- 1Robarts Research Institute, London, ON, Canada
- 2Department of Medical Biophysics, London, ON, Canada
- 3Department of Medical Imaging, Western University, London, ON, Canada
Introduction: The pulmonary effects of e-cigarette use (or vaping) became a healthcare concern in 2019, following the rapid increase of e-cigarette-related or vaping-associated lung injury (EVALI) in young people, which resulted in the critical care admission of thousands of teenagers and young adults. Pulmonary functional imaging is well-positioned to provide information about the acute and chronic effects of vaping. We generated a systematic review to retrieve relevant imaging studies that describe the acute and chronic imaging findings that underly vaping-related lung structure-function abnormalities.
Methods: A systematic review was undertaken on June 13th, 2023 using PubMed to search for published manuscripts using the following criteria: [(“Vaping” OR “e-cigarette” OR “EVALI”) AND (“MRI” OR “CT” OR “Imaging”)]. We included only studies involving human participants, vaping/e-cigarette use, and MRI, CT and/or PET.
Results: The search identified 445 manuscripts, of which 110 (668 unique participants) specifically mentioned MRI, PET or CT imaging in cases or retrospective case series of patients who vaped. This included 105 manuscripts specific to CT (626 participants), three manuscripts which mainly used MRI (23 participants), and two manuscripts which described PET findings (20 participants). Most studies were conducted in North America (n = 90), with the remaining studies conducted in Europe (n = 15), Asia (n = 4) and South America (n = 1). The vast majority of publications described case studies (n = 93) and a few described larger retrospective or prospective studies (n = 17). In e-cigarette users and patients with EVALI, key CT findings included ground-glass opacities, consolidations and subpleural sparing, MRI revealed abnormal ventilation, perfusion and ventilation/perfusion matching, while PET showed evidence of pulmonary inflammation.
Discussion and conclusion: Pulmonary structural and functional imaging abnormalities were common in patients with EVALI and in e-cigarette users with or without respiratory symptoms, which suggests that functional MRI may be helpful in the investigation of the pulmonary health effects associated with e-cigarette use.
Introduction
In the summer of 2019, public health authorities in the US issued a public health alert and launched an investigation which resulted in the reporting of a large cluster of hospitalizations in young electronic cigarette (e-cigarette) users (1). The outbreak, first identified in July 2019 (2), stemmed from the hospital admission of five previously healthy teens who were recent e-cigarette users, which was then reported by the Centers for Disease Control (CDC) in the US (3). Because of this concerted public health approach, nearly 3,000 cases of idiopathic acute lung injury were reported in the fall of 2019, a few months prior to the beginning of the coronavirus disease 2019 (COVID-19) pandemic. Serious, life-threatening lung injury requiring critical care and mechanical ventilation and, in some cases, extracorporeal membrane oxygenation (ECMO), was reported primarily in previously healthy adolescents, which lead to the deaths of at least 68 adolescents and young adults (4), the youngest of whom was 13 years of age (5). Lung injury related to e-cigarette use was contemporaneously termed EC-related or vaping product use-associated lung injury (EVALI) (6). While the number and intensity of vaping related hospitalizations diminished during the pandemic years, many unanswered questions remain about the impact of vaping on lung structure and function in combustible cigarette users and in people who had never smoked combustible cigarettes prior. Such questions included: “what was the exact compound and pathophysiologic mechanisms responsible for the e-cigarette-related acute lung injury in 2019?”; “what are the acute and chronic effects of vaping on pulmonary health?”; “with the public health alert now passed, do unreported intensive care hospital admissions still occur in young adults and adolescents?; “how do the different e-liquid components impact the lungs?”; and, finally, “how does the apparent lung damage that stems from vaping or e-cigarette use directly compare to the acute and long term effects of combustible cigarette use?”
Unfortunately, there are very few studies designed to answer these questions, and even fewer studies which provide high quality evidence based on randomized, controlled prospective research designs. This systematic review was undertaken to uncover and summarize the pulmonary imaging evidence already published and explore some of these unanswered questions.
Historical context and technical developments
Historical development of vaping-related devices
The first e-cigarette was developed and patented in 1930 (U.S. Patent No. 1775947A) for individual use and a similar device was patented in 1963 (U.S. Patent No. 3200819A); neither of these devices were manufactured for commercialization. In 2003, a Chinese pharmacist, Hon Lik, initially developed the e-cigarette as an alternative to combustible cigarettes. It was commercialized in 2004 in Canada and China and was on the market in Europe and the United States in 2006 (7).
As shown in Figure 1, modern e-cigarette devices consist of a battery, mouthpiece, heating element, liquid solution reservoir and disposable cartridge or pod. The battery powers a heating element which, when applied to the liquid solution, rapidly increases the temperature enabling the transition of the e-liquid to a gaseous or aerosol state, which is subsequently inhaled via a mouthpiece into the lungs.
E-cigarette versus combustible cigarette risk
The long-term use of combustible cigarettes can cause various cardiopulmonary health risks such as chronic obstructive pulmonary disease, pulmonary hypertension and cancer. Despite some national advisory committees suggesting that the relative health risks of e-cigarette use are reduced as compared to combustible cigarettes (8), very few head-to-head comparison studies have been completed. Previous investigations suggest that e-cigarette use, either alone or in combination with combustible cigarettes, is associated with reduced overall health, breathing difficulties, and cardiovascular abnormalities (9, 10). Several systematic reviews have been conducted to examine the impact of e-cigarette use on respiratory illness (11) and human health (12); here, we summarize pulmonary imaging findings in e-cigarette users.
Composition of vaping e-liquids
e-liquids contain flavors, solvent carriers and active ingredients such as tetrahydrocannabinol (THC) or nicotine. The solvent carriers typically consist of propylene glycol, ethylene glycol, glycerol, tobacco-specific nitrosamines, volatile organic compounds, phenolic compounds, tobacco alkaloids, aldehydes, free radicals, reactive oxygen species, furans and metals (i.e., nickel, lead, chromium). Flavoring substances include menthol, ethyl maltol and diacetyl which are present in the most popular flavors of e-cigarettes (13), as shown in Figure 2.
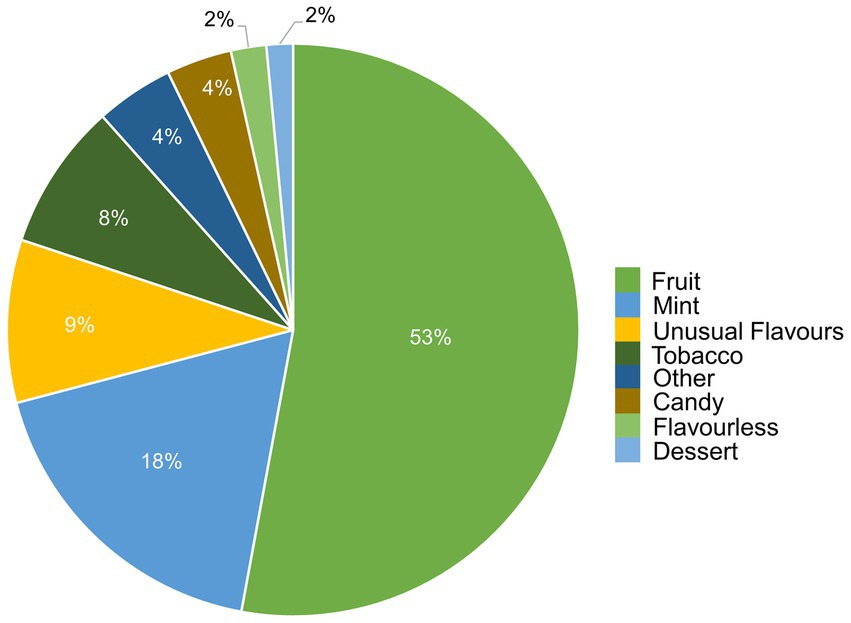
Figure 2. Distribution of vape flavors by preference. Figure was created using data from Canadian Tobacco and Nicotine Survey (CTNS) (14).
Flavors and excipients
Table 1 lists common excipients and flavors present in e-liquids. Active ingredients and concentrated flavoring compounds are dissolved in inactive excipients, which are then delivered to users as an aerosol.
There are more than 7,000 vape liquid flavors and over 450 brands available on the market (27). Though many flavorings fall under the “generally recognized as safe” (GRAS) provision by the US Food and Drug Administration (FDA), the GRAS status only applies to the use of such flavorings in ingested foods and not for inhaled products (28). In fact, at least 65 individual flavoring ingredients in flavored e-liquids were observed to cause toxicity in the respiratory track by inducing cytotoxicity, generating reactive oxygen species and impairing clearance mechanisms (21). Furthermore, cinnamaldehyde, vanillin, menthol, ethyl vanillin, benzaldehyde, ethyl maltol and linalool were present in the flavors that caused the most toxicity in in vitro studies (21). Unfortunately, the variety of products available as well as the ongoing modifications of e-cigarette and vaping devices makes it difficult to comprehensively evaluate the biological risk of vaping and specific e-liquids (29).
As early as 2000 (15, 30), the excipient diacetyl, which provides a buttery flavor in e-liquids (31), was associated with the development of bronchiolitis obliterans or “popcorn lung” in workers at a microwave popcorn plant; inflammation and fibrosis in the lung can result in the partial or complete obstruction of the peripheral airway lumen, thus leading to bronchiolitis obliterans.
Previous work has demonstrated that propylene glycol, which acts as an emulsifier for active ingredients in e-liquids (32), may also damage peripheral airways by harming epithelial cells and reducing cell proliferation; e-cigarette users with underlying chronic obstructive pulmonary disease (COPD) were more susceptible to small airway epithelial damage than those without (33).
Vegetable glycerin serves as a delivery vehicle for the active contents in e-liquids (34). Vegetable glycerin e-cigarette aerosols disturb the human nasal cystic fibrosis transmembrane conductance regulator, resulting in consequent mucus hyperconcentration and potentially harming the airway by inducing inflammation and ion channel dysfunction (34).
Vitamin E acetate is commonly used as a thickening agent in vaping products that contain cannabis derivatives (31). The inhalation of vitamin E acetate may lead to impaired pulmonary function (35–37). Furthermore, when heated, vitamin E acetate thermally degrades into ketene, alkene and benzene, all of which may contribute to epithelial lung injury (18). Additional studies observed that vitamin E acetate alters surfactant expansion and compression cycles, potentially compromising surfactant function (38). With compromised lung surfactant, the alveolar surface tension would increase and may cause an inflammatory cascade in lung tissue. The CDC previously observed vitamin E acetate in the bronchoalveolar lavage fluid of 94% of EVALI patients (19, 39), pointing to an association between vitamin E acetate and the development of EVALI.
Active ingredients
Commonly used active ingredients in e-liquids include THC and nicotine. THC is the psychoactive compound in cannabis and may be added to e-liquids (21). While THC can be degraded to toxins such as methacrolein and benzene when heated in vapes (18), other compounds in THC-based e-liquids may also have negative effects on respiratory health. THC-based e-liquids differ from nicotine-based liquids because THC (a highly hydrophobic chemical) requires a hydrophobic emulsifier to be incorporated into the vape liquid (18). Thus, vitamin E acetate, described in the previous section, is commonly used in THC-based e-liquids. While the vast majority of EVALI cases (80%–86%) are associated with vaping THC products, the overall health risks of cannabis vaping is largely unknown (18, 29, 39, 40).
e-cigarettes are marketed as a safe alternative to traditional combustible cigarettes as they do not contain carcinogenic incomplete combustion byproducts nor tobacco nitrosamines (41). However, previous investigations have demonstrated an association between nicotine and vaping-induced COPD pathologies (42, 43), such as emphysema, as well as an increased risk of lung cancer among nicotine e-cigarette users (44). Although vaping does not require tobacco or combustion, stimulant nicotine in e-liquids may still cause DNA damage (45) and may mediate tumor growth by promoting the self-renewal of stem-like cells in tumor initiation and metastasis (46).
Pathophysiology of vaping-induced lung injury
Symptoms
The majority of EVALI patients experience respiratory symptoms, including cough, chest pain, shortness of breath and hemoptysis (2, 39, 47), in addition to gastrointestinal symptoms, such as abdominal pain, nausea, vomiting and diarrhea; gastrointestinal symptoms may precede respiratory symptoms in some patients (47). Additional common symptoms include unexplained weight loss, headache and fatigue (2), and, more generally, EVALI patients are frequently admitted with tachycardia, tachypnea, fever and hypoxemia (39). Because lung biopsies not commonly obtained in EVALI patients, the pathological drivers of symptoms and disease progression remain poorly understood.
The respiratory system
The respiratory system consists of two main parts: the upper and lower respiratory tract. The upper tract includes the nose, nasal cavity, throat and larynx. This tract is responsible for bringing in air from outside the body, through the nose and mouth.
As shown in Figure 3, the lower respiratory tract is divided into two zones, known as the conducting and respiratory zones. These zones encompass a total of 23 generations of airways. The conducting zone, which includes airway generations 0–16, is responsible for guiding inhaled air towards the alveoli and humidifying it. The conducting zone begins with the trachea which then bifurcates across 16 generations of airways to the terminal bronchioles, which conclude the conducting zone. As the airways progress into further generations, their diameter gradually decreases. The airways within the conducting zone consist of thick walls of mucosa, smooth muscle and cartilage and are lined with cilia to remove dust and foreign particles from the lung.
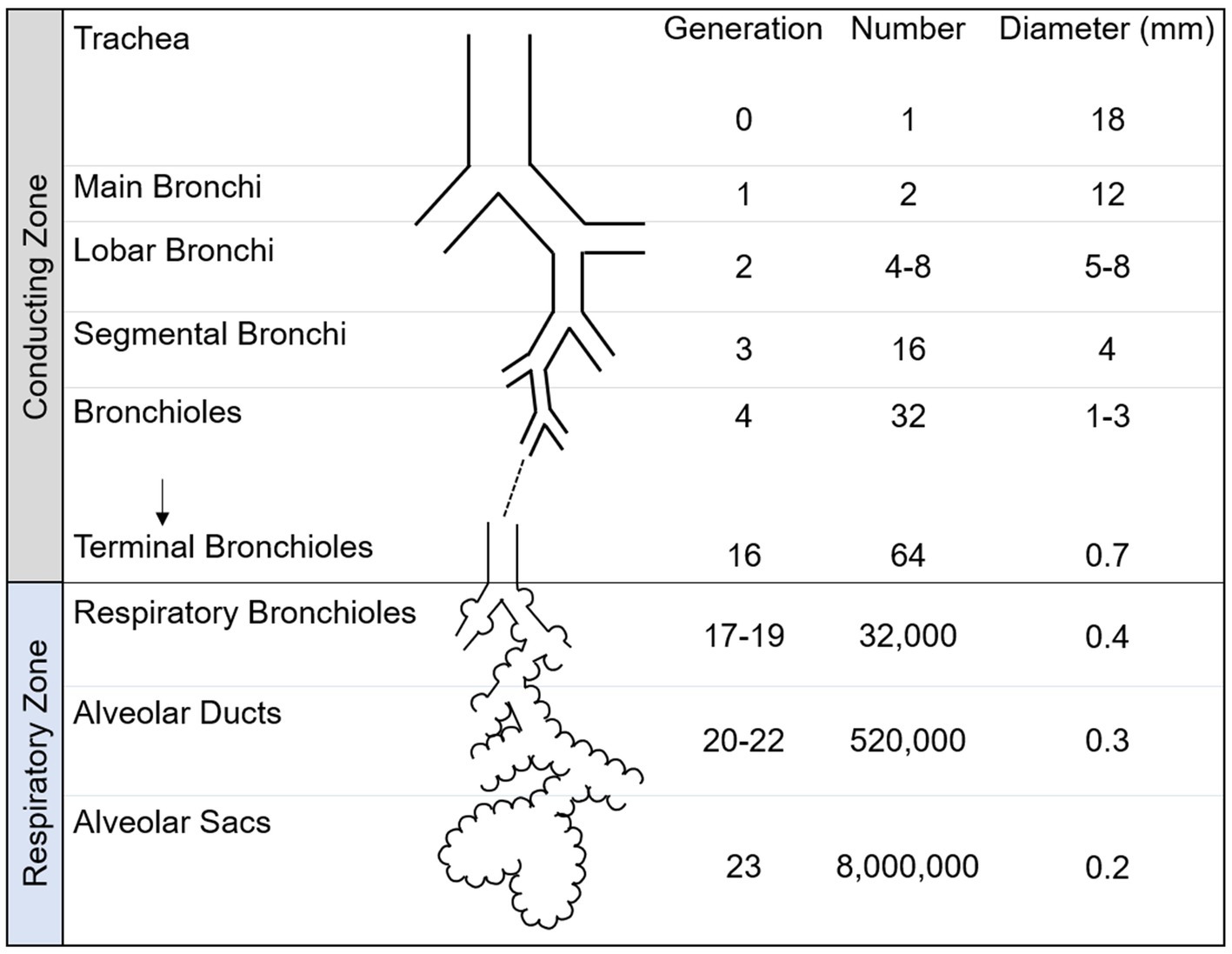
Figure 3. Schematic of airway tree conducting and respiratory zone. The human airway tree consists of the conducting zone and respiratory zone, with corresponding generation, number and diameter shown. Adapted from Nunn’s Applied Respiratory Physiology, 8th edition.
The respiratory zone is responsible for facilitating gas exchange and contains airway generations 17–23. The respiratory zone contains respiratory bronchioles, alveolar ducts and alveolar sacs. Within the lungs, there are numerous microscopic sacs known as alveoli, which begin to bud along the walls of the respiratory bronchioles and become increasingly abundant with each subsequent airway generation. These alveoli are surrounded by a network of capillaries, whose primary function is to facilitate the exchange of inhaled oxygen with carbon dioxide. The alveolar sacs complete the respiratory zone and the airway tree.
Pathophysiology
Potential mechanisms of vaping-related lung injury are provided in schematic in Figure 4. When aerosolized e-liquids are inhaled, the particles come in direct contact with the entire respiratory system. Consequently, chemicals in e-cigarette aerosols, such as menthol and ethyl maltol may lead to inflammation, which is thought to be the dominant cause or contribution to vaping-related lung injury (18, 31, 48–50). It has also been postulated that the pro-inflammatory effects caused by vape aerosols are partially mediated by reactive oxygen species (ROS) (27), which may lead to cellular apoptosis through ROS-mediated autophagy (51); this cellular death pathway is also mechanistically attributed to emphysema (27). In addition, pulmonary inflammation and fibrosis may result in bronchiolitis obliterans, which is characterized by hypertrophy of the bronchiolar smooth muscle, peribronchiolar inflammatory infiltrates, mucus accumulation in the bronchiolar lumen and bronchiolar scarring (52). This scarring is irreversible and bronchiolitis obliterans has no known cure. While healthy patients may achieve complete improvement, the pulmonary health of most patients progressively worsens and some may even require mechanical ventilation or lung transplants in severe cases (53).
Heavy metals have been detected in the vape aerosols produced from pod-type vapes (27), including chromium, nickel, copper, zinc, cadmium, tin, manganese and lead (54). The metallic components of e-cigarette devices, such as the filaments and coils, are comprised of such heavy metals and can degrade when exposed to oxidized acidic e-liquids (54). While metal exposure is a risk factor for multiple pulmonary diseases including respiratory inflammation, asthma, COPD and respiratory cancer (55–57), the health effects of metal exposure in e-cigarette users is still largely unknown (58).
Patients with EVALI or vaping-related lung injury consistently present with lipid laden alveolar macrophages or foam cells in the lung or in bronchoalveolar lavage fluid (59). These macrophages serve as the main phagocytes in the innate immune system, clearing the airspaces of potentially harmful particles (48). Recent work has observed lipid laden alveolar macrophages in the bronchoalveolar lavage fluid of 80% of EVALI patients, demonstrating its potential as a biomarker of vaping-related lung injury. Nevertheless, the presence of lipid laden alveolar macrophages is not unique to vaping, which has been reported in a number of other pulmonary conditions (18). Thus, they are a non-specific marker of vape product use, and do not provide a direct prognosis of vaping-related lung injury (60).
Knowledge and health care gaps
There are currently many unanswered questions associated with vaping. An unexhausted list of these questions includes: “what are the longitudinal effects of vaping on pulmonary health?”; “what is the safety profile of e-cigarette use relative combustible cigarettes?”; “what are the effects of each e-liquid component on respiratory health?”; and “what role can pulmonary imaging play in the diagnosis and monitoring of vaping-related lung injury?”
This review is designed to explore some of these unanswered questions in the context of pulmonary imaging.
Research questions
We aim to investigate the current understanding of vaping in literature, the conditions associated with it, and the common imaging modalities used to evaluate vaping-related lung injury, specifically magnetic resonance imaging (MRI), computed tomography (CT) and positron emission tomography (PET).
Methods
This review was conducted according to the preferred reporting items for systematic reviews and meta-analyses (PRISMA). We used PubMed to search for manuscripts related to vaping/e-cigarette use or EVALI and pulmonary imaging on June 13, 2023 using the terms [(“Vaping” OR “e-cigarette” OR “EVALI”) AND (“MRI” OR “CT” OR “Imaging”)].
Inclusion and exclusion criteria
The inclusion and exclusion criteria are presented in Table 2. The inclusion criteria were: (a) imaging modalities: strictly MRI, CT and PET; (b) bodily systems scanned; strictly structures in the respiratory system: such as lungs, respiratory epithelium, and alveoli; (c) test subjects: living human subjects who had previously used e-cigarettes/vapes. Exclusion criteria included: (1) any types of non-electric cigarette such as conventional cigarettes, joints, cigars, and hookahs; (2) any articles that were reviews or not directly treating a single/group of patients.
Selection process and data collection
Four reviewers (JH, VP, JZ, and GP) independently screened the abstracts of the retrieved reports to evaluate whether they met the predetermined inclusion/exclusion criteria. A full text review of the remaining studies was performed to further evaluate the inclusion eligibility of the reports and any discrepancies were presented and discussed between the four reviewers to ensure a consensus was made regarding the inclusion of each report.
Risk of bias assessment
Three reviewers (JH, VP, and JZ) independently appraised the methodological qualities of the included studies in accordance with two risk of bias tools, detailed further in Supplementary Figure S1. The JBI critical appraisal checklist for case reports was deemed most appropriate for one report [Eddy et al. (61)] while the Cochran risk of bias in non-randomized studies-of exposure tool (ROBINS-E) was used for the remaining studies. The JBI tool assesses bias arising from: the patient’s demographics (D1), history (D2) and clinical condition on presentation (D3), the diagnostic tests or assessment methods (D4), the interventions or treatments used (D5), the post-intervention clinical condition (D6), the adverse effects on unanticipated events (D7), and the takeaway lessons (D8). The ROBINS-E tool assesses bias arising from: confounds (D1), measurement of the exposure (D2), selection of the participants in the study (D3), post-exposure interventions (D4), missing data (D5), measurement of the outcome (D6), and selection of the reported result (D7). The level of risk judgement for each domain was categorized into four categories: high risk, some concerns, low risk, or no information.Synthesis methods
The criteria used to determine which studies were eligible for each synthesis was that, for each patient, the study must report age, gender, location, and history of e-cigarette or vape use. We used a table to separate patient characteristics such as age, type of e-device vaped and symptoms. No data conversions were necessary, as we qualitatively analysed patient symptoms and characteristics. The method used to prepare missing summary statistics of age range was to report the mean age.
Evaluation methods
Computed tomography
Chest CT measures x-ray attenuation coefficients to determine lung tissue density and is routinely used in clinic to evaluate a variety of respiratory diseases, including but not limited to pneumonia, emphysema and interstitial lung disease. CT may be used to discern between pulmonary diseases and evaluate severity, as well as monitor disease progression and treatment response.
Positron emission tomography
PET is a quantitative molecular imaging modality that utilizes radiotracers to measure pulmonary ventilation, perfusion and blood flow as well as metabolic activity. The radiotracers used in PET imaging emit positrons, which will typically travel a few millimeters before colliding with an electron in the surrounding tissue, resulting in the emission of two 511 keV photons in opposite directions that are then detected by PET detectors oriented around the patient. In the context of lung disease, PET imaging is mostly commonly used to detect cancerous cells. However, PET radiotracers may also be used to evaluate the deposition of inhaled substances using carbon-11 (11C) and fluorine-18 (18F) (62), inflammation using 18F-fluorodeoxyglucose (18F-FDG) (63) and ventilation using nitrogen-13 (13N2) (63).
Pulmonary functional MRI
Hyperpolarized noble gas, oxygen-enhanced 1H, free-breathing 1H and arterial spin labelling methods are employed in order to acquire pulmonary functional MR images. These MRI techniques, in combination, may be used to measure lung ventilation and/or perfusion (64, 65), and are sensitive to early disease changes not easily detected with conventional pulmonary function tests (64, 66).
Hyperpolarized noble gas MRI allows for the visualization of the gas distribution in vivo, and is sensitive to the transitional and respiratory zones of the lung (67). Hyperpolarized 3He or 129Xe act as inhaled contrast agents, providing three-dimensional images with increased spatial and temporal resolution as compared to other functional imaging methods such as PET (68, 69), 133Xe scintigraphy (70), Xe-enhanced CT (71) and single photon emission computed tomography (SPECT) (68, 69). Ventilation abnormalities depicted on hyperpolarized MR images are associated with pulmonary structural abnormalities including luminal plugging, air trapping, airway inflammation and emphysema (68, 72–76). Hyperpolarized gas MRI has mainly been used as a research tool to investigate respiratory diseases including COPD (77), asthma (67), cystic fibrosis (78) and idiopathic pulmonary fibrosis (79). Recently, hyperpolarized 129Xe was approved by the US FDA for clinical use (80).
Oxygen-enhanced 1H MRI is a research technique that relies on the weak paramagnetic properties in oxygen, which decreases the longitudinal relaxation time (T1) of tissues, thus increasing the signal (81). By using O2 as a contrast agent, the presence and/or absence of ventilation may be evaluated and specific ventilation may be quantified (81). Oxygen-enhanced MRI has previously been used to investigate patients with COPD (82), interstitial lung disease (83) and asthma (84).
Free-breathing 1H MRI is also mainly a research tool which can be used to evaluate pulmonary ventilation and perfusion without the need for an endogenous contrast agent. This technique has been applied to patients with various cardiopulmonary diseases including COPD, CTEPH and asthma (85–88). These images are post-processed using non-rigid registration which compensates for respiratory motion, eliminating the need for patient compliance during a breathhold. Commonly used image processing techniques for free-breathing MRI include phase-resolved functional lung (PREFUL) (88) and matrix pencil decomposition (89).
Arterial spin labelling MRI can be used to evaluate pulmonary blood flow and its heterogeneity. This technique involves the acquisition of two images, which are subsequently subtracted to remove the blood signal from the tissue (90). This results in a perfusion-weighted image of the blood delivered within one cardiac cycle.
Results
Participants and report characteristics
A flowchart is provided in Figure 5; Supplementary Figure S2 which show that the PubMed search conducted on June 132,023 yielded 445 manuscripts, one of which was identified as a duplicate and was excluded. The abstracts of the remaining 444 manuscripts were screened and an additional 235 manuscripts were excluded. Following a thorough full text evaluation, 45 review articles, 27 non-pulmonary articles, 15 letters to the editor, five editorials, four non-vape related and three non-English articles were excluded. Ultimately, 110 manuscripts met the inclusion criteria and were included in this review.
The characteristics of the included studies are listed in Supplementary Tables S1, S2. The vast majority of studies were conducted in North America (n = 90) with the remaining studies having been conducted in Europe (n = 15), Asia (n = 4) and South America (n = 1). A total of 668 e-cigarette users or patients that were exposed to vaping performed MRI, CT and/or PET scans; the primary imaging modality for 625 patients was CT, for 20 patients was PET and for 22 patients was MRI; a single participant was presented in two separate manuscripts, where one report described the clinical case and CT findings (91) and the other described research MRI and quantitative CT findings (61). In addition, each PET scan was accompanied by an anatomical CT. The minimum number of patients included in each study was 1 and the maximum was 160. Nearly all (n = 109) studies evaluated current or former e-cigarette users; one study (92) prospectively evaluated the acute effects of nicotine-based vaping in 15 healthy adults. The reported active ingredients in the e-liquid used by patients is summarized in Table 3. A total of 354 participants exclusively used nicotine (n = 101), THC (n = 247) or other substances (n = 6) in vape devices, 233 participants used both nicotine and either THC (n = 230) or containing other substances (n = 3) and 10 participants used a combination of three substances, while the contents of the vape devices of the remaining 71 participants was unspecified or unknown.
CT findings
Of the included manuscripts, CT was the most common imaging modality used to evaluate pulmonary abnormalities in vaping-related lung injury (105/110 manuscripts; 95%). Table 4; Supplementary Table S1 provide summaries of the findings from the included CT manuscripts. The majority of manuscripts reported on patients that were adolescents or young adults (61/105; 58%), with patient age ranging from 13 to 68 years old. Of the CT manuscripts included, 92 (88%) were case studies and 13 (12%) were retrospective studies. Acute lung injury was reported in 97 (92%) studies and 600 (98%) patients, chronic lung injury was reported in 7 (7%) studies and 13 (2%) patients, and both were reported in 2 (2%) studies. Nineteen (18%) studies involved participants who had previous respiratory diseases including: asthma (n = 15), tuberculosis (n = 1), allergic rhinitis (n = 1) and COPD (n = 1).
Ground-glass opacities, defined as a hazy increase in lung density observed on chest CT (93), were the most common CT finding in the included manuscripts, with 84 (80%) studies and 347 (57%) participants reporting evidence of this pulmonary abnormality. Consolidation opacities (37 studies/176 participants), subpleural sparing (32 studies/230 participants), septal thickening (24 studies/145 participants) and patchy opacities (22 studies/44 participants) were also common.
Figure 6 shows axial CT slices of three patients, each at two separate time-points, previously published and described by Kligerman et al. (94). P01 was a 35 years-old female who reported the use of THC-based e-cigarettes and who presented with CT evidence of ground-glass opacities and consolidations as well as subpleural and perilobular sparing (P01a). CT images that were acquired 2 weeks later (P01b) showed extensive consolidation along with areas of bronchial dilation and the development of a right pneumothorax; this patient died 5 days later. P02 was a 51 years-old female who reported the use of nicotine-based e-cigarettes and who presented with CT evidence of ground-glass opacities and subpleural sparing (P02a). Two months later (P02b), CT images revealed more extensive ground-glass opacities and septal thickening which presented as “crazy paving” pattern. P03 was a 20 years-old male who reported the use of both THC- and nicotine-based e-cigarettes. CT imaging (P03a) in this patient revealed organizing pneumonia, peribronchiolar ground-glass opacities and subpleural sparing. CT imaging 4 weeks later (P03b) normalized, after the patient was treated with steroid therapy.
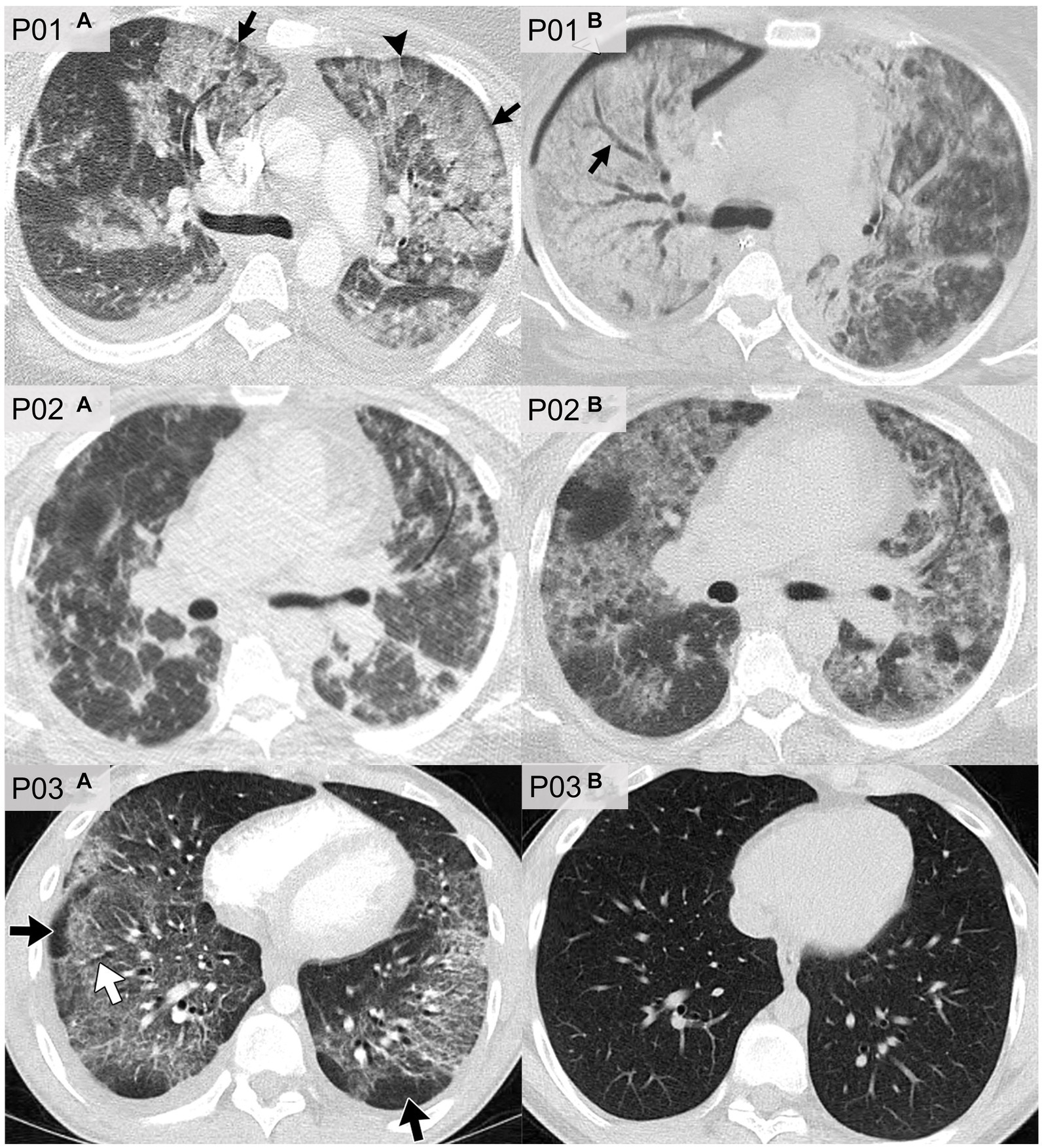
Figure 6. CT imaging of e-cigarette-related or vaping-associated lung injury (EVALI) with short-term follow-up. P01: axial CT of a 35 years-old female with diffuse alveolar damage pattern who vaped THC. (A) CT imaging showed ground-glass opacities with areas of consolidation, subpleural and perilobular sparing (arrows) and septal thickening (arrowhead). (B) CT 2 weeks later showed extensive right lung consolidation with areas of bronchial dilation (arrow) and internal development of right pneumothorax. Patient died 5 days later. P02: axial CT of a 51 years-old female showing multiple episodes of EVALI following repeated vaping of nicotine with mint flavoring. (A) CT imaging showed scattered areas of ground-glass opacities with subpleural sparing. (B) Two months later, the patient returned to emergency department with dyspnea and fever. CT findings included more extensive ground-glass opacities with areas of lobular and subpleural sparing. Septal thickening is present creating “crazy paving” pattern. Patient’s condition deteriorated, and was complicated by aspiration pneumonia and bilateral lower-lobe collapse. P03: axial CT of organizing pneumonia pattern in a 20 years-old male who vapes nicotine and THC products daily. (A) CT imaging showed peribronchiolar ground-glass opacities with subpleural sparing (black arrows). Areas of bronchial dilation are seen in areas of ground-glass opacities (white arrow). (B) Four weeks following steroid therapy, the patient’s CT scan was normal. Images reproduced with permission from Kligerman et al. (40).
Table 5 summarizes CT findings from the 13 included retrospective studies. All patients described in these studies presented with probable or confirmed EVALI. Six of these studies reported on pediatric patients and four reported findings in adult patients, while the remaining three described findings in both pediatric and adult populations.
Nearly all (10/13) of the retrospective studies reported that ground-glass opacities, subpleural sparing and/or consolidation were among the most common CT findings in patients with EVALI (94–98, 102–106). Three investigations included short-term (within 30 days of initial presentation) follow-up imaging (95, 101, 104). Kalininskiy et al. (101) reported that CT abnormalities in patients treated with antibiotics were largely resolved within 17 days of their initial emergency department visit; Aberegg et al. (95) also reported resolution of CT opacities after treatment with antibiotics and/or corticosteroids. CT abnormalities cleared in some patients described by Panse et al. (104) despite extensive injury at initial presentation, although some patients described showed evidence of residual imaging findings. Prospective longitudinal imaging studies will help elucidate whether such residual abnormalities are the result of slow healing areas of injury or point to the development of permanent scarring.
PET findings
Table 6 summarizes the included PET studies (n = 2). In total, 20 patients were either current or former e-cigarette users, or were acutely exposed to e-cigarettes.
Wetherill et al. (109) used the radiotracer 18F-nitric oxide synthase (NOS) to characterize oxidative stress and inflammation in the lungs of e-cigarette users (n = 5) and compare with combustible cigarette smokers (n = 5) and healthy controls (n = 5). They revealed that e-cigarette users showed greater non-displaceable binding potential (Figure 7A) than combustible cigarette smokers and healthy controls, which is associated with the pro-inflammatory cytokine TNF-alpha that is involved in the inflammatory cascade of acute lung injury. They also observed that pulmonary inflammation was greater in e-cigarette users than combustible cigarette smokers and healthy controls.
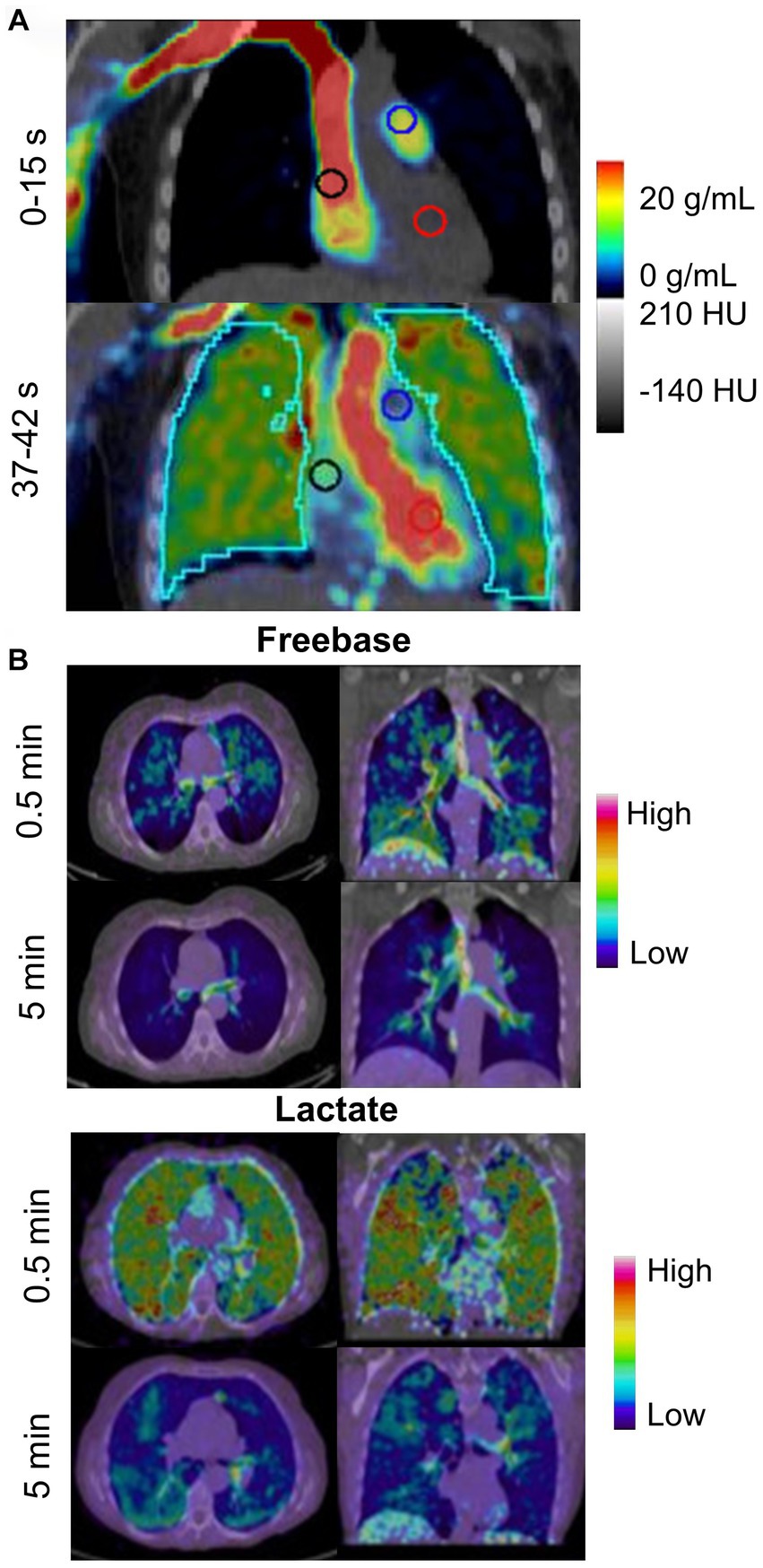
Figure 7. PET imaging tracking metabolic activity in e-cigarette users and smokers. (A) Oronal PET/CT 18F-NOS in an e-cigarette user (unspecified active ingredient). Uptake is shown 0–15 s and 37–42 s after injection of 18F-NOS to quantify oxidative stress and inflammation in the lungs. Adapted from Wetherill et al. (109) under Creative Commons License. (B) Representative distribution of 11C-nicotine freebase and lactate in lungs at 0.5 and 5 min after inhalation of the tagged nicotine salts through an e-cigarette in a healthy adult smoker. Adapted from Wall et al. (92) under Creative Commons License.
In contrast, Wall et al. (92) investigated the acute deposition of inhaled 11C-nicotine in 15 healthy adults using two nicotine formulations—freebase and lactate salt. In this study, the authors showed that freebase nicotine exhibited high uptake and deposition in the upper respiratory pathways while lactate nicotine was deposited throughout the entire lung and bronchial tree (Figure 7B). Lactate nicotine was also distributed more rapidly than freebase nicotine.
MRI findings
Table 6 also summarizes the included MRI reports (n = 3). A total of 23 patients who were current or former e-cigarette users were reported.
Figure 8 shows hyperpolarized 129Xe MRI ventilation (cyan) co-registered with anatomical 1H MRI (greyscale) for one participant with EVALI, one asymptomatic e-cigarette user and one healthy participant; all images were acquired by the authors’ group. The participant with EVALI demonstrates a heterogenous ventilation pattern with multiple ventilation defects, represented as black regions, throughout the entire lung. In contrast, the asymptomatic e-cigarette user demonstrates a relatively homogenous ventilation pattern with evidence of small, peripheral ventilation defects in the lung apices. Finally, the healthy participant has a homogenous ventilation distribution with no ventilation defects.
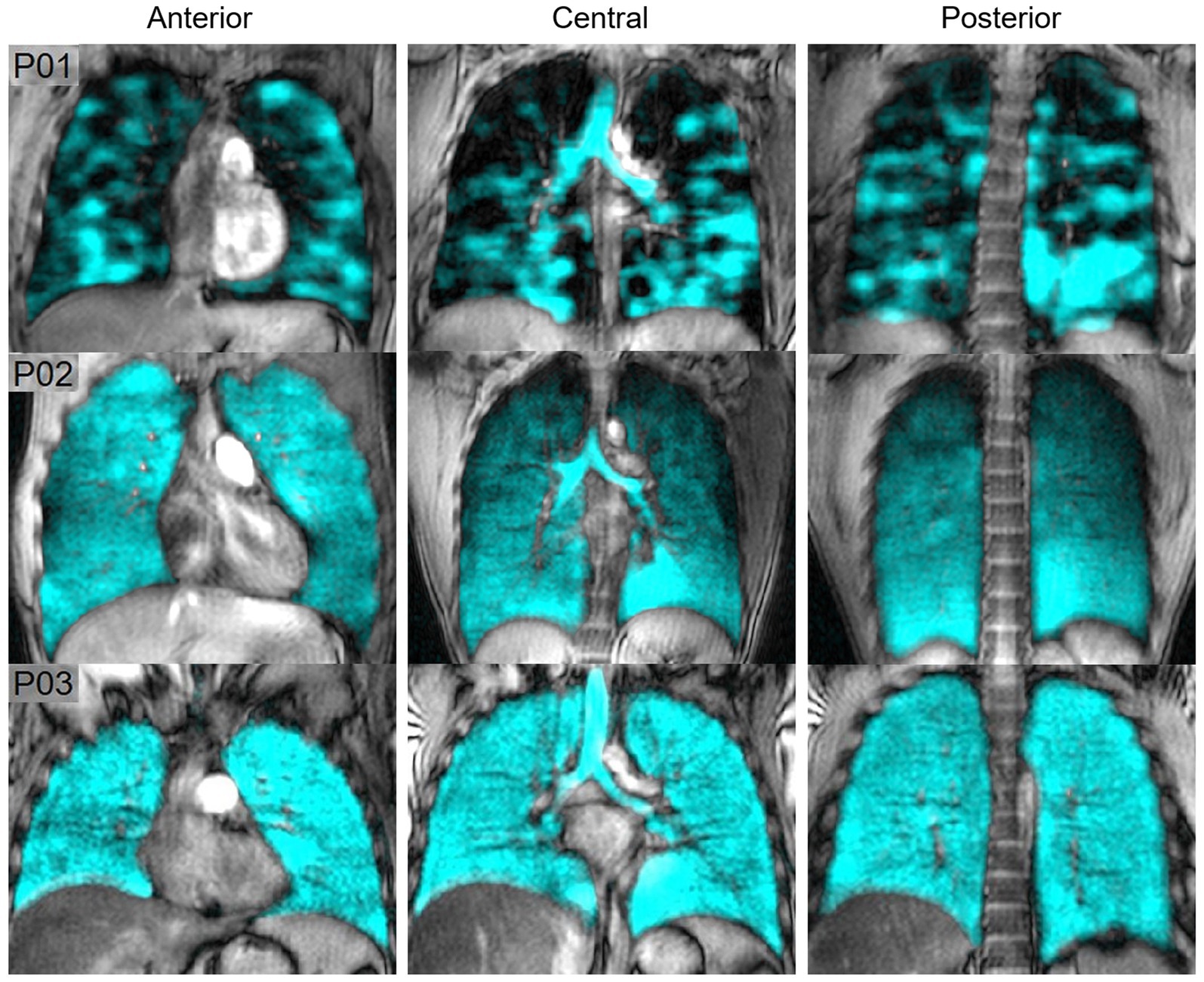
Figure 8. Hyperpolarized 129Xe MR ventilation imaging in chronic vapers and similar-aged healthy volunteer. Anterior, central, and posterior coronal slices of 129Xe ventilation (cyan) co-registered with anatomical 1H (greyscale) MRI. All images were acquired by the authors’ group. P01 is an 18 years-old male with severe bronchiolitis and respiratory failure caused by e-cigarette use, 1 month post discharge after a 6 months history of vaping (VDP = 21%). P02 is a 29 years-old male with a 2.5 years history of vaping nicotine (3/4 pods daily) (VDP = 4.7%). P03 is a 22 years-old male with no history of chronic respiratory abnormalities, vaping or combustible cigarette use (VDP = 1.5%).
Two studies compared the acute effects of e-cigarette use on ventilation. In the first, alveolar ventilation and ventilation heterogeneity in asymptomatic e-cigarette users were similar to healthy controls prior to exposure, however both measurements worsened following exposure to vaping in e-cigarette users (107). In contrast, while the second study observed a nominal increase in ventilation impairment post-exposure, this change was not statistically significant (108). In a similar manner, the 129Xe MRI ventilation pattern of a patient recovering from EVALI was highly abnormal and dissimilar to the patterns previously observed in either asthma or COPD (61). Furthermore, these ventilation abnormalities persisted for at least 8 months (61).
MRI perfusion maps are shown for four e-cigarette users in Figure 9, demonstrating changes in regional perfusion following exposure to e-cigarettes. Two studies have evaluated pulmonary perfusion in e-cigarette users prior to and following acute exposure to e-cigarettes and both observed similar findings. Perfusion prior to exposure was similar between healthy controls and e-cigarette users, and following exposure, perfusion was increased (107) while perfusion heterogeneity was decreased (108). Furthermore, e-cigarette users who were exposed to nicotine-based e-liquids demonstrated significant increases in perfusion while those who were exposed to nicotine-free e-liquids were not. Finally, ventilation/perfusion heterogeneity was increased throughout the lungs of e-cigarette users as compared to healthy controls, both prior to and following acute exposure to e-cigarettes (107); the extent of this disruption in ventilation/perfusion matching was similar to what has previously been observed in COPD.
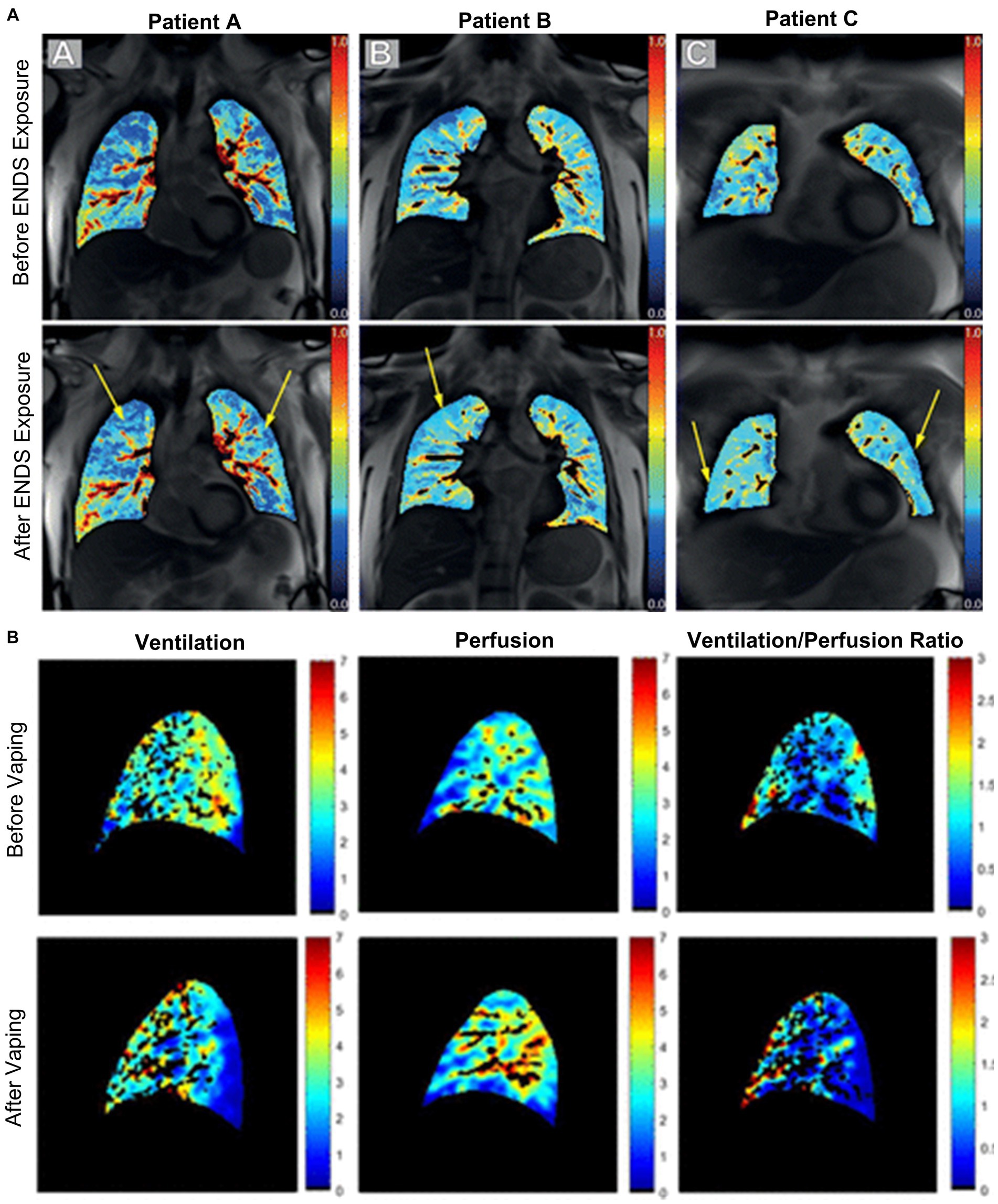
Figure 9. Free-breathing 1H MRI of acute effects of vaping. (A) Pulmonary perfusion images obtained by using non-contrast matrix pencil MRI in three electronic nicotine vape users before and after exposure. The arrows indicate lung regions with increased regional perfusion post-exposure. Red corresponds to greater perfusion amplitude and blue corresponds to lower values (108). (B) Spatial maps of ventilation, perfusion, and ventilation-perfusion ratios, before and after vaping, in a single sagittal slice of one representative subject. Black regions within the lung field represent regions excluded from analysis, including conducting airways and vessels, and regions with poor signal to noise (107).
Discussion
Despite nearly 3,000 EVALI cases having been reported in the US in less than 12 months in 2019, the impact of e-cigarette use or vaping on respiratory health is still largely unknown. This is, in part, due to a lack of prospective studies designed to sensitively evaluate the pathological mechanisms responsible for the development of this disease. In this systematic review we summarize in e-cigarette users and patients with EVALI the following points: (1) CT sensitively revealed pulmonary structural abnormalities, (2) PET measured pulmonary inflammation and the deposition of e-cigarette aerosolized particles, and (3) ventilation and perfusion measured via MRI was abnormal. These findings demonstrated that pulmonary functional and structural abnormalities were common in both patients with vaping-induced lung injury and in e-cigarette users with or without respiratory symptoms, and that these abnormalities may be sensitively measured using CT, PET and pulmonary functional MRI. Furthermore, these findings support the use of imaging modalities in prospective studies to help uncover the pathological drivers and mechanisms underlying respiratory symptoms and the development of EVALI in previously healthy adolescents and young adults.
Our systematic review included any report which used CT, PET or MRI to evaluate patients with EVALI or e-cigarette users. CT was the most commonly reported imaging modality, with 105 of the 110 included studies primarily reporting CT findings. Ground-glass opacities, consolidation opacities, subpleural sparing, septal thickening and patchy opacities were among the most common CT findings, in patients with EVALI and e-cigarette users. These findings demonstrated that CT is highly useful in the diagnosis and monitoring of patients with EVALI. Moreover, the worsening or resolution of CT abnormalities, with or without therapeutic intervention, may help inform on the pathological drivers of EVALI, point towards potential treatment options or help identify characteristics of patients at risk of developing serious and/or permanent lung injury as a result of e-cigarette use.
PET showed that there were differences in the deposition distribution of freebase or lactate salt nicotine aerosolized particles (92). This is important because early e-liquids primarily used freebase nicotine however, since 2017, lactate salt nicotine is more commonly used (110). The health risks of lactate salt and freebase nicotine have not yet been studied. PET also revealed a unique inflammatory response to acute e-cigarette exposure, in comparison with acute combustible cigarette exposure or in healthy controls. These findings point towards possible mechanisms of acute lung injury following e-cigarette use. Together, these preliminary studies may drive further hypothesis-driven research into the pulmonary health effects associated with e-cigarette use.
Unlike CT and PET, pulmonary functional MRI does not require ionizing radiation and multiple acquisitions can be completed over either short or long periods of time. In the context of vaping, pulmonary functional MRI sensitively revealed ventilation and perfusion abnormalities in e-cigarette users following acute exposure to e-cigarettes (107, 108), and in a patient recovering from EVALI (61). Ventilation abnormalities in e-cigarette users may be driven by airflow obstruction via inflammation, impaired mucus clearance, constriction or collapse (107). In addition, perfusion abnormalities were worse in patients using nicotine-based e-liquids as compared to those using nicotine-free e-liquids, which points towards known impacts of nicotine on cardiovascular hemodynamics, increases in heart rate, systolic blood pressure and cardiac output (107). Furthermore, findings of increased perfusion and decreased perfusion heterogeneity in e-cigarette users, regardless of e-liquid content, may suggest hypoxic vasoconstriction as a compensatory mechanism in the presence of abnormal ventilation (107). This idea is further supported by the observation of increased ventilation/perfusion heterogeneity. Together, these findings show that MRI may be used to sensitively measure both the acute and chronic effects of e-cigarette use on both pulmonary ventilation and perfusion.
Limitations
The reviewed manuscripts have a number of important limitations. First, our search criteria were quite specific and thus we may have excluded relevant studies which did not use our precise terminology. Second, technical CT parameters were not commonly described. The variation of CT parameters between studies and patients may have impacted the radiologist’s findings and thus, it may be possible that some CT abnormalities were undetected. Third, the commercialization and distribution of e-cigarette devices is recent and thus, the majority of the included studies reported on data acquired within the past 4 years. It is not possible at this time to comment on the long-term health effects associated with either continuous or sporadic e-cigarette use as this has not yet been investigated and/or reported. Fourth, a large proportion (26% of studies and 11% of patients) of the included studies did not report or were not able to ascertain the specific ingredients present in the e-liquids used by the studied patients. With the wide variety of e-liquids available, as well as the potential combination of multiple e-liquids by users, the effect of individual or combinations of e-liquids and their ingredients on respiratory health is largely unknown. Finally, e-cigarette devices have evolved and many components of these may be customized, including the voltage of the battery, the e-liquid temperature, nicotine yield or puff volume (111–113), introducing a wide number of variables that may contribute to vaping-induced lung injury.
Conclusion
With the growing popularity of e-cigarettes for recreational use in people who have not previously smoked combustible cigarettes, it is important to understand the short- and long-term effects of vaping on respiratory health. This systematic review, while revealing only a handful of functional imaging studies, showed that pulmonary imaging and in particular functional imaging is helpful in generating a better understanding of the acute and chronic effects of vaping on pulmonary structure and function. The time is right to explore larger scale, multi-centre studies using pulmonary functional imaging methods to uncover the pathological mechanisms driving vaping-induced lung injury and respiratory symptoms, and to evaluate interventions.
Data availability statement
The original contributions presented in the study are included in the article/Supplementary material, further inquiries can be directed to the corresponding author.
Author contributions
JH: Data curation, Formal analysis, Investigation, Visualization, Writing – original draft. VP: Data curation, Formal analysis, Investigation, Visualization, Writing – original draft. JZ: Data curation, Formal analysis, Investigation, Visualization, Writing – original draft. MS: Supervision, Visualization, Writing – review & editing. GP: Conceptualization, Investigation, Methodology, Supervision, Writing – review & editing. MM: Supervision, Visualization, Writing – review & editing.
Funding
The author(s) declare financial support was received for the research, authorship, and/or publication of this article. MS and MM acknowledge doctoral awards from the Natural Sciences and Engineering Research Council (NSERC) of Canada. MM acknowledges a studentship training award from Canadian Respiratory Research Network. GP acknowledges funding from the Canadian Institutes of Health Research, NSERC Discovery Grant and she holds a Tier 1 Canada Research Chair.
Conflict of interest
GP discloses investigator initiated study funding from AstraZeneca, Novartis and GSK, study funding from the Ministry of Health and Long-term Care Ontario, and honoraria from AstraZeneca for speaking engagements, outside the submitted work.
The remaining authors declare that the research was conducted in the absence of any commercial or financial relationships that could be construed as a potential conflict of interest.
The reviewer LS declared a past co-authorship with the authors MS and GP to the handling editor.
Publisher’s note
All claims expressed in this article are solely those of the authors and do not necessarily represent those of their affiliated organizations, or those of the publisher, the editors and the reviewers. Any product that may be evaluated in this article, or claim that may be made by its manufacturer, is not guaranteed or endorsed by the publisher.
Supplementary material
The Supplementary material for this article can be found online at: https://www.frontiersin.org/articles/10.3389/fmed.2024.1285361/full#supplementary-material
References
1. Siegel, DA, Jatlaoui, TC, Koumans, EH, Kiernan, EA, Layer, M, Cates, JE, et al. Update: interim guidance for health care providers evaluating and caring for patients with suspected e-cigarette, or vaping, product use associated lung injury—United States, October 2019. MMWR Morb Mortal Wkly Rep. (2019) 68:919–27. doi: 10.15585/mmwr.mm6841e3
2. Layden, JE, Ghinai, I, Pray, I, Kimball, A, Layer, M, Tenforde, MW, et al. Pulmonary illness related to e-cigarette use in Illinois and Wisconsin—final report. N Engl J Med. (2020) 382:903–16. doi: 10.1056/NEJMoa1911614
3. Centers for Disease Control and Prevention. Outbreak of lung injury associated with the use of e-cigarette, or vaping, products. (2021). Available at: https://www.cdc.gov/tobacco/basic_information/e-cigarettes/severe-lung-disease.html
4. Schaffer, S, Strang, A, Saul, D, Krishnan, V, and Chidekel, A. Adolescent e-cigarette or vaping use-associated lung injury in the Delaware Valley: a review of hospital-based presentation, management, and outcomes. Cureus. (2022) 14:e21988. doi: 10.7759/cureus.21988
5. Hall, W, Gartner, C, and Bonevski, B. Lessons from the public health responses to the US outbreak of vaping-related lung injury. Addiction. (2021) 116:985–93. doi: 10.1111/add.15108
6. Zulfiqar, H, Sankari, A, and Rahman, O. Vaping-associated pulmonary injury In: StatPearls. Treasure Island, FL: StatPearls Publishing LLC (2023)
7. National Center for Chronic Disease Prevention and Health Promotion. e-cigarette use among youth and young adults: a report of the surgeon general. Rockville, MD: National Center for Chronic Disease Prevention and Health Promotion (U.S.) (2016).
8. National Academies of Sciences, Engineering, and Medicine. Public health consequences of e-cigarettes. Washington DC: The National Academies Press (2018).
9. Wang, JB, Olgin, JE, Nah, G, Vittinghoff, E, Cataldo, JK, Pletcher, MJ, et al. Cigarette and e-cigarette dual use and risk of cardiopulmonary symptoms in the health eHeart study. PLoS One. (2018) 13:e0198681. doi: 10.1371/journal.pone.0198681
10. Moheimani, RS, Bhetraratana, M, Yin, F, Peters, KM, Gornbein, J, Araujo, JA, et al. Increased cardiac sympathetic activity and oxidative stress in habitual electronic cigarette users: implications for cardiovascular risk. JAMA Cardiol. (2017) 2:278–84. doi: 10.1001/jamacardio.2016.5303
11. Wills, TA, Soneji, SS, Choi, K, Jaspers, I, and Tam, EK. e-cigarette use and respiratory disorders: an integrative review of converging evidence from epidemiological and laboratory studies. Eur Respir J. (2021) 57:1901815. doi: 10.1183/13993003.01815-2019
12. Marques, P, Piqueras, L, and Sanz, MJ. An updated overview of e-cigarette impact on human health. Respir Res. (2021) 22:151. doi: 10.1186/s12931-021-01737-5
13. Banks, E, Yazidjoglou, A, Brown, S, Nguyen, M, Martin, M, Beckwith, K, et al. Electronic cigarettes and health outcomes: umbrella and systematic review of the global evidence. Med J Aust. (2023) 218:267–75. doi: 10.5694/mja2.51890
14. Canada Go. Canadian tobacco and nicotine survey (CTNS): summary of results for 2021 (2021) Available at: https://www.canada.ca/en/health-canada/services/canadian-tobacco-nicotine-survey/2021-summary.html
15. White, AV, Wambui, DW, and Pokhrel, LR. Risk assessment of inhaled diacetyl from electronic cigarette use among teens and adults. Sci Total Environ. (2021) 772:145486. doi: 10.1016/j.scitotenv.2021.145486
16. Cao, DJ, Aldy, K, Hsu, S, McGetrick, M, Verbeck, G, De Silva, I, et al. Review of health consequences of electronic cigarettes and the outbreak of electronic cigarette, or vaping, product use-associated lung injury. J Med Toxicol. (2020) 16:295–310. doi: 10.1007/s13181-020-00772-w
17. Gotts, JE, Jordt, SE, McConnell, R, and Tarran, R. What are the respiratory effects of e-cigarettes? BMJ. (2019) 366:l5275. doi: 10.1136/bmj.l5275
18. Jonas, A. Impact of vaping on respiratory health. BMJ. (2022) 378:e065997. doi: 10.1136/bmj-2021-065997
19. Blount, BC, Karwowski, MP, Shields, PG, Morel-Espinosa, M, Valentin-Blasini, L, Gardner, M, et al. Vitamin E acetate in bronchoalveolar-lavage fluid associated with EVALI. N Engl J Med. (2020) 382:697–705. doi: 10.1056/NEJMoa1916433
20. Clapp, PW, Pawlak, EA, Lackey, JT, Keating, JE, Reeber, SL, Glish, GL, et al. Flavored e-cigarette liquids and cinnamaldehyde impair respiratory innate immune cell function. Am J Physiol Lung Cell Mol Physiol. (2017) 313:L278–92. doi: 10.1152/ajplung.00452.2016
21. Stefaniak, AB, LeBouf, RF, Ranpara, AC, and Leonard, SS. Toxicology of flavoring- and cannabis-containing e-liquids used in electronic delivery systems. Pharmacol Ther. (2021) 224:107838. doi: 10.1016/j.pharmthera.2021.107838
22. Smith, MR, Jarrell, ZR, Orr, M, Liu, KH, Go, Y-M, and Jones, DP. Metabolome-wide association study of flavorant vanillin exposure in bronchial epithelial cells reveals disease-related perturbations in metabolism. Environ Int. (2021) 147:106323. doi: 10.1016/j.envint.2020.106323
23. Hickman, E, Herrera, CA, and Jaspers, I. Common e-cigarette flavoring chemicals impair neutrophil phagocytosis and oxidative burst. Chem Res Toxicol. (2019) 32:982–5. doi: 10.1021/acs.chemrestox.9b00171
24. Durrani, K, Din, S-MAE, Sun, Y, Rule, AM, and Bressler, J. Ethyl maltol enhances copper mediated cytotoxicity in lung epithelial cells. Toxicol Appl Pharmacol. (2021) 410:115354. doi: 10.1016/j.taap.2020.115354
25. Nair, V, Tran, M, Behar, RZ, Zhai, S, Cui, X, Phandthong, R, et al. Menthol in electronic cigarettes: a contributor to respiratory disease? Toxicol Appl Pharmacol. (2020) 407:115238. doi: 10.1016/j.taap.2020.115238
26. Cherinka, B, Andrews, BH, Sanchez-Gallego, J, Brownstein, J, Argudo-Fernandez, M, Blanton, M, et al. Marvin: a tool kit for streamlined access and visualization of the SDSS-IV MaNGA data set. Astron J. (2019) 158:74. doi: 10.3847/1538-3881/ab2634
27. Traboulsi, H, Cherian, M, Rjeili, MA, Preteroti, M, Bourbeau, J, Smith, BM, et al. Inhalation toxicology of vaping products and implications for pulmonary health. Int J Mol Sci. (2020) 21:3495. doi: 10.3390/ijms21103495
28. Federal Food, Drug, and Cosmetic Act. Federal Food, Drug, and Cosmetic Act. Section 201. Washington DC: US Food and Drug Administration. (1938). 409 p.
29. Cherian, SV, Kumar, A, and Estrada, YMRM. e-cigarette or vaping product-associated lung injury: a review. Am J Med. (2020) 133:657–63. doi: 10.1016/j.amjmed.2020.02.004
30. Kreiss, K, Gomaa, A, Kullman, G, Fedan, K, Simoes, EJ, and Enright, PL. Clinical bronchiolitis obliterans in workers at a microwave-popcorn plant. N Engl J Med. (2002) 347:330–8. doi: 10.1056/NEJMoa020300
31. Bracken-Clarke, D, Kapoor, D, Baird, AM, Buchanan, PJ, Gately, K, Cuffe, S, et al. Vaping and lung cancer—a review of current data and recommendations. Lung Cancer. (2021) 153:11–20. doi: 10.1016/j.lungcan.2020.12.030
32. Jacob, SE, Scheman, A, and McGowan, MA. Propylene glycol. Dermatitis. (2018) 29:3–5. doi: 10.1097/DER.0000000000000315
33. Komura, M, Sato, T, Yoshikawa, H, Nitta, NA, Suzuki, Y, Koike, K, et al. Propylene glycol, a component of electronic cigarette liquid, damages epithelial cells in human small airways. Respir Res. (2022) 23:216. doi: 10.1186/s12931-022-02142-2
34. Kim, MD, Chung, S, Dennis, JS, Yoshida, M, Aguiar, C, Aller, SP, et al. Vegetable glycerin e-cigarette aerosols cause airway inflammation and ion channel dysfunction. Front Pharmacol. (2022) 13:1012723. doi: 10.3389/fphar.2022.1012723
35. Massey, JB, She, HS, and Pownall, HJ. Interaction of vitamin E with saturated phospholipid bilayers. Biochem Biophys Res Commun. (1982) 106:842–7. doi: 10.1016/0006-291X(82)91787-9
36. Kamal, MA, and Raghunathan, VA. Modulated phases of phospholipid bilayers induced by tocopherols. Biochim Biophys Acta. (2012) 1818:2486–93. doi: 10.1016/j.bbamem.2012.06.016
37. Casals, C, and Canadas, O. Role of lipid ordered/disordered phase coexistence in pulmonary surfactant function. Biochim Biophys Acta. (2012) 1818:2550–62. doi: 10.1016/j.bbamem.2012.05.024
38. Van Bavel, N, Lai, P, Amrein, M, and Prenner, EJ. Pulmonary surfactant function and molecular architecture is disrupted in the presence of vaping additives. Colloids Surf B. (2023) 222:113132. doi: 10.1016/j.colsurfb.2023.113132
39. Winnicka, L, and Shenoy, MA. EVALI and the pulmonary toxicity of electronic cigarettes: a review. J Gen Intern Med. (2020) 35:2130–5. doi: 10.1007/s11606-020-05813-2
40. Kligerman, S, Raptis, C, Larsen, B, Henry, TS, Caporale, A, Tazelaar, H, et al. Radiologic, pathologic, clinical, and physiologic findings of electronic cigarette or vaping product use-associated lung injury (EVALI): evolving knowledge and remaining questions. Radiology. (2020) 294:491–505. doi: 10.1148/radiol.2020192585
41. Lee, HW, Park, SH, Weng, MW, Wang, HT, Huang, WC, Lepor, H, et al. e-cigarette smoke damages DNA and reduces repair activity in mouse lung, heart, and bladder as well as in human lung and bladder cells. Proc Natl Acad Sci U S A. (2018) 115:E1560–9. doi: 10.1073/pnas.1718185115
42. Ghosh, A, Coakley, RD, Ghio, AJ, Muhlebach, MS, Esther, CR Jr, Alexis, NE, et al. Chronic e-cigarette use increases neutrophil elastase and matrix metalloprotease levels in the lung. Am J Respir Crit Care Med. (2019) 200:1392–401. doi: 10.1164/rccm.201903-0615OC
43. Garcia-Arcos, I, Geraghty, P, Baumlin, N, Campos, M, Dabo, AJ, Jundi, B, et al. Chronic electronic cigarette exposure in mice induces features of COPD in a nicotine-dependent manner. Thorax. (2016) 71:1119–29. doi: 10.1136/thoraxjnl-2015-208039
44. Herriges, MJ, Pinkhasov, R, Shapiro, O, Jacob, JM, Basnet, A, Bratslavsky, G, et al. e-cigarette use and the risk of bladder and lung cancer. J Clin Oncol. (2022) 40:443. doi: 10.1200/JCO.2022.40.6_suppl.443
45. Saji, S, Patil, SS, Alleyn, M, Lockey, R, and Kolliputi, N. Nicotine in e-cigarette smoke: cancer culprit? J Cell Commun Signal. (2020) 14:127–8. doi: 10.1007/s12079-019-00519-5
46. Schaal, CM, Bora-Singhal, N, Kumar, DM, and Chellappan, SP. Regulation of Sox2 and stemness by nicotine and electronic-cigarettes in non-small cell lung cancer. Mol Cancer. (2018) 17:149. doi: 10.1186/s12943-018-0901-2
47. Salzman, GA, Alqawasma, M, and Asad, H. Vaping associated lung injury (EVALI): an explosive United States epidemic. Mo Med. (2019) 116:492–6.
48. Muthumalage, T, Lamb, T, Friedman, MR, and Rahman, I. e-cigarette flavored pods induce inflammation, epithelial barrier dysfunction, and DNA damage in lung epithelial cells and monocytes. Sci Rep. (2019) 9:19035. doi: 10.1038/s41598-019-51643-6
49. Bitzer, ZT, Goel, R, Reilly, SM, Elias, RJ, Silakov, A, Foulds, J, et al. Effect of flavoring chemicals on free radical formation in electronic cigarette aerosols. Free Radic Biol Med. (2018) 120:72–9. doi: 10.1016/j.freeradbiomed.2018.03.020
50. Tsai, M, Byun, MK, Shin, J, and Crotty Alexander, LE. Effects of e-cigarettes and vaping devices on cardiac and pulmonary physiology. J Physiol. (2020) 598:5039–62. doi: 10.1113/JP279754
51. Bodas, M, Van Westphal, C, Carpenter-Thompson, R, Arnold, EC 4th, Johnson, SC, and Harb, A. Nicotine exposure induces bronchial epithelial cell apoptosis and senescence via ROS mediated autophagy-impairment. Free Radic Biol Med. (2016) 97:441–53. doi: 10.1016/j.freeradbiomed.2016.06.017
52. Krishna, R, Anjum, F, and Oliver, TI. Bronchiolitis obliterans In: StatPearls. Treasure Island, FL: StatPearls (2023)
53. Yang, X, Kong, J, Yang, MX, Xie, WP, Wang, H, Rong, R, et al. Analysis of clinical characteristics of 21 cases of acute fibrinous and organizing pneumonia. Zhonghua Jie He He Hu Xi Za Zhi. (2020) 43:670–6. doi: 10.3760/cma.j.cn112147-20191014-00685
54. Jeon, J, Zhang, Q, Chepaitis, PS, Greenwald, R, Black, M, and Wright, C. Toxicological assessment of particulate and metal hazards associated with vaping frequency and device age. Toxics. (2023) 11:155. doi: 10.3390/toxics11020155
55. Taucher, E, Mykoliuk, I, Lindenmann, J, and Smolle-Juettner, FM. Implications of the immune landscape in COPD and lung cancer: smoking versus other causes. Front Immunol. (2022) 13:846605. doi: 10.3389/fimmu.2022.846605
56. Fei, QY, Weng, XQ, Liu, K, Liu, S, Chen, JM, Guo, XR, et al. The relationship between metal exposure and chronic obstructive pulmonary disease in the general US population: NHANES 2015–2016. Int J Environ Res Pub Health. (2022) 19:2085. doi: 10.3390/ijerph19042085
57. McRae, N, Gennings, C, Rivera, NR, Tamayo-Ortiz, M, Pantic, I, Amarasiriwardena, C, et al. Association between prenatal metal exposure and adverse respiratory symptoms in childhood. Environ Res. (2022) 205:112448. doi: 10.1016/j.envres.2021.112448
58. Ko, TJ, and Kim, SA. Effect of heating on physicochemical property of aerosols during vaping. Int J Environ Res Public Health. (2022) 19:1892. doi: 10.3390/ijerph19031892
59. Guerrini, V, Panettieri, RA Jr, and Gennaro, ML. Lipid-laden macrophages as biomarkers of vaping-associated lung injury. Lancet Respir Med. (2020) 8:e6. doi: 10.1016/S2213-2600(19)30476-X
60. Ghosh, A, Ahmad, S, Coakley, RD, Sassano, MF, Alexis, NE, and Tarran, R. Lipid-laden macrophages are not unique to patients with e-cigarette or vaping product use-associated lung injury. Am J Respir Crit Care Med. (2021) 203:1030–3. doi: 10.1164/rccm.202009-3507LE
61. Eddy, RL, Serajeddini, H, Knipping, D, Landman, ST, Bosma, KJ, Mackenzie, CA, et al. Pulmonary functional MRI and CT in a survivor of bronchiolitis and respiratory failure caused by e-cigarette use. Chest. (2020) 158:e147–51. doi: 10.1016/j.chest.2020.06.005
62. Conway, J. Lung imaging - two dimensional gamma scintigraphy, SPECT, CT and PET. Adv Drug Deliv Rev. (2012) 64:357–68. doi: 10.1016/j.addr.2012.01.013
63. Iking, J, Staniszewska, M, Kessler, L, Klose, JM, Luckerath, K, Fendler, WP, et al. Imaging inflammation with positron emission tomography. Biomedicine. (2021) 9:212. doi: 10.3390/biomedicines9020212
64. Nyilas, S, Bauman, G, Pusterla, O, Ramsey, K, Singer, F, Stranzinger, E, et al. Ventilation and perfusion assessed by functional MRI in children with CF: reproducibility in comparison to lung function. J Cyst Fibros. (2019) 18:543–50. doi: 10.1016/j.jcf.2018.10.003
65. Nyilas, S, Bauman, G, Pusterla, O, Sommer, G, Singer, F, Stranzinger, E, et al. Structural and functional lung impairment in primary ciliary dyskinesia. Assessment with magnetic resonance imaging and multiple breath washout in comparison to spirometry. Ann Am Thorac Soc. (2018) 15:1434–42. doi: 10.1513/AnnalsATS.201712-967OC
66. Ebner, L, Kammerman, J, Driehuys, B, Schiebler, ML, Cadman, RV, and Fain, SB. The role of hyperpolarized 129xenon in MR imaging of pulmonary function. Eur J Radiol. (2017) 86:343–52. doi: 10.1016/j.ejrad.2016.09.015
67. Kooner, HK, McIntosh, MJ, Desaigoudar, V, Rayment, JH, Eddy, RL, Driehuys, B, et al. Pulmonary functional MRI: detecting the structure-function pathologies that drive asthma symptoms and quality of life. Respirology. (2022) 27:114–33. doi: 10.1111/resp.14197
68. Fain, S, Schiebler, ML, McCormack, DG, and Parraga, G. Imaging of lung function using hyperpolarized helium-3 magnetic resonance imaging: review of current and emerging translational methods and applications. J Magn Reson Imaging. (2010) 32:1398–408. doi: 10.1002/jmri.22375
69. Vidal Melo, MF, Layfield, D, Harris, RS, O’Neill, K, Musch, G, Richter, T, et al. Quantification of regional ventilation-perfusion ratios with PET. J Nucl Med. (2003) 44:1982–91.
70. Altes, TA, Rehm, PK, Harrell, F, Salerno, M, Daniel, TM, and De Lange, EE. Ventilation imaging of the lung: comparison of hyperpolarized helium-3 MR imaging with Xe-133 scintigraphy. Acad Radiol. (2004) 11:729–34. doi: 10.1016/j.acra.2004.04.001
71. Santyr, GE, Couch, MJ, Lam, WW, Ouriadov, A, Drangova, M, McCormack, DG, et al. Comparison of hyperpolarized 3He MRI with Xe-enhanced computed tomography imaging for ventilation mapping of rat lung. NMR Biomed. (2011) 24:1073–80. doi: 10.1002/nbm.1659
72. Mummy, DG, Dunican, EM, Carey, KJ, Evans, MD, Elicker, BM, Newell, JD Jr, et al. Mucus plugs in asthma at CT associated with regional ventilation defects at helium 3 MRI. Radiology. (2021) 303:184–90. doi: 10.1148/radiol.2021204616
73. Svenningsen, S, Eddy, RL, Lim, HF, Cox, PG, Nair, P, and Parraga, G. Sputum eosinophilia and magnetic resonance imaging ventilation heterogeneity in severe asthma. Am J Respir Crit Care Med. (2018) 197:876–84. doi: 10.1164/rccm.201709-1948OC
74. Svenningsen, S, Haider, E, Boylan, C, Mukherjee, M, Eddy, RL, Capaldi, DPI, et al. CT and functional MRI to evaluate airway mucus in severe asthma. Chest. (2019) 155:1178–89. doi: 10.1016/j.chest.2019.02.403
75. Svenningsen, S, Kirby, M, Starr, D, Coxson, HO, Paterson, NA, McCormack, DG, et al. What are ventilation defects in asthma? Thorax. (2014) 69:63–71. doi: 10.1136/thoraxjnl-2013-203711
76. Kirby, M, Svenningsen, S, Kanhere, N, Owrangi, A, Wheatley, A, Coxson, HO, et al. Pulmonary ventilation visualized using hyperpolarized helium-3 and xenon-129 magnetic resonance imaging: differences in COPD and relationship to emphysema. J Appl Physiol. (2013) 114:707–15. doi: 10.1152/japplphysiol.01206.2012
77. Sheikh, K, Coxson, HO, and Parraga, G. This is what COPD looks like. Respirology. (2016) 21:224–36. doi: 10.1111/resp.12611
78. Santyr, G, Kanhere, N, Morgado, F, Rayment, JH, Ratjen, F, and Couch, MJ. Hyperpolarized gas magnetic resonance imaging of pediatric cystic fibrosis lung disease. Acad Radiol. (2019) 26:344–54. doi: 10.1016/j.acra.2018.04.024
79. Mammarappallil, JG, Rankine, L, Wild, JM, and Driehuys, B. New developments in imaging idiopathic pulmonary fibrosis with hyperpolarized xenon magnetic resonance imaging. J Thorac Imaging. (2019) 34:136–50. doi: 10.1097/RTI.0000000000000392
80. FDA approves Polarean’s XENOVIEWTM (xenon Xe 129 hyperpolarized) for use with MRI for the evaluation of lung ventilation. North Carolina USA: Polarean Imaging PLC. (Press release). (2022).
81. Sa, RC, Cronin, MV, Henderson, AC, Holverda, S, Theilmann, RJ, Arai, TJ, et al. Vertical distribution of specific ventilation in normal supine humans measured by oxygen-enhanced proton MRI. J Appl Physiol. (1985) 109:1950–9. doi: 10.1152/japplphysiol.00220.2010
82. Morgan, AR, Parker, GJ, Roberts, C, Buonaccorsi, GA, Maguire, NC, Hubbard Cristinacce, PL, et al. Feasibility assessment of using oxygen-enhanced magnetic resonance imaging for evaluating the effect of pharmacological treatment in COPD. Eur J Radiol. (2014) 83:2093–101. doi: 10.1016/j.ejrad.2014.08.004
83. Ohno, Y, Nishio, M, Koyama, H, Yoshikawa, T, Matsumoto, S, Seki, S, et al. Oxygen-enhanced MRI for patients with connective tissue diseases: comparison with thin-section CT of capability for pulmonary functional and disease severity assessment. Eur J Radiol. (2014) 83:391–7. doi: 10.1016/j.ejrad.2013.11.001
84. Zhang, WJ, Niven, RM, Young, SS, Liu, YZ, Parker, GJ, and Naish, JH. Dynamic oxygen-enhanced magnetic resonance imaging of the lung in asthma—initial experience. Eur J Radiol. (2015) 84:318–26. doi: 10.1016/j.ejrad.2014.10.021
85. Capaldi, DP, Sheikh, K, Guo, F, Svenningsen, S, Etemad-Rezai, R, Coxson, HO, et al. Free-breathing pulmonary 1H and hyperpolarized 3He MRI: comparison in COPD and bronchiectasis. Acad Radiol. (2015) 22:320–9. doi: 10.1016/j.acra.2014.10.003
86. Klimes, F, Voskrebenzev, A, Gutberlet, M, Kern, AL, Behrendt, L, Grimm, R, et al. 3D phase-resolved functional lung ventilation MR imaging in healthy volunteers and patients with chronic pulmonary disease. Magn Reson Med. (2021) 85:912–25. doi: 10.1002/mrm.28482
87. Matheson, AM, Capaldi, DP, Guo, F, Eddy, RL, McCormack, DG, and Parraga, G. Fourier decomposition free-breathing 1H MRI perfusion maps in asthma Medical Imaging 2019: Image Processing; (2019)
88. Voskrebenzev, A, Gutberlet, M, Klimes, F, Kaireit, TF, Schonfeld, C, Rotarmel, A, et al. Feasibility of quantitative regional ventilation and perfusion mapping with phase-resolved functional lung (PREFUL) MRI in healthy volunteers and COPD, CTEPH, and CF patients. Magn Reson Med. (2018) 79:2306–14. doi: 10.1002/mrm.26893
89. Bauman, G, and Bieri, O. Matrix pencil decomposition of time-resolved proton MRI for robust and improved assessment of pulmonary ventilation and perfusion. Magn Reson Med. (2017) 77:336–42. doi: 10.1002/mrm.26096
90. Bolar, DS, Levin, DL, Hopkins, SR, Frank, LF, Liu, TT, Wong, EC, et al. Quantification of regional pulmonary blood flow using ASL-FAIRER. Magn Reson Med. (2006) 55:1308–17. doi: 10.1002/mrm.20891
91. Landman, ST, Dhaliwal, I, Mackenzie, CA, Martinu, T, Steel, A, and Bosma, KJ. Life-threatening bronchiolitis related to electronic cigarette use in a Canadian youth. CMAJ. (2019) 191:E1321–31. doi: 10.1503/cmaj.191402
92. Wall, A, Roslin, S, Borg, B, McDermott, S, Walele, T, Nahde, T, et al. e-cigarette aerosol deposition and disposition of [11C]nicotine using positron emission tomography: a comparison of nicotine uptake in lungs and brain using two different nicotine formulations. Pharmaceuticals. (2022) 15:367. doi: 10.3390/ph15030367
93. Hansell, DM, Bankier, AA, MacMahon, H, McLoud, TC, Muller, NL, and Remy, J. Fleischner society: glossary of terms for thoracic imaging. Radiology. (2008) 246:697–722. doi: 10.1148/radiol.2462070712
94. Kligerman, SJ, Kay, FU, Raptis, CA, Henry, TS, Sechrist, JW, Walker, CM, et al. CT findings and patterns of e-cigarette or vaping product use-associated lung injury: a multicenter cohort of 160 cases. Chest. (2021) 160:1492–511. doi: 10.1016/j.chest.2021.04.054
95. Aberegg, SK, Cirulis, MM, Maddock, SD, Freeman, A, Keenan, LM, Pirozzi, CS, et al. Clinical, bronchoscopic, and imaging findings of e-cigarette, or vaping, product use-associated lung injury among patients treated at an Academic Medical Center. JAMA Netw Open. (2020) 3:e2019176. doi: 10.1001/jamanetworkopen.2020.19176
96. Artunduaga, M, Rao, D, Friedman, J, Kwon, JK, Pfeifer, CM, Dettori, A, et al. Pediatric chest radiographic and CT findings of electronic cigarette or vaping product use-associated lung injury (EVALI). Radiology. (2020) 295:430–8. doi: 10.1148/radiol.2020192778
97. Carroll, BJ, Kim, M, Hemyari, A, Thakrar, P, Kump, TE, Wade, T, et al. Impaired lung function following e-cigarette or vaping product use associated lung injury in the first cohort of hospitalized adolescents. Pediatr Pulmonol. (2020) 55:1712–8. doi: 10.1002/ppul.24787
98. Chidambaram, AG, Dennis, RA, Biko, DM, Hook, M, Allen, J, and Rapp, JB. Clinical and radiological characteristics of e-cigarette or vaping product use associated lung injury. Emerg Radiol. (2020) 27:495–501. doi: 10.1007/s10140-020-01796-z
99. Harry-Hernandez, S, Thiboutot, J, Wahidi, MM, Giovacchini, CX, De Cardenas, J, Meldrum, C, et al. Bronchoalveolar lavage (BAL) and pathologic assessment of electronic cigarette or vaping product use-associated lung injury (EVALI): the EVALI-BAL study, a multicenter cohort. J Bronchology Interv Pulmonol. (2023) 30:144–54. doi: 10.1097/LBR.0000000000000890
100. Helfgott, D, Capozzoli, G, Madray, J, Baig, A, Uppaluri, L, Gaur, S, et al. e-cigarette or vaping product use associated lung injury (EVALI) in the time of COVID-19: a clinical dilemma. Pediatr Pulmonol. (2022) 57:623–30. doi: 10.1002/ppul.25804
101. Kalininskiy, A, Bach, CT, Nacca, NE, Ginsberg, G, Marraffa, J, Navarette, KA, et al. e-cigarette, or vaping, product use associated lung injury (EVALI): case series and diagnostic approach. Lancet Respir Med. (2019) 7:1017–26. doi: 10.1016/S2213-2600(19)30415-1
102. Layden, JE, and Meiman, JG. Pulmonary disease related to e-cigarette use. Reply. N Engl J Med. (2020) 383:793. doi: 10.1056/NEJMc2009932
103. Pajak, A, Bascoy, S, Li, JC, Benninghoff, M, and Deitchman, A. e-cigarette or vaping product use associated lung injury among three young adults: a retrospective case series from Delaware. Cureus. (2020) 12:e11031. doi: 10.7759/cureus.11031
104. Panse, PM, Feller, FF, Butt, YM, Smith, ML, Larsen, BT, Tazelaar, HD, et al. Pulmonary injury resulting from vaping or e-cigarette use: imaging appearances at presentation and follow-up. Radiol Cardiothorac Imaging. (2020) 2:e200081. doi: 10.1148/ryct.2020200081
105. Rao, DR, Maple, KL, Dettori, A, Afolabi, F, Francis, JKR, Artunduaga, M, et al. Clinical features of e-cigarette, or vaping, product use-associated lung injury in teenagers. Pediatrics. (2020) 146:e20194104. doi: 10.1542/peds.2019-4104
106. Wang, KY, Jadhav, SP, Yenduri, NJS, Lee, SA, Farber, HJ, and Guillerman, RP. e-cigarette or vaping product use-associated lung injury in the pediatric population: imaging features at presentation and short-term follow-up. Pediatr Radiol. (2020) 50:1231–9. doi: 10.1007/s00247-020-04698-x
107. Kizhakke Puliyakote, AS, Elliott, AR, Sá, RC, Anderson, KM, Crotty Alexander, LE, and Hopkins, SR. Vaping disrupts ventilation-perfusion matching in asymptomatic users. J Appl Physiol. (1985) 130:308–17. doi: 10.1152/japplphysiol.00709.2020
108. Nyilas, S, Bauman, G, Korten, I, Pusterla, O, Singer, F, Ith, M, et al. MRI shows lung perfusion changes after vaping and smoking. Radiology. (2022) 304:195–204. doi: 10.1148/radiol.211327
109. Wetherill, RR, Doot, RK, Young, AJ, Lee, H, Schubert, EK, Wiers, CE, et al. Molecular imaging of pulmonary inflammation in users of electronic and combustible cigarettes: a pilot study. J Nucl Med. (2023) 64:797–802. doi: 10.2967/jnumed.122.264529
110. Han, S, Liu, C, Chen, H, Fu, Y, Zhang, Y, Miao, R, et al. Pharmacokinetics of freebase nicotine and nicotine salts following subcutaneous administration in male rats. Drug Test Anal. (2022) 15:1099–106. doi: 10.1002/dta.3363
111. Etter, JF. Explaining the effects of electronic cigarettes on craving for tobacco in recent quitters. Drug Alcohol Depend. (2015) 148:102–8. doi: 10.1016/j.drugalcdep.2014.12.030
112. Etter, JF. Characteristics of users and usage of different types of electronic cigarettes: findings from an online survey. Addiction. (2016) 111:724–33. doi: 10.1111/add.13240
113. Talih, S, Balhas, Z, Eissenberg, T, Salman, R, Karaoghlanian, N, El Hellani, A, et al. Effects of user puff topography, device voltage, and liquid nicotine concentration on electronic cigarette nicotine yield: measurements and model predictions. Nicotine Tob Res. (2015) 17:150–7. doi: 10.1093/ntr/ntu174
Keywords: e-cigarettes, MRI, CT, PET, EVALI
Citation: Hofmann JJ, Poulos VC, Zhou J, Sharma M, Parraga G and McIntosh MJ (2024) Review of quantitative and functional lung imaging evidence of vaping-related lung injury. Front. Med. 11:1285361. doi: 10.3389/fmed.2024.1285361
Edited by:
Mark O. Wielpütz, Heidelberg University, GermanyReviewed by:
Gaetano Rea, Monaldi Hospital, ItalyLaura Saunders, The University of Sheffield, United Kingdom
Copyright © 2024 Hofmann, Poulos, Zhou, Sharma, Parraga and McIntosh. This is an open-access article distributed under the terms of the Creative Commons Attribution License (CC BY). The use, distribution or reproduction in other forums is permitted, provided the original author(s) and the copyright owner(s) are credited and that the original publication in this journal is cited, in accordance with accepted academic practice. No use, distribution or reproduction is permitted which does not comply with these terms.
*Correspondence: Grace Parraga, Z3BhcnJhZ2FAdXdvLmNh
†These authors have contributed equally to this work and share first authorship