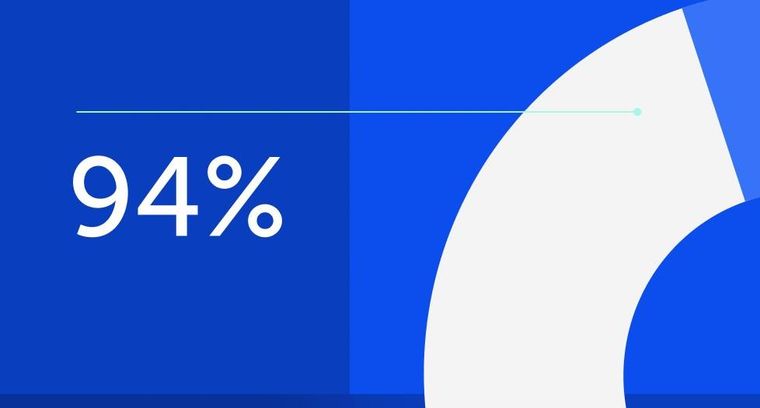
94% of researchers rate our articles as excellent or good
Learn more about the work of our research integrity team to safeguard the quality of each article we publish.
Find out more
ORIGINAL RESEARCH article
Front. Med., 09 February 2024
Sec. Precision Medicine
Volume 10 - 2023 | https://doi.org/10.3389/fmed.2023.1340703
This article is part of the Research TopicMolecular Informatics in Precision MedicineView all 10 articles
Introduction: Psoriasis and vitiligo are inflammatory autoimmune skin disorders with remarkable genetic involvement. Mannose-binding lectin (MBL) represents a significant immune molecule with one of its gene variants strongly linked to autoimmune diseases. Therefore, in this study, we investigated the role of the MBL variant, rs1800450, in psoriasis and vitiligo disease susceptibility.
Methods: The study comprised performing in silico analysis, performing an observational study regarding psoriasis patients, and performing an observational study regarding vitiligo patients. Various in silico tools were used to investigate the impact of the selected mutation on the function, stability, post-translational modifications (PTMs), and secondary structures of the protein. In addition, a total of 489 subjects were enrolled in this study, including their demographic and clinicopathological data. Genotyping analysis was performed using real-time PCR for the single nucleotide polymorphism (SNP) rs1800450 on codon 54 of the MBL gene, utilizing TaqMan genotyping technology. In addition, implications of the studied variant on disease susceptibility and various clinicopathological data were analyzed.
Results: Computational analysis demonstrated the anticipated effects of the mutation on MBL protein. Furthermore, regarding the observational studies, rs1800450 SNP on codon 54 displayed comparable results in our population relative to global frequencies reported via the 1,000 Genomes Project. This SNP showed no significant association with either psoriasis or vitiligo disease risk in all genetic association models. Furthermore, rs1800450 SNP did not significantly correlate with any of the demographic or clinicopathological features of both psoriasis and vitiligo.
Discussion: Our findings highlighted that the rs1800450 SNP on the MBL2 gene has no role in the disease susceptibility to autoimmune skin diseases, such as psoriasis and vitiligo, among Egyptian patients. In addition, our analysis advocated the notion of the redundancy of MBL and revealed the lack of significant impact on both psoriasis and vitiligo disorders.
Psoriasis and vitiligo are inflammatory autoimmune diseases with genetic factors playing a remarkable role in both diseases (1). Psoriasis is an inflammatory skin disease that is characterized by the development of scaly and erythematous plaques with a global prevalence of approximately 2% (2, 3). Its pathogenesis comprises intricate interactions between the adaptive immune system and the innate immune system (4). Meanwhile, vitiligo represents an autoimmune disease of the skin characterized by autoimmune melanocyte destruction, resulting in the related depigmentation patches affecting the skin in addition to mucosa (5). The prevalence of vitiligo is estimated at approximately 0.2–2% in varied populations (6). Genetic factors have a remarkable role in vitiligo and the risk related to these factors is estimated to reach 75–83%, leaving only approximately 20% for the other environmental factors (7).
One of the important components of innate immunity is mannose-binding lectin (MBL), and the defects of this molecule have been linked with different autoimmune diseases (8). This protein is one of the pattern-recognition molecules that are responsible for activating the complement system through the lectin pathway (9, 10). It is encoded by the MBL2 gene located on chromosome 10, and the presence of certain missense single nucleotide polymorphisms (SNPs) in this gene was associated with notable low levels of MBL (11). One of these specific mutations is rs1800450 in codon 54, which leads to the change of glycine to aspartic acid (12), with the variant allele represented by allele B and the wild-type one represented by allele A (13). Moreover, this missense mutation could impact the binding activity of MBL, leading to implications on its function (14). The presence of this SNP was linked with several autoimmune diseases such as rheumatoid arthritis (15) and systemic lupus erythematosus (16). However, the relationship between this SNP and autoimmune skin diseases, specifically vitiligo and psoriasis, was investigated by only a few studies showing conflicting results (17–20).
More studies are required to investigate the impact of this missense mutation on psoriasis and vitiligo diseases, especially in light of the increasing interest in the genetic association studies with different human diseases (21–24). By deciphering the nature of the relationship between this mutation and such chronic autoimmune diseases and their features, hopes could be raised to improve the guidelines for the prediction and management of these autoimmune conditions.
The study comprised an in silico analysis, an observational study regarding psoriasis patients, and an observational study regarding vitiligo patients. A chart demonstrating the outline of the study research is displayed in Figure 1.
For estimating how the SNP might affect protein function, MutPred2 was used.1 MutPred2 represents a software package and machine learning technique that combines genetic and molecular data for reasoning probabilistically regarding the pathogenicity of substitutions of amino acids by providing (1) a general prediction regarding pathogenicity and (2) a list describing certain molecular changes that may affect the phenotype (25).
The stability of the protein was examined with regard to the chosen mutation using the Mu-Pro tool. The Mu-Pro tool utilizes a strong support vector machine technique that, when used for cross-validation and verification, demonstrates an accuracy of 84%2 (26).
The secondary structure of the studied protein was analyzed using the SOPMA server, and the precise alignment regarding the modified amino acids in the secondary structure was figured out.3 In addition, the secondary structures with the selected SNP were examined as well. The SOPMA server could forecast the secondary structures of a given protein by examining the numerous alignments regarding the protein’s sequence (27).
The MusiteDeep server4 was employed to forecast the positions of various types of post-translational modifications. Due to the significant role of PTMs in controlling how proteins function, the recognition of PTMs is crucial for understanding the pathogenesis of diseases (28–30).
The GeneMANIA tool was employed to produce the MBL2 gene–gene interaction network.5 Using a range of resources and data types, GeneMANIA could predict the genes that strongly interact with a given gene (31).
The STRING database6 was adopted to predict the MBL protein–protein interaction network. The STRING database represents a database into which protein–protein interactions, encompassing both functional and physical relationships, are methodically gathered and integrated (32). The prediction was restricted to the 10 proteins representing the most interactive ones.
This is a case–control study in which the study participants were recruited from the outpatient dermatology department clinic, Suez Canal University Hospital (SCUH), Ismailia, Egypt, with the study participants classified into three main groups: Group 1 (vitiligo patient group), Group 2 (psoriasis patient group), and Group 3 (control group). All subjects or their next of kin provided informed consent.
After being diagnosed with vitiligo through clinical examination, supplemented with Wood’s lamp, 90 patients from both sexes were included. All the necessary clinicopathological data, such as sex, age, BMI, family history, previous history regarding other autoimmune diseases (e.g., Hashimoto’s thyroiditis, diabetes mellitus, psoriasis, and Addison’s disease), age of the disease onset, severity, disease duration, and treatment, were collected depending on patients’ history. Furthermore, a thorough dermatological examination was applied to all patients to assess the size, site, pattern, and distribution of individual lesions. In addition, disease severity assessment was performed depending on the criteria of the Vitiligo Area Severity Index (VASI) in addition to the Vitiligo European Task Force (VETF).
After being diagnosed with chronic plaque psoriasis, 99 patients of both sexes were included in the study, with the exclusion of those suffering from other autoimmune diseases. All needed clinicopathological data, such as sex, age, BMI, family history, severity, age of the disease onset, lines of treatment, and disease duration, were collected depending on patients’ history. Moreover, a thorough dermatological examination was carried out on all patients to assess the size, site, pattern, and distribution of individual lesions. In addition, disease severity assessment was performed depending on the Psoriasis Area Severity Index (PASI) score. According to the European consensus, PASI is interpreted as mild in cases where the PASI score is less than 10, moderate in cases where the score is between 10 and 20, and severe in cases where the score is greater than 20.
A total of 300 healthy volunteers were recruited from the SCUH blood bank. The members of this third group were matched with the members of the other two groups in terms of sex and age.
The molecular analysis was conducted at the Center of Excellence of Molecular and Cellular Medicine at the Faculty of Medicine, Suez Canal University and Hospital. The extraction of DNA was conducted from venous blood depending on the QIAamp DNA Blood Mini Kit (Cat. No. 51104, QIAGEN; Hilden, Germany). The concentration and purity of DNA were checked depending on the NanoDrop 2000 1C spectrophotometer (NanoDrop Tech., Inc. Wilmington, DE, USA). The DNA samples were stored at −20°C until further analysis. MBL2 SNP rs1800450 was identified by relying on real-time PCR (RT-PCR) utilizing TaqMan genotyping assays (assay ID: C___2336609_20). The reaction components were obtained from Applied Biosystems (Foster City, CA, USA). A 25-mL reaction volume was used for the PCR, containing 1.25 mL of TaqMan SNP genotyping assay mix, 12.5 mL of TaqMan genotyping master mix, no AmpErase UNG (2×), and 20 ng of genomic DNA, which was diluted to 11.25 mL, utilizing DNase-RNase-free water. After that, the amplification was done using the StepOne™ real-time PCR equipment (Applied Biosystems, Foster City, CA, USA). SDS software version 1.3.1 (Applied Biosystems) was utilized for allelic discrimination. The procedures were conducted blindly with respect to the vitiligo/psoriasis/control groups.
For the statistical analysis, we depended on Statistical Package for the Social Sciences (SPSS) software, version 24 in addition to Microsoft® Excel 2010. Percentage and frequency were adopted to express qualitative variables, with the usage of the chi-squared (χ2) test besides Fisher’s exact tests to compare between groups. Mean ± standard deviation (SD) was adopted to express quantitative variables, with the use of Student’s t-test and one-way ANOVA tests to compare quantitative variables with a normal distribution, while the Mann–Whitney U test and Kruskal–Wallis test were used to compare quantitative variables with a non-normal distribution. Statistical significance was determined by a value of p below 0.05. Moreover, the calculation of odds ratios (OR) was performed using a 95% confidence interval (CI). Hardy–Weinberg equilibrium (HWE) was calculated as well. In addition, SNPSTAT was used for genetic models.
The utilization of the MutPred2 tool revealed a score of 0.849 with rs1800450 SNP, which suggested pathogenicity. Furthermore, molecular alterations were predicted, including losing acetylation at K59 with a probability of 0.27 and a value of p of 7.3e-03, gaining SUMOylation at K56 with a probability of 0.25 and a value of p of 7.8e-03, losing methylation at K56 with a probability of 0.23 and a value of p of 3.1e-03, gaining ubiquitylation at K49 with a probability of 0.17 and a value of p of 0.02, and gaining proteolytic cleavage at D53 with a probability of 0.13 and a value of p of 0.02.
The analysis of how the nominated SNP could affect MBL stability was conducted using the Mu-Pro tool. The SNP was predicted by the Mu-Pro tool to decrease the stability with ΔΔG of −0.69653508.
The SOPMA tool was used to analyze the anticipated secondary structures of MBL, as demonstrated in Figure 2A. SOPMA revealed that 75 residues were linked with an alpha helix (30.24%), 39 with an extended strand (15.37%), 122 with a random coil (49.19%), and 12 with a beta turn (4.84%). Position 54 was found to be associated with the random coil. Furthermore, the secondary structures with the presence of the selected mutation were predicted, as shown in Figure 2B. In this case, SOPMA exposed that 69 residues were linked with an alpha helix (27.82%), 38 with an extended strand (15.32%), 133 with a random coil (53.63%), and 8 with a beta turn (3.23%). Position 54 was found to be associated with the random coil in this case as well.
Figure 2. Secondary structure analysis: (A) Secondary structure with wild MBL protein and (B) secondary structure with MBL protein in case of the selected mutation, produced by the SOPMA server. Alpha helix: (h), Extended strand: (e), Beta turn: (t), Random coil: (c).
To identify the expected post-translational modification sites, the MusiteDeep server was utilized; the anticipated post-translational modification sites with MBL protein are shown in Figure 3A. Moreover, the anticipated post-translational modification sites with MBL in the case of the nominated mutation are shown in Figure 3B, and loss of SUMOylation at position 56 could be noticed in this case.
Figure 3. Post-translational modification analysis (A) post-translational modification sites with wild MBL protein and (B) post-translational modification sites with MBL protein in case of the selected mutation, produced by the MusiteDeep server. P, Phosphorylation; me, Methylation; gl, Glycosylation; Su, SUMOylation; Ub, Ubiquitination; ac, Acetyllysine; pa, Palmitoylation; Pc, Pyrrolidone carboxylic acid; Hy, Hydroxylation.
Utilizing the GeneMANIA tool, the MBL2 gene’s gene–gene interaction was examined, and the results showed 20 genes with the strongest connections to the MBL2 gene (Figure 4). Among these genes, the mannan-binding lectin serine peptidase 1 gene (MASP1) ranked first. Then, the mannan-binding lectin serine peptidase 2 gene (MASP2) held the second rank. After that, complement C2 (C2) held the third rank.
Depending on the STRING database, the MBL protein–protein interaction network was generated, and the 10 most interactive proteins were predicted, as could be shown in detail in Figure 5.
Figure 5. Protein–protein interaction network related to MBL protein, generated by the STRING databases (version 12). The nodes signify proteins, whereas the edges indicate protein–protein associations. The edges could possess any of the seven distinct colored lines, with distinct indications. Black lines: co-expression evidence; light blue lines: database evidence; yellow lines: text-mining evidence; purple lines: experimental evidence; blue lines: co-occurrence evidence; green lines: neighborhood evidence; red lines: fusion evidence. MASP2: Mannan-binding lectin serine protease 2 A chain, FCN2: Ficolin-2, MASP1: Mannan-binding lectin serine peptidase 1, PRSS2: Trypsin-2, FCN3: Ficolin-3, CALR: Calreticulin, C4A: Complement C4-A alpha chain, SERPING1: Plasma protease C1 inhibitor, PTX3: Pentraxin-related protein PTX, C4B: Complement C4-B alpha chain (https://string-db.org/ accessed on 23 December 2023).
Our study comprised a total of 489 subjects, including 90 vitiligo patients, 99 psoriasis patients, and a comparable number of healthy control subjects, i.e., 300. The baseline features of enrolled subjects are shown in Table 1. The age of the control, vitiligo, and psoriasis groups ranged from 18 to 63 years, from 6 to 60 years, and from 18 to 60 years, respectively. Meanwhile, the percentage of men in these three groups was 50.3, 61.1, and 45.5%. Considering special habits among the study groups, 53.8% of the control group participants were smokers, 64.4% of vitiligo patients were smokers, and 56.6% of psoriasis patients were smokers. Regarding family history, 18.9% of vitiligo patients had a positive family history, while 15.2% of psoriasis patients had a positive history. No significant statistical difference was found with these baseline characteristics except with the age variable.
Different clinicopathological features between patient groups are shown in Table 2. The mean of disease duration for vitiligo patients and psoriasis patients was 9.2 ± 9.61 and 7.0 ± 6.25, respectively, with no statistical significance. Furthermore, the mean age regarding disease onset for these two groups was 25.93 ± 15.98 and 35.07 ± 13.34, respectively, with statistically significant differences found between these groups. Studying Vitiligo Disease Activity (VIDA) revealed the presence of 37.5, 3.4, 13.6, 20.5, 22.7, and 2.3% of vitiligo patients in stages −1, 0, 1, 2, 3, and 4, respectively, showing statistical significance. Meanwhile, studying psoriasis severity revealed the presence of 45% of psoriasis patients in the mild subgroup, 31% in the moderate subgroup, and 24% in the severe subgroup, with statistical significance found as well. The mean of the Vitiligo Area Severity Index (VASI) was 0.51 ± 0.30 in the vitiligo group, while the mean of the Psoriasis Area Severity Index (PASI) was 13.66 ± 9.05 in the psoriasis group, with statistical significance indicated by a value of p less than 0.001.
Table 3 shows the genotype and allele frequencies of MBL2 gene rs1800450 SNP among the studied skin autoimmune diseases. As shown, the allele frequencies in the control group were 514 (85.67%) and 86 (14.33%) for allele A and allele B, respectively. Regarding the psoriasis group, allele frequencies were 174 (87.88%) and 24 (12.12%), respectively, while with the vitiligo group, allele frequencies were 164 (91.11%) and 16 (8.89%), respectively (Figure 6A).
Figure 6. Genotype and allele frequencies of MBL2 gene rs1800450 SNP among skin autoimmune diseases. (A) Allele frequencies and (B) genotype frequencies (controls = 300 subjects, psoriasis = 99 patients, vitiligo = 90 patients).
Meanwhile, the genotype frequencies for wild genotype, heterozygous genotype, and mutant genotype with the control group were 222 (74%), 70 (23.3%), and 8 (2.7%), respectively. Regarding the psoriasis group, the genotype frequencies were 76 (76.8%), 22 (22.2%), and 1 (1%), respectively, while with the vitiligo group, the genotype frequencies were 75 (83.3%), 14 (15.6%), and 1 (1.1%), respectively (Figure 6B). The genotype distribution in study groups showed consistency with Hardy–Weinberg equilibrium. In addition, allele frequencies for allele B in different populations for the rs1800450 SNP are shown in Figure 7, according to the 1,000 Genomes project, along with the allele frequencies in the study groups.
Figure 7. Allele frequencies for MBL2 gene rs1800450 SNP according to the 1,000 Genomes Project. This diagram was constructed based on Ensembl, https://www.ensembl.org/index.html (accessed on 17 July 2023).
The analysis of MBL2 gene rs1800450 SNP with psoriasis risk was performed under all genetic association models, as shown in Table 4; nevertheless, no statistical significance was uncovered. In addition, the analysis of the rs1800450 variant with vitiligo risk was conducted under all genetic association models as well; however, no significant statistical difference was found, as shown in Table 5.
Table 4. Genetic association models for the MBL2 gene rs1800450 SNP with psoriasis risk (total n = 399, controls = 300, psoriasis patients = 99, adjusted by age and sex).
Table 5. Genetic association models for the MBL2 gene rs1800450 SNP with vitiligo risk (total n = 390, controls = 300, vitiligo patients = 90, adjusted by age and sex).
The inter-relationships between rs1800450 SNP genotyping, psoriasis cases’ demographic data, and their clinicopathological features were analyzed and shown in Table 6. A direct and significant correlation was found between age and duration (r = 0.366; p < 0.001), disease onset (r = 0.890; p < 0.001), and disease (r = 0.310; p < 0.001). Sex had a direct and significant correlation with family history (r = 0.890; p < 0.05). Moreover, duration had a direct and significant correlation with age (r = 0.366; p < 0.001), PASI (r = 0.381; p < 0.001), and severity (r = 0.297; p = 0.003). Furthermore, PASI showed a direct and significant correlation with duration (r = 0.381; p < 0.001) as well as with severity and vice versa (r = 0.930; p < 0.001).
Table 6. Correlation matrix between psoriasis patients’ demographic data, clinicopathological features, and the MBL2 gene rs1800450 SNP genotyping.
The analysis of interrelationships between rs1800450 SNP and vitiligo cases’ demographic data and their clinicopathological features was performed, as shown in Table 7. Age showed a direct and significant correlation with VASI (r = 0.271; p = 0.01), duration (r = 0.438; p < 0.001), and disease onset (r = 0.812; p < 0.001). VASI showed a direct and significant correlation with age (r = 0.271; p = 0.01) as well as with duration (r = 0.447; p < 0.001) and vice versa.
Table 7. Correlation matrix between vitiligo patients’ demographic data, clinicopathological features, and the MBL2 gene rs1800450 SNP genotyping.
Genetics were found to have primary roles in both psoriasis and vitiligo disorders (7, 33–36). SNPs signify the most prevalent type of genetic variation (37), with massive attention attracted to the missense SNP subtype that may result in pathogenic consequences (38). Despite the importance of MBL in the immune system and the discovered links between MBL2 polymorphisms and autoimmune diseases (39), only a few studies to our knowledge have addressed the association between these variants with vitiligo and psoriasis disorders.
By applying the in silico methods, the SNP was predicted to affect protein function and protein stability. Furthermore, a protein’s secondary structure plays crucial functions in both the structure and folding of the protein, demonstrating the relevance of this structure (40); therefore, secondary structure analysis was performed using the SOPMA server. The secondary structure analysis detected no change regarding the alignment of the selected position. Moreover, PTMs could influence protein functions and have the potential to impact many aspects of protein biology, such as stability, cellular localization, and interaction with co-factors (41). Therefore, PTM analysis was performed, which revealed a loss of SUMOylation in position 56 with this mutation. In addition, gene–gene interaction examination revealed the 20 genes with the strongest connections to the MBL2 gene, which might be affected by MBL2 variants. The significance of researching gene–gene interactions while examining disease–gene associations was demonstrated by the verified presence of interaction between various genetic loci (42). Furthermore, mutations that cause diseases show a tendency to cause instability in protein–protein interactions (43). Consequently, the interaction pattern of MBL in conjunction with other proteins was predicted using the STRING database showing the 10 most prominent interactors.
Our experimental analysis revealed genotype frequencies of 76.3, 21.7, and 2% for AA, AB, and BB, respectively, which were similar to previously conducted studies on Egyptian populations. A 2018 study conducted by Hammad et al. showed genotype frequencies of 79.7, 18.6, and 1.7% with AA, AB, and BB, respectively (44). Moreover, genotype frequencies in another Egyptian study by Gomaa et al. exposed genotype frequencies of 64.6, 28.8, and 6.7%, respectively (12). Although the heterozygous allele displayed a high frequency in these studies along with our study, other previous studies showed even more elevated frequencies with the AB allele (9, 45, 46). With only limited exceptions, these remarkably elevated frequencies of this variant allele and other MBL deficiency variant alleles were detected worldwide. It is noteworthy that the allele B has nearly substituted the allele A in specific Indian populations in South America (47). This distinct pattern has raised inquiries about MBL significance and whether these mutations are associated with some kind of biological benefits (48). Different models were introduced in pursuit of the clarification of this phenomenon, resulting in an unsettled debate (49, 50). One explanation assumed a gained protection with these variants due to the associating low MBL levels that could be advantageous in case of tissue damage resulting from a strong inflammatory response (51). This postulation gave explanation to some previous findings that favored the clinical outcome with genotypes of low MBL in case of some disorders; these genotypes were associated with less severe autoimmune manifestations in primary Sjögren’s syndrome (10); moreover, tuberculosis protection was correlated with heterozygosity in MBL alleles, which is responsible for low levels of MBL (52). Furthermore, similar protective benefit was found for these genotypes against visceral leishmaniasis (53). In addition, the elevated MBL levels were identified to confer an augmented risk of ischemic heart disease along with myocardial infarction in rheumatoid arthritis (54, 55). On the other hand, another explanation excluded the existence of selective pressure and displayed the absence of statistical support for this pressure (56, 57), further advocating the redundancy of MBL in human immunity as a result (57).
Subsequently, the association between codon 54 polymorphism and psoriasis risk was investigated. Nevertheless, no significant association was revealed with all genetic association models. Moreover, no significant correlation was discovered between rs1800450 SNP and the clinicopathological features of psoriasis. The significance of MBL and MBL deficiency in disease pathogenesis has been a source of ambiguity and queries as studies indicate that low or elevated MBL levels show a damaging, protecting, or no effect on susceptibility to diseases (51), which could also be the case here, as recent studies have revealed the important role of MBL in psoriasis pathogenesis. One study found that MBL may aggravate psoriatic skin inflammation by assisting the infiltration of the neutrophils in psoriatic lesions (58). Another study revealed that psoriasis patients displayed higher MBL levels than control subjects and that the level of MBL correlated positively with PASI score and psoriasis severity, and found that MBL assisted the differentiating and infiltrating processes of plasmacytoid dendritic cells in initial skin lesions of psoriasis, leading to the aggravation of disease severity (59). Therefore, the reason for the lack of protective effect with MBL-deficient genotypes needs to be explained. One suggested explanation for the neutrality of MBL deficiency in previous studies was by assuming the redundancy of MBL and the existence of adequate substituting immune mechanisms (9, 60), as other pathways or molecules have demonstrated that they may make up for the deficiency of MBL (57, 61, 62).
The scarcity of previous related studies addressing MBL2 variants and psoriasis was noticed. The previously conducted study in the Turkish population included 50 psoriasis vulgaris patients along with 53 control subjects and found a significant association between rs1800450 SNP and psoriasis (20), which differs from our findings and with the potential protective role of MBL as well. However, previous analyses related to the impacts of MBL2 variants have pointed out the role of underpowered studies in inconsistent results (63).
In addition, the analysis was extended to examine the relationship between codon 54 polymorphism and vitiligo risk as well. However, the analysis also did not reveal a significant association with all genetic association models. Furthermore, no significant correlation was uncovered between this SNP and clinicopathological features of vitiligo. To our knowledge, only a limited number of previous studies dealt with this topic. Our result complies with the findings of both Karkucak et al. and Dwivedi et al., who investigated this issue; Karkucak et al. conducted their study on a Turkish population comprising 101 vitiligo cases and 101 control subjects, reporting the absence of the association of vitiligo risk with rs1800450 SNP (18). Moreover, Dwivedi et al. conducted their study on an Indian population comprising 92 cases affected by generalized vitiligo and 94 control subjects, revealing the absence of the association of this disease with rs1800450 SNP and other MBL2 SNPs (17). However, one more Turkish study, including 40 vitiligo patients and 50 healthy subjects, was conducted that suggested a role for rs1800450 in vitiligo susceptibility (19). Complying with the findings of the two studies with a larger sample size gave robustness to our findings. These findings also suggested the notion of redundancy regarding MBL. Finally, the current study had its limitations as well. The patient sample size included in this preliminary study was relatively small. As a result, there is a need for further studies to be conducted on the Egyptian population with a larger patient sample size that encompasses diverse geographic areas. Furthermore, despite investigating the selected variant’s relationship with two autoimmune skin disorders through a combination of experimental and computational approaches, there is still a need for more forthcoming studies that analyze several genetic factors and combine them with environmental factors to understand the full picture regarding these complex diseases.
In conclusion, the in silico analysis forecasted the consequences of the presence of the selected mutation on the protein’s function, stability, PTMs, and secondary structures. Moreover, our analysis emphasized the same frequency pattern of the codon 54 variant among the Egyptian population in comparison with other populations and highlighted the unsettled ambiguity regarding this pattern. The studied variant showed no association with the risk of psoriasis and vitiligo as well. Furthermore, this mutation did not significantly correlate with the clinicopathological data for both diseases. These findings supported the redundancy of MBL and the worth of the compensatory mechanisms.
The original contributions presented in the study are included in the article/supplementary material, further inquiries can be directed to the corresponding authors.
The studies involving humans were approved by the Research Ethics Committee at the Faculty of Medicine, Suez Canal University, Egypt. The studies were conducted in accordance with the local legislation and institutional requirements. Written informed consent for participation in this study was provided by the participants or their next of kin.
MB: Conceptualization, Investigation, Methodology, Writing – original draft. NT: Investigation, Writing – review & editing. RE: Funding acquisition, Writing – review & editing. DN: Funding acquisition, Writing – review & editing. DA: Funding acquisition, Writing – review & editing. EF: Funding acquisition, Writing – review & editing. WE: Writing – review & editing. HA: Conceptualization, Funding acquisition, Investigation, Methodology, Supervision, Writing – original draft.
The author(s) declare financial support was received for the research, authorship, and/or publication of this article. The authors extend their appreciation to the Deanship of Scientific Research at King Khalid University for funding this research through large group research project under grant number RGP2/17/44. This research was also supported by Princess Nourah bint Abdulrahman University Researchers Supporting Project number (PNURSP2024R155), Princess Nourah bint Abdulrahman University, Riyadh, Saudi Arabia.
The authors extend their appreciation to the Deanship of Scientific Research at King Khalid University for funding this work through large group Research Project under grant number RGP2/17/44. The authors extend their appreciation also to Princess Nourah bint Abdulrahman University Researchers Supporting Project number (PNURSP2024R155), Princess Nourah bint Abdulrahman University, Riyadh, Saudi Arabia.
The authors declare that the research was conducted in the absence of any commercial or financial relationships that could be construed as a potential conflict of interest.
All claims expressed in this article are solely those of the authors and do not necessarily represent those of their affiliated organizations, or those of the publisher, the editors and the reviewers. Any product that may be evaluated in this article, or claim that may be made by its manufacturer, is not guaranteed or endorsed by the publisher.
1. ^ http://mutpred2.mutdb.org/index.html
2. ^ http://mupro.proteomics.ics.uci.edu/
3. ^ https://npsa-prabi.ibcp.fr/cgi-bin/npsa_automat.pl?page=/NPSA/npsa_sopma.html
1. Sharquie, KE, Salman, HA, and Yaseen, AK. Psoriasis and vitiligo are close relatives. Clin Cosmet Investig Dermatol. (2017) 10:341–5. doi: 10.2147/CCID.S142819
2. Rendon, A, and Schäkel, K. Psoriasis pathogenesis and treatment. Int J Mol Sci. (2019) 20:1475. doi: 10.3390/ijms20061475
3. Tokuyama, M, and Mabuchi, T. New treatment addressing the pathogenesis of psoriasis. Int J Mol Sci. (2020) 21:7488. doi: 10.3390/ijms21207488
4. Kamiya, K, Kishimoto, M, Sugai, J, Komine, M, and Ohtsuki, M. Risk factors for the development of psoriasis. Int J Mol Sci. (2019) 20:4347. doi: 10.3390/ijms20184347
5. Feng, Y, and Lu, Y. Advances in vitiligo: update on therapeutic targets. Front Immunol. (2022) 13:986918. doi: 10.3389/fimmu.2022.986918
6. Spritz, RA, and Santorico, SA. The genetic basis of vitiligo. J Invest Dermatol. (2021) 141:265–73. doi: 10.1016/j.jid.2020.06.004
7. Marchioro, HZ, Silva de Castro, CC, Fava, VM, Sakiyama, PH, Dellatorre, G, and Miot, HA. Update on the pathogenesis of vitiligo. An Bras Dermatol. (2022) 97:478–90. doi: 10.1016/j.abd.2021.09.008
8. Kalia, N, Singh, J, and Kaur, M. The ambiguous role of mannose-binding lectin (MBL) in human immunity. Open Med (Warsaw, Poland). (2021) 16:299–310. doi: 10.1515/med-2021-0239
9. Behairy, MY, Abdelrahman, AA, Abdallah, HY, Ibrahim, EE-DA, Hashem, HR, Sayed, AA, et al. Role of MBL2 polymorphisms in Sepsis and survival: a pilot study and in silico analysis. Diagnostics. (2022) 12:460. doi: 10.3390/diagnostics12020460
10. Ramos-Casals, M, Brito-Zerón, P, Soria, N, Nardi, N, Vargas, A, Muñoz, S, et al. Mannose-binding lectin-low genotypes are associated with milder systemic and immunological disease expression in primary Sjögren’s syndrome. Rheumatology (Oxford). (2009) 48:65–9. doi: 10.1093/rheumatology/ken411
11. Jacobson, S, Larsson, P, Åberg, A-M, Johansson, G, Winsö, O, and Söderberg, S. Levels of mannose-binding lectin (MBL) associates with sepsis-related in-hospital mortality in women. J Inflamm (Lond). (2020) 17:28. doi: 10.1186/s12950-020-00257-1
12. Gomaa, MH, Ali, SS, Fattouh, AM, Hamza, HS, and Badr, MM. MBL2 gene polymorphism rs1800450 and rheumatic fever with and without rheumatic heart disease: an Egyptian pilot study. Pediatr Rheumatol Online J. (2018) 16:24. doi: 10.1186/s12969-018-0245-x
13. Speletas, M, Dadouli, K, Syrakouli, A, Gatselis, N, Germanidis, G, Mouchtouri, VA, et al. MBL deficiency-causing B allele (rs1800450) as a risk factor for severe COVID-19. Immunobiology. (2021) 226:152136. doi: 10.1016/j.imbio.2021.152136
14. Tiyo, BT, Vendramini, ECL, de Souza, VH, Colli, CM, Alves, HV, Sell, AM, et al. Association of MBL2 exon 1 polymorphisms with multibacillary leprosy. Front Immunol. (2020) 11:1927. doi: 10.3389/fimmu.2020.01927
15. Zhang, C, Zhu, J, Li, S-L, Wang, H, and Zhu, Q-X. The association of mannose-binding lectin genetic polymorphisms with the risk of rheumatoid arthritis: a meta-analysis. J Recept Signal Transduct Res. (2015) 35:357–62. doi: 10.3109/10799893.2014.975247
16. Lee, YH, Lee, H-S, Choi, SJ, Ji, JD, and Song, GG. The association between the mannose-binding lectin codon 54 polymorphism and systemic lupus erythematosus: a meta-analysis update. Mol Biol Rep. (2012) 39:5569–74. doi: 10.1007/s11033-011-1361-6
17. Dwivedi, M, Gupta, K, Gulla, KC, Laddha, NC, Hajela, K, and Begum, R. Lack of genetic association of promoter and structural variants of mannan-binding lectin (MBL2) gene with susceptibility to generalized vitiligo. Br J Dermatol. (2009) 161:63–9. doi: 10.1111/j.1365-2133.2009.09140.x
18. Karkucak, M, Solak, B, Turan, H, Uslu, E, Yakut, T, Aliagaoglu, C, et al. MBL2 gene polymorphism and risk of vitiligo in Turkish patients. Int J Hum Genet. (2015) 15:93–6. doi: 10.1080/09723757.2015.11886257
19. Onay, H, Pehlivan, M, Alper, S, Ozkinay, F, and Pehlivan, S. Might there be a link between mannose binding lectin and vitiligo? Eur J Dermatol. (2007) 17:146–8. doi: 10.1684/ejd.2007.0128
20. Turan, H, Karkucak, M, Yakut, T, Ozsahin, M, Gurlevik, Z, Yanik, ME, et al. Does MBL2 codon 54 polymorphism play a role in the pathogenesis of psoriasis? Int J Dermatol. (2014) 53:34–8. doi: 10.1111/j.1365-4632.2012.5657.x
21. Behairy, MY, Abdelrahman, AA, Toraih, EA, Ibrahim, EE-DA, Azab, MM, Sayed, AA, et al. Investigation of TLR2 and TLR4 polymorphisms and Sepsis susceptibility: computational and experimental approaches. Int J Mol Sci. (2022) 23:10982. doi: 10.3390/ijms231810982
22. Behairy, MY, Abdelrahman, A, Abdallah, HY, Ibrahim, EE-DA, Sayed, AA, and Azab, MM. In silico analysis of missense variants of the C1qA gene related to infection and autoimmune diseases. J Taibah Univ Med Sci. (2022) 17:1074–82. doi: 10.1016/j.jtumed.2022.04.014
23. Choi, BG, Hong, JY, Hong, JR, Hur, MS, Kim, SM, Lee, YW, et al. The IL17F His161Arg polymorphism, a potential risk locus for psoriasis, increases serum levels of interleukin-17F in an Asian population. Sci Rep. (2019) 9:18921. doi: 10.1038/s41598-019-55062-5
24. Zhang, Y, Liu, J, Wang, C, Liu, J, and Lu, W. Toll-like receptors gene polymorphisms in autoimmune disease. Front Immunol. (2021) 12:672346. doi: 10.3389/fimmu.2021.672346
25. Pejaver, V, Urresti, J, Lugo-Martinez, J, Pagel, KA, Lin, GN, Nam, H-J, et al. Inferring the molecular and phenotypic impact of amino acid variants with MutPred2. Nat Commun. (2020) 11:5918. doi: 10.1038/s41467-020-19669-x
26. Cheng, J, Randall, A, and Baldi, P. Prediction of protein stability changes for single-site mutations using support vector machines. Proteins. (2006) 62:1125–32. doi: 10.1002/prot.20810
27. Geourjon, C, and Deléage, G. SOPMA: significant improvements in protein secondary structure prediction by consensus prediction from multiple alignments. Comput Appl Biosci. (1995) 11:681–4. doi: 10.1093/bioinformatics/11.6.681
28. Wang, D, Liang, Y, and Xu, D. Capsule network for protein post-translational modification site prediction. Bioinformatics. (2019) 35:2386–94. doi: 10.1093/bioinformatics/bty977
29. Wang, D, Liu, D, Yuchi, J, He, F, Jiang, Y, Cai, S, et al. MusiteDeep: a deep-learning based webserver for protein post-translational modification site prediction and visualization. Nucleic Acids Res. (2020) 48:W140–w146. doi: 10.1093/nar/gkaa275
30. Wang, D, Zeng, S, Xu, C, Qiu, W, Liang, Y, Joshi, T, et al. MusiteDeep: a deep-learning framework for general and kinase-specific phosphorylation site prediction. Bioinformatics. (2017) 33:3909–16. doi: 10.1093/bioinformatics/btx496
31. Warde-Farley, D, Donaldson, SL, Comes, O, Zuberi, K, Badrawi, R, Chao, P, et al. The GeneMANIA prediction server: biological network integration for gene prioritization and predicting gene function. Nucleic Acids Res. (2010) 38:W214–20. doi: 10.1093/nar/gkq537
32. Szklarczyk, D, Kirsch, R, Koutrouli, M, Nastou, K, Mehryary, F, Hachilif, R, et al. The STRING database in 2023: protein-protein association networks and functional enrichment analyses for any sequenced genome of interest. Nucleic Acids Res. (2023) 51:D638–46. doi: 10.1093/nar/gkac1000
33. Abdallah, HY, Abdelhamid, NR, Mohammed, EA, AbdElWahab, NY, Tawfik, NZ, Gomaa, AHA, et al. Investigating melanogenesis-related microRNAs as disease biomarkers in vitiligo. Sci Rep. (2022) 12:13526. doi: 10.1038/s41598-022-17770-3
34. Abdallah, HY, Faisal, S, Tawfik, NZ, Soliman, NH, Kishk, RM, and Ellawindy, A. Expression signature of immune-related MicroRNAs in autoimmune skin disease: psoriasis and vitiligo insights. Mol Diagn Ther. (2023) 27:405–23. doi: 10.1007/s40291-023-00646-1
35. Raharja, A, Mahil, SK, and Barker, JN. Psoriasis: a brief overview. Clin Med. (2021) 21:170–3. doi: 10.7861/clinmed.2021-0257
36. Tawfik, NZ, Abdallah, HY, Hassan, R, Hosny, A, Ghanem, DE, Adel, A, et al. PSORS1 locus genotyping profile in psoriasis: a pilot case-control study. Diagnostics (Basel, Switzerland). (2022) 12:1035. doi: 10.3390/diagnostics12051035
37. Behairy, MY, Soltan, MA, Eldeen, MA, Abdulhakim, JA, Alnoman, MM, Abdel-Daim, MM, et al. HBD-2 variants and SARS-CoV-2: new insights into inter-individual susceptibility. Front Immunol. (2022) 13:1008463. doi: 10.3389/fimmu.2022.1008463
38. Behairy, MY, Soltan, MA, Adam, MS, Refaat, AM, Ezz, EM, Albogami, S, et al. Computational analysis of deleterious SNPs in NRAS to assess their potential correlation with carcinogenesis. Front Genet. (2022) 13:872845. doi: 10.3389/fgene.2022.872845
39. Araujo, J, Segat, L, Guimarães, RL, Brandão, LAC, Souza, PER, Santos, S, et al. Mannose binding lectin gene polymorphisms and associated auto-immune diseases in type 1 diabetes Brazilian patients. Clin Immunol. (2009) 131:254–9. doi: 10.1016/j.clim.2008.12.010
40. Ji, Y-Y, and Li, Y-Q. The role of secondary structure in protein structure selection. Eur Phys J E Soft Matter. (2010) 32:103–7. doi: 10.1140/epje/i2010-10591-5
41. Brunmeir, R, and Xu, F. Functional regulation of PPARs through post-translational modifications. Int J Mol Sci. (2018) 19:1738. doi: 10.3390/ijms19061738
42. Cordell, HJ. Detecting gene-gene interactions that underlie human diseases. Nat Rev Genet. (2009) 10:392–404. doi: 10.1038/nrg2579
43. Teng, S, Madej, T, Panchenko, A, and Alexov, E. Modeling effects of human single nucleotide polymorphisms on protein-protein interactions. Biophys J. (2009) 96:2178–88. doi: 10.1016/j.bpj.2008.12.3904
44. Hammad, NM, El Badawy, NE, Nasr, AM, Ghramh, HA, and Al Kady, LM. Mannose-binding lectin gene polymorphism and its association with susceptibility to recurrent vulvovaginal candidiasis. Biomed Res Int. (2018) 2018:7648152–8. doi: 10.1155/2018/7648152
45. Badawy, M, Mosallam, DS, Saber, D, and Madani, H. Use of mannose-binding lectin gene polymorphisms and the serum MBL level for the early detection of neonatal Sepsis. J Pediatr Genet. (2018) 7:150–7. doi: 10.1055/s-0038-1675801
46. Nasr, M, Marie, A, Boghdadi, G, Elsaid, R, and Salah, E. Role of mannose binding lectin in response to candida antigen immunotherapy of warts. J Dermatolog Treat. (2021) 32:376–80. doi: 10.1080/09546634.2019.1662365
47. Garred, P, Larsen, F, Seyfarth, J, Fujita, R, and Madsen, HO. Mannose-binding lectin and its genetic variants. Genes Immun. (2006) 7:85–94. doi: 10.1038/sj.gene.6364283
48. Turner, MW. Mannose-binding lectin (MBL) in health and disease. Immunobiology. (1998) 199:327–39. doi: 10.1016/S0171-2985(98)80037-5
49. Eisen, DP, and Osthoff, M. If there is an evolutionary selection pressure for the high frequency of MBL2 polymorphisms, what is it? Clin Exp Immunol. (2014) 176:165–71. doi: 10.1111/cei.12241
50. Tereshchenko, SY, Smolnikova, MV, and Freidin, MB. Mannose-binding lectin gene polymorphisms in the East Siberia and Russian Arctic populations. Immunogenetics. (2020) 72:347–54. doi: 10.1007/s00251-020-01175-5
51. Heitzeneder, S, Seidel, M, Förster-Waldl, E, and Heitger, A. Mannan-binding lectin deficiency – good news, bad news, doesn’t matter? Clin Immunol. (2012) 143:22–38. doi: 10.1016/j.clim.2011.11.002
52. Søborg, C, Madsen, HO, Andersen, AB, Lillebaek, T, Kok-Jensen, A, and Garred, P. Mannose-binding lectin polymorphisms in clinical tuberculosis. J Infect Dis. (2003) 188:777–82. doi: 10.1086/377183
53. Alonso, DP, Ferreira, AFB, Ribolla, PEM, Santos, IKFM, Cruz, MSP, Carvalho, FA, et al. Genotypes of the mannan-binding lectin gene and susceptibility to visceral leishmaniasis and clinical complications. J Infect Dis. (2007) 195:1212–7. doi: 10.1086/512683
54. Troelsen, LN, Garred, P, and Jacobsen, S. Mortality and predictors of mortality in rheumatoid arthritis--a role for mannose-binding lectin? J Rheumatol. (2010) 37:536–43. doi: 10.3899/jrheum.090812
55. Troelsen, LN, Garred, P, Madsen, HO, and Jacobsen, S. Genetically determined high serum levels of mannose-binding lectin and agalactosyl IgG are associated with ischemic heart disease in rheumatoid arthritis. Arthritis Rheum. (2007) 56:21–9. doi: 10.1002/art.22302
56. Boldt, ABW, Messias-Reason, IJ, Meyer, D, Schrago, CG, Lang, F, Lell, B, et al. Phylogenetic nomenclature and evolution of mannose-binding lectin (MBL2) haplotypes. BMC Genet. (2010) 11:38. doi: 10.1186/1471-2156-11-38
57. Verdu, P, Barreiro, LB, Patin, E, Gessain, A, Cassar, O, Kidd, JR, et al. Evolutionary insights into the high worldwide prevalence of MBL2 deficiency alleles. Hum Mol Genet. (2006) 15:2650–8. doi: 10.1093/hmg/ddl193
58. Zeng, J, Chen, X, Lei, K, Wang, D, Lin, L, Wang, Y, et al. Mannan-binding lectin promotes keratinocyte to produce CXCL1 and enhances neutrophil infiltration at the early stages of psoriasis. Exp Dermatol. (2019) 28:1017–24. doi: 10.1111/exd.13995
59. Zeng, J, Wang, D, Luo, J, Li, L, Lin, L, Li, J, et al. Mannan-binding lectin exacerbates the severity of psoriasis by promoting plasmacytoid dendritic cell differentiation via the signal transducer and activator of transcription 3-interferon regulatory factor 8 axis. J Dermatol. (2022) 49:496–507. doi: 10.1111/1346-8138.16323
60. Casanova, J-L, and Abel, L. Human genetics of infectious diseases: unique insights into immunological redundancy. Semin Immunol. (2018) 36:1–12. doi: 10.1016/j.smim.2017.12.008
61. Lynch, NJ, Roscher, S, Hartung, T, Morath, S, Matsushita, M, Maennel, DN, et al. L-ficolin specifically binds to lipoteichoic acid, a cell wall constituent of gram-positive bacteria, and activates the lectin pathway of complement. J Immunol. (2004) 172:1198–202. doi: 10.4049/jimmunol.172.2.1198
62. Roos, A, Garred, P, Wildenberg, ME, Lynch, NJ, Munoz, JR, Zuiverloon, TCM, et al. Antibody-mediated activation of the classical pathway of complement may compensate for mannose-binding lectin deficiency. Eur J Immunol. (2004) 34:2589–98. doi: 10.1002/eji.200324401
Keywords: psoriasis, vitiligo, MBL, innate immunity, SNP, rs1800450
Citation: Behairy MY, Tawfik NZ, Eid RA, Nasser Binjawhar D, Alshaya DS, Fayad E, Elkhatib WF and Abdallah HY (2024) Mannose-binding lectin gene polymorphism in psoriasis and vitiligo: an observational study and computational analysis. Front. Med. 10:1340703. doi: 10.3389/fmed.2023.1340703
Received: 18 November 2023; Accepted: 28 December 2023;
Published: 09 February 2024.
Edited by:
Abdul Azeez Sayed, Imam Abdulrahman Bin Faisal University, Saudi ArabiaReviewed by:
Padmaja Mummaneni, United States Food and Drug Administration, United StatesCopyright © 2024 Behairy, Tawfik, Eid, Nasser Binjawhar, Alshaya, Fayad, Elkhatib and Abdallah. This is an open-access article distributed under the terms of the Creative Commons Attribution License (CC BY). The use, distribution or reproduction in other forums is permitted, provided the original author(s) and the copyright owner(s) are credited and that the original publication in this journal is cited, in accordance with accepted academic practice. No use, distribution or reproduction is permitted which does not comply with these terms.
*Correspondence: Mohammed Y. Behairy, bW9oYW1lZHllaHlhOTUwQGdtYWlsLmNvbQ==; Dalal Sulaiman Alshaya, ZHNhbHNoYXlhQHBudS5lZHUuc2E=
Disclaimer: All claims expressed in this article are solely those of the authors and do not necessarily represent those of their affiliated organizations, or those of the publisher, the editors and the reviewers. Any product that may be evaluated in this article or claim that may be made by its manufacturer is not guaranteed or endorsed by the publisher.
Research integrity at Frontiers
Learn more about the work of our research integrity team to safeguard the quality of each article we publish.