- 1Division of Pulmonary and Critical Care Medicine, Department of Medicine, Lenox Hill Hospital - Northwell Health, New York, NY, United States
- 2Donald and Barbara Zucker School of Medicine at Hofstra/Northwell, Hempstead, NY, United States
- 3Department of Medicine, Lenox Hill Hospital - Northwell Health, New York, NY, United States
- 4Office of Clinical Research, The Feinstein Institutes for Medical Research, Northwell Health, Manhasset, NY, United States
Purpose: To systematically review and compare ultrasonographic methods and their utility in predicting non-invasive ventilation (NIV) outcomes.
Methods: A systematic review was performed using the PubMed, Medline, Embase, and Cochrane databases from January 2015 to March 2023. The search terms included the following: ultrasound, diaphragm, lung, prediction, non-invasive, ventilation, and outcomes. The inclusion criteria were prospective cohort studies on adult patients requiring non-invasive ventilation in the emergency department or inpatient setting.
Results: Fifteen studies were analyzed, which comprised of 1,307 patients (n = 942 for lung ultrasound score studies; n = 365 patients for diaphragm dysfunction studies). Lung ultrasound scores (LUS) greater than 18 were associated with NIV failure with a sensitivity 62–90.5% and specificity 60–91.9%. Similarly, a diaphragm thickening fraction (DTF) of less than 20% was also associated with NIV failure with a sensitivity 80–84.6% and specificity 76.3–91.5%.
Conclusion: Predicting NIV failure can be difficult by routine initial clinical impression and diagnostic work up. This systematic review emphasizes the importance of using lung and diaphragm ultrasound, in particular the lung ultrasound score and diaphragm thickening fraction respectively, to accurately predict NIV failure, including the need for ICU-level of care, requiring invasive mechanical ventilation, and resulting in higher rates of mortality.
Introduction
There are several contributors in predicting the outcome of non-invasive ventilation (NIV). Historically, multiple articles have demonstrated that the prediction of noninvasive ventilation (NIV) failure was based on components of the patient’s vital signs, level of consciousness, and degree of acidosis (1–6). This led to the development of a scale that considers heart rate, acidosis, consciousness, oxygenation, and respiratory rate (referred to as the HACOR scale) and is used to predict NIV failure, defined as the need for intubation after NIV intervention (7, 8). The scale was however limited in terms of predictive value in respiratory illnesses and thus an updated HACOR score was developed that considered baseline data such as acute respiratory distress syndrome (ARDS), septic shock, immunosuppression, organ failure, among other data (9). Additional risk factors beyond the HACOR scale for NIV failure include patient baseline severity scores (SOFA, APACHE II and SAPS II), delay between admission and NIV use, duration of NIV use, patient-ventilator asynchrony, number of fiberoptic bronchoscopies performed, and increased radiographic infiltrates within the first 24 hours (5, 10–13).
Point of care ultrasound (POCUS) in the fields of pulmonary and critical care medicine has received increased attention because of its rapid availability to assess and diagnose patients, accuracy, reproducibility, low-cost profile, and lack of harmful radiation. Numerous studies have attempted to utilize various ultrasonographic methods to ultimately predict NIV outcomes. However, given the variability of each technique and the multiple sites of interest for bedside ultrasound, it is challenging to comprehend the clinical significance and the generalized acceptance of specific ultrasound modalities.
The purpose of this study is to systematically review the literature to highlight the predictiveness of bedside ultrasound with regards to NIV outcomes as well as to compare the various ultrasonographic techniques that have been used.
Materials and methods
This study was conducted in accordance with the 2020 PRISMA (Preferred Reporting Items for Systematic Reviews and Meta-Analyses) statement and the PRISMA checklist (14). A systematic review of the literature from 2015 to 2023 on the role of ultrasound in predicting NIV outcomes was performed across a 4-database wide search including PubMed, Medline, Embase, and Cochrane. The queries were performed in March 2023 with the following search terms: ultrasound, diaphragm, lung, prediction, non-invasive, ventilation, and outcomes.
Inclusion/exclusion criteria
The inclusion criteria were adults 18 years of age and older, patients in the emergency department or hospitalized, with acute respiratory failure, receiving ultrasound assessments, and on non-invasive ventilation. The exclusion criteria were studies in languages other than English, pregnant patients, post-surgical patients, patients with neuromuscular disorders, using ultrasound to determine if patient needs to be advanced to NIV, using ultrasound to evaluate if patient can be extubated or weaned from invasive mechanical ventilation, and if the study involved drug interventions. Two investigators (M.K. and V.D.) reviewed the titles and abstracts of all articles that met the inclusion and exclusion criteria, and full-text articles were obtained to verify that the criteria were met.
Results
A flow diagram documenting the method of article identification and selection is shown in Figure 1. A total of 318 studies remained after eliminating 36 duplicate studies. The investigators independently reviewed the titles and abstracts, identifying 26 full-text articles for review. Application of the inclusion and exclusion criteria yielding 15 full-text articles for inclusion in the review (15–29).
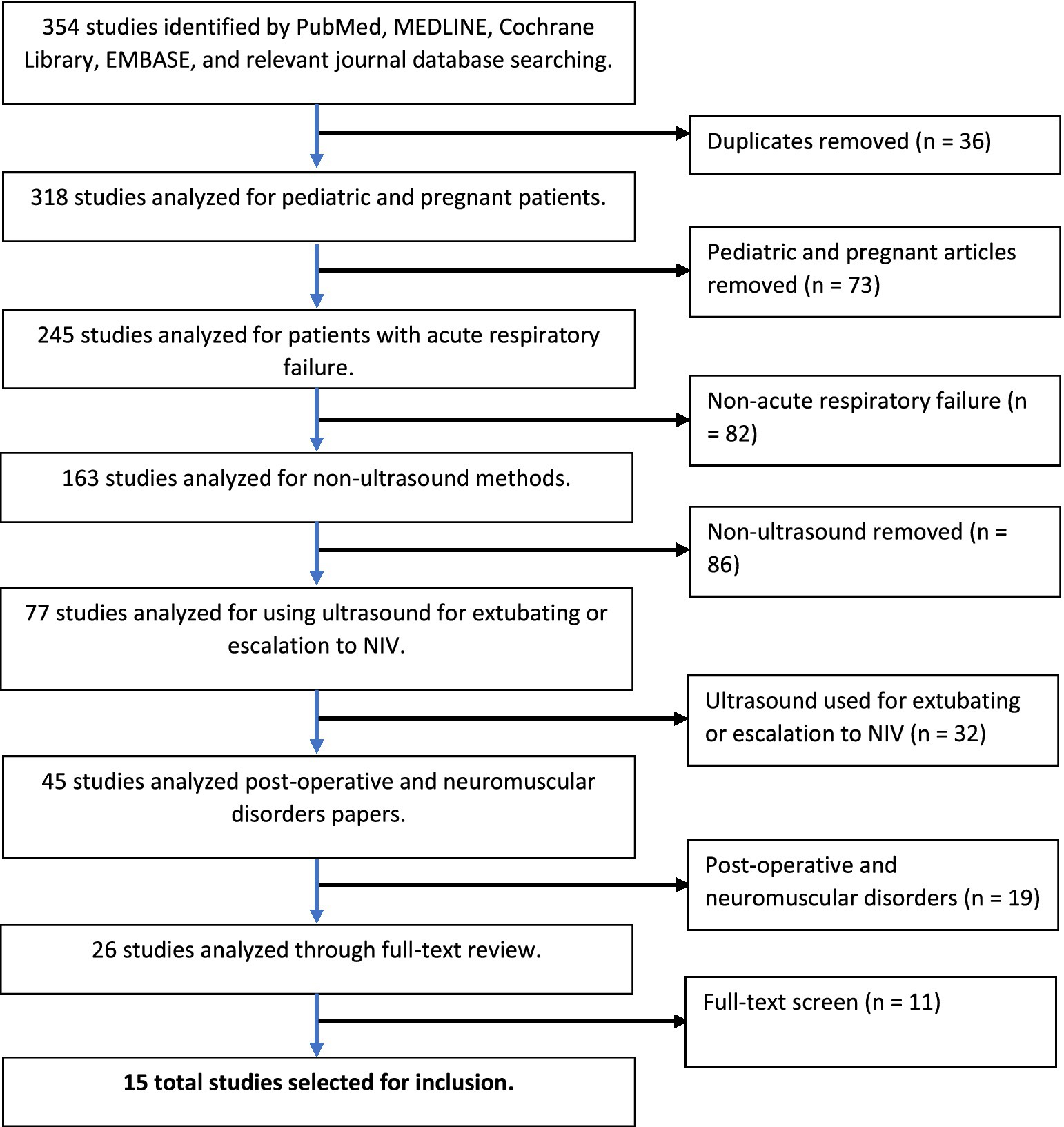
Figure 1. PRISMA (preferred reporting items for systematic reviews and meta-analyses) flowchart of literature search.
Lung ultrasound score
Several studies employ an ultrasonographic method of scoring lung pathology, namely the lung ultrasound score (LUS) (Table 1). A lung ultrasound score assessment is performed for multiple reasons, such as: perioperative oxygenation (31), predicting disease severity and mortality in ARDS (32), monitoring of lung aeration changes (after proning in ARDS, antimicrobial therapy in ventilator-associated pneumonia, selecting the ideal positive end-expiratory pressure, etc.) (33–35), weaning from mechanical ventilation (36), and as a prognosticator for respiratory failure (15, 18–20, 22, 28). There are multiple variations of the LUS, however arguably the more commonly utilized version of the LUS assessment consists of scanning a predetermined 6 regions per lung. Lung aeration of each region is graded between 0 to 3 depending on the ultrasound pattern visualized. In each region, points are allocated according to the following ultrasound pattern: normal = 0, well-defined B-lines = 1, coalescent B-lines = 2, and consolidation = 3. The total score for the LUS assessment therefore ranges from 0 to 36 (37) (Figure 2). A broader score, known as the integrated lung ultrasound score (i-LUS), was implemented during the coronavirus disease 2019 (COVID-19) pandemic that incorporates other important parameters seen with COVID-19 pneumonia. These additional parameters include: presence of pleural effusion (absent = 0, present = 1), presence of pericardial effusion (absent = 0, present = 1), measurement of IVC respiratory variation (<0–33%; absent = 0, present = 1), and diaphragm excursion (excursion >2 ± 0.5 cm was considered normal = 0, values below = 1) (18). Again, there are several other variations of the standard LUS (most of which are described in this present article) and these lung ultrasound score assessments have demonstrated positively in predicting outcomes for patients on NIV.
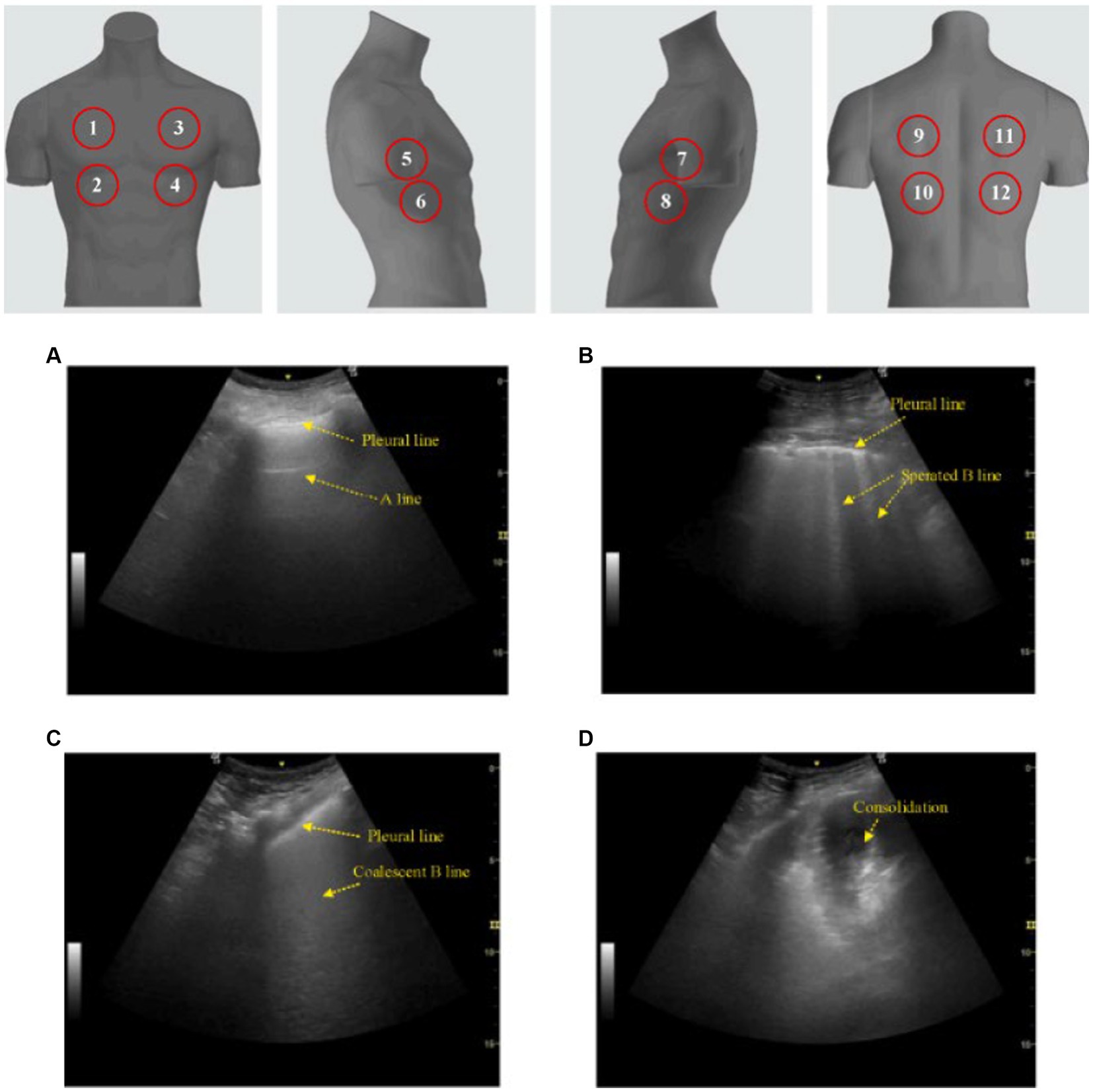
Figure 2. Lung ultrasound score (LUS). The classic LUS requires scanning 12 zones (upper image; 6 on each hemithorax) while scoring each zone from 0 to 3 based on pattern. (A) A score of 0 is a normal pattern. (B) A score of 1 requires at minimum 3 isolated or coalescent B-lines covering <50% of the screen without clear subpleural alterations. (C) A score of 2 is given when B-lines encompass >50% of the screen without clear subpleural alterations. (D) And finally, a score of 3 is made when consolidation is observed. Total scores range from 0 to 36. This image is reproduced with permission from the Elsevier Novel Coronavirus Center. No changes were made to this image (38).
Ahmed et al. performed a prospective observational study of 50 ICU patients who presented with acute respiratory failure and were indicated for NIV (including COPD with exacerbation, pneumonia with no or mild secretions, acute lung injury manifested by PaO2/FiO2 < 200, and acute congestive heart failure with pulmonary edema). Lung ultrasound score, HACOR score, and serum lactate were recorded on admission and after 12 h from NIV application. To predict NIV failure, LUS with a cut-off value of 18 on admission showed sensitivity 77.0%, specificity 60.0% (p = 0.001). A cut-off value of 15 after 12 h NIV use showed a sensitivity of 87.0% and a specificity of 75.0% (p < 0.001) (15).
A similar study of 120 patients admitted for COVID-19 pneumonia showed that the optimal cutoff for the baseline LUS score was 18 (sensitivity 62%, specificity 74%). Both mortality and requirement for invasive mechanical ventilation were increased with a baseline LUS score > 18 compared to patients with baseline LUS score between 0 and 18. The hazard ratio of invasive mechanical ventilation or death for LUS score was 1.12 per point (p = 0.0008) (30). Dell’Aquila et al. also examined COVID-19 patients in a prospective study of 143 patients. They noted that in the survivor group, patients had a median i-LUS score of 16, while the score was 20 in the non-survivor group (interquartile range 12–20 vs. 15–24; p = 0.005) (18).
In a prospective cohort study of 85 patients with COVID-19 respiratory failure, Biasucci et al. showed that LUS (denoted as “LUSsc”; median score of 12 for NIV failure and median score of 6 for NIC success) as well as the amount of involved sonographic lung areas (denoted as “LUSq”; median areas involved were 6 for NIV failure and median areas involved were 3 for NIC success) were significantly higher in patients who failed NIV (p < 0.001) (28). This was also seen in a larger study of 280 patients with COVID-19 pneumonia by Ji et al., which demonstrated that patients with a high LUS score (defined as LUS > 12) were more likely to have poor outcomes including requiring invasive mechanical ventilation, higher incidence of ARDS, and higher mortality rates compared to the lowest LUS score group (defined as LUS 0–1), with a specificity and sensitivity of 90.5 and 91.9%, respectively (19). Blair et al. utilized the mean LUS (mLUS) score (ranging from 0 to 3) across 12 lung zones. The study revealed that every increase in mLUS score at enrollment was associated with disease progression to ICU-level of care (adjusted hazard ratio [aHR], 3.61; 95% confidence interval [CI], 1.27–10.2; p = 0.016) as well as 28-day mortality (aHR, 3.10; 95% CI, 1.29–7.50; p = 0.012) (22).
Diaphragm dysfunction
In addition to the lung ultrasound score, point of care ultrasonography can be used to rapidly assess for many pathologies including diaphragm dysfunction (DD) (Table 2). Similar to other ultrasound modalities, diaphragm-related ultrasound is noninvasive, safe, and can serve as a repeatable bedside tool. Patients with significant hypoxia who require NIV have a risk of developing diaphragmatic impairment which may poorly affect outcomes, including the need for invasive mechanical ventilation. Numerous studies have employed ultrasonography to assess functionality of the diaphragm to assist in predicting outcomes for patients on NIV (16, 17, 21, 23–27). These studies assessed diaphragm thickness (DT) or the variation of diaphragmatic thickness fraction (DTF) between end-inspiration and end-expiration. DTF is calculated from the following formula: [(Thickness at end-inspiration − Thickness at end-expiration)/(Thickness at end expiration)] × 100, see Figure 3. A DTF, also known as change in diaphragmatic thickness (ΔTdi), <20% is consistent with diaphragmatic dysfunction (39) (Figure 4). For sake of consistency, this article will use the term DTF with knowledge that it is interchangeable with ΔTdi.
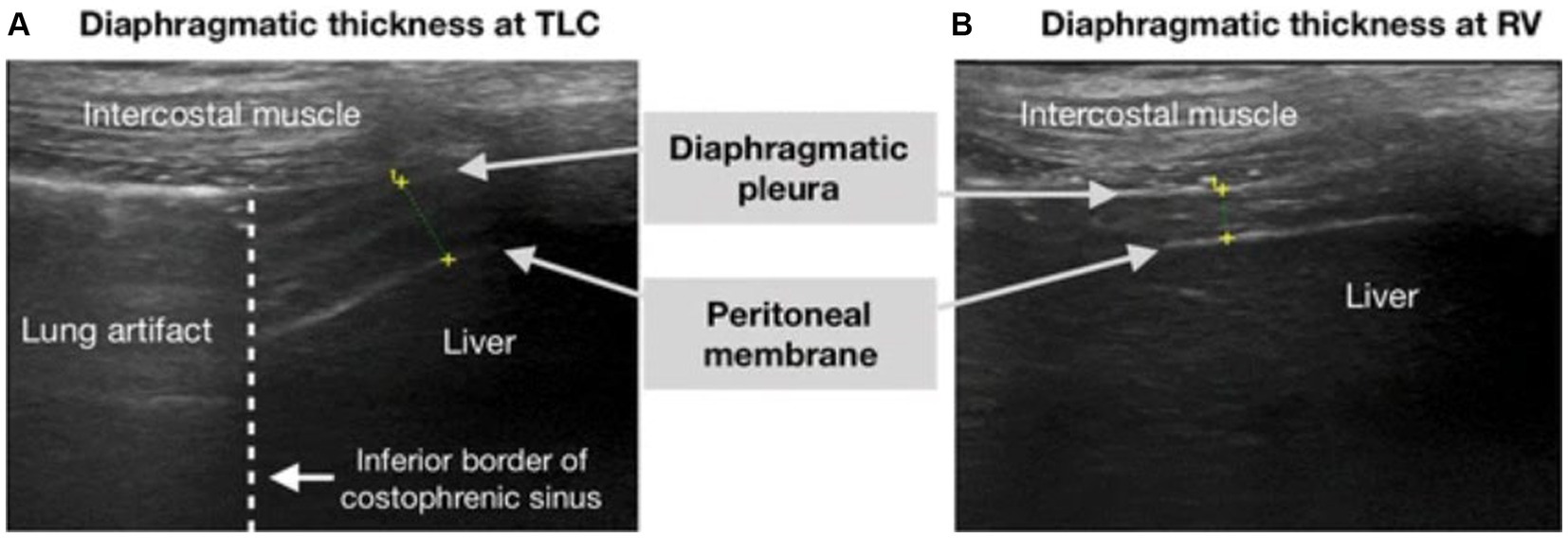
Figure 4. Obtaining diaphragm thickness fraction on ultrasound. Ultrasound image of the diaphragm on B-mode, showing the three layers of the diaphragm (hypoechoic diaphragm bordered by hyperechoic diaphragmatic pleura and the peritoneal membrane). Seen in (A) total lung capacity (TLC) and (B) residual volume, which is equivalent to end-inspiration and end-expiration, respectively. This image was reproduced with the permission of the Creative Commons license. No changes were made to this image. The Creative Commons Public Domain Dedication waiver (http://creativecommons.org/publicdomain/zero/1.0/) applies to this image (41).
Across the more recent studies which are highlighted in this article, diaphragm evaluation was performed on patients with an acute exacerbation of COPD (AECOPD) or with acute respiratory failure from SARS-COV2 infection. Marchioni et al. performed a prospective observational study using ultrasound to assess diaphragm dysfunction in patients with AECOPD and reported that patients with diaphragmatic dysfunction had a higher risk for NIV failure than those without DD (risk ratio = 4.4; p < 0.001). Change in diaphragm thickness (DTF) <20% during tidal volume was the predefined cutoff for identifying DD. The study investigated the correlation between ultrasound-assessed DD and the transdiaphragmatic pressure (Pdi) assessed using an invasive sniff maneuver [esophageal pressure (Pes) and gastric pressure (Pga) levels are recorded using balloon catheters before starting NIV; transdiaphragmatic generating pressure capacity (Pdi) was obtained by subtracting Pes from Pga (Pga – Pes) during a sniff maneuver], see Figure 5. It was reported that DTF highly correlated and had similar accuracy in identifying diaphragmatic dysfunction as with Pdi sniff (Pearson’s r = 0.81; p = 0.004). Lastly, the study demonstrated that DTF < 20% had better accuracy in predicting NIV failure than baseline pH value <7.25, as well as early changes in arterial pH and PaCO2 following initiation of NIV (AUCs 0.84, 0.51, 0.56, and 0.54, respectively; p < 0.0001) (17).
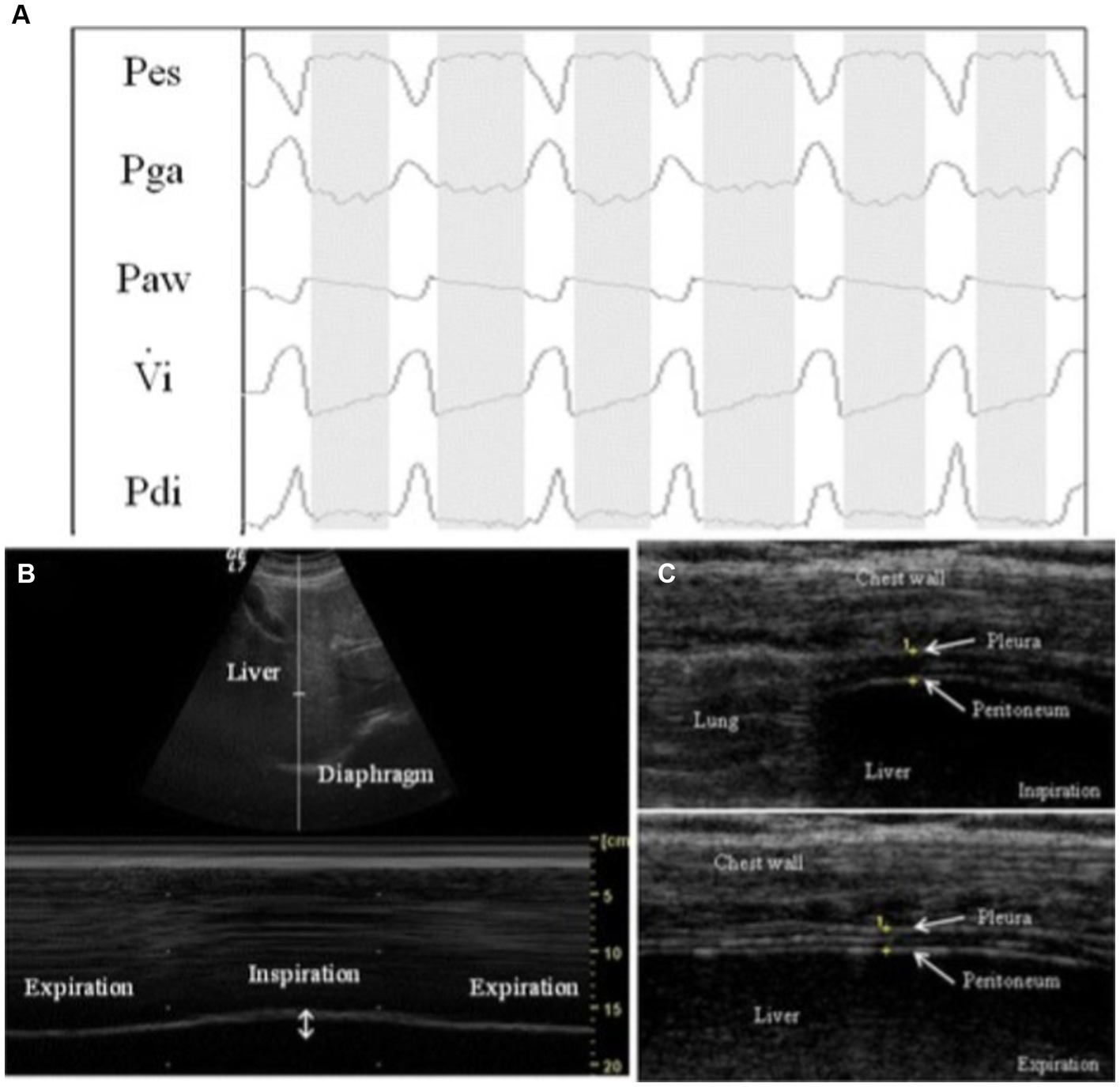
Figure 5. Example of respiratory tracings with ultrasound measurements during a pressure support of 0 cm H2O (PS0 step). (A) Respiratory tracings showing: Pes, esophageal pressure; Pga, gastric pressure; Paw, airway pressure; Vi, respiratory flow; Pdi, transdiaphragmatic pressure. The white columns represent inspiration and gray columns represent expiration phases. (B) Ultrasound view of diaphragm excursion during spontaneous breathing in B-mode (upper) and M-mode (lower). (C) Ultrasound view of the diaphragm in the zone of apposition during inspiration (upper) and during expiration (lower). The diaphragm is the three-layer structure in the middle consisting of a hypoechoic central layer sounded by an echogenic diaphragmatic pleurae and peritoneum, as indicated by the yellow arrows. This image was adopted with the permission of the Creative Commons license. No changes were made to this image. The Creative Commons Public Domain Dedication waiver (http://creativecommons.org/publicdomain/zero/1.0/) applies to this image (40).
Cammarota et al. similarly reported diaphragmatic excursion assessment 2 h after NIV initiation was a better predictor of NIV failure than pH, PaCO2, and left expiratory diaphragmatic thickness. Diaphragmatic excursion was greater in NIV successes than in NIV failures at initiation of NIV (1.92 [1.22–2.54] cm vs. 1.00 [0.60–1.41] cm, p = 0.02) and at 2 h after initiation of NIV (1.99 [1.63–2.54] cm vs. 1.20 [0.79–1.41] cm, p = 0.008), respectively (27). Diaphragmatic dysfunction was also evaluated through diaphragmatic excursion by Barbariol et al. in ICU patients admitted for acute respiratory failure. Data was collected right before starting NIV as well as 1 h after initiating NIV. Diaphragmatic dysfunction was defined as a diaphragmatic excursion of less than 1.00 cm. Out of 47 patients, the patients without diaphragmatic dysfunction had about a 10% increase in NIV success than patients with diaphragmatic dysfunction, which was not statistically significant (p = 0.54). They also performed ROC analysis and noted that when using diaphragmatic excursion as a predictor of NIV response the area under the curve was 0.53; the best sensitivity (58.1%) and specificity (62.5%) was obtained with a diaphragmatic excursion cut-off of 1.37 cm (29).
Elsayed et al. conducted a prospective observational study where patients with acute exacerbation of COPD (AECOPD) were categorized into successful and failed NIV groups and DTF was measured. Readings of DTF in the successful NIV group were ≥ 33–38% (p < 0.001) while failed NIV had DTF values ≤16–18% (p < 0.001). In addition, cut-off value of DTF < 26–29% on both sides (left hemidiaphragm% - right hemidiaphragm%) was associated with NIV failure with 96.67% sensitivity, 80–82.22% specificity, 76.3–78.4% positive predictive value (PPV), and a 97.3–97.4% negative predictive value (NPV) (16). In a similar study by Kocyigit et al. on patients with AECOPD in the emergency department, DD (defined as DTF of <20% during spontaneous breathing) had a high sensitivity of 84.6% (95% CI:54.6–98.1), specificity of 91.5% (95% CI:79.6–97.6), PPV 73.3% (95% CI:51.2–87.8), and NPV 95.6% (95% CI:85.7–98.7) (24). Antenora et al. underwent a pilot study on the prevalence and clinical consequences of diaphragmatic dysfunction diagnosed by ultrasonography during AECOPD and reported that DD (defined as DTF < 20% during spontaneous breathing) was found to be strongly correlated with NIV failure (p < 0.001, R2 = 0.27), longer ICU stay (p = 0.02, R2 = 0.13), prolonged mechanical ventilation (p = 0.023, R2 = 0.15), and need for tracheostomy (p = 0.006, R2 = 0.20) (21). In a study by Mercurio et al. on patients with de-novo acute respiratory failure, cut-off values for DTF were explored that would accurately predict NIV failure. It was determined that the cut-off value of DTF < 36.3% significantly predicted NIV failure (p < 0.0001) with sensitivity of 71.7% (95% CI 56.5–84.0) and specificity of 94.3% (95% CI 80.8–99.3) (26).
In SARS-COV2 patients, Corradi et al. underwent a single-center pilot study that reported in their univariate logistic regression analysis that CPAP failure was significantly associated with a low DTF (odds ratio [OR]: 0.673; p < 0.001) and high respiratory rate (OR: 1.572; p < 0.001), but only DTF reached statistical significance at multivariate analysis (OR: 0.681; p < 0.001) (23). The same investigators performed a separate study and noted that patients on NIV who developed adverse outcomes had thinner diaphragms than those who did not (2.0 vs. 2.2 mm, p = 0.001) (25).
Discussion
Predicting NIV failure remains a diagnostic dilemma in general practice. Recent literature shows that patients presenting with acute respiratory failure have an NIV failure rate around 31–50% with nearly 65% of NIV failures occurring within 1–48 h of NIV use (8, 42–44). Current methods of evaluating for impending NIV failure is based on a multitude of parameters, of which some include monitoring vital signs, evaluating for signs of respiratory distress on physical examination, obtaining arterial blood gas values, calculating severity scores (e.g., SOFA, APACHE II, etc.), serial chest radiographs, and so forth (3, 6–9, 13, 43, 45). Undoubtedly these measures are crucial, but ongoing monitoring and the time required to obtain all this information can be tedious and laborious. The main findings of this systematic review emphasize the effective ability of utilizing bedside ultrasound as a potential independent predictive tool for NIV outcomes.
This study demonstrates that point of care ultrasound of the lung (e.g., lung ultrasound score) and diaphragm (e.g., diaphragm thickening fraction) can predict NIV failure early on (15–19, 21–30). This is pivotal as a delay in early detection of potential NIV failure has been shown to increase length of hospital stay, delay endotracheal intubation and increase hospital morbidity and mortality (45–48). Also, this may serve as an additional tool in guiding clinicians when considering patient disposition with regard to deciding escalating level of monitoring to an intermediate care unit (i.e., stepdown unit) vs. an intensive care unit. Clinicians may also use these ultrasound tools to communicate with family members and/or healthcare proxies about suspected outcomes for the patient. Additionally, clinicians may use this information to proactively prepare for anticipated outcomes of NIV failure (such as longer ICU stay, prolonged mechanical ventilation, mortality, and need for tracheostomy) by either providing closer patient monitoring or even considering additional treatment modalities. A recent emergency department study evaluating COVID-19 patients showed that LUS improved prognostic stratification over clinical judgment alone and supported standardized disposition decisions (49).
In a more pragmatic way of interpreting these articles, one may suggest based on these findings that perhaps a lung ultrasound score greater than 18 (out of 36 total) in a patient places the individual at a higher risk for NIV failure (e.g., ICU admission, invasive mechanical ventilation, and mortality). Likewise, a low diaphragm thickness fraction (also known as change in diaphragm thickness) accurately predicts diaphragm dysfunction. A corresponding DTF value of less than 20% highly correlates with NIV failure. Diaphragm thickness and diaphragmatic excursion were evaluated in three of the nine diaphragm ultrasound studies that met inclusion criteria (25, 27, 29). The two studies in this review examining diaphragmatic excursion showed mixed results in terms of predicting NIV failure, whereas one of those studies concluded that diaphragm thickness was a better predictor of NIV outcomes compared to diaphragmatic excursion. The inconsistency of the results and lack of studies evaluating these two variables, diaphragm thickness and diaphragmatic excursion, make it difficult to interpret and utilize these ultrasonographic methods clinically. Currently, additional research is required to evaluate these ultrasound techniques in the setting of predicting NIV outcomes.
Although the outcomes of many of these studies are promising and exemplify the independent utility of performing bedside ultrasound to predict NIV failure, there are some limitations. Firstly, all of the aforementioned studies are small unblinded prospective observational studies and many are conducted at a single institution. In addition, a vast majority of these studies focus on acute respiratory failure from either COVID-19 or AECOPD, which decreases generalizability to other patient populations (e.g., congestive heart failure, post-operative, bacterial pneumonia, etc.). Moreover, the timing of the ultrasound evaluation and the treatment course of the patients are not standardized, making the external validity of the results difficult to establish. There were also many lung ultrasound scoring systems (e.g., LUS, mLUS, i-LUS, etc.) and different cut-off values for both LUS and DTF to determine the same outcomes. This heterogeneity makes it difficult to perform a pooled analysis. Finally, there were unfortunately only two studies that fit the inclusion criteria that examined diaphragmatic thickness and two studies that examined diaphragmatic excursion as predictors for NIV failure. Hence, much of the conversation regarding diaphragmatic dysfunction centered on the diaphragm thickening fraction instead.
Conclusion
The results of this systematic review emphasize the clinical utility of performing bedside ultrasonography to predict non-invasive ventilation outcomes. Lack of early recognition of impending NIV failure in patients can be deleterious as these individuals often require ICU admission, invasive mechanical ventilation, and have higher mortality rates. Both the LUS and DTF (i.e., indicators of lung parenchymal injury and diaphragmatic dysfunction, respectively) have been shown to accurately predict NIV outcomes. Further larger prospective cohort studies are warranted to contribute to standardizing LUS and DTF across a wide range of etiologies of acute respiratory failure. Moreover, additional investigations are necessary to evaluate whether a combination of the LUS, DTF, and HACOR score can best predict NIV outcomes.
Data availability statement
The original contributions presented in the study are included in the article/supplementary material, further inquiries can be directed to the corresponding author.
Ethics statement
Ethical approval was not required for the study involving humans in accordance with the local legislation and institutional requirements. Written informed consent to participate in this study was not required from the participants or the participants’ legal guardians/next of kin in accordance with the national legislation and the institutional requirements.
Author contributions
MK and BM: conceptualization. MK, VD, and BM: methodology and writing – review and editing. MK and VD: investigation, data curation, and original draft preparation. MK and VR: image contributions. BM: visualization and supervision. All authors contributed to the article and approved the submitted version.
Conflict of interest
The authors declare that the research was conducted in the absence of any commercial or financial relationships that could be construed as a potential conflict of interest.
Publisher’s note
All claims expressed in this article are solely those of the authors and do not necessarily represent those of their affiliated organizations, or those of the publisher, the editors and the reviewers. Any product that may be evaluated in this article, or claim that may be made by its manufacturer, is not guaranteed or endorsed by the publisher.
References
1. Antonelli, M, Conti, G, Esquinas, A, Montini, L, Maggiore, SM, Bello, G, et al. A multiple-center survey on the use in clinical practice of noninvasive ventilation as a first-line intervention for acute respiratory distress syndrome. Crit Care Med. (2007) 35:18–25. doi: 10.1097/01.CCM.0000251821.44259.F3
2. Nicolini, A, Ferraioli, G, Ferrari-Bravo, M, Barlascini, C, Santo, M, and Ferrera, L. Early non-invasive ventilation treatment for respiratory failure due to severe community-acquired pneumonia. Clin Respir J. (2016) 10:98–103. doi: 10.1111/crj.12184
3. Yoshida, Y, Takeda, S, Akada, S, Hongo, T, Tanaka, K, and Sakamoto, A. Factors predicting successful noninvasive ventilation in acute lung injury. J Anesth. (2008) 22:201–6. doi: 10.1007/s00540-008-0637-z
4. Rana, S, Jenad, H, Gay, PC, Buck, CF, Hubmayr, RD, and Gajic, O. Failure of non-invasive ventilation in patients with acute lung injury: observational cohort study. Crit Care. (2006) 10:R79. doi: 10.1186/cc4923
5. Carrillo, A, Gonzalez-Diaz, G, Ferrer, M, Martinez-Quintana, ME, Lopez-Martinez, A, Llamas, N, et al. Non-invasive ventilation in community-acquired pneumonia and severe acute respiratory failure. Intensive Care Med. (2012) 38:458–66. doi: 10.1007/s00134-012-2475-6
6. Antonelli, M, Conti, G, Moro, ML, Esquinas, A, Gonzalez-Diaz, G, Confalonieri, M, et al. Predictors of failure of noninvasive positive pressure ventilation in patients with acute hypoxemic respiratory failure: a multi-center study. Intensive Care Med. (2001) 27:1718–28. doi: 10.1007/s00134-001-1114-4
7. Duan, J, Han, X, Bai, L, Zhou, L, and Huang, S. Assessment of heart rate, acidosis, consciousness, oxygenation, and respiratory rate to predict noninvasive ventilation failure in hypoxemic patients. Intensive Care Med. (2017) 43:192–9. doi: 10.1007/s00134-016-4601-3
8. Duan, J, Wang, S, Liu, P, Han, X, Tian, Y, Gao, F, et al. Early prediction of noninvasive ventilation failure in COPD patients: derivation, internal validation, and external validation of a simple risk score. Ann Intensive Care. (2019) 9:108. doi: 10.1186/s13613-019-0585-9
9. Duan, J, Chen, L, Liu, X, Bozbay, S, Liu, Y, Wang, K, et al. An updated HACOR score for predicting the failure of noninvasive ventilation: a multicenter prospective observational study. Crit Care. (2022) 26:196. doi: 10.1186/s13054-022-04060-7
10. Di Marco, F, Centanni, S, Bellone, A, Messinesi, G, Pesci, A, Scala, R, et al. Optimization of ventilator setting by flow and pressure waveforms analysis during noninvasive ventilation for acute exacerbations of COPD: a multicentric randomized controlled trial. Crit Care. (2011) 15:R283. doi: 10.1186/cc10567
11. Demoule, A, Girou, E, Richard, J-C, Taille, S, and Brochard, L. Benefits and risks of success or failure of noninvasive ventilation. Intensive Care Med. (2006) 32:1756–65. doi: 10.1007/s00134-006-0324-1
12. Riviere, S, Monconduit, J, Zarka, V, Massabie, P, Boulet, S, Dartevelle, P, et al. Failure of noninvasive ventilation after lung surgery: a comprehensive analysis of incidence and possible risk factors. Eur J Cardiothorac Surg. (2011) 39:769–76. doi: 10.1016/j.ejcts.2010.08.016
13. Adda, M, Coquet, I, Darmon, M, Thiery, G, Schlemmer, B, and Azoulay, E. Predictors of noninvasive ventilation failure in patients with hematologic malignancy and acute respiratory failure. Crit Care Med. (2008) 36:2766–72. doi: 10.1097/CCM.0b013e31818699f6
14. Page, MJ, McKenzie, JE, Bossuyt, PM, Boutron, I, Hoffmann, TC, Mulrow, CD, et al. The PRISMA 2020 statement: an updated guideline for reporting systematic reviews. BMJ. (2021) 372:n71. doi: 10.1136/bmj.n71
15. Ahmed, WMK, Samir, M, Andraos, AW, and Hosny, M. Radiological and clinical perspectives to predict failure of non-invasive ventilation in acute respiratory failure. Egypt J Crit Care Med. (2022). doi: 10.1097/EJ9.0000000000000047
16. Elsayed, AA, Neanaa, EHM, and Beshey, BN. Diaphragmatic impairment as a predictor of invasive ventilation in acute exacerbation of chronic obstructive pulmonary disease patients. Egypt J Anaesth. (2022) 38:334–41. doi: 10.1080/11101849.2022.2085975
17. Marchioni, A, Castaniere, I, Tonelli, R, Fantini, R, Fontana, M, Tabbì, L, et al. Ultrasound-assessed diaphragmatic impairment is a predictor of outcomes in patients with acute exacerbation of chronic obstructive pulmonary disease undergoing noninvasive ventilation. Crit Care. (2018) 22:109. doi: 10.1186/s13054-018-2033-x
18. Dell’Aquila, P, Raimondo, P, Racanelli, V, de Luca, P, de Matteis, S, Pistone, A, et al. Integrated lung ultrasound score for early clinical decision-making in patients with COVID-19: results and implications. Ultrasound J. (2022) 14:21. doi: 10.1186/s13089-022-00264-8
19. Ji, L, Cao, C, Gao, Y, Zhang, W, Xie, Y, Duan, Y, et al. Prognostic value of bedside lung ultrasound score in patients with COVID-19. Crit Care. (2020) 24:700. doi: 10.1186/s13054-020-03416-1
20. Yasukawa, K, Minami, T, Boulware, DR, Shimada, A, and Fischer, EA. Point-of-care lung ultrasound for COVID-19: findings and prognostic implications from 105 consecutive patients. J Intensive Care Med. (2021) 36:334–42. doi: 10.1177/0885066620988831
21. Antenora, F, Fantini, R, Iattoni, A, Castaniere, I, Sdanganelli, A, Livrieri, F, et al. Prevalence and outcomes of diaphragmatic dysfunction assessed by ultrasound technology during acute exacerbation of COPD: a pilot study. Respirology. (2017) 22:338–44. doi: 10.1111/resp.12916
22. Blair, PW, Siddharthan, T, Liu, G, Bai, J, Cui, E, East, J, et al. Point-of-care lung ultrasound predicts severe disease and death due to COVID-19: a prospective cohort study. Crit Care Explor. (2022) 4:e0732. doi: 10.1097/CCE.0000000000000732
23. Corradi, F, Vetrugno, L, Orso, D, Bove, T, Schreiber, A, Boero, E, et al. Diaphragmatic thickening fraction as a potential predictor of response to continuous positive airway pressure ventilation in Covid-19 pneumonia: a single-center pilot study. Respir Physiol Neurobiol. (2021) 284:103585. doi: 10.1016/j.resp.2020.103585
24. Kocyigit, H, Gunalp, M, Genc, S, Oguz, AB, Koca, A, and Polat, O. Diaphragm dysfunction detected with ultrasound to predict noninvasive mechanical ventilation failure: a prospective cohort study. Am J Emerg Med. (2021) 45:202–7. doi: 10.1016/j.ajem.2020.08.014
25. Corradi, F, Isirdi, A, Malacarne, P, Santori, G, Barbieri, G, Romei, C, et al. Low diaphragm muscle mass predicts adverse outcome in patients hospitalized for COVID-19 pneumonia: an exploratory pilot study. Minerva Anestesiol. (2021) 87:432–8. doi: 10.23736/S0375-9393.21.15129-6
26. Mercurio, G, D’Arrigo, S, Moroni, R, Grieco, DL, Menga, LS, Romano, A, et al. Diaphragm thickening fraction predicts noninvasive ventilation outcome: a preliminary physiological study. Crit Care. (2021) 25:219. doi: 10.1186/s13054-021-03638-x
27. Cammarota, G, Sguazzotti, I, Zanoni, M, Messina, A, Colombo, D, Vignazia, GL, et al. Diaphragmatic ultrasound assessment in subjects with acute Hypercapnic respiratory failure admitted to the emergency department. Respir Care. (2019) 64:1469–77. doi: 10.4187/respcare.06803
28. Biasucci, DG, Buonsenso, D, Piano, A, Bonadia, N, Vargas, J, Settanni, D, et al. Lung ultrasound predicts non-invasive ventilation outcome in COVID-19 acute respiratory failure: a pilot study. Minerva Anestesiol. (2021) 87:1006–16. doi: 10.23736/S0375-9393.21.15188-0
29. Barbariol, F, Deana, C, Guadagnin, GM, Cammarota, G, Vetrugno, L, and Bassi, F. Ultrasound diaphragmatic excursion during non-invasive ventilation in ICU: a prospective observational study. Acta Biomed. (2021) 92:e2021269. doi: 10.23750/abm.v92i3.11609
30. Lichter, Y, Topilsky, Y, Taieb, P, Banai, A, Hochstadt, A, Merdler, I, et al. Lung ultrasound predicts clinical course and outcomes in COVID-19 patients. Intensive Care Med. (2020) 46:1873–83. doi: 10.1007/s00134-020-06212-1
31. Patel, SK, Bansal, S, Puri, A, Taneja, R, and Sood, N. Correlation of perioperative atelectasis with duration of anesthesia, pneumoperitoneum, and length of surgery in patients undergoing laparoscopic cholecystectomy. Cureus. (2022) 14:e24261. doi: 10.7759/cureus.24261
32. Skopljanac, I, Ivelja, MP, Barcot, O, Brdar, I, Dolic, K, Polasek, O, et al. Role of lung ultrasound in predicting clinical severity and fatality in COVID-19 pneumonia. J Pers Med. (2021) 11:757. doi: 10.3390/jpm11080757
33. Heldeweg, MLA, Mousa, A, van Ekeren, J, Lieveld, AWE, Walburgh-Schmidt, RS, Smit, JM, et al. Lung ultrasound to predict gas-exchange response to prone positioning in COVID-19 patients: a prospective study in pilot and confirmation cohorts. J Crit Care. (2023) 73:154173. doi: 10.1016/j.jcrc.2022.154173
34. Ammar, MA, Hilal, A, and Abdalla, W. The role of lung ultrasound in procalcitonin-guided antibiotic discontinuation in ventilator-associated pneumonia. Indian J Anaesth. (2022) 66:431–5. doi: 10.4103/ija.ija_989_21
35. Tang, K-Q, Yang, S-L, Zhang, B, Liu, H-X, Ye, D-Y, Zhang, H-Z, et al. Ultrasonic monitoring in the assessment of pulmonary recruitment and the best positive end-expiratory pressure. Medicine (Baltimore). (2017) 96:e8168. doi: 10.1097/MD.0000000000008168
36. Soliman, SB, Ragab, F, Soliman, RA, Gaber, A, and Kamal, A. Chest ultrasound in predication of weaning failure. Open Access Maced J Med Sci. (2019) 7:1143–7. doi: 10.3889/oamjms.2019.277
37. Dargent, A, Chatelain, E, Si-Mohamed, S, Simon, M, Baudry, T, Kreitmann, L, et al. Lung ultrasound score as a tool to monitor disease progression and detect ventilator-associated pneumonia during COVID-19-associated ARDS. Heart Lung. (2021) 50:700–5. doi: 10.1016/j.hrtlng.2021.05.003
38. Xing, W, He, C, Li, J, Qin, W, Yang, M, Li, G, et al. Automated lung ultrasound scoring for evaluation of coronavirus disease 2019 pneumonia using two-stage cascaded deep learning model. Biomed Signal Process Control. (2022) 75:103561. doi: 10.1016/j.bspc.2022.103561
39. Ali, ER, and Mohamad, AM. Diaphragm ultrasound as a new functional and morphological index of outcome, prognosis and discontinuation from mechanical ventilation in critically ill patients and evaluating the possible protective indices against VIDD. Egypt J Chest Dis Tuberculosis. (2017) 66:339–51. doi: 10.1016/j.ejcdt.2016.10.006
40. Umbrello, M, Formenti, P, Longhi, D, Galimberti, A, Piva, I, Pezzi, A, et al. Diaphragm ultrasound as indicator of respiratory effort in critically ill patients undergoing assisted mechanical ventilation: a pilot clinical study. Crit Care. (2015) 19:161. doi: 10.1186/s13054-015-0894-9
41. Pirompanich, P, and Romsaiyut, S. Use of diaphragm thickening fraction combined with rapid shallow breathing index for predicting success of weaning from mechanical ventilator in medical patients. J Intensive Care. (2018) 6:6. doi: 10.1186/s40560-018-0277-9
42. Korula, PJ, Nayyar, V, Stachowski, E, Karuppusami, R, and Peter, JV. An observational study on the practice of noninvasive ventilation at a tertiary level Australian intensive care unit. Aust Crit Care. (2020) 33:89–96. doi: 10.1016/j.aucc.2018.11.067
43. Chen, Q, Liu, M, Liu, B, Li, W, Gao, D, Xie, L, et al. Predictive factors for failure of noninvasive ventilation in adult intensive care unit: a retrospective clinical study. Can Respir J. (2020) 2020:1324348–7. doi: 10.1155/2020/1324348
44. Ozyilmaz, E, Ugurlu, AO, and Nava, S. Timing of noninvasive ventilation failure: causes, risk factors, and potential remedies. BMC Pulm Med. (2014) 14:19. doi: 10.1186/1471-2466-14-19
45. Ghazala, L, Hatipoğlu, U, Devnani, T, Covert, E, Hanks, J, Edwards, K, et al. Duration of noninvasive ventilation is not a predictor of clinical outcomes in patients with acute exacerbation of COPD and respiratory failure. Can J Respir Ther. (2021) 57:113–8. doi: 10.29390/cjrt-2021-021
46. Liengswangwong, W, Yuksen, C, Thepkong, T, Nakasint, P, and Jenpanitpong, C. Early detection of non-invasive ventilation failure among acute respiratory failure patients in the emergency department. BMC Emerg Med. (2020) 20:80. doi: 10.1186/s12873-020-00376-1
47. Bellani, G, Laffey, JG, Pham, T, Madotto, F, Fan, E, Brochard, L, et al. Noninvasive ventilation of patients with acute respiratory distress syndrome. Insights from the LUNG SAFE study. Am J Respir Crit Care Med. (2017) 195:67–77. doi: 10.1164/rccm.201606-1306OC
48. Bai, L, Ding, F, Xiong, W, Shu, W, Jiang, L, Liu, Y, et al. Early assessment of the efficacy of noninvasive ventilation tested by HACOR score to avoid delayed intubation in patients with moderate to severe ARDS. Ther Adv Respir Dis. (2022) 16:17534666221081042. doi: 10.1177/17534666221081042
Keywords: non-invasive, ventilation, outcomes, lung, ultrasound, diaphragm, dysfunction
Citation: Kheir M, Dong V, Roselli V and Mina B (2023) The role of ultrasound in predicting non-invasive ventilation outcomes: a systematic review. Front. Med. 10:1233518. doi: 10.3389/fmed.2023.1233518
Edited by:
Jun Duan, First Affiliated Hospital of Chongqing Medical University, ChinaReviewed by:
Carmen Silvia Valente Barbas, University of São Paulo, BrazilCristian Deana, Azienda Sanitaria Universitaria Integrata di Udine, Italy
Copyright © 2023 Kheir, Dong, Roselli and Mina. This is an open-access article distributed under the terms of the Creative Commons Attribution License (CC BY). The use, distribution or reproduction in other forums is permitted, provided the original author(s) and the copyright owner(s) are credited and that the original publication in this journal is cited, in accordance with accepted academic practice. No use, distribution or reproduction is permitted which does not comply with these terms.
*Correspondence: Matthew Kheir, bWtoZWlyMUBub3J0aHdlbGwuZWR1