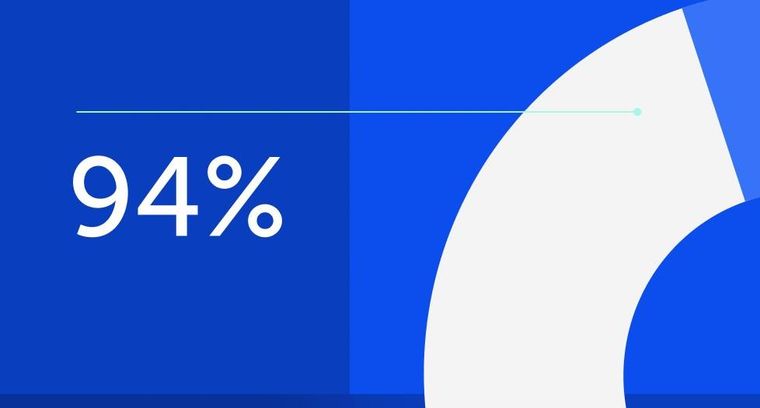
94% of researchers rate our articles as excellent or good
Learn more about the work of our research integrity team to safeguard the quality of each article we publish.
Find out more
REVIEW article
Front. Med., 08 January 2024
Sec. Rheumatology
Volume 10 - 2023 | https://doi.org/10.3389/fmed.2023.1272291
This article is part of the Research TopicInflammatory or Autoimmune Manifestations Associated with Hematological MalignanciesView all 4 articles
Background: CAR-T-related deaths observed worldwide are rare. The underlying pathogenetic mechanisms are the subject of study, as are the findings that enable diagnosis. A systematic literature search of the PubMed database and a critical review of the collected studies were conducted from the inception of this database until January 2023. The aim of the study is to determine when death is related to CAR-T cell therapy and to develop a shareable diagnostic algorithm.
Methods: The database was searched by combining and meshing the terms (“CAR-t” OR “CART”) AND (“Pathology” OR “Histology” OR “Histological” OR “Autopsy”) AND (“Heart” OR “Cardiac” OR “Nervous System” OR “Kidney” OR “Liver”) with 34 results and also the terms: [(Lethal effect) OR (Death)] AND (CAR-T therapy) with 52 results in titles, abstracts, and keywords [all fields]. One hundred scientific articles were examined, 14 of which were additional records identified through other sources. Fifteen records were included in the review.
Results: Neuronal death, neuronal edema, perivascular edema, perivascular and intraparenchymal hemorrhagic extravasation, as well as perivascular plasmatodendrosis, have been observed in cases with fatal cerebral edema. A cross-reactivity of CAR-T cells in cases of fatal encephalopathy can be hypothesized when, in addition to the increased vascular permeability, there is also a perivascular lymphocyte infiltrate, which appears to be a common factor among most authors.
Conclusion: Most CAR-T-related deaths are associated with blood–brain barrier breakdown, central nervous system cell damage, and infiltrated T cells. Further autopsies and microscopic investigations would shed more light on the lethal toxicity related to CAR-T cells. A differential diagnosis of CAR-T-related death is crucial to identifying adverse events. In this article, we propose an algorithm that could facilitate the comparison of findings through a systematic approach. Despite toxicity cases, CAR-T therapy continues to stand out as the most innovative treatment within the field of oncology, and emerging strategies hold the promise of delivering safer therapies in future.
Chimeric antigen receptor (CAR)-T cell therapy is a novel immunotherapy that has demonstrated remission responses in relapsed/refractory (R/R) hematological cancers (1, 2). CAR-T cells are derived from the patient’s own cells to overcome allogeneic rejection or graft-versus-host disease (GvHD). However, this approach requires individual, bespoke manufacturing. Autologous CAR-T cells are activated T cells transduced with a retroviral or lentiviral vector containing the CAR gene.
The first generation of CARs consisted of a transmembrane domain and an intracellular domain derived from the CD3ζ molecule of the endogenous T-cell receptor (2). Second-generation CARs contain a CD3ζ chain and an intracellular signaling domain of various costimulatory molecules, such as 4-1BB (CD137), CD28, OX40 (CD134), and induction T-cell stimulators (ICOS or CD278), which have improved the efficacy and persistence of CAR-T cells (3). Third-generation CARs have two signaling domains and a CD3ζ chain, such as CD3ζ–CD28–OX40, which, compared to second-generation CARs, provides increased activation signals, cytokine production, and anti-tumor activity in these cells (4). The latest generation of CAR-T cells is currently programmed to inhibit the tumor microenvironment (5).
CAR-T cells allow for high specificity and a sustained response that is HLA-independent, owing to the ability of these “living drugs” to bind to specific targets for which they have been programmed. The consistent expression of B-cell markers, such as CD19, CD20, and CD22 (6), or maturation antigens (e.g., BCMA) (7, 8), in many B-cell malignancies, has made them excellent targets for CAR-T cell therapy.
In order to address the need for new therapeutic options that ensure a high standard of safety for human health, numerous clinical CAR-T studies are being conducted worldwide (9). To illustrate, various European centers, universities, and academic institutions are actively pursuing research in this field, including studies such as NCT03373097 for neuroblastoma and NCT03373071 for CD19+ diseases, both conducted in Italy at the Bambino Gesù Pediatric Hospital in Rome. Nevertheless, the largest number of clinical trials currently takes place in the United States and China. Several clinical trials have explored CD19- and CD22-targeted CAR-T cells (NCT01044069 and NCT02315612), showing promising results in acute lymphoid leukemia (ALL), as well as CD19-targeted CAR-T cells in various non-Hodgkin lymphoma (NHL) subtypes (10–19).
Axicabtagene ciloleucel (axi-cel), INN-lisocabtagene maraleucel (liso-cell), brexucabtagene autoleucel (brexu-cel), and tisagenlecleucel (tisa-cel) represent second-generation autologous CD19-targeted CAR-T cell therapies approved for the treatment of relapsed/refractory large B-cell lymphoma (LBCL) based on phase II trials ZUMA-1 (15, 20) and JULIET (21).
More recently, several clinical trials have demonstrated promising results in patients with relapsed/refractory multiple myeloma (MM) treated with CAR-T cell therapy (8, 22–26). Various antigens, including CD33 and CD123, which are overexpressed on acute myeloid leukemia (AML) leukemic stem cells, have been extensively studied in preclinical models (27–29).
In parallel with the onco-hematological success of this new therapy, the European Society for Blood and Marrow Transplantation (EBMT), the Joint Accreditation Committee of ISCT and EBMT (JACIE), and the European Hematology Association (EHA) have issued reference guidelines. These guidelines not only delineate the treatment strategies developed thus far but also encompass the most frequently encountered adverse effects associated with CAR-T cell therapy. While therapy-related toxicities are generally rare, among them, the most commonly encountered are cytokine release syndrome (CRS) and immune effector cell-associated neurotoxicity syndrome (ICANS). Typically, these toxicities manifest within the first 2 weeks (1–14 days) after infusion and may persist for days, weeks, or, in rare cases, even months. Other side effects include cytopenia, hypogammaglobulinemia, B-cell aplasia, and infection. Although death related to CAR-T therapy is uncommon, some authors have estimated a therapy-related mortality rate of 5% (2).
CRS represents the predominant complication associated with CAR-T cell treatment, occurring in 30–100% of patients. Its onset usually occurs within the first week after CAR-T cell infusion and coincides with the peak of CAR-T cell expansion. According to the grading scale established by the American Society for Transplantation and Cellular Therapy (ASTCT), grade 1 CRS entails only fever (≥38°C). Grade 2 is characterized by fever, mild hypoxia, and hypotension. Grade 3 involves fever, hypoxia requiring high-flow oxygen, and hypotension necessitating vasopressor therapy. Grade 4 presents as fever accompanied by severe hypotension, profound hypoxia requiring oxygen-positive pressure therapy (e.g., BiPAP, CPAP, and mechanical intubation), corticosteroid drugs, and tocilizumab (30). Due to gravity, treatment for CRS (cytokine release syndrome) is initiated when the grading reaches ≥G2.
ICANS is a neurotoxic syndrome occurring in 67% of patients with leukemia and 62% of patients with lymphoma treated with CD19-targeted CAR-T cell therapy. It is characterized by symptoms such as aphasia, tremor, dysgraphia, and lethargy, which can progress to global aphasia, seizures, obtundation, stupor, and coma (30). The risk of developing high-grade ICANS can be attributed to factors such as the pre-treatment tumor immune microenvironment, elevated levels of inflammatory cytokines (e.g., IL-6), C-reactive protein (CRP), ferritin, and D-dimer, as well as the infused product (e.g., CAR-T cells with CD28 as a costimulatory domain) and pre-existing central nervous system (CNS) disorders (31). It has been reported that high fever and neurological symptoms in the first few days after infusion can predict severe ICANS (6). ICANS gravity is established by a score system (CARTOX-10), which allows for the classification of severity into four grades (0–3).
Understanding the pathophysiological mechanisms underlying ICANS is of significant importance and will help interpret the histopathological findings to date. It appears that the symptomatic manifestation is primarily driven by increased vascular permeability linked to elevated cytokines associated with endothelial cell activation. Additionally, macrophage activation syndrome (MAS) and tumor lysis syndrome (TLS) have been documented as side effects of therapy. In this highly pre-treated population, prior cardiotoxic chemotherapy or underlying cardiac conditions in these patients warrant assessment. By the way, some authors have reported cardiovascular complications in approximately 20% of CAR-T therapy cases, including hypotension, arrhythmias, myocardial damage, and even death from heart failure.
In the literature, adverse effects related to CAR-T cell therapy (ICANS, CRS, TLS, and infections) have been reported even several months after treatment. Some authors suggest that the potential risk of developing cardiovascular toxicity should prompt more stringent surveillance protocols (32). Given the growing utilization of these drugs in oncology, it is imperative for pathologists to possess knowledge of the forensic implications associated with CAR-T therapy, particularly in cases involving medical negligence or issues related to the administration of CAR-T therapy.
The objective of this study is to gain insights, through a systematic review of the scientific literature, into the elements that define CAR-T-related deaths and to highlight findings from cases involving autopsies and/or histopathological investigations.
This systematic review was conducted from the inception of these databases until January 2023 and followed the Preferred Reporting Items for Systematic Reviews and Meta-Analyses (PRISMA) guidelines (33). A systematic literature search of the PubMed database and a critical review of the collected studies were conducted. The database was searched by combining and meshing the terms: (“CAR-T” OR “CART”) AND (“pathology” OR “Histology” OR “histological” OR “autopsy”) AND (“heart” OR “cardiac” OR “Nervous System” OR “kidney” OR “liver”) with 34 results. We also searched the terms: [(lethal effect) OR (death)] AND (CAR-T therapy) with 52 results in titles, abstracts, and keywords [all fields]. The references of all identified articles were examined and cross-referenced to further isolate the relevant literature. A methodologic appraisal of each study was conducted, including an evaluation of bias. The data collection process included study selection and data extraction. This study was exempt from institutional review board approval as it did not involve human subjects. A waiver of patient consent was provided due to the use of deidentified patient data. Inclusion criteria included case reports, research articles, autopsy series reports, and papers describing CAR-T-related deaths. Exclusion criteria included items that did not have deaths related to CAR-T therapy; deaths related to underlying disease or leukocyte depletion/aplasia infections were excluded (Figure 1).
Figure 1. Preferred reporting items for systematic review (PRISMA) flow chart—search strategy. Study designs comprised retrospective and prospective studies, original articles, and reviews. An appraisal based on titles and abstracts as well as a hand search of reference lists were carried out. A total of 15 studies fulfilled the inclusion criteria.
This systematic review possesses several strengths, one of which is the international scope of the scientific literature it encompasses. In fact, the studies investigating CAR-related toxicities and deaths originate from various countries across different regions of the world. It must be noted that this review includes studies that have few cases. Therefore, the information that emerges must necessarily be updated by increasing the number of cases. There is also a gap between the number of clinically described CAR-T cell-related deaths and the number of autopsies and histopathological investigations performed.
The literature research has uncovered significant involvement of the central nervous system, with a few cases of cardiotoxicity. In the investigation, the primary causes of death were neurotoxic, followed by cardiotoxicity, as reported in Table 1. As illustrated below, cerebral involvement was present in most autopsy cases.
Despite the little information deduced from the scientific data examined, the review of the literature of autopsy cases of CAR-T-related death allows to know the consequences of adverse events related to therapy. The damages described by the MRI were also observed in autopsies.
The primary information gleaned from autopsy reports concerns the involvement of the central nervous system (CNS) in CAR-T-related deaths, characterized by an enlargement of cerebral volume with augmented convolutions observed in situ. Following dissection, the key features that could be observed include small petechial hemorrhagic areas, especially in caudal regions, the cerebellar zone, and intraparenchymal spaces such as the medulla, pons, midbrain, posterior thalamus, and cerebellum. These microscopic findings confirmed signs of CAR-T-induced encephalopathy and were observed in the absence of any herniation.
Cerebral infarcted areas, characterized by large necrotic and plaque-like lesions, are frequently described. These gross features were confirmed during the histological examination. As seen in MRI scans, acute cerebral edema was present in all cases of neurotoxicity. Notably, edema constitutes the primary histological finding, manifested as optically empty vacuoles in which cells are immersed, along with dilatation of perivascular spaces (vasogenic and cytotoxic edema).
Microscopic examinations revealed multifocal microhemorrhages and patchy parenchymal necrosis. In association with small areas of infarction, severe vascular lesions were observed, featuring karyorrhexis, fibrinoid vessel wall necrosis, and perivascular CD8+ infiltration. In one case, the authors highlighted a significant activation of macrophages/microglia (CD68+). Most slides exhibited the presence of lymphocytes, CAR-T cells, macrophages, and signs of endothelial damage.
Examination of the heart revealed hypertrophic changes with volume enlargement in both ventricles. In one case, coronary atherosclerosis was observed, but it was not linked to CAR-T therapy-related death. Histological examination showed monocyte infiltrates and lipofuscin pigmentation around some nuclei, associated with interstitial edema in the right ventricle. Scattered mast cells CD117+ were also observed throughout the myocardium, along with patchy lymphocytic infiltrate CD3+ (no CAR-T), focal monocyte necrosis, and perivascular fibrosis. Cardiac findings do not always allow for a diagnosis of cardiac death.
CAR-T cell therapy has demonstrated a significant and long-lasting response in different types of tumors. However, if, on the one hand, CAR-T cells meet cancer cells, inducing their apoptosis, on the other, the release of inflammatory cytokines and the recruitment of myeloid cells lead to systemic inflammation that, in some cases, induces damage to healthy tissues, causing the death of the subject. The high presence of circulating inflammatory cytokines and endothelial activation driven by myeloid cells can determine the dysfunction of the blood–brain barrier (BBB) and ICANS. Capillary leak syndrome, hypotension, organ failure, disseminated intravascular coagulation, and macrophage activation syndrome have been observed in treated patients developing ICANS. Aberrant macrophages were found around the brain vasculature in a case study of fatal CAR-T-associated ICANS (45). These mechanisms would agree with what some authors have found: high levels of glial fibrillary acidic protein (GFAP), S100 calcium-binding protein B, white blood cells, protein, interferon-gamma, granzyme, granulocyte–macrophage colony-stimulating factor, macrophage inflammatory protein-1α, and TNF-α in the cerebrospinal fluid of patients experiencing ICANS (34, 45).
In patients with severe ICANS, an evolution to fatal cerebral edema has been observed, characterized by swollen brain with flattened gyri, ventricles, cerebral aqueduct, and narrowed sulci; however, no herniations or hemorrhages of Duret have been observed. Both at the level of the cortex and the white matter of the brain, brainstem, and cerebellum, some areas of reddish-brown color have been highlighted and confirmed as intraparenchymal hemorrhagic extravasations (31, 41, 46), sometimes in the context of frank multifocal necrotizing leukoencephalopathy (46).
From a histopathological and immunohistochemical point of view, the findings highlighted in ICANS cases are neuronal death, neuronal edema, perivascular edema, perivascular and intraparenchymal hemorrhagic extravasation, as well as perivascular clasmatodendrosis in cases of fatal cerebral edema (37, 45); at H&E staining, dilations of perivascular spaces present infiltration of inflammatory cells surrounded by eosinophilic amorphous matrix, indicative of fluid extravasation (31, 41). In some cases, areas characterized by the presence of ACL-, CD3-, and CD68-positive inflammatory cells have also been observed (40). Immunohistochemistry stains for GLUT1 were used to highlight endothelial damage, while staining for GFAP showed damage to the astrocyte component in both white matter and the cortex.
According to Gust et al. (31), severe neurotoxicity related to CAR-T cells is also characterized by intraparenchymal hemorrhages, fibrinoid vessel wall necrosis, intravascular microthrombi, infarctions, and infiltrations by CAR-T cells. These last findings find confirmation in animal models (microthrombi, microinfarcts, and infiltrations of intraparenchymal and intrameningeal CAR-T cells). In the literature, there are also cases of inflammation extended to the trunk, limbic system, and cerebellum, characterized by astrocyte damage, macrophage/microglia activation, and diffuse and scattered infiltrates of cytotoxic T lymphocytes (e.g., CD8+ at hippocampal level), where the extraction of CAR-T cell DNA from the most affected areas has allowed a causal correlation (CAR-T—brain damage, concluding for CAR-T-cell-triggered encephalitis) (40). A more extensive encephalic involvement would also be confirmed by other authors, where vacuolization and axonal spheroids in the pontine white matter, a finding consistent with Wallerian degeneration, have also been documented through LFB-PAS stains (41).
In some cases, the areas of encephalopathy were considered with caution since they could not be uniquely related to the infusion of CAR-T; these findings are also present in cases of encephalopathy or multifocal leukoencephalopathy linked to chemotherapy, hypoxia, AIDS, JC viruses, drugs, genetic factors, etc., which were excluded before correlating deaths to CAR-T cell infusion.
The authors who have dealt with the topic so far agree with the hypothesis of cross-reactivity of CAR-T cells in cases of fatal encephalopathy when, in addition to the increase in vascular permeability, there is also a perivascular lymphocyte infiltrate (31, 37, 40, 41, 47, 48). A recent study (January 2023) proposes a multi-phase model that would explain a common mechanism underlying neuropathological findings and the greater severity of ICANS in cases of oligodendrocytic cell line involvement (38). The latter provides a clear illustrative representation of the mechanisms underlying CAR-T-related deaths, making it a useful guide in forensic cases. An algorithm proposal for the diagnosis of CAR-T-related deaths is shown in Figure 2.
Table 3. Collection data of autopsy and microscopic evaluation according to the liquor and plasma concentration of protein and inflammatory cells.
Emphasizing the significance of correlating clinical data with both pre- and post-mortem instrumental analyses in conjunction with autopsy findings is crucial. For an exhaustive examination of the central nervous system, the authors suggest the simultaneous performance of cerebral evisceration and vertebral specum dissection. Subsequently, these specimens should be assessed as a unified entity following formalin fixation, and then examined microscopically.
In addition to histopathology, magnetic resonance imaging (MRI) is applied in the post-mortem examination of CAR-T-related neurotoxicity. Further studies are needed to estimate the correlation between CAR-T and brain toxicity, as it is a matter of fact that MRI could see the common findings of neurotoxicity (49, 50). A recent review underlines the role of MRI in stressing on how the infusion of CAR-T may result in an impairment of the blood–brain barrier, with signs of demyelination, micro-thrombosis, microhemorrhages/infarcts, edema, and expansive lesions related to infiltrations of intraparenchymal and intrameningeal CAR-T cells (51). It is of the utmost importance that future experimental studies give a thorough description of the post-mortem features in the neuroimaging of ICANS.
The data collected during forensic investigations with these points can help the neuropathologist to have sufficient evidence to support a CAR-T-related death. Although the toxicity of CAR-T on the cardiovascular system is known, supporting a causal correlation appears more difficult when autopsy and immunohistochemistry do not reveal relevant elements (52, 53).
To diagnose CAR-T therapy-related deaths, it is crucial to have access to the patients’ clinical history and knowledge of the timing of CAR-T cell treatment. This is especially important because, to date, the number of reported deaths in this context is limited, and microscopic evidence is not pathognomonic. Distinguishing the effects of the chemotherapeutics used and opportunistic viruses from those linked to CAR-T therapy is of paramount importance. Most CAR-T therapy-related deaths manifest with blood–brain barrier disruption and damage to the central nervous system cell components, along with T-cell infiltration, as per the observed mechanism.
This study presents highly significant advancements as it enables clinicians to provide valuable information for distinguishing post-mortem deaths resulting from the therapy from those due to the natural course of the underlying disease. In this context, the remnants described can provide valuable insights into understanding the life-threatening consequences of the clinical situation and detecting them early. These findings can be used by the scientific community to investigate new methods for diagnosing fatalities associated with CAR-T therapy.
However, conducting additional autopsies and microscopic investigations would provide further insights into this subject. The investigation of CAR-T DNA presence in directly affected tissues presents an intriguing avenue for research that should be rigorously explored, validated, and potentially standardized in future (54–57). In the field of forensics, a more comprehensive understanding of CAR-T therapy-related deaths would help in determining the causes of death and resolving issues related to any legal disputes. From a forensic perspective, another intriguing area to explore is the possibility of detecting CAR-T cells even several years after treatment. This information could prove valuable and guide investigations in cases of personal identification.
FD: Formal analysis, Writing – original draft. GN: Writing – original draft. GV: Writing – original draft. AM: Conceptualization, Project administration, Writing – original draft. RR: Conceptualization, Investigation, Writing – original draft. MP: Data curation, Writing – original draft. SM: Validation, Writing – review & editing. PF: Visualization, Writing – original draft. MB: Writing – review & editing. VF: Supervision, Writing – original draft.
The author(s) declare that no financial support was received for the research, authorship, and/or publication of this article.
The authors declare that the research was conducted in the absence of any commercial or financial relationships that could be construed as a potential conflict of interest.
All claims expressed in this article are solely those of the authors and do not necessarily represent those of their affiliated organizations, or those of the publisher, the editors and the reviewers. Any product that may be evaluated in this article, or claim that may be made by its manufacturer, is not guaranteed or endorsed by the publisher.
1. Pasqui, DM, Latorraca, CDOC, Pacheco, RL, and Riera, R. CAR-T cell therapy for patients with hematological malignancies. A systematic review. Eur J Haematol. (2022) 109:601–18. doi: 10.1111/ejh.13851
2. Kröger, N, Gribben, J, Chabannon, C, Yakoub-Agha, I, and Einsele, H eds. The EBMT/EHA CAR-T cell handbook. Cham: Springer International Publishing (2022).
3. Till, BG, Jensen, MC, Wang, J, Qian, X, Gopal, AK, Maloney, DG, et al. CD20-specific adoptive immunotherapy for lymphoma using a chimeric antigen receptor with both CD28 and 4-1BB domains: pilot clinical trial results. Blood. (2012) 119:3940–50. doi: 10.1182/blood-2011-10-387969
4. Lock, D, Mockel-Tenbrinck, N, Drechsel, K, Barth, C, Mauer, D, Schaser, T, et al. Automated manufacturing of potent CD20-directed chimeric antigen receptor T cells for clinical use. Hum Gene Ther. (2017) 28:914–25. doi: 10.1089/hum.2017.111
5. Cheng, J, Zhao, L, Zhang, Y, Qin, Y, Guan, Y, Zhang, T, et al. Understanding the mechanisms of resistance to CAR T-cell therapy in malignancies. Front Oncol. (2019) 9:1237. doi: 10.3389/fonc.2019.01237
6. Portuguese, AJ, Gauthier, J, Tykodi, SS, Hall, ET, Hirayama, A, Yeung, CCS, et al. CD19 CAR-T therapy in solid organ transplant recipients: case report and systematic review. Bone Marrow Transplant. (2022) 58:353–9. doi: 10.1038/s41409-022-01907-z
7. Roex, G, Timmers, M, Wouters, K, Campillo-Davo, D, Flumens, D, Schroyens, W, et al. Safety and clinical efficacy of BCMA CAR-T-cell therapy in multiple myeloma. J Hematol Oncol. (2020) 13:164. doi: 10.1186/s13045-020-01001-1
8. Raje, N, Berdeja, J, Lin, Y, Siegel, D, Jagannath, S, Madduri, D, et al. Anti-BCMA CAR T-cell therapy bb 2121 in relapsed or refractory multiple myeloma. N Engl J Med. (2019) 380:1726–37. doi: 10.1056/NEJMoa1817226
9. Barros, LRC, Couto, SCF, da Silva Santurio, D, Paixão, EA, Cardoso, F, da Silva, VJ, et al. Systematic review of available CAR-T cell trials around the world. Cancers. (2022) 14:2667. doi: 10.3390/cancers14112667
10. Wang, N, Hu, X, Cao, W, Li, C, Xiao, Y, Cao, Y, et al. Efficacy and safety of CAR19/22 T-cell cocktail therapy in patients with refractory/relapsed B-cell malignancies. Blood. (2020) 135:17–27. doi: 10.1182/blood.2019000017
11. Yan, N, Wang, N, Wang, G, Huang, L, Li, C, Wang, D, et al. CAR19/22 T cell cocktail therapy for B-ALL relapsed after allogeneic hematopoietic stem cell transplantation. Cytotherapy. (2022) 24:841–9. doi: 10.1016/j.jcyt.2022.01.011
12. Mueller, KT, Waldron, E, Grupp, SA, Levine, JE, Laetsch, TW, Pulsipher, MA, et al. Clinical pharmacology of tisagenlecleucel in B-cell acute lymphoblastic leukemia. Clin Cancer Res. (2018) 24:6175–84. doi: 10.1158/1078-0432.CCR-18-0758
13. Ying, Z, Huang, XF, Xiang, X, Liu, Y, Kang, X, Song, Y, et al. A safe and potent anti-CD19 CAR T cell therapy. Nat Med. (2019) 25:947–53. doi: 10.1038/s41591-019-0421-7
14. Shah, NN, Johnson, BD, Schneider, D, Zhu, F, Szabo, A, Keever-Taylor, CA, et al. Bispecific anti-CD20, anti-CD19 CAR T cells for relapsed B cell malignancies: a phase 1 dose escalation and expansion trial. Nat Med. (2020) 26:1569–75. doi: 10.1038/s41591-020-1081-3
15. Locke, FL, Ghobadi, A, Jacobson, CA, Miklos, DB, Lekakis, LJ, Oluwole, OO, et al. Long-term safety and activity of axicabtagene ciloleucel in refractory large B-cell lymphoma (ZUMA-1): a single-arm, multicentre, phase 1–2 trial. Lancet Oncol. (2019) 20:31–42. doi: 10.1016/S1470-2045(18)30864-7
16. Frey, NV, Shaw, PA, Hexner, EO, Pequignot, E, Gill, S, Luger, SM, et al. Optimizing chimeric antigen receptor T-cell therapy for adults with acute lymphoblastic leukemia. J Clin Oncol. (2020) 38:415–22. doi: 10.1200/JCO.19.01892
17. Abramson, JS, Palomba, ML, Gordon, LI, Lunning, MA, Wang, M, Arnason, J, et al. Lisocabtagene maraleucel for patients with relapsed or refractory large B-cell lymphomas (TRANSCEND NHL 001): a multicentre seamless design study. Lancet. (2020) 396:839–52. doi: 10.1016/S0140-6736(20)31366-0
18. Wang, M, Munoz, J, Goy, A, Locke, FL, Jacobson, CA, Hill, BT, et al. KTE-X19 CAR T-cell therapy in relapsed or refractory mantle-cell lymphoma. N Engl J Med. (2020) 382:1331–42. doi: 10.1056/NEJMoa1914347
19. Yassine, F, Iqbal, M, Murthy, H, Kharfan-Dabaja, MA, and Chavez, JC. Real world experience of approved chimeric antigen receptor T-cell therapies outside of clinical trials. Curr Res Transl Med. (2020) 68:159–70. doi: 10.1016/j.retram.2020.05.005
20. Neelapu, SS, Locke, FL, Bartlett, NL, Lekakis, LJ, Miklos, DB, Jacobson, CA, et al. Axicabtagene ciloleucel CAR T-cell therapy in refractory large B-cell lymphoma. N Engl J Med. (2017) 377:2531–44. doi: 10.1056/NEJMoa1707447
21. Schuster, SJ, Bishop, MR, Tam, CS, Waller, EK, Borchmann, P, McGuirk, JP, et al. Tisagenlecleucel in adult relapsed or refractory diffuse large B-cell lymphoma. N Engl J Med. (2019) 380:45–56. doi: 10.1056/NEJMoa1804980
22. Mei, H, Li, C, Jiang, H, Zhao, X, Huang, Z, Jin, D, et al. A bispecific CAR-T cell therapy targeting BCMA and CD38 in relapsed or refractory multiple myeloma. J Hematol Oncol. (2021) 14:161. doi: 10.1186/s13045-021-01170-7
23. Cohen, AD, Garfall, AL, Stadtmauer, EA, Melenhorst, JJ, Lacey, SF, Lancaster, E, et al. B cell maturation antigen–specific CAR T cells are clinically active in multiple myeloma. J Clin Investig. (2019) 129:2210–21. doi: 10.1172/JCI126397
24. Li, C, Mei, H, Hu, Y, Guo, T, Liu, L, Jiang, H, et al. A bispecific CAR-T cell therapy targeting BCMA and CD38 for relapsed/refractory multiple myeloma: updated results from a phase 1 dose-climbing trial. Blood. (2019) 134:930–10. doi: 10.1182/blood-2019-130340
25. Zah, E, Nam, E, Bhuvan, V, Tran, U, Ji, BY, Gosliner, SB, et al. Systematically optimized BCMA/CS1 bispecific CAR-T cells robustly control heterogeneous multiple myeloma. Nat Commun. (2020) 11:2283. doi: 10.1038/s41467-020-16160-5
26. Popat, R, Zweegman, S, Cavet, J, Yong, K, Lee, L, Faulkner, J, et al. Phase 1 first-in-human study of AUTO2, the first chimeric antigen receptor (CAR) T cell targeting APRIL for patients with relapsed/refractory multiple myeloma (RRMM). Blood. (2019) 134:3112–2. doi: 10.1182/blood-2019-126689
27. Haubner, S, Perna, F, Köhnke, T, Schmidt, C, Berman, S, Augsberger, C, et al. Coexpression profile of leukemic stem cell markers for combinatorial targeted therapy in AML. Leukemia. (2019) 33:64–74. doi: 10.1038/s41375-018-0180-3
28. Fiorenza, S, and Turtle, CJ. CAR-T cell therapy for acute myeloid leukemia: preclinical rationale, current clinical progress, and barriers to success. BioDrugs. (2021) 35:281–302. doi: 10.1007/s40259-021-00477-8
29. Yao, S, Jianlin, C, Yarong, L, Botao, L, Qinghan, W, Hongliang, F, et al. Donor-derived CD123-targeted CAR T cell serves as a RIC regimen for Haploidentical transplantation in a patient with FUS-ERG+ AML. Front Oncol. (2019) 9:1358. doi: 10.3389/fonc.2019.01358
30. Lee, DW, Santomasso, BD, Locke, FL, Ghobadi, A, Turtle, CJ, Brudno, JN, et al. ASTCT consensus grading for cytokine release syndrome and neurologic toxicity associated with immune effector cells. Biol Blood Marrow Transplant. (2019) 25:625–38. doi: 10.1016/j.bbmt.2018.12.758
31. Gust, J, Hay, KA, Hanafi, LA, Li, D, Myerson, D, Gonzalez-Cuyar, LF, et al. Endothelial activation and blood–brain barrier disruption in neurotoxicity after adoptive immunotherapy with CD19 CAR-T cells. Cancer Discov. (2017) 7:1404–19. doi: 10.1158/2159-8290.CD-17-0698
32. Ghosh, AK, Chen, DH, Guha, A, Mackenzie, S, Walker, JM, and Roddie, C. CAR T cell therapy–related cardiovascular outcomes and management. JACC Cardio Oncol. (2020) 2:97–109. doi: 10.1016/j.jaccao.2020.02.011
33. Page, MJ, McKenzie, JE, Bossuyt, PM, Boutron, I, Hoffmann, TC, Mulrow, CD, et al. The PRISMA 2020 statement: an updated guideline for reporting systematic reviews. Int J Surg. (2021) 88:105906. doi: 10.1016/j.ijsu.2021.105906
34. Gust, J, Finney, OC, Li, D, Brakke, HM, Hicks, RM, Futrell, RB, et al. Glial injury in neurotoxicity after pediatric CD19-directed chimeric antigen receptor T cell therapy. Ann Neurol. (2019) 86:42–54. doi: 10.1002/ana.25502
35. Benjamin, R, Graham, C, Yallop, D, Jozwik, A, Mirci-Danicar, OC, Lucchini, G, et al. Genome-edited, donor-derived allogeneic anti-CD19 chimeric antigen receptor T cells in paediatric and adult B-cell acute lymphoblastic leukaemia: results of two phase 1 studies. Lancet. (2020) 396:1885–94. doi: 10.1016/S0140-6736(20)32334-5
36. Afzal, A, Farooque, U, Gillies, E, and Hassell, L. T-cell therapy-mediated myocarditis secondary to cytokine release syndrome. Cureus. (2020) 12:e10022. doi: 10.7759/cureus.10022
37. Torre, M, and Feany, MB. Iatrogenic neuropathology of systemic therapies. Surg Pathol Clin. (2020) 13:331–42. doi: 10.1016/j.path.2020.01.004
38. Nie, EH, Ahmadian, SS, Bharadwaj, SN, Acosta-Alvarez, L, Threlkeld, ZD, Frank, MJ, et al. Multifocal demyelinating leukoencephalopathy and oligodendroglial lineage cell loss with immune effector cell-associated neurotoxicity syndrome (ICANS) following CD19 CAR T-cell therapy for mantle cell lymphoma. J Neuropathol Exp Neurol. (2023) 82:160–8. doi: 10.1093/jnen/nlac121
39. Berdeja, JG, Madduri, D, Usmani, SZ, Jakubowiak, A, Agha, M, Cohen, AD, et al. Ciltacabtagene autoleucel, a B-cell maturation antigen-directed chimeric antigen receptor T-cell therapy in patients with relapsed or refractory multiple myeloma (CARTITUDE-1): a phase 1b/2 open-label study. Lancet. (2021) 398:314–24. doi: 10.1016/S0140-6736(21)00933-8
40. Jung, S, Greiner, J, von Harsdorf, S, Popovic, P, Moll, R, Schittenhelm, J, et al. Fatal late-onset CAR T-cell–mediated encephalitis after axicabtagene-ciloleucel in a patient with large B-cell lymphoma. Blood Adv. (2021) 5:3789–93. doi: 10.1182/bloodadvances.2021004889
41. Karschnia, P, Strübing, F, Teske, N, Blumenberg, V, Bücklein, VL, Schmidt, C, et al. Clinicopathologic findings in fatal neurotoxicity after adoptive immunotherapy with CD19-directed CAR T-cells. Hema. (2021) 5:e533. doi: 10.1097/HS9.0000000000000533
42. Goldman, A, Maor, E, Bomze, D, Liu, JE, Herrmann, J, Fein, J, et al. Adverse cardiovascular and pulmonary events associated with chimeric antigen receptor T-cell therapy. J Am Coll Cardiol. (2021) 78:1800–13. doi: 10.1016/j.jacc.2021.08.044
43. Jacobson, CA, Chavez, JC, Sehgal, AR, William, BM, Munoz, J, Salles, G, et al. Axicabtagene ciloleucel in relapsed or refractory indolent non-Hodgkin lymphoma (ZUMA-5): a single-arm, multicentre, phase 2 trial. Lancet Oncol. (2022) 23:91–103. doi: 10.1016/S1470-2045(21)00591-X
44. Pemmaraju, N, Wilson, NR, Senapati, J, Economides, MP, Guzman, ML, Neelapu, SS, et al. CD123-directed allogeneic chimeric-antigen receptor T-cell therapy (CAR-T) in blastic plasmacytoid dendritic cell neoplasm (BPDCN): clinicopathological insights. Leuk Res. (2022) 121:106928. doi: 10.1016/j.leukres.2022.106928
45. Torre, M, Solomon, IH, Sutherland, CL, Nikiforow, S, DeAngelo, DJ, Stone, RM, et al. Neuropathology of a case with fatal CAR T-cell-associated cerebral edema. J Neuropathol Exp Neurol. (2018) 77:877–82. doi: 10.1093/jnen/nly064
46. Marker, DF, Kofler, JK, Mettenburg, JA, Agha, ME, and Wiley, CA. Multifocal necrotizing leukoencephalopathy with preferential microglia toxicity in a patient treated with chimeric antigen receptor T-cells and review of the literature. J Neuropathol Exp Neurol. (2020) 79:1115–21. doi: 10.1093/jnen/nlaa099
47. Schuster, SJ, Svoboda, J, Chong, EA, Nasta, SD, Mato, AR, Anak, Ö, et al. Chimeric antigen receptor T cells in refractory B-cell lymphomas. N Engl J Med. (2017) 377:2545–54. doi: 10.1056/NEJMoa1708566
48. Mackenzie, S, Shafat, M, Roddy, H, Hyare, H, Neill, L, and Marzolini, MA v.,, et al. Pembrolizumab for the treatment of progressive multifocal leukoencephalopathy following anti-CD19 CAR-T therapy: a case report. EJHaem (2021); 2: 848–853. doi: 10.1002/jha2.274
49. Pensato, U, Muccioli, L, Zinzani, P, D’Angelo, R, Pierucci, E, Casadei, B, et al. Correction to: fulminant cerebral edema following CAR T-cell therapy: case report and pathophysiological insights from literature review. J Neurol. (2022) 269:4564–4. doi: 10.1007/s00415-022-11156-1
50. Pensato, U, de Philippis, C, Pistolese, F, Mannina, D, Marcheselli, S, Politi, LS, et al. Case report: reversible punctate inflammatory foci in the corpus callosum: a novel radiological finding of CAR T-cell therapy-related neurotoxicity. Front Neurol. (2023) 14:1125121. doi: 10.3389/fneur.2023.1125121
51. Pinto, SN, Liu, CJ, Nelson, MD, Bluml, S, Livingston, D, and Tamrazi, B. Neuroimaging of complications arising after CD19 chimeric antigen receptor T-cell therapy: a review. J Neuroimaging. (2023) 33:703–15. doi: 10.1111/jon.13138
52. Neri, M, Frati, A, Turillazzi, E, Cantatore, S, Cipolloni, L, Di Paolo, M, et al. Immunohistochemical evaluation of Aquaporin-4 and its correlation with CD68, IBA-1, HIF-1α, GFAP, and CD15 expressions in fatal traumatic brain injury. Int J Mol Sci. (2018) 19:3544. doi: 10.3390/ijms19113544
53. Turillazzi, E, Di Paolo, M, Neri, M, Riezzo, I, and Fineschi, V. A theoretical timeline for myocardial infarction: immunohistochemical evaluation and western blot quantification for Interleukin-15 and monocyte chemotactic protein-1 as very early markers. J Transl Med. (2014) 12:188. doi: 10.1186/1479-5876-12-188
54. Morbelli, S, Gambella, M, Raiola, AM, Ghiggi, C, Bauckneht, M, Raimondo, TD, et al. Brain FDG-PET findings in chimeric antigen receptor T-cell therapy neurotoxicity for diffuse large B-cell lymphoma. J Neuroimaging. (2023) 33:825–36. doi: 10.1111/jon.13135
55. Qian, S, Villarejo-Campos, P, Guijo, I, Hernández-Villafranca, S, García-Olmo, D, González-Soares, S, et al. Update for advance CAR-T therapy in solid tumors, clinical application in peritoneal Carcinomatosis from colorectal Cancer and future prospects. Front Immunol. (2022) 13:841425. doi: 10.3389/fimmu.2022.841425
56. Hayden, PJ, Roddie, C, Bader, P, Basak, GW, Bonig, H, Bonini, C, et al. Management of adults and children receiving CAR T-cell therapy: 2021 best practice recommendations of the European Society for Blood and Marrow Transplantation (EBMT) and the joint accreditation committee of ISCT and EBMT (JACIE) and the European Haematology Association (EHA). Ann Oncol. (2022) 33:259–75. doi: 10.1016/j.annonc.2021.12.003
Keywords: CAR-T, death, edema, blood–brain barrier breakdown, autopsy
Citation: Del Duca F, Napoletano G, Volonnino G, Maiese A, La Russa R, Di Paolo M, De Matteis S, Frati P, Bonafè M and Fineschi V (2024) Blood–brain barrier breakdown, central nervous system cell damage, and infiltrated T cells as major adverse effects in CAR-T-related deaths: a literature review. Front. Med. 10:1272291. doi: 10.3389/fmed.2023.1272291
Received: 03 August 2023; Accepted: 17 December 2023;
Published: 08 January 2024.
Edited by:
Thibault Comont, Centre Hospitalier Universitaire de Toulouse, FranceReviewed by:
Luca Castagna, Azienda Ospedaliera Ospedali Riuniti Villa Sofia Cervello, ItalyCopyright © 2024 Del Duca, Napoletano, Volonnino, Maiese, La Russa, Di Paolo, De Matteis, Frati, Bonafè and Fineschi. This is an open-access article distributed under the terms of the Creative Commons Attribution License (CC BY). The use, distribution or reproduction in other forums is permitted, provided the original author(s) and the copyright owner(s) are credited and that the original publication in this journal is cited, in accordance with accepted academic practice. No use, distribution or reproduction is permitted which does not comply with these terms.
*Correspondence: Vittorio Fineschi, dml0dG9yaW8uZmluZXNjaGlAdW5pcm9tYTEuaXQ=
Disclaimer: All claims expressed in this article are solely those of the authors and do not necessarily represent those of their affiliated organizations, or those of the publisher, the editors and the reviewers. Any product that may be evaluated in this article or claim that may be made by its manufacturer is not guaranteed or endorsed by the publisher.
Research integrity at Frontiers
Learn more about the work of our research integrity team to safeguard the quality of each article we publish.