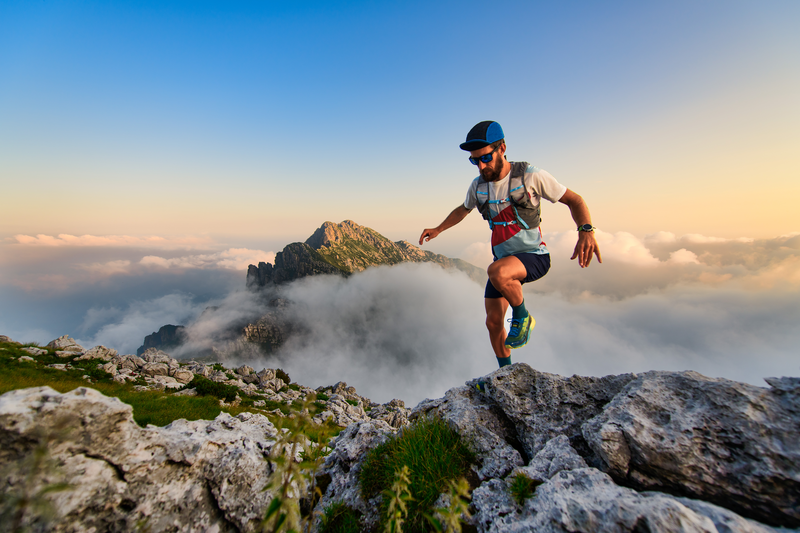
94% of researchers rate our articles as excellent or good
Learn more about the work of our research integrity team to safeguard the quality of each article we publish.
Find out more
ORIGINAL RESEARCH article
Front. Med. , 11 January 2024
Sec. Regulatory Science
Volume 10 - 2023 | https://doi.org/10.3389/fmed.2023.1308047
This article is part of the Research Topic Women in Science - Regulatory Science 2023 View all 6 articles
Strategic regulatory development is essential to ensure that new innovations in nanotechnology-enabled health products (NHPs) successfully reach the market and benefit patients. Currently, the lack of specific regulatory guidelines for NHPs is considered one of the primary causes of the so-called “valley of death” in these products, impacting both current and future advancements. In this study, we have implemented a methodology to anticipate key trends in NHP development and compare them with the current regulatory landscape applicable to NHPs. This methodology relies on Horizon Scanning, a tool commonly used by policymakers to foresee future needs and proactively shape a regulatory framework tailored to those needs. Through the application of this methodology, different trends in NHP have been identified, notably NHPs for drug delivery and dental applications. Furthermore, the most disruptive elements involve NHPs that are multicomposite and multifunctional, harnessing nano-scale properties to combine therapeutic and diagnostic purposes within a single product. When compared with the regulatory landscape, current regulations are gradually adapting to accommodate emerging trends, with specific guidelines being developed. However, for the most disruptive elements, multicomposite and multifunctional NHPs, their novelty still poses significant regulatory challenges, requiring a strategic development of guidelines by regulatory agencies to ensure their safe and effective integration into healthcare practices. This study underscores the importance of proactive regulatory planning to bridge the gap between NHP innovation and market implementation.
The global nanotechnology market is expected to see a significant growth throughout the current decade (1). Predictions suggest a compound annual growth rate (CAGR)1 ranging from 9.2% to 36.4% between 2000 and 2030. The global nanomaterials market was valued at USD 7.1 billion in 2020, and it is predicted to grow to USD 13.60 billion by 2027. This trend is largely driven by their increasing incorporation into nanotechnology-enabled health products (NHPs),2 particularly in applications for drug delivery. Namely, the fastest growth is expected to occur in the Asia-Pacific region (2).
However, the successful innovation of NHPs must be supported by the development of a robust regulatory framework to ensure that the quality, safety, and efficacy of these products can be adequately assessed by health authorities. In 2020, the European Medicines Agency (EMA) acknowledged the need to “develop understanding of, and regulatory response to, nanotechnology and new materials in pharmaceuticals” (3). Among different underlying actions, the development and standardisation of novel testing methodologies for nanomedicines were identified as paramount, particularly for safety and quality assessments.
In line with this, the European Technology Platform for Nanomedicine’s 2016 report on the “Strategic Research and Innovation Agenda for Nanomedicine 2016–2030” emphasised early engagement with regulators about nanomedical trends to ensure adaptable regulations and a “fast but safe track” to innovation (4).
Despite these initiatives, the pace of regulations advancement is lagging behind that of NHP innovation (5). This discrepancy is frequently identified as a contributing factor to the “valley of death” in NHPs, a term used to describe the significant gap between the number of applications in research and development and the number of products (both medicinal products and medical devices) on the market (6, 7).
To prevent this situation, policy makers can utilize various tools to anticipate and address future regulatory needs. One such tool is Horizon Scanning, which is a systematic methodology designed to identify early signs of significant developments, opportunities and threats that could impact a specific area (8). The use of Horizon Scanning methodology is well-established among legislators. In health politics for instance, it is used by the governments of United Kingdom, the Netherlands and Singapore. In addition, international organizations like the World Health Organization (WHO) (9) and regulatory bodies such as the EMA, the British Medicines and Healthcare Products Regulatory Agency (MHRA) and the Japanese Pharmaceuticals and Medical Devices Agency (PMDA) also use Horizon Scanning-based methodologies for strategic regulatory development (10–13).
Horizon Scanning can be commonly implemented through two distinct approaches: exploratory scanning and issue-centred scanning. Exploratory scanning is a heuristic search for information aimed at identifying potential issues and signs by reviewing various sources of information or “scans” (see Table 1 for key terms used in Horizon Scanning). Conversely, issue-centred scanning begins with the analysis of a set of core documents to identify signals within a specific field of study (15). Although there is no standardised description for implementing the Horizon Scanning methodology (17), it is traditionally described as a process that includes the following steps: signal detection, filtration, prioritization, assessment, and dissemination (18). It is important to note that Horizon Scanning is not a simple prediction tool; predictions usually deal with broader topics and have longer-term impacts. In contrast, Horizon Scanning focuses more narrowly on specific topics in the short to medium term (18).
Again, for regulatory agencies, the application of future-oriented methodologies, such as Horizon Scanning, serves to pre-emptively identify upcoming needs. This foresight facilitates the creation of specific guidelines or legislation, ensuring that all innovations are well addressed within their applicable regulatory framework. The objective is to establish expertise in advance of evaluating forthcoming innovative products, thereby streamlining their path to market by minimizing potential developmental, legal or regulatory bottlenecks (12).
As previously discussed, the current regulatory state of the art applicable to NHPs, understood as the most recent versions of regulatory guidelines and technical standards published and under development (19), is a known cause of the “valley of death” for this kind of products. This situation is considered a challenge that could potentially escalate with the swift progression of nanotechnology. In this paper, we suggest utilizing the Horizon Scanning methodology to focus on the advancements in NHPs. The aim is to predict upcoming trends and improve the strategic development of regulatory guidelines.
Guidelines and other regulatory documents applicable to NHPs have been gathered in a regulatory database that includes references identified up to August 31st 2023 from the following sources:
• Competent authorities:
o European Medicines Agency (EMA): https://www.ema.europa.eu/en/human-regulatory/research-development/scientific-guidelines/multidisciplinary/multidisciplinary-nanomedicines.
o United States Food and Drug Administration (FDA): https://www.fda.gov/science-research/nanotechnology-programs-fda/nanotechnology-guidance-documents.
• European Commission:
o EU Science Hub: https://joint-research-centre.ec.europa.eu/scientific-activities-z/nanotechnology_en.
o Scientific Committee on Emerging and Newly Identified Health Risks (SCENHIR): https://health.ec.europa.eu/scientific-committees/former-scientific-committees/scientific-committee-emerging-and-newly-identified-health-risks-scenihr_en.
• Organization for the Economic Co-operation and Development (OECD), Safety of manufactured nanomaterials: https://www.oecd.org/science/nanosafety/.
• Standards emitting organizations:
o International Organization for Standardization (ISO), Technical committee ISO/TC 229 on Nanotechnologies: https://www.iso.org/committee/381983.html.
o American Society for Testing and Materials (ASTM), Technical subcommittee E56.08 on Nano-enabled Medicinal Products: https://www.astm.org/get-involved/technical-committees/committee-e56/subcommittee-e56.
The adaptation and implementation of the Horizon Scanning methodology has been developed as part of the objectives of this manuscript. It is applied in four stages based on exploratory scanning approach: (i) signal detection, (ii) filtration, (iii) prioritization, and (iv) assessment (18).
Various scanners were used for signal detection such as scientific publication databases, clinical study databases, and international patent registries that encompass NHPs at different developmental stages. To effectively handle the volume of information, searches were performed during three distinct periods of time, ranging from January 2020 to June 2022 (refer to Table 2). Any results not directly relevant to a specific NHP were discarded.
Novelty was employed as a filtration criterion to eliminate irrelevant signals and as a prioritization standard for categorizing results into potential trends (weak signals) or disruptive elements (wild cards). Besides, the methodology for novelty quantification was adapted based on the novelty metrics proposed by Shah et al. (20). This widely recognised approach, originating from the field of design creativity research, characterises novelty as a measure of how unusual or unexpected an idea is compared to other ideas (20, 21).
The detected trends and wild cards were assessed for their potential impact on the current regulatory state-of-the art (i.e., guidelines and standards). This assessment involved a detailed analysis of how these detected signals fit within the scope of the existing regulatory science, as reflected in the regulatory database. The aim of this assessment was to identify potential areas of insufficient regulation.
Detected signals on NHP development have been indexed following the classification system described in a previous work of our group (22). This classification system considers both scientific and regulatory criteria and allows the grouping of NHPs into different categories, expected to share similar relevant characteristics when evaluated by regulatory authorities (refer to Figure 1). The system assigns a unique four-digit code, or classification signature, to each category of NHP. This code is based on the NHP’s principal mode of action, chemical composition, intended purpose, and the approach followed in its nanomanufacturing (22).
Figure 1. Nanotechnology-enabled health products classification system [taken from Rodríguez-Gómez et al. (22)].
Databases have been generated with Excel macro-enabled workbook format (Microsoft Office Professional Plus 2019). Data processing and plotting was carried out by using dynamic tables.
The scanners chosen for signal detection included databases that may represent NHPs at various development stages. Data obtained from patent registers typically represent NHPs in the early stages of development. These NHPs might either be newly described or not yet evaluated by a regulatory authority in terms of quality, safety, and performance. Conversely, the clinical studies database represents NHPs that have been assessed by a regulatory authority. These products have already initiated at least initial first-in-human studies. Finally, data obtained from scientific literature databases can cover the entire spectrum: newly developed NHPs or already approved NHPs.
Scopus was selected as the database for signal detection among scientific publications. It is a comprehensive database comprising peer-reviewed literature from scientific journals, books, and conference proceedings. Compared to PubMed and Web of Science, Scopus was chosen due to its broader spectrum of journals (23). An extensive literature search was conducted on NHPs with various applications (treatment, diagnosis, prophylaxis). A total of 330 articles were analysed, and 102 articles were selected. The literature search strategy is described in Table 2 and the search results are summarised in Table 3.
The European Patent Office (EPO) Database is a crucial resource, providing access to an extensive collection of over 90 million patent documents sourced from more than 90 countries worldwide. This comprehensive database was chosen for signal detection among patents as it illustrates the evolving landscape of global technological advancements, incorporating detailed information on patent applications, patents granted, and their respective status (24). Based on search strategy described in Table 2 a total of 659 patents documents were analysed. From those, 524 were selected for the first stage of signal detection in Horizon Scanning. A summary of the results is listed in Table 3.
ClinicalTrials.gov is a web-based resource that provides free access to data on publicly and privately supported clinical studies. Sponsored by the U.S. National Library of Medicine, this database offers information about a trial’s purpose, assessed products and participation criteria among other details (25). All clinical studies registered under key prefix nano* were retrieved as described in Table 2. A total of 405 studies were analysed, and 195 were selected for applying the Horizon Scanning methodology. The results are summarised in Table 3.
Each detected signal was stored in a database. In this database, every record was linked to its original source of information and classified according to the system defined by Rodríguez-Gómez et al. (22). Figure 2 includes a summary of all the detected signals among the three scans.
Novelty was used as a key criterion for filtering and prioritizing the detected signals. A novelty quantification methodology was applied for this purpose. Originally developed for design creativity research, this methodology provides a holistic approach to assess the creativity of various ideas or “design solutions” by contrasting each idea with the entire set of design solutions (20). This structured approach aids objective research in design creativity (21, 26).
As proposed by Shah et al. (20), a design problem often leads to the generation of multiple potential solutions, termed as “design artefacts.” Each artefact possesses an “attribute” that outlines its potential to resolve the design problem, referred to as “design solution.” In a situation where various unique solutions are proposed for a design problem, the artefact with the least frequently repeated attribute is considered more novel (Figure 3). For example, given the design problem of developing propulsion systems, if four artefacts are proposed, with three based on sail (design solution 1) and one on jet (design solution 2), the jet-based artefact is deemed more novel due to its uniqueness (20, 27).
Figure 3. Novelty quantification rationale as per Shah et al. (20).
Shah et al. (20) methodology has been adapted to quantify the novelty of detected signals. In this context, the various design problems correspond to six categories, which are derived from a combination of two criteria of the applied NHP classification system. Specifically, these criteria are the primary mode of action and medical purpose, resulting in the following design problems:
• Products with pharmacological, immunological, or metabolic action of therapeutic/treating effect.
• Products with pharmacological, immunological, or metabolic action of therapeutic/prophylactic effect.
• Products with non-pharmacological, immunological, or metabolic action of therapeutic effect.
• Products with non-pharmacological, immunological, or metabolic action of not in vitro diagnostic effect.
• Products with non-pharmacological, immunological, or metabolic action of in vitro diagnostic effect.
• Products with non-pharmacological, immunological, or metabolic action of galenic effect.
Each of these design problems would be the same as the above-mentioned problem of designing a propulsion system and, throughout the design process, NHPs with diverse attributes may be generated to resolve each problem category. For instance, the first category could include NHPs with antifungal and anti-inflammatory actions, while the third might encompass NHPs with structural and moisturizing actions. Each attribute corresponds to a distinct design solution. Supplementary Table S1 provides a comprehensive list of all design solutions (identified by a design solution code, DSC) defined for all NHPs in the detected signals.
For each detected signal, the corresponding NHP and DSC are determined, and novelty is quantified according to Eq. 1. Signals with a novelty score of 10 or closer are deemed more novel. Additionally, the cumulative percentage of the frequency of design solutions can be displayed in descending order of frequency per design solution. This approach, as established by Sluis-Thiescheffer et al. (27), allows setting the third quartile (Q3) as the threshold to identify the most disruptive and novel elements (wild cards), while the first quartile (Q1) can denote trends in design solutions. Figure 4 provides an overview of this representation encompassing the complete spectrum of identified signals sourced from scientific literature, patent registries, and clinical study databases.
Novelty score calculation:
S1 is the “novelty score” for design solution 1. C1 is the frequency for design solution 1. T is the total number of design solutions.
Novelty analysis of the design solutions of detected signals. Design solutions are in descending order of frequency (i.e., in ascending order of novelty). The red squares show the 0.25 and 0.75 percentiles, Q1 and Q3, respectively. All design solutions with a frequency higher or equal to Q1 are considered tentative trends, all design solutions with a frequency lower or equal to Q3 are considered tentative wild cards.
As initially outlined, the identification of trends among all detected signals is determined by the frequency distribution of each DSC. NHPs whose DSCs fall within the top quartile of the total distribution are classified as trends, or weak signals. Figure 4 shows that frequencies exceeding the Q1 correspond to three distinct DSCs, representing 217 of the 836 detected NHPs. Table 4 compiles the DSCs classified as trends, while Figure 5 presents the distribution of NHPs linked to these trends across various information sources.
Based on the results, a clear trend towards the development of drug delivery systems (DDS) is evident. The most prevalent systems are based on polymer (DSC: B.T.2.3) and lipid (DSC: C.T.2.3) chemistry (95 and 61 selected signals respectively). Moreover, a growing trend in the development of nanomaterials for dental applications (DSC: E.T.2.1), such as surface filling or tooth replacement, is also notable (61 selected signals).
Figure 6 summarises the types of NHPs associated with the DSCs B.T.2.3, C.T.2.3, and E.T.2.1. As expected, all identified NHPs linked to DDS, i.e., B.T.2.3 and C.T.2.3, possess a classification signature featuring “2.3.n.n”, indicating a non-pharmacological, immunological, or metabolic action (“2.n.n.n”) and a galenic function (“n.3.n.n”). For NHPs with DSC B.T.2.3, those with a classification signature “2.3.2.1” predominate. This indicates a molecular building block composition (“n.n.2.n”) and a bottom-up nanofabrication process (“n.n.n.1”). For NHPs with DSC C.T.2.3, carbon-based NHPs, particularly those with self-assembling structures in physiological medium (“n.n.3s.n”), are dominant. All these NHPs are produced using a bottom-up nanofabrication approach (“n.n.n.1”).
Figure 6. NHP classification code of signals identified as trends (weak signals). (A) Represents NHPs for trends identified as molecular building block nanocarrier (B.T.2.3). (B) Represents NHPs for trends identified as dental filling/replacement material (E.T.2.1). (C) Represents NHPs for trends identified as lipid nanocarrier (C.T.2.3).
In the case of NHPs with DSC E.T.2.1, dental filling/replacement material, all detected signals correspond to NHPs with a primary action that is not based on pharmacological, immunological, or metabolic means and whose medical indication is therapeutic (signature featuring “2.1.n.n”). The chemical composition varies, with metallic (“n.1.n.n”) composition being predominant, followed by polymeric (“n.2.n.n”), carbon-based (“n.3.n.n”), or occasionally, undefined compositions (“n.0.n.n”). The nanofabrication approach is often undefined, but some NHPs are produced using bottom-up (“n.n.n.1”), top-down techniques (“n.n.n.2”), or combinations thereof.
Overall, the three DSCs identified as trends correspond to NHPs with relatively consistent classification signatures.
The increasing interest within the medical community towards DDS reflects their potential to significantly transform therapeutic interventions (28, 29), particularly in the principal therapeutic areas of nanotechnology development, which are predominantly cancer and then infectious diseases (1, 2). These complex systems serve to improve solubility, encapsulation efficacy and therefore the pharmacokinetic properties of medicinal products. In addition, DDS can be designed for targeting specific cells or tissues, thereby enhancing efficacy while reducing systemic side effects (30). DDS have the ability to control the time and location of drug release, assuring a consistent therapeutic effect and overcoming the limitations of traditional dosage forms. Moreover, DDS can be designed to overcome biological barriers, enabling the delivery of a wider range of therapeutics, including genes and biologics. Eventually, it is estimated that 90% of new medicinal products developed by the pharmaceutical industry are discarded despite their effectivity due to solubility issues (31). In response to the decreasing number of new pharmaceuticals, there is a growing trend towards exploring alternative drug delivery methods to increase the safety and efficacy of existing pharmaceuticals (31, 32). In addition, the global pharmaceutical drug delivery market, indicative of the potential and growth of DDS, is projected to reach a staggering USD 2206.5 billion by 2026, exhibiting a CAGR of 5.9% (33). In this context, lipid and polymer platforms have been identified as trends in NHP development based on Horizon Scanning methodology (Table 4).
The integration of nanomaterials into dentistry represents a significant advancement in enhancing oral health care. These nano-based structures, with their unique physicochemical properties, have led to the development of innovative dental materials and techniques. Nanocomposites and nanoceramics, for example, offer excellent aesthetic and mechanical properties, thereby improving the durability and appearance of restorations. Furthermore, the application of nanotechnology in dentistry goes beyond restorative materials, with nanoparticle-based antimicrobials offering a promising strategy in combating oral biofilms (34).
Importantly, many nanomaterial applications in dentistry are based on structural action, suggesting possible classification within the regulatory category of medical devices in the European Union (35). This hypothesis is backed by the majority of detected signals related to these types of products coming from clinical trial registries (Figure 5). This is also in agreement with the fact that medical devices undergo a shorter regulatory preclinical development compared to pharmaceuticals, thus enabling earlier evaluation in human studies.
Among the total detected signals, the most innovative NHPs correspond to those with the highest novelty scores. These are the NHPs with design solutions falling within the third quartile as shown in Figure 4. However, when analysing the 219 results obtained, prioritizing some NHPs over others in terms of novelty proved challenging due to the similar and near-maximum (between 9.89 and 9.99) novelty scores (Figure 7). Hence, a novelty-enrichment method has been proposed to adjust the novelty scores based on various criteria. Table 5 presents the criteria employed for novelty-correction and the rationale for each.
After implementing this adjustment, the distribution of corrected novelty scores becomes segregated (Figure 8). Consequently, five NHPs with the highest novelty scores are identified. These are NHPs whose combinations of design solutions appeared only once in the total of 836 detected signals (Table 6). It can be inferred that the NHPs identified as the most innovative (wild cards) correspond to systems integrating nanomaterials with diverse chemical compositions, capable of possessing various therapeutic, diagnostic, or galenic effects in a single entity.
The emergence of multicomponent and multifunctional nanomaterials in healthcare signifies a transformative leap in medical therapeutics and diagnosis (36). These complex structures offer enhanced functionality by facilitating the concurrent delivery of diverse therapeutics and diagnostic agents. This multifunctionality not only amplifies therapeutic efficacy, but also enables real-time monitoring of treatment efficacy, representing the essence of theranostics (37). Furthermore, these nanomaterials can be engineered to treat pathologies with multiple mechanisms of action simultaneously (38, 39). This capacity is largely attributed to the unique properties that materials exhibit at the nanometric scale (40), providing a level of therapeutic control unparalleled in conventional treatment modalities. Consequently, the exploration of these sophisticated nanomaterials heralds a promising future in personalised medicine, paving the way for more efficient and customised healthcare solutions (41).
In the final phase of the Horizon Scanning process, signals detected date from June 2022. There exists roughly a one-year gap between the last update of the regulatory database (August 31st, 2023) and the detection of these signals. However, this gap is not expected to considerably affect the conclusions drawn. As previously stated, the rate of innovation in NHPs outpaces the progress of their regulations. Thus, despite the one-year difference, there may be areas still insufficiently regulated, as highlighted in this study.
In broad terms, the European legal basis of NHPs intended for dental use, classified under the DSC E.T.2.1, is the Regulation (EU) 2017/745 of the European Parliament and of the Council of 5 April 2017 on medical devices which refers to products with a medical purpose exerting a physical/mechanical primary mode of action. In the regulatory database, only 2 documents were found to directly address NHPs under E.T.2.1: “ISO/TS 23367-1:2022 – Nanotechnologies — Performance characteristics of nanosensors for chemical and biomolecule detection – Part 1: Detection performance” and the European Commission’s “Opinion on Titanium Dioxide (nano form) as UV-Filter in sprays” (refer to the complete Regulatory database attached in Supplementary Table S2). However, it is important to note that none of these documents refer to dental application products.
Contrarily, in Europe DDS are governed by Directive 2001/83/EC of the European Parliament and of the Council of 6 November 2001 on the Community code relating to medicinal products for human use. Table 7 provides a thorough list of all identified regulatory documents specific to NHPs with a galenic action. Out of the 9 references, 6 are dedicated to nanoliposomes (NHP signature 2.3.3 s.1) and 1 document is addressing polymer-based DDS (NHP signature 2.3.2.1).
A closer look at the Nanotechnology Product Database (NPD), recognised as a reliable resource for institutions and policymakers establishing national policies and strategic plans (42), reveals a specific category for dental applications of NHPs. This category includes a total of 190 approved products worldwide (as of 11 December 2023). The NPD website has also a dedicated section for pharmaceutical products, which includes a total of 432 approved products worldwide (as of 11 December 2023). In this section, products utilising polymeric nanoparticles and nanoliposomes are the most common among the approved products (59 and 15, respectively).
This information suggests that despite the relatively low number of specific regulatory documents for NHPs for dental applications or galenic action (based on polymers and lipids), these types of NHPs have most frequently achieved regulatory approval. One possibility for this is that development and evaluation of these NHPs may have been based on other more general, less specific regulatory guidelines to achieve regulatory approval.
On the one hand, nano-filled composite dental materials demonstrate superior physical properties, such as compressive strength, tensile strength, toughness, flexural strength, and abrasion resistance, compared to their micro-particle filled counterparts. Historically, nanocomposite materials have been utilised in dentistry, and this remains a growing area of research and innovation (34). Given its established nature, regulatory hurdles are not anticipated in this specific area.
On the other hand, although polymer and lipid-based nanoparticles appear to be achieving more regulatory approval success due to extensive experience and knowledge within the scientific community, along with their generally optimal controlled-release and stability profile, there are still substantial challenges for DDSs (43). These challenges mainly include the need for safety and biological behaviour assessment prior to approval. In biological environments, the constituents of the medium are typically adsorbed onto the nanoparticle surface due to their small size, creating a hybrid system where the nanoparticle becomes enveloped with organic particles, predominantly proteins, forming a corona (44, 45). This phenomenon alters the expected pharmacokinetic compartment for small drug molecules, which is not equivalent for NHPs (46). The connection between the physicochemical properties of NHPs and their pharmacokinetic behaviour and safety profile requires a more thorough understanding, and is considered one of the main challenges in meeting regulatory expectations (47–49). In fact, in light of these complexities, the EMA has underscored the importance of establishing guidelines for the evaluation of pharmacodynamics and pharmacokinetics of NHPs, as a fundamental part of its regulatory science strategy towards 2025 (3).
The innovation of nanotechnology is introducing a new era of multifunctional and multicomposite NHPs, each with unique properties and potential applications (50). These innovative products are often developed by adopting one of two primary strategies, commonly referred to as the “one-for-all” and “all-in-one” approaches. The “one-for-all” approach involves the development of a single building block with inherent multifunctional properties (51, 52), whereas the “all-in-one” approach combines different agents into a single nanoparticle with distinct sections, each serving a different function (29, 39, 53).
NHPs produced using the “all-in-one” approach are often designed with stealth-like features to evade the immune system and prevent opsonization, protective layers to prevent degradation, targeting moieties for improved specificity, membrane-permeation moieties for enhanced cell uptake, as well as imaging agents for delivery and dosing control, and monitoring. However, each additional functionality increases complexity and cost, particularly due to the heterogeneous nature of the formulations (38). Notwithstanding, “all-in-one” NHPs offer the opportunity to utilise components that have already been approved or have other healthcare applications, potentially mitigating challenges for regulatory approval. In contrast, the “one-for-all” approach often requires the exploration of novel materials with inherent multifunctionality, which can complicate regulatory approval due to their novelty (39).
Despite their complexities, multifunctional NHPs offer considerable advantages such as the potential to combine different imaging techniques that provide complementary information, or to deliver substances with synergistic mechanisms of action that can enhance treatment efficacy. However, no specific regulatory guidelines have been identified that address specifically multifunctional NHPs. These disruptive elements, indeed, mandate strategic reinforcement to existing regulations. The goal shall be to facilitate the development of these innovative health products, ensure their comprehensive evaluation, and realise their full benefits, all the while ensuring patient safety. As such, filling up the current regulatory landscape becomes essential to appropriately address these uniquely complex and promising products.
To conclude, a notable disparity exists between the innovative medical products being developed and those that achieve regulatory approval, especially in the case of NHPs. This is primarily due to the lack of adequate regulatory guidelines that can effectively evaluate the quality, safety, and efficacy of these products. The application of Horizon Scanning methodology, a standard practice among legislators and health authorities, can be key for anticipating future regulatory needs. Using Horizon Scanning methodology, this study identifies DDSs and dental applications as leading trends in NHP development. In turn, multifunctional and multicomposite products are identified as the most novel.
While the regulatory landscape is becoming more accommodating for emerging trends, substantial challenges still exist for the most innovative technologies. As regulatory science works to keep up with technological innovation, the continued focus on developing robust and adaptable regulatory guidelines for NHPs is of utmost importance. This will facilitate the safe and effective transition of these groundbreaking products from the research stage to clinical application, ultimately serving the best interests of patients and propelling the progress of nanomedicine.
The raw data supporting the conclusions of this article will be made available by the authors, without undue reservation.
FDRG: Conceptualization, Data curation, Formal analysis, Investigation, Methodology, Project administration, Visualization, Writing – original draft. DM: Conceptualization, Funding acquisition, Project administration, Supervision, Validation, Writing – review & editing. OP: Conceptualization, Funding acquisition, Project administration, Supervision, Validation, Writing – review & editing. PRG: Conceptualization, Funding acquisition, Supervision, Validation, Writing – review & editing.
The author(s) declare financial support was received for the research, authorship, and/or publication of this article. This work was supported by the Industrial Doctorates Plan of the Department of Research and Universities of the Generalitat de Catalunya (grant no 202015). PRG acknowledges the Ministry of Science, Innovation and Universities (MICINN-AEI) (AEI-PID2019-106755RB-I00 and AEI-PID2022-140423NB-I00) and the AGAUR (2021 SGR 00175 and 2021 PROD 00041) for financial support.
FDRG, OP, and DM were employed by company Asphalion SL.
The remaining author declares that the research was conducted in the absence of any commercial or financial relationships that could be construed as a potential conflict of interest.
PRG declared that she was an editorial board member of Frontiers, at the time of submission. This had no impact on the peer review process and the final decision.
All claims expressed in this article are solely those of the authors and do not necessarily represent those of their affiliated organizations, or those of the publisher, the editors and the reviewers. Any product that may be evaluated in this article, or claim that may be made by its manufacturer, is not guaranteed or endorsed by the publisher.
The Supplementary material for this article can be found online at: https://www.frontiersin.org/articles/10.3389/fmed.2023.1308047/full#supplementary-material
1. ^“Compound Annual Growth Rate (CAGR)” average yearly growth rate over a specified period of time, providing a smoothed annual growth perspective.
2. ^Nanotechnology-enabled health product’ is an overarching term for nanomedicines (health products including nanomaterials that are regulated as medicinal products) and nanomedical devices (health products including nanomaterials that are regulated as medical devices).
1. Bosetti, R, and Jones, SL. Cost-effectiveness of nanomedicine: estimating the real size of nano-costs. Nanomedicine (Lond). (2019) 14:1367–70. doi: 10.2217/nnm-2019-0130
2. Domingues, C, Santos, A, Alvarez-Lorenzo, C, Concheiro, A, Jarak, I, Veiga, F, et al. Where is Nano today and where is it headed? A review of nanomedicine and the dilemma of Nanotoxicology. ACS Nano. (2022) 16:9994–10041. doi: 10.1021/acsnano.2c00128
3. European Medicines Agency, E. (2020). Ema regulatory science to 2025 [Online]. Amsterdam: European Medicines Agency. Available at: https://www.ema.europa.eu/en/documents/regulatory-procedural-guideline/ema-regulatory-science-2025-strategic-reflection_en.pdf [Accessed].
4. European Technology Platform For Nanomedicine. (2016). Strategic research and innovation agenda for nanomedicine 2016–2030 [online]. Available at: https://etp-nanomedicine.eu/wp-content/uploads/2018/09/Nanomedicine-Sria-2016-2030.pdf (Accessed).
5. Ali, F, Neha, K, and Parveen, S. Current regulatory landscape of nanomaterials and nanomedicines: a global perspective. J Drug Deliv Sci Technol. (2023) 80:104118. doi: 10.1016/j.jddst.2022.104118
6. Coty, JB, and Vauthier, C. Characterization of nanomedicines: a reflection on a field under construction needed for clinical translation success. J Control Release. (2018) 275:254–68. doi: 10.1016/j.jconrel.2018.02.013
7. Ferreri, A, Matteucci, F, Giannantonio, R, Grande, S, and Allan, J. Technology transfer in nanotechnology. Luxembourg: Publications Office of the European Union (2019).
8. Mhra. (2013). Review of cross-government horizon scanning [online]. London (UK): Medicines and Healthcare Products Regulatory Agency. Available at: https://assets.publishing.service.gov.uk/government/uploads/system/uploads/attachment_data/file/79252/Horizon_Scanning_Review_20121003.pdf [Accessed 27/07/2023].
9. WHO. (2022). Emerging trends and technologies: A horizon scan for global public health [online]. Available at: https://www.who.int/publications/i/item/9789240044173 (Accessed July 27, 2023).
11. Fukaya-Shiba, A, Shimokawa, M, Sasaki, H, and Wakao, R. Pharmaceuticals and medical devices Agency's horizon scanning and the science board: cooperation toward extracellular vesicle-based products. Br J Clin Pharmacol. (2022) 88:1392–4. doi: 10.1111/bcp.15065
12. Shimokawa, M, Sato, D, Wakao, R, and Arai, H. Pmda's vision for horizon scanning of emerging technologies potentially relevant to the development of new medical products: the regulatory challenge. Clin Pharmacol Ther. (2021) 109:295–8. doi: 10.1002/cpt.1986
13. Vignali, V, Hines, PA, Cruz, AG, Ziętek, B, and Herold, R. Health horizons: future trends and technologies from the European medicines Agency’s horizon scanning collaborations. Front Med. (2022) 9:1064003. doi: 10.3389/fmed.2022.1064003
14. Saritas, O, and Smith, JE. The big picture – trends, drivers, wild cards, discontinuities and weak signals. Futures. (2011) 43:292–312. doi: 10.1016/j.futures.2010.11.007
15. Amanatidou, E, Butter, M, Carabias, V, Könnölä, T, Leis, M, Saritas, O, et al. On concepts and methods in horizon scanning: lessons from initiating policy dialogues on emerging issues. Sci Public Policy. (2012) 39:208–21. doi: 10.1093/scipol/scs017
16. MHRA. (2017). The futures toolkit. Toold for futures thinking and foresight across Uk government [online]. London (Uk): Medicines and Healthcare Products Regulatory Agency. Available at: https://assets.publishing.service.gov.uk/government/uploads/system/uploads/attachment_data/file/674209/futures-toolkit-edition-1.pdf [Accessed 27/07/2023].
17. Malin Nuth, W-S, Gloria, T, and Eli, F. Barriers and facilitators to adopting horizon scanning to identify novel integrated care models: a qualitative interview study. BMJ Innov. (2022) 8:65–71. doi: 10.1136/bmjinnov-2021-000804
18. Philip, H, Li Hiu, Y, Richard, HG, Angela, B, and Marisa, P-A. Scanning the horizon: a systematic literature review of methodologies. BMJ Open. (2019) 9:e026764. doi: 10.1136/bmjopen-2018-026764.
19. Medical Device Coordination Group. (2021). Mdcg 2021–5: Guidance on standardisation for medical devices [online]. Brussels, Belgium. Available at: https://health.ec.europa.eu/system/files/2021-04/md_mdcg_2021_5_en_0.pdf (Accessed 27/07/2023).
20. Shah, JJ, Smith, SM, and Vargas-Hernandez, N. Metrics for measuring ideation effectiveness. Des Stud. (2003) 24:111–34. doi: 10.1016/S0142-694X(02)00034-0
21. Fiorineschi, L, and Rotini, F. Uses of the novelty metrics proposed by Shah et al.: what emerges from the literature? Design Sci. (2023) 9:e11. doi: 10.1017/dsj.2023.9
22. Rodríguez-Gómez, FD, Penon, O, Monferrer, D, and Rivera-Gil, P. Classification system for nanotechnology-enabled health products with both scientific and regulatory application. Front Med. (2023) 10:1212949. doi: 10.3389/fmed.2023.1212949
23. Falagas, ME, Pitsouni, EI, Malietzis, GA, and Pappas, G. Comparison of PubMed, Scopus, web of science, and Google scholar: strengths and weaknesses. FASEB J. (2008) 22:338–42. doi: 10.1096/fj.07-9492LSF
24. European Patent Office, E. (2023). European Patent Register [Online]. Munich: European Patent Office, Epo. Available at: https://register.epo.org/regviewer [Accessed 2022].
25. Medicine, N. L. O. (2000). ClinicalTrials.Gov [online]. Bethesda. Available at: https://clinicaltrials.gov/ (Accessed July 15, 2022).
26. Ruiz-Pastor, L, Chulvi, V, Mulet, E, and Royo, M. A metric for evaluating novelty and circularity as a whole in conceptual design proposals. J Clean Prod. (2022) 337:130495. doi: 10.1016/j.jclepro.2022.130495
27. Sluis-Thiescheffer, W, Bekker, T, Eggen, B, Vermeeren, A, and De Ridder, H. Measuring and comparing novelty for design solutions generated by young children through different design methods. Des Stud. (2016) 43:48–73. doi: 10.1016/j.destud.2016.01.001
28. An, W, Defaus, S, Andreu, D, and Rivera-Gil, P. In vivo sustained release of peptide vaccine mediated by dendritic mesoporous silica Nanocarriers. Front Immunol. (2021) 12:684612. doi: 10.3389/fimmu.2021.684612
29. Ganas, C, Weiß, A, Nazarenus, M, Rösler, S, Kissel, T, Rivera Gil, P, et al. Biodegradable capsules as non-viral vectors for in vitro delivery of Pei/sirna polyplexes for efficient gene silencing. J Control Release. (2014) 196:132–8. doi: 10.1016/j.jconrel.2014.10.006
30. Yusuf, A, Almotairy, ARZ, Henidi, H, Alshehri, OY, and Aldughaim, MS. Nanoparticles as drug delivery systems: a review of the implication of nanoparticles’ physicochemical properties on responses in biological systems. Polymers. (2023) 15:1596. doi: 10.3390/polym15071596
31. Sun, D, Gao, W, Hu, H, and Zhou, S. Why 90% of clinical drug development fails and how to improve it? Acta Pharm Sin B. (2022) 12:3049–62. doi: 10.1016/j.apsb.2022.02.002
32. Vargason, AM, Anselmo, AC, and Mitragotri, S. The evolution of commercial drug delivery technologies. Nat Biomed Eng. (2021) 5:951–67. doi: 10.1038/s41551-021-00698-w
33. Gao, J, Karp, JM, Langer, R, and Joshi, N. The future of drug delivery. Chem Mater. (2023) 35:359–63. doi: 10.1021/acs.chemmater.2c03003
34. Kochan, O, Boitsaniuk, S, Levkiv, M, Przystupa, K, Manashchuk, N, Pohoretska, K, et al. Emergence of Nano-dentistry as a reality of contemporary dentistry. Appl Sci. (2022) 12:2008. doi: 10.3390/app12042008
35. Ec (2017). Regulation (Eu) 2017/745. "Regulation (Eu) 2017/745 of the European Parliament and of the council of 5 April 2017 on medical devices, amending directive 2001/83/Ec, regulation (Ec) no 178/2002 and regulation (Ec) no 1223/2009 and repealing council directives 90/385/Eec and 93/42/Eec". Official Journal of the European Union (Eu). Brussels, Belgium: European Commission (Ec).
36. Seleci, M, Seleci, DA, Joncyzk, R, Stahl, F, Blume, C, and Scheper, T. Smart multifunctional nanoparticles in nanomedicine. BioNanoMaterials. (2016) 17:33–41. doi: 10.1515/bnm-2015-0030
37. Nazarenus, M, Abasolo, I, García-Aranda, N, Voccoli, V, Rejman, J, Cecchini, M, et al. Polymer capsules as a Theranostic tool for a universal in vitro screening assay—the case of lysosomal storage diseases. Part Part Syst Charact. (2015) 32:991–8. doi: 10.1002/ppsc.201500156
38. Cheng, Z, Al Zaki, A, Hui, JZ, Muzykantov, VR, and Tsourkas, A. Multifunctional nanoparticles: cost versus benefit of adding targeting and imaging capabilities. Science. (2012) 338:903–10. doi: 10.1126/science.1226338
39. Huynh, E, and Zheng, G. Engineering multifunctional nanoparticles: all-in-one versus one-for-all. Wiley Interdiscip Rev Nanomed Nanobiotechnol. (2013) 5:250–65. doi: 10.1002/wnan.1217
40. Tsoutsi, D, Sanles-Sobrido, M, Cabot, A, and Gil, PR. Common aspects influencing the translocation of Sers to biomedicine. Curr Med Chem. (2018) 25:4638–52. doi: 10.2174/0929867325666180105101841
41. Chakraborty, K, Tripathi, A, Mishra, S, Mallick, AM, and Roy, RS. Emerging concepts in designing next-generation multifunctional nanomedicine for cancer treatment. Biosci Rep. (2022) 42:BSR20212051. doi: 10.1042/BSR20212051
42. Nourizadeh, H., Doostkam, M., Seyfi, M., Nori, M., Mirzaei, H., Abbasnia, M., et al. (2021). Nano-pharmaceutical products.
43. Lu, H, Zhang, S, Wang, J, and Chen, Q. A review on polymer and lipid-based Nanocarriers and its application to Nano-Pharmaceutical and food-based systems. Front Nutr. (2021) 8:783831. doi: 10.3389/fnut.2021.783831
44. Zamora-Perez, P, Pelaz, B, Tsoutsi, D, Soliman, MG, Parak, WJ, and Rivera-Gil, P. Hyperspectral-enhanced dark field analysis of individual and collective photo-responsive gold–copper sulfide nanoparticles. Nanoscale. (2021) 13:13256–72. doi: 10.1039/D0NR08256B
45. Zamora-Perez, P, Tsoutsi, D, Xu, R, and Rivera Gil, P. Hyperspectral-enhanced dark field microscopy for single and collective nanoparticle characterization in biological environments. Materials (Basel). (2018) 11:243. doi: 10.3390/ma11020243
46. Rivera-Gil, P, Jimenez De Aberasturi, D, Wulf, V, Pelaz, B, Del Pino, P, Zhao, Y, et al. The challenge to relate the physicochemical properties of colloidal nanoparticles to their cytotoxicity. Acc Chem Res. (2013) 46:743–9. doi: 10.1021/ar300039j
47. Foulkes, R, Man, E, Thind, J, Yeung, S, Joy, A, and Hoskins, C. The regulation of nanomaterials and nanomedicines for clinical application: current and future perspectives. Biomater Sci. (2020) 8:4653–64. doi: 10.1039/D0BM00558D
48. Halwani, AA. Development of pharmaceutical nanomedicines: from the bench to the market. Pharmaceutics. (2022) 14:106. doi: 10.3390/pharmaceutics14010106
49. Hühn, D, Kantner, K, Geidel, C, Brandholt, S, De Cock, I, Soenen, SJH, et al. Polymer-coated nanoparticles interacting with proteins and cells: focusing on the sign of the net charge. ACS Nano. (2013) 7:3253–63. doi: 10.1021/nn3059295
50. Peteiro-Cartelle, J, Rodríguez-Pedreira, M, Zhang, F, Gil, PR, Del Mercato, LL, and Parak, WJ. One example on how colloidal nano- and microparticles could contribute to medicine. Nanomedicine (Lond). (2009) 4:967–79. doi: 10.2217/nnm.09.84
51. Li, W, Zamani, R, Rivera Gil, P, Pelaz, B, Ibáñez, M, Cadavid, D, et al. CuTe nanocrystals: shape and size control, plasmonic properties, and use as Sers probes and photothermal agents. J Am Chem Soc. (2013) 135:7098–101. doi: 10.1021/ja401428e
52. Zamora-Perez, P, Xiao, C, Sanles-Sobrido, M, Rovira-Esteva, M, Conesa, JJ, Mulens-Arias, V, et al. Multiphoton imaging of melanoma 3D models with plasmonic nanocapsules. Acta Biomater. (2022) 142:308–19. doi: 10.1016/j.actbio.2022.01.052
Keywords: regulatory science, horizon scanning, nanomedicine, nanotechnology-enabled health product, regulatory guideline
Citation: Rodríguez-Gómez FD, Monferrer D, Penon O and Rivera-Gil P (2024) Implementing Horizon Scanning as a tool for the strategic development of regulatory guidelines for nanotechnology-enabled health products. Front. Med. 10:1308047. doi: 10.3389/fmed.2023.1308047
Received: 05 October 2023; Accepted: 26 December 2023;
Published: 11 January 2024.
Edited by:
Jacques Joubert, University of the Western Cape, South AfricaReviewed by:
Alireza Khadem Broojerdi, World Health Organization, SwitzerlandCopyright © 2024 Rodríguez-Gómez, Monferrer, Penon and Rivera-Gil. This is an open-access article distributed under the terms of the Creative Commons Attribution License (CC BY). The use, distribution or reproduction in other forums is permitted, provided the original author(s) and the copyright owner(s) are credited and that the original publication in this journal is cited, in accordance with accepted academic practice. No use, distribution or reproduction is permitted which does not comply with these terms.
*Correspondence: Francisco D. Rodríguez-Gómez, ZnJhbmNpc2NvZGllZ28ucm9kcmlndWV6MDFAZXN0dWRpYW50LnVwZi5lZHU=; Pilar Rivera-Gil, cGlsYXIucml2ZXJhQHVwZi5lZHU=
Disclaimer: All claims expressed in this article are solely those of the authors and do not necessarily represent those of their affiliated organizations, or those of the publisher, the editors and the reviewers. Any product that may be evaluated in this article or claim that may be made by its manufacturer is not guaranteed or endorsed by the publisher.
Research integrity at Frontiers
Learn more about the work of our research integrity team to safeguard the quality of each article we publish.