- Heart and Vascular Center, Semmelweis University, Budapest, Hungary
Cardiogenic shock (CS) in acute coronary syndrome (ACS) is a critical disease with high mortality rates requiring complex treatment to maximize patient survival chances. Emergent coronary revascularization along with circulatory support are keys to saving lives. Mechanical circulatory support may be instigated in severe, yet still reversible instances. Of these, the peripheral veno-arterial extracorporeal membrane oxygenator (pVA-ECMO) is the most widely used system for both circulatory and respiratory support. The aim of our work is to provide a review of our current understanding of the pVA-ECMO when used in the catheterization laboratory in a CS ACS setting. We detail the workings of a Shock Team: pVA-ECMO specifics, circumstances, and timing of implantations and discuss possible complications. We place emphasis on how to select the appropriate patients for potential pVA-ECMO support and what characteristics and parameters need to be assessed. A detailed, stepwise implantation algorithm indicating crucial steps is also featured for practitioners in the catheter laboratory. To provide an overall aspect of pVA-ECMO use in CS ACS we further gave pointers including relevant human resource, infrastructure, and consumables management to build an effective Shock Team to treat CS ACS via the pVA-ECMO method.
1. Introduction
Cardiogenic shock (CS) is defined as generalized hypoperfusion and organ hypoxia due to primary cardiac dysfunction, which, if left untreated, leads to multiple organ failure and eventual death. A decrease in systolic blood pressure (<90 mmHg), and cardiac index (<2.2 l/min/m2), as well as an increase in pulmonary capillary wedge pressure (PCWP ≥ 15 mmHg) are parametrical values indicating the presence or onset of CS (1–4).
Acute coronary syndrome (ACS) presents as an emergency medical condition requiring urgent coronary revascularization, usually by means of percutaneous coronary intervention (PCI) performed at specialized cardiovascular centers. In cases of severe ACS, clinical manifestations of shock (CS ACS) may develop in 3–13% of patients due to acute left ventricular failure, significantly increasing the risk of mortality by over tenfold (1, 4–6).
The pathophysiological progression toward CS, especially CS ACS is frequently described as a complex spiral, culminating in an irreversible state (4) (Figure 1). Consequently, the primary objective revolves around the timely identification of the condition prompting immediate and simultaneous treatment of the underlying ACS and circulatory failure in its early stages. In approximately half of CS ACS cases, comprehensive and accurate medical and interventional treatment can effectively halt the deterioration of the condition and provide a cure for the patients. However, if CS worsens and potentially or clinically proves refractory to lesser treatment methods, the utilization of extracorporeal life support (ECLS) devices becomes a viable option in dedicated cases (4, 7, 8).
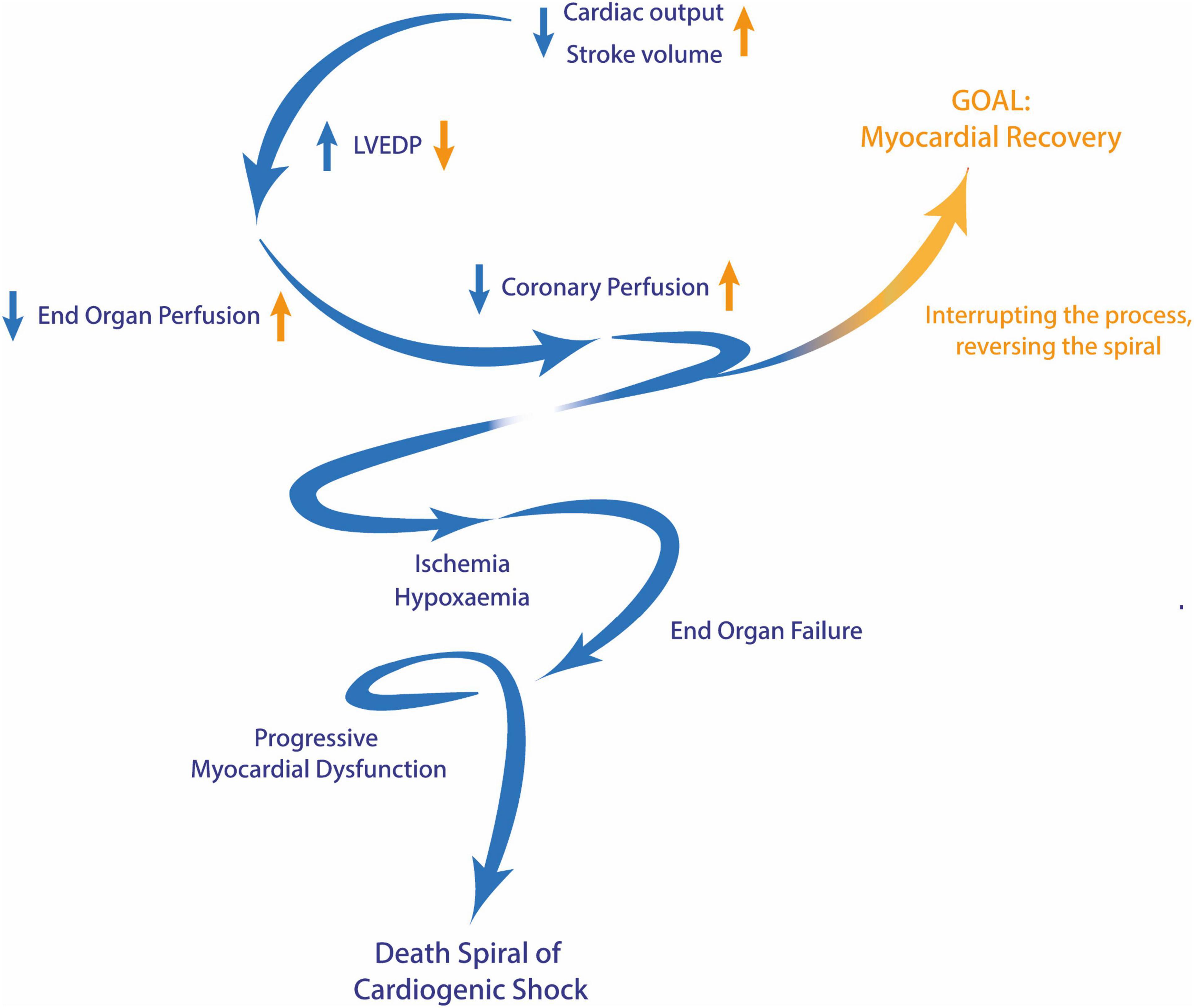
Figure 1. The death spiral of cardiogenic shock. Blue arrows represent the changes observed during the progression of cardiogenic shock, while yellow arrows depict the opposing changes achieved through effective and timely intervention. LVEDP, left ventricular end-diastolic pressure.
The use of ECLS devices has been a longstanding practice in the field of cardiology, dating back to the 1960s (4). Among the array of available options, veno-arterial (VA) extracorporeal membrane oxygenator (ECMO) may be the optimal choice for cases of refractory cardiogenic shock, however, there is a notable absence of comprehensive guidelines concerning many aspects related to this device, particularly in terms of appropriate patient selection, timing and duration of VA-ECMO, and also the management of associated complications.
Our aim in this work is to offer insightful information regarding the utilization and management of peripheral VA-ECMO within the catheterization laboratory (cath lab) in cases of CS ACS, from the viewpoint of a high-volume tertiary Shock Center. Our objective is to present an overview of both scientific and empirical recommendations on this important topic.
2. Veno-arterial extracorporeal membrane oxygenator (VA-ECMO)
As a specific type of ECLS, VA-ECMO enables the temporary replacement of both respiratory and cardiovascular functions, thus providing support in acute, life-threatening clinical scenarios (9, 10). If initiated in properly selected patients in a timely manner, VA-ECMO can significantly mitigate the risk of multiple organ failure and eventual death (11, 12).
Veno-arterial extracorporeal membrane oxygenator supports cardiorespiratory function by providing continuous flow and oxygenation using an external capillary biomatrix. It concurrently maintains effective cardiac output and relieves pressure in the central veins, right atrium, and right ventricle, which ensures organ protection for the time required to evaluate the prognosis and allows for a decision to be made on the optimal therapeutic method (bridge to decision/bridge to bridge) (4, 10, 13). Furthermore, it serves as a viable option until either reversible damage is restored (bridge to recovery), or the implantation of a long-term mechanical circulatory support (MCS) device (destination therapy) becomes necessary. VA-ECMO treatment might ultimately be extended until heart transplantation (bridge to transplantation) becomes feasible (14, 15).
The newest data on cardiogenic shock-associated pVA-ECMO outcomes were recently published by Thiele et al. (16), as they randomized CS ACS early revascularization treatment to an ECLS-supported and a conservative (non-ECLS) arm. The study showed no 30-day mortality differences between the groups. However, interpreting these results requires understanding several limiting factors that the authors themselves emphasized. First, left ventricular unloading in the ECLS group was undertaken at a low percentage (5.8%). Additionally, severe bleeding complications reduced the potential benefits of the ECLS group, which however, may be mitigated or somewhat minimized by an experienced Shock Team. Most importantly, despite randomization crossover/bail-out ECLS was initiated in 12.5% of the control group, furthermore, other forms of MCS (other than ECMO, primarily a microaxial transvalvular device) were used in an additional 15.4% of the control patients. Thus, altogether, nearly one-third of the control group did eventually receive mechanical circulatory support in one form or another. These statistics underscore the importance of utilizing ECLS or other MCS devices in managing critically ill patients, especially those facing hemodynamic deterioration despite appropriate medical interventions.
Among these devices, the microaxial transvalvular device (Impella) is increasingly deployed in cases of CS ACS, either alongside or concurrently with VA-ECMO. Impella operates as a catheter-based, continuous axial flow pump, allowing for active propulsion of blood into the aorta, while reducing myocardial stress and enhancing systemic circulation (9, 17). The safety and efficacy of Impella and VA-ECMO in treating critically ill patients with CS ACS remains a topic of debate due to conflicting evidence. Most studies suggest little difference in mortality rates, although specific outcomes rates vary. Lemor et al. (18) found higher in-hospital mortality and complication rates in the ECMO group, while others reported no significant differences in short-term or long-term mortality (19–21). Given these discrepancies and the complex nature of CS ACS, ongoing research is crucial to guide device selection in clinical practice.
We believe, that within the context of acute coronary syndrome complicated by cardiogenic shock, the utilization of VA-ECMO remains fundamental. Nonetheless, its effectiveness depends on careful patient selection, accurate timing of insertion, and a keen awareness of the specific conditions of each case. By adopting a patient-focused strategy, the administration and management of VA-ECMO can be improved, potentially leading to better results in this critically ill patient population.
3. Circumstances and timing of implantation
The peripheral iteration of the system (pVA-ECMO) is a widely utilized therapeutic approach as a component of the complex management of refractory CS ACS (22, 23). The implantation is typically conducted in the cath lab or the intensive care unit of the cardiology department but may also be implemented beyond the confines of an in-hospital setting (24–27). It involves percutaneous cannulation of the major blood vessels of the inguinal (femoral artery and/or vein) or jugular (carotid artery and/or internal jugular vein) region with a large bore cannula for use as outflow and inflow sites of the pVA-ECMO (28, 29).
As per the recommendations of the European Society of Cardiology (14, 15), cardiovascular centers are encouraged to establish designated teams to define the most appropriate strategy for managing patients with cardiogenic shock. These Shock Teams should typically consist of a multidisciplinary group of experts specialized in cardiology, cardiothoracic surgery, critical care, and nursing, working collaboratively to provide optimal care for patients in such critical conditions (9, 15, 30).
3.1. Patient selection
Establishing selection criteria for appropriate candidates and determining the optimal timing for MCS is of paramount importance, especially considering that presently approximately 40–50% of CS ACS patients survive with standard medical therapy, while another 25–35% of cases may not respond to MCS effectively. The remaining 15–35% of patients with CS ACS, however, show clear benefits from MCS and eventually survive due to pVA-ECMO support (2, 4).
Several scoring systems have been developed to assess the risk classification of patients based on specific clinical and biological indicators. These scoring systems, such as the SAVE Score (31), ENCOURAGE (32), and PREDICT-VA ECMO (33), have the potential to enhance patient selection and optimize outcomes. The most prevalent SAVE Score (31) is based on a cohort analysis combining data from multiple international centers. This scoring system classifies patients into five distinct risk categories based on a comprehensive set of criteria pertaining to their medical history and pre-ECMO implantation condition. In terms of prognosis, it assigns hospital survival rates ranging from 75 to 18%. According to the findings of the study, primary contributors to higher mortality rates include pre-ECMO organ failure, chronic renal failure, an extended period of intubation before ECMO, pre-ECMO cardiac arrest, congenital heart disease, pulse pressure before ECMO ≤ 20 mmHg, and HCO3– before ECMO ≤ 15 mmol/L. Conversely, factors that offer protection against increased mortality are younger age, lower body weight, and the underlying causes of cardiogenic shock being myocarditis, refractory VT/VF, or post-heart or lung transplantation, respectively.
Nonetheless, in the absence of internationally accepted CS ACS pVA-ECMO initialization guidelines, our center has developed its own protocol (Figure 2), based on available literature data and empirical findings from our own clinical study using one of the largest single-center databases among the specialized institutions worldwide (34). The protocol considers multiple factors, such as clinical condition, age, low-flow times, and comorbidities. It also incorporates the recommendations of the European Society of Cardiology (14, 15) (IIb/C), the American Heart Association (35) (IIa/C), and the Society for Cardiovascular Angiography and Interventions (36) concerning the initialization of MCS (Table 1).
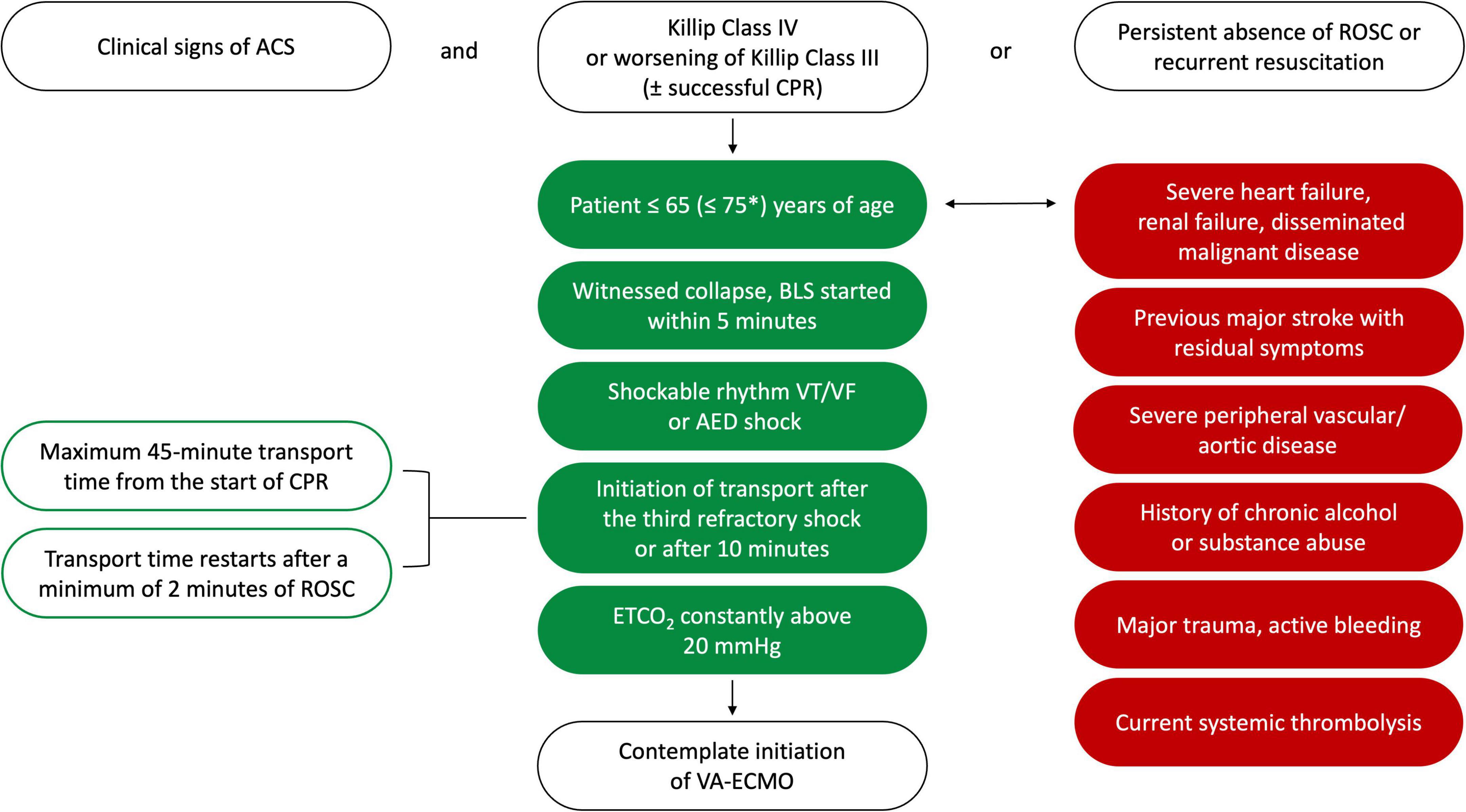
Figure 2. Protocol for peripheral VA-ECMO implantation in acute cardiogenic shock at the heart and vascular center. *If cardiac arrest occurs in a hospital setting, where the initiation of VA-ECMO is feasible within a 20-min timeframe. ACS, acute coronary syndrome; AED, automated external defibrillator; BLS, basic life support; CPR, cardiopulmonary resuscitation; ETCO2, end-tidal CO2; ROSC, return of spontaneous circulation; VA-ECMO, veno-arterial extracorporeal membrane oxygenator; VF, ventricular fibrillation; VT, ventricular tachycardia.
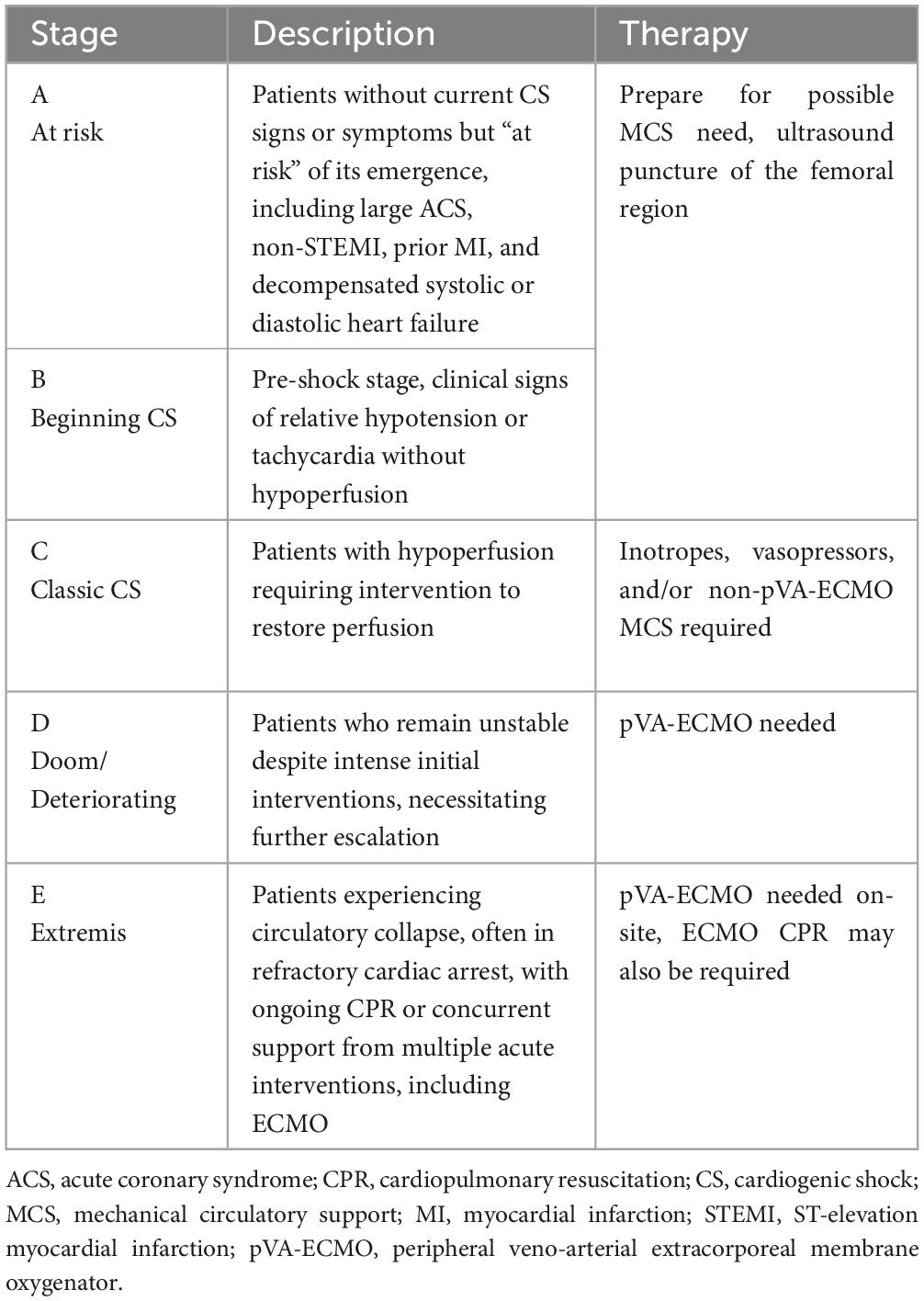
Table 1. Stages and management of cardiogenic shock based on the expert consensus of the Society for Cardiovascular Angiography and Interventions (36).
3.2. Time management and eCPR
Currently, advancements in infrastructure, individual circumstances, financial considerations, and other factors have enabled the initiation of out-of-hospital pVA-ECMO augmented cardiopulmonary resuscitation (eCPR), possibly enhancing the prospects of long-term survival with favorable neurological outcomes for such patients (24, 37). These, however, impose an extreme burden on human resources and equipment allocation, yet robust data suggests a genuine increase in the survival of this patient population is not self-evident (26, 27, 38). A possible alternative option is the transportation of patients to a nearby tertiary shock center as soon as possible with maintained continuous advanced life support for precise and fast-paced pVA-ECMO implantation and initiation in a hospital setting (39, 40).
The present literature underscores the crucial role of immediate and effective CPR as a critical determinant in the feasibility of implementing VA-ECMO and ultimately, patient survival (41). Specifically, it is emphasized that the “no-flow period,” which refers to the interval from the onset of cardiac arrest to the initiation of CPR should be limited to 5 min, whereas each second without CPR, the prospects for favorable neurological recovery diminish significantly (10, 41). Research indicates that an acceptable neurological outcome reduces to below 2% after 20 min (42) and below 1% after 30 min of CPR (“low-flow period”) (43).
Regarding the recommended time frame for transitioning to eCPR, it is suggested that consideration for this intervention be made at the 21-min mark of continuous CPR efforts (44). Patients with a low-flow period exceeding 60 min are less likely to benefit from the implementation of VA-ECMO, and beyond 90 min there is limited expectation of a favorable outcome (45, 46). The Extracorporeal Life Support Organization (ELSO) recommends conducting a prompt evaluation of the feasibility of eCPR and aims to keep the elapsed time from cardiac arrest to ECMO initiation within 60 min (47).
Our CS ACS protocol also defines clear parameters in case of CPR need, paying particular attention to cases of out-of-hospital cardiac arrest (OHCA), accounting for transportation times and other delays (48) (see Figure 2).
The optimal timing of VA-ECMO implantation compared to PCI in CS ACS patients is a subject of considerable investigation. Research revealed that among this patient population, VA-ECMO initiation before revascularization results in significantly better short- and long-term outcomes compared to cases where VA-ECMO insertion occurs subsequent to revascularization (49–52). The recommended protocol from our center underscores the critical importance of prompt implementation of VA-ECMO before proceeding with revascularization procedures (see Figure 3).
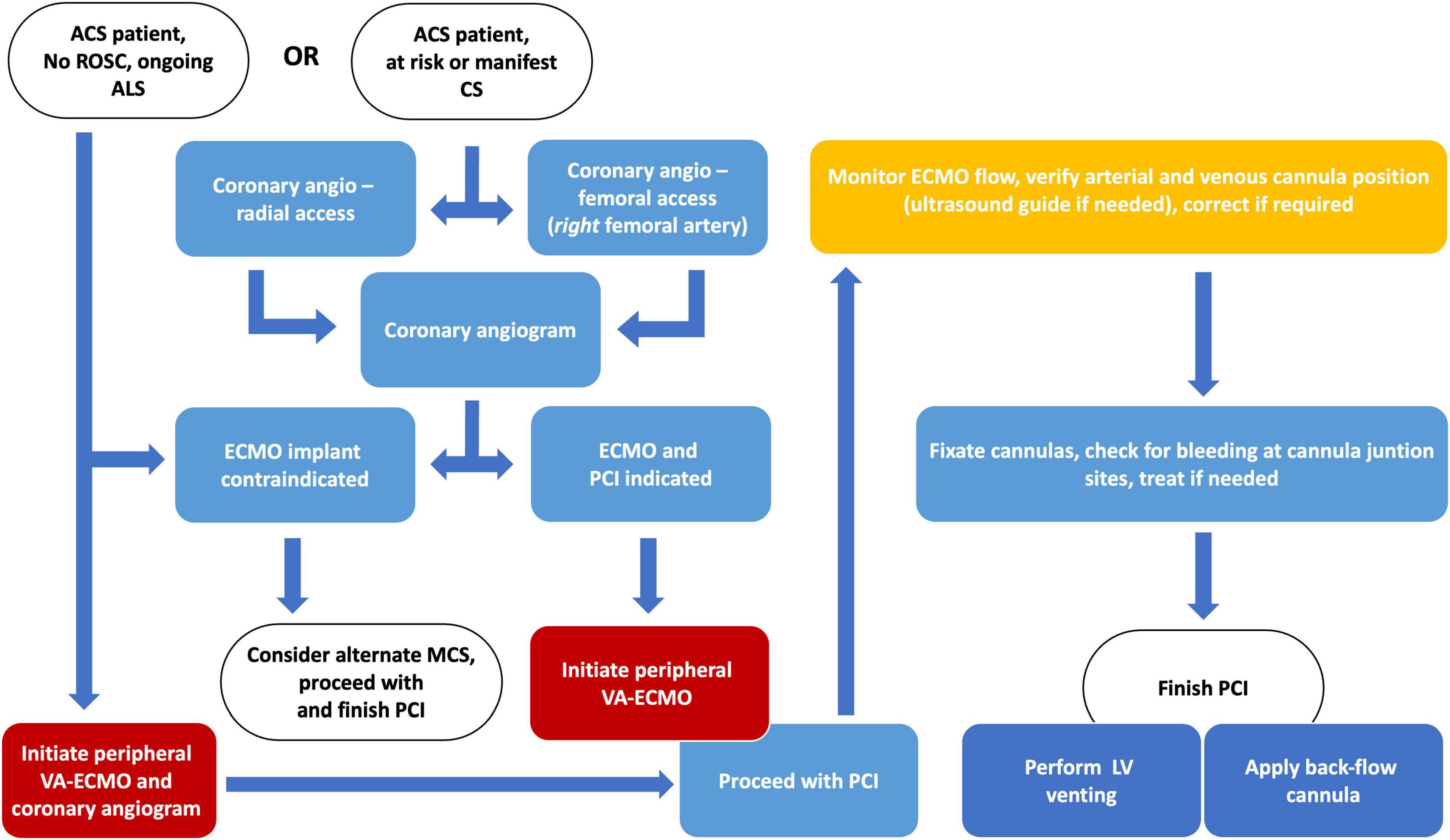
Figure 3. Algorithm and suggested workflow for the initiation of peripheral VA-ECMO in cardiogenic shock due to acute coronary syndrome. ACS, acute coronary syndrome; ALS, advanced life support; CS, cardiogenic shock; LV, left ventricle; MCS, mechanical circulatory support; PCI, percutaneous coronary intervention; ROSC, return of spontaneous circulation; VA-ECMO, veno-arterial extracorporeal membrane oxygenator.
4. Complications and management during pVA-ECMO care
4.1. Left ventricular unloading
In the case of femoral cannulation, the arterial cannula of the device, functioning as the outflow part, initiates a strong retrograde flow in the aorta, resulting in an elevation of left ventricular end-diastolic pressure (LVEDP) and increased wall tension, of an already failing heart in CS ACS. It may also increase myocardial oxygen demand and decrease the trans-coronary pressure gradient. This cascade of events may potentially lead to further myocardial necrosis, additionally compromising the unstable hemodynamic conditions and the function of a weak left ventricle (LV) (13, 53, 54). These factors can significantly contribute to unfavorable outcomes, especially in CS ACS where the sudden and significant reduction in cardiac function is primarily due to the imbalance in oxygen demand and supply. These processes are greatly influenced by the rate of ECMO flow, an increase of which can result in pulmonary stasis (so-called “ECMO lung”) and a decrease or even total cessation in cardiac output, potentially leading to left ventricular thrombosis, which harbors an extremely high mortality rate (55).
Based on the presented facts and observations, there is a clear indication of methods that can potentially reduce left ventricular pressure and congestion during VA-ECMO support. Two questions arise: what device to utilize and when should we implant it?
Although various methods are available for this purpose, decision-making can be challenging in the absence of clear guidelines. The two viable approaches are pharmacological therapy and the concomitant implantation of further invasive devices in conjunction with pVA-ECMO support (56).
The former method primarily entails the administration of inotropes, which may be supplemented by diuretics or potentially continuous veno-venous hemofiltration (CVVH) to effectively decrease the intravascular fluid volume (10). The option of combined device utilization encompasses several techniques: initiation of intra-aortic balloon pump (IABP) counterpulsation to decrease left ventricular pressure and pulmonary edema by reducing the afterload (57) (I); percutaneous implantation of a pigtail catheter into the LV and connection of this unit to the pVA-ECMO venous cannula (58) (IIa); using a transaortic axial flow heart pump to enhance forward flow and reduce pulmonary capillary wedge pressure (59) (IIb); performing mechanical left ventricular decompression through a transseptal puncture and creation of iatrogenic atrial septal defect (ASD), resulting in a left-right shunt (60) (III) (see Figure 4).
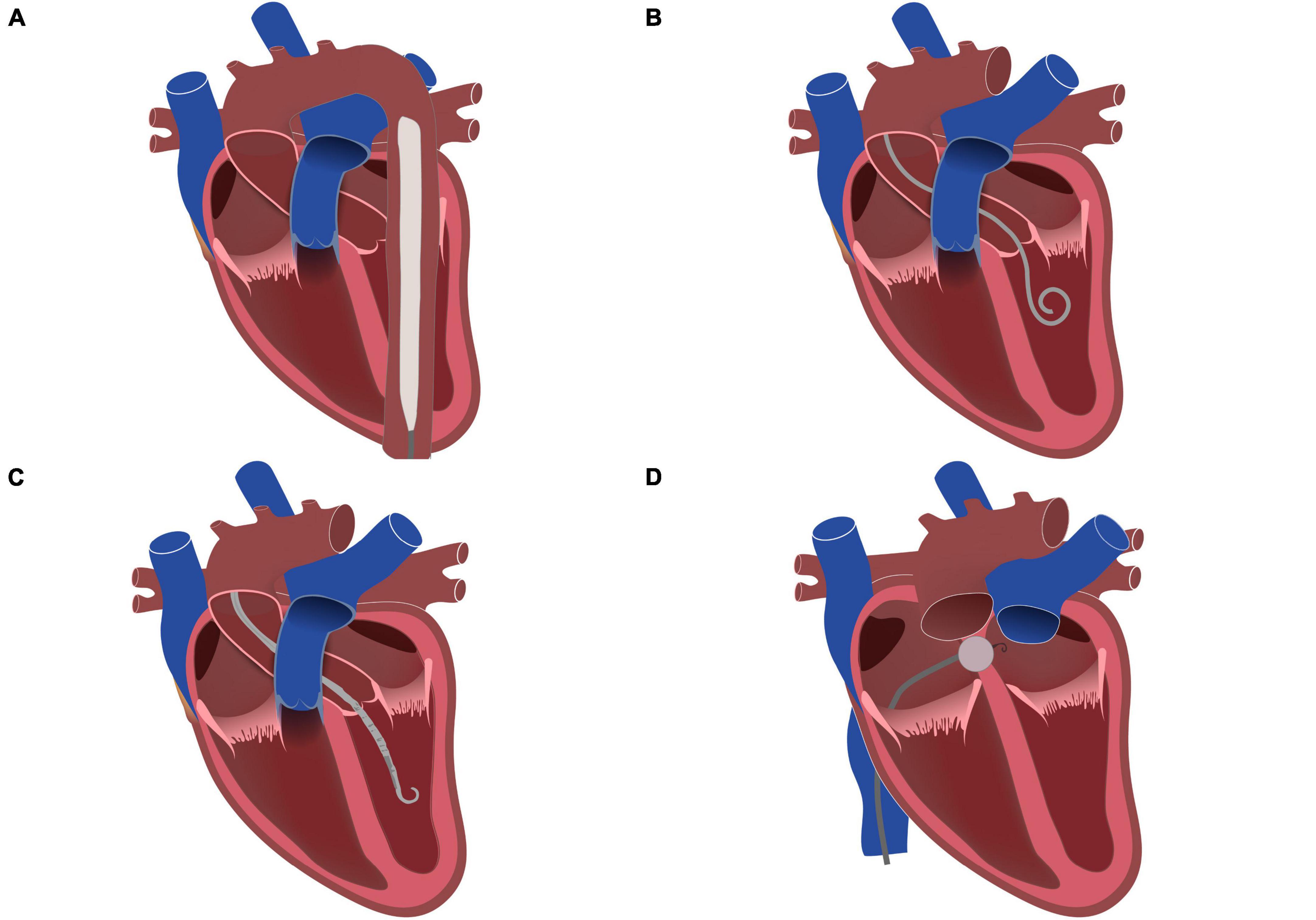
Figure 4. Left ventricular unloading methods during VA-ECMO treatment. (A) Intra-aortic balloon pump (IABP); (B) Pigtail catheter; (C) Microaxial transvalvular assist device (Impella); (D) Interatrial septostomy.
The indication for left ventricular unloading in general, the preferred method, and the appropriate timing of unloading present critical, yet unsettled aspects. Hemodynamic monitoring plays a crucial role in comprehensive patient care and should encompass the examination of pulmonary arterial diastolic pressure and PCWP using a Swan–Ganz catheter (61). Furthermore, with the advancements in echocardiography, it became feasible to not only monitor the opening of the aortic valve and chamber dimensions but also to assess left ventricular function in greater detail using functional parameters like global longitudinal strain, determined by the preload- and afterload-dependent speckle-tracking echocardiography (62, 63). The findings should guide the decision in the indication, selection, and timing of the left ventricular unloading devices.
In a comparison between prophylactic (initiated simultaneously with VA-ECMO) and therapeutic (post-symptomatic) left ventricular unloading, Na et al. (64) found that the prophylactic approach resulted in reduced early mortality rates and higher success rates of bridging to cardiac replacement therapy. These findings were corroborated by a multicenter study, demonstrating that unloading initiated before or within 2 h of VA-ECMO implantation led to a lower 30-day mortality rate compared to ECMO alone. The research team also revealed that every hour spent without unloading increases the risk of mortality in this critically ill patient population (65, 66). These results emphasize the importance of prompt left ventricular unloading in improving patient outcomes.
4.2. Harlequin syndrome
Another phenomenon to account for that may occur during pVA-ECMO care is the mixing of ECLS oxygenated blood from the arterial cannula and the oxygen-deprived ejected blood from the LV in the proximal part of the descending aorta. Since CS ACS always involves some degree of pulmonary failure, blood exiting the left ventricle is left at low oxygen saturation levels. Mixing usually occurs at the site of the major supra-aortic branches, involving either one of these (brachiocephalic trunk, left carotid, and left subclavian artery). This is the phenomenon of differential hypoxemia, the so-called Harlequin or North-South Syndrome (10, 67), which may compromise effective arterial ECMO blood flow to the central nervous system, especially limiting oxygen-rich blood flow to the right carotid and vertebral arteries. Also, the upper, mainly right side of the body, especially the upper extremity may be affected causing severe ischemia on occasion. Yet, as in CS ACS left ventricular ejection is usually minimal or completely absent due to severe myocardial stunning, this phenomenon rarely occurs in the early stages of care. Later, during intensive care as LV function improves, this phenomenon may manifest and thus, must be monitored (10, 67).
Treatment of apparent Harlequin syndrome is urgent and involves potentially restoring pulmonary function to allow for physiological blood oxygenation and/or aggressive venting of the LV to decrease the volume of ejected oxygen-deprived blood. Additional methods involve converting to a V-AV system arrangement using a return cannula to the superior vena cava, transitioning to a jugulo-femoral V-A, and opting for a VV-ECMO configuration. A markedly invasive option to solve the problem is converting the peripheral ECLS system to a central configuration which allows for a near-physiological distribution of arterial blood (67–69).
4.3. Critical ischemia of the lower limb
Ipsilateral ischemia in the lower limb containing the arterial cannula of the device is a frequently observed manifestation. With the utilization of large-bore cannulas operating at high flow rates, there is an occurrence of flow obstruction to distal arterial segments, potentially leading to severe or even critical ischemia in the lower limb. Methods on how to manage this situation are not uniform, although the application of a back-flow cannula is the most recommended approach to address this issue (70, 71). It involves the ipsilateral anterograde puncture and sheath cannulation of the affected limb at a distal segment of the superficial femoral artery. This second sheath is afterward connected to the arterial cannula of the pVA-ECMO, restoring peripheral arterial circulation in the lower extremities. Similar to ventricular unloading, prophylactic and symptomatic back-flow cannula implementation methods may also be used (72).
4.4. Bleeding complications
Hemorrhagic events are one of the primary complications associated with VA-ECMO treatment, typically arising due to vascular cannulation, anticoagulation, and antiplatelet strategies, or perturbations induced in the coagulation cascade (9, 54).
Patients under ECLS support require comprehensive anticoagulation using unfractionated heparin (UFH), maintaining elevated activated clotting time levels (180 to 200 s) to prevent oxygenator and cannula clotting issues. Evaluation of activated partial thromboplastin time (aPTT) serves as a superior indicator to assess potential thrombotic events. These are to be adjusted to between 40 and 60 s and may be increased to the 60 to 80-s range in case of increased thrombotic risk (10, 73, 74). Newer literature emphasizes the concomitant measurement of anti-Xa factor in conjunction with aPTT for the possible best conjunction when administering UFH anticoagulation. Target levels for anti-Xa factors range from 0.3 to 0.7 IU/mL (75).
To further complicate bleeding issues percutaneous revascularization treatment of CS ACS is almost always indicated, and implantation of one or more coronary stents is performed. The existing evidence concerning the simultaneous use of antiplatelet and anticoagulant therapy in cardiogenic shock patients on VA-ECMO is notably limited (76). These require dual antiplatelet therapy for a certain period varying from days to months, often encompassing highly effective P2Y12 antagonist administration such as prasugrel of ticagrelor.
Therefore, significant external and internal (mainly gastrointestinal) bleeding complications may often occur in association with pVA-ECMO, requiring intensive care measures such as blood transfusions and adjustments to anticoagulant treatment (10).
Implanting large-bore cannulas entails the use of highly invasive techniques, often necessitating incisions at the insertion sites. Despite sutures being the common method for securing cannulas, there is currently no standardized approach for addressing para-sheath and para-cannula bleeding. According to the ELSO (77), securing peripheral cannulas must involve at least two sites to prevent malpositioning and decannulation. Ensuring effective fixation of the cannulas is essential due to the potential life-threatening complications it can avert, including ECMO cannula-related infections (CRI), as well as mechanical circuit dysfunction that can lead to air embolism or severe bleeding (78, 79).
When considering alternative sutureless securement devices (such as semi-occlusive dressings, modified tapes, and adhesive anchors), caution should be exercised, as these products are not specific for the purpose of ECMO cannula fixation and, therefore may not be recommended in this context without further evidence (79, 80).
5. Weaning from VA-ECMO
Standardized guidelines for weaning patients from pVA-ECMO are currently lacking. Usage is typically limited to a maximum of 21 days, mainly due to oxygenator failure, major bleeding, or limb ischemia, however, early weaning is recommended, whenever possible.
The decision to discontinue pVA-ECMO support should be based on objective clinical factors, and closely monitored indicators such as increasing blood pressure and decreasing central venous and pulmonary pressures. Acute de-cannulation in the catheterization laboratory, even if the clinical scenario is very hopeful, should be avoided. To assess true cardiac recovery and response during a weaning process, the utilization of trans-esophageal echocardiography (TEE) is recommended (81).
Weaning should be carried out gradually, following a standard reduction of flow rate by 1 l/h every 3–4 h (so-called ECMO challenge). Decannulation can be considered once the patient exhibits stability and is able to sustain adequate cardiovascular function without ECMO support for a minimum duration of 1–2 h. Methods of decannulation also vary from percutaneous closure to vascular surgery.
6. Our recommendations regarding pVA-ECMO use in the catheterization laboratory
6.1. Who requires pVA-ECMO support?
The clinical decision to implement pVA-ECMO support requires swift and decisive action. If an “at risk” or clinically manifest CS ACS patient is identified in the cath lab, the multidisciplinary Shock Team should be notified without hesitation (9, 15, 30). We recommend a delay of five to a maximum of 10 min from the initial identification of the patient to the first decision and reassessment of any prior decision by the Shock Team should there be a change in the clinical course of the patient. We strongly urge the Shock Team to develop and use an evidence-backed decision-making checklist, adjusted to hospital standards of care. This may be altered and perfected as the Shock Team gains experience and most importantly: it will negate the possibility of missing crucial inclusion or exclusion criteria and allow for a homogenized selection of potentially treatable patients (Figure 2).
Based on published recommendations (10, 47), our previous results and experiences (34), the most important inclusion criteria are genuine ACS clinical features (I), age of subject (II), and Killip presentation (III). Exclusion criteria deal with issues that either hinder effective pVA-ECMO function: severe peripheral artery and/or aortic disease, including but not limited to aortic dissection, surgically repaired aorta, iliac or femoral arteries (I), severe, acute bleeding (II), clinical presentation after systemic thrombolysis (III); or correspond to severe co-morbidities that render overall survival questionable, such as severe symptomatic chronic heart failure, significant valvular dysfunction, renal insufficiency or disseminated malignant disease (IV), and history of chronic alcohol, drug or other substance abuse (V).
It is important to note that patients who require ongoing or recurrent CPR demand further attention to detail from the Shock Team, to individually determine eligibility criteria. Here the main objective is to establish if the patient has any medical chance of survival in case of pVA-ECMO support initiation. We recommend focusing on clinical and time-based parameters to establish appropriateness. Sudden death occurring due to ventricular arrhythmias (I) with minimal no-circulation time, quick initiation of basic life support (II), and high exhaled CO2 levels (III) are important hallmarks of potential benefit from MCS. Furthermore, especially during ongoing advanced life support (ALS), transport time constraints play a crucial role, regarding the initiation of transport to the shock center (IV) and the transport time itself (V).
If the Shock Team deems the CS ACS subject eligible for pVA-ECMO support, initiation of MCS should be the priority after coronary anatomy assessment. With patients arriving undergoing continuous ALS, initiation before diagnostic coronary anatomy assessment should be carried out. If personnel exchange is required in the cath lab for pVA-ECMO implantation, it should be performed immediately. After the successful establishment of cannulation and flow support, subsequent care, often involving complex PCI, becomes considerably simplified, leading to improved potential outcomes (49–52).
6.2. How to implant the pVA-ECMO in the cath lab?
Our recommendation is that either a senior interventional cardiologist, or in specific cases, a qualified heart surgeon should perform implantation of the pVA-ECMO. The operator should be experienced with large bore (16–24F) catheter devices, ultrasound-based bi-femoral puncture techniques, and suture procedures. The summary of the proposed algorithm is illustrated in Figure 3.
6.2.1. Puncture and cannulation
A bi-femoral cannulation method should be utilized in the cath lab during CS ACS. The right inguinal/femoral region is to be used for the venous cannula, ranging in size from 22 to 26F and lengths of up to 70 cm. The contralateral, left side is for the arterial cannula, with sizes between 14 to 18F (28, 29, 82). Our recommendation is to puncture the femoral vascular structures via ultrasound guidance only, ideally with one penetration of the needle. We propose maintaining this course of action even when cannulation is being performed during continuous chest compressions as part of ongoing ALS. Additionally, if patients are in the early stages of CS ACS (see Table 1), yet significant worsening is probable during the clinical course of PCI, pre-emptive cannulation of the femoral regions is preferable with 6F standard sheaths, which can either be efficiently exchanged to the pVA-ECMO cannula if needed or removed via commercially available closure devices (especially in case of the artery) if MCS is not required (36). These steps are essential for simplified and faster cannulation and are efficient in preventing later episodes of significant bleeding from puncture sites.
Cannula insertion is only advised using extra stiff or super stiff standard guidewires, using sequentially larger dilators, and finally the cannula itself. Based on our experience, it is often necessary to make a scalpel incision in the vessel before the final insertion of the cannula.
We also recommend placing pressure-resistant three-way valves on the arterial cannula side-port at this point during the procedure, which will serve as a connection point for the back-flow sheath. If left ventricular venting is planned via a pigtail catheter, then, the venous cannula side-port requires a similar valve as well. The distal end of the venous cannula needs to be placed into the right atrium (83), with the arterial cannula in the distal part of the abdominal aorta (28, 29, 82). Placement, depth, and positions of both cannulas need to be verified via fluoroscopy. If any uncertainties arise, positions may be checked and adjusted via ultrasound guidance (e.g., TEE for the venous side) (28, 29, 82). Connection of the pVA-ECMO circuit and cannulas requires the use of clamps and a syringe-based manual infusion of sterile saline into all four ends with meticulous attention to detail, to eliminate air bubbles from the system during connection, as any residuals can potentially cause catastrophic air emboli in the motor unit (84). Although unlikely to be swapped due to color coding, verifying arterial-arterial and venous-venous connections of the circuit and cannulas is mandatory as the last checkpoint prior to system launch.
6.2.2. Cannula fixation and local bleeding management
After the system is correctly set up, the pVA-ECMO may be started after the removal of all clamps. Ideally, the puncture to pVA-ECMO start time should be below 15 min. After system startup, safely and effectively securing the cannulas is of paramount importance requiring at least three subsequent cutaneous sutures or multiple custom-made adhesive clasps (77). Para-cannula and para-sheath bleeding are commonly present and require attention. If bleeding from the access site is significant, we advise practitioners to not only suture the bleeding site but use commercially available potassium-ferrate-based hemostatic powders/paste or similar, specifically designed incised discs to cover the junction of the skin and cannula. We have found this method particularly effective in solving this problematic issue.
6.2.3. Left ventricular unloading
Handling and fine-tuning the pVA-ECMO system should be left to dedicated personnel, ideally an expert perfusionist technician (77). With MCS stabilized circulatory and pulmonary support, revascularization should be completed as required in the CS ACS patient. Following successful PCI, there are two substantial matters to address concerning the pVA-ECMO system. The first one involves the decompression of the LV. Our standard of care mandates active LV venting for every CS ACS patient on pVA-ECMO support, regardless of the acute state of the chamber, or ejection fraction thereof. An additional ultrasound-guided arterial puncture of the right femoral region is required if not already present as PCI approach site. If accessible and available, the transaortic axial flow heart pump is the best option for active LV decompression as the device is adjustable to meet the clinical demands of the patient (59). The less sophisticated, non-adjustable alternative way with a similar mechanism of action involves a 7F pigtail catheter in the LV connected to the venous side of the pVA-ECMO cannula may also be recommended (58). We advise practitioners utilizing this technique to pay close attention to the venous cannula when attaching the catheter, as it can easily aspirate large amounts of air, rendering the motor unit and thus the whole MCS system useless. This is where the previously attached three-way valve plays a significant role, as it decreases the possibility of air emboli. A transseptal puncture to iatrogenic ASD formation is only recommended in case of a prosthetic aortic valve, it should not be undertaken otherwise (60). In a similar fashion use of the IABP for LV unloading is also contraindicated as it lacks the efficacy of active alternatives (57).
6.2.4. Prophylactic back-flow cannula
As the final step of the procedure, the back-flow cannula should be implanted in the femoral artery distal to the arterial pVA-ECMO cannula (70, 71). We recommend applying this device to every patient, in a prophylactic manner. An ultrasound-based anterograde, precise puncture of the superficial femoral artery is required. We recommend using commercially available 7F metallic sheaths with reinforced ports, capable of handling the pressure of the arterial outflow cannula. Drawing from our practical knowledge, to facilitate connection, the three-way valve plays an important role in attaching the devices safely without compromising blood flow, or the danger of bleeding from the cannula side port.
6.3. Infrastructure requirement and training
To successfully initiate a pVA-ECMO-based MCS program for an institution aiming to take on shock center responsibilities, we recommend the following resources in terms of human resources: ECMO-trained senior interventional cardiologist or heart surgeon (I), ECMO-trained perfusionist (II), ECMO-trained interventional assistant (III), and anesthesiologist (IV). With respect to the infrastructure: a pVA-ECMO system inside the cath lab 24/7 with an attached circuit, ready for the priming sequence. If the MCS device itself is outside of the cath lab, especially in a storage facility, this leads to further, significant time delays and reduces effectiveness. Our data shows that having the system inside the cath lab can halve puncture-to-ECMO start times.
Furthermore, an ECMO kit should be compiled encompassing everything that is needed in the cath lab for percutaneous implantation, fixation, and initiation of the system. We recommend including all consumables, e.g., the initial 6F sheaths, extra stiff guidewires, hemostatic devices, three-way valves, suturing and fixating materials, and all required clamps into the kit. Using this approach simplifies peri-procedural care.
Usually, that cath lab is a crowded environment, with most of the available space being taken up by the angiography system, its examination table, monitor and gantry. Also, other essential infrastructure is located in the examination room. As such, the available space is constrained, allowing only a few points of contact with the patient. Thus, it is advisable to pre-ordain the exact location of the pVA-ECMO machine, ensuring generous cable length compatibility and allocating applicable places for all medical staff. We support the idea of boot-camp-style training with a virtual patient (e.g., dummy) to focus on individual responsibilities, coordination, communication, and appropriate locations of staff and machinery.
6.4. Summary of recommendations
As a short summary of our recommendations, we advise always assessing the problem of CS ACS from a three-way perspective: patient (I), ECMO implant procedure (II), and infrastructure (III). These are three clearly definable, yet different issues when working as a Shock Team.
– Assessing the appropriate CS ACS patient for pVA-ECMO support needs to happen swiftly. The Shock Team must reach a clinically sound, unanimous verdict in a matter of minutes, for which a checklist is recommended to be used as discussed previously.
– The exact steps of the pVA-ECMO implementation need to be followed in a precise and calm manner for optimal performance. This is required to minimize puncture-to-ECMO time and prevent possibly catastrophic complications.
– The maintenance and replacement of the MCS system, other infrastructure and required consumables need to be undertaken at regular intervals and fully replenished after each implantation procedure.
Following the above recommendations and thought processes we hope that practitioners will be able to gain meaningful additional knowledge for their own Shock Team operations.
Author contributions
RE: Writing–original draft. BN: Writing–review and editing. PK: Writing–review and editing. GF: Writing–review and editing. DB: Writing–review and editing. BK: Writing–review and editing. EZ: Writing–review and editing. BM: Writing–review and editing. IÉ: Writing–original draft.
Funding
The authors declare that no financial support was received for the research, authorship, and/or publication of this article.
Conflict of interest
The authors declare that the research was conducted in the absence of any commercial or financial relationships that could be construed as a potential conflict of interest.
The authors declared that they were an editorial board member of Frontiers, at the time of submission. This had no impact on the peer review process and the final decision.
Publisher’s note
All claims expressed in this article are solely those of the authors and do not necessarily represent those of their affiliated organizations, or those of the publisher, the editors and the reviewers. Any product that may be evaluated in this article, or claim that may be made by its manufacturer, is not guaranteed or endorsed by the publisher.
Abbreviations
ACS, acute coronary syndrome; AED, automated external defibrillator; ALS, advanced life support; ASD, atrial septal defect; BLS, basic life support; CPR, cardiopulmonary resuscitation; CS, cardiogenic shock; CVVH, continuous veno-venous hemofiltration; ECLS, extracorporeal life support; IABP, intra-aortic balloon pump; LV, left ventricle; LVEDP, left ventricular end-diastolic pressure; MCS, mechanical circulatory support; OHCA, out-of-hospital cardiac arrest; PCI, percutaneous coronary intervention; PCWP, pulmonary capillary wedge pressure; ROSC, return of spontaneous circulation; TEE, transesophageal echocardiography; VAECMO, veno-arterial extracorporeal membrane oxygenator; VF, ventricular fibrillation; VT, ventricular tachycardia.
References
1. Reynolds HR, Hochman JS. Cardiogenic shock: current concepts and improving outcomes. Circulation. (2008) 117:686–97.
2. Thiele H, Zeymer U, Neumann FJ, Ferenc M, Olbrich HG, Hausleiter J, et al. Intraaortic balloon support for myocardial infarction with cardiogenic shock. N Engl J Med. (2012) 367:1287–96.
3. van Diepen S, Katz JN, Albert NM, Henry TD, Jacobs AK, Kapur NK, et al. Contemporary management of cardiogenic shock: a scientific statement from the american heart association. Circulation. (2017) 136:e232–68.
4. Thiele H, Ohman EM, de Waha-Thiele S, Zeymer U, Desch S. Management of cardiogenic shock complicating myocardial infarction: an update 2019. Eur Heart J. (2019) 40:2671–83.
5. Kolte D, Khera S, Aronow WS, Mujib M, Palaniswamy C, Sule S, et al. Trends in incidence, management, and outcomes of cardiogenic shock complicating ST-elevation myocardial infarction in the United States. J Am Heart Assoc. (2014) 3:e000590.
6. Goldberg RJ, Makam RC, Yarzebski J, McManus DD, Lessard D, Gore JM. Decade-long trends (2001-2011) in the incidence and hospital death rates associated with the in-hospital development of cardiogenic shock after acute myocardial infarction. Circ Cardiovasc Qual Outcomes. (2016) 9:117–25.
7. Chieffo A, Dudek D, Hassager C, Combes A, Gramegna M, Halvorsen S, et al. Joint EAPCI/ACVC expert consensus document on percutaneous ventricular assist devices. EuroIntervention. (2021) 17:e274–86.
8. Kulyassa P, Németh BT, Hartyánszky I, Szilveszter B, Fazekas L, Pólos M, et al. The use of VA-ECMO, our experiences at Semmelweis University’s heart and vascular center. Cardiol Hungarica. (2021) 51:320–30.
9. Combes A, Price S, Slutsky AS, Brodie D. Temporary circulatory support for cardiogenic shock. Lancet. (2020) 396:199–212. doi: 10.1016/S0140-6736(20)31047-3
10. Guglin M, Zucker MJ, Bazan VM, Bozkurt B, El Banayosy A, Estep JD, et al. Venoarterial ECMO for adults: JACC scientific expert panel. J Am Coll Cardiol. (2019) 73:698–716. doi: 10.1016/j.jacc.2018.11.038
11. Ouweneel DM, Schotborgh JV, Limpens J, Sjauw KD, Engstrom AE, Lagrand WK, et al. Extracorporeal life support during cardiac arrest and cardiogenic shock: a systematic review and meta-analysis. Intensive Care Med. (2016) 42:1922–34. doi: 10.1007/s00134-016-4536-8
12. Shin TG, Choi JH, Jo IJ, Sim MS, Song HG, Jeong YK, et al. Extracorporeal cardiopulmonary resuscitation in patients with inhospital cardiac arrest: a comparison with conventional cardiopulmonary resuscitation. Crit Care Med. (2011) 39:1–7. doi: 10.1097/CCM.0b013e3181feb339
13. Baran DA. Extracorporeal membrane oxygenation (ECMO) and the Critical Cardiac Patient. Curr Transplant Rep. (2017) 4:218–25. doi: 10.1007/s40472-017-0158-5
14. McDonagh TA, Metra M, Adamo M, Gardner RS, Baumbach A, Bohm M, et al. 2021 ESC guidelines for the diagnosis and treatment of acute and chronic heart failure. Eur Heart J. (2021) 42:3599–726. doi: 10.1093/eurheartj/ehab368
15. Ponikowski P, Voors AA, Anker SD, Bueno H, Cleland JGF, Coats AJS, et al. 2016 ESC guidelines for the diagnosis and treatment of acute and chronic heart failure: the task force for the diagnosis and treatment of acute and chronic heart failure of the European Society of Cardiology (ESC) developed with the special contribution of the heart failure association (HFA) of the ESC. Eur Heart J. (2016) 37:2129–200. doi: 10.1093/eurheartj/ehw128
16. Thiele H, Zeymer U, Akin I, Behnes M, Rassaf T, Mahabadi AA, et al. Extracorporeal life support in infarct-related cardiogenic shock. N Engl J Med. (2023) [Epub ahead of print].
17. Vora N, Chaudhary R, Upadhyay HV, Konat A, Zalavadia P, Padaniya A, et al. Mechanical assist device-assisted percutaneous coronary intervention: the use of impella versus extracorporeal membrane oxygenation as an emerging frontier in revascularization in cardiogenic shock. Cureus. (2023) 15:e33372. doi: 10.7759/cureus.33372
18. Lemor A, Hosseini Dehkordi SH, Basir MB, Villablanca PA, Jain T, Koenig GC, et al. Impella versus extracorporeal membrane oxygenation for acute myocardial infarction cardiogenic shock. Cardiovasc Revasc Med. (2020) 21:1465–71. doi: 10.1016/j.carrev.2020.05.042
19. Lamarche Y, Cheung A, Ignaszewski A, Higgins J, Kaan A, Griesdale DE, et al. Comparative outcomes in cardiogenic shock patients managed with impella microaxial pump or extracorporeal life support. J Thorac Cardiovasc Surg. (2011) 142:60–5. doi: 10.1016/j.jtcvs.2010.07.075
20. Schurtz G, Rousse N, Saura O, Balmette V, Vincent F, Lamblin N, et al. IMPELLA((R)) or extracorporeal membrane oxygenation for left ventricular dominant refractory cardiogenic shock. J Clin Med. (2021) 10:759. doi: 10.3390/jcm10040759
21. Schiller P, Hellgren L, Vikholm P. Survival after refractory cardiogenic shock is comparable in patients with impella and veno-arterial extracorporeal membrane oxygenation when adjusted for SAVE score. Eur Heart J Acute Cardiovasc Care. (2019) 8:329–37. doi: 10.1177/2048872618799745
22. Combes A, Leprince P, Luyt CE, Bonnet N, Trouillet JL, Leger P, et al. Outcomes and long-term quality-of-life of patients supported by extracorporeal membrane oxygenation for refractory cardiogenic shock. Crit Care Med. (2008) 36:1404–11.
23. Thiagarajan RR, Barbaro RP, Rycus PT, McMullan DM, Conrad SA, Fortenberry JD, et al. Extracorporeal life support organization registry international report 2016. ASAIO J. (2017) 63:60–7. doi: 10.1097/MAT.0000000000000475
24. Chen YS, Lin JW, Yu HY, Ko WJ, Jerng JS, Chang WT, et al. Cardiopulmonary resuscitation with assisted extracorporeal life-support versus conventional cardiopulmonary resuscitation in adults with in-hospital cardiac arrest: an observational study and propensity analysis. Lancet. (2008) 372:554–61. doi: 10.1016/S0140-6736(08)60958-7
25. Kagawa E, Dote K, Kato M, Sasaki S, Nakano Y, Kajikawa M, et al. Should we emergently revascularize occluded coronaries for cardiac arrest: rapid-response extracorporeal membrane oxygenation and intra-arrest percutaneous coronary intervention. Circulation. (2012) 126:1605–13. doi: 10.1161/CIRCULATIONAHA.111.067538
26. Bougouin W, Dumas F, Lamhaut L, Marijon E, Carli P, Combes A, et al. Extracorporeal cardiopulmonary resuscitation in out-of-hospital cardiac arrest: a registry study. Eur Heart J. (2020) 41:1961–71. doi: 10.1093/eurheartj/ehz753
27. Choi DS, Kim T, Ro YS, Ahn KO, Lee EJ, Hwang SS, et al. Extracorporeal life support and survival after out-of-hospital cardiac arrest in a nationwide registry: a propensity score-matched analysis. Resuscitation. (2016) 99:26–32. doi: 10.1016/j.resuscitation.2015.11.013
28. Pavlushkov E, Berman M, Valchanov K. Cannulation techniques for extracorporeal life support. Ann Transl Med. (2017) 5:70.
29. Gajkowski EF, Herrera G, Hatton L, Velia Antonini M, Vercaemst L, Cooley E. ELSO guidelines for adult and pediatric extracorporeal membrane oxygenation circuits. ASAIO J. (2022) 68:133–52. doi: 10.1097/MAT.0000000000001630
30. Crespo-Leiro MG, Metra M, Lund LH, Milicic D, Costanzo MR, Filippatos G, et al. Advanced heart failure: a position statement of the heart failure association of the European Society of Cardiology. Eur J Heart Fail. (2018) 20:1505–35.
31. Schmidt M, Burrell A, Roberts L, Bailey M, Sheldrake J, Rycus PT, et al. Predicting survival after ECMO for refractory cardiogenic shock: the survival after veno-arterial-ECMO (SAVE)-score. Eur Heart J. (2015) 36:2246–56.
32. Muller G, Flecher E, Lebreton G, Luyt CE, Trouillet JL, Brechot N, et al. The ENCOURAGE mortality risk score and analysis of long-term outcomes after VA-ECMO for acute myocardial infarction with cardiogenic shock. Intensive Care Med. (2016) 42:370–8. doi: 10.1007/s00134-016-4223-9
33. Wengenmayer T, Duerschmied D, Graf E, Chiabudini M, Benk C, Muhlschlegel S, et al. Development and validation of a prognostic model for survival in patients treated with venoarterial extracorporeal membrane oxygenation: the PREDICT VA-ECMO score. Eur Heart J Acute Cardiovasc Care. (2019) 8:350–9.
34. Edes IF, Nemeth BT, Hartyanszky I, Szilveszter B, Kulyassa P, Fazekas L, et al. Predictors of mortality following extracorporeal membrane oxygenation support in an unselected, critically ill patient population. Postepy Kardiol Interwencyjnej. (2021) 17:290–7.
35. Peura JL, Colvin-Adams M, Francis GS, Grady KL, Hoffman TM, Jessup M, et al. Recommendations for the use of mechanical circulatory support: device strategies and patient selection: a scientific statement from the American Heart Association. Circulation. (2012) 126:2648–67. doi: 10.1161/CIR.0b013e3182769a54
36. Baran DA, Grines CL, Bailey S, Burkhoff D, Hall SA, Henry TD, et al. SCAI clinical expert consensus statement on the classification of cardiogenic shock: this document was endorsed by the American College of Cardiology (ACC), the American Heart Association (AHA), the Society of Critical Care Medicine (SCCM), and the Society of Thoracic Surgeons (STS) in April 2019. Catheter Cardiovasc Interv. (2019) 94:29–37. doi: 10.1002/ccd.28329
37. Bartos JA, Grunau B, Carlson C, Duval S, Ripeckyj A, Kalra R, et al. Improved survival with extracorporeal cardiopulmonary resuscitation despite progressive metabolic derangement associated with prolonged resuscitation. Circulation. (2020) 141:877–86.
38. Gottula AL, Neumar RW, Hsu CH. Extracorporeal cardiopulmonary resuscitation for out-of-hospital cardiac arrest - who, when, and where? Curr Opin Crit Care. (2022) 28:276–83.
39. Papolos AI, Kenigsberg BB, Berg DD, Alviar CL, Bohula E, Burke JA, et al. Management and outcomes of cardiogenic shock in cardiac ICUs with versus without shock teams. J Am Coll Cardiol. (2021) 78:1309–17.
40. Rab T, Ratanapo S, Kern KB, Basir MB, McDaniel M, Meraj P, et al. Cardiac shock care centers: JACC review topic of the week. J Am Coll Cardiol. (2018) 72:1972–80.
41. Rajan S, Wissenberg M, Folke F, Hansen SM, Gerds TA, Kragholm K, et al. Association of bystander cardiopulmonary resuscitation and survival according to ambulance response times after out-of-hospital cardiac arrest. Circulation. (2016) 134:2095–104.
42. Goto Y, Funada A, Goto Y. Relationship between the duration of cardiopulmonary resuscitation and favorable neurological outcomes after out-of-hospital cardiac arrest: a prospective, nationwide, population-based cohort study. J Am Heart Assoc. (2016) 5:e002819. doi: 10.1161/JAHA.115.002819
43. Reynolds JC, Frisch A, Rittenberger JC, Callaway CW. Duration of resuscitation efforts and functional outcome after out-of-hospital cardiac arrest: when should we change to novel therapies? Circulation. (2013) 128:2488–94. doi: 10.1161/CIRCULATIONAHA.113.002408
44. Kim SJ, Jung JS, Park JH, Park JS, Hong YS, Lee SW. An optimal transition time to extracorporeal cardiopulmonary resuscitation for predicting good neurological outcome in patients with out-of-hospital cardiac arrest: a propensity-matched study. Crit Care. (2014) 18:535. doi: 10.1186/s13054-014-0535-8
45. Chen YS, Yu HY, Huang SC, Lin JW, Chi NH, Wang CH, et al. Extracorporeal membrane oxygenation support can extend the duration of cardiopulmonary resuscitation. Crit Care Med. (2008) 36:2529–35.
46. Wengenmayer T, Rombach S, Ramshorn F, Biever P, Bode C, Duerschmied D, et al. Influence of low-flow time on survival after extracorporeal cardiopulmonary resuscitation (eCPR). Crit Care. (2017) 21:157.
47. Richardson ASC, Tonna JE, Nanjayya V, Nixon P, Abrams DC, Raman L, et al. Extracorporeal cardiopulmonary resuscitation in adults. Interim guideline consensus statement from the extracorporeal life support organization. ASAIO J. (2021) 67:221–8. doi: 10.1097/MAT.0000000000001344
48. Kiss B, Nagy B, Pal-Jakab A, Lakatos B, Soltesz A, Osztheimer I, et al. Early application of ECMO after sudden cardiac arrest to prevent further deterioration: a review and case report. J Clin Med. (2023) 12:4249. doi: 10.3390/jcm12134249
49. Choi KH, Yang JH, Hong D, Park TK, Lee JM, Song YB, et al. Optimal timing of venoarterial-extracorporeal membrane oxygenation in acute myocardial infarction patients suffering from refractory cardiogenic shock. Circ J. (2020) 84:1502–10. doi: 10.1253/circj.CJ-20-0259
50. Del Rio-Pertuz G, Benjanuwattra J, Juarez M, Mekraksakit P, Argueta-Sosa E, Ansari MM. Efficacy of mechanical circulatory support used before versus after primary percutaneous coronary intervention in patients with cardiogenic shock from ST-elevation myocardial infarction: a systematic review and meta-analysis. Cardiovasc Revasc Med. (2022) 42:74–83. doi: 10.1016/j.carrev.2022.05.002
51. Huang CC, Hsu JC, Wu YW, Ke SR, Huang JH, Chiu KM, et al. Implementation of extracorporeal membrane oxygenation before primary percutaneous coronary intervention may improve the survival of patients with ST-segment elevation myocardial infarction and refractory cardiogenic shock. Int J Cardiol. (2018) 269:45–50. doi: 10.1016/j.ijcard.2018.07.023
52. Kuroki N, Abe D, Iwama T, Suzuki K, Sugiyama K, Akashi A, et al. Association between delay to coronary reperfusion and outcome in patients with acute coronary syndrome undergoing extracorporeal cardiopulmonary resuscitation. Resuscitation. (2017) 114:1–6. doi: 10.1016/j.resuscitation.2017.02.007
53. Cevasco M, Takayama H, Ando M, Garan AR, Naka Y, Takeda K. Left ventricular distension and venting strategies for patients on venoarterial extracorporeal membrane oxygenation. J Thorac Dis. (2019) 11:1676–83. doi: 10.21037/jtd.2019.03.29
54. Cheng R, Hachamovitch R, Kittleson M, Patel J, Arabia F, Moriguchi J, et al. Complications of extracorporeal membrane oxygenation for treatment of cardiogenic shock and cardiac arrest: a meta-analysis of 1,866 adult patients. Ann Thorac Surg. (2014) 97:610–6. doi: 10.1016/j.athoracsur.2013.09.008
55. Weber C, Deppe AC, Sabashnikov A, Slottosch I, Kuhn E, Eghbalzadeh K, et al. Left ventricular thrombus formation in patients undergoing femoral veno-arterial extracorporeal membrane oxygenation. Perfusion. (2018) 33:283–8. doi: 10.1177/0267659117745369
56. Donker DW, Brodie D, Henriques JPS, Broome M. Left ventricular unloading during veno-arterial ECMO: a review of percutaneous and surgical unloading interventions. Perfusion. (2019) 34:98–105.
57. Patel SM, Lipinski J, Al-Kindi SG, Patel T, Saric P, Li J, et al. Simultaneous venoarterial extracorporeal membrane oxygenation and percutaneous left ventricular decompression therapy with impella is associated with improved outcomes in refractory cardiogenic shock. ASAIO J. (2019) 65:21–8. doi: 10.1097/MAT.0000000000000767
58. Barbone A, Malvindi PG, Ferrara P, Tarelli G. Left ventricle unloading by percutaneous pigtail during extracorporeal membrane oxygenation. Interact Cardiovasc Thorac Surg. (2011) 13:293–5. doi: 10.1510/icvts.2011.269795
59. Akanni OJ, Takeda K, Truby LK, Kurlansky PA, Chiuzan C, Han J, et al. EC-VAD: combined use of extracorporeal membrane oxygenation and percutaneous microaxial pump left ventricular assist device. ASAIO J. (2019) 65:219–26. doi: 10.1097/MAT.0000000000000804
60. Seib PM, Faulkner SC, Erickson CC, Van Devanter SH, Harrell JE, Fasules JW, et al. Blade and balloon atrial septostomy for left heart decompression in patients with severe ventricular dysfunction on extracorporeal membrane oxygenation. Catheter Cardiovasc Interv. (1999) 46:179–86. doi: 10.1002/(SICI)1522-726X(199902)46:2<179::AID-CCD13>3.0.CO;2-W
61. Mosier JM, Kelsey M, Raz Y, Gunnerson KJ, Meyer R, Hypes CD, et al. Extracorporeal membrane oxygenation (ECMO) for critically ill adults in the emergency department: history, current applications, and future directions. Crit Care. (2015) 19:431. doi: 10.1186/s13054-015-1155-7
62. Kim D, Jang WJ, Park TK, Cho YH, Choi JO, Jeon ES, et al. Echocardiographic predictors of successful extracorporeal membrane oxygenation weaning after refractory cardiogenic shock. J Am Soc Echocardiogr. (2021) 34:414–22 e4. doi: 10.1016/j.echo.2020.12.002
63. Lakatos BK, Ruppert M, Tokodi M, Olah A, Braun S, Karime C, et al. Myocardial work index: a marker of left ventricular contractility in pressure- or volume overload-induced heart failure. ESC Heart Fail. (2021) 8:2220–31. doi: 10.1002/ehf2.13314
64. Na SJ, Yang JH, Yang JH, Sung K, Choi JO, Hahn JY, et al. Left heart decompression at venoarterial extracorporeal membrane oxygenation initiation in cardiogenic shock: prophylactic versus therapeutic strategy. J Thorac Dis. (2019) 11:3746–56. doi: 10.21037/jtd.2019.09.35
65. Schrage B, Becher PM, Bernhardt A, Bezerra H, Blankenberg S, Brunner S, et al. Left ventricular unloading is associated with lower mortality in patients with cardiogenic shock treated with venoarterial extracorporeal membrane oxygenation: results from an international, multicenter cohort study. Circulation. (2020) 142:2095–106. doi: 10.1161/CIRCULATIONAHA.120.048792
66. Schrage B, Sundermeyer J, Blankenberg S, Colson P, Eckner D, Eden M, et al. Timing of active left ventricular unloading in patients on venoarterial extracorporeal membrane oxygenation therapy. JACC Heart Fail. (2023) 11:321–30. doi: 10.1016/j.jchf.2022.11.005
67. Falk L, Sallisalmi M, Lindholm JA, Lindfors M, Frenckner B, Broome M, et al. Differential hypoxemia during venoarterial extracorporeal membrane oxygenation. Perfusion. (2019) 34(1_suppl):22–9. doi: 10.1177/0267659119830513
68. Contento C, Battisti A, Agro B, De Marco M, Iaiza A, Pietraforte L, et al. A novel veno-arteriovenous extracorporeal membrane oxygenation with double pump for the treatment of Harlequin syndrome. Perfusion. (2020) 35(1_suppl):65–72. doi: 10.1177/0267659120908409
69. Wilson J, Fisher R, Caetano F, Soliman-Aboumarie H, Patel B, Ledot S, et al. Managing harlequin syndrome in VA-ECMO - do not forget the right ventricle. Perfusion. (2022) 37:526–9. doi: 10.1177/02676591211020895
70. Madershahian N, Nagib R, Wippermann J, Strauch J, Wahlers T. A simple technique of distal limb perfusion during prolonged femoro-femoral cannulation. J Card Surg. (2006) 21:168–9. doi: 10.1111/j.1540-8191.2006.00201.x
71. Juo YY, Skancke M, Sanaiha Y, Mantha A, Jimenez JC, Benharash P. Efficacy of distal perfusion cannulae in preventing limb ischemia during extracorporeal membrane oxygenation: a systematic review and meta-analysis. Artif Organs. (2017) 41:E263–73. doi: 10.1111/aor.12942
72. Lamb KM, DiMuzio PJ, Johnson A, Batista P, Moudgill N, McCullough M, et al. Arterial protocol including prophylactic distal perfusion catheter decreases limb ischemia complications in patients undergoing extracorporeal membrane oxygenation. J Vasc Surg. (2017) 65:1074–9. doi: 10.1016/j.jvs.2016.10.059
73. Esper SA, Levy JH, Waters JH, Welsby IJ. Extracorporeal membrane oxygenation in the adult: a review of anticoagulation monitoring and transfusion. Anesth Analg. (2014) 118:731–43. doi: 10.1213/ANE.0000000000000115
74. Rajsic S, Breitkopf R, Jadzic D, Popovic Krneta M, Tauber H, Treml B. Anticoagulation strategies during extracorporeal membrane oxygenation: a narrative review. J Clin Med. (2022) 11:5147. doi: 10.3390/jcm11175147
75. Levy JH, Staudinger T, Steiner ME. How to manage anticoagulation during extracorporeal membrane oxygenation. Intensive Care Med. (2022) 48:1076–9. doi: 10.1007/s00134-022-06723-z
76. Staudacher DL, Biever PM, Benk C, Ahrens I, Bode C, Wengenmayer T. Dual antiplatelet therapy (DAPT) versus no antiplatelet therapy and incidence of major bleeding in patients on venoarterial extracorporeal membrane oxygenation. PLoS One. (2016) 11:e0159973. doi: 10.1371/journal.pone.0159973
77. ELSO. Guidelines for Cardiopulmonary Extracorporeal Life Support Extracorporeal Life Support Organization, Version 1.4. Ann Arbor, MI: ELSO (2017).
78. Biffi S, Di Bella S, Scaravilli V, Peri AM, Grasselli G, Alagna L, et al. Infections during extracorporeal membrane oxygenation: epidemiology, risk factors, pathogenesis and prevention. Int J Antimicrob Agents. (2017) 50:9–16. doi: 10.1016/j.ijantimicag.2017.02.025
79. Bull T, Corley A, Lye I, Spooner AJ, Fraser JF. Cannula and circuit management in peripheral extracorporeal membrane oxygenation: an international survey of 45 countries. PLoS One. (2019) 14:e0227248. doi: 10.1371/journal.pone.0227248
80. Inwood S. An exploration of the past, present and future of catheter securement. Br J Nurs. (2014) 23:S26–7. doi: 10.12968/bjon.2014.23.Sup8.S26
81. Tsiouris A, Protos AN, Saikus CE, Jeyakumar AKC. Fundamentals of weaning veno-arterial and veno-venous extracorporeal membrane oxygenation. Indian J Thorac Cardiovasc Surg. (2023) 39(Suppl 1):1–11. doi: 10.1007/s12055-023-01474-y
82. Napp LC, Kuhn C, Hoeper MM, Vogel-Claussen J, Haverich A, Schafer A, et al. Cannulation strategies for percutaneous extracorporeal membrane oxygenation in adults. Clin Res Cardiol. (2016) 105:283–96. doi: 10.1007/s00392-015-0941-1
83. Lindfors M, Frenckner B, Sartipy U, Bjallmark A, Broome M. Venous cannula positioning in arterial deoxygenation during veno-arterial extracorporeal membrane oxygenation-a simulation study and case report. Artif Organs. (2017) 41:75–81. doi: 10.1111/aor.12700
Keywords: cardiogenic shock (CS), percutaneous coronary intervention (PCI), acute coronary syndrome (ACS), extracorporeal life support (ECLS), veno-arterial extracorporeal membrane oxygenator (VA-ECMO)
Citation: Ehrenberger R, Németh BT, Kulyassa P, Fülöp GA, Becker D, Kiss B, Zima E, Merkely B and Édes IF (2023) Acute coronary syndrome associated cardiogenic shock in the catheterization laboratory: peripheral veno-arterial extracorporeal membrane oxygenator management and recommendations. Front. Med. 10:1277504. doi: 10.3389/fmed.2023.1277504
Received: 14 August 2023; Accepted: 18 September 2023;
Published: 07 November 2023.
Edited by:
Luo Zhe, Fudan University, ChinaReviewed by:
Andrea Glotta, Ospedale Regionale di Lugano, SwitzerlandAhmet Karabulut, Acibadem MAA Üniversite Atakent Hospital, Türkiye
Copyright © 2023 Ehrenberger, Németh, Kulyassa, Fülöp, Becker, Kiss, Zima, Merkely and Édes. This is an open-access article distributed under the terms of the Creative Commons Attribution License (CC BY). The use, distribution or reproduction in other forums is permitted, provided the original author(s) and the copyright owner(s) are credited and that the original publication in this journal is cited, in accordance with accepted academic practice. No use, distribution or reproduction is permitted which does not comply with these terms.
*Correspondence: István F. Édes, ZWRlczc4OUBnbWFpbC5jb20=