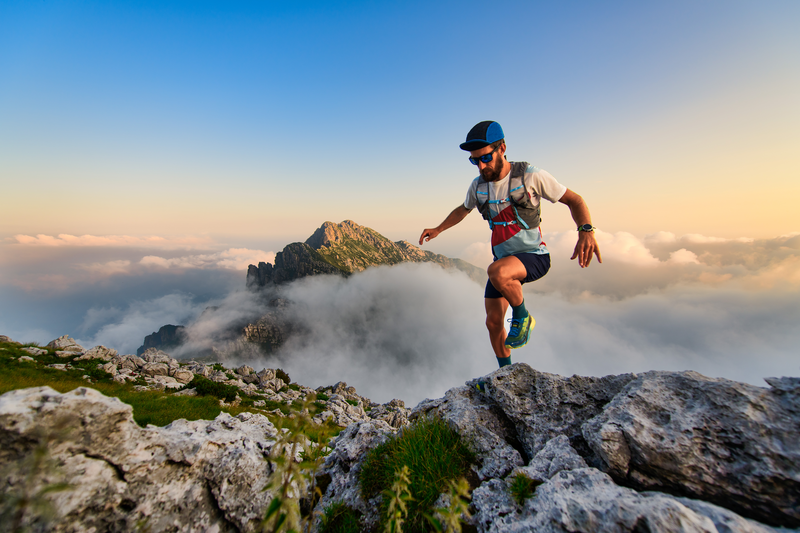
95% of researchers rate our articles as excellent or good
Learn more about the work of our research integrity team to safeguard the quality of each article we publish.
Find out more
SYSTEMATIC REVIEW article
Front. Med. , 28 December 2023
Sec. Intensive Care Medicine and Anesthesiology
Volume 10 - 2023 | https://doi.org/10.3389/fmed.2023.1250845
This article is part of the Research Topic Carbon Monoxide Poisoning: Updates on Prevention, Diagnosis, and Treatment View all 6 articles
Introduction: Acute carbon monoxide poisoning (COP) is one of the leading causes of intoxication among patients presenting to the emergency department (ED). COP symptoms are not always specific and may vary from mild to critical. In the last few years, COHb pulse oximeters have been developed and applied to the setting of suspected COP. The aim of this systematic review is to assess the diagnostic accuracy of CO pulse oximetry (SpCO) with carboxyhemoglobin (COHb) levels measured by blood gas analysis, used as a reference standard, in patients with suspected COP.
Methods: We developed our search strategy according to the PICOS framework, population, index/intervention, comparison, outcome, and study, considering the diagnostic accuracy of SpCO compared to COHb levels measured by blood gas analysis, used as a reference standard, in patients with suspected COP enrolled in cross-sectional studies in English. The search was performed on MEDLINE/PubMed and EMBASE in February 2022. Quality assessment was performed using the QUADAS-2 methodology. A COHb cutoff of 10% was chosen to test the sensitivity and specificity of the index test. A bivariate model was used to perform the meta-analysis. The protocol was registered on PROSPERO (CRD42022359144).
Results: A total of six studies (1734 patients) were included. The pooled sensitivity of the test was 0.65 (95% CI 0.44–0.81), and the pooled specificity was 0.93 (95% CI 0.83–0.98). The pooled LR+ was 9.4 (95% CI 4.4 to 20.1), and the pooled LR- was 0.38 (95% CI 0.24 to 0.62).
Conclusion: Our results show that SpCO cannot be used as a screening tool for COP in the ED due to its low sensitivity. Because of its high LR+, it would be interesting to evaluate, if SpCO could have a role in the prehospital setting as a tool to quickly identify COP patients and prioritize their transport to specialized hospitals on larger samples with a prospective design.
Carbon monoxide (CO) is a colorless, odorless, non-irritant, toxic gas. The incomplete combustion of hydrocarbons leads to CO production. In nature, the principal CO sources are volcanoes and forest fires (1, 2). On the other hand, artificial CO production comes mainly from motor vehicles, poorly functioning heating systems, gas-powered equipment, house fires, boat engines, and cigar or waterpipe smoking. These factors contribute to most cases of accidental human CO poisoning (COP) (3). CO intoxication can also result from indirect sources, such as dichloromethane ingestion or inhalation and its subsequent metabolism to CO (4).
CO intoxication is one of the leading poisoning causes of admission to the ED, with an exposure that may be accidental or intentional (3, 5). According to European registries on CO intoxications and deaths, from 1980 to 2008, there were 140,490 CO-related deaths in 28 countries. CO intoxication was involved in 31,473 hospital admissions. Death due to unintentional intoxication (mostly caused by accidental exposure, such as that due to faulty heating systems and appliances) accounts for 54.7% of all CO-related deaths (6). Intentional COP can take place by several means, such as self-intoxication with internal combustion engine exhaust fumes in an enclosed space.
CO is inhaled and then diffuses from the alveoli into the blood, with its blood levels depending upon its concentration in the air and the duration of exposure (1). After absorption, CO partially binds to myoglobin and cytochrome C, but mainly to iron molecules of hemoglobin, so that it forms carboxyhemoglobin (COHb) (1, 5, 7). Hemoglobin affinity for CO is up to 250 times higher than oxygen affinity (1, 5). This implies that oxygen delivery to tissues is severely impaired, and hypoxia and anaerobic metabolism result as a consequence (1, 3). In addition, CO affects platelet function and may lead to a hypercoagulable state and intravascular thrombosis (8). Furthermore, oxidative metabolism is affected by CO and leads to free radical formation (9).
COP symptoms are not always specific and have a wide range, from mild to critical (5, 9). Mild symptoms such as headache, nausea, dizziness, and vomiting may be present. In more severe cases, patients may present with syncope or altered mental status. Moreover, CO may cause cardiac ischemia or failure due to hypoxia and endothelial dysfunction of myocytes in combination with mitochondrial inhibition, promoting a syndrome similar to myocardial infarction (5, 9).
Since the diagnosis of COP is complicated by the variability in its clinical presentations, it can sometimes go undetected (10). Consequently, the absence of a clear history and the non-specific symptoms make blood CO-oximetry a fundamental adjunct to confirm or exclude a suspected exposure in different settings. Blood gas analysis (BGA) is the most widespread method for the diagnosis of COP because the measurement of COHb levels is reliable and quickly available. Nonetheless, BGA is an invasive method and is usually not available out of the hospital, in particular, in emergency situations with contingent severity and an urgent need for therapy (7, 10). Since standard pulse oximetry devices are not able to distinguish between oxyhemoglobin and COHb, oxygen saturation levels in patients with COP are wrongly reported as normal (11). Since 2005, the Rad-57 signal extraction pulse CO-oximeter (RAD; Maximo Corporation, Irvine, CA) has been approved for clinical use. This device has the ability to discriminate between oxyhemoglobin, COHb, and methemoglobin and therefore may be used to assess the COHb percentage level. Several studies have been published addressing this issue, with partially discordant results (11, 12).
The aim of this systematic review is to evaluate the diagnostic accuracy of CO-oximetry (SpCO) using blood COHb as a reference standard in adult patients with suspected COP.
This systematic review was conducted and reported in accordance with the Preferred Reporting Items for Systematic Reviews and Meta-Analyses (PRISMA) statement. The protocol of this systematic review was registered on PROSPERO with the registration number CRD42022359144.
Studies that enrolled patients suspected of COP, published in English, with a cross-sectional design were eligible if data to construct a 2×2 table for SpCO accuracy at a cutoff of 10% were available. The 10% cutoff was chosen because it is outside of the physiological range for heavy smokers. The index test had to be peripheral SpCO and the reference standard COHb measured by BGA.
We developed our search strategy according to the PICOS framework, population, index/intervention, comparison, outcome, and study, considering the diagnostic accuracy of SpCO compared to COHb levels measured by BGA in patients with suspected COP enrolled in cross-sectional studies in English.
MEDLINE/PubMed and EMBASE were searched for cross-sectional studies in February 2022. The search strategy was ((diagnosis) OR (sensitivity) OR (specificity)) OR (cross-sectional) AND (carbon monoxide).
Reference lists of individual articles were manually screened for evaluation and possible inclusion of other relevant studies.
After removing duplicates, abstracts were independently screened by two review authors (EK and FA). Full texts of the potentially eligible studies were retrieved, and two review authors (GR and FA) independently assessed the full-text publications for eligibility according to inclusion criteria. Disagreements between investigators in the choice of article inclusion (such as those due to the relevance of the article to the research question, study design, and patient selection criteria) were resolved by means of inter-investigator discussion and eventual agreement. When disagreement persisted, a third investigator (GC) was asked to make the eventual decision.
The following data were extracted: study title, author, country, design, language and year of publication, number of centers involved, funding, the total number of patients, age range, sex, and data for a 2×2 table (numbers of true positive, TN; false positive, FP; false negative, FN; and true negative, TN) at a cutoff of 10%. For studies that did not report in the main text or tables, accuracy data and data for the 2×2 table were derived from figures reported in the article, if available.
The Quality Assessment of Diagnostic Accuracy Studies (QUADAS-2) tool was used for the assessment of the risk of bias in the included studies and the applicability of their results.
All data were extracted by two review authors (FA and GR). Disagreements were resolved through discussion.
First, we performed a graphical descriptive analysis of the included studies. We presented forest plots [sensitivity and specificity separately, with their 95% confidence intervals (CIs)], and we provided a graphical representation of studies in the receiver operating characteristic space (sensitivity against 1 – specificity). Second, we performed the meta-analyses using the bivariate model and provided estimates of pooled sensitivity and specificity. We used the summary pooled estimates obtained from the fitted models to calculate summary estimates of positive (LR+) and negative (LR–) likelihood ratios.
All the statistical analyses were performed using SAS (release 9.4) and Rev Man (release 5.4) software.
The search, after removing duplicates, yielded 13,394 results, of which 13,342 were excluded after screening the title or the abstract of the studies. The remaining 52 studies were assessed for inclusion. Five additional studies were included after the manual screening of the 52 full-text references and were also read in full. Out of the 57 articles evaluated for full assessment, 38 studies were not relevant to the study question, 10 studies were not cross-sectional, and data could not be retrieved for three additional studies. Finally, six studies were included in the systematic review and meta-analysis (7, 10, 11, 13–15). The study selection process is summarized in Figure 1.
All the studies included were monocentric, with prospective data collection conducted from 2005 to 2014. A total of 1734 patients were included. The mean age was in the fifth decade for most of the studies. Females accounted for 40–55% of the subjects. The number of participants for each study varies from 12 to 1,363. Smoking habits were reported in three out of six studies (11, 13, 14) and smokers percentages ranged from 23 to 40%. Out of 1734 patients, 106 had COP. The overall prevalence of COP was 6.1%, ranging from 1.7% (in the largest study) to 83% (in the smallest study). Pediatric patients were only present in Piatkowski’s study (one patient, age 8), in Coulange’s study (one patient, age 8), in Weaver’s study (at least one patient, age 3), and possibly in Touger’s study (not specified). The characteristics of the included studies are presented in Table 1.
The risk of bias and applicability concerns evaluated according to QUADAS-2 is reported in Figure 2. Patient selection (lack of consecutive or random enrollment, inappropriate exclusions) and flow and timing (mostly due to the exclusion of patients from the analysis) were deemed to be at high risk of bias in three studies. While the reference standard was not deemed to be at high risk of bias in any study, it was unclear in six studies.
For the six studies included in the meta-analysis of SpCO at a 10% cutoff, sensitivity ranged from 30 to 100% and specificity ranged from 75 to 100% (Figure 3). The pooled sensitivity was 0.65 (95% CI 0.44–0.81), the pooled specificity was 0.93 (95% CI 0.83–0.98), the pooled LR+ was 9.4 (95% CI 4.4 to 20.1), and the pooled LR- was 0.38 (95% CI 0.24 to 0.62) (Figure 4).
Figure 4. Plot of the studies in the ROC space. Circles represent individual studies. The solid black circle represents the pooled estimate of sensitivity and specificity. The dotted border represents 95% confidence region.
The results of our study show that non-invasive SpCO at a COHb cutoff of 10% has high specificity (specificity 0.93; LR+ 9.4) but low sensitivity (sensitivity 0.65; LR- 0.38). These data suggest that SpCO is not sufficiently accurate to be used as a screening test in patients with suspected COP.
The ideal non-invasive method to triage patients with suspected COP in the ED should be highly sensitive. However, the sensitivity reported in our study is not sufficient to allow SpCO to be used as a screening test.
A COP diagnosis relies on clinical suspicion and increased levels of COHb. Blood COHb levels are usually considered normal, up to 10% in smokers and 5% in non-smokers (16). In our study, the defining CO cutoff for COP was 10%. This rather high upper limit was chosen because it is clinically relevant, it is outside of the CO physiological range for both smokers and non-smokers, and it was previously used to compare SpCO to blood COHb levels in a prospective observational study (15, 17). Lowering the COHb cutoff would likely increase SpCO sensitivity at the cost of specificity. While reducing the COHb cutoff to 5% could be a feasible strategy to improve the sensitivity of the test, it should be considered that the resulting decrease in specificity would result in a heightened rate of false positives and a consequent challenge in the management of resources. In addition, COHb levels may normally reach levels up to 10% in smokers, as compared to 2–3% in non-smokers, which further complicates diagnostic assessment (5). According to previous studies, each pack of cigarettes smoked a day increases COHb levels by approximately 2.5%, and in selected heavy smokers, COHb levels can also increase above 10% (17).
Importantly, the high LR+ obtained in our study suggests that SpCO could be useful to confirm a suspect COP in settings where BGA is not available. SpCO could help first responders rapidly recognize patients who need to be transported to a specialized hospital setting with hyperbaric treatment capability. A triage algorithm for COP based on SpCO was proposed by Hampson et al. in 2006 (18). In Italy, SpCO prehospital measurement has been explored in one district, with a reduction in the time elapsed from rescue to hyperbaric oxygen treatment (19). Similar results were observed in a group of patients in the US, in which SpCO measurement performed either at the scene by first responders or in the ED led to significantly shorter times for hyperbaric oxygen treatment, which could in turn lead to a clinical benefit (20).
In addition, on-site measurement of SpCO would be of high value in situations, such as mass casualty incidents (e.g., fires and explosions), in which the resources needed (such as helicopters and, in a severe crisis, even ambulances) to rapidly distribute the high number of patients to different facilities may be limited. The rapidity of use, the lack of necessity of invasive procedures (BGA), and the portability are the main advantages of the CO pulse oximeter. These features could make these devices particularly useful in disaster medicine. Implementing SpCO measurement within the initial triage procedure of patients involved in a fire would offer a quick estimate of the number of patients requiring hyperbaric treatment and therefore allow a timelier coordination with the emergency center and the receiving hospitals. In addition, a quick detection of COP with a SpCO device could be particularly useful to identify environments that could possibly be dangerous for rescuers and could be a valuable alternative to BGA in situations where it is not readily available, such as in disaster medicine. In a mass COP event that occurred in Switzerland, SpCO measurement was integrated with symptoms (e.g., transient loss of consciousness) and history (e.g., known pregnancy) in order to develop a rapid triage system that allowed for the identification of patients requiring transport to an HBO center, transport to a general hospital, or who could be treated on-site or directly discharged (21). SpCO measurement could also be of value in mass COP events following an abrupt power outage (e.g., as a result of storms, floods, and earthquakes) and the consequent use of improvised heating or burning devices (22, 23).
A recently published systematic review and meta-analysis evaluating SpCO accuracy to estimate blood COHb quantification reported a mean sensitivity of 0.77 [95% CI (0.66–0.85)] and a mean specificity of 0.83 [95% CI (0.74–0.89)] (24). Several reasons might explain the slightly higher sensitivity and lower specificity of SpCO observed in Papin’s study compared to ours. First, due to less stringent inclusion criteria, a higher number of articles with both observational and experimental designs were analyzed. Second, the COHb cutoff was not selected a priori and ranged from 5 to 23%. This wide range of COHb thresholds could have influenced SpCO diagnostic accuracy. However, even with these limitations, the meta-analysis results are similar to ours regarding the low sensitivity, while the reported specificity is much lower than ours. For these reasons, our conclusions are slightly different: they suggest that SpCO is currently an unreliable diagnostic tool and cannot be used as a substitute for blood COHb to identify COP cases, while we think that, in specific settings such as prehospital emergency medicine, SpCO could have some role.
The most important limitation of our meta-analysis is the origin of the data. Our results might have been influenced by the small number of patients enrolled in most of the studies. Moreover, the report with the highest number of subjects (14) included only a minority of patients with proven COP. Of note, the low prevalence of COP might make enrollment of consecutive patients difficult, therefore exposing studies to possible biases.
Moreover, the choice of a single COHb cutoff for smokers and non-smokers could be a limitation since these two populations have different baseline COHb levels. In particular, the choice of a 10% cutoff may be inappropriate and too high in some special populations, such as pregnant women, children, and patients with chronic CO intoxication. Nonetheless, the available data do not allow an accurate distinction between these subgroups of patients. Further studies are needed to evaluate if setting distinct cutoffs in different patient populations could improve the diagnostic accuracy of SpCO.
Our results show that SpCO cannot be used as a screening tool for COP in the ED due to its low sensitivity. Because of its high LR+, it would be interesting to evaluate if SpCO could have a role in the prehospital setting as a tool to quickly identify COP patients and prioritize their transport to specialized hospitals on larger samples with a prospective design.
The raw data supporting the conclusions of this article will be made available by the authors, without undue reservation.
GR, EK, AG, FA, IP, RC, and Gcol did the search strategy extracted the data and quality assessment. GR, GCos, GCa, and FG wrote the first draft of the manuscript. All authors discuss the results and contribute in writing the final manuscript.
The author(s) declare financial support was received for the research, authorship, and/or publication of this article. This study was partially funded by Italian Ministry of Health, Current research IRCCS.
The authors declare that the research was conducted in the absence of any commercial or financial relationships that could be construed as a potential conflict of interest.
All claims expressed in this article are solely those of the authors and do not necessarily represent those of their affiliated organizations, or those of the publisher, the editors and the reviewers. Any product that may be evaluated in this article, or claim that may be made by its manufacturer, is not guaranteed or endorsed by the publisher.
CO, carbon monoxide; COHb, carboxyhemoglobin; SpCO, carbon monoxide pulse oximetry; COP, acute carbon monoxide poisoning; ED, emergency department; BGA, blood gas analysis.
1. Chenoweth, JA, Albertson, TE, and Greer, MR. Carbon monoxide poisoning. Crit Care Clin. (2021) 37:657–72. doi: 10.1016/j.ccc.2021.03.010
2. Wilbur, S, Williams, M, Williams, R, Scinicariello, F, Klotzbach, JM, Diamond, GL, et al. Toxicological profile for carbon monoxide. Atlanta, GA: Agency for Toxic Substances and Disease Registry (US) (2012).
3. Bleecker, ML. Carbon monoxide intoxication. Handb Clin Neurol. (2015) 131:191–203. doi: 10.1016/B978-0-444-62627-1.00024-X
4. Pacheco, C, Magalhaes, R, Fonseca, M, Silveira, P, and Brandao, I. Accidental intoxication by dichloromethane at work place: clinical case and literature review. J Acute Med. (2016) 6:43–5. doi: 10.1016/j.jacme.2016.03.008
5. Ernst, A, and Zibrak, JD. Carbon monoxide poisoning. N Engl J Med. (1998) 339:1603–8. doi: 10.1056/NEJM199811263392206
6. Braubach, M, Algoet, A, Beaton, M, Lauriou, S, Héroux, ME, and Krzyzanowski, M. Mortality associated with exposure to carbon monoxide in WHO European member states. Indoor Air. (2012) 23:115–25. doi: 10.1111/ina.12007
7. Touger, M, Birnbaum, A, Wang, J, Chou, K, Pearson, D, and Bijur, P. Performance of the RAD-57 pulse CO-oximeter compared with standard laboratory carboxyhemoglobin measurement. Ann Emerg Med. (2010) 56:382–8. doi: 10.1016/j.annemergmed.2010.03.041
8. Nielsen, VG, and Pretorius, E. Carbon monoxide: anticoagulant or procoagulant? Thromb Res. (2014) 133:315–21. doi: 10.1016/j.thromres.2013.12.004
9. Rose, JJ, Wang, L, Xu, Q, McTiernan, CF, Shiva, S, Tejero, J, et al. Carbon monoxide poisoning: pathogenesis, management, and future directions of therapy. Am J Respir Crit Care Med. (2017) 195:596–606. doi: 10.1164/rccm.201606-1275CI
10. Piatkowski, A, Ulrich, D, Grieb, G, and Pallua, N. A new tool for the early diagnosis of carbon monoxide intoxication. Inhal Toxicol. (2009) 21:1144–7. doi: 10.3109/08958370902839754
11. Sebbane, M, Claret, PG, Mercier, G, Lefebvre, S, Théry, R, Dumont, R, et al. Emergency department management of suspected carbon monoxide poisoning: role of pulse CO-oximetry. Respir Care. (2013) 58:1614–20. doi: 10.4187/respcare.02313
12. Zaouter, C, and Zavorsky, GS. The measurement of carboxyhemoglobin and methemoglobin using a non-invasive pulse CO-oximeter. Respir Physiol Neurobiol. (2012) 182:88–92. doi: 10.1016/j.resp.2012.05.010
13. Coulange, M, Barthelemy, A, Hug, F, Thierry, AL, and De Haro, L. Reliability of new pulse CO-oximeter in victims of carbon monoxide poisoning. Undersea Hyperb Med. (2008) 35:107–11.
14. Weaver, LK, Churchill, SK, Deru, K, and Cooney, D. False positive rate of carbon monoxide saturation by pulse oximetry of emergency department patients. Respir Care. (2013) 58:232–40. doi: 10.4187/respcare.01744
15. Villalba, N, Osborn, ZT, Derickson, PR, Manning, CT, Herrington, RR, Kaminsky, DA, et al. Diagnostic performance of carbon monoxide testing by pulse oximetry in the emergency department. Respir Care. (2019) 64:1351–7. doi: 10.4187/respcare.06365
16. Suner, S, Partridge, R, Sucov, A, Valente, J, Chee, K, Hughes, A, et al. Non-invasive pulse CO-oximetry screening in the emergency department identifies occult carbon monoxide toxicity. J Emerg Med. (2008) 34:441–50. doi: 10.1016/j.jemermed.2007.12.004
17. Hampson, NB, Piantadosi, CA, Thom, SR, and Weaver, LK. Practice recommendations in the diagnosis, management, and prevention of carbon monoxide poisoning (2013) 186:1095–101. doi: 10.1164/rccm.201207-1284CI,
18. Hampson, N, and Weaver, LK. Noninvasive CO measurement by first responders. A suggested management algorithm. J Emerg Med Serv. (2006) 24:10–2.
19. Martani, L, Cantadori, L, Paganini, M, Camporesi, EM, and Bosco, G. Carbon monoxide intoxication: prehospital diagnosis and direct transfer to the hyperbaric chamber. Minerva Anestesiol. (2019) 85:920–2. doi: 10.23736/S0375-9393.19.13648-6
20. Hampson, NB. Noninvasive pulse CO-oximetry expedites evaluation and management of patients with carbon monoxide poisoning. Am J Emerg Med. (2012) 30:2021–4. doi: 10.1016/j.ajem.2012.03.026
21. Pasquier, M, Dami, F, Carron, PN, Yersin, B, Pignel, R, and Hugli, O. Mass casualty triage in the case of carbon monoxide poisoning: lessons learned. Disaster Med Public Health Prep. (2018) 12:373–8. doi: 10.1017/dmp.2017.65
22. Iqbal, S, Clower, JH, Hernandez, SA, Damon, SA, and Yip, FY. A review of disaster-related carbon monoxide poisoning: surveillance, epidemiology, and opportunities for prevention. Am J Public Health. (2012) 102:1957–63. doi: 10.2105/AJPH.2012.300674
23. Nakajima, M, Aso, S, Matsui, H, Fushimi, K, Yamaguchi, Y, and Yasunaga, H. Disaster-related carbon monoxide poisoning after the great East Japan earthquake, 2011: a nationwide observational study. Acute Med Surgery. (2019) 6:294–300. doi: 10.1002/ams2.417
Keywords: carbon monoxide, carbon monoxide poisoning, pulse oximetry, diagnostic accuracy, carboxyhemoglobin, oximetry, intoxication, systematic review & meta-analysis
Citation: Ramponi G, Gianni F, Karlafti E, Piazza I, Albertoni F, Colombo G, Casazza G, Garegnani A, Casella R and Costantino G (2023) The diagnostic accuracy of carbon monoxide pulse oximetry in adults with suspected acute carbon monoxide poisoning: a systematic review and meta-analysis. Front. Med. 10:1250845. doi: 10.3389/fmed.2023.1250845
Received: 30 June 2023; Accepted: 09 October 2023;
Published: 28 December 2023.
Edited by:
Matteo Paganini, University of Padua, ItalyReviewed by:
John Lange, Envirosafe Training and Consultants, United StatesCopyright © 2023 Ramponi, Gianni, Karlafti, Piazza, Albertoni, Colombo, Casazza, Garegnani, Casella and Costantino. This is an open-access article distributed under the terms of the Creative Commons Attribution License (CC BY). The use, distribution or reproduction in other forums is permitted, provided the original author(s) and the copyright owner(s) are credited and that the original publication in this journal is cited, in accordance with accepted academic practice. No use, distribution or reproduction is permitted which does not comply with these terms.
*Correspondence: Giorgio Costantino, Z2lvcmdpYzJAZ21haWwuY29t
Disclaimer: All claims expressed in this article are solely those of the authors and do not necessarily represent those of their affiliated organizations, or those of the publisher, the editors and the reviewers. Any product that may be evaluated in this article or claim that may be made by its manufacturer is not guaranteed or endorsed by the publisher.
Research integrity at Frontiers
Learn more about the work of our research integrity team to safeguard the quality of each article we publish.