- 1Division of Cardiology, Hospital de Clínicas de Porto Alegre, Porto Alegre, Brazil
- 2Postgraduate Program in Cardiology, Universidade Federal do Rio Grande do Sul, Rio de Janeiro, Brazil
- 3Ted Rogers Centre for Heart Research, Peter Munk Cardiac Centre, University of Toronto, Toronto, ON, Canada
- 4Social Responsibility, Hospital Moinhos de Vento, Porto Alegre, Brazil
- 5Hasselt University, REVAL/BIOMED, Hasselt, Belgium
- 6Health Sciences and Technologies Graduate Program, University of Brasilia (UnB), Brasilia, Brazil
- 7General Coordination, Chronic and Airborne Disease Surveillance Coordination, Health Surveillance Secretariat, Brazilian Ministry of Health, Brasilia, Brazil
- 8General Coordination, National Immunization Program, Health Surveillance Secretariat, Brazilian Ministry of Health, Brasilia, Brazil
Background: Persistent symptoms and exercise intolerance have been reported after COVID-19, even months after the acute disease. Although, the long-term impact on exercise capacity and health-related quality of life (HRQoL) is still unclear.
Research question: To assess the long-term functional capacity and HRQoL in patients hospitalized due to COVID-19.
Study design and methods: This is a prospective cohort study, conducted at two centers in Brazil, that included post-discharge COVID-19 patients and paired controls. The cohort was paired by age, sex, body mass index and comorbidities, using propensity score matching in a 1:3 ratio. Patients were eligible if signs or symptoms suggestive of COVID-19 and pulmonary involvement on chest computed tomography. All patients underwent cardiopulmonary exercise testing (CPET) and a HRQoL questionnaire (SF-36) 6 months after the COVID-19. The main outcome was the percentage of predicted peak oxygen consumption (ppVO2). Secondary outcomes included other CPET measures and HRQoL.
Results: The study sample comprised 47 post-discharge COVID-19 patients and 141 healthy controls. The mean age of COVID-19 patients was 54 ± 14 years, with 19 (40%) females, and a mean body mass index of 31 kg/m2 (SD, 6). The median follow-up was 7 months (IQR, 6.5–8.0) after hospital discharge. PpVO2 in COVID-19 patients was lower than in controls (83% vs. 95%, p = 0.002) with an effect size of 0.38 ([95%CI], 0.04–0.70). Mean peak VO2 (22 vs. 25 mL/kg/min, p = 0.04) and OUES (2,122 vs. 2,380, p = 0.027) were also reduced in the COVID-19 patients in comparison to controls. Dysfunctional breathing (DB) was present in 51%. HRQoL was significantly reduced in post COVID patients and positively correlated to peak exercise capacity.
Interpretation: Hospitalized COVID-19 patients presented, 7 months after discharge, with a reduction in functional capacity and HRQoL when compared to historical controls. HRQoL were reduced and correlated with the reduced peak VO2 in our population.
Introduction
The COVID-19 pandemic declared in March of 2020 resulted in a massive number of cases in several countries (1). SARS-Cov-2 infection overloaded healthcare systems and was responsible for over 450 million cases worldwide (2). Viral pneumonia is the hallmark of hospitalized COVID-19 patients, and, in severe forms, progress to acute respiratory distress syndrome (ARDS), the most worrying presentation with a high mortality rate and associated with long-term disabilities (3).
Experience from the previous severe acute respiratory syndrome (SARS-CoV-1) epidemic, suggests that pulmonary function at rest and exercise capacity could be profoundly impaired, either by the virus action or because of post-intensive care syndrome, but its long-term impact is unknown (4–6). Studies conducted in patients who recovered from COVID-19 have related a myriad of symptoms, including chest pain, fatigue, dyspnea, leg pain and weakness (7, 8). A case-control study conducted at 2–3 months from disease onset showed that a significant proportion of hospital discharged patients reported symptoms such as breathlessness, fatigue, depression and limited exercise capacity (9). Furthermore, cross-sectional studies performing cardiopulmonary exercise testing (CPET), the gold-standard for functional capacity assessment, elucidated some exercise limitation pathophysiological mechanisms (10, 11). Studies conducted 3 months after discharge had shown reduced functional capacity in 33 to 50% of patients post COVID-19 (12, 13). However, these studies only evaluated short-term physical impairment after COVID-19 infection, with uncertainty about causality, mechanisms of limitation and persistence of this limitation. Possible underlying mechanisms for these persistent complaints can include cardiac, pulmonary and peripheral (oxygen extraction) limitations, with either two or more combined.
The impact in health-related quality of life (HRQol) have been shown to be impaired in patients post COVID-19 (14). Countless patients affected by COVID-19 are returning to their work activities, and the real burden of this disease is still being discovered. Therefore, the aim of this study was to assess long-term functional capacity and HRQoL, among survivors of hospitalization due to COVD-19, comparing the results with those of historical controls matched by age, sex, body mass index, and comorbidities.
Methods
This is a prospective cohort study of COVID-19 patients who required hospitalization due to respiratory symptoms between June 2020 and December 2020 and paired historical controls. Participants were recruited from a previous cohort, in which adult patients (≥18 years) were eligible if admitted with signs or symptoms suggestive of COVID-19 (cough, fever, or sore throat) within 14 days of onset and hospitalized in the prior 2 days (15). All patients were hospitalized at a private hospital in Porto Alegre, southern Brazil. This private institution is the reference hospital in the care of COVID-19 cases in Porto Alegre, RS, Brazil, with 372 infirmary beds, and 113 ICU beds.
Between six and nine months after hospital discharge, patients with confirmed COVID-19 by RT-PCR and pulmonary involvement on chest computed tomography were contacted by telephone to perform a CPET and a clinical evaluation through a HRQoL questionnaire. A physician assessed the presence of persistent symptoms during the clinical evaluation. Exclusion criteria were inability to perform CPET due to musculoskeletal limitation, absence of radiologic pulmonary involvement and patient refusal. The project was submitted to the local ethics committee and complied with both the National Health Council Resolution 466/12 and the Declaration of Helsinki. All patients signed an informed consent.
Data collection
All data were collected prospectively including demographic, symptoms at admission, comorbidities, need for oxygen support, supplemental ventilatory support type, need for intensive care and length of stay. Oxygen support therapy was defined as the therapy used with the highest oxygen concentration supply and invasiveness during hospital admission. Patients were also classified according to the World Heart Organization COVID-19 severity classification: mild (symptomatic patients meeting the case definition for COVID-19 without evidence of viral pneumonia or hypoxia); moderate (adults with clinical signs of pneumonia (fever, cough, dyspnea, fast breathing) but no signs of severe pneumonia, including SpO2 ≥ 90% on room air); severe (adults with clinical signs of pneumonia plus one of the following: respiratory rate > 30 breaths/min; severe respiratory distress; or SpO2 < 90% on room air); and critical patients with acute respiratory distress syndrome (ARDS) or sepsis or septic shock (16). At the follow-up visit, patients were interviewed to assess persistent symptoms, medications in use, current exercise activity and other clinically relevant information.
Cardiopulmonary exercise testing
CPET was performed on a treadmill (General Electric T-2100, GE Healthcare, United States) with breath-by-breath gas analysis (Metalyzer 3B, Cortex, Leipzig, Germany) between January 2021 to March 2021. Symptom-limited maximal exercise testing with an individualized ramp protocol was used to yield fatigue-limited exercise duration of 8 to 12 min. Peak VO2 was determined by the higher measure of 20 s averaging of breath-by-breath values. Other prognostic variables were also measured, such as first and second ventilatory thresholds, which were defined by V-slope for first ventilatory threshold and ventilatory equivalent method to confirm first and determine second ventilatory threshold, minute ventilation-carbon dioxide output relationship (VE/VCO2 slope), oxygen uptake efficiency slope (OUES) and resting end tidal carbon dioxide tension. Maximal effort was considered when respiratory exchange ratio (RER) was equal to or above 1.05. Before each test, brief spirometry was performed before each test, to assess forced vital capacity (FVC) and forced expiratory volume in the 1st second (FEV1). Maximal voluntary ventilation (MVV) was estimated by FEV1x 37.5 (17). Peak VE was also compared as a percentage of maximal predicted using a validated equation (18). For the percentage predicted peak VO2 (ppVO2) both the Wasserman’s and Hansen algorithm and FRIEND equations were used (19). Dysfunctional breathing (DB) was defined by pattern recognition as described by previous studies (20, 21). For this classification, we considered the graphs of minute ventilation (VE) versus time, VE/VCO2 slope and respiratory rate (breaths per minute), tidal volume (mL/min) vs. VE (L/min). CPET and spirometry were performed following current guidelines for exercise testing (22).
Quality of life assessment
Short Form36 (SF-36) physical and mental health questionnaire was completed by all post COVID-19 patients. The SF-36 addresses HRQoL in eight domains (general health, physical functioning, physical role function, bodily pain, vitality, emotional role function, mental health, and social functioning) that are summarized in two dimensions: physical and mental. Scores range from worst to best (0–100). The eight different scales scores were calculated and computed. For construction of summary measures, scales were standardized using a Z-score transformation, providing both physical and mental composite scores (PCS and MCS). We used national normative data for both z-scores calculations and for comparison purpose with our sample (23).
Selection of healthy controls and pairing
Control subjects were selected from a CPET database of 4,957 test subjects without diagnosed cardiovascular or pulmonary disease, evaluated at an experienced laboratory in the Brazilian Midwest region from 2011 to 2020. CPET were mainly performed for cardiorespiratory fitness assessment and exercise prescription. Test subjects who did not fulfill ventilatory maximality criterion (RER ≥1.05) were excluded before pairing. COVID-19 patients were matched with controls by a 1:3 ratio for age, sex, BMI, hypertension and diabetes. A nearest neighbor matching method was applied with a caliper of 0.2 without replacement. After matching, included variables were compared between groups to confirm that there were no significant differences.
Statistical analysis
Continuous data were tested for normality with Shapiro–Wilk test and presented as mean (standard deviation) or median (interquartile range). Categorical data are presented as absolute count and relative frequency. Comparisons between COVID-19 and matched controls were performed by independent samples Student’s t-test and chi-square test. The effect size was calculated by dividing the mean difference between groups by the standard deviation of the population. Spearman’s rank correlation coefficient was performed to test association of HRQoL and CPET data. Non-linear regression with curve fitting was used to examine the relationship between peak VO2 and PCS of HRQol. We used a generalized linear model to estimate the association of COVID-19 infection in comparison to healthy controls for the ppVO2. An adjusted model including age, sex, height, and weight was also performed. This study used a convenience cohort of patients. We performed a post-hoc power calculation for the observed differences of the ppVO2 among COVID-19 and healthy controls resulting in a power of 89.94% for an alpha value of 5%. Significance was accepted at p < 0.05 for all tests. Data were analyzed in SPSS, Version 25.0 for Windows (SPSS Inc., Chicago, IL, United States) and R 4.1.1 statistical software (R: The R Project for Statistical Computing, https://www.r-project.org).
Results
From 110 screened patients with confirmed SARS-CoV-2 infection, 63 were excluded due to an absence of radiological abnormalities at time of admission or did not consent to perform CPET. The flowchart of the study is shown in Figure 1. Our sample comprised 47 previously hospitalized COVID-19 patients with a mean age of 54 years (standard deviation [SD], 14), 19 (40%) females, 24 (51%) with hypertension and 12 (26%) with diabetes. There were no significant differences found in baseline characteristics between cases and the 141 matched controls. COVID-19 patients required supplementary oxygen in 26 (55%) cases, but only 3 (6%) required high-flow nasal cannula, 1 (2%) Bilevel, and 2 (4%) mechanical ventilation. Patients were hospitalized for a median of 7 days (interquartile range [IQR], 4–10) and 5 (11%) required ICU admission. Twenty-six (55%) subjects reported persistent symptoms, being fatigue (46%), dyspnea (38%), and leg pain/weakness (21%) the most common. Table 1 summarizes demographic and clinical data from COVID-19 patients and healthy controls.
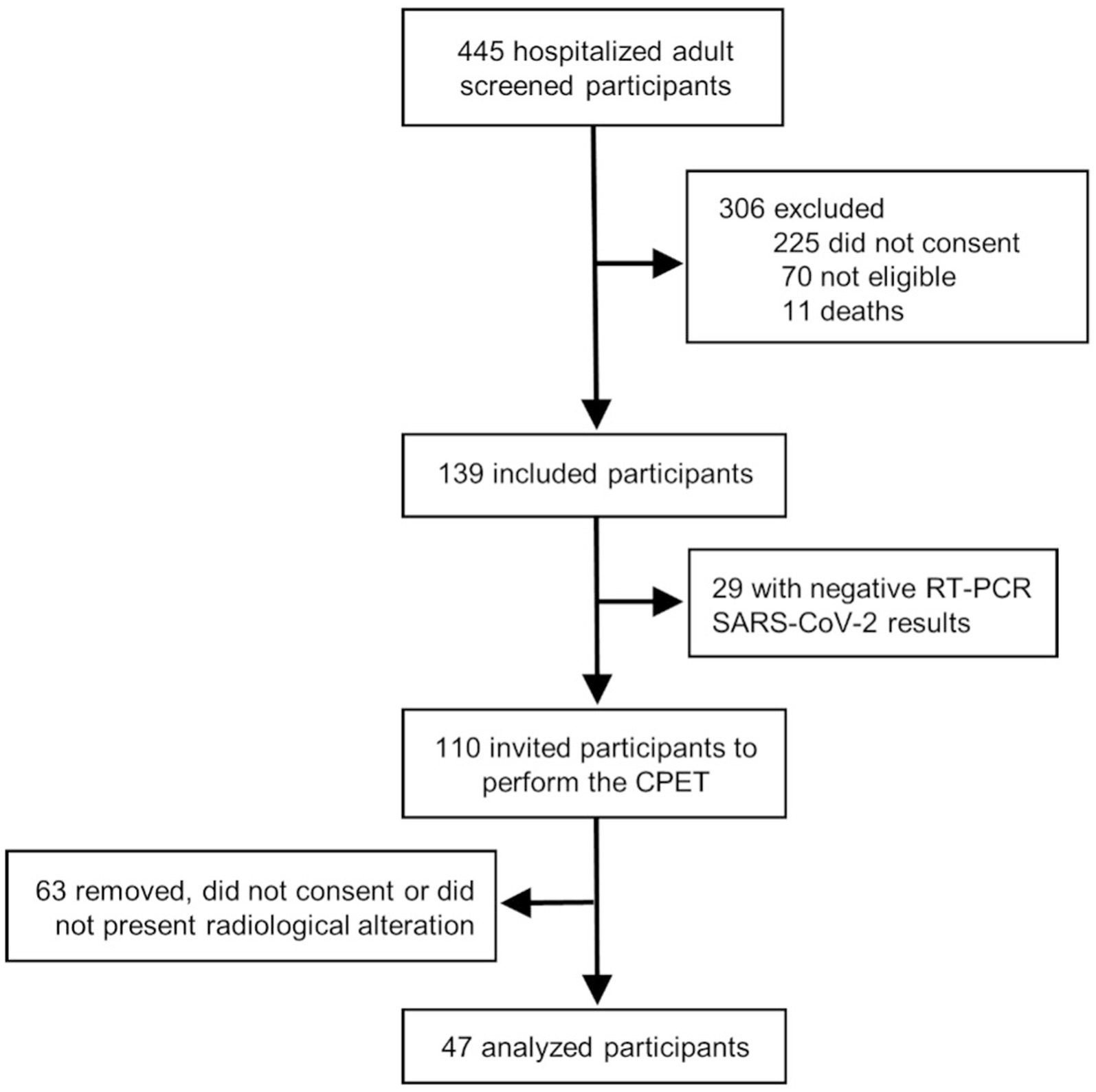
Figure 1. Study design. We screened 445 hospitalized patients diagnosed with COVID-19 infection and selected for eligibility when a positive polymerase chain reaction test and signs of lung involvement evaluated by computed tomography chest imaging. Of the 110 eligible patients, 47 accepted the invitation to perform a cardiopulmonary exercise test study 6 months after hospital discharge.
Exercise capacity and CPET results
Table 2 and Figure 2 summarize the CPET variables compared between COVID-19 and controls. The median time from hospital discharge to CPET was 7 months (IQR, 6.5–8). The mean FEV1 was 3.34 L [SD, 0.7], and the mean FVC was 4.3 L [SD, 0.9]. Among the patients, 1 presented with a restrictive pattern, while 2 exhibited an obstructive pattern during spirometry. COVID-19 patients showed lower mean ppVO2 by the Wasserman and Hansen algorithm (83% [SD, 15] vs. 95% [SD, 35]; p = 0.002), and by the FRIEND equation (81% [SD, 18] vs. 88% [SD, 18]; p = 0.039). Peak VO2 (22.5 [SD, 6] vs. 25.0 [SD, 7] mL/kg/min; p = 0.048), VO2@AT (14.4 [SD, 4] vs. 12.8 [SD, 3] mL/kg/min; p = 0.007), and OUES (2,122 [SD, 611] vs. 2,380 [SD, 860]; p = 0.02) were also impaired in COVID-19 patients when compared to healthy controls. The greatest effect size was observed for VO2@AT, ppVO2, peak VO2 and OUES, respectively. Peak VE and percent-predicted VE showed lower values for COVID-19 patients (76 [SD, 23] vs. 84 [SD, 27], p = 0.08 and 103 [SD, 40] vs. 93 [SD, 39]; p = 0.116, respectively), but without statistical significance. Breathing reserve was normal in all post-COVID patients, with the average peak VE/MVV relation of 0.63 (SD, 0.2).
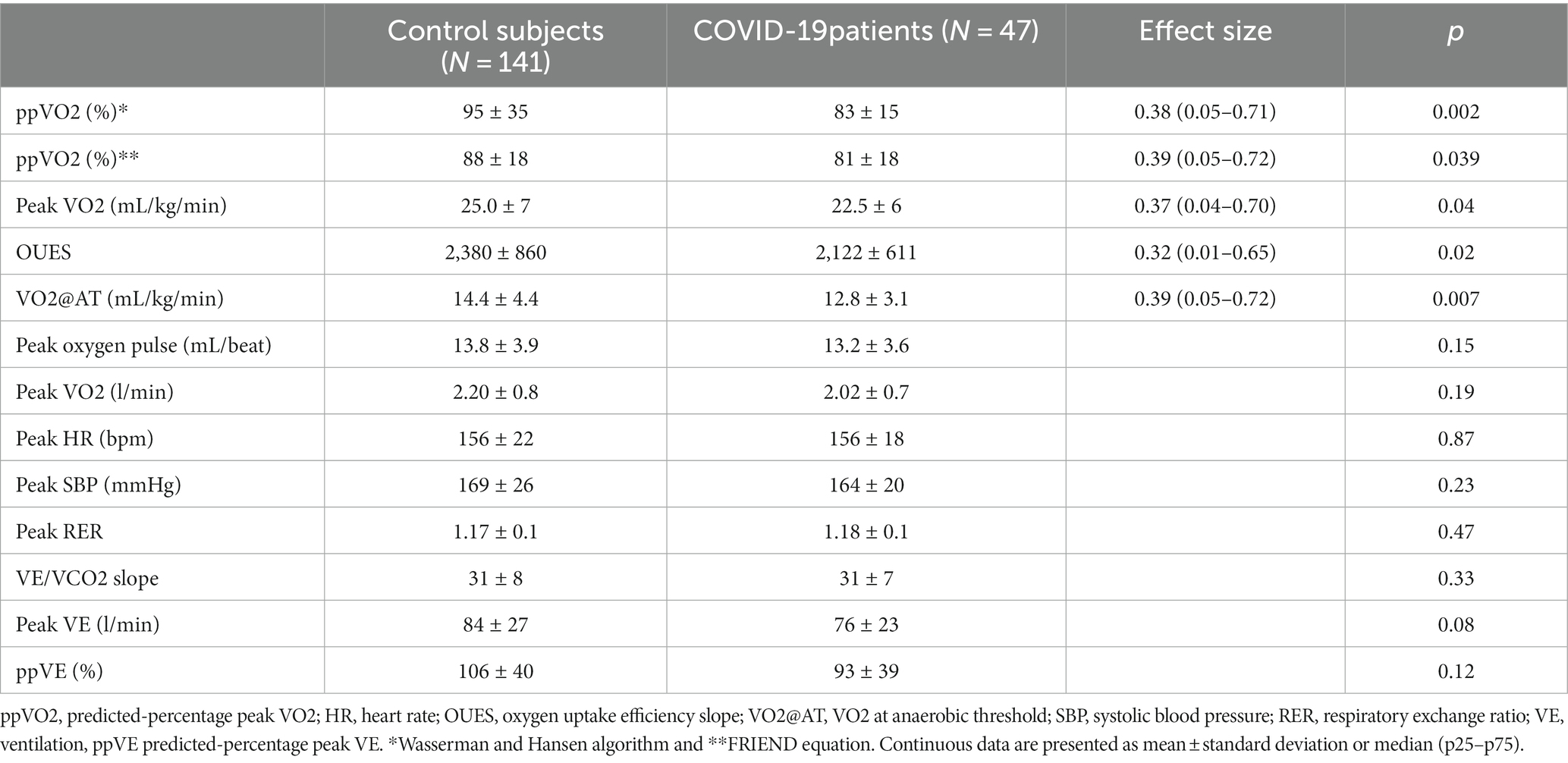
Table 2. Comparison of cardiopulmonary exercise testing between healthy controls and hospitalized COVID-19 patients.
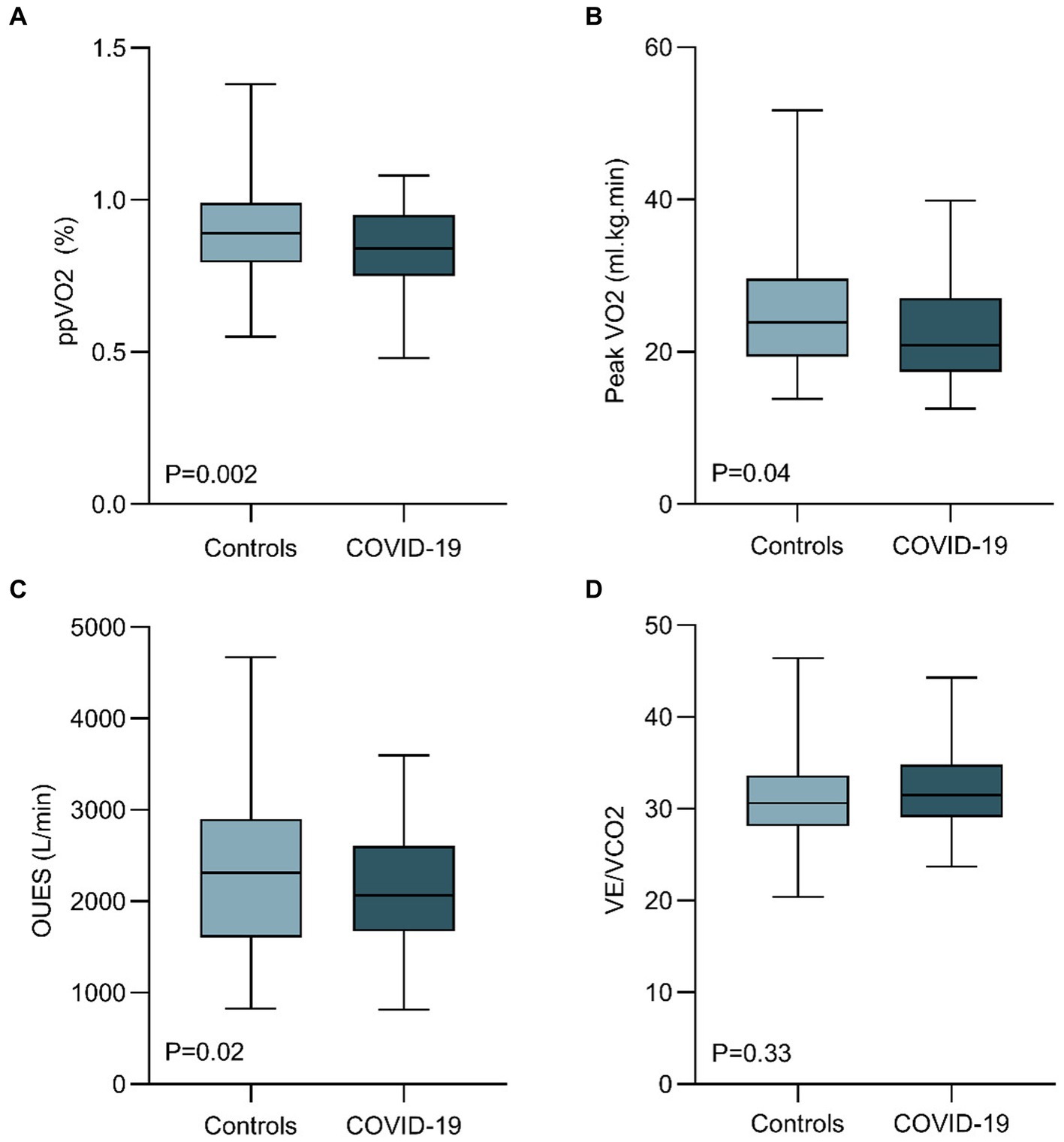
Figure 2. Comparison of cardiopulmonary exercise test parameters between healthy controls and hospitalized COVID-19 patients 6 months after discharge. COVID-19 patients 6 months after hospital discharge showed a reduced ppVO2 (calculated by the Wasserman and Hansen Algorithm), peak VO2, and OUES. VE/VCO2 were similar between cohorts. (OUES, oxygen uptake efficiency slope; ppVO2, predicted-percentage peak VO2; VE, ventilation).
When considering those participants with ppVO2 less than 80% of predicted, 21 (45%) COVID-19 patients had values below this threshold, in comparison to only 12 (8.5%) of healthy controls (p = 0.01). When using ERS algorithm (24) for determining causes of exercise limitation of these 21 COVID-19 patients, 12 (57%) showed findings consistent with cardiocirculatory limitation and nine subjects (43%), findings suggesting peripheral muscle limitation. It is noteworthy that none of the patients showed reduced breathing reserve or signs of pulmonary limitation.
Dysfunctional breathing was prevalent among COVID-19 patients (51%). Persistence of symptoms (dyspnea, fatigue, leg weakness) was associated with the DB ventilatory pattern (OR, 3.8; 95% CI, 1.3–12.1). DB was more common in patients who had lower ppVO2 (78% vs. 89%, p = 0.012) and among those with peripheral muscle limitation than cardiocirculatory or normal findings (89% vs. 66% vs.31%, respectively, p = 0.005). The relationship between symptoms, DB and reduced ppVO2 is displayed in Figure 3A.
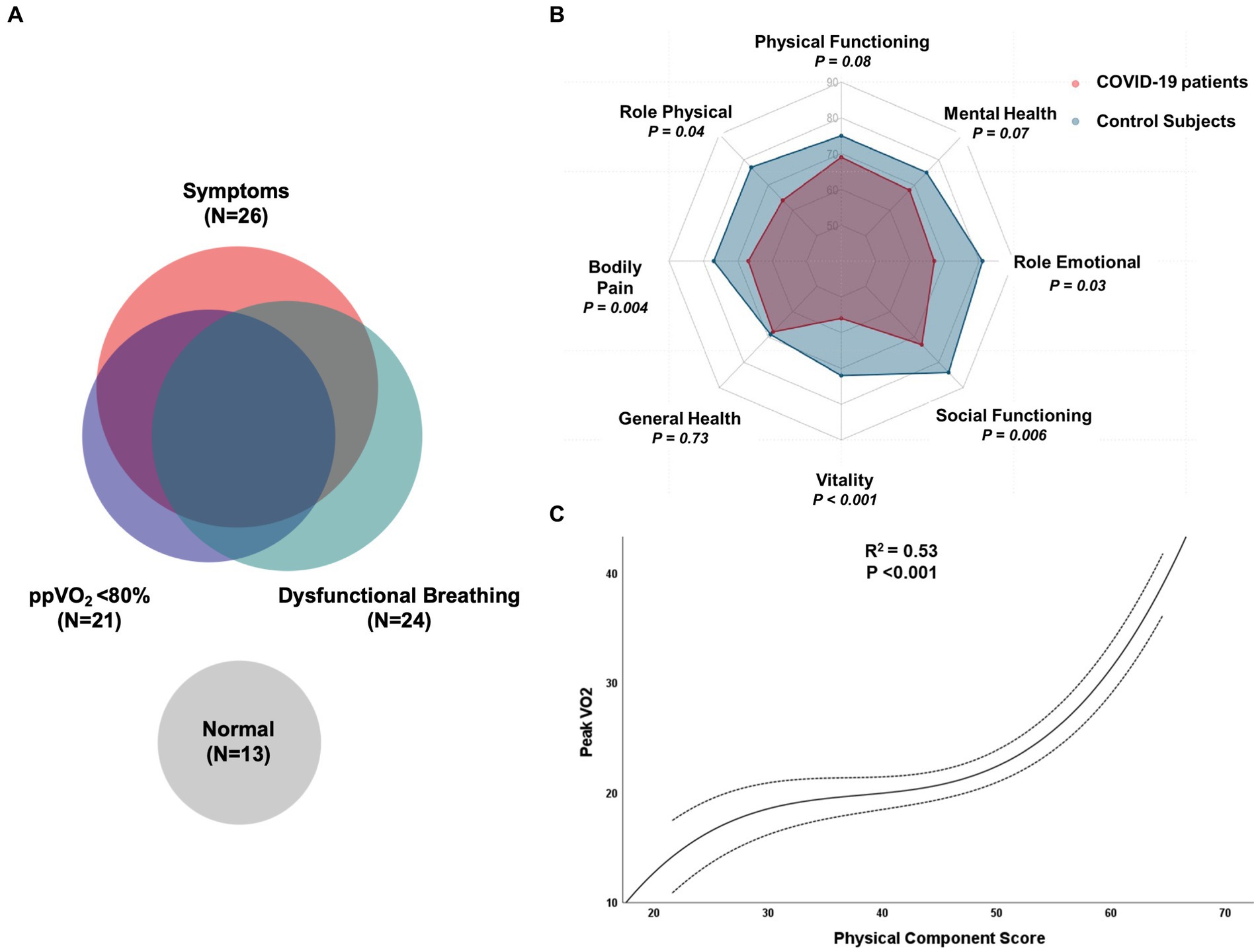
Figure 3. (A) Venn diagram illustrating the relationship between symptoms, reduced percent-predicted peak oxygen consumption, dysfunctional breathing and normal evaluation in COVID-19 patients. (B) Evaluation of quality-of-life domains of SF-36 between healthy controls and hospitalized COVID-19 patients six months after discharge; (C) Cubic regression between peak oxygen consumption during CPET and physical component score of HRQol in COVID-19 patients.
Predictors of decrease predicted peak VO2
We subsequently performed an analysis to evaluate the impact of COVID-19 in the observed ppVO2 (by Wasserman and Hansen algorithm) in comparison to matched controls (Table 3). COVID-19 patients had a reduced ppVO2 with an unadjusted odds ratio (OR) of 0.89 (95%CI, 0.82–0.95; p = 0.002) and an adjusted OR of 0.88 (95%CI, 0.82–0.95, p = 0.002). We then sought to evaluate which characteristics were associated with the ppVO2 in hospitalized COVID-19 patients after discharge (Table 3). The presence of coronary artery disease (OR, 0.83; 95%CI, 0.76–0.89; p < 0.001), the use of Bilevel (OR, 0.83; 95%CI, 0.79–0.88; p < 0.001), mechanical ventilation (OR, 0.88; 95%CI, 0.79–0.98; p = 0.02), and in-hospital length of stay (OR, 0.99; 95%CI, 0.99–0.99; p = 0.001) were associated with lower ppVO2.
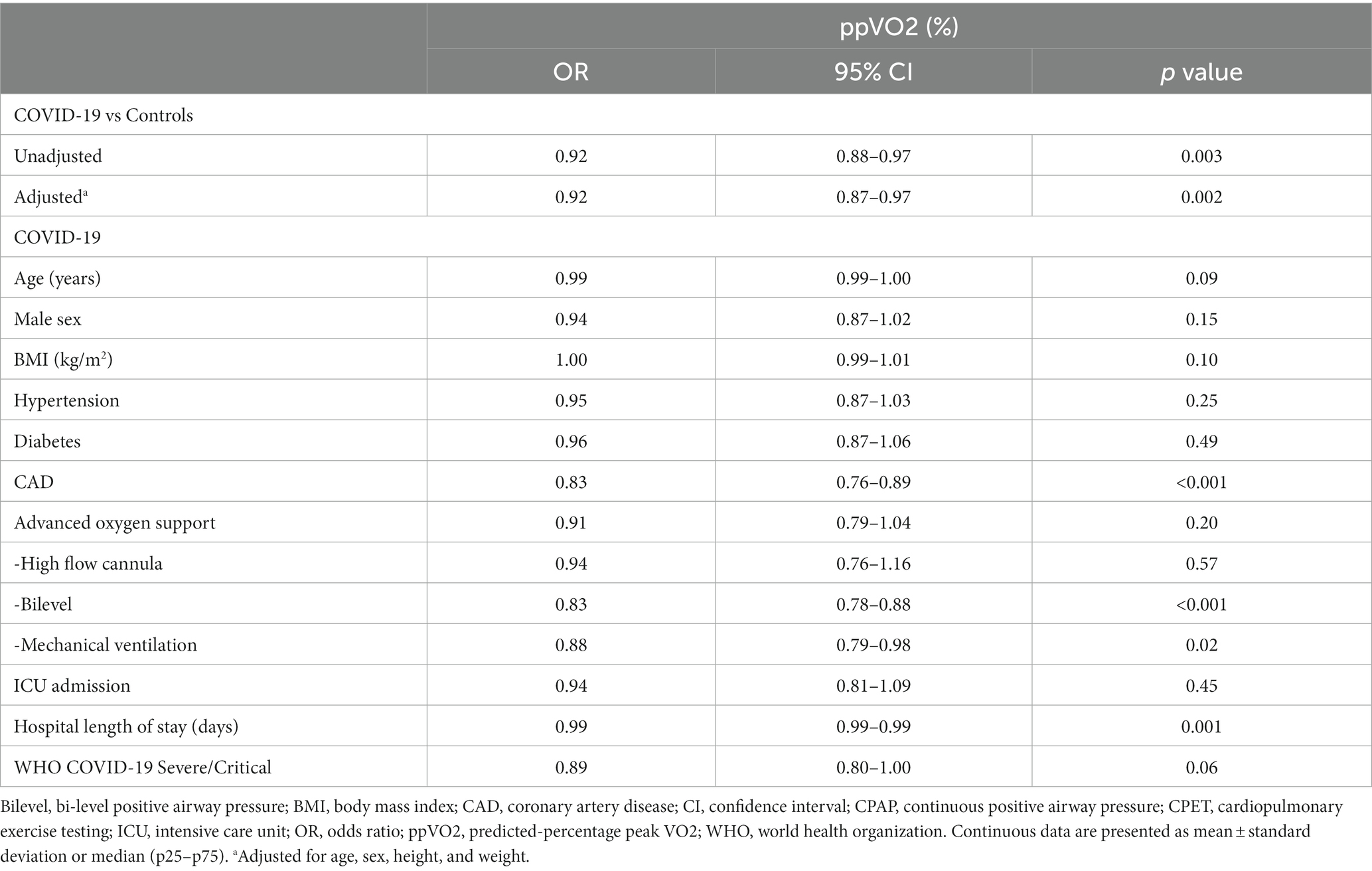
Table 3. Crude and adjusted analysis of variables related to percent-predicted peak VO2 after COVID-19 hospitalization.
Quality of life assessment
Quality of life measurements were compared to national normative data stratified by age and sex. Both physical (45 vs. 49; p = 0.01) and mental (47 vs. 51; p = 0.04) composite mean scores were significantly reduced in COVID-19 patients. Regarding the domains of physical and mental health, vitality (55 vs. 71; p < 0.001), bodily pain (66 vs. 77, p = 0.004), role physical (64 vs. 76, p = 0.04), role emotional (68 vs. 81, p = 0.03) and social functioning (72 vs. 84, p = 0.006) were significantly reduced in post COVID-19 subjects. Physical functioning (69 vs. 75, p = 0.08) and mental health (69 vs. 74, p = 0.07) also were reduced in post-COVID-19 patients, but without statistical significancy. Interestingly, the global health perception of patients was not reduced when compared to controls (68 vs. 69, p = 0.73). HRQol results are summarized in Figure 3B.
Physical composite score (Spearman’s ρ = 0.654; p < 0.001), functional capacity (ρ = 0.649; p < 0.001) and bodily pain (ρ = 0.637; p < 0.001) showed a significant, moderate correlation with peak VO2 in our sample. The relationship between peak VO2 and PCS was best described as a third-degree polynomial, presenting a moderate coefficient of determination (R2 = 0.53; p < 0.001) as shown in Figure 3C.
Discussion
Our study shows that hospitalized COVID-19 patients, even after more than 6 months post-discharge, can still demonstrate reduced functional capacity and HRQoL compared to matched controls. Several CPET prognostic markers, physical and mental aspects of HRQoL were also significantly reduced 6 months after hospital discharge in COVID-19 patients, demonstrating the long-term impact of the disease. Moreover, more than half of the patients has persistent symptoms at 6 months follow-up, increasing the burden of disease.
Our results are consistent with those found in previous studies evaluating patients in the short-term after COVID-19 infection (12, 13). Skjorten and colleagues, using a treadmill, found one-third of patients with a ppVO2 less than 80%, additionally, 15% percent of these patients had shown reduced ventilatory efficiency (12). Clavario et al. reported one-third of patients with a reduced peak VO2 3 months post-discharge on cycle ergometer CPET, mostly due to muscular impairment (13). Many recent data suggest that peripheral factors are incriminated in persistent functional impairment in post COVID-19 patients. A small study was conducted in 10 patients without cardiopulmonary disease who recovered from COVID-19. Patients were investigated with invasive cardiopulmonary exercise testing (iCPET) and compared to 10 age- and sex-matched controls (25). The reduction in peak VO2 was associated with impaired systemic oxygen extraction, depicting a peripheral rather than a central cardiac limitation.
Functional impairment after COVID-19 infection remains a major concern. We demonstrated that after 6 months of discharge, COVID-19 patients had a reduction in ppVO2 and peak VO2 when compared to matched controls. The observed higher peak VO2 in males was not confirmed by the ppVO2, suggesting an absence of sex-related post-COVID-19 hospitalization functional impairment. Interestingly, we did not find any exercise limitation due to pulmonary gas exchange or ventilatory mechanics. In keeping with previous reports, cardiocirculatory limitation was the predominant deficit encountered in our study. A recent meta-analysis explored the utility of CPET to evaluate long COVID-19 symptoms in adults, showing that exercise capacity was reduced in these patients and that CPET may provide insight into the mechanisms for this impairment (26).
Several patients after COVID-19 had presented a rapid and irregular breathing pattern consistent with DB, which is characterized sometimes by rapid shallow breaths or other erratic ventilatory patterns (20, 21). It was associated with persistent symptoms such as dyspnea and fatigue, and with a reduced ppVO2 as well. We have found a similar prevalence of DB when comparing our data to other studies, also showing a positive correlation of this ventilatory abnormality with symptoms (20, 27). Nevertheless, identification of DB is subjective and requires pattern recognition, without any strict criteria. The development of quantitative methods would help us to diagnose this entity.
Notably, the requirement of Bilevel support, mechanical ventilation, ICU admission, hospital length of stay, and COVID-19 severity were all associated with a reduced ppVO2. The high number of COVID-19 infected patients will certainly impact the demand for dyspnea evaluation and referrals for rehabilitation soon. We should be aware that symptoms persist even 6 months after hospital discharge in COVID-19 patients. A preemptive approach towards rehabilitation could be beneficial, especially in those more likely to be impacted such as in those with severe disease presentations. Physical rehabilitation after discharge could improve these symptoms, especially in patients with a severe initial COVID-19 presentation, but the efficacy of this intervention is yet to be established in this scenario (27).
Mental and physical aspects of HRQoL were significantly reduced in COVID-19 patients 6 months after discharge. A reduced mental aspect of HRQoL is consistent with the findings of sleep disturbances, depression, anxiety, and cognitive impairment as reported in a systematic review (28). Of note, the comparison of HRQoL scores was adjusted by age and sex according to national normative data, which strengthens the evidence for this impairment when compared to the general population. Both peak VO2 and ppVO2 were positively correlated with several aspects of HRQoL, not only physical, but also social and mental. It provides a better understanding of persistent impairment after moderate to severe COVID-19: there is a pathophysiological basis for these symptoms associated with a documented reduction in exercise capacity.
Our study has several limitations. Although we used a 3:1 control ratio, our study cannot support that the late exercise impairment observed in COVID-19 patients is related exclusively to this etiology. Comparing CPET parameters after hospital discharge with a population affected by another viral pneumonia could better clarify if COVID-19 is responsible for these symptoms or they are merely due to the hospital stay. One of the variables most affected in post COVID subjects is the diffusion capacity, which was not measured in our study. Recruitment to the study is another limitation. The stigma related to COVID-19 infection and the environmental safety for a CPET study were barriers to patient recruitment. Although the selection was not based on the presence of symptoms, patients more likely to present dyspnea or fatigue could be more prone to accept the research invitation. Our inclusion criteria limited the results to hospitalized patients with pulmonary involvement, so caution should be taken in extrapolating these findings to less severe patients.
Conclusion
Hospitalized COVID-19 patients showed decreased exercise capacity after 6 months from discharge related mainly to cardiocirculatory impairment and peripheral muscle limitation. Dysfunctional breathing was common and associated with persistent symptoms. Both physical and mental quality of life domains were reduced in these patients. The requirement of higher level of oxygen support, intensive care admission, longer hospital stay, and COVID-19 severity were the main predictors of reduced peak VO2. Our results highlight the health support required by these patients even after more than 6 months from hospital discharge.
Data availability statement
The raw data supporting the conclusions of this article will be made available by the authors, without undue reservation.
Ethics statement
The studies involving humans were approved by Comitê de Ética do Hospital de Clínicas de Porto Alegre. The studies were conducted in accordance with the local legislation and institutional requirements. The participants provided their written informed consent to participate in this study.
Author contributions
AS: Writing – original draft, Writing – review & editing. FS: Writing – original draft, Writing – review & editing. MSa: Writing – original draft, Writing – review & editing. DB: Writing – review & editing. MM: Writing – original draft. JM: Writing – review & editing. GJ: Writing – review & editing. IS: Writing – review & editing. GZ: Writing – review & editing. MT: Writing – review & editing. MC: Writing – review & editing. MSc: Writing – original draft. RS: Writing – review & editing. RR: Writing – review & editing.
Funding
The author(s) declare financial support was received for the research, authorship, and/or publication of this article. This work was supported by the Brazilian Ministry of Health, through the Institutional Development Program of the Brazilian National Health System (PROADI-SUS) in collaboration with Hospital Moinhos de Vento. Financial partial support for this work was also provided by public research grants and scholarships from Conselho Nacional de Desenvolvimento Científico e Tecnológico (CNPq) and Coordenação de Aperfeiçoamento de Pessoal de Nível Superior (CAPES). The authors also received scholarships from the Special Research Fund (BOF) from Hasselt University/Belgium (Number: BOF23DOCBL10 and BOF23KV10). This study was partially funded by the Coordenação de Aperfeiçoamento de Pessoal de Nivel Superior (CAPES) - Brasil - Finance Code 001. This study was partially funded by Hospital de Clínicas de Porto Alegre (Fundo de Incentivo à Pesquisa e Eventos; FIPE/HCPA).
Acknowledgments
We thank the assistance team, laboratory team, and local staff from Hospital Moinhos de Vento. We also thank the support from the coordinators and the staff of Brazilian National Immunization Program and the Coordination of Surveillance of Respiratory Transmitted Diseases and Chronic Conditions from the Brazilian Ministry of Health. We thank to the COVIDa study group staff for the support. Amanda Paz Santos, Caroline Nespolo de David, Luciane Beatriz Kern, Fernanda Lutz Tolves, Jaina da Costa Pereira, Shirlei Villanova Ribeiro, Ingrid Rodrigues Fernandes, Fernanda Hammes Varela, Márcia Polese-Bonatto, and Thais Raupp Azevedo.
Conflict of interest
The authors declare that the research was conducted in the absence of any commercial or financial relationships that could be construed as a potential conflict of interest.
Publisher’s note
All claims expressed in this article are solely those of the authors and do not necessarily represent those of their affiliated organizations, or those of the publisher, the editors and the reviewers. Any product that may be evaluated in this article, or claim that may be made by its manufacturer, is not guaranteed or endorsed by the publisher.
References
1. Murthy, S, Gomersall, CD, and Fowler, RA. Care for critically ill patients with COVID-19. JAMA. (2020) 323:1499–500. doi: 10.1001/jama.2020.3633
2. Berlin, DA, Gulick, RM, and Martinez, FJ. Severe COVID-19. N Engl J Med. (2020) 383:2451–60. doi: 10.1056/NEJMcp2009575
3. Marini, JJ, and Gattinoni, L. Management of COVID-19 respiratory distress. JAMA. (2020) 323:2329–30. doi: 10.1001/jama.2020.6825
4. Lau, HMC, Lee, EWC, Wong, CNC, Ng, GYF, and Jones, AYM. The impact of severe acute respiratory syndrome on the physical profile and quality of life. Arch Phys Med Rehabil. (2005) 86:1134–40. doi: 10.1016/j.apmr.2004.09.025
5. Lau, HMC, Ng, GYF, Jones, AYM, Lee, EWC, Siu, EHK, and Hui, DSC. A randomised controlled trial of the effectiveness of an exercise training program in patients recovering from severe acute respiratory syndrome. Aust J Physiother. (2005) 51:213–9. doi: 10.1016/s0004-9514(05)70002-7
6. Hui, DS, Wong, KT, Ko, FW, Tam, LS, Chan, DP, and Woo, J. The 1-year impact of severe acute respiratory syndrome on pulmonary function, exercise capacity, and quality of life in a cohort of survivors. Chest. (2005) 128:2247–61. doi: 10.1378/chest.128.4.2247
7. Goërtz, YMJ, Van Herck, M, Delbressine, J, et al. Persistent symptoms 3 months after a SARS-CoV2 infection: the post-COVID-19 syndrome? ERJ Open Res. (2020) 6:542. doi: 10.1183/23120541.00542-2020
8. Carfì, A, and Bernabei, R. Against COVID-19 post-acute care study group. Persistent symptoms in patients after acute COVID-19. JAMA. (2020) 324:603–5. doi: 10.1001/jama.2020.12603
9. Raman, B, Cassar, MP, Tunnicliffe, EM, Filippini, N, Griffanti, L, and Alfaro-Almagro, F. Medium-term effects of SARS-CoV-2 infection on multiple vital organs, exercise capacity, cognition, quality of life and mental health, post-hospital discharge. E Clin Med. (2021) 31:100683. doi: 10.1016/j.eclinm.2020.100683
10. Guenette, JA, Chin, RC, Cory, JM, Webb, KA, and O'Donnell, DE. Inspiratory capacity during exercise: measurement, analysis, and interpretation. Pulm Med. (2013) 2013:1–13. doi: 10.1155/2013/956081
11. Vogiatzis, I, Zakynthinos, G, and Andrianopoulos, V. Mechanisms of physical activity limitation in chronic lung diseases. Pulm Med. (2012) 2012:1–11. doi: 10.1155/2012/634761
12. Skjørten, I, Ankerstjerne, OAW, Trebinjac, D, Brønstad, E, Rasch-Halvorsen, Ø, and Einvik, G. Cardiopulmonary exercise capacity and limitations 3 months after COVID-19 hospitalisation. Eur Respir J. (2021) 58:2100996. doi: 10.1183/13993003.00996-2021
13. Clavario, P, De Marzo, V, and Lotti, R. Cardiopulmonary exercise testing in COVID-19 patients at 3 months follow-up. Int J Cardiol. (2021) 340:113–8. doi: 10.1016/j.ijcard.2021.07.033
14. Garrigues, E, Janvier, P, Kherabi, Y, le Bot, A, Hamon, A, and Gouze, H. Post-discharge persistent symptoms and health-related quality of life after hospitalization for COVID-19. J Infect. (2020) 81:e4–6. doi: 10.1016/j.jinf.2020.08.029
15. David, CN, Varela, FH, Sartor, IT, Polese-Bonatto, M, Fernandes, IR, and Zavaglia, GO. Diagnostic accuracy of a SARS-CoV-2 rapid test and optimal time to seropositivity according to the onset of symptoms. Cad Saude Publica. (2021) 38:9921. doi: 10.1590/0102-311x00069921
16. Williams, J, et al. Predicting maximal exercise ventilation in patients with chronic obstructive pulmonary disease. Chest. (1987) 92:253–9. doi: 10.1378/chest.92.2.253
17. Kaminsky, LA, Harber, MP, Imboden, MT, Arena, R, and Myers, J. Peak ventilation reference standards from exercise testing: from the FRIEND registry. Med Sci Sports Exerc. (2018) 50:2603–8. doi: 10.1249/MSS.0000000000001740
18. Myers, J, Kaminsky, LA, Lima, R, Christle, JW, Ashley, E, and Arena, R. A reference equation for Normal standards for VO 2 max: analysis from the fitness registry and the importance of exercise National Database (FRIEND registry). Prog Cardiovasc Dis. (2017) 60:21–9. doi: 10.1016/j.pcad.2017.03.002
19. Mancini, D., Brunjes, D., and Lala, A., Use of cardiopulmonary stress testing for patients with unexplained dyspnea post-coronavirus disease. J am Coll Cardiol, (2021); 9: 927–937, doi: 10.1016/j.jchf.2021.10.002
20. Motiejunaite, J, Balagny, P, Arnoult, F, et al. Hyperventilation: a possible explanation for long-lasting exercise intolerance in mild COVID-19 survivors? Front Physiol. 11:614590. doi: 10.3389/fphys.2020.614590
21. ATS/ACCP statement on cardiopulmonary exercise testing. Am J Respir Crit Care Med. 167:211–77. doi: 10.1164/rccm.167.2.211
22. Laguardia, J, Campos, M R, Travassos, CC, Najar, AL, Anjos,, and Vasconcellos, MM Dados normativos brasileiros do questionário Short Form-36 versão 2. Rev Bras Epidemiol (2013). doi: 10.1590/S1415-790X2013000400009, 16, 889–897
23. Radtke, T, Crook, S, Kaltsakas, G, Louvaris, Z, Berton, D, and Urquhart, DS. ERS statement on standardisation of cardiopulmonary exercise testing in chronic lung diseases. Eur. Resp. Rev. (2019) 28:180101. doi: 10.1183/16000617.0101-2018
24. Singh, I, Joseph, P, Heerdt, PM, Cullinan, M, Lutchmansingh, DD, and Gulati, M. Persistent exertional intolerance after COVID-19: insights from invasive cardiopulmonary exercise testing. Chest. (2022) 161:54–63. doi: 10.1016/j.chest.2021.08.010
25. Durstenfeld, MS, Sun, K, Tahir, P, Peluso, MJ, Deeks, SG, and Aras, MA. Use of cardiopulmonary exercise testing to evaluate Long COVID-19 symptoms in adults: a systematic review and Meta-analysis. JAMA Netw Open. (2022) 5:e2236057. doi: 10.1001/jamanetworkopen.2022.36057
26. Brito, GM, Prado, DML, Rezende, DA, de Matos, LDNJ, Loturco, I, and Vieira, MLC. The utility of cardiopulmonary exercise testing in athletes and physically active individuals with or without persistent symptoms after COVID-19. Front Med. (2023) 10:1128414. doi: 10.3389/fmed.2023.1128414
27. Vries, H, Kemps, H, Engen-Verheul, MM, et al. Cardiac rehabilitation and survival in a large representative community cohort of Dutch patients. Eur Heart J. (2015) 36:1519–28. doi: 10.1093/eurheartj/ehv111
Keywords: CPET cardiopulmonary exercise testing, COVID-19, long COVID, HRQOL, functional capacity
Citation: da Silveira AD, Scolari FL, Saadi MP, Brahmbhatt DH, Milani M, Milani JGPO, Junior GC, Sartor ITS, Zavaglia GO, Tonini ML, da Costa MSC, Scotta MC, Stein RT and Rosa RG (2024) Long-term reduced functional capacity and quality of life in hospitalized COVID-19 patients. Front. Med. 10:1289454. doi: 10.3389/fmed.2023.1289454
Edited by:
Eric S. Hall, Nemours Foundation, United StatesReviewed by:
Luis V. F. Oliveira, Evangelical University of Goiás – UniEVANGÉLICA, BrazilRodrigo Torres-Castro, University of Chile, Chile
Copyright © 2024 da Silveira, Scolari, Saadi, Brahmbhatt, Milani, Milani, Junior, Sartor, Zavaglia, Tonini, da Costa, Scotta, Stein and Rosa. This is an open-access article distributed under the terms of the Creative Commons Attribution License (CC BY). The use, distribution or reproduction in other forums is permitted, provided the original author(s) and the copyright owner(s) are credited and that the original publication in this journal is cited, in accordance with accepted academic practice. No use, distribution or reproduction is permitted which does not comply with these terms.
*Correspondence: Anderson Donelli da Silveira, adsilveira@hcpa.edu.br