- 1Department of Reproductive Medicine, The Puer People's Hospital, Pu'er, China
- 2Department of Pharmacy, The Puer People's Hospital, Pu'er, China
- 3School of Pharmaceutical Science and Yunnan Key Laboratory of Pharmacology for Natural Products, Kunming Medical University, Kunming, China
Background: The effect of booster vaccinations with the coronavirus virus disease (COVID-19) vaccine on people living with HIV (PLWH) remains unknown. In this study, we aimed to investigate the immunogenicity and effectiveness of booster doses of the COVID-19 vaccine in PLWH.
Methods: Literature research was done through the PubMed, Embase, Cochrane Review, and Web of Science databases up to 4 July 2023. Pooled estimates were calculated and compared using the DerSimonian and Laird method for a random effects model. Randomized control trials and observational studies were both considered for inclusion.
Results: We included 35 eligible studies covering 30,154 PLWH. The pooled immune response rate (IRR) of PLWH after the COVID-19 booster vaccination was 97.25% (95% confidence interval [CI], 93.81–99.49), and similar to healthy control (HC) (risk ratio [RR] = 0.98, 95% CI, 0.96–1.00). The pooled IRR for PLWH with CD4+ T-cell counts ≤ 200 was 86.27 (95% CI, 65.35–99.07). For Omicron variants, the pooled IRR for PLWH after booster dose was 74.07% (95% CI, 58.83–89.30), and the risk of IRR was reduced by 10% in PLWH compared with HC (RR = 0.90, 95% CI, 0.80–1.00). The T-cell immune response of PLWH was found to be comparable to HC (p ≥ 0.05). Subgroup analyses revealed that mRNA vaccines produced a relatively high IRR in PLWH compared to other vaccines. In addition, the results showed that booster vaccination appeared to further reduce the risk of COVID-19-related infections, hospitalizations, and deaths compared with the primary vaccination.
Conclusion: It was shown that booster vaccination with the COVID-19 vaccine provided a high IRR in PLWH and still produced a desirable moderate IRR in PLWH with a CD4+ T-cell count of ≤ 200. Importantly, the humoral and T-cell responses to booster vaccination in PLWH were comparable to HC, and similar results were observed with the SARS-CoV-2 Omicron variant. Our review strongly emphasizes the effect of mRNA vaccine booster vaccination in PLWH on eliciting desirable protective IRR. Furthermore, booster vaccination appears to further reduce the risk of COVID-19 infection, hospitalization, and death in PLWH compared to primary vaccination. However, more evidence is needed to confirm its effectiveness.
Introduction
The World Health Organization (WHO) has declared the end of the coronavirus disease 2019 (COVID-19) emergency. However, the severe acute respiratory syndrome coronavirus 2 (SARS-CoV-2) variants and the risk of breakthrough infections make the post-pandemic era challenging (1–3). At present, the impact of impaired immunity on the progression of SARS-CoV-2 infection and COVID-19 remains difficult to account for in individuals with immunodeficiency diseases such as people living with HIV (PLWH) (4, 5). Immune perturbations from innate and adaptive immunity, chronic inflammation, accelerated immune senescence, a high prevalence of comorbidities, and sociodemographic factors may contribute to the increased risk of SARS-CoV-2 infection in PLWH compared with HIV-negative individuals (6). A WHO report states that PLWH appears to be a significant independent risk factor for SARS-CoV-2 infection (7, 8). This risk is particularly higher for PLWH with advanced disease, low CD4+ T-cell levels, or detectable viremia, as they may be more susceptible to severe COVID-19 and death (9–14).
The current meta-analyses on COVID-19 have mainly focused on primary vaccination, and there is a lack of evidence-based data for booster doses (15–18). In the original COVID-19 cohort studies, particularly in developed countries, comparable clinical outcomes were found in PLWH and HIV-negative individuals (19, 20). In contrast, in other cohort studies, PLWH demonstrated worse outcomes, including higher rates of hospitalization and mortality (7, 21). Timely evaluation of the evidence for booster doses of vaccination is necessary to ensure strategy adaptation for high protection of PLWH. Previous studies have shown (22) that COVID-19 mortality is similar in PLWH and HIV-negative individuals when Alpha, Beta, and Delta variants predominate. However, it has been shown that the immunocompromised population is associated with an increased risk of COVID-19-associated mortality in the face of Omicron variants (23, 24). Providing booster doses to the general population may help overcome this problem (25). However, in PLWH, especially in those individuals with severe immunosuppression, the extent of antibody protection regarding additional doses is unknown.
Evidence suggests that the induction of neutralizing antibody responses alone does not adequately protect the organism during SARS-CoV-2 virus infection, and the involvement of T-cell immune responses is pivotal (26, 27). CD4+ T-cell and CD8+ T-cell levels and the use of antiretroviral therapy (ART) were shown to be correlates of effective immune responses to influenza or pneumococcal vaccines (28, 29). Recent immunization studies in PLWH have shown that assessment of a third dose of the COVID-19 vaccine elicited a strong humoral immune response but reduced T-cell stimulation compared to healthy control (HC) (30, 31). However, deviations in the CD4+ to CD8+ ratio may also contribute to the decreased responsiveness of PLWH to SARS-CoV-2 infection (32). Further investigations are needed to elucidate the effect of booster vaccines on T-cell immune responses in PLWH.
In summary, the current data on SARS-CoV-2-infected individuals and PLWH raise the question of whether booster vaccinations with COVID-19 vaccines produce the desired immune response. This is especially relevant when faced with conditions such as severe immunosuppression or the Omicron variant. In this meta-analysis and systematic review, we systematically assessed humoral and cellular immune responses after booster doses of COVID-19 vaccination in PLWH and compared them with HC. We also analyzed clinical effectiveness outcome indicators.
Methods
Search strategy
We conducted a systematic review and meta-analysis according to the Preferred Reporting Items for Systematic Reviews and Meta-Analyses (PRISMA) recommendations (33). Systematic literature research was completed through PubMed, Embase, Cochrane Review, and Web of Science, with a search date from inception to 4 July 2023. Search terms included (“COVID-19” or “SARS-CoV-2”), (“vaccine” or “vaccination” or “booster”), and (“HIV” or “acquired immunodeficiency syndrome”). More details of the search strategy are provided in the Supplementary material. Two authors (CMQ and LR) independently reviewed the titles, abstracts, and full text of the articles, and disagreements were resolved by two other researchers (SG and WZY).
Inclusion and exclusion criteria
The inclusion criteria were as follows: (1) studies that reported on PLWH receiving booster doses (≥3 doses) of the COVID-19 vaccine; (2) observational studies (including case-control and cohort studies), randomized clinical trials (RCTs), and non-RCTs; and (3) studies from which data on immunogenicity (humoral and T-cell immunity) and effectiveness (clinical outcome metrics such as associated infections, symptomatic infections, hospitalizations, and deaths) could be extracted. The excluded studies were as follows: (1) non-original articles, such as reviews, commentaries, and meta-analyses; (2) preprints; (3) studies for which data could not be obtained to calculate immune response rates (IRRs) and risk ratio (RR); and (4) those in which the interval between the booster and primary vaccination was less than 3 months or unspecified.
Definitions of IRR may vary between studies (refer to Supplementary Table S1). Booster vaccination was defined as the completion of the vaccination schedule followed by one or more injections. For multiple articles from the same cohort reporting the same results, we selected those with the largest and most recent studies.
Data extraction
Two researchers (CMQ and LR) independently extracted data based on a predetermined form in Microsoft Excel. Information collected included first author, year of publication, country, age, booster vaccine type and dose, timing after vaccination, previous vaccinations, interval between booster doses and primary vaccination, CD4+ T-cell count, antiretroviral therapy (ART), immunoassay, cellular immune response test, unit, positive response threshold, and effectiveness outcome.
Risk of bias assessment
We assessed cohort studies and case-control studies using the Newcastle-Ottawa Quality Assessment Scale (34). Two investigators (CMQ and LR) independently conducted the quality assessment. Disagreements were resolved by two additional reviewers (SG and WZY).
Outcomes of interest
The evaluation of immunological effects included both humoral and cellular immunity.
The primary outcome of humoral immunity evaluation is the immune response rate of neutralizing antibodies (nAb) and other antibodies (such as detection of anti-S protein IgG or anti-RBD IgG). The formula was calculated as the IRR = number of immune responses/number of COVID-19 vaccinations × 100%. The evaluation of cellular immunity mainly includes the level of specific T cells: CD4+, CD8+, and IFN-γ.
Effectiveness outcomes were assessed primarily in terms of COVID-19-associated infections, hospitalizations, serious illnesses, and deaths.
Data synthesis and statistical analysis
All analyses were visualized using the STATA 17 statistical software. Pooled estimates were calculated and compared using the DerSimonian and Laird method for a random effects model with a 95% confidence interval (CI) (35). Randomized control trials and observational studies were both considered for inclusion. The statistical heterogeneity of the results was calculated using the I2 statistic. An I2 statistic value >50% was considered indicative of substantial heterogeneity (36). We performed subgroup analyses by year of publication, continents, booster vaccine type, time after vaccination, interval between booster doses and primary vaccination, ART, and immunological outcomes. Q-tests were used for subgroup comparisons; variables between subgroups were considered significant when the p-value for subgroup differences was < 0.05. The publication bias was studied by visual inspection of the funnel plot symmetry as well as by Egger's test (asymmetry is considered if p < 0.05) (37). Sensitivity analysis was performed for outcomes that included more than 10 studies. p-values of < 0.05 were considered statistically significant.
Results
Characteristics of included studies
In Figure 1, we summarize the selection process, which culminated in the inclusion of 35 studies, of which 31 involved (27, 30, 31, 38–65) immunogenicity assessments (2,652 PLWH) and 4 involved (66–69) effectiveness assessments (27,502 PLWH). Of the 31 immunogenicity studies analyzed (Tables 1, 2; Supplementary Tables S1–S6), all were observational, including 1 case-control study (3.33%) and 30 cohort studies (96.77%). These studies were published in 14 (45.16%) and 17 (54.84%) in 2022 and 2023, respectively. Notably, 9 (29.03%), 17 (54.84%), and 5 (16.13%) studies were conducted in Asia, Europe, and North America, respectively. In addition, 20 (64.52%), 8 (25.81%), and 3 (9.67%) studies were conducted on mRNA vaccine, inactivated vaccine, and multivaccine, respectively. In terms of effectiveness studies, four studies were analyzed. Among the clinical outcome indicators involved, 1 (33.33%) COVID-19-related infection, 1 (33.33%) symptomatic infection, 2 (66.67%) hospitalizations, and 1 (33.33%) death were included (Table 3).
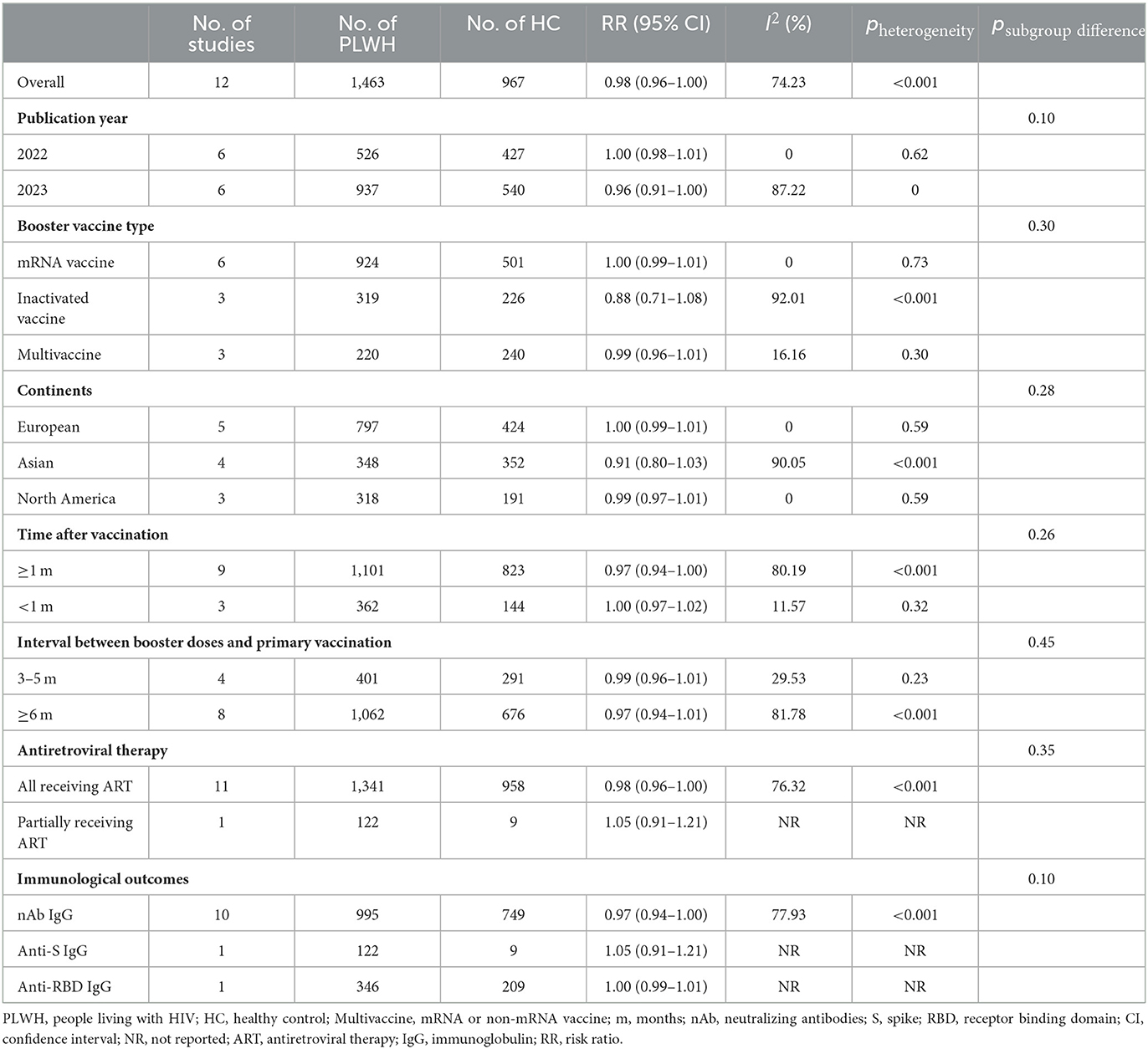
Table 2. Immune response rates of PLWH compared with HC after COVID-19 booster vaccination by subgroup.
The risk of bias was assessed for 31 meta-analyzed literature. Of these, 6 (19.35%) studies were assessed as low risk of bias, 15 (48.39%) were assessed as moderate risk of bias, and 10 (32.26%) were assessed as high risk of bias. For detailed information on the results of the risk of bias assessment, refer to Supplementary Tables S7, S8.
Immune response rates among PLWH
The pooled IRR was 97.25% (95% CI, 93.81–99.49) in 29 studies (27, 30, 31, 39, 41–65) involving 2,599 PLWH recipients of booster vaccination, with a high degree of heterogeneity between studies (I2 = 93.25%) (Table 1; Supplementary Table S2). However, subgroup analyses showed no significant differences between the groups (p ≥ 0.05). The IRRs were relatively lower in inactivated vaccine (92.48%, 95% CI, 80.95–99.20), Asian (93.68%, 95% CI, 83.24–99.54), time ≥1 m after vaccination (96.68%, 95% CI, 92.10–99.51), interval between booster doses and primary vaccination ≥6 m (95.77%, 95% CI, 89.40–99.54), and nAb type (96.17%, 95% CI, 91.03–99.41) (Table 1).
A total of seven eligible studies (43, 47, 51, 53, 54, 59, 61) involved PLWH with T-cell counts < 200, none of which had HC. The results showed a pooled IRR of 86.27% (95% CI, 65.35–99.07). A subgroup analysis showed that the IRR was significantly higher for the mRNA vaccine than for the inactivated vaccine (p < 0.05) (Figure 2; Supplementary Table S3).
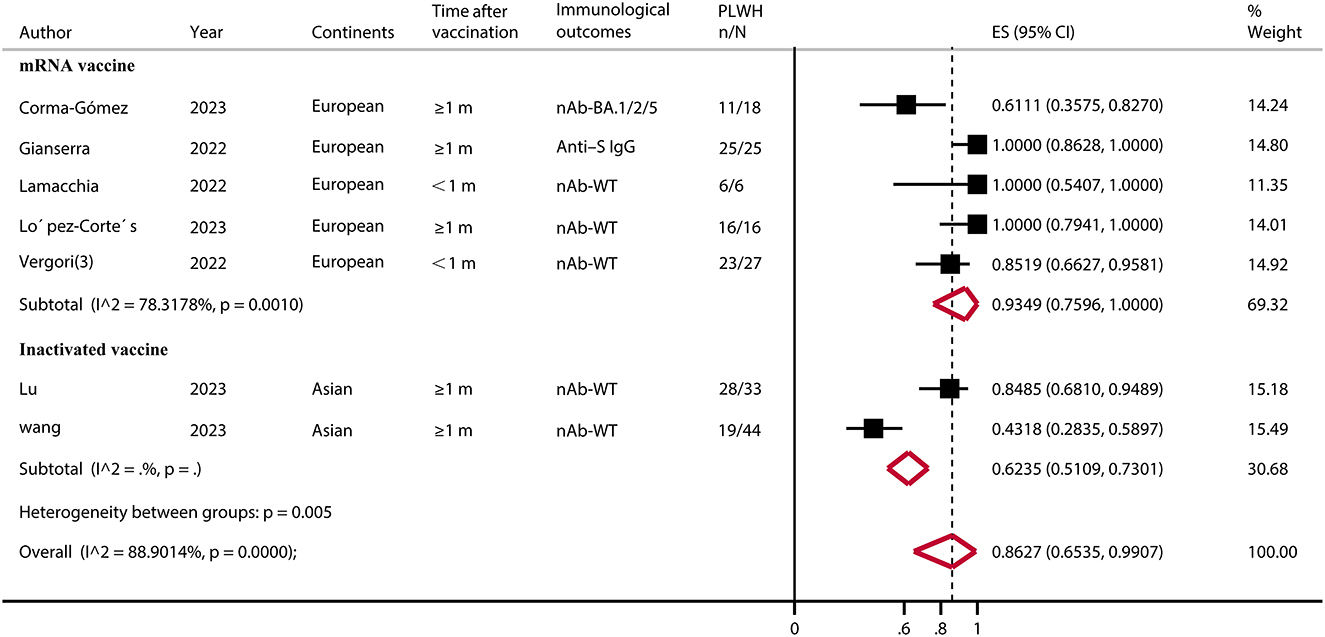
Figure 2. Immune response rate of PLWH (CD4+ T-cell count of ≤ 200) after COVID-19 booster vaccination. PLWH, people living with HIV; CI, confidence interval; m, months; ART, antiretroviral therapy; nAb, neutralizing antibodies; S, spike; IgG, immunoglobulin; WT, wild type; ES, effect size (proportions); N, total number of PLWH; n, number of immune responsed PLWH.
Comparison of immune responses between PLWH and HC
In 12 studies (27, 30, 31, 44, 50, 52, 54, 56–59, 64), consisting of 1,463 PLWH and 967 HC, there was no significant difference in IRR between PLWH and HC after the booster dose (RR = 0.98, 95% CI, 0.96–1.00). There was a high heterogeneity among the studies (I2 = 75.90%, p < 0.001) (Table 2, Supplementary Table S2). There was no significant difference in IRR between the PLWH and the HC in each subgroup (p ≥ 0.05). Of these, the PLWH booster vaccination with mRNA vaccine had the closest degree of immune response to HC (RR = 1.00, 95% CI, 0.99–1.01) and low heterogeneity (I2 = 0). In addition, a meta-analysis of 6 months after booster vaccination showed a reduction in IRR produced by PLWH compared to the healthy population, but it was not statistically significant (RR = 0.71, 95% CI, 0.35–1.42) (Supplementary Table S4).
Immune response rates of SARS-CoV-2 Omicron variant among PLWH
For the SARS-CoV-2 Omicron variant, in 13 studies (30, 42, 43, 49, 50, 52, 56, 58–62, 64) involving 1,136 PLWH who received a booster vaccination, the pooled IRR was 74.07% (95% CI, 58.83–89.30) (Figure 3; Supplementary Table S5). The IRR after booster vaccination was similar (RR = 0.90, 95% CI, 0.80–1.00) between PLWH and HC in seven studies consisting of 696 PLWH and 547 HCs. A subgroup analysis of vaccine types showed relatively high IRR and RR for mRNA vaccines (IRR = 89.88%, 95% CI, 84.75–95.00; RR = 0.98, 95% CI, 0.94–1.03) (Figure 4; Supplementary Table S5).
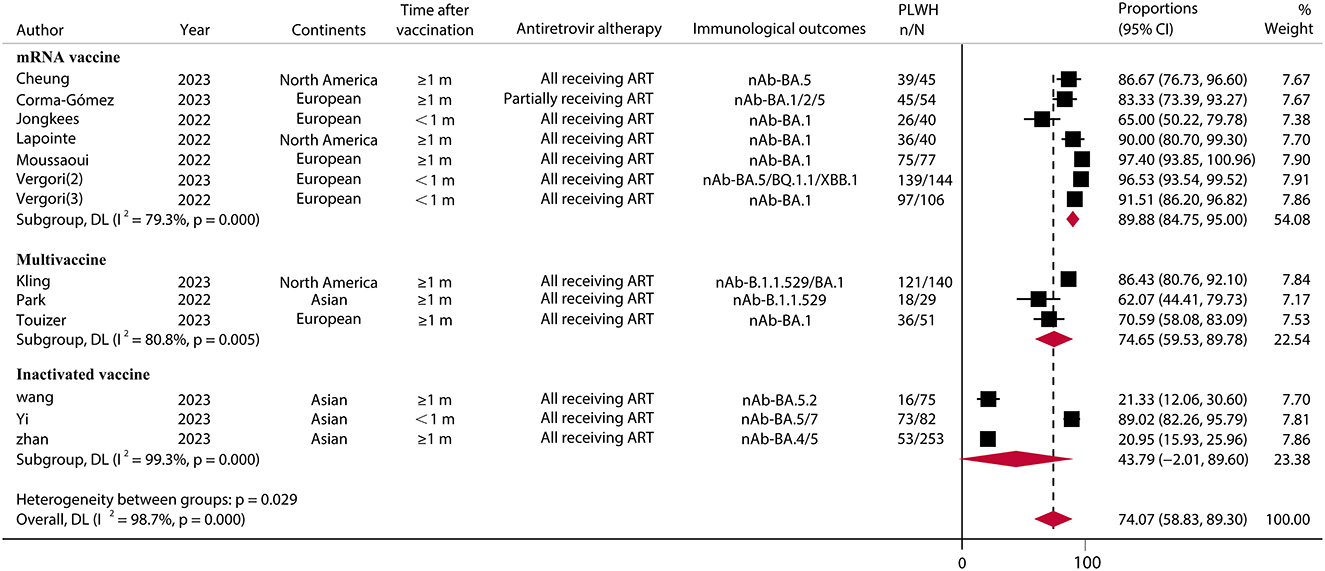
Figure 3. Immune response rates of SARS-CoV-2 omicron variants to PLWH after COVID-19 booster vaccination. PLWH, people living with HIV; CI, confidence interval; m, months; ART, antiretroviral therapy; nAb, neutralizing antibodies; N, the total number of PLWH; n, the number of PLWH with immune responses.
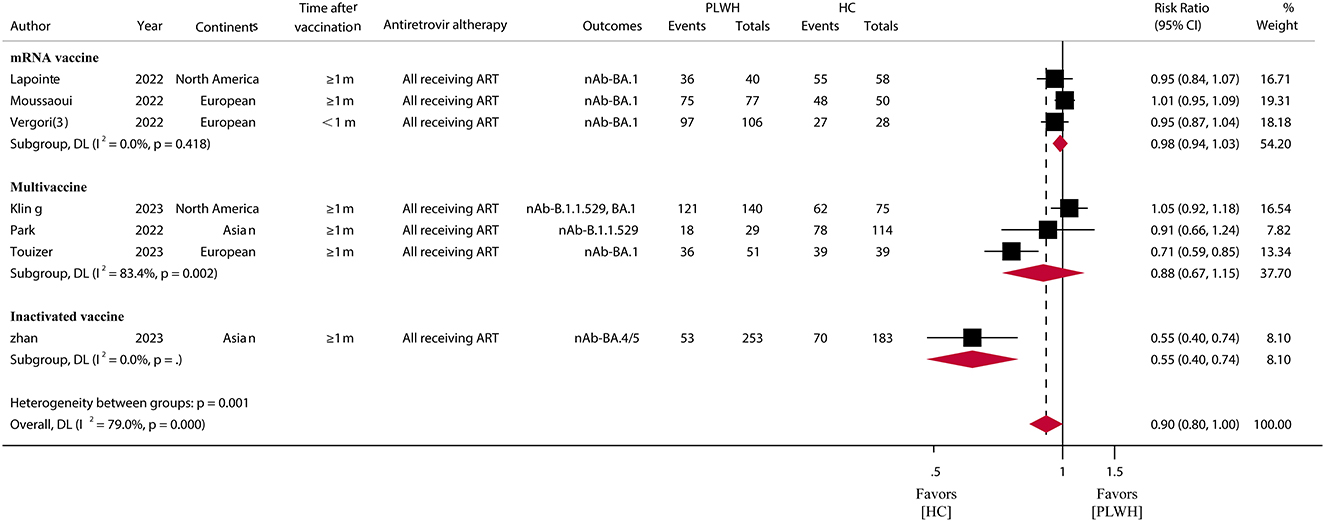
Figure 4. Immune response rates of SARS-CoV-2 omicron variants among PLWH compared to HC after COVID-19 booster vaccination. PLWH, people living with HIV; HC, healthy control; CI, confidence interval; m, months; ART, antiretroviral therapy; nAb, neutralizing antibodies.
T-cells immune responses among PLWH
In terms of T-cell immune response, a total of six eligible studies (26, 27, 30, 31, 40, 56) were included in the meta-analysis. The results showed that the immune levels of CD4+ T cells and IFN-γ were lower in PLWH than in the HC (standardized mean difference (SMD) = −0.34, 95% CI, −0.98 to 0.29; SMD = −0.20, 95% CI, −0.60 to 0.20), but there was no statistically significant difference (p > 0.05). The CD8+ T-cell levels were higher in PLWH than in the HC (SMD = 0.76, 95% CI, −0.79 to 2.31); however, there was also no statistically significant difference (p > 0.05) (Figure 5; Supplementary Table S6).
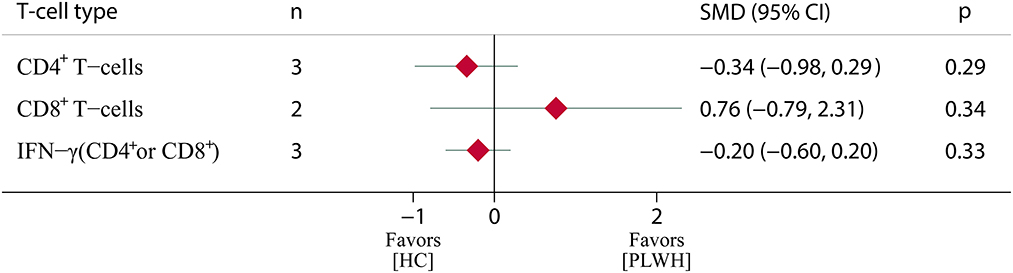
Figure 5. T-cell immune response among PLWH compared to HC after COVID-19 booster vaccination. PLWH, people living with HIV; HC, healthy control; CI, confidence interval; SMD, standardized mean difference; N, number of included studies; p < 0.05 represents a statistically significant difference.
Effectiveness of PLWH vaccination between boosters compared to primary
Four studies (66–69), including 27,502 PLWH, reported on vaccine effectiveness following the COVID-19 booster vaccination (Table 3). Of these, three cohort studies and one retrospective study were included. Two study sites were from the United States (US), one from Denmark, and one from Poland. Unfortunately, none were compared with healthy populations. Our results showed that the risk of COVID-19-associated infections, hospitalization, and death appeared to be reduced in PLWH who received a booster dose compared to the primary vaccine.
Publication bias and sensitivity analysis
Funnel plots and Egger's test indicated a possible publication bias in the meta-analysis of immune response to the original strains after receiving booster doses of the COVID-19 vaccine (test result: t = −2.93, df = 11, p = 0.0167). To address the issue of publication bias, the trim-and-fill method was used, and the adjusted risk (RR = 1.00, 95% CI, 0.94–1.01) was very close to the original result (RR = 0.98, 95% CI, 0.96–1.00) (Supplementary Figure S1).
To explore the stability of meta-analysis results, sensitivity analysis was performed by excluding each study one by one. For the immune response rate among PLWH compared to HC, after excluding some literature, the results showed that the immune-antibody response of PLWH may be lower than that of the healthy population (Supplementary Figure S2).
Discussion
This systematic review and meta-analysis provides a comprehensive analysis of the immunogenicity and effectiveness of COVID-19 booster vaccination in PLWH using data from 35 eligible studies and more than 30,000 PLWH individuals with important implications for the field. Our study showed that PLWH had an IRR of 97.25% after COVID-19 booster immunization. It provided a moderate immune response even in PLWH with low CD4+ T-cell counts. Importantly, the humoral and cellular immune response to COVID-19 booster immunization was comparable in PLWH compared to HC. The immune response rate was reduced in the face of the Omicron variant but was comparable compared to HC. In terms of effectiveness evaluation, booster vaccination appears to be effective in reducing the risk of COVID-19 infection, hospitalization, and death in PLWH.
There is less evidence-based research regarding booster doses of the COVID-19 vaccine for PLWH, and so far we have found only one study addressing this (18). The meta-analysis study by Zhou et al. (18), which included six studies, calculated a seroconversion rate of 98.4% (95% CI, 94.8–100%) after vaccine boosters for PLWH, and in three of these studies, PLWH had a reduced antibody response compared to the HC (RR = 0.97, 95% CI, 0.94–0.99). Our findings suggest that PLWH vaccinated with vaccine boosters produced humoral immune responses comparable to those of the healthy population (RR = 0.98, 95% CI, 0.96–1.00). However, it is worth noting that the immune response to PLWH may be weaker than that of the healthy population after study-by-study exclusion in stability analyses. In comparison to Zhou et al. (18), our study delved into a more extensive and detailed analysis. We explored various aspects such as subgroup analysis, antibody responses to the Omicron variant, and T-cell immune responses.
In the subgroup analysis, we only found that immunological outcomes may have a significant effect on the pooled immune response rate (pbetween groups= 0.005). The subgroup analysis of immunological outcomes showed that the immune response rate of nAb was lower than that of other types of antibodies. Previous studies have suggested that inactivated vaccine recipients may be at higher risk of breakthrough infections compared to mRNA vaccines (70, 71). This may also be true in PLWH as our study showed a relatively high immune response to the mRNA vaccine and a relatively weak response to the inactivated vaccine. There is a correlation between the strength of the antibody response and clinical endpoints. In terms of study sites, the pooled IRR was lower in Asia and relatively higher in Europe and North America. This may be due to differences in the type of vaccination, with the majority of vaccines administered in Asian countries being inactivated, but ethnic differences cannot be ruled out (72). A subgroup analysis of vaccination intervals and time to immunoassay after booster showed no significant differences between groups (p > 0.05). The results indirectly suggest that vaccine boosters given 3 months after primary vaccination can also produce a relatively favorable immune response. Furthermore, it has been shown that under an effective ART, SARS-CoV-2-infected PLWH have good immune recovery and adequate virological control to generate humoral and T-cell immune responses similar to those of HC (30, 38). In this regard, our findings are similar.
CD4+ T-cell counts have been shown to be negatively associated with increased morbidity and mortality in COVID-19 (43, 59). Our results showed that the IRR of PLWH with low CD4+ T-cell levels ( ≤ 200 per μl) after vaccine boosters was 86.27% (95% CI, 65.35–99.07). Critically, subgroups showed that the mRNA vaccine still produced a high antibody response (IRR = 93.49%, 95% CI, 75.96–100.00). mRNA vaccines may provide effective antibody protection in severely immunosuppressed PLWH. However, more data support from studies comparing healthy populations is still needed.
Although the emergence of Omicron variants has been associated with a reduction in the severity of COVID-19 (73), recent data suggest that immunocompromised patients are still at high risk of COVID-19 morbidity and mortality, which may be due to the lack of vaccine efficacy in this population (74). Our study showed that vaccine boosters in PLWH provided moderate protection against immune responses in the face of Omicron variants. However, the risk of immune protection was reduced by 10% (RR = 0.90, 95% CI, 0.80–1.00) compared to the healthy population, but there was no statistically significant difference (p = 0.05).
While the level of nAb is strongly associated with the protective efficacy of vaccines (75, 76), the massive activation and expansion of antigen-specific CD4+ and CD8+ T cells are also critical for improving the immune duration and immune memory (77, 78). The immune response of T cells can be divided into two main categories: CD8+ and CD4+ T-cell responses. CD4+ lymphocytes are a group of “helper” T cells that mediate the activity of other immune cells through direct and indirect mechanisms. CD8+ T cells, on the other hand, play an important role in attenuating, controlling, and removing intracellular pathogens. Among the included T-cell studies, 3, 2, and 3 studies involved CD4+, CD8+, and IFN-γ, respectively. The results showed comparable T-cell immune responses in PLWH compared to the healthy population (p > 0.05). Of course, this may be attributed to the fact that the included studies were mRNA vaccine-related. Polvere et al. (79) demonstrated that the second vaccination of mRNA vaccine elicits B-cell responses that are quantitatively similar between PLWH and HC, but there are important differences in terms of antibody functionality and phenotypes of memory B cells. More evidence is needed to support changes in B-cell antibody function in PLWH after booster vaccination with COVID-19.
There are fewer studies on the effectiveness of vaccine boosters for PLWH, and no studies with healthy populations as controls were identified, so we included studies comparing only with primary vaccination. It was found that PLWH receiving booster doses appeared to have a reduced risk of COVID-19 infections, hospitalization, and death. Previous studies vaccinating PLWH with influenza (80), hepatitis B (81), and pneumococcal (82) vaccines have demonstrated the presence of a poorer initial antibody response, shorter persistence of the immune response, and more breakthrough infections. The translation of a poorer immune response may have poorer clinical outcomes (70, 75). However, our study showed that booster vaccination has a high and durable immune response in PLWH, and in combination with the currently limited evidence of efficacy, PLWH may benefit from booster vaccinations. However, more research evidence is needed to support this.
There are several limitations to this study. First, the studies included in the literature were observational. Although the researchers attempted to control for potential confounders in the analysis, there may still be unknown confounders that could have affected the results of the study. Furthermore, the use of different immunoassay kits and immunoreactivity thresholds may have biased the results. Predicting protection against COVID-19 has been the subject of debate, and given the paucity of data on clinical efficacy endpoints, nAb levels have recently been recognized as a reliable predictor of protection against COVID-19. In the present study, serum nAb data were preferentially included, and if nAb data were not available, other antibodies, such as Spike-IgG or RBD-IgG antibody data, were included. Additionally, most of the included studies were rated as having a moderate or severe risk of bias, which may affect the reliability and generalizability of the results. Attention also needs to be paid to the presence of instability in the sensitivity analyses, and the results need to be interpreted with caution. Finally, there may be differences in data sources, such as studies eligible for inclusion that were not conducted in Africa, and results from the African PLWH cohort should be interpreted with caution.
Conclusion
It was shown that booster vaccination with the COVID-19 vaccine provided a high IRR in PLWH and still produced a desirable moderate IRR in PLWH with a CD4+ T-cell count of ≤ 200. Importantly, the humoral and T-cell responses to booster vaccination in PLWH were comparable to HC, and similar results were observed with the SARS-CoV-2 Omicron variant. Our review strongly emphasizes the effect of mRNA vaccine booster vaccination on PLWH in eliciting desirable protective IRR. Furthermore, booster vaccination appears to further reduce the risk of COVID-19 infection, hospitalization, and death in PLWH compared to primary vaccination. However, more evidence is needed to confirm its effectiveness.
Data availability statement
The original contributions presented in the study are included in the article/Supplementary material, further inquiries can be directed to the corresponding authors.
Author contributions
M-QC: Conceptualization, Data curation, Visualization, Validation, Writing—original draft, Writing—review and editing. RL: Data curation, Formal analysis, Methodology, Validation, Writing—review and editing. Z-YW: Conceptualization, Investigation, Project administration, Resources, Supervision, Writing—review and editing. GS: Conceptualization, Data curation, Formal analysis, Funding acquisition, Investigation, Project administration, Resources, Software, Supervision, Visualization, Writing—original draft, Writing—review and editing.
Funding
The author(s) declare that financial support was received for the research, authorship, and/or publication of this article. This study was funded by the Yunnan Provincial Department of Science and Technology and the Kunming Medical University Joint Foundation for Applied Basic Research (No. 202201AY070001-294).
Conflict of interest
The authors declare that the research was conducted in the absence of any commercial or financial relationships that could be construed as a potential conflict of interest.
Publisher's note
All claims expressed in this article are solely those of the authors and do not necessarily represent those of their affiliated organizations, or those of the publisher, the editors and the reviewers. Any product that may be evaluated in this article, or claim that may be made by its manufacturer, is not guaranteed or endorsed by the publisher.
Supplementary material
The Supplementary Material for this article can be found online at: https://www.frontiersin.org/articles/10.3389/fmed.2023.1275843/full#supplementary-material
References
1. Meng Y, Irwin DM, Shen Y. Ecology of SARS-CoV-2 in the post-pandemic era. Lancet Microbe. (2023) 4:e208. doi: 10.1016/S2666-5247(22)00361-5
2. Terpos E, Musto P, Engelhardt M, Delforge M, Cook G, Gay F, et al. Management of patients with multiple myeloma and COVID-19 in the post pandemic era: a consensus paper from the European Myeloma Network (EMN). Leukemia. (2023) 37:1175–85. doi: 10.1038/s41375-023-01920-1
3. Wu N, Joyal-Desmarais K, Ribeiro PAB, Vieira AM, Stojanovic J, Sanuade C, et al. Long-term effectiveness of COVID-19 vaccines against infections, hospitalisations, and mortality in adults: findings from a rapid living systematic evidence synthesis and meta-analysis up to December, 2022. Lancet Respir Med. (2023) 11:439–52. doi: 10.1016/S2213-2600(23)00015-2
4. Fung M, Babik JM. COVID-19 in Immunocompromised Hosts: What We Know So Far. Clin Infect Dis. (2021) 72:340–50. doi: 10.1093/cid/ciaa863
5. Mirzaei H, McFarland W, Karamouzian M, Sharifi H. COVID-19 Among People Living with HIV: A Systematic Review. AIDS Behav. (2021) 25:85–92. doi: 10.1007/s10461-020-02983-2xs
6. Spinelli MA, Jones BLH, Gandhi M. COVID-19 outcomes and risk factors among people living with HIV. Curr HIV/AIDS Rep. (2022) 19:425–32. doi: 10.1007/s11904-022-00618-w
7. Geretti AM, Stockdale AJ, Kelly SH, Cevik M, Collins S, Waters L, et al. Outcomes of Coronavirus disease 2019 (COVID-19) related hospitalization among people with human immunodeficiency virus (HIV) in the ISARIC world health organization (WHO) clinical characterization protocol (UK): a prospective observational study. Clin Infect Dis. (2021) 73:e2095–106. doi: 10.1093/cid/ciaa1605
8. Shields AM, Tadros S, Al-Hakim A, Nell JM, Lin MMN, Chan M, et al. Impact of vaccination on hospitalization and mortality from COVID-19 in patients with primary and secondary immunodeficiency: the United Kingdom experience. Front Immunol. (2022) 13:984376. doi: 10.3389/fimmu.2022.984376
9. Kanwugu ON, Adadi P. HIV/SARS-CoV-2 coinfection: a global perspective. J Med Virol. (2021) 93:726–32. doi: 10.1002/jmv.26321
10. Karim F, Gazy I, Cele S, Zungu Y, Krause R, Bernstein M, et al. HIV status alters disease severity and immune cell responses in Beta variant SARS-CoV-2 infection wave. Elife. (2021) 10:e67397. doi: 10.7554/eLife.67397
11. Yang X, Sun J, Patel RC, Zhang J, Guo S, Zheng Q, et al. Associations between HIV infection and clinical spectrum of COVID-19: a population level analysis based on US National COVID Cohort Collaborative (N3C) data. Lancet HIV. (2021) 8:e690–700. doi: 10.1016/S2352-3018(21)00239-3
12. Western Cape Department of Health in collaboration with the National Institute for Communicable Diseases, South Africa. Risk factors for coronavirus disease 2019 (COVID-19) death in a population cohort study from the Western Cape Province, South Africa. Clin Infect Dis. (2021) 73:e2005–015. doi: 10.1093/cid/ciaa1198
13. Nomah DK, Reyes-Urueña J, Díaz Y, Moreno S, Aceiton J, Bruguera A, et al. Sociodemographic, clinical, and immunological factors associated with SARS-CoV-2 diagnosis and severe COVID-19 outcomes in people living with HIV: a retrospective cohort study. Lancet HIV. (2021) 8:e701–10. doi: 10.1016/S2352-3018(21)00240-X
14. Spinelli MA, Lynch KL, Yun C, Glidden DV, Peluso MJ, Henrich TJ, et al. SARS-CoV-2 seroprevalence, and IgG concentration and pseudovirus neutralising antibody titres after infection, compared by HIV status: a matched case-control observational study. Lancet HIV. (2021) 8:e334–41. doi: 10.1016/S2352-3018(21)00072-2
15. Griffin DWJ, Pai Mangalore R, Hoy JF, McMahon JH. Immunogenicity, effectiveness, and safety of SARS-CoV-2 vaccination in people with HIV. AIDS. (2023) 37:1345–60. doi: 10.1097/QAD.0000000000003579
16. Kang L, Shang W, Gao P, Wang Y, Liu J, Liu M. Immunogenicity and safety of COVID-19 vaccines among people living with HIV: a systematic review and meta-analysis. Vaccines. (2022) 10:1569. doi: 10.3390/vaccines10091569
17. Yin J, Chen Y, Li Y, Wang C, Zhang X. Immunogenicity and efficacy of COVID-19 vaccines in people living with HIV: a systematic review and meta-analysis. Int J Infect Dis. (2022) 124:212–23. doi: 10.1016/j.ijid.2022.10.005
18. Zhou Q, Zeng F, Meng Y, Liu Y, Liu H, Deng G. Serological response following COVID-19 vaccines in patients living with HIV: a dose-response meta-analysis. Sci Rep. (2023) 13:9893. doi: 10.1038/s41598-023-37051-x
19. Byrd KM, Beckwith CG, Garland JM, Johnson JE, Aung S, Cu-Uvin S, et al. SARS-CoV-2 and HIV coinfection: clinical experience from Rhode Island, United States. J Int AIDS Soc. (2020) 23:e25573. doi: 10.1002/jia2.25573
20. Vizcarra P, Pérez-Elías MJ, Quereda C, Moreno A, Vivancos MJ, Dronda F, et al. Description of COVID-19 in HIV-infected individuals: a single-centre, prospective cohort. Lancet HIV. (2020) 7:e554–64. doi: 10.1016/S2352-3018(20)30164-8
21. Bhaskaran K, Rentsch CT, MacKenna B, Schultze A, Mehrkar A, Bates CJ, et al. HIV infection and COVID-19 death: a population-based cohort analysis of UK primary care data and linked national death registrations within the OpenSAFELY platform. Lancet HIV. (2021) 8:e24–32. doi: 10.1016/S2352-3018(20)30305-2
22. Danwang C, Noubiap JJ, Robert A, Yombi JC. Outcomes of patients with HIV and COVID-19 co-infection: a systematic review and meta-analysis. AIDS Res Ther. (2022) 19:3. doi: 10.1186/s12981-021-00427-y
23. Lafont E, Pere H, Lebeaux D, Cheminet G, Thervet E, Guillemain R, et al. Targeted SARS-CoV-2 treatment is associated with decreased mortality in immunocompromised patients with COVID-19. J Antimicrob Chemother. (2022) 77:2688–92. doi: 10.1093/jac/dkac253
24. Totschnig D, Augustin M, Niculescu I, Laferl H, Jansen-Skoupy S, Lehmann C, et al. SARS-CoV-2 pre-exposure prophylaxis with sotrovimab and tixagevimab/cilgavimab in immunocompromised patients-a single-center experience. Viruses. (2022) 14:2278. doi: 10.3390/v14102278
25. Carreño JM, Alshammary H, Tcheou J, Singh G, Raskin AJ, Kawabata H, et al. Activity of convalescent and vaccine serum against SARS-CoV-2 Omicron. Nature. (2022) 602:682–8. doi: 10.1038/s41586-022-04399-5
26. Alexandrova Y, Costiniuk CT, Jenabian MA. Pulmonary immune dysregulation and viral persistence during HIV infection. Front Immunol. (2022) 12:808722. doi: 10.3389/fimmu.2021.808722
27. Heftdal LD, Pérez-Alós L, Hasselbalch RB, Hansen CB, Hamm SR, Møller DL, et al. Humoral and cellular immune responses eleven months after the third dose of BNT162b2 an mRNA-based COVID-19 vaccine in people with HIV - a prospective observational cohort study. EBioMedicine. (2023) 93:104661. doi: 10.1016/j.ebiom.2023.104661
28. Lombardi F, Belmonti S, Fabbiani M, Morandi M, Rossetti B, Tordini G, et al. Immunogenicity and Safety of the 13-Valent pneumococcal conjugate vaccine versus the 23-valent polysaccharide vaccine in unvaccinated HIV-infected adults: a pilot, prospective controlled study. PLoS ONE. (2016) 11:e0156523. doi: 10.1371/journal.pone.0156523
29. Fabbiani M, Sidella L, Corbi M, Martucci R, Sali M, Colafigli M, et al. HIV-infected patients show impaired cellular immune response to influenza vaccination compared to healthy subjects. Vaccine. (2013) 31:2914–8. doi: 10.1016/j.vaccine.2013.04.033
30. Moussaoui ME, Desmecht S, Tashkeev A, Lambert N, Maes N, Braghini J, et al. Reduced T-cell response following a third dose of SARS-CoV-2 vaccine in infection-naïve people living with HIV. J Infect. (2022) 85:702–69. doi: 10.1016/j.jinf.2022.09.006
31. Vergori A, Cozzi Lepri A, Cicalini S, Matusali G, Bordoni V, Lanini S, et al. Immunogenicity to COVID-19 mRNA vaccine third dose in people living with HIV. Nat Commun. (2022) 13:4922. doi: 10.1038/s41467-022-32263-7
32. Alrubayyi A, Gea-Mallorquí E, Touizer E, Hameiri-Bowen D, Kopycinski J, Charlton B, et al. Characterization of humoral and SARS-CoV-2 specific T cell responses in people living with HIV. Nat Commun. (2021) 12:5839. doi: 10.1038/s41467-021-26137-7
33. Page MJ, McKenzie JE, Bossuyt PM, Boutron I, Hoffmann TC, Mulrow CD, et al. The PRISMA 2020 statement: an updated guideline for reporting systematic reviews. BMJ. (2021) 372:n71. doi: 10.1136/bmj.n71
34. Wells G, Shea B, O'Connell D, Peterson J, Welch V, Lososet M, et al. The Newcastle-Ottawa Scale (NOS) for Assessing the Quality of Non-randomised Studies in Meta-analyses. (2007). Available online at: https://www.ohri.ca/programs/clinical_epidemiology/oxford.asp (accessed Sepember 11, 2023).
35. Nyaga VN, Arbyn M, Aerts M. Metaprop: a stata command to perform meta-analysis of binomial data. Arch Public Health. (2014) 72:39. doi: 10.1186/2049-3258-72-39
36. Higgins JP, Thompson SG. Quantifying heterogeneity in a meta-analysis. Stat Med. (2002) 21:1539–58. doi: 10.1002/sim.1186
37. Duval S, Tweedie R. Trim and fill: A simple funnel-plot-based method of testing and adjusting for publication bias in meta-analysis. Biometrics. (2000) 56:455–63. doi: 10.1111/j.0006-341x.2000.00455.x
38. Alexandrova Y, Yero A, Mboumba Bouassa RS, Comeau E, Samarani S, Brumme ZL, et al. SARS-CoV-2 vaccine-induced T-cell response after three doses in people living with HIV on antiretroviral therapy compared to seronegative controls (CTN 328 COVAXHIV Study). Viruses. (2023) 15:575. doi: 10.3390/v15020575
39. Basso M, Pirola N, Pascoli S, Bragato B, Vinci A, Iannetta M, et al. Humoral Response after Two Doses of BNT162b2 mRNA vaccine has a role in predicting response after three doses that is related to plasma HIV viremia and nadir CD4+ Cell Count in HIV-positive patients. Vaccines. (2022) 11:82. doi: 10.3390/vaccines11010082
40. Bessen C, Plaza-Sirvent C, Simsek A, Bhat J, Marheinecke C, Urlaub D, et al. Impact of SARS-CoV-2 vaccination on systemic immune responses in people living with HIV. Front Immunol. (2022) 13:1049070. doi: 10.3389/fimmu.2022.1049070
41. Chan DPC, Wong NS, Wong BCK, Chan JMC, Lee SS. Three-dose primary series of inactivated COVID-19 vaccine for persons living with HIV, Hong Kong. Emerg Infect Dis. (2022) 28:2130–2. doi: 10.3201/eid2810.220691
42. Cheung PK, Lapointe HR, Sang Y, Ennis S, Mwimanzi F, Speckmaier S, et al. SARS-CoV-2 live virus neutralization after four COVID-19 vaccine doses in people with HIV receiving suppressive antiretroviral therapy. AIDS. (2023) 37:F11–8. doi: 10.1097/QAD.0000000000003519
43. Corma-Gómez A, Fernández-Fuertes M, Viñuela L, Domínguez C, Santos M, Fuentes-López A, et al. Reduced neutralizing antibody response to SARS-CoV-2 vaccine booster dose in people living with HIV with severe immunosuppression. J Med Virol. (2023) 95:e28602. doi: 10.1002/jmv.28602
44. Costiniuk CT, Singer J, Lee T, Langlois MA, Arnold C, Galipeau Y, et al. COVID-19 vaccine immunogenicity in people with HIV. AIDS. (2023) 37:F1–10. doi: 10.1097/QAD.0000000000003429
45. Fidler S, Fox J, Tipoe T, Longet S, Tipton T, Abeywickrema M, et al. Booster vaccination against SARS-CoV-2 induces potent immune responses in people with human immunodeficiency virus. Clin Infect Dis. (2023) 76:201–9. doi: 10.1093/cid/ciac796
46. Fusco FM, Carleo MA, Sangiovanni N, D'Abbraccio M, Tambaro O, Borrelli F, et al. Does COVID-19 vaccination with BNT162b2 influence HIV-related immunological and virological markers? Data from 235 persons living with HIV at cotugno hospital, Naples, Italy: immune response after second and third doses, and influence on immunovirological markers. Viral Immunol. (2023) 36:360–5. doi: 10.1089/vim.2022.0182
47. Gianserra L, Donà MG, Giuliani E, Stingone C, Pontone M, Buonomini AR, et al. Immunogenicity and safety of BNT162b2 homologous booster vaccination in people living with HIV under effective cART. Vaccines. (2022) 10:1243. doi: 10.3390/vaccines10081243
48. Hassold N, Brichler S, Gater Y, Leclerc D, Gordien E, Bouchaud O, et al. Mixed outcomes following a third SARS-CoV-2 vaccine dose in previously unresponsive people with HIV. J Clin Virol. (2023) 158:105347. doi: 10.1016/j.jcv.2022.105347
49. Jongkees MJ, Geers D, Hensley KS, Huisman W, GeurtsvanKessel CH, Bogers S, et al. Immunogenicity of an additional mRNA-1273 SARS-CoV-2 vaccination in people with HIV with hyporesponse after primary vaccination. J Infect Dis. (2023) 227:651–62. doi: 10.1093/infdis/jiac451
50. Kling KD, Janulis P, Demonbreun AR, Sancilio A, Berzins B, Krueger K, et al. No difference in anti-spike antibody and surrogate viral neutralization following SARS-CoV-2 booster vaccination in persons with HIV compared to controls (CO-HIV Study). Front Immunol. (2023) 13:1048776. doi: 10.3389/fimmu.2022.1048776
51. Lamacchia G, Salvati L, Kiros ST, Mazzoni A, Vanni A, Capone M, et al. Fourth Dose of mRNA COVID-19 vaccine transiently reactivates spike-specific immunological memory in people living with HIV (PLWH). Biomedicines. (2022) 10:3261. doi: 10.3390/biomedicines10123261
52. Lapointe HR, Mwimanzi F, Cheung PK, Sang Y, Yaseen F, Umviligihozo G, et al. People with human immunodeficiency virus receiving suppressive antiretroviral therapy show typical antibody durability after dual coronavirus disease 2019 vaccination and strong third dose responses. J Infect Dis. (2023) 227:838–49. doi: 10.1093/infdis/jiac229
53. López-Cortés LF, Saborido-Alconchel A, Trujillo-Rodríguez M, Serna-Gallego A, Llaves-Flores S, Muñoz-Muela E, et al. Humoral and cellular immunity to SARS-CoV-2 after vaccination with mRNA vaccines in PLWH with discordant immune response. Influence of the vaccine administered. Front Immunol. (2023) 14:1129753. doi: 10.3389/fimmu.2023.1129753
54. Lu T, Chen Z, Cao Y, Ao L, Li Z, Gu X, et al. Dynamic immunogenicity after primary and booster inactivated SARS-CoV-2 vaccination in people living with HIV: a longitudinal observational study. J Med Virol. (2023) 95:e28730. doi: 10.1002/jmv.28730
55. Malin JJ, Suárez I, Biehl LM, Schommers P, Knops E, Di Cristanziano V, et al. Immune response to mRNA-based COVID-19 booster vaccination in people living with HIV. HIV Med. (2023) 24:785–93. doi: 10.1111/hiv.13481
56. Park JH, Chung H, Kim MC, Choi SH, Chung JW. Immune responses against the omicron variant of SARS-CoV-2 after a third dose of COVID-19 vaccine in patients living with human immunodeficiency virus (PLWH): comparison with healthcare workers. Vaccines. (2022) 10:2129. doi: 10.3390/vaccines10122129
57. Tan Y, Zou S, Ming F, Zhang Z, Xing Z, Wu S, et al. Early efficacy and safety of the third dose inactivated COVID-19 vaccine among people living with HIV. J Acquir Immune Defic Syndr. (2022) 90:e1–3. doi: 10.1097/QAI.0000000000002953
58. Touizer E, Alrubayyi A, Ford R, Hussain N, Gerber PP, Shum HL, et al. Attenuated humoral responses in HIV after SARS-CoV-2 vaccination linked to B cell defects and altered immune profiles. iScience. (2023) 26:105862. doi: 10.1016/j.isci.2022.105862
59. Vergori A, Cozzi-Lepri A, Matusali G, Colavita F, Cicalini S, Gallì P, et al. SARS-CoV-2 omicron variant neutralization after third dose vaccination in PLWH. Viruses. (2022) 14:1710. doi: 10.3390/v14081710
60. Vergori A, Matusali G, Lepri AC, Cimini E, Fusto M, Colavita F, et al. Neutralizing activity and T-cell response after bivalent fifth dose of messenger RNA vaccine in people living with HIV. Int J Infect Dis. (2023) 134:195–9. doi: 10.1016/j.ijid.2023.06.010
61. Wang Y, Li J, Zhang W, Liu S, Miao L, Li Z, et al. Extending the dosing interval of COVID-19 vaccination leads to higher rates of seroconversion in people living with HIV. Front Immunol. (2023) 14:1152695. doi: 10.3389/fimmu.2023.1152695
62. Yi Y, Han X, Cui X, Wang P, Wang X, Liu H, et al. Safety and immunogenicity of the inactivated COVID-19 vaccine booster in people living with HIV in China. Vaccines (Basel). (2023) 11:1019. doi: 10.3390/vaccines11061019
63. Zeng G, He F, Zhang X, Li G, Wang X, Gan Y, et al. Antibody response to the third dose of inactivated COVID-19 vaccine in people living with HIV (PLWH): a longitudinal cohort. J Med Virol. (2023) 95:e28797. doi: 10.1002/jmv.28797
64. Zhan H, Gao H, Liu Y, Zhang X, Li H, Li X, et al. Booster shot of inactivated SARS-CoV-2 vaccine induces potent immune responses in people living with HIV. J Med Virol. (2023) 95:e28428. doi: 10.1002/jmv.28428
65. Zhang W, Liu S, Miao L, Fu A, Bao J, Zheng L, et al. Dynamics of CD4+ T-cells and neutralizing antibody responses to three consecutive doses of inactivated COVID-19 vaccines in PLWH. Infect Drug Resist. (2023) 16:2695–707. doi: 10.2147/IDR.S409147
66. Bieńkowski C, Skrzat-Klapaczyńska A, Firlag-Burkacka E, Horban A, Kowalska JD. The clinical effectiveness and safety of vaccinations against COVID-19 in HIV-positive patients: data from observational study in Poland. Vaccines. (2023) 11:514. doi: 10.3390/vaccines11030514
67. Coburn SB, Humes E, Lang R, Stewart C, Hogan BC, Gebo KA, et al. Analysis of post-vaccination breakthrough COVID-19 infections among adults with HIV in the United States. JAMA Netw Open. (2022) 5:e2215934. doi: 10.1001/jamanetworkopen.2022.15934
68. Rasmussen LD, Cowan S, Gerstoft J, Kronborg G, Johansen IS, Larsen CS, et al. Outcomes following severe acute respiratory syndrome coronavirus 2 infection among individuals with and without HIV in Denmark. AIDS. (2023) 37:311–21. doi: 10.1097/QAD.0000000000003393
69. Rosenthal EM, Patterson W, Chicoine J, Dorabawila V, Adamashvili N, Rajulu DT, et al. COVID-19 vaccination and hospitalization among persons living with diagnosed HIV in New York State. J Acquir Immune Defic Syndr. (2023) 93:92–100. doi: 10.1097/QAI.0000000000003177
70. Wu JD Li JX, Liu J, Wang HM, Zhou GH Li J, et al. Safety, immunogenicity, and efficacy of the mRNA vaccine CS-2034 as a heterologous booster versus homologous booster with BBIBP-CorV in adults aged ≥18 years: a randomised, double-blind, phase 2b trial. Lancet Infect Dis. (2023) 23:1020–30. doi: 10.1016/S1473-3099(23)00199-8
71. Peng Q, Zhou R, Wang Y, Zhao M, Liu N, Li S, et al. Waning immune responses against SARS-CoV-2 variants of concern among vaccinees in Hong Kong. EBioMedicine. (2022) 77:103904. doi: 10.1016/j.ebiom.2022.103904
72. Valier MR, Elam-Evans LD, Mu Y, Santibanez TA, Yankey D, Zhou T, et al. Racial and ethnic differences in COVID-19 vaccination coverage among children and adolescents aged 5–17 years and parental intent to vaccinate their children - National immunization survey-child COVID Module, United States, December 2020-September 2022. MMWR Morb Mortal Wkly Rep. (2023) 72:1–8. doi: 10.15585/mmwr.mm7201a1
73. Ulloa AC, Buchan SA, Daneman N, Brown KA. Estimates of SARS-CoV-2 Omicron Variant Severity in Ontario, Canada. JAMA. (2022) 327:1286–8. doi: 10.1001/jama.2022.2274
74. Al Jurdi A, Gassen RB, Borges TJ, Lape IT, Morena L, Efe O, et al. Suboptimal antibody response against SARS-CoV-2 Omicron variant after third dose of mRNA vaccine in kidney transplant recipients. Kidney Int. (2022) 101:1282–6. doi: 10.1016/j.kint.2022.04.009
75. Khoury DS, Cromer D, Reynaldi A, Schlub TE, Wheatley AK, Juno JA, et al. Neutralizing antibody levels are highly predictive of immune protection from symptomatic SARS-CoV-2 infection. Nat Med. (2021) 27:1205–11. doi: 10.1038/s41591-021-01377-8
76. Earle KA, Ambrosino DM, Fiore-Gartland A, Goldblatt D, Gilbert PB, Siber GR, et al. Evidence for antibody as a protective correlate for COVID-19 vaccines. Vaccine. (2021) 39:4423–8. doi: 10.1016/j.vaccine.2021.05.063
77. Palm AE, Henry C. Remembrance of things past: long-term B cell memory after infection and vaccination. Front Immunol. (2019) 10:1787. doi: 10.3389/fimmu.2019.01787
78. Dan JM, Mateus J, Kato Y, Hastie KM, Yu ED, Faliti CE, et al. Immunological memory to SARS-CoV-2 assessed for up to 8 months after infection. Science. (2021) 371:eabf4063. doi: 10.1126/science.abf4063
79. Polvere J, Fabbiani M, Pastore G, Rancan I, Rossetti B, Durante M, et al. B cell response after SARS-CoV-2 mRNA vaccination in people living with HIV. Commun Med. (2023) 3:13. doi: 10.1038/s43856-023-00245-5
80. Zhang W, Sun H, Atiquzzaman M, Sou J, Anis AH, Cooper C. Influenza vaccination for HIV-positive people: systematic review and network meta-analysis. Vaccine. (2018) 36:4077–86. doi: 10.1016/j.vaccine.2018.05.077
81. Farooq PD, Sherman KE. Hepatitis B Vaccination and waning hepatitis B immunity in persons living with HIV. Curr HIV/AIDS Rep. (2019) 16:395–403. doi: 10.1007/s11904-019-00461-6
Keywords: COVID-19 vaccines, booster vaccination, immunogenicity, effectiveness, meta-analysis, systematic review, people living with HIV
Citation: Cheng M-Q, Li R, Weng Z-Y and Song G (2023) Immunogenicity and effectiveness of COVID-19 booster vaccination among people living with HIV: a systematic review and meta-analysis. Front. Med. 10:1275843. doi: 10.3389/fmed.2023.1275843
Received: 10 August 2023; Accepted: 18 September 2023;
Published: 09 October 2023.
Edited by:
Yanmin Wan, Fudan University, ChinaReviewed by:
Marco Massari, IRCCS Local Health Authority of Reggio Emilia, ItalyFabio Fiorino, LUM University Giuseppe Degennaro, Italy
Copyright © 2023 Cheng, Li, Weng and Song. This is an open-access article distributed under the terms of the Creative Commons Attribution License (CC BY). The use, distribution or reproduction in other forums is permitted, provided the original author(s) and the copyright owner(s) are credited and that the original publication in this journal is cited, in accordance with accepted academic practice. No use, distribution or reproduction is permitted which does not comply with these terms.
*Correspondence: Gao Song, 387925693@qq.com; Zhi-Ying Weng, 24286630433@qq.com