = 0.03). In that analysis, the Alaris infusion pump’s accuracy was similar to that of the DripAssist and the DripAssist combination stations, but it was significantly more accurate than drop counting and the manual flow regulator methods.
3.2 Setup times
Setup time differed significantly between stations (p < 0.001); the manual flow regulator was the least time consuming (mean [SD], 71 [56.2] seconds), and the combined DripAssist station was the most time consuming (365 [291.2] seconds; Table 5). Setup time for the manual flow regulator was not significantly different from that of the infusion pump, which had the second fastest time of 116 (77.9) seconds. The drop-counting method (225 [187.2] seconds) was the third fastest station to set up and was significantly different from the other 4 stations. The DripAssist station and the combined manual flow regulator and DripAssist station took the most time to set up (352 [240.1] seconds and 365 [291.2] seconds, respectively), but they were not significantly different from one another. Setup time for drop counting was significantly different between types of practitioners (p = 0.001), with nurses taking significantly less time than others.
The mean bag setup time was 100 s for those who set up the bag before setting up the infusion rate.
3.3 Participant ratings of the infusion methods
Ratings of understandability, operability, and perceived time consumption of the infusion methods differed significantly between stations (p < 0.001). The manual flow regulator was considered to be the most operable, most understandable, and least time consuming, and the DripAssist stations were considered the most time consuming (Table 5).
Understandability also varied among stations (p < 0.001). The drop-counting method and the manual flow regulator (stations 1 and 2) were rated the easiest to understand (mean [SD], 4.5 [0.92] and 4.8 [0.57], respectively) but were not statistically different from each other. The Alaris infusion pump and the DripAssist stations were the next easiest to understand (4.0 [1.2] and 3.8 [1.1], respectively) but were not statistically different from each other. The combined DripAssist and manual flow regulator station was the most difficult to understand (3.5 [1.3]) but was not statistically different from the DripAssist alone.
Analysis of operability (p < 0.001) showed that the manual flow regulator was the most operable device (4.7 [0.73]) by a statistically significant margin. The Alaris infusion pump was the second most easy station to operate (4.2 [1.1]), and this again was statistically different from other methods. Stations 1, 4, and 5 were the most difficult to operate (3.1 [1.6], 2.8 [1.2], and 3.0 [1.2], respectively) and were not statistically different from each other.
For the perceived time-consuming nature of the stations (p < 0.001), the manual flow regulator (station 2) was rated as the least time consuming (1.2 [0.58]), and this was statistically significant. The Alaris infusion pump (station 3) was perceived as the next least time-consuming device (1.7 [0.91]) and was also statistically significant. Stations 1, 4, 5 were rated as being the most time consuming, and the differences between these 3 were not statistically significant (3.3, [1.4], 3.7 [1.2], 3.4 [1.2], respectively).
3.4 Content analysis of participant survey comments
Several common themes emerged from participants’ responses: perceived accuracy, time-consuming nature of method, willingness to use in low-resource settings, favorites, and suggestions for implementation in low-resource settings.
3.4.1 Perceived accuracy
Participants thought that the manual flow regulator was less accurate than other methods because the flow rates required estimates that were not on the device. For example, one participant mentioned that “the dial needs to be more accurate. Cannot tell if it is set up to 240. Numbers go from 200 to 250.” Perceptions were mixed regarding whether the DripAssist was more accurate than drop counting. Although one participant preferred drop counting because the DripAssist “takes so long to set,” another participant preferred the DripAssist, “especially in critical care situations in which the rate needs to be very accurate - it’s better than nothing. However if I was just infusing fluids, I might just drop count.”
3.4.2 Time consuming
Participants found the 2 DripAssist stations to be the most time consuming. One participant remarked that it was “painful,” and another pointed out that it “requires no movement,” because the device is motion-sensitive during setup. Yet another participant wrote, “I wanted to like the DripAssist, and it was more convenient than counting drops, but it is also time consuming trying to get the exact number. It’s easier for a nurse to come by and do a quick check” on the flow rate “to make sure the rate has not changed due to patient movement compared to drop count.” In comparison with other methods, “the Alaris was the fastest but using the DripAssist and manual regulator together were easy to operate and not that time consuming with practice.” Although most participants thought that the manual flow regulator alone was least time consuming, participants who liked the combination station thought that more experience with the DripAssist could decrease the time for setup and allow the accuracy to approach that of the Alaris infusion pump.
3.4.3 Willingness to use in low-resource settings
Many participants stated that all of the devices could be used in a low-resource setting and that they can help improve the safety of medications administered. For example:
- “If people are taught” and oriented to the new technologies, such implementation “will be a great way to learn and it will be useful in a low-resource setting.”
- These various technology methods “will help patients get more medications in a safe and timely manner.”
3.4.4 Favorites
Participants had a variety of favorites among the 5 stations for different reasons. Manual drop counting was favored because it was the “most fun.” The manual flow regulator was favored because it was not as motion-sensitive as the DripAssist and was “not bad.” The DripAssist station was favored, with reservation, because participants “would have loved this in the ICU,” but “there may be a lot of [number] rounding.” The combination of the DripAssist and manual flow regulator was favored by some because it “made me feel more confident.” The combination station was the least favorite for some participants because “positioning is key for the DripAssist” and it was “overwhelming because you have to go back and forth between devices.”
3.4.5 Suggestions for implementation in low-resource settings
Participants’ suggestions for the manual flow regulator and the DripAssist ranged from improving efficiencies with the flow of patient care to using one infusion method as a backup to the other. The following are the most noteworthy feedback points gathered from the participants:
- Caution is recommended with “checking and changing tubing,” as this is one of the most important steps.
- Because of its motion sensitivity, “changes in gravity (higher/lower IV bag) affect the DripAssist” accuracy measurements.
- One suggestion targeted cost savings with DripAssists. The participant recommended running the DripAssist “to spot check-- like after running for a few minutes.” This use could allow low-resource settings to purchase fewer DripAssists.
- Another participant further suggested that in the healthcare setting, the staff could “just undershoot the rate and then supplement if necessary” as another cost-saving measure.
- A concern was raised with the manual flow regulator with regard to “[changing] the device for each patient.”
3.5 Cost-effectiveness analysis
The results of our study indicated that all infusion methods reached levels of accuracy that were comparable to the gold standard of the IV infusion pump (p = 0.8). Therefore, each method can achieve an optimal sedation state to reduce in-hospital mortality and increase quality of life. Therefore, we assigned QALY = 1 for all methods. The infusion pump would be the most expensive method in both Senegal and India, and the manual flow regulator would be the least expensive (Table 6). Therefore, the incremental cost-effectiveness ratio, or ICER, helps to relate the cost to the effectiveness of the type of IV method as illustrated below. A negative ICER indicates cost savings.
Comparison of manual flow regulator to drop counting.
ICER = 409.56–411.77/1 QALY = −$2.21 per QALY in Senegal.
ICER = 113.26–110.04/1 QALY = $3.22 per QALY in India.
Comparison of DripAssist to drop counting.
ICER = 707.76–411.77/1 QALY = $295.99 per QALY in Senegal.
ICER = 410.57–110.04/1 QALY = $300.53 per QALY in India.
Comparison of DripAssist/manual flow regulator to drop counting.
ICER = 711.85–411.77/1 QALY = $300.08 per QALY in Senegal.
ICER = 414.63–110.04/1 QALY = $304.59 per QALY in India.
Comparison of IV infusion pump to DripAssist.
ICER = 707.76–2404.92/1 QALY = −1697.16 per QALY in Senegal.
ICER = 410.57–2108.16/1 QALY = −1697.59 per QALY in India.
Comparison of IV infusion pump to DripAssist/manual flow regulator.
ICER = 711.85–2404.92/1 QALY = −1693.07 per QALY in Senegal.
ICER = 414.63–2108.16/1 QALY = −1693.53 per QALY in India.
Comparison of IV infusion pump to manual flow regulator.
ICER = 409.56–2404.92/1 QALY = −1995.36 per QALY in Senegal.
ICER = 113.26–2108.16/1 QALY = −1994.90 per QALY in India.
With the exception of the IV infusion pump, all methods met acceptable cost-effectiveness thresholds for implementation in Senegal (adjusted for purchasing power parity: $73–$1,166; actual $34–$544) or India (adjusted for purchasing power parity: $416–$2,781; actual: $115–$770) (25). The manual flow regulator appeared to have the highest cost-effectiveness. The DripAssist also met acceptable levels of cost-effectiveness in both countries and theoretically yielded cost savings when compared with implementing the IV infusion pump.
4 Discussion
In this study, we examined new devices for delivering IV infusions, compared their accuracy and precision, and evaluated their cost-effectiveness in low-resource settings. Additionally, we assessed the perceptions of these devices among a diverse group of healthcare workers. These participants offered additional insights into the use of the technologies in low-resource settings.
Both the DripAssist and the manual flow regulator are portable, superior low-cost alternatives to drop counting. The manual flow regulator alone was best understood and easiest to operate for the participants and had a high level of precision, making this a very favorable option for a low-resource setting. Although the DripAssist/manual flow regulator combination increased accuracy compared with manual drop counting, this combination was the most difficult to use and most time consuming, making it a less favorable option for a low-resource setting. Comments from participants suggested that the DripAssist could achieve as much accuracy as the infusion pump but that it was very sensitive, position-dependent, and more time consuming than other systems tested (Table 7).
An additional consideration was that setup time for drop counting differed significantly between types of practitioners (p < 0.001), with nurses taking significantly less time than others. The setup time factored into our cost analysis with physician and nurse wages. Furthermore, because the Alaris infusion pump is only offered in English, language may present as another barrier to implementation in non-English speaking countries, such as Senegal.
Based on these results, we believe that healthcare providers can adapt these devices to their practice environments and thereby improve the safety of rate-sensitive IV medications over the traditional method of drop counting without significant strain on electricity, time, or personnel resources.
4.1 Accuracy and healthcare worker perceptions
A key finding in this study is the similarity in accuracy between the different infusion methods. As mentioned previously, the DripAssist has demonstrated accuracy and can be used in low-resource settings such as in prehospital locations and military medicine (10, 11). In addition, Couperus et al. (11) assessed the perceptions of healthcare workers who compared the DripAssist to drop counting and infusion pumps and concluded that the DripAssist was easier to use than either drop counting or infusion pumps and that the DripAssist can be accurate in a low-resource setting. Participants in our study believed the DripAssist to be as accurate as the infusion pump, but they did not find it to be easier to operate than either the infusion pump or drop counting. Of note, a disadvantage of the free drop method was the decrease in the rate as the IV bag decreases in volume. Nevertheless, participants thought that with practice, DripAssists may become easier to use. Participants cited the motion sensitivity of the DripAssist as the reason for it being the most time consuming and least operable of the devices tested.
Another consideration is the possibility of low infusion rates. In one study by Vieira et al, low infusion rates were shown to cause a startup delay, that is, the period between the start of the infusion at a desired infusion rate and the actual delivery of the medication. Startup delay not only delays the delivery of medication to the patient, it also creates a discrepancy between the delivered volume as recorded by the pump and the volume that is actually delivered to the patient. The startup delay was smaller at higher infusion rates. Accuracy was not affected at any of the flow rates, though total volume delivered may have been affected (28).
Additionally, our study demonstrated a significant difference in flow rate accuracy (p = 0.03) among the more experienced healthcare workers, namely nurses and residents. Flow rate accuracy of the DripAssist was statistically similar to that of the infusion pump and statistically different from those of the manual flow regulator and drop-counting methods. Thus, when looking to apply these methods in low-resource settings, it is important to note that nurses and residents are the backbone of the workforce. Nevertheless, medical students also learned the setup and had infusion method ratings similar to those of other health professionals.
Other considerations are that in Dakar, Senegal, the manual flow regulator is commonly used, whereas in India, manual counting of drops or milliliters is most commonly used. Despite unfamiliarity with the manual flow regular and its lower accuracy, participants demonstrated higher precision with this device than with other devices. This finding implies that healthcare settings, including those in low-resource areas, may use other metrics for deciding between methods.
4.2 Cost
We conducted a cost-effectiveness analysis of the 5 methods for India and Senegal as representative low-resource settings in sub-Saharan Africa and in South Asia. We included health-related costs, worker-related costs, and the direct costs of each infusion method. The health measure of interest was based on QALY after sedation in the ICU. The infusion pump appeared to be least cost-effective and the manual flow regulator appeared to be most cost effective in a low-resource environment (demonstrated for both Senegal and India). With the exception of the IV infusion pump, all methods met globally acceptable cost-effectiveness thresholds (29). It is interesting to note that in Senegal, ventilator use does not factor into the pay structure. It could be that the use of ventilators lags behind owing to lack of training; thus most ventilators in the country are not being used. Another reason could be that the use of ventilators is factored into the “oxygen need” of the patients. Conversely, in India, use of ventilators is a large contributor to cost, making it very expensive to keep a patient on the machine for more than 2 days.
4.3 Limitations and future areas of research
This study had some limitations. For example, not every participant had to set up an IV bag from scratch, reducing the sample size for this metric. The study was not conducted in low-resource settings, but rather in high-resource institutions, and it was not conducted at the patient bedside. It is possible that results for flow rate may differ in clinical settings, especially in low-resource areas. Finally, our cost-effectiveness analysis addressed only one outcome that was based on the accuracy of sedation flow rates, which we found differs minimally between methods. Although statistical significance was not demonstrated, statistical significance may differ from clinical significance of the variation in flow rates demonstrated in this study.
Further study of the manual flow regulator may be warranted to determine how many times it can be reused, how long on average it takes to break, and how regularly it should be tested for accuracy. In addition, future studies of the experimental methods used here should be conducted as a clinical study in a low-resource clinical setting. Such a study could be conducted in Sierra Leone, as Johns Hopkins sent 30 DripAssists there in 2020 to bolster their critical care capacity. Lastly, the devices should be trialed at other flow rates and with other fluids or medications, perhaps tailored to the most frequently used rate-sensitive medications in these settings.
4.4 Conclusion
Both the DripAssist and manual flow regulator are portable, superior low-cost alternatives to drop counting. The combination of the 2 devices has accuracy similar to that of manual drop counting. However, this combination was the most difficult and most time-consuming method tested in the study. The manual flow regulator alone was most understood and easiest to operate by healthcare worker participants and was determined to be the most cost effective in low-resource settings. The DripAssist followed as the next most cost-effective method. These methods can be considered for implementation in acute care environments when sedation infusions are part of IV therapy. Healthcare providers can easily adapt these devices to their practice environments and improve the safety of rate-sensitive IV medications without significant strain on electricity, time, or personnel resources.
Data availability statement
The original contributions presented in the study are included in the article/Supplementary material, further inquiries can be directed to the corresponding author.
Ethics statement
The studies involving humans were approved by the Johns Hopkins School of Medicine Institutional Review Board and the Howard Community College Institutional Review Board. The studies were conducted in accordance with the local legislation and institutional requirements. The participants provided their written informed consent to participate in this study.
Author contributions
OT: Conceptualization, Data curation, Formal analysis, Investigation, Project administration, Resources, Supervision, Writing – original draft, Writing – review & editing. SA: Data curation, Formal analysis, Investigation, Validation, Writing – original draft, Writing – review & editing, Project administration. IE: Investigation, Supervision, Writing – review & editing. SW: Data curation, Investigation, Methodology, Writing – review & editing. FD: Data curation, Validation, Writing – review & editing. GD: Data curation, Formal analysis, Investigation, Project administration, Writing – review & editing. OO: Data curation, Writing – review & editing, Project administration, Visualization. KS: Data curation, Investigation, Supervision, Writing – review & editing. EA: Data curation, Investigation, Supervision, Writing – review & editing. VK: Data curation, Investigation, Writing – review & editing. JS: Conceptualization, Investigation, Supervision, Writing – review & editing. YS: Data curation, Investigation, Writing – review & editing.
Funding
The author(s) declare financial support was received for the research, authorship, and/or publication of this article. This project was made possible by the Johns Hopkins Institute for Clinical and Translational Research (ICTR), which is funded in part by grant UL1 TR003098 from the National Center for Advancing Translational Sciences (NCATS), a component of the National Institutes of Health (NIH), and NIH Roadmap for Medical Research. The contents of this article are solely the responsibility of the authors and do not necessarily represent the official view of the Johns Hopkins ICTR, NCATS, or NIH. This project was made possible in part by Shift Labs, the source of the DripAssists. Shift Labs had no influence on the project design, implementation, or analysis. This project was made possible in part by a grant from UT Southwestern Office of Global Health.
Acknowledgments
The authors would like to acknowledge the support of the Howard Community College (HCC) Division of Health, Science & Technology for laboratory supplies, space, and medical equipment to enable parallel setup at both the Hopkins and HCC sites; Tina Satapathy, MD, MPH, HCC Adjunct faculty, for assistance with data collection at the HCC site and public health data collection; the Indian Society of Critical Care, for public health data collection; Kingsley Baidoo, MSN, for assistance with data collection; Christaphine Bob-Ray, MS, for partial provision of participant tokens; Michael Banks, MD, MEHP, for encouragement of the visiting student study team members, and the Johns Hopkins Global Alliance of Perioperative Professionals for support throughout all phases of this study.
Conflict of interest
The authors declare that the research was conducted in the absence of any commercial or financial relationships that could be construed as a potential conflict of interest.
Publisher’s note
All claims expressed in this article are solely those of the authors and do not necessarily represent those of their affiliated organizations, or those of the publisher, the editors and the reviewers. Any product that may be evaluated in this article, or claim that may be made by its manufacturer, is not guaranteed or endorsed by the publisher.
Supplementary material
The Supplementary material for this article can be found online at: https://www.frontiersin.org/articles/10.3389/fmed.2024.1326144/full#supplementary-material
References
1. Kazory, A, Ronco, C, and McCullough, PA. SARS-CoV-2 (COVID-19) and intravascular volume management strategies in the critically ill. Proc (Bayl Univ Med Cent). (2020) 33:370–5. doi: 10.1080/08998280.2020.1754700
2. Claure-Del Granado, R, and Mehta, RL. Fluid overload in the ICU: evaluation and management. BMC Nephrol. (2016) 17:109. doi: 10.1186/s12882-016-0323-6
3. World Health Organization. WHO coronavirus (COVID-19) dashboard. (2022). Available at: https://covid19.who.int/. Accessed November 22, 2022.
4. Poston, JT, Patel, BK, and Davis, AM. Management of critically ill adults with COVID-19. JAMA. (2020) 323:1839–41. doi: 10.1001/jama.2020.4914
5. Loner, C, Acquisto, NM, Lenhardt, H, Sensenbach, B, Purick, J, Jones, C, et al. Accuracy of intravenous infusion flow regulators in the prehospital environment. Prehosp Emerg Care. (2018) 22:645–9. doi: 10.1080/10903127.2018.1436208
6. Song, Y, Wang, W, Zhang, L, Sha, L, and Lu, G. Optimization of the intravenous infusion workflow in the isolation ward for patients with coronavirus disease 2019. Int J Nurs Sci. (2020) 7:148–52. doi: 10.1016/j.ijnss.2020.03.009
7. Kress, JP, Pohlman, AS, O'Connor, MF, and Hall, JB. Daily interruption of sedative infusions in critically ill patients undergoing mechanical ventilation. N Engl J Med. (2000) 342:1471–7. doi: 10.1056/NEJM200005183422002
8. DiaMedical: Advancing Training & Simulation for the next generation of medical professionals. Available at: Diamedicalusa.com (Accessed: 14 September 2023).
9. Atanda, O, West, J, Stables, T, Johnson, C, Merrifield, R, and Kinross, J. Flow rate accuracy of infusion devices within healthcare settings: a systematic review. Ther Adv Drug Saf. (2023) 14:20420986231188602. doi: 10.1177/20420986231188602
10. BuyMedical.Com: Wholesale medical supplies. Available at: buymedical.com (Accessed: 14 September 2023).
11. Buonora, MJE. Management of Gravity Intravenous Infusions in an austere environment using the DripAssist infusion rate monitor. AANA J. (2019) 87:65–70.
12. Couperus, K, Kmiecik, K, and Kang, C. IV DripAssist: an innovative way to monitor intravenous infusions away from an outlet? Mil Med. (2019) 184:322–5. doi: 10.1093/milmed/usy365
13. Li, J, Dong, P, Lai, Y, Qin, X, Bai, J, Wang, H, et al. Intelligent infusion controller with a physiological information feedback function. Technology and health care: Official J European Society for Engineer Med. (2020) 28:37–46. doi: 10.3233/THC-209005
14. Juarez, A, Maynard, K, Skerrett, E, Molyneux, E, Richards-Kortum, R, Dube, Q, et al. AutoSyP: a low-cost, low-power syringe pump for use in low-resource settings. Am J Trop Med Hyg. (2016) 95:964–9. doi: 10.4269/ajtmh.16-0285
15. Skerrett, E, Kommwa, E, Maynard, K, Juarez, A, Mataya, R, Richards-Kortum, R, et al. Evaluation of a low-cost, low-power syringe pump to deliver magnesium sulfate intravenously to pre-eclamptic women in a Malawian referral hospital. BMC Pregnancy Childbirth. (2017) 17:191. doi: 10.1186/s12884-017-1382-9
16. Ouedraogo, NS, and Schimanski, C. Energy poverty in healthcare facilities: a "silent barrier" to improved healthcare in sub-Saharan Africa. J Public Health Policy. (2018) 39:358–71. doi: 10.1057/s41271-018-0136-x
17. Barghouth, A, Brower, A, Larson, J, and Shaw, G. Beyond access: What does electricity mean for healthcare facilities in Senegal? (2021). Accessed June 3, 2023. Available at: https://www.climatelinks.org/blog/beyond-access-what-does-electricity-mean-healthcare-facilities-senegal#:~:text=The%20lack%20of%20electricity%20restricts,far%20too%20many%20healthcare%20workers
18. Carayon, P, Wetterneck, TB, Hundt, AS, Ozkaynak, M, Ram, P, DeSilvey, J, et al. Observing nurse interaction with infusion pump technologies In: K Henriksen, JB Battles, and ES Marks, et al., editors. Advances in patient safety: From research to implementation (volume 2: Concepts and methodology). Rockville (MD): Agency for Healthcare Research and Quality (US) (2005)
19. Manrique-Rodríguez, S, Sánchez-Galindo, AC, de Lorenzo-Pinto, A, González-Vives, L, López-Herce, J, Carrillo-Álvarez, Á, et al. Implementation of smart pump technology in a paediatric intensive care unit. Health Informatics J. (2015) 21:209–22. doi: 10.1177/1460458213518058
20. Fox, J. E. The science of usability testing. Bureau of Labor Statistics. Accessed May 24, 2023. (2015). Available at: nces.ed.gov/fcsm/pdf/C2_Fox_2015FCSM.pdf
21. Kulkarni, AP, and Divatia, JV. A prospective audit of costs of intensive care in cancer patients in India. Indian J Crit Care Med. (2013) 17:292–7. doi: 10.4103/0972-5229.120321
23. Clinique Madeleine, Clinique Casahous, Hopital Principal, Hôpital Aristide Le Dantec, Clinique Pasteur.
25. Wade, KA, Diop, ZB, Diop, EHN, Niang, B, Sow, A, Kounta, MB, et al. Facteurs Predictives de Mortalite en reanimation a L'hopital principal de Dakar/Senegal. Mali Med. (2022) 37:25–9.
26. Sessler, CN, Gosnell, MS, Grap, MJ, Brophy, GM, O'Neal, PV, Keane, KA, et al. The Richmond agitation-sedation scale: validity and reliability in adult intensive care unit patients. Am J Respir Crit Care Med. (2002) 166:1338–44. doi: 10.1164/rccm.2107138
27. American Thoracic Society. Understanding costs and cost-effectiveness in critical care: report from the second American Thoracic Society workshop on outcomes research. Am J Respir Crit Care Med. (2002) 165:540–50. doi: 10.1164/ajrccm.165.4.16541
28. Vieira, NM, Pires, MPO, Crespo, GB, Nascimento, LPP, Peterlini, MAS, and Pedreira, MLG. Start-up delay in syringe infusion pumps with different rates and priming techniques of intravenous sets. Rev Gaucha Enferm. (2022) 43:e20210071. doi: 10.1590/1983-1447.2022.20210071.en
Keywords: cost-effectiveness analysis, global health disparities, critically ill, low-resource setting, intravenous infusion therapy, sedation
Citation: Tomobi O, Avoian S, Ekwere I, Waghmare S, Diaban F, Davis G, Sy Y, Ogbonna O, Streete K, Aryee E, Kulasingham V and Sampson JB (2024) A comparative analysis of intravenous infusion methods for low-resource environments. Front. Med. 11:1326144. doi: 10.3389/fmed.2024.1326144
Edited by:
Ata Murat Kaynar, University of Pittsburgh, United StatesReviewed by:
Richard M. Pino, Louisiana State University, United StatesDmytro Dmytriiev, National Pirogov Memorial Medical University, Ukraine
Copyright © 2024 Tomobi, Avoian, Ekwere, Waghmare, Diaban, Davis, Sy, Ogbonna, Streete, Aryee, Kulasingham and Sampson. This is an open-access article distributed under the terms of the Creative Commons Attribution License (CC BY). The use, distribution or reproduction in other forums is permitted, provided the original author(s) and the copyright owner(s) are credited and that the original publication in this journal is cited, in accordance with accepted academic practice. No use, distribution or reproduction is permitted which does not comply with these terms.
*Correspondence: John B. Sampson, anNhbXBzbzRAamhtaS5lZHU=