- 1Creighton University School of Medicine, Omaha, NE, United States
- 2College of Medicine, University of Nebraska Medical Center, Omaha, NE, United States
- 3Department of Ophthalmology and Visual Sciences, Stanley M. Truhlsen Eye Institute, University of Nebraska Medical Center, Omaha, NE, United States
- 4Department of Ophthalmology, University of Illinois-Chicago, Chicago, IL, United States
- 5Department of Microbiology and Pathology, University of Nebraska Medical Center, Omaha, NE, United States
- 6Walter Reed National Military Medical Center, Bethesda, MD, United States
- 7Department of Ophthalmology, Emory University School of Medicine, Atlanta, GA, United States
- 8Global Center for Health Security, University of Nebraska Medical Center, Omaha, NE, United States
- 9National Strategic Research Institute, University of Nebraska Medical Center, Omaha, NE, United States
As technology continues to evolve, the possibility for a wide range of dangers to people, organizations, and countries escalate globally. The United States federal government classifies types of threats with the capability of inflicting mass casualties and societal disruption as Chemical, Biological, Radiological, Nuclear, and Energetics/Explosives (CBRNE). Such incidents encompass accidental and intentional events ranging from weapons of mass destruction and bioterrorism to fires or spills involving hazardous or radiologic material. All of these have the capacity to inflict death or severe physical, neurological, and/or sensorial disabilities if injuries are not diagnosed and treated in a timely manner. Ophthalmic injury can provide important insight into understanding and treating patients impacted by CBRNE agents; however, improper ophthalmic management can result in suboptimal patient outcomes. This review specifically addresses the biological agents the Center for Disease Control and Prevention (CDC) deems to have the greatest capacity for bioterrorism. CBRNE biological agents, encompassing pathogens and organic toxins, are further subdivided into categories A, B, and C according to their national security threat level. In our compendium of these biological agents, we address their respective CDC category, systemic and ophthalmic manifestations, route of transmission and personal protective equipment considerations as well as pertinent vaccination and treatment guidelines.
1 Introduction
Recent outbreaks of Ebola virus disease, monkeypox, and the COVID-19 pandemic, which are public health emergencies of international concern (PHEIC) per World Health Organization (WHO) criteria, have provided insights into the clinical impact, socioeconomic implications, and widespread disruption of society (1–3). Identifying and understanding these infectious disease threats could be extended to potential engineered pathogens as well.
As technology evolves, a wide range of threats to service members, diplomats, and United States (US) citizens emerges. The US federal government classifies types of threats with the capability of inflicting mass casualties and societal disruption as Chemical, Biological, Radiological, Nuclear, and Energetics/Explosives (CBRNE) (4, 5). These incidents include accidental events like chemical waste spills to intentional use of technology like weapons of mass destruction (5, 6). CBRNE events have the potential to inflict death or severe disabilities in the absence of accurate diagnosis and timely treatment. There are various echelons of CBRNE initiatives within the US aimed to prevent, prepare for, respond to, and recover from these incidents (4–6). However, to date, we are unaware of a centralized resource for CBRNE incidents as they pertain to ophthalmic disease and countermeasures. Ophthalmic injury can provide key insight into understanding and treating patients impacted by CBRNE agents, while improper diagnosis and management can lead to debilitating implications for patients’ vision and quality of life.
CBRNE biological agents encompass pathogens and toxins from microbes and plants. The Center for Disease Control and Prevention (CDC) classifies biological agents into categories A, B, and C according to their national security threat level using the following elements: ease of dissemination; mortality and morbidity rates; capacity for inciting public panic and social disruption; and requirements for special public health preparations (Table 1) (7). Category A agents are of highest priority, Category B of moderate importance, and Category C agents are emerging in nature and require further research for detection, diagnosis and treatment (8).
This is a comprehensive summary of the biological agents with special focus in relation to CBRNE preparation and management as identified by the CDC. We address the CDC category, the clinical manifestations and ophthalmic findings, the route of transmission and personal protective equipment (PPE) considerations, and the current vaccination and treatment guidelines for each identified biological agent in this review.
2 Viruses
A wide array of viruses is found in biological agent Categories A, B, and C. Proper hand washing and surface disinfection practices aid in prevention of nosocomial dissemination (9). Since a considerable number of viral infections are zoonotic, general preventative measures involve limiting contact with vectors and reducing direct and indirect contact with natural reservoirs and intermediate hosts. For example, removal of standing water sources when handling mosquito-borne infections reduces vector propagation, thus reducing disease burden (10).
Multiple viruses may be shed in the tear film and subsequently pose additional risk to eye care providers; therefore, ophthalmologic environmental interventions may include transparent shields on slit lamps, disinfection of potentially contaminated surfaces and instruments between patients, and implementation of telemedicine initiatives. Awareness of potential aerosol generation such as air puff-like tonometry is also critical to risk mitigation (11, 12). Appendix 1 summarizes key viruses according to the CDC categories.
2.1 Category A
Category A viruses encompass viral hemorrhagic fevers (VHFs) including arenaviruses (Lassa and Machupo viruses) and filoviruses (Marburg and Ebola viruses), and smallpox (variola major) (7).
2.1.1 Arenaviruses: Lassa fever and Machupo virus
Arenaviruses cause zoonotic hemorrhagic diseases via rodents and include Lassa fever and Machupo viruses (13). Rodent-to-human transmission occurs through contact with urine, feces, and saliva in contaminated food, aerosolized particles, and epidermal barrier lesions. Human-to-human transmission occurs through direct contact with infectious body fluids and contaminated fomites (14). Lassa fever is an arenavirus endemic to Africa and transmitted by the Mastomys natalensis mouse (13). Systemic manifestations include fever, exudative pharyngitis, proteinuria, emesis, sensorineural hearing loss, and other neurologic complications. Patients may progress into acute hemorrhagic fever and multi-organ failure. Ophthalmic findings of Lassa fever are conjunctivitis and subconjunctival hemorrhage in an acute disease state, with potential for visual acuity decline over time due to anterior and posterior segment pathology (15).
Machupo virus is the etiologic agent of Bolivian Hemorrhagic Fever and is transmitted by the Calomys callosus vesper mouse (16, 17). Systemic manifestations are a flu-like syndrome (16). Less than one third of cases may progress to neurologic and hemorrhagic syndromes culminating in multi-organ failure and death. Ophthalmic findings include conjunctivitis, conjunctival congestion, and periorbital edema. Current treatment is supportive care. Some early animal trials and per os administration suggest potential benefits of ribavirin and favipiravir as treatment and prophylaxis for Lassa fever and Machupo viral infections (13, 16).
2.1.2 Filoviruses: Marburg and Ebola virus diseases
Marburg and Ebola virus diseases are caused by members of the Filoviridae family—Marburg virus (MARV) and Ebola virus (EBOV), respectively (18). MARV is transmitted from its reservoir host, the African fruit bat, to humans and nonhuman primates (NHPs) through infectious body fluids and contaminated fruits (19). Infected NHPs can also serve as intermediate hosts for MARV transmission to humans through direct contact and bushmeat consumption while human-to-human transmission occurs via body fluids and contaminated fomites. High-risk populations for filovirus infection include health care workers and those involved in the burial of infected human corpses (19, 20). Systemic MARV manifestations are grouped into three phases: Phase 1: flu-like illness and fever; Phase 2: neurological and hemorrhagic symptoms; and Phase 3: prolonged phase of restoration or multi-organ failure and death (19). There have been reports of acute anterior uveitis three months after initial disease onset (21, 22). Current treatment is supportive care with no effective vaccines or therapeutics available (19).
EBOV is highly fatal and demonstrates similar human-to-human transmission mechanisms as MARV especially in instances of accidental needlesticks by healthcare workers (20, 23). Although EBOV’s natural reservoir is unknown, bats are often implicated (20). Systemic manifestations are divided into three phases: Phase 1: Nonspecific symptoms and fever that progresses to intractable vomiting and watery diarrhea; Phase 2: Illness peaks with meningoencephalitis, acute kidney injury, adrenal insufficiency, pulmonary vascular leakage, pericarditis, and pancreatitis; and Phase 3: development of late-onset sequelae including ophthalmic and otologic complications, cognitive difficulties, and musculoskeletal pain and weakness (24). Ophthalmic findings during acute infection are conjunctival hemorrhages and vision loss, and after convalescence patients may present with anterior uveitis followed by posterior uveitis (25–27). Other ophthalmic sequelae include eye pain, redness, photophobia with acute or chronic unilateral vision loss, episcleritis, interstitial keratitis, and cataracts. A slit lamp exam may reveal nonspecific signs of active or old inflammation, as well as retinal and peripapillary lesions (Figure 1). Like MARV, current treatment is supportive care with no effective vaccines or therapeutics available.
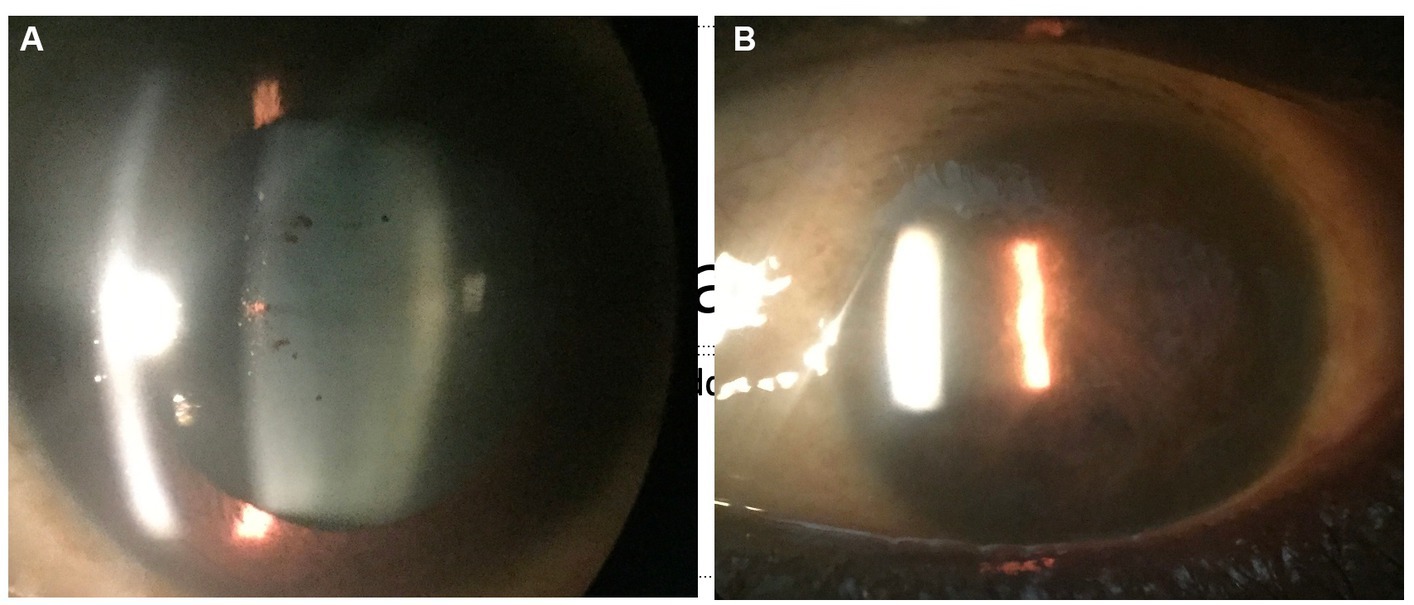
Figure 1. Anterior segment photograph of an Ebola virus disease survivor shows pigment on the lens capsule (A), indicative of prior uveitis. In a West African survivor who was seen late in the uveitis disease course, complete seclusion of the pupil from extensive posterior synechiae and chronic inflammation is observed (B).
2.1.3 Variola major: smallpox
Variola major virus, the etiological agent of smallpox, is an Orthopoxvirus that significantly impacted human populations for centuries until vaccination efforts globally eradicated this notorious pathogen in 1980 (28). Changes in global population health, vaccination hesitance, and increased intercontinental contact portend risks for pandemics and bioterrorism (28, 29). Transmission of variola virus involves inhalation of microdroplets from the respiratory tract, skin, and body fluids of infected patients. Systemic manifestations include a nonspecific prodromal phase followed by a rash of characteristic small cutaneous lesions that synchronously progress to scabs and pock scars (28). Vaccination with the vaccinia virus, a live attenuated poxvirus like smallpox and treatment with cidofovir are used to prevent and manage systemic infection (28, 29). Ophthalmic findings include pustular rash, edema, discharge, and dried secretions of the eyelids; conjunctival pustules that induce pain, photophobia, and lacrimation; corneal ulceration and perforation, iris prolapse, hypopyon, staphyloma, and endophthalmitis (9). Additional ophthalmic findings may include iritis, iridocyclitis, secondary glaucoma, and disciform keratitis, among others (9, 30–32). Subsequent treatments may involve topical antivirals, vaccinia immunoglobulins, and combination therapy with antivirals and topical steroids.
2.2 Category B
2.2.1 Togaviruses: Venezuelan encephalomyelitis and Western equine encephalomyelitis
Category B viruses consist of three alphaviruses from the Togaviridae family with severe morbidity and mortality—Venezuelan encephalomyelitis (VEEV) and Eastern and Western Equine encephalomyelitides (EEEV, WEEV) (7, 33, 34). Although primarily transmitted by mosquitos between equine, the natural reservoir, and humans, these viruses have been aerosolized as highly infectious virions employed in biowarfare (34, 35). Shared systemic manifestations for all three encephalomyelitides includes an asymptomatic febrile incubation period, symptomatic phase encephalopathy, and high case fatalities, especially with EEEV (50–75%) (33). Severe neurologic sequelae present in survivors (VEEV 4–14%, EEEV 50–90%, WEEV 15–30%) include emotional instability, seizures, and cognitive, sensory, and motor deficits (10, 33).
Ophthalmic findings in addition to those seen in encephalitis with papilledema from elevated intracranial pressure involve occasional optic neuritis and/or cranial nerve (CN) palsies (CN-VI, CN-VII) affecting vision (10). Those with VEEV and WEEV may also exhibit conjunctivitis, eye pain, and photophobia. Current treatment is supportive care. Although several vaccine candidates are under investigation, none have been approved for use in humans (34, 35). Preventive methods entail vector control by thorough elimination of standing water sources, bed nets, insect repellents and mosquito-repellent clothing (10).
2.3 Category C
Category C agents include Nipah virus (NiV) and Hantaviruses (7).
2.3.1 Hantavirus
Hantaviruses are rodent-carried viruses transmitted by aerosols of excreta, saliva, and urine; however, they are rarely transmitted human-to-human (36, 37). These viruses are subdivided according to their geographic distribution and unique systemic manifestations following a nonspecific symptom phase. ‘New World’ hantaviruses, predominately in the Americas, present with cardiopulmonary syndrome (HCPS) that progresses to organ failure. ‘Old World’ hantaviruses are endemic to Europe and Asia and present as hemorrhagic fever with renal syndrome (HFRS) and potential renal failure (36, 38). The first ophthalmic finding is acute, transient myopia, which is primarily associated with the HFRS-causing Puumala hantavirus (39, 40). One case reported a co-presentation of assumed hantavirus necrotizing retinitis and HFRS (41). Additional manifestations include anterior segment changes such as conjunctival chemosis, lens thickening, vitreous length shallowing, and macular features such as intraretinal hemorrhages, in addition to disc streak hemorrhages (42). Current treatment is supportive care with no effective vaccines or therapeutics available (43).
2.3.2 Nipah virus
Nipah virus (NiV), a member of the Paramyxoviridae family, is a zoonotic disease naturally found in fruit bats with spillover into intermediate hosts (pigs and horses) and humans (44). Reported transmission from bats was associated with bat-bitten fruit and bat saliva- and excreta-contaminated date palm sap (44–47). Human-to-intermediate hosts and human-to-human NiV transmission has been recorded in association with urine, saliva and respiratory secretions in addition to direct contact, fomites, and aerosols (45, 46, 48, 49). Severe respiratory and neurologic systemic manifestations encompass acute respiratory distress syndrome pneumonia, encephalitis, meningitis, seizures, and multiple organ dysfunction syndrome with a 40–70% case fatality (44–47). Several small studies report the following ophthalmic findings: nystagmus, CN-VI palsy and transient blindness during the acute phase of the illness; branch retinal artery occlusion, CN-VI palsy and Horner’s syndrome upon follow up with a higher mortality associated with doll’s eye reflex and pin-point pupils (50, 51). Current treatment is supportive care with no effective vaccines or therapeutics available (47).
3 Bacteria
Many bacteria are noted in the CDC’s tripartite partitioning of biological agents within the CBRNE framework. Appendix 2 contains a summary of the bacteria and toxins according to CDC categories.
3.1 Category A
Category A bacteria include Bacillus anthracis, Yersinia pestis, and Francisella tularensis, and the botulinum toxin of Clostridium botulinum (7).
3.1.1 Bacillus anthracis: anthrax
Bacillus anthracis is a spore-forming, aerobic, Gram-positive rod that rose to public notoriety following the 2001 Anthrax letters (52, 53). Produced by the Soviet Union in at least one military research facility in 1979 (54), the bacterium is capable of cutaneous, respiratory, and gastrointestinal forms, which arise from the entrance of endospores into the body via breaks in the skin, by inhalation, or by ingestion. The vast majority of reported cases are cutaneous, and the gastrointestinal form is quite rare (55). Inhalational anthrax, also known as wool sorter’s disease, is the cause of bioterror potential and involves germination of endospores in the lungs (55). Systemic manifestations of the disease include an early phase of fever, malaise, headache, and a nonproductive cough followed by a secondary phase of dyspnea and hypoxemia that can progress to septic shock (55). Ophthalmic findings of anthrax are limited and seen in the cutaneous form, with the characteristic black eschar seen on the eyelids (56). Current treatment of inhalational anthrax includes two months of intravenous antimicrobial combination therapy of at least one bactericidal drug and one protein synthesis inhibitor, an antitoxin, and postexposure prophylactic vaccination (57–59).
3.1.2 Botulinum toxin (Clostridium botulinum): botulism
The botulinum toxin, produced by Clostridium botulinum, can be spread via foodborne vectors (consumption of spores in children or preformed toxin in adults), direct wound colonization and toxin production by C. botulinum, inhalation of aerosolized toxin, or an iatrogenic route via exposure to injectable therapeutic toxin (60). Regardless of the mode of transmission, infection from botulinum toxin results in a characteristic bilateral, symmetric, flaccid paralysis that can lead to respiratory failure. Ophthalmic manifestations include photophobia, ptosis, diplopia, mydriasis, and extraocular/eyelid paralysis (61). Treatment of botulism includes antitoxin administration and supportive care, and intubation may be required in instances of airway protection and respiratory failure (60, 62).
3.1.3 Francisella tularensis: tularemia
Francisella tularensis, like Yersinia pestis, is a poorly staining Gram-negative coccobacillus that can be transmitted by ingestion or inhalation and via tick bites (63). Studied by several countries in the 20th century and probably used to some degree in World War II (WWII), Tularemia presents with six forms – ulceroglandular, glandular, oculoglandular, oropharyngeal, typhoidal and respiratory (64–66). Each of these forms begins with a nonspecific, flu-like phase of headache, fever, fatigue, chills, and myalgias (64). Given the scope of this article, the focus will be on the oculoglandular form. As the only ophthalmic form of tularemia, this localized form manifests with symptoms of photophobia, lacrimation, conjunctivitis, yellow conjunctival ulcers, chemosis, and eyelid edema (10, 63, 67). These symptoms coincide with the formation of regional pre-auricular or submandibular lymphadenopathy, and treatment should include 10–21 days of tetracycline, aminoglycoside, or fluoroquinolone antibiotics (10).
3.1.4 Yersinia pestis: plague
Yersinia pestis, commonly known as plague, is a Gram-negative coccobacillus transmitted by flea bites, infected animal contact, and inhalation (68). Plague can manifest in bubonic, septicemic, and pneumonic forms, with pneumonic being the most likely bioterror threat due to the potential for particles to be aerosolized. In WWII, the Japanese military used plague on prisoners at Manchuria, and even deployed plague-infected fleas in a number of cities in China (69, 70). This form begins with a sudden onset of headache, chills, fever, tachypnea, tachycardia, and a cough that progresses from dry to hemoptysis (68, 71). Ocular plague has been described in mule deer, but no cases have been reported in humans (72). Infections can be successfully treated with an aminoglycoside, such as streptomycin (68, 71, 73).
3.2 Category B
The CDC has designated the following ten bacteria and two bacterial toxins as Category B agents.
3.2.1 Brucella spp.: brucellosis
Brucellosis is the clinical disease caused by species of the Brucella genus. Like many bacteria, Brucella can be transmitted by ingestion of contaminated food, inhalation, or contact with mucous membranes (74). The clinical manifestations of brucellosis are both numerous and nonspecific. Patients may present with flu-like illness, abdominal pain, hepatomegaly and splenomegaly, or arthralgia, with more severe cases reporting endocarditis, motor and cranial nerve deficits, meningitis, seizures, bronchopneumonia, and pleural adhesion (75). Despite the many clinical manifestations reported, ophthalmic manifestation is infrequent. One 26-year study in Peru described 52 patients with ocular brucellosis, with uveitis being the most common presentation although keratitis and conjunctivitis were also reported (76). Treatment of brucellosis is difficult, requiring a combination therapy of doxycycline plus streptomycin/gentamicin or doxycycline plus rifampin (77).
3.2.2 Burkholderia mallei: glanders
Burkholderia mallei is a Gram-negative intracellular bacterium, and the causative agent of glanders. While glanders is rarely seen in developed countries today, it was one of the first biological agents used in warfare during the World War I and employed to impact adversarial transport animals (78). Transmission and human infection can occur through direct contact with damaged skin or mucosal membranes and inhalation (79, 80). Clinical presentation of glanders can range from a localized infection to septicemia. Generalized symptoms include fever, fatigue, headache, myalgias, and lymphadenopathy, and localized infections characterized by focal areas of suppuration that may ulcerate. Ophthalmic symptoms are generally due to localized eye infection of the conjunctiva, resulting in photophobia and excessive lacrimation (80). Pulmonary infection due to inhalation can cause a productive cough, dyspnea, and chest pain with pneumonia, pleuritis, or abscess formation. Dissemination of infection to the bloodstream can lead to bacterial colonization and abscess formation in nearly any organ (79, 80). Due to the lack of recent human glanders cases, treatment options have not been well described, but B. mallei has been shown to be susceptible to doxycycline, imipenem, ceftazidime, ciprofloxacin, piperacillin, and aminoglycosides (81).
3.2.3 Burkholderia pseudomallei: melioidosis
Despite being from the same genus, Burkholderia pseudomallei causes a separate clinical disease known as Melioidosis. Unlike glanders, human melioidosis cases are still known to occur in tropical and subtropical regions with endemic areas including Australia, southeast Asia, and India (82). Human infection typically occurs via inhalation or contact with contaminated water or soil (83). Pneumonia is the most common presentation and is associated with subsequent bacteremia. These patients typically present with a productive cough and dyspnea with fever and abscess formation following dissemination like glanders. Melioidosis can also present with a localized ulcerative infection (79, 83). Ocular melioidosis is rare but may present with symptoms such as orbital cellulitis, endophthalmitis, corneal ulceration, and dacryocystitis (84). Current regimens include ceftazidime or a carbapenem followed by trimethoprim-sulfamethoxazole (81, 83). Employment of biosafety level 3 precautions for laboratory workers has been suggested by some researchers (82, 83).
3.2.4 Chlamydia psittaci: psittacosis
Psittacosis is considered an atypical pneumonia caused by the Gram negative, intracellular bacterium Chlamydia psittaci (85, 86). The bacterium commonly infects both domestic and wild birds and can be transmitted to humans via inhalation of aerosolized feces or feather dust (85, 86). Systemic signs of psittacosis include an abrupt onset flu-like illness of fever, headache, chills, myalgias, fatigue, and cough, with less common manifestations of hepatosplenomegaly and peri-, endo-, or myocarditis (86, 87). The most commonly reported ophthalmic symptom of psittacosis is keratoconjunctivitis although this is still rare and typically reported in bird fanciers or laboratory workers (88). An association between psittacosis and ocular adnexal lymphoma has been described although this is still contested (89). Treatment of psittacosis with oral doxycycline is effective, and a macrolide such as azithromycin is considered a second line agent (85, 87).
3.2.5 Coxiella burnetii: Q fever
Coxiella burnetii, the causative agent of Q fever, is a Gram-negative, obligate intracellular bacterium that spreads via inhalation of aerosolized body fluids or consumption of contaminated food material (90). In humans, the clinical disease begins with a sudden onset of flu-like symptoms with pneumonia and hepatitis (91). Q fever can progress to a chronic form which can involve endocarditis. In pregnant women, Q fever has been linked to both spontaneous abortion and stillbirth (92). Ophthalmic manifestations of Q fever are limited to case reports and include acute multifocal retinitis, optic neuritis, and bilateral exudative retinal detachment (93–95). Both acute (2–3 weeks) and chronic (18–24 months) Q fever should be treated with doxycycline and hydroxychloroquine (91). Due to the aerosolized nature of transmission, PPE should include respirators (96).
3.2.6 Enterotoxin B (Staphylococcus spp.)
Comparable to C. botulinum, Staphylococcus species can produce a Category B toxin known as Enterotoxin B. It was previously studied for use as an aerosol biological weapon, but the toxin can also spread via contaminated food (97, 98). The toxin is a superantigen that causes widespread stimulation of the immune system, inducing fever, hypotension, pulmonary edema, acute respiratory distress syndrome, or septic shock (97–99). Ophthalmic symptoms are typically not well characterized, although purulent conjunctivitis following exposure to the toxin has been reported (100). The rapid and widespread onset of symptoms from Enterotoxin B make treatment difficult, and there are currently no approved antitoxins for clinical use (97–99).
3.2.7 Epsilon toxin (Clostridium perfringens)
The capacity for aerosolization of the epsilon toxin produced by Clostridium perfringens makes it a possible biowarfare agent (101). Despite this, little is known about the clinical manifestations, with only two cases ever being reported, both from 1955 (102, 103). One of the patients presented with only profuse diarrhea while the other developed a peritoneal effusion with a gangrenous ileum (102, 103). No ophthalmic symptoms have been reported. Moreover, there is currently no known treatment for infection with epsilon toxin (101).
3.2.8 Food safety threats: non-typhoid Salmonella spp., Shigella dysenteriae, Escherichia coli O157:H7
Non-typhoid Salmonella species, Shigella dysenteriae, and Escherichia coli O157:H7 together are classified as food safety threats by the CDC (7). Each bacterium is spread via the fecal-oral route through the consumption of contaminated food or water (Salmonella can also be transmitted via contact with reptiles) (104–106). While these pathogens exhibit shared clinical manifestations, Salmonella has some pertinent differences. Salmonella infection has a nonspecific presentation that may include fever, diarrhea, or pneumonia with hepatosplenomegaly common with bacteremia (105, 107). Shigella and E. coli O157:H7 can cause a watery diarrhea that progresses to a bloody diarrhea, or, in severe cases, hemolytic uremic syndrome (HUS) (104, 106). Infection of Shigella has been linked to reactive arthritis, previously known as Reiter’s syndrome, which is a rare presentation of infection-induced arthritis that can cause conjunctivitis, among other non-ocular symptoms (108). Non-typhoidal Salmonella species have also been implicated in reactive arthritis, causing keratitis, uveitis and conjunctivitis (109, 110). While some strains of E.coli are known to impact the eye, the O157:H7 strain is not known to cause ocular disease. Unless immunocompromised, Salmonella infections are typically self-limited and should be treated supportively (111). The recommendation for E. coli treatment is similar, with antibiotic treatment demonstrating increased potential for developing HUS (104). Shigella, however, should be treated in both children and adults with ciprofloxacin or ceftriaxone (112, 113).
3.2.9 Rickettsia prowazekii: typhus fever
Rickettsia prowazekii is a Gram-negative bacilli and the causative agent of typhus fever. The disease is spread to humans via deposition of louse feces into bites or mucosal surfaces, or inhalation of aerosolized feces (114, 115). Clinical onset includes high fever, headache, and a rash due to hematogenous dissemination of R. prowazekii. Other symptoms can include nausea, vomiting, pneumonia, myocarditis, thrombocytopenia, jaundice, seizures, confusion, or even coma (114). Despite the numerous potential clinical manifestations of typhus fever, ophthalmic manifestations have not been described. Treatment of typhus fever is tetracyclines, with doxycycline being the preferred agent (115). Due to the louse-borne transmission of typhus fever, proper use of gowns, gloves, and caps should be used to prevent louse spread.
3.2.10 Water safety threats: Vibrio cholerae and Cryptosporidium parvum
Akin to the previous bacteria, Vibrio cholerae is designated by the CDC as a water safety threat, along with the protozoan Cryptosporidium parvum (7). Despite its eukaryotic classification, this review includes C. parvum here due to the CDC grouping with V. cholerae. While the classical transmission route of both organisms is the consumption of contaminated water, both can also be acquired via contaminated food, and C. parvum is capable of respiratory transmission (116–118). Infection from either organism leads to a watery diarrhea. V. cholerae causes pathognomonic “rice water” stool that leads to severe dehydration and electrolyte imbalances (116). In contrast, C. parvum infection typically presents with less severe diarrhea along with abdominal pain, nausea, flatulence, anorexia, and fatigue. If inhaled, one may also demonstrate a productive cough (117).
While neither are widely known for ophthalmic manifestations, some cases have been reported including a single case report of keratitis with corneal scraping cultures that grew V. cholerae after the patient was struck in the right eye by a marine shrimp (119). It should be noted that this infection was presumably caused by the direct contact of V. cholerae to the mucosal surface of the eye, and not the typical water-borne route. One study also reported that 9% of patients reported eye pain one-year post infection with C. parvum, but the protozoan is otherwise not linked to any other ophthalmic disease (120). The hallmark of cholera treatment is volume replacement and rehydration therapy with doxycycline indicated in severe cases (116). C. parvum infection should be treated with nitazoxanide (117).
4 Miscellaneous
4.1 Category B
4.1.1 Ricin toxin (Ricinus communis)
The ricin toxin is the only bioterrorism agent classified by the CDC that is neither viral, nor bacterial (or designated as a specific threat with a bacterium, as is the case with C. parvum) (Appendix 3). The toxin is produced by Ricinus communis, the castor bean, and when extracted, it can be disseminated by a number of modalities including aerosol, injection, or ingestion pathways (121). Physical symptoms vary by route of intoxication.
If ricin is aerosolized, the inhaled toxin can cause dyspnea, fever, cough, nausea, chest tightness, pulmonary edema, and skin erythema. If injected, myalgias and circulatory collapse are common. Ingested ricin typically causes abdominal pain, diarrhea, cramping, and dehydration (121). Ophthalmic implications of ricin toxin have yet to be described other than conjunctival injection. An antitoxin has been shown to be an effective countermeasure against ricin toxin in swine models (122).
5 COVID-19
Although not included in the CBRNE framework, response efforts to novel agents such as SARS-CoV-2 involve principles shared with other biological pathogens. The WHO COVID-19 Dashboard shows that estimates of COVID-19 has exceeded 770 million affected individuals and nearly 7 million deaths (123). Transmission is variable and includes SARS-CoV-2 particles landing on or otherwise coming into contact with the eyes, mouth, or nose, as well as inhalation of aerosol particles or droplets that contain the virus (124). Those infected may experience a range of symptoms, including those that are mild, moderate, and severe, but asymptomatic infection and transmission may also occur. Reported symptoms include malaise, fever or chills, cough, new loss of taste or smell, muscle or body aches, and difficulty breathing (125).
Ophthalmic manifestations associated with COVID-19 have been reported and include findings involving both the anterior and posterior segments of the eye. Reported findings include conjunctival hyperemia and injection, eye pain and redness, photophobia, cotton wool spots, retinal artery occlusion, retinal hemorrhage, and retinopathy (126–131). In addition to these ophthalmic findings, SARS-CoV-2 RNA has been previously detected in the tear film of 25% of patients in a hospitalized COVID-19 cohort (126). These ocular findings may provide insight into the physiologic changes of COVID-19, as well as the behavior of SARS-CoV-2 on the ocular surface.
6 Personal protective equipment
Due to the variable nature of the above biological agents, PPE decision-making relies on an understanding of the agent(s) of interest including the specific route(s) of transmission (e.g., respiratory, aerosolized droplets, contact), and the potential for spread during asymptomatic or presymptomatic infection. Agencies within the United States federal government, such as the National Institute for Occupational Safety and Health (NIOSH) and the Occupational Safety and Health Administration (OSHA), among others, have developed specific guidelines for CBRNE incident response that consider transmission routes (132–134).
Droplets of variola major virus, for example, can be spread through a respiratory route and guidance from the CDC indicates appropriate PPE as: eye protection, a NIOSH-certified N95 respirator, and disposable gown and gloves (135). Alternatively, contact transmission has been implicated in a number of VHFs so the CDC and WHO advocate for transmission-specific PPE provisions, such as a disposable facemask, full face shield, fluid-resistant gown, and two pairs of gloves (136, 137). Other key factors related to PPE include the specific tasks to be performed, duration of PPE wear, and the environmental conditions where patient care activities occur (e.g., forward, resource-austere settings vs. high resource settings).
7 Conclusion
Biologic agents can precipitate great ophthalmic injury and cause significant morbidity. With overlapping or limited ophthalmic findings, further investigations and close clinical monitoring of impacted patients are critical. As the global public health community continues to learn more about these agents, the CBRNE biological agent list and associated classification framework will require subsequent reevaluation, including potential revision of PPE guidance. Additionally, the appearance of emerging infectious diseases, especially zoonoses, necessitates continual pathogen surveillance, investigation, characterization, and assessment of merit for CBRNE status. Several zoonotic agents described here also require vector and/or source control measures for biohazard containment, and although an in-depth review of vector control is out of scope for this work, its importance should not be minimized. Future research to improve understanding of known pathogens will require reevaluation of the current CBRNE biological agent list and pathogen classification.
As globalization continues to expand, the risk for CBRNE incidents increases and subsequently prompts the need for progressive, vigilant surveillance and timely response to incidents to ensure a healthy global health community. Improving healthcare response and outcomes starts with accurate diagnosis, agent control, and treatment. This paper summarizes the clinical and ophthalmic manifestations, transmission routes and PPE considerations, as well as the current management guidelines for the biologic agents the CDC deems to be most dangerous to public health.
Author contributions
EC: Writing – original draft, Writing – review & editing. MDD: Writing – original draft, Writing – review & editing. CH: Writing – review & editing. YH: Writing – review & editing. CC: Writing – review & editing. MRD: Writing – review & editing. GJ: Writing – review & editing. JT: Supervision, Writing – review & editing. SY: Conceptualization, Supervision, Writing – review & editing.
Funding
The author(s) declare financial support was received for the research, authorship, and/or publication of this article. This project was supported by the National Eye Institute of the National Institutes of Health under award number R01 EY029594 (SY). Grant support was also provided by the Macula Society Retina Research Foundation, ARVO Mallinckrodt Young Investigator Grant, and the Stanley M. Truhlsen Family Foundation, Inc. CC was supported in part by a Knights Templar Eye Foundation career development award and competitive renewal, IDeA-CTR career development award, and National Eye Institute of the National Institutes of Health award number K08 EY034892.
Conflict of interest
The authors declare that the research was conducted in the absence of any commercial or financial relationships that could be construed as a potential conflict of interest.
Publisher’s note
All claims expressed in this article are solely those of the authors and do not necessarily represent those of their affiliated organizations, or those of the publisher, the editors and the reviewers. Any product that may be evaluated in this article, or claim that may be made by its manufacturer, is not guaranteed or endorsed by the publisher.
Author disclaimer
The content is solely the responsibility of the authors and does not necessarily represent official views of the National Institutes of Health or the views or policies of the Department of Health and Human Services, nor does mention of trade names, commercial products, or organizations imply endorsement by the U.S. Government.
Supplementary material
The Supplementary material for this article can be found online at: https://www.frontiersin.org/articles/10.3389/fmed.2023.1349571/full#supplementary-material
References
1. The lancet infectious D. Ebola Pheic is over but emergency continues. Lancet Infect Dis. (2016) 16:507. doi: 10.1016/s1473-3099(16)30013-5
2. Shadmi, E, Chen, Y, Dourado, I, Faran-Perach, I, Furler, J, Hangoma, P, et al. Health equity and COVID-19: global perspectives. Int J Equity Health. (2020) 19:104. doi: 10.1186/s12939-020-01218-z
3. Tambo, E, and Al-Nazawi, AM. Combating the global spread of poverty-related monkeypox outbreaks and beyond. Infect Dis Poverty. (2022) 11:80. doi: 10.1186/s40249-022-01004-9
4. U.S. Department of Homeland Security. (2022). Cbrne Program. Available at: https://www.dhs.gov/hazardous-response-program (Accessed October 8, 2023).
5. U.S. Department of Homeland Security. (2022). National Strategy for Chemical, Biological, Radiological, Nuclear, and Explosives (Cbrne) Standards. Available at: https://www.dhs.gov/national-strategy-chemical-biological-radiological-nuclear-and-explosives-cbrne-standards (Accessed October 8, 2023).
6. U.S. Department of Homeland Security. (2023). Office of Emerging Threats. Available at: https://www.fema.gov/about/offices/response-recovery/emerging-threats (Accessed October 8, 2023).
7. Centers for Disease Control and Prevention. (2018). Emergency preparedness and response-bioterrorism agents/diseases: U.S. Department of Health & Human Services. Available at: https://emergency.cdc.gov/agent/agentlist-category.asp#catdef (Accessed March 22, 2023).
8. Mushtaq, A, El-Azizi, M, and Khardori, N. Category C potential bioterrorism agents and emerging pathogens. Infect Dis Clin N Am. (2006) 20:423–41, x. doi: 10.1016/j.idc.2006.03.003
9. Semba, RD. The ocular complications of smallpox and smallpox immunization. Arch Ophthalmol. (2003) 121:715–9. doi: 10.1001/archopht.121.5.715
10. Karesh, JW, Mazzoli, RA, and Heintz, SK. Ocular manifestations of mosquito-transmitted diseases. Mil Med. (2018) 183:450–8. doi: 10.1093/milmed/usx183
11. Walker, DH, McCormick, JB, Johnson, KM, Webb, PA, Komba-Kono, G, Elliott, LH, et al. Pathologic and virologic study of fatal Lassa fever in man. Am J Pathol. (1982) 107:349–56.
12. Britt, JM, Clifton, BC, Barnebey, HS, and Mills, RP. Microaerosol formation in noncontact 'Air-Puff' tonometry. Arch Ophthalmol. (1991) 109:225–8. doi: 10.1001/archopht.1991.01080020071046
13. Aloke, C, Obasi, NA, Aja, PM, Emelike, CU, Egwu, CO, Jeje, O, et al. Combating Lassa fever in West African sub-region: Progress, challenges, and future perspectives. Viruses. (2023) 15:146. doi: 10.3390/v15010146
14. Centers for Disease Control and Prevention. (2021). Viral hemorrhagic fevers (Vhfs) – arenaviruses (Arenaviridae): U.S. Department of Health & Human Services. Available at: https://www.cdc.gov/vhf/virus-families/arenaviridae.html (Accessed July 31, 2023).
15. Li, AL, Grant, D, Gbakie, M, Kanneh, L, Mustafa, I, Bond, N, et al. Ophthalmic manifestations and vision impairment in Lassa fever survivors. PLoS One. (2020) 15:e0243766. doi: 10.1371/journal.pone.0243766
16. Frank, MG, Beitscher, A, Webb, CM, and Raabe, V. South American hemorrhagic fevers: a summary for clinicians. Int J Infect Dis. (2021) 105:505–15. doi: 10.1016/j.ijid.2021.02.046
17. Silva-Ramos, CR, Faccini-Martínez, ÁA, Calixto, OJ, and Hidalgo, M. Bolivian hemorrhagic fever: a narrative review. Travel Med Infect Dis. (2021) 40:102001. doi: 10.1016/j.tmaid.2021.102001
18. Centers for Disease Control and Prevention. (2021). Viral hemorrhagic fevers (Vhfs) – filoviruses (filoviridae): U.S. Department of Health & Human Services. Available at: https://www.cdc.gov/vhf/virus-families/filoviridae.html (Accessed April 14, 2023).
19. Abir, MH, Rahman, T, Das, A, Etu, SN, Nafiz, IH, Rakib, A, et al. Pathogenicity and virulence of Marburg virus. Virulence. (2022) 13:609–33. doi: 10.1080/21505594.2022.2054760
20. Baseler, L, Chertow, DS, Johnson, KM, Feldmann, H, and Morens, DM. The pathogenesis of Ebola virus disease. Annu Rev Pathol. (2017) 12:387–418. doi: 10.1146/annurev-pathol-052016-100506
21. Gear, JS, Cassel, GA, Gear, AJ, Trappler, B, Clausen, L, Meyers, AM, et al. Outbreake of Marburg virus disease in Johannesburg. Br Med J. (1975) 4:489–93. doi: 10.1136/bmj.4.5995.489
22. Kuming, BS, and Kokoris, N. Uveal involvement in Marburg virus disease. Br J Ophthalmol. (1977) 61:265–6. doi: 10.1136/bjo.61.4.265
23. Vingolo, EM, Messano, GA, Fragiotta, S, Spadea, L, and Petti, S. Ocular manifestations of Ebola virus disease: an Ophthalmologist's guide to prevent infection and panic. Biomed Res Int. (2015) 2015:487073. doi: 10.1155/2015/487073
24. Beeching, NJ, Fenech, M, and Houlihan, CF. Ebola virus disease. BMJ. (2014) 349:g7348. doi: 10.1136/bmj.g7348
25. Varkey, JB, Shantha, JG, Crozier, I, Kraft, CS, Lyon, GM, Mehta, AK, et al. Persistence of Ebola virus in ocular fluid during convalescence. N Engl J Med. (2015) 372:2423–7. doi: 10.1056/NEJMoa1500306
26. Shantha, JG, Crozier, I, Hayek, BR, Bruce, BB, Gargu, C, Brown, J, et al. Ophthalmic manifestations and causes of vision impairment in Ebola virus disease survivors in Monrovia, Liberia. Ophthalmology. (2017) 124:170–7. doi: 10.1016/j.ophtha.2016.10.011
27. Mattia, JG, Vandy, MJ, Chang, JC, Platt, DE, Dierberg, K, Bausch, DG, et al. Early clinical sequelae of Ebola virus disease in Sierra Leone: a cross-sectional study. Lancet Infect Dis. (2016) 16:331–8. doi: 10.1016/s1473-3099(15)00489-2
28. Meyer, H, Ehmann, R, and Smith, GL. Smallpox in the post-eradication era. Viruses. (2020) 12:2. doi: 10.3390/v12020138
29. Centers for Disease Control and Prevention. (2017). Smallpox: U.S. Department of Health & Human Services Available at: https://www.cdc.gov/smallpox/index.html (Accessed April 15, 2023).
31. Smith, CL. Disciform keratitis following smallpox. Am J Ophthalmol. (1922) 5:32–4. doi: 10.1016/S0002-9394(22)91025-6
32. Fleck, HK. Disciform keratitis secondary to smallpox. Am J Ophthalmol. (1921) 4:573–9. doi: 10.1016/S0002-9394(21)90487-2
33. Ronca, SE, Dineley, KT, and Paessler, S. Neurological sequelae resulting from encephalitic alphavirus infection. Front Microbiol. (2016) 7:959. doi: 10.3389/fmicb.2016.00959
34. Kehn-Hall, K, and Bradfute, SB. Understanding host responses to equine encephalitis virus infection: implications for therapeutic development. Expert Rev Anti-Infect Ther. (2022) 20:1551–66. doi: 10.1080/14787210.2022.2141224
35. Sharma, A, and Knollmann-Ritschel, B. Current understanding of the molecular basis of Venezuelan equine encephalitis virus pathogenesis and vaccine development. Viruses. (2019) 11:164. doi: 10.3390/v11020164
36. Wells, RM, Sosa Estani, S, Yadon, ZE, Enria, D, Padula, P, Pini, N, et al. An unusual hantavirus outbreak in southern Argentina: person-to-person transmission? Hantavirus pulmonary syndrome study Group for Patagonia. Emerg Infect Dis. (1997) 3:171–4. doi: 10.3201/eid0302.970210
37. Enría, D, Padula, P, Segura, EL, Pini, N, Edelstein, A, Posse, CR, et al. Hantavirus pulmonary syndrome in Argentina. Possibility of person to person transmission. Medicina (B Aires). (1996) 56:709–11.
38. Krüger, DH, Schönrich, G, and Klempa, B. Human pathogenic hantaviruses and prevention of infection. Hum Vaccin. (2011) 7:685–93. doi: 10.4161/hv.7.6.15197
39. Theiler, G, Langer-Wegscheider, B, Zollner-Schwetz, I, Valentin, T, Hönigl, M, Schnedl, W, et al. Blurred vision and myopic shift in Puumala virus infections are independent of disease severity. Clin Microbiol Infect. (2012) 18:E435–7. doi: 10.1111/j.1469-0691.2012.03997.x
40. Kontkanen, M, Puustjärvi, T, and Lähdevirta, J. Myopic shift and its mechanism in Nephropathia Epidemica or Puumala virus infection. Br J Ophthalmol. (1994) 78:903–6. doi: 10.1136/bjo.78.12.903
41. Cao, Y, Zhao, X, Yi, J, Tang, R, and Lei, S. Hantavirus retinitis and concurrent hemorrhagic fever with renal syndrome. Can J Ophthalmol. (2017) 52:e41–4. doi: 10.1016/j.jcjo.2016.09.017
42. Mehta, S, and Jiandani, P. Ocular features of hantavirus infection. Indian J Ophthalmol. (2007) 55:378–80. doi: 10.4103/0301-4738.33827
43. Singh, S, Numan, A, Sharma, D, Shukla, R, Alexander, A, Jain, GK, et al. Epidemiology, virology and clinical aspects of hantavirus infections: an overview. Int J Environ Health Res. (2022) 32:1815–26. doi: 10.1080/09603123.2021.1917527
44. Bruno, L, Nappo, MA, Ferrari, L, Di Lecce, R, Guarnieri, C, Cantoni, AM, et al. Nipah virus disease: epidemiological, clinical, diagnostic and legislative aspects of this unpredictable emerging zoonosis. Animals (Basel). (2022) 13:159. doi: 10.3390/ani13010159
45. Luby, SP, Hossain, MJ, Gurley, ES, Ahmed, BN, Banu, S, Khan, SU, et al. Recurrent zoonotic transmission of Nipah virus into humans, Bangladesh, 2001-2007. Emerg Infect Dis. (2009) 15:1229–35. doi: 10.3201/eid1508.081237
46. Chua, KB. Nipah Virus Outbreak in Malaysia. J Clin Virol. (2003) 26:265–75. doi: 10.1016/s1386-6532(02)00268-8
47. Singh, RK, Dhama, K, Chakraborty, S, Tiwari, R, Natesan, S, Khandia, R, et al. Nipah virus: epidemiology, pathology, immunobiology and advances in diagnosis, vaccine designing and control strategies – a comprehensive review. Vet Q. (2019) 39:26–55. doi: 10.1080/01652176.2019.1580827
48. Luby, SP. The pandemic potential of Nipah virus. Antivir Res. (2013) 100:38–43. doi: 10.1016/j.antiviral.2013.07.011
49. Islam, MS, Sazzad, HM, Satter, SM, Sultana, S, Hossain, MJ, Hasan, M, et al. Nipah virus transmission from bats to humans associated with drinking traditional liquor made from date palm sap, Bangladesh, 2011-2014. Emerg Infect Dis. (2016) 22:664–70. doi: 10.3201/eid2204.151747
50. Goh, KJ, Tan, CT, Chew, NK, Tan, PS, Kamarulzaman, A, Sarji, SA, et al. Clinical features of Nipah virus encephalitis among pig farmers in Malaysia. N Engl J Med. (2000) 342:1229–35. doi: 10.1056/nejm200004273421701
51. Lim, CC, Lee, WL, Leo, YS, Lee, KE, Chan, KP, Ling, AE, et al. Late clinical and magnetic resonance imaging follow up of Nipah virus infection. J Neurol Neurosurg Psychiatry. (2003) 74:131–3. doi: 10.1136/jnnp.74.1.131
52. Dixon, TC, Meselson, M, Guillemin, J, and Hanna, PC. Anthrax. N Engl J Med. (1999) 341:815–26. doi: 10.1056/nejm199909093411107
53. Bush, LM, Abrams, BH, Beall, A, and Johnson, CC. Index case of fatal inhalational anthrax due to bioterrorism in the United States. N Engl J Med. (2001) 345:1607–10. doi: 10.1056/NEJMoa012948
54. Meselson, M, Guillemin, J, Hugh-Jones, M, Langmuir, A, Popova, I, Shelokov, A, et al. The Sverdlovsk anthrax outbreak of 1979. Science. (1994) 266:1202–8. doi: 10.1126/science.7973702
55. Kamal, SM, Rashid, AK, Bakar, MA, and Ahad, MA. Anthrax: an update. Asian Pac J Trop Biomed. (2011) 1:496–501. doi: 10.1016/s2221-1691(11)60109-3
56. Amraoui, A, Tabbara, KF, and Zaghloul, K. Anthrax of the eyelids. Br J Ophthalmol. (1992) 76:753–4. doi: 10.1136/bjo.76.12.753
57. Hendricks, KA, Wright, ME, Shadomy, SV, Bradley, JS, Morrow, MG, Pavia, AT, et al. Centers for Disease Control and Prevention expert panel meetings on prevention and treatment of anthrax in adults. Emerg Infect Dis. (2014) 20:e130687. doi: 10.3201/eid2002.130687
58. Pillai, SK, Huang, E, Guarnizo, JT, Hoyle, JD, Katharios-Lanwermeyer, S, Turski, TK, et al. Antimicrobial treatment for systemic anthrax: analysis of cases from 1945 to 2014 identified through a systematic literature review. Health Secur. (2015) 13:355–64. doi: 10.1089/hs.2015.0033
59. Chateau, A, Van der Verren, SE, Remaut, H, and Fioravanti, A. The Bacillus Anthracis cell envelope: composition, physiological role, and clinical relevance. Microorganisms. (2020) 8:1864. doi: 10.3390/microorganisms8121864
60. Rao, AK, Sobel, J, Chatham-Stephens, K, and Luquez, C. Clinical guidelines for diagnosis and treatment of botulism, 2021. MMWR Recomm Rep. (2021) 70:1–30. doi: 10.15585/mmwr.rr7002a1
61. Caya, JG. Clostridium botulinum and the ophthalmologist: a review of botulism, including biological warfare ramifications of botulinum toxin. Surv Ophthalmol. (2001) 46:25–34. doi: 10.1016/s0039-6257(01)00227-2
62. Yu, PA, Lin, NH, Mahon, BE, Sobel, J, Yu, Y, Mody, RK, et al. Safety and improved clinical outcomes in patients treated with new equine-derived heptavalent botulinum antitoxin. Clin Infect Dis. (2017) 66:S57. doi: 10.1093/cid/cix816
63. Wawszczak, M, Banaszczak, B, and Rastawicki, W. Tularaemia – a diagnostic challenge. Ann Agric Environ Med. (2022) 29:12–21. doi: 10.26444/aaem/139242
64. World Health Organization. Who Guidelines on Tularaemia; Epidemic and Pandemic Alert and Response. Geneva: World Health Organization (2007).
65. Hirschmann, JV. From squirrels to biological weapons: the early history of tularemia. Am J Med Sci. (2018) 356:319–28. doi: 10.1016/j.amjms.2018.06.006
66. Croddy, E, and Krčálová, S. Tularemia, biological warfare, and the Battle for Stalingrad (1942–1943). Mil Med. (2001) 166:837–8. doi: 10.1093/milmed/166.10.837
67. Kantardjiev, T, Padeshki, P, and Ivanov, IN. Diagnostic approaches for Oculoglandular tularemia: advantages of Pcr. Br J Ophthalmol. (2007) 91:1206–8. doi: 10.1136/bjo.2007.117523
68. Rosario-Acevedo, R, Biryukov, SS, Bozue, JA, and Cote, CK. Plague prevention and therapy: perspectives on current and future strategies. Biomedicine. (2021) 9:1421. doi: 10.3390/biomedicines9101421
70. Dennis, DT. Plague as a biological weapon. Bioterror Infect Agents. (2009):37–70. doi: 10.1007/978-1-4419-1266-4_2
71. Kool, JL. Risk of person-to-person transmission of pneumonic plague. Clin Infect Dis. (2005) 40:1166–72. doi: 10.1086/428617
72. Edmunds, DR, Williams, ES, O'Toole, D, Mills, KW, Boerger-Fields, AM, Jaeger, PT, et al. Ocular plague (Yersinia Pestis) in mule deer (Odocoileus Hemionus) from Wyoming and Oregon. J Wildl Dis. (2008) 44:983–7. doi: 10.7589/0090-3558-44.4.983
73. Inglesby, TV, Dennis, DT, Henderson, DA, Bartlett, JG, Ascher, MS, Eitzen, E, et al. Plague as a biological weapon: medical and public health management. Working Group on Civilian Biodefense. JAMA. (2000) 283:2281–90. doi: 10.1001/jama.283.17.2281
74. de Figueiredo, P, Ficht, TA, Rice-Ficht, A, Rossetti, CA, and Adams, LG. Pathogenesis and immunobiology of brucellosis: review of brucella-host interactions. Am J Pathol. (2015) 185:1505–17. doi: 10.1016/j.ajpath.2015.03.003
75. Dean, AS, Crump, L, Greter, H, Hattendorf, J, Schelling, E, and Zinsstag, J. Clinical manifestations of human brucellosis: a systematic review and meta-analysis. PLoS Negl Trop Dis. (2012) 6:e1929. doi: 10.1371/journal.pntd.0001929
76. Rolando, I, Olarte, L, Vilchez, G, Lluncor, M, Otero, L, Paris, M, et al. Ocular manifestations associated with brucellosis: a 26-year experience in Peru. Clin Infect Dis. (2008) 46:1338–45. doi: 10.1086/529442
77. Solera, J, Martínez-Alfaro, E, and Espinosa, A. Recognition and optimum treatment of brucellosis. Drugs. (1997) 53:245–56. doi: 10.2165/00003495-199753020-00005
79. Johns Hopkins Center for Health Security. (2011). Burkholderia Mallei (Glanders) and Burkholderia (Melioidosis) Fact Sheet: Johns Hopkins Bloomberg School of Public Health. Available at: https://www.centerforhealthsecurity.org/our-work/publications/glanders-and-melioidosis-fact-sheet (Accessed March 26, 2023).
80. Van Zandt, KE, Greer, MT, and Gelhaus, HC. Glanders: an overview of infection in humans. Orphanet J Rare Dis. (2013) 8:131. doi: 10.1186/1750-1172-8-131
81. Estes, DM, Dow, SW, Schweizer, HP, and Torres, AG. Present and future therapeutic strategies for melioidosis and glanders. Expert Rev Anti-Infect Ther. (2010) 8:325–38. doi: 10.1586/eri.10.4
82. Currie, BJ, Dance, DA, and Cheng, AC. The global distribution of Burkholderia Pseudomallei and melioidosis: an update. Trans R Soc Trop Med Hyg. (2008) 102:S1–4. doi: 10.1016/s0035-9203(08)70002-6
83. Gassiep, I, Armstrong, M, and Norton, R. Human Melioidosis. Clin Microbiol Rev. (2020) 33:2. doi: 10.1128/cmr.00006-19
84. Yaisawang, S, Asawaphureekorn, S, Chetchotisakd, P, Wongratanacheewin, S, and Pakdee, P. Ocular involvement in melioidosis: a 23-year retrospective review. J Ophthalmic Inflamm Infect. (2018) 8:5. doi: 10.1186/s12348-018-0147-6
85. Basarab, M, Macrae, MB, and Curtis, CM. Atypical pneumonia. Curr Opin Pulm Med. (2014) 20:247–51. doi: 10.1097/mcp.0000000000000048
86. Knittler, MR, and Sachse, K. Chlamydia Psittaci: update on an underestimated zoonotic agent. Pathog Dis. (2015) 73:1–15. doi: 10.1093/femspd/ftu007
87. West, A. A brief review of Chlamydophila Psittaci in birds and humans. J Exotic Pet Med. (2011) 20:18–20. doi: 10.1053/j.jepm.2010.11.006
88. Dean, D, Shama, A, Schachter, J, and Dawson, CR. Molecular identification of an avian strain of Chlamydia Psittaci causing severe keratoconjunctivitis in a bird fancier. Clin Infect Dis. (1995) 20:1179–85. doi: 10.1093/clinids/20.5.1179
89. Raderer, M, Kiesewetter, B, and Ferreri, AJ. Clinicopathologic characteristics and treatment of marginal zone lymphoma of mucosa-associated lymphoid tissue (malt lymphoma). CA Cancer J Clin. (2016) 66:153–71. doi: 10.3322/caac.21330
90. Van Leuken, JPG, Swart, AN, Brandsma, J, Terink, W, Van de Kassteele, J, Droogers, P, et al. Human Q fever incidence is associated to spatiotemporal environmental conditions. One Health. (2016) 2:77–87. doi: 10.1016/j.onehlt.2016.03.004
91. Ullah, Q, Jamil, T, Saqib, M, Iqbal, M, and Neubauer, H. Q fever-a neglected zoonosis. Microorganisms. (2022) 10:8. doi: 10.3390/microorganisms10081530
92. Kampschreur, L, Dekker, S, Hagenaars, JCJP, Lestrade, P, Renders, NHM, de Jager-Leclercq, MGL, et al. Identification of risk factors for chronic Q fever, the Netherlands. Emerg Infect Dis J. (2012) 18:563–70. doi: 10.3201/eid1804.111478
93. Mahmoud, A, Abid, F, Khairallah, M, Affes, S, Mbarek, S, Amor, HH, et al. Acute multifocal retinitis in a patient with Q fever (Coxiella Burnetii infection) with endocarditis. J Ophthalmic Inflamm Infect. (2022) 12:19. doi: 10.1186/s12348-022-00295-1
94. Ong, C, Ahmad, O, Senanayake, S, Buirski, G, and Lueck, C. Optic neuritis associated with Q fever: case report and literature review. Int J Infect Dis. (2010) 14:e269–73. doi: 10.1016/j.ijid.2009.11.010
95. Udaondo, P, Garcia-Delpech, S, Salom, D, Garcia-Pous, M, and Diaz-Llopis, M. Q fever: a new ocular manifestation. Clin Ophthalmol. (2011) 5:1273–5. doi: 10.2147/opth.S18771
96. Ganter, M. Zoonotic risks from small ruminants. Vet Microbiol. (2015) 181:53–65. doi: 10.1016/j.vetmic.2015.07.015
97. Pinchuk, IV, Beswick, EJ, and Reyes, VE. Staphylococcal enterotoxins. Toxins (Basel). (2010) 2:2177–97. doi: 10.3390/toxins2082177
98. Fries, BC, and Varshney, AK. Bacterial toxins-staphylococcal enterotoxin B. Microbiol Spectr. (2013) 1:2. doi: 10.1128/microbiolspec.AID-0002-2012
99. Krakauer, T. Therapeutic Down-modulators of staphylococcal superantigen-induced inflammation and toxic shock. Toxins (Basel). (2010) 2:1963–83. doi: 10.3390/toxins2081963
100. Rusnak, JM, Kortepeter, M, Ulrich, R, Poli, M, and Boudreau, E. Laboratory exposures to staphylococcal enterotoxin B. Emerg Infect Dis. (2004) 10:1544–9. doi: 10.3201/eid1009.040250
101. Alves, GG, Machado de Ávila, RA, Chávez-Olórtegui, CD, and Lobato, FC. Clostridium Perfringens epsilon toxin: the third Most potent bacterial toxin known. Anaerobe. (2014) 30:102–7. doi: 10.1016/j.anaerobe.2014.08.016
102. Gleeson-White, MH, and Bullen, JJ. Clostridium Welchii epsilon toxin in the intestinal contents of man. Lancet. (1955) 268:384–5. doi: 10.1016/s0140-6736(55)91275-7
103. Kohn, J, and Warrack, GH. Recovery of clostridium Welchii type D from man. Lancet. (1955) 268:385. doi: 10.1016/s0140-6736(55)91276-9
104. Rahal, EA, Kazzi, N, Nassar, FJ, and Matar, GM. Escherichia Coli O157:H7-clinical aspects and novel treatment approaches. Front Cell Infect Microbiol. (2012) 2:138. doi: 10.3389/fcimb.2012.00138
105. Crump, JA, Sjölund-Karlsson, M, Gordon, MA, and Parry, CM. Epidemiology, clinical presentation, laboratory diagnosis, antimicrobial resistance, and antimicrobial Management of Invasive Salmonella Infections. Clin Microbiol Rev. (2015) 28:901–37. doi: 10.1128/cmr.00002-15
106. Pakbin, B, Brück, WM, and Brück, TB. Molecular mechanisms of shigella pathogenesis; recent advances. Int J Mol Sci. (2023) 24:2448. doi: 10.3390/ijms24032448
107. Gilchrist, JJ, and MacLennan, CA. Invasive nontyphoidal salmonella disease in Africa. EcoSal Plus. (2019) 8:2018. doi: 10.1128/ecosalplus.ESP-0007-2018
108. Hannu, T, Mattila, L, Siitonen, A, and Leirisalo-Repo, M. Reactive arthritis attributable to shigella infection: a clinical and epidemiological Nationwide study. Ann Rheum Dis. (2005) 64:594–8. doi: 10.1136/ard.2004.027524
109. Collins, NE, Fitzgerald, O, and Murphy, CC. Clinical image: keratitis in reactive arthritis. Arthritis Rheum. (2011) 63:2522. doi: 10.1002/art.30397
110. Saari, KM, Vilppula, A, Lassus, A, Leirisalo, M, and Saari, R. Ocular inflammation in Reiter's disease after salmonella enteritis. Am J Ophthalmol. (1980) 90:63–8. doi: 10.1016/s0002-9394(14)75077-9
111. Onwuezobe, IA, Oshun, PO, and Odigwe, CC. Antimicrobials for treating symptomatic non-typhoidal salmonella infection. Cochrane Database Syst Rev. (2012) 11:CD001167. doi: 10.1002/14651858.CD001167.pub2
112. Christopher, PRH, David, KV, John, SM, and Sankarapandian, V. Antibiotic therapy for shigella dysentery. Cochrane Database Syst Rev. (2009):4. doi: 10.1002/14651858.CD006784.pub2
113. Traa, BS, Walker, CL, Munos, M, and Black, RE. Antibiotics for the treatment of dysentery in children. Int J Epidemiol. (2010) 39 Suppl 1:i70–4. doi: 10.1093/ije/dyq024
114. Angelakis, E, Bechah, Y, and Raoult, D. The history of epidemic typhus. Microbiol Spectr. (2016) 4, 2–3. doi: 10.1128/microbiolspec.PoH-0010-2015
115. Blanton, LS. The rickettsioses: a practical update. Infect Dis Clin N Am. (2019) 33:213–29. doi: 10.1016/j.idc.2018.10.010
116. Chowdhury, F, Ross, AG, Islam, MT, McMillan, NAJ, and Qadri, F. Diagnosis, management, and future control of cholera. Clin Microbiol Rev. (2022) 35:e0021121. doi: 10.1128/cmr.00211-21
117. Helmy, YA, and Hafez, HM. Cryptosporidiosis: from prevention to treatment, a narrative review. Microorganisms. (2022) 10:2456. doi: 10.3390/microorganisms10122456
118. DuPont, HL, Chappell, CL, Sterling, CR, Okhuysen, PC, Rose, JB, and Jakubowski, W. The infectivity of Cryptosporidium Parvum in healthy volunteers. N Engl J Med. (1995) 332:855–9. doi: 10.1056/NEJM199503303321304
119. Chen, WD, Lai, LJ, Hsu, WH, and Huang, TY. Vibrio Cholerae non-O1 – the first reported case of keratitis in a healthy patient. BMC Infect Dis. (2019) 19:916. doi: 10.1186/s12879-019-4475-4
120. Stiff, RE, Davies, AP, Mason, BW, Hutchings, HA, and Chalmers, RM. Long-term health effects after resolution of acute Cryptosporidium Parvum infection: a 1-year follow-up of outbreak-associated cases. J Med Microbiol. (2017) 66:1607–11. doi: 10.1099/jmm.0.000609
121. Rasetti-Escargueil, C, and Avril, A. Medical countermeasures against ricin intoxication. Toxins (Basel). (2023) 15:2. doi: 10.3390/toxins15020100
122. Falach, R, Sapoznikov, A, Evgy, Y, Aftalion, M, Makovitzki, A, Agami, A, et al. Post-exposure anti-ricin treatment protects swine against lethal systemic and pulmonary exposures. Toxins (Basel). (2020) 12:354. doi: 10.3390/toxins12060354
123. World Health Organization. (2023). Who coronavirus (COVID-19) dashboard: World Health Organization. Available at: https://covid19.who.int/ (Accessed December 13, 2023).
124. Centers for Disease Control and Prevention. (2023). COVID-19 overview and infection prevention and control priorities in non-U.S. healthcare settings: Centers for Disease Control and Prevention. Available at: https://www.cdc.gov/coronavirus/2019-ncov/hcp/non-us-settings/overview/index.html#r1 (Accessed December 14, 2023).
125. Centers for Disease Control and Prevention. (2022). Symptoms of COVID-19: Centers for Disease Control and Prevention. Available at: https://www.cdc.gov/coronavirus/2019-ncov/symptoms-testing/symptoms.html (Accessed December 13, 2023).
126. Shantha, JG, Fashina, T, Stittleburg, V, Randleman, C, Ward, L, Regueiro, M, et al. COVID-19 and the eye: systemic and laboratory risk factors for retinopathy and detection of tear film Sars-Cov-2 Rna with a triplex rt-Pcr assay. PLoS One. (2022) 17:e0277301. doi: 10.1371/journal.pone.0277301
127. Shantha, JG, Auld, SC, Anthony, C, Ward, L, Adelman, MW, Maier, CL, et al. Retinopathy and systemic disease morbidity in severe COVID-19. Ocul Immunol Inflamm. (2021) 29:743–50. doi: 10.1080/09273948.2021.1952278
128. Guan, WJ, Ni, ZY, Hu, Y, Liang, WH, Ou, CQ, He, JX, et al. Clinical characteristics of coronavirus disease 2019 in China. N Engl J Med. (2020) 382:1708–20. doi: 10.1056/NEJMoa2002032
129. Gangaputra, SS, and Patel, SN. Ocular symptoms among nonhospitalized patients who underwent COVID-19 testing. Ophthalmology. (2020) 127:1425–7. doi: 10.1016/j.ophtha.2020.06.037
130. Ma, N, Li, P, Wang, X, Yu, Y, Tan, X, Chen, P, et al. Ocular manifestations and clinical characteristics of children with laboratory-confirmed COVID-19 in Wuhan, China. JAMA Ophthalmol. (2020) 138:1079–86. doi: 10.1001/jamaophthalmol.2020.3690
131. Invernizzi, A, Torre, A, Parrulli, S, Zicarelli, F, Schiuma, M, Colombo, V, et al. Retinal findings in patients with COVID-19: results from the Serpico-19 study. EClinicalMedicine. (2020) 27:100550. doi: 10.1016/j.eclinm.2020.100550
132. Brown, CK, Matthews, DL, Thomas, RJ, and Edens, AL. Developing a personal protective equipment selection matrix for preventing occupational exposure to Ebola virus. Health Secur. (2019) 17:213–28. doi: 10.1089/hs.2019.0014
133. National Institute for Occupational Safety and Health. Guidance on emergency responder personal protective equipment (Ppe) for response to Cbrn terrorism incidents. Ctr Dis Control Prev. (2008) 1–13.
134. Occupational Safety and Health Administration. Chemical-biological-radiological-nuclear (CBRN) personal protective equipment selection matrix for emergency responders: U.S. Department of Labor. Occupational Safety & Health Administration, U.S. Department of Labor, Washington, DC, USA (2005).
135. Centers for Disease Control and Prevention. Smallpox: Prevent Spread of Disease: U.S. Department of Health and Human Services. Centers for Disease Control and Prevention, Atlanta, GA, USA (2017).
136. World Health Organization. Infection control for viral Haemorrhagic fevers in the African health care setting. Geneva: World Health Organization (1998).
137. Centers for Disease Control and Prevention. (2023). Guidance on personal protective equipment (Ppe) in U.S. healthcare settings for evaluating patients suspected to have selected viral hemorrhagic fevers who are clinically stable and do not have bleeding, vomiting, or diarrhea: U.S. Department of Health and Human Service. Available at: https://www.cdc.gov/vhf/ebola/healthcare-us/ppe/guidance-clinically-stable-puis.html (Accessed November 14, 2023).
Keywords: ophthalmic, biological agents, CBRNE, management, public health
Citation: Curran EH, Devine MD, Hartley CD, Huang Y, Conrady CD, Debiec MR, Justin GA, Thomas J and Yeh S (2024) Ophthalmic implications of biological threat agents according to the chemical, biological, radiological, nuclear, and explosives framework. Front. Med. 10:1349571. doi: 10.3389/fmed.2023.1349571
Edited by:
Kai Jin, Zhejiang University, ChinaReviewed by:
Zhi-Qin Huang, Capital Medical University, ChinaCopyright © 2024 Curran, Devine, Hartley, Huang, Conrady, Debiec, Justin, Thomas and Yeh. This is an open-access article distributed under the terms of the Creative Commons Attribution License (CC BY). The use, distribution or reproduction in other forums is permitted, provided the original author(s) and the copyright owner(s) are credited and that the original publication in this journal is cited, in accordance with accepted academic practice. No use, distribution or reproduction is permitted which does not comply with these terms.
*Correspondence: Joanne Thomas, joanne.jess.thomas@emory.edu; Steven Yeh, syeh@unmc.edu
†These authors have contributed equally to this work and share first authorship