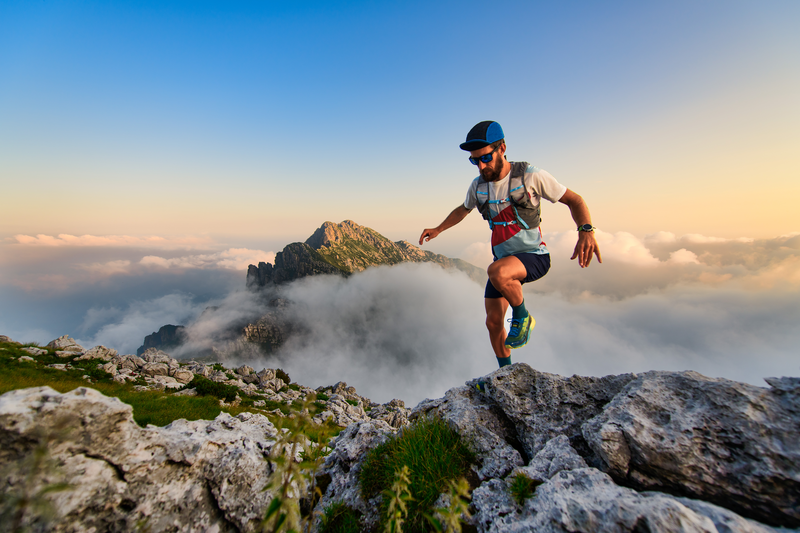
94% of researchers rate our articles as excellent or good
Learn more about the work of our research integrity team to safeguard the quality of each article we publish.
Find out more
MINI REVIEW article
Front. Med. , 08 August 2023
Sec. Nephrology
Volume 10 - 2023 | https://doi.org/10.3389/fmed.2023.1250457
This article is part of the Research Topic Pathogenic Aspects of the Innate Immune System of the Kidney View all 5 articles
Heatstroke can cause multiple organ failure and systemic inflammatory response syndrome as the body temperature rises beyond the body’s ability to regulate temperature in a hot environment. Previous studies have indicated that heatstroke-induced acute kidney injury (AKI) can lead to chronic kidney disease. Therefore, there is an urgent need to elucidate the mechanism of heatstroke-induced AKI and to establish methods for its prevention and treatment. Recent reports have revealed that innate immunity, including neutrophils, macrophages, lymphocytes, and mast cells, is deeply involved in heat-induced AKI. In this review, we will discuss the roles of each immune cell in heat-induced renal injury and their potential therapeutic use.
Due to global warming and heat waves, humans are being increasingly exposed to high temperatures, and the rate of heat-related illnesses is consequently increasing as well.
Heatstroke (HS), which is the most severe condition of heat-related illness, is characterized by central nervous system dysfunction and a core body temperature of greater than 40°C (1). Normally, the body temperature is maintained at approximately 37°C. However, when heat gain exceeds heat loss (noncompensatory phase), the body temperature continues to rise, triggering circulatory disturbances, cytotoxicity, and maladaptive inflammatory responses, which induce multiorgan dysfunction, including acute kidney injury (AKI) (2). Recent studies have shown that HS-induced AKI is not temporary but actually progresses to chronic kidney disease (CKD) (3, 4). Therefore, there is an urgent need to elucidate the exact mechanisms underlying HS-induced AKI and to explore prevention and treatment strategies for HS-induced AKI.
Many studies have reported that HS can cause a systemic inflammatory response syndrome (SIRS), which is defined as ≥2 of the following: body temperature >38°C or <36°C, heart rate >90/min, respiratory rate >20/min or PaCO2 <32 mmHg, and white blood cell count >12,000/mm3 or <4,000/mm3 or >10% immature bands (5). SIRS can cause severe shock, disseminated intravascular coagulation (DIC), multiple organ dysfunction, and death (6). Proinflammatory cytokines, such as interleukin (IL)-6, increase following endotoxemia or hyperthermia due to heat exposure (7). Sustained elevation of circulating IL-6 could induce further inflammation and result in poor outcome of HS (8).
Recently, increasing evidence has supported that kidney immune cells play important roles in early tissue injury, repair, and fibrosis after AKI (9). In this review, we will discuss the roles of each immune cell in heat-induced renal injury and therapeutic strategies targeting immune cells.
Neutrophils rapidly respond to injured organs. In animal experiments, neutrophils infiltrated injured kidneys as early as 4 h and reached a maximum at 24 h after ischemia-reperfusion injury (10, 11). Consistently, infiltrated neutrophils in the kidneys were also reported to start increasing immediately after heat exposure and increase statistically significantly at 24 h after heat stress (12). It has also been reported that neutrophils increase in bronchoalveolar lavage fluid (BALF) as early as 2 h after heat exposure in a mouse heat-induced acute lung injury model (13). In humans, renal biopsies are rarely performed to confirm the diagnosis of HS-induced AKI, and whether or not neutrophils are involved in AKI after heat stress is unclear. However, one study indirectly showed the relationship between neutrophils and HS-induced AKI. A retrospective study enrolled 187 intensive-care unit (ICU) patients with exertional HS (EHS) and showed that an increase in neutrophils and a decrease in lymphocytes are risk factors for AKI (14).
Neutrophil infiltration into damaged kidneys could contribute to cytotoxicity through phagocytosis, chemotaxis, and oxidative burst (10). A recent study showed that citrullinated histone H3 (Cit-H3), which is involved in the formation of neutrophil extracellular traps (NETs), appeared in the peripheral blood of HS patients (15). In a murine septic AKI model, NETs were induced by necrotic tubule-derived DAMPs and histones, leading to further cytokine release and resulting in additional tubular necrosis (16–18). Furthermore, these Cit-H3-positive cells possessed a multisegmented nucleus, and most were immunoreactive for CD66b (15). Multisegmented neutrophils, the so-called “botryoid nucleus,” were also observed in HS patients and mouse HS models (19, 20). These changes seem to result from nuclear degeneration induced by hyperthermia.
As a therapeutic target, oxytocin has been reported to reduce neutrophil infiltration, myeloperoxidase activity, and oxidative damage markers in acute lung injury after HS (21). Furthermore, CRRT in an early phase (≤8 h after admission) reduced percentages of neutrophils and APACHE II scores, which resulted in an improved survival of HS patients (22).
Mononuclear phagocytes, including monocytes and macrophages, have crucial and distinct roles in tissue homeostasis (23). In experimental animals, several studies have shown that macrophage infiltration into the kidney increased as early as 24 h after heat stress (12, 24). Furthermore, these increases in infiltrated macrophages were associated with the extent of core temperature elevation and exacerbation of tubular damage and fibrosis after heat stress. Using the mitochondrial coupling agent 2,3-dinitrophenol (DNP) with exposure to heat stress, mice expressed higher core temperature, more tubular damage, and more F4/80-positive macrophages in kidney tissue than those who did not use DNP (24).
Kidney macrophages can be classified into two types: bone marrow (monocyte)-derived macrophages and tissue-resident macrophages. Monocytes and bone marrow-derived macrophages are key players in inflammation and pathogen challenge. These cells produce proinflammatory cytokines, such as tumor necrosis factor-α (TNF-α) (25–27). In contrast, tissue-resident macrophages have important roles in the development and resolution of inflammation as well as tissue repair (23, 28–30). Tissue-resident macrophages were reported to enhance Wingless-type MMTV integration site family (Wnt) signaling after injury, which seemed to advance tissue repair (29). Using flow cytometry, we and others have reported that these two subsets of macrophages can be identified based on the differential expression of F4/80 and CD11b in mice: tissue-resident macrophages express F4/80highCD11blow, and bone marrow-derived macrophages express F4/80lowCD11bhigh (27–31). In our previous study, tissue-resident macrophages expressing F4/80high were decreased after heat stress in a mouse heat stress model. These macrophages seemed to become nonviable after heat stress (31). Furthermore, a decrease in the number of tissue-resident macrophages was coincident with a decrease in the number of PCNA-positive tubular cells, indicating delayed tubular regeneration.
Macrophages are also classified into two phenotypes: pro-inflammatory M1 phenotype and anti-inflammatory M2 phenotype (32). This classification was based on the in vitro observation that macrophages activated classically [activated by interferon (IFN)-γ] or alternatively (activated by IL-4/IL-13) (32). We and others have reported that kidney macrophages polarize to the M1 phenotype after heat stress (12, 31). These changes occurred immediately after exposure to heat stress, before monocyte-and bone marrow-derived macrophage infiltration into the kidneys. Furthermore, we also showed that not only bone marrow-derived macrophages but also tissue-resident macrophages were polarized to the M1 phenotype, suggesting that the kidney microenvironment after heat exposure might be involved in these phenotypical changes (31). In vivo, however, these phenotypes can coexist, so caution should be practiced when interpreting these results (33). For example, M2 macrophages contribute to both tissue repair and fibrosis in the context of AKI-to-CKD progression (34).
Regarding therapeutic targets, one study showed that electrical vagus nerve stimulation reduced the infiltration of CD11b-positive macrophages into the lung and spleen, resulting in a decrease in serum proinflammatory cytokines and an improved survival rate in a rat heat stress model (35). We recently evaluated the effect of heat acclimation in mice and found that heat acclimation ameliorated the decrease in tissue-resident macrophages and polarization to the M1 phenotype after heat stress in both bone marrow-derived and tissue-resident macrophages, which improved tubular damage and kidney fibrosis due to heat stress (31). We also found increased levels of heat shock protein (Hsp) 70, which induces stress resilience in each cell, in renal tubular cells and tissue-resident macrophages after heat acclimation. Therefore, heat acclimation can induce cytoprotective effects in tissue-resident macrophages, resulting in preservation of the number of these cells after heat exposure (Figure 1). Furthermore, these results from our study were consistent with a recent clinical study that showed that heat acclimation was associated with a decrease in AKI incidence. The incidence rate of AKI was reported to significantly decrease after a 23 days heat acclimation program in the United Kingdom military, which was coincident with the decrease in the levels of IL-6, one of the proinflammatory cytokines released by M1 macrophages (36).
Figure 1. The mechanisms by which heat acclimation (HA) prevents heat stress-induced AKI. HA induced intracellular heat shock protein (Hsp) 70 in tubular cells, which resulted in increased heat tolerance of tubular cells and prevented tubular injury (mechanism 1). HA also increased the levels of intracellular Hsp70 in tissue-resident macrophages and preserved the number of these cells after heat stress. As tissue-resident macrophages play an important role in tissue repair, regeneration of tubules occurred earlier with HA than without HA (mechanism 2).
Lymphocytes also play important roles in HS. Early studies demonstrated that CD8-positive cytotoxic T cells and natural killer (NK) cells are increased in the peripheral blood of HS patients, whereas CD4-positive helper T cells are decreased (37, 38). Furthermore, the number of CD8-positive T cells in the peripheral blood of HS patients was positively correlated with the rectal temperature (37). However, a recent study showed that a decreased number of lymphocytes was associated with increased 90 days mortality (39) and incidence of AKI (15) in HS patients. These controversial results might be due to the focus on whole lymphocytes or specific subpopulations thereof.
NK cells and NKT cells have been reported to play key roles in the early innate response in a mouse AKI model (11, 40, 41). These cells exert cytotoxic activities by releasing cytotoxic mediators and proinflammatory cytokines (42). Furthermore, when NK cells were depleted, the cytotoxic function of NKT cells was enhanced in a mouse α-GalCer-induced AKI model (43).
Regulatory T cells (Tregs), expressing forkhead/winged-helix transcription factor (Foxp) 3, release inhibitory cytokines and negative regulators of inflammation (44). In an animal experiment, decreases in the numbers of splenic Tregs and helper T cells and the production levels of anti-inflammatory cytokines were observed after heat stress (12, 45). Intestinal barrier dysfunction, which can cause leaky gut and endotoxemia, is also affected by a decrease in Tregs after heat exposure (46). These studies implied that Tregs might serve as potential therapeutic targets for HS patients.
Mast cells play an important role in the innate immune system. They are activated by antigen-specific immunoglobulin (Ig) E via high-affinity receptors for IgE (FcεRI), leading to degranulation and release of mediators, including histamine (47). Furthermore, mast cell-derived cytokines and other mediators affect immune cells, such as dendritic cells, T cells, and B cells (48). A recent study showed that mast cell tryptase (MCT), which is relatively specific for histamine release, was significantly increased in participants who developed post-exercise hypotension compared with those who did not (49). The authors suggest that mast cell degranulation is a vasodilatory mechanism underlying post-exercise hypotension and exercise associated collapse. However, one study showed that heat stress suppressed the IgE-induced degranulation of mast cells (50). A more recent study showed that exercise-associated changes in skeletal muscle temperature generated elevations in intramuscular histamine concentrations, although heating to comparable temperatures did not activate mast cell degranulation in an in vitro experiment (51). Therefore, the release of histamine without degranulation may cause post-exercise hypotension. In contrast, some studies have shown that the elevation of histamine levels in skeletal muscle might be important in generating positive adaptations to exercise training (51–53). An increase in histamine concentration induces an increase in post-exercise muscle perfusion (51, 52). Furthermore, with chronic interval training, histamine H1/H2 receptor signaling increases in the skeletal muscle, which results in an enhanced insulin sensitivity, aerobic capacity, and vascular function (NO production) (53). Further studies are needed to investigate whether or not increased histamine and its receptor signaling after chronic training and/or heat acclimation affects systemic adaptation to heat stress.
It is now clear that HS-induced AKI is due not only to dehydration or a decrease in renal blood flow but also to tubular damage caused by heat stress itself and the inflammatory immune response. Although the contributions of these studies are mentioned above, the precise immune response involved in HS-induced AKI remains unclear due to the wide distribution of involved immune cells and the complex interaction of these cells. Further studies focused on comprehensive changes in kidney immune cells after heat exposure, including studies using single-cell RNA sequencing, are needed to better understand the roles of each immune cell and the interactions of these cells, which might result in the development of new therapeutic approaches.
HG elaborated this mini-review. HG, MK, and NO edited and revised manuscript. All authors contributed to the article and approved the submitted version.
This work was supported by grants from the National Defense Medical College to our department.
The authors declare that the research was conducted in the absence of any commercial or financial relationships that could be construed as a potential conflict of interest.
All claims expressed in this article are solely those of the authors and do not necessarily represent those of their affiliated organizations, or those of the publisher, the editors and the reviewers. Any product that may be evaluated in this article, or claim that may be made by its manufacturer, is not guaranteed or endorsed by the publisher.
1. Sorensen, C, and Hess, J. Treatment and prevention of heat-related illness. N Engl J Med. (2022) 387:1404–13. doi: 10.1056/NEJMcp2210623
2. Epstein, Y, and Yanovich, R. Heatstroke. N Engl J Med. (2019) 380:2449–59. doi: 10.1056/NEJMra1810762
3. Kupferman, J, Ramírez-Rubio, O, Amador, JJ, López-Pilarte, D, Wilker, EH, Laws, RL, et al. Acute kidney injury in sugarcane workers at risk for mesoamerican nephropathy. Am J Kidney Dis. (2018) 72:475–82. doi: 10.1053/j.ajkd.2018.04.014
4. Tseng, MF, Chou, CL, Chung, CH, Chen, YK, Chien, WC, Feng, CH, et al. Risk of chronic kidney disease in patients with heat injury: a nationwide longitudinal cohort study in Taiwan. PLoS One. (2020) 15:e0235607. doi: 10.1371/journal.pone.0235607
5. Singer, M, Deutschman, CS, Seymour, CW, Shankar-Hari, M, Annane, D, Bauer, M, et al. The third international consensus definitions for sepsis and septic shock (Sepsis-3). JAMA. (2016) 315:801–10. doi: 10.1001/jama.2016.0287
6. Bone, RC . Sir Isaac Newton, sepsis, SIRS, and CARS. Crit Care Med. (1996) 24:1125–8. doi: 10.1097/00003246-199607000-00010
7. Garcia, CK, Renteria, LI, Leite-Santos, G, Leon, LR, and Laitano, O. Exertional heat stroke: pathophysiology and risk factors. BMJ Med. (2022) 1:e000239. doi: 10.1136/bmjmed-2022-000239
8. Hammami, MM, Bouchama, A, Al-Sedairy, S, Shail, E, AlOhaly, Y, and Mohamed, GE. Concentrations of soluble tumor necrosis factor and interleukin-6 receptors in heatstroke and heatstress. Crit Care Med. (1997) 25:1314–9. doi: 10.1097/00003246-199708000-00017
9. Lee, SA, Noel, S, Sadasivam, M, Hamad, ARA, and Rabb, H. Role of immune cells in acute kidney injury and repair. Nephron. (2017) 137:282–6. doi: 10.1159/000477181
10. Friedewald, JJ, and Rabb, H. Inflammatory cells in ischemic acute renal failure. Kidney Int. (2004) 66:486–91. doi: 10.1111/j.1523-1755.2004.761_3.x
11. Li, L, Huang, L, Sung, S-s J, Lobo, PI, Brown, MG, Gregg, RK, et al. NKT cell activation mediates neutrophil IFN-γ production and renal ischemia-reperfusion injury. J Immunol. (2007) 178:5899–911. doi: 10.4049/jimmunol.178.9.5899
12. Hu, J, Kang, H, Liu, C, Yang, M, and Zhou, F. Acute immune responses are involved in liver and kidney injury in heat stroke. Eur J Inflamm. (2019) 17:205873921983870. doi: 10.1177/2058739219838709
13. Zhou, G, Chen, Z, Li, J, Guo, X, Qin, K, Luo, J, et al. Role of the receptor for advanced glycation end products in heat stress-induced endothelial hyperpermeability in acute lung injury. Front Physiol. (2020) 11:1087. doi: 10.3389/fphys.2020.01087
14. Wu, M, Wang, C, Liu, Z, Zhong, L, Yu, B, Cheng, B, et al. Clinical characteristics and risk factors associated with acute kidney injury inpatient with exertional heatstroke: an over 10-year intensive care survey. Front Med. (2021) 8:678434. doi: 10.3389/fmed.2021.678434
15. Hirose, T, Hamaguchi, S, Matsumoto, N, Irisawa, T, Seki, M, Tasaki, O, et al. Presence of neutrophil extracellular traps and citrullinated histone H3 in the bloodstream of critically ill patients. PLoS One. (2014) 9:e111755. doi: 10.1371/journal.pone.0111755
16. Sharfuddin, AA, and Molitoris, BA. Pathophysiology of ischemic acute kidney injury. Nat Rev Nephrol. (2011) 7:189–200. doi: 10.1038/nrneph.2011.16
17. Czaikoski, PG, Mota, JM, Nascimento, DC, Sônego, F, Castanheira, FV, Melo, PH, et al. Neutrophil extracellular traps induce organ damage during experimental and clinical sepsis. PLoS One. (2016) 11:e0148142. doi: 10.1371/journal.pone.0148142
18. Nakazawa, D, Marschner, JA, Platen, L, and Anders, HJ. Extracellular traps in kidney disease. Kidney Int. (2018) 94:1087–98. doi: 10.1016/j.kint.2018.08.035
19. Im, DD, Ho, CH, and Chan, RY. Hypersegmented neutrophils in an adolescent male with heatstroke. J Pediatr Hematol Oncol. (2015) 37:488. doi: 10.1097/MPH.0000000000000356
20. Iba, T, Sawada, T, Kondo, Y, Kondo, K, and Levy, JH. Morphological changes in blood cells in a rat model of heatstroke: a pilot study. J Clin Med. (2022) 11:4821. doi: 10.3390/jcm11164821
21. Lin, CH, Tsai, CC, Chen, TH, Chang, CP, and Yang, HH. Oxytocin maintains lung histological and functional integrity to confer protection in heat stroke. Sci Rep. (2019) 9:18390. doi: 10.1038/s41598-019-54739-1
22. Chen, GM, Chen, YH, Zhang, W, Yu, Y, Chen, JH, and Chen, J. Therapy of severe heatshock in combination with multiple organ dysfunction with continuous renal replacement therapy: a clinical study. Medicine. (2015) 94:e1212. doi: 10.1097/MD.0000000000001212
23. Ginhoux, F, and Jung, S. Monocytes and macrophages: developmental pathways and tissue homeostasis. Nat Rev Immunol. (2014) 14:392–404. doi: 10.1038/nri3671
24. Sato, Y, Roncal-Jimenez, CA, Andres-Hernando, A, Jensen, T, Tolan, DR, Sanchez-Lozada, LG, et al. Increase of core temperature affected the progression of kidney injury by repeated heat stress exposure. Am J Physiol Renal Physiol. (2019) 317:F1111–21. doi: 10.1152/ajprenal.00259.2019
25. Li, L, Huang, L, Sung, SS, Vergis, AL, Rosin, DL, Rose, CE Jr, et al. The chemokine receptors CCR2 and CX3CR1 mediate monocyte/macrophage trafficking in kidney ischemia-reperfusion injury. Kidney Int. (2008) 74:1526–37. doi: 10.1038/ki.2008.500
26. Kawakami, T, Lichtnekert, J, Thompson, LJ, Karna, P, Bouabe, H, Hohl, TM, et al. Resident renal mononuclear phagocytes comprise five discrete populations with distinct phenotypes and functions. J Immunol. (2013) 191:3358–72. doi: 10.4049/jimmunol.1300342
27. Ito, S, Nakashima, H, Ishikiriyama, T, Nakashima, M, Yamagata, A, Imakiire, T, et al. Effects of a CCR2 antagonist on macrophages and toll-like receptor 9 expression in a mouse model of diabetic nephropathy. Am J Physiol Renal Physiol. (2021) 321:F757–70. doi: 10.1152/ajprenal.00191.2021
28. Salei, N, Rambichler, S, Salvermoser, J, Papaioannou, NE, Schuchert, R, Pakalniškytė, D, et al. The kidney contains ontogenetically distinct dendritic cell and macrophage subtypes throughout development that differ in their inflammatory properties. J Am Soc Nephrol. (2020) 31:257–78. doi: 10.1681/ASN.2019040419
29. Lever, JM, Hull, TD, Boddu, R, Pepin, ME, Black, LM, Adedoyin, OO, et al. Resident macrophages reprogram toward a developmental state after acute kidney injury. JCI Insight. (2019) 4:e125503. doi: 10.1172/jci.insight.125503
30. Ito, S, Nakashima, M, Ishikiriyama, T, Nakashima, H, Yamagata, A, Imakiire, T, et al. Effects of L-carnitine treatment on kidney mitochondria and macrophages in mice with diabetic nephropathy. Kidney Blood Press Res. (2022) 47:277–90. doi: 10.1159/000522013
31. Goto, H, Nakashima, M, Nakashima, H, Noguchi, M, Imakiire, T, Oshima, N, et al. Heat acclimation ameliorated heat stress-induced acute kidney injury and prevented changes in kidney macrophages and fibrosis. Am J Physiol Renal Physiol. (2022) 323:F243–54. doi: 10.1152/ajprenal.00065.2022
32. Chen, T, Cao, Q, Wang, Y, and Harris, DCH. M2 macrophages in kidney disease: biology, therapies, and perspectives. Kidney Int. (2019) 95:760–73. doi: 10.1016/j.kint.2018.10.041
33. Sica, A, and Mantovani, A. Macrophage plasticity and polarization: in vivo veritas. J Clin Invest. (2012) 122:787–95. doi: 10.1172/JCI59643
34. Yang, Q, Wang, Y, Pei, G, Deng, X, Jiang, H, Wu, J, et al. Bone marrow-derived Ly6C-macrophages promote ischemia-induced chronic kidney disease. Cell Death Dis. (2019) 10:291. doi: 10.1038/s41419-019-1531-3
35. Yamakawa, K, Matsumoto, N, Imamura, Y, Muroya, T, Yamada, T, Nakagawa, J, et al. Electrical vagus nerve stimulation attenuates systemic inflammation and improves survival in a rat heatstroke model. PLoS One. (2013) 8:e56728. doi: 10.1371/journal.pone.0056728
36. Omassoli, J, Hill, NE, Woods, DR, Delves, SK, Fallowfield, JL, Brett, SJ, et al. Variation in renal responses to exercise in the heat with progressive acclimatisation. J Sci Med Sport. (2019) 22:1004–9. doi: 10.1016/j.jsams.2019.04.010
37. Bouchama, A, Al Hussein, K, Adra, C, Rezeig, M, Al Shail, E, and Al Sedairy, S. Distribution of peripheral blood leukocytes in acute heatstroke. J Appl Physiol. (1992) 73:405–9. doi: 10.1152/jappl.1992.73.2.405
38. Hammami, MM, Bouchama, A, Shail, E, Aboul-Enein, HY, and Al-Sedairy, S. Lymphocyte subsets and adhesion molecules expression in heatstroke and heat stress. J Appl Physiol. (1998) 84:1615–21. doi: 10.1152/jappl.1998.84.5.1615
39. Ji, J, Su, P, Lin, W, Ouyang, L, Wang, C, Jia, J, et al. Immune cells characteristics and their prognostic effects in exertional heatstroke patients: a retrospective cohort study. Front Med. (2022) 9:867774. doi: 10.3389/fmed.2022.867774
40. Uchida, T, Ito, S, Kumagai, H, Oda, T, Nakashima, H, and Seki, S. Roles of natural killer T cells and natural killer cells in kidney injury. Int J Mol Sci. (2019) 20:2487. doi: 10.3390/ijms20102487
41. Zhang, Z-X, Wang, S, Huang, X, Min, W-P, Sun, H, Liu, W, et al. NK cells induce apoptosis in tubular epithelial cells and contribute to renal ischemia-reperfusion injury. J Immunol. (2008) 181:7489. doi: 10.4049/jimmunol.181.11.7489
42. Seki, S, Habu, Y, Kawamura, T, Takeda, K, Dobashi, H, Ohkawa, T, et al. The liver as a crucial organ in the first line of host defense: the roles of Kupffer cells, natural killer (NK) cells and NK1.1 Ag+ T cells in T helper 1 immune responses. Immunol Rev. (2000) 174:35–46. doi: 10.1034/j.1600-0528.2002.017404.x
43. Uchida, T, Nakashima, H, Ito, S, Ishikiriyama, T, Nakashima, M, Seki, S, et al. Activated natural killer T cells in mice induce acute kidney injury with hematuria through possibly common mechanisms shared by human CD56+ T cells. Am J Physiol Renal Physiol. (2018) 315:F618–27. doi: 10.1152/ajprenal.00160.2018
44. Belkaid, Y, and Rouse, BT. Natural regulatory T cells in infectious disease. Nat Immunol. (2005) 6:353–60. doi: 10.1038/ni1181
45. Hu, J, Kang, HJ, Liu, C, Hu, P, Yang, MM, and Zhou, FH. Response of regulatory T cells to classic heat stroke in mice. Exp Ther Med. (2018) 16:4609–15. doi: 10.3892/etm.2018.6766
46. Hu, J, Kang, H, Liu, C, Hu, P, Yang, M, and Zhou, F. Regulatory T cells could improve intestinal barrier dysfunction in heatstroke. Inflammation. (2019) 42:1228–38. doi: 10.1007/s10753-019-00983-6
47. Galli, SJ . New concepts about the mast cell. N Engl J Med. (1993) 328:257–65. doi: 10.1056/NEJM199301283280408
48. Mack, M, and Rosenkranz, AR. Basophils and mast cells in renal injury. Kidney Int. (2009) 76:1142–7. doi: 10.1038/ki.2009.320
49. Parsons, IT, Stacey, MJ, Faconti, L, Hill, N, O'Hara, J, Walter, E, et al. Histamine, mast cell tryptase and post-exercise hypotension in healthy and collapsed marathon runners. Eur J Appl Physiol. (2021) 121:1451–9. doi: 10.1007/s00421-021-04645-0
50. Mortaz, E, Redegeld, FA, van der Heijden, MW, Wong, HR, Nijkamp, FP, and Engels, F. Mast cell activation is differentially affected by heat shock. Exp Hematol. (2005) 33:944–52. doi: 10.1016/j.exphem.2005.05.004
51. Mangum, JE, Needham, KW, Sieck, DC, Ely, MR, Larson, EA, Peck, MC, et al. The effect of local passive heating on skeletal muscle histamine concentration: implications for exercise-induced histamine release. J Appl Physiol. (2022) 132:367–74. doi: 10.1152/japplphysiol.00740.2021
52. Romero, SA, McCord, JL, Ely, MR, Sieck, DC, Buck, TM, Luttrell, MJ, et al. Mast cell degranulation and de novo histamine formation contribute to sustained postexercise vasodilation in humans. J Appl Physiol. (2017) 122:603–10. doi: 10.1152/japplphysiol.00633.2016
Keywords: heat-related illness (HRI), macrophage, neutrophil, NKT (natural killer T) cells, mast cell (MC), NK cells
Citation: Goto H, Kinoshita M and Oshima N (2023) Heatstroke-induced acute kidney injury and the innate immune system. Front. Med. 10:1250457. doi: 10.3389/fmed.2023.1250457
Received: 30 June 2023; Accepted: 28 July 2023;
Published: 08 August 2023.
Edited by:
Takashi Oda, Tokyo Medical University Hachioji Medical Center, JapanReviewed by:
Eiji Kawamoto, Mie University Hospital, JapanCopyright © 2023 Goto, Kinoshita and Oshima. This is an open-access article distributed under the terms of the Creative Commons Attribution License (CC BY). The use, distribution or reproduction in other forums is permitted, provided the original author(s) and the copyright owner(s) are credited and that the original publication in this journal is cited, in accordance with accepted academic practice. No use, distribution or reproduction is permitted which does not comply with these terms.
*Correspondence: Hiroyasu Goto, aGdvdG9AbmRtYy5hYy5qcA==
Disclaimer: All claims expressed in this article are solely those of the authors and do not necessarily represent those of their affiliated organizations, or those of the publisher, the editors and the reviewers. Any product that may be evaluated in this article or claim that may be made by its manufacturer is not guaranteed or endorsed by the publisher.
Research integrity at Frontiers
Learn more about the work of our research integrity team to safeguard the quality of each article we publish.