- 1Laboratory of Clinical Virology, WHO Regional Reference Laboratory for Poliomyelitis and Measles for the EMR, Institut Pasteur de Tunis, University of Tunis El Manar, Tunis, Tunisia
- 2LR 20 IPT 02 Laboratory of Virus, Host and Vectors, Institut Pasteur de Tunis, University of Tunis El Manar, Tunis, Tunisia
- 3Istituto Zooprofilattico Sperimentale dell'Abruzzo e del Molise, Teramo, Italy
- 4Service of External Consultants, Institut Pasteur de Tunis, Tunis, Tunisia
Background: The severe acute respiratory syndrome coronavirus 2 (SARS-CoV-2) is responsible for serious respiratory infections in humans. Even in the absence of respiratory symptoms, gastrointestinal (GI) signs were commonly reported in adults and children. Thus, oral–fecal transmission was suspected as a possible route of infection. The objective of this study was to describe RNA shedding in nasopharyngeal and stool samples obtained from asymptomatic and symptomatic children and to investigate virus viability.
Methods: This study included 179 stool and 191 nasopharyngeal samples obtained from 71 children, which included symptomatic (n = 64) and asymptomatic (n = 7) ones. They were collected every 7 days from the onset of the infection until negativation. Viral RNA was detected by real-time RT-PCR, targeting the N and ORF1 genes. Whole-genome sequencing was performed for positive cases. Viral isolation was assessed on Vero cells, followed by molecular detection confirmation.
Results: All cases included in this study (n = 71) were positive in their nasopharyngeal samples. SARS-CoV-2 RNA was detected in 36 stool samples obtained from 15 out of 71 (21.1%) children; 13 were symptomatic and two were asymptomatic. Excretion periods varied from 7 to 21 days and 7 to 14 days in nasopharyngeal and fecal samples, respectively. Four variants were detected: Alpha (n = 3), B.1.160 (n = 3), Delta (n = 7), and Omicron (n = 1). Inoculation of stool samples on cell culture showed no specific cytopathic effect. All cell culture supernatants were negative for RT-qPCR.
Conclusion: Our study demonstrated nasopharyngeal and fecal shedding of SARS-CoV-2 RNA by children up to 21 and 14 days, respectively. Fecal shedding was recorded in symptomatic and asymptomatic children. Nevertheless, SARS-CoV-2 was not isolated from positive stool samples.
1. Introduction
At the beginning of the coronavirus disease 2019 (COVID-19) pandemic, available data suggested that the severe acute respiratory syndrome coronavirus 2 virus (SARS-CoV-2) was able to affect adults more than children. Up to May 2020, pediatric infections were limited to 1–5% of total recorded cases (1–4). Rapidly, several waves of COVID-19 have occurred around the world as a consequence of the emergence of multiple variants of concern (VOCs) (5–9). An increase in the number of pediatric cases was recorded, especially with the emergence of the Delta and Omicron variants (4, 10–14).
COVID-19 is mainly characterized by severe upper and lower respiratory tract infections in humans (15–19). Pediatric disease was, in general, less severe; most of the cases were asymptomatic or developed mild signs (14). Nevertheless, severe cases were also reported, requiring hospitalization and intensive care unit admission (4, 14, 20–22). Gastrointestinal (GI) signs were frequently reported, even in the absence of respiratory symptoms (3), estimated between 2 and 79% of cases according to different studies (3, 23–26). Diarrhea, vomiting, and nausea were estimated at 8.8–49.5%, 4.2–15.9%, and 4.2–29.4% of cases, respectively (26–33). Indeed, SARS-CoV-2 S protein binding to the host cell angiotensin-converting enzyme 2 (ACE2) receptor mediates viral entry. Although ACE2 is present throughout the respiratory tract (34), its expression is relatively low compared to the gastrointestinal tract, kidney, and myocardium (35). The most common GI symptom was diarrhea, which was generally noticed during the first 8 days of the infection (33, 36). Regarding child infection, GI was described as having a higher incidence, especially diarrhea and vomiting, estimated at 8–35.6% and 6.5–66.7%, respectively (33, 37–40). Shedding of SARS-CoV-2 RNA in stool samples was reported (3, 19, 41, 42). Thus, fecal–oral transmission was considered a possible route for SARS-CoV-2 transmission (41). Nevertheless, little is known about the virus shedding according to different variants and the duration of excretion, especially in pediatric patients and in symptomatic and asymptomatic cases. Furthermore, data on virus viability in positive stool samples using real-time PCR (RT-qPCR) are controversial (43). Several studies proved the absence of the live SARS-CoV-2 virus in feces (44–47), while two studies reported the possible presence of an infectious virus (48, 49).
This study aimed to investigate the excretion of SARS-CoV-2 RNA in nasopharyngeal and stool samples obtained from symptomatic and asymptomatic COVID-19 pediatric cases. Additionally, the virus viability of positive stool samples was explored by cell culture and confirmed by specific molecular detection applied to the cell culture product.
2. Materials and methods
2.1. Ethics statement
This study was approved by the Medical Ethics Committee of the Bechir Hamza Children’s Hospital of Tunis, Tunisia, under the reference “12/2021.” It was performed in accordance with the ethical standards of the 1964 Declaration of Helsinki and its later amendments, or comparable ethical standards. Written consent was obtained from their parents or their legal tutors.
2.2. Studied samples
A total of 179 stool samples and 191 nasopharyngeal swabs were collected from 71 children between February 2021 and January 2022 at the Pasteur Institute of Tunis, in a pandemic context and after the obtention of their parents or their legal tutors’ consent. Details of each collected sample are listed in Supplementary Table 1. The study included 28 boys and 43 girls, with a sex ratio equivalent to 0.65. Their age ranged from 1 month to 18 years old, with a median age of 15 years. Patients who tested positive for SARS-CoV-2 RNA in the nasopharyngeal swab further underwent stool sample collection from these patients; nasopharyngeal swabs and stools were collected every 7 days until negative results were obtained (Figure 1). Two groups were considered. Group 1 included 160 stool samples collected from COVID-19 symptomatic children (n = 64), and Group 2 included 19 stool samples collected from COVID-19 asymptomatic children (n = 7), among the contacts of symptomatic cases (Table 1). According to the WHO Living Guidance for Clinical Management of COVID-19, symptomatic cases were defined as patients with mild, moderate, severe, or critical signs of COVID-19. Asymptomatic cases were defined as COVID-19 patients, confirmed by SARS-CoV-2 real-time PCR on nasopharyngeal samples with no signs or symptoms of an illness or disease (50).
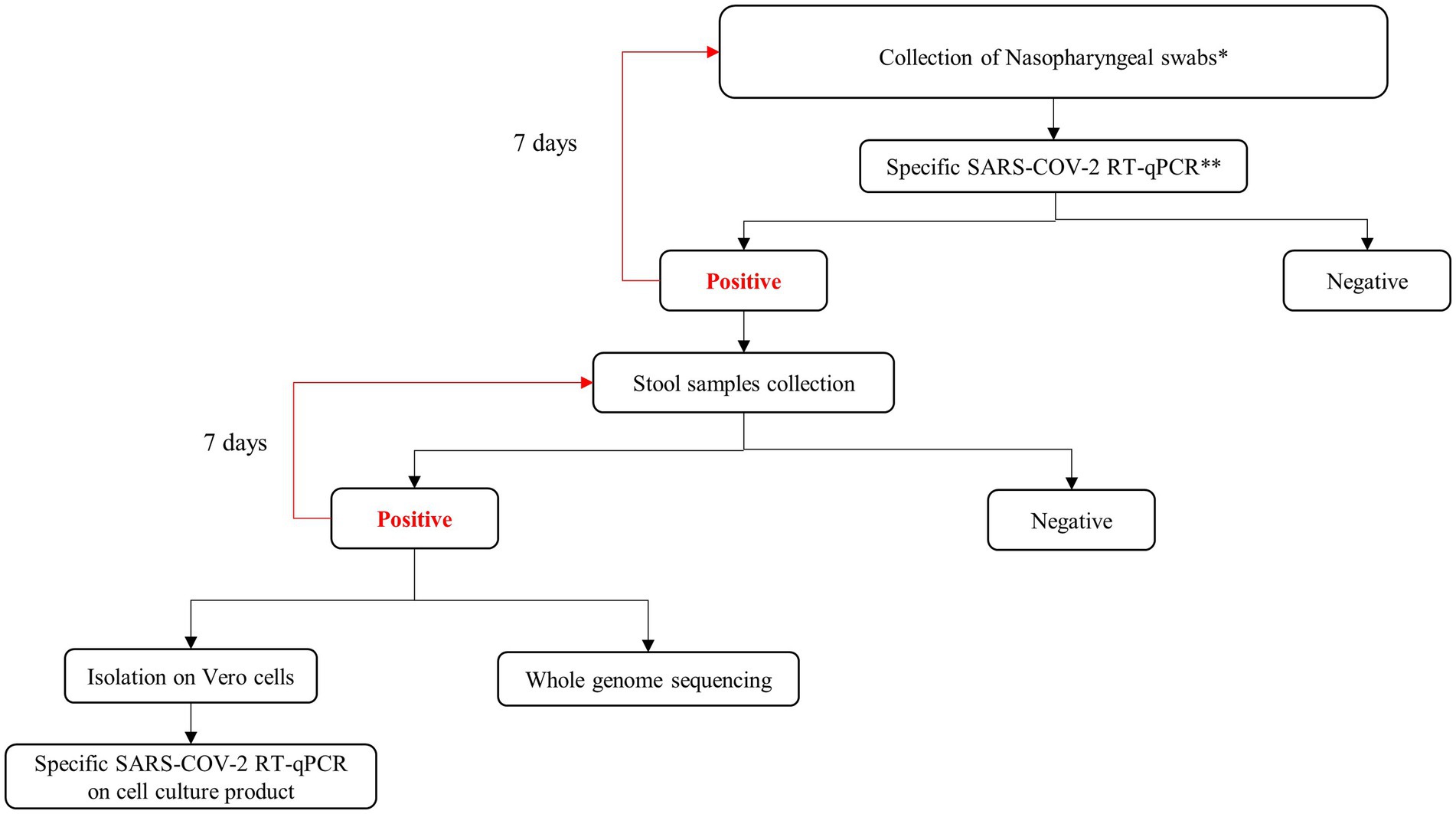
Figure 1. Workflow presenting the methodology used in this study. *Sample were collected from suspected COVID-19 pediatric cases and pediatric contact of confirmed adult cases; **WHO recommended protocol.
2.3. Nucleic acid extraction and detection by PCR
Nasopharyngeal and stool samples were processed in accordance with recommended good laboratory practices. Stool samples were treated with PBS/chloroform (1%) and centrifuged at 2500× g for 30 min, according to the WHO protocol for stool sample treatment (51–53). Viral RNA was extracted from 140 μL of the supernatants of nasopharyngeal and stool samples using the QIAamp Viral RNA Mini Kit (Qiagen, Hilden, Germany), according to the manufacturer’s instructions (54). The presence of SARS-CoV-2 RNA was determined with RT-qPCR using HKU (55) and IPT2-IPT4 protocols (Institut Pasteur, Paris) (56) as previously described (57) (Figure 1).
2.4. Whole-genome sequencing (WGS)
The whole genome of SARS-CoV-2 was obtained by next-generation sequencing using the COVIDSeq Test (Illumina Inc., San Diego, CA, USA) as previously described (58, 59) (Figure 1). The library preparation process used validated protocols at the “National Reference Centre for Whole-Genome Sequencing of microbial pathogens at Istituto Zooprofilattico Sperimentale dell’Abruzzo e del Molise IZSAM,” with the Hamilton Microlab STAR Liquid Handling System (Hamilton Robotics, Reno, NV, USA). NGS sequencing was achieved with the NextSeq 1,000 (Illumina Inc., San Diego, CA, USA), which provided read length data of 2 × 150 bp.
2.5. SARS-CoV-2 variant identification
Data analysis was automatically performed at the end of the sequencing run using the GENPAT platform at IZSAM in Teramo, as described in Molini et al. (59) and Di Pasquale et al. (60). Mapping to the Wuhan-Hu-1 reference genome (accession number NC_045512) was performed with the BWA tool (61), after quality control and trimming of the reads using FastQC and Trimmomatic (62). The consensus sequence was obtained using the iVar tool (63). The identification of SARS-CoV-2 lineage and sub-lineage was performed with the Pangolin (64) and Nextclade tools via the web1, 2 (65). Boxplot was used to visualize our results (66).
2.6. Statistical analysis
A chi-square test was performed using R software (67), which evaluated whether there was a significant association between symptomatic and asymptomatic children. Statistical significance was determined using 95% confidence intervals.
2.7. Virus isolation
The stool sample was treated as previously described and inoculated on Vero cells (African green monkey kidney cells) obtained from ATCC (CCL-81) in a biosafety level 3 laboratory at the Pasteur Institute of Tunis (67). The inoculated cells were then maintained in Minimum Essential Medium (MEM) supplemented with 5% fetal bovine serum (FBS), incubated at 36°C and 5% CO2, and observed for cytopathic effect (CPE) for 7 days. In the absence of CPE, inoculated cells were harvested and clarified by centrifugation, and 200 μL of supernatant was inoculated onto a fresh cell culture monolayer and observed for an additional 7 days (53). Specimens were considered negative if no cytopathic effect was detected during 14 days after initial inoculation. For samples showing a cytopathic effect (CPE), the infected cells were then harvested and clarified by centrifugation. Virus suspensions were used for confirmation by SARS-CoV-2 real-time PCR.
3. Results
3.1. Positive SARS-CoV-2 RNA in nasopharyngeal swabs
All investigated cases presented an initial positive SARS-CoV-2 nasopharyngeal sample. Among them, 7 out of 71 (9.9%) children were asymptomatic, and 64 out of 71 (90.1%) were symptomatic.
They presented mild clinical signs, including fever (n = 46), cough (n = 43), headache (n = 32), loss of taste smell (n = 36), tiredness (n = 21), diarrhea (n = 32), vomiting (n = 16), muscle pain (n = 29), breathing difficulty (n = 4), conjunctivitis (n = 2), dyspnea (n = 3), urticaria (n = 2), and vertigo (n = 1). The duration of SARS-CoV-2 shedding in nasopharyngeal swabs varied between 7 and 21 days (average equal to 8.7 days). By days 7, 14, and 21, 42 (59%), 11 (15.5%), and 4 (5.6%) out of 71 children continued to shed SARS-CoV-2 RNA. All children stopped shedding SARS-CoV-2 RNA on day 28 (Figure 2A). The values of the threshold cycle (Ct values) varied from 13 to 34, 21 to 38, 22 to 37, and 28 to 35 on days 1, 7, 14, and 21, respectively. The median Ct values were 22, 27.3, 33.75, and 33.5 on days 1, 7, 14, and 21, respectively, as described in Table 2.
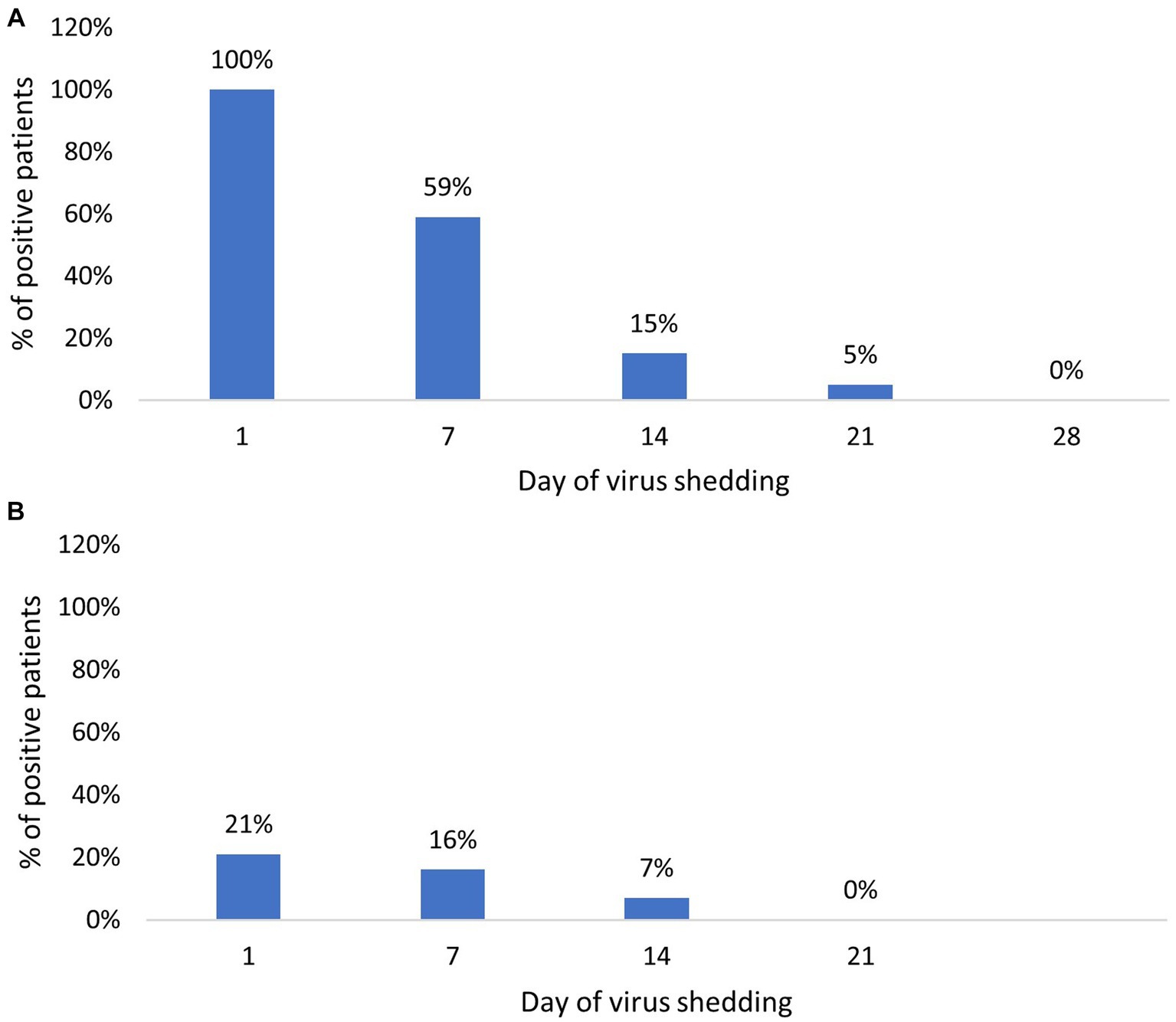
Figure 2. Percentage of SARS-CoV-2 positive patients over time: (A) Percentage of SARS-CoV-2 positive cases in nasopharyngeal samples; (B) Percentage of SARS-CoV-2 positive cases in stool samples.
3.2. Positive SARS-CoV-2 RNA in stool samples
Among the studied population, SARS-CoV-2 RNA was detected in stool samples of 15 out of 71 (21.1%) children. They are constituted by 13 out of 64 (20.3%) symptomatic and 2 out of 7 (28.6%) asymptomatic patients, with no statistically significant difference between the two groups (value of p > 0.05; x squared = 0.00042451, df = 1, value of p = 0.9836; Table 3).

Table 3. Prevalence of positive stool sample shedding cases among symptomatic and asymptomatic cases.
A total of 36 positive samples were detected, with a threshold cycle (Ct) varying between 23 and 37 (Table 2). For samples obtained from the symptomatic group, the Ct values were between 23 and 37, while samples obtained from the asymptomatic group presented Ct values varying between 24 and 32 (Table 3).
The RNA-shedding duration was between 7 and 14 days. On days 7 and 14, 12 and 5 of 71 (16% and 7%) children remained positive by RT-PCR, respectively. No one of them continued to shed the RNA in stool samples on day 21 (Figure 2B). In symptomatic children, the duration of SARS-CoV-2 RNA excretion in stool samples varied between 7 and 14 days (average equivalent to 9.4), while in asymptomatic cases, the duration of viral RNA excretion was 7 days (Table 2).
3.3. SARS-CoV-2 variant identification
With the aim of identifying variants of excreted viruses in stool samples, 14 full genome sequences were obtained from samples of 14 out of 15 children presenting fecal shedding (Table 2). For one child, sequences were not generated given the high real-time PCR Ct value. Four SARS-CoV-2 variants were detected: Alpha (B.1.1.7) (n = 3), B.1.160 (n = 3), Delta (AY.122 sub-variant) (n = 7), and Omicron (BA.1.1.1 sub-variant)(n = 1). The Delta variant was the most excreted variant (value of p < 0.05). The duration of excretion of positive fecal samples was variable among those variants (Figure 3). Patients infected with the Alpha and B.1.160 variants presented positive RNA results during 7 (n = 2) to 14 (n = 1) days of the infection, for each one. On the other hand, 7 children infected with the Delta variant (AY.122 sub-variant) presented positive results up to 14 days (n = 4) and 7 days (n = 3). For the Omicron variant (BA.1.1.1 sub-variant), the RNA shedding was limited to 7 days.
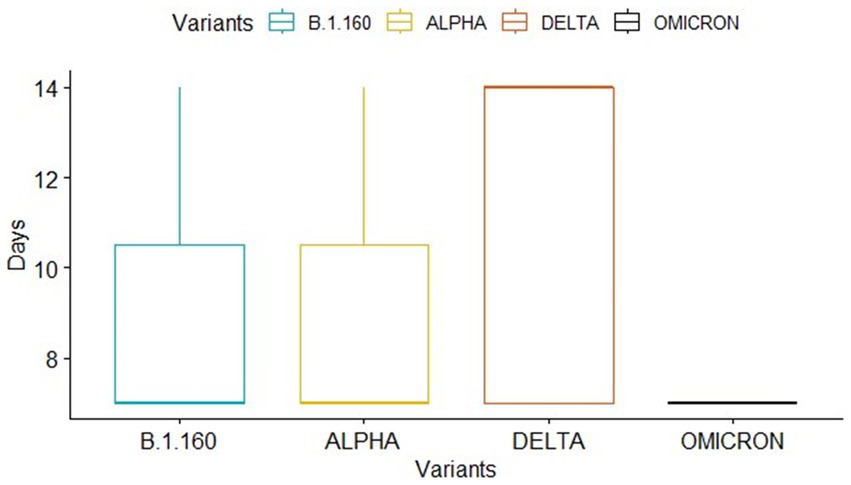
Figure 3. Presentation of SARS-CoV-2 RNA shedding duration for each variant using the boxplot package which describes data sets using 5 particular numbers: the minimum, first quartile, median, third quartile, and maximum. The box in the diagram begins with the first quartile and ends with the third quartile. Lines extend from the first quartile down to the minimum and from the third quartile up to the maximum.
3.4. Virus isolation
Virus isolation using Vero cells showed non-specific modification of the cell aspect in positive stool samples. The observed modifications appeared between 4 and 7 days, respectively. After passaging, slight modifications appeared after 5 days. Specific SARS-CoV-2 RT-qPCR detection was negative for all obtained cell culture supernatants.
4. Discussion
Since the beginning of the COVID-19 pandemic, the fecal–oral excretion of infectious SARS-CoV-2 has been a matter of debate. Transmission via the fecal–oral route was previously demonstrated for other coronaviruses, such as MERS-CoV and SARS-CoV-1 (3, 68). Thus, in this study, we investigated the SARS-CoV-2 RNA shedding in stool samples obtained from symptomatic and asymptomatic COVID-19 pediatric cases infected with different virus variants between February 2021 and January 2022, along the Alpha, B.1.160, Delta, and Omicron waves in Tunisia (58, 69). To support the hypothesis of possible fecal–oral transmission, the virus viability in positive samples was investigated by inoculation with cell culture.
A proportion of 21.1% of infected children showed SARS-CoV-2 RNA shedding. The shedding occurred similarly among symptomatic and asymptomatic children. Previous studies showed that children with fecal excretion of viral RNA may be asymptomatic or present with clinical respiratory or gastrointestinal signs (19, 41, 42). The rate of positive RNA in fecal specimens of COVID-19 patients was controversial among the different published studies. An overview of the gastrointestinal shedding of SARS-CoV-2 in infected children suggested an average of 20–30% of positive fecal shedding in infants with and without gastrointestinal signs (38). However, other studies, mainly achieved rapidly at the beginning of the pandemic, reported 83.3 to 91.4% of RNA shedding in stool samples from children (3, 42, 70, 71). Other authors reported rates between 47 and 69% (72, 73).
Moreover, several data points regarding the shedding duration in stool samples suggest prolonged periods of up to 70 days or more (3, 19, 42, 74). The prolonged shedding period was mainly related to severe cases requiring hospitalization or immunocompromised patients (39, 75). Many studies reporting asymptomatic and moderate cases showed a shedding period between 18 and 32 days through the digestive tract (3, 72). In our series of investigations, the shedding period was at least 21 days in nasopharyngeal samples and at least 14 days in stool samples. Indeed, they present mild clinical forms with moderate respiratory and gastrointestinal signs.
In our study, the excreted viral RNA belonged to four variants of SARS-CoV-2: Alpha (B.1.1.7), B.1.160, Delta (B.1.617.2), and Omicron (B.1.1.529). The Delta variant (sub-variant AY.122) appears to be the most excreted, during the longest period, and with the highest viral load. In the literature, limited information was available about the rate of SARS-CoV-2 RNA shedding by children and adults according to the variant. Available data suggested increasing SARS-CoV-2 infection in the pediatric population, especially during the Delta variant wave, with higher transmissibility and pathogenicity than other variants (4, 10−14). It was suggested that the Delta variant is 60% more transmissible than the Alpha variant (32, 76). Furthermore, disease duration in children infected with the Delta variant was reported to be longer, in some cases exceeding 29 days (7, 77, 78).
It is worth noting that the detection of SARS-CoV-2 RNA in the framework of wastewater-based genomic surveillance is of great interest for tracking SARS-CoV-2 variants and the early management of new waves of the infection. It can complement clinical surveillance efforts and also offer more details about the evolutionary dynamics of SARS-CoV-2 (79).
To investigate the hypothesis of possible fecal–oral transmission, SARS-CoV-2 RNA detection and virus isolation on cell culture, followed by molecular confirmation, were used. Indeed, RNA shedding in stool samples could not reflect systematically the possible fecal–oral transmission, as the detection of SARS-CoV-2 RNA might be the result of virus replication into the gastrointestinal tract (38). In our study, a total of 36 samples were inoculated, and none of them showed any specific CPE until 14 days. Moreover, SARS-CoV-2 RNA detection in the cell culture supernatant was also negative. In this regard, the data in the literature are conflicting. Many studies have demonstrated the absence of cytopathic effect (CPE) in Vero cells after inoculation with stool samples (44, 45), while others have suggested the presence of infectious particles by the use of cell culture and electron microscopy (EM) visualization (48, 49). Reasonably, isolation in cell culture and observation of virus particles in EM are not sufficient to confirm the presence of viable particles. The use of more specific methods, such as molecular detection of sub-genomic RNA, is therefore highly recommended for investigating virus viability in infected biological specimens (80).
In our setting, the presence of CPE or cell modifications generated with stool samples could be the result of the multiplication of other enteric viruses, especially on Vero cells, which are permissive for the majority of cultured viruses. In this case, the initial evidence of SARS-CoV-2 RNA from the stool sample reflects only the presence of SARS-CoV-2 genomic RNA.
Indeed, in our study, investigated stool samples were all showing high CT values, evidence that makes virus isolation unlikely.
Nevertheless, the different parameters used for cell culture isolation may impact the sensitivity of virus detection. Furthermore, it is worth mentioning that our findings are derived from a relatively small number of positive stool samples. Conducting an analysis on a larger dataset of fecal specimens, if accessible, would support our findings. It is worth highlighting that during the study, a significant number of children, particularly adolescents, declined to provide stool samples.
From another point of view, it will be very interesting to investigate the potential correlations between fecal shedding patterns and variables such as the type of vaccine administered to participants and the severity of COVID-19 disease.
5. Conclusion
In conclusion, the exploration of SARS-CoV-2 shedding in stool samples bears significant relevance as it could contribute to a wider spread of the virus and environmental contamination. Our study has revealed that children can shed SARS-CoV-2 RNA in their nasopharyngeal and fecal samples for up to 21 and 14 days, respectively, particularly when infected with the Delta variant. However, none of the positive samples exhibited the presence of viable SARS-CoV-2 particles. Consequently, the likelihood of SARS-CoV-2 transmission via the fecal–oral route appears to be low. Further investigation involving a larger and more diverse population can provide additional support for our findings.
Data availability statement
The datasets presented in this study can be found in online repositories. The names of the repository/repositories and accession number(s) can be found in the article/Supplementary material.
Ethics statement
The studies involving humans were approved by the local Medical Ethics Committee of Bechir Hamza Children’s Hospital of Tunis, Tunisia, under the reference “12/2021.” The studies were conducted in accordance with the local legislation and institutional requirements. Written informed consent for participation in this study was provided by the participants’ legal guardians/next of kin.
Author contributions
SH-B and HTr: conceptualization. HKh, MG, and SH-B: data curation. HKh, MG, SBH, HKr, HTo, AC, AS, ZM, NH, CC, MA, AP, and RA: investigation. HKh, MG, SBH, HKr, HTo, AC, AS, ZM, NH, CC, MA, AP, and RA: resources. HTr, SH-B, CC, AL, and AP: funding acquisition. HTr, SH-B, MA, and AP: supervision. HTr, AL, and SH-B: validation. HKh, HTr, AL, and SH-B: visualization, original draft preparation, and writing—review and editing. HKh and SH-B: writing. All authors reviewed the manuscript and agreed to its submission to this journal.
Funding
This study was co-funded by the Italian Ministry of Health (IZSAM05/20 Ricerca Corrente 2020 PanCO: epidemiologia e patogenesi dei coronavirus umani e animali) via the project MEDNET coordinated by the World Health Organization Tunisia office, the Pasteur Institute of Tunis, the Tunisian Ministry of Health, and the Tunisian Ministry of Higher Education and Research (LR20IPT02). This research was also partially supported by EU funding within the NextGenerationEU-MUR PNRR Extended Partnership initiative on Emerging Infectious Diseases (Project no. PE00000007, INF-ACT).
Acknowledgments
The authors gratefully acknowledge the Instituto Zooprofilattico Sperimentale dell’Abruzzo e del Molise G. Caporale (IZSAM), Teramo, Italy, for their contribution to whole-genome sequencing (WGS) and the data analysis. The authors also acknowledge Samia Rourou for providing Vero cell lines.
Conflict of interest
The authors declare that the research was conducted in the absence of any commercial or financial relationships that could be construed as a potential conflict of interest.
The reviewer SM declared a past co-authorship with the author SH-B to the handling editor.
Publisher’s note
All claims expressed in this article are solely those of the authors and do not necessarily represent those of their affiliated organizations, or those of the publisher, the editors and the reviewers. Any product that may be evaluated in this article, or claim that may be made by its manufacturer, is not guaranteed or endorsed by the publisher.
Supplementary material
The Supplementary material for this article can be found online at: https://www.frontiersin.org/articles/10.3389/fmed.2023.1226207/full#supplementary-material
Footnotes
References
1. Mannheim, J, Gretsch, S, Layden, JE, and Fricchione, MJ. Characteristics of hospitalized pediatric coronavirus disease 2019 cases in Chicago, Illinois, march-April 2020. J Pediatr Infect Dis Soc. (2020) 9:519–22. doi: 10.1093/jpids/piaa070
2. Tsabouri, S, Makis, A, Kosmeri, C, and Siomou, E. Risk factors for severity in children with coronavirus disease 2019: a comprehensive literature review. Pediatr Clin N Am. (2021) 68:321–38. doi: 10.1016/j.pcl.2020.07.014
3. Xu, CLH, Raval, M, Schnall, JA, Kwong, JC, and Holmes, NE. Duration of respiratory and gastrointestinal viral shedding in children with SARS-CoV-2: a systematic review and synthesis of data. Pediatr Infect Dis J. (2020) 39:e249–56. doi: 10.1097/INF.0000000000002814
4. Khemiri, H, Ayouni, K, Triki, H, and Haddad-Boubaker, S. SARS-CoV-2 infection in pediatric population before and during the Delta (B.1.617.2) and Omicron (B.1.1.529) variants era. Virol J. (2022) 19:144. doi: 10.1186/s12985-022-01873-4
5. World Health Organization, COVID-19 variants. (2023). Available at: https://www.who.int/westernpacific/emergencies/covid-19/information/covid-19-variants, Accessed 28 june 2022
6. Dubey, A, Choudhary, S, Kumar, P, and Tomar, S. Emerging SARS-CoV-2 variants: genetic variability and clinical implications. Curr Microbiol. (2021) 79:20. doi: 10.1007/s00284-021-02724-1
7. Bard, JD, Bootwalla, M, Leber, A, Planet, P, Moustafa, AM, Harris, R, et al. Emergence of SARS-CoV-2 variants of concern in the pediatric population of the United States. bioRxiv. (2021). doi: 10.1101/2021.05.22.21257660
8. Mascola, JR, Graham, BS, and Fauci, AS. SARS-CoV-2 viral variants—tackling a moving target. JAMA. (2021) 325:1261–2. doi: 10.1001/jama.2021.2088
9. Kunal, S, Ish, P, and Aditi, GK. Emergence of COVID-19 variants and its global impact In: S Adibi, P Griffin, M Sanicas, M Rashidi, and F Lanfranchi, editors. Frontiers of COVID-19: Scientific and clinical aspects of the novel coronavirus 2019 [internet]. Cham: Springer International Publishing (2022). 183–201.
10. Torjesen, I. Covid-19: Delta variant is now UK’s most dominant strain and spreading through schools. BMJ. (2021) 373:n1445. doi: 10.1136/bmj.n1445
11. Molteni, E, Sudre, CH, Canas, LDS, Bhopal, SS, Hughes, RC, Chen, L, et al. Illness characteristics of COVID-19 in children infected with the SARS-CoV-2 Delta variant. Children. (2022) 9:652. doi: 10.3390/children9050652
12. Bittmann, S. Role of Omicron variant of SARS-CoV-2 in children in Germany. World J Pediatr WJP. (2022) 18:283–4. doi: 10.1007/s12519-021-00511-3
13. Torjesen, I. Covid-19: Omicron variant is linked to steep rise in hospital admissions of very young children. BMJ. (2022) 376:o110. doi: 10.1136/bmj.o110
14. Alteri, C, Scutari, R, Costabile, V, Colagrossi, L, Yu la Rosa, K, Agolini, E, et al. Epidemiological characterization of SARS-CoV-2 variants in children over the four COVID-19 waves and correlation with clinical presentation. Sci Rep. (2022) 12:10194. doi: 10.1038/s41598-022-14426-0
15. Lotfi, M, and Rezaei, N. SARS-CoV-2: a comprehensive review from pathogenicity of the virus to clinical consequences. J Med Virol. (2020) 92:1864–74. doi: 10.1002/jmv.26123
16. Hindson, J. COVID-19: faecal–oral transmission? Nat Rev Gastroenterol Hepatol. (2020) 17:259. doi: 10.1038/s41575-020-0295-7
17. Rathore, JS, and Ghosh, C. Severe acute respiratory syndrome coronavirus-2 (SARS-CoV-2), a newly emerged pathogen: an overview. Pathog Dis. (2020) 78:ftaa042. doi: 10.1093/femspd/ftaa042
18. Huang, C, Wang, Y, Li, X, Ren, L, Zhao, J, Hu, Y, et al. Clinical features of patients infected with 2019 novel coronavirus in Wuhan. China Lancet Lond Engl. (2020) 395:497–506. doi: 10.1016/S0140-6736(20)30183-5
19. Zhang, T, Cui, X, Zhao, X, Wang, J, Zheng, J, Zheng, G, et al. Detectable SARS-CoV-2 viral RNA in feces of three children during recovery period of COVID-19 pneumonia. J Med Virol. (2020) 92:909–14. doi: 10.1002/jmv.25795
20. Callaway, E. Delta coronavirus variant: scientists brace for impact. Nature. (2021) 595:17–8. doi: 10.1038/d41586-021-01696-3
21. Delahoy, MJ, Ujamaa, D, Whitaker, M, O’Halloran, A, Anglin, O, Burns, E, et al. Hospitalizations associated with COVID-19 among children and adolescents - COVID-NET, 14 states, march 1, 2020-august 14, 2021. MMWR Morb Mortal Wkly Rep. (2021) 70:1255–60. doi: 10.15585/mmwr.mm7036e2
22. Wang, L, Berger, NA, Kaelber, DC, Davis, PB, Volkow, ND, and Xu, R. COVID infection severity in children under 5 years old before and after Omicron emergence in the US. MedRxiv Prepr Serv Health Sci. (2022):2022.01.12.22269179. doi: 10.1101/2022.01.12.22269179
23. Yeo, C, Kaushal, S, and Yeo, D. Enteric involvement of coronaviruses: is faecal-oral transmission of SARS-CoV-2 possible? Lancet Gastroenterol Hepatol. (2020) 5:335–7. doi: 10.1016/S2468-1253(20)30048-0
24. Ong, J, Young, BE, and Ong, S. COVID-19 in gastroenterology: a clinical perspective. Gut. (2020) 69:1144–5. doi: 10.1136/gutjnl-2020-321051
25. Ng, SC, and Tilg, H. COVID-19 and the gastrointestinal tract: more than meets the eye. Gut. (2020) 69:973–4. doi: 10.1136/gutjnl-2020-321195
26. Jin, X, Lian, JS, Hu, JH, Gao, J, Zheng, L, Zhang, YM, et al. Epidemiological, clinical and virological characteristics of 74 cases of coronavirus-infected disease 2019 (COVID-19) with gastrointestinal symptoms. Gut. (2020) 69:1002–9. doi: 10.1136/gutjnl-2020-320926
27. Lu, X, Zhang, L, Du, H, Zhang, J, Li, YY, Qu, J, et al. SARS-CoV-2 infection in children. N Engl J Med. (2020) 382:1663–5. doi: 10.1056/NEJMc2005073
28. Lin, L, Jiang, X, Zhang, Z, Huang, S, Zhang, Z, Fang, Z, et al. Gastrointestinal symptoms of 95 cases with SARS-CoV-2 infection. Gut. (2020) 69:997–1001. doi: 10.1136/gutjnl-2020-321013
29. Young, BE, Ong, SWX, Kalimuddin, S, Low, JG, Tan, SY, Loh, J, et al. Epidemiologic features and clinical course of patients infected with SARS-CoV-2 in Singapore. JAMA. (2020) 323:1488–94. doi: 10.1001/jama.2020.3204
30. Fang, D, Ma, J, Guan, J, Wang, M, Song, Y, Tian, D, et al. Manifestations of digestive system in hospitalized patients with novel coronavirus pneumonia in Wuhan, China: a single-center, descriptive study. Chin J Dig. (2020):E005–5.
31. Gu, J, Han, B, and Wang, J. COVID-19: gastrointestinal manifestations and potential fecal-Oral transmission. Gastroenterology. (2020) 158:1518–9. doi: 10.1053/j.gastro.2020.02.054
32. Cheung, KS, Hung, IFN, Chan, PPY, Lung, KC, Tso, E, Liu, R, et al. Gastrointestinal manifestations of SARS-CoV-2 infection and virus load in fecal samples from a Hong Kong cohort: systematic review and Meta-analysis. Gastroenterology. (2020) 159:81–95. doi: 10.1053/j.gastro.2020.03.065
33. Matthai, J, Shanmugam, N, and Sobhan, P. Indian Society of Pediatric Gastroenterology Hepatology and Nutrition, pediatric gastroenterology chapter of Indian academy of pediatrics. Coronavirus disease (COVID-19) and the gastrointestinal system in children. Indian Pediatr. (2020) 57:533–5. doi: 10.1007/s13312-020-1851-5
34. Hou, YJ, Okuda, K, Edwards, CE, Martinez, DR, Asakura, T, Dinnon, KH, et al. SARS-CoV-2 reverse genetics reveals a variable infection gradient in the respiratory tract. Cells. (2020) 182:429–446.e14. doi: 10.1016/j.cell.2020.05.042
35. Puray-Chavez, M, LaPak, KM, Schrank, TP, Elliott, JL, Bhatt, DP, Agajanian, MJ, et al. Systematic analysis of SARS-CoV-2 infection of an ACE2-negative human airway cell. Cell Rep. (2021) 36:109364. doi: 10.1016/j.celrep.2021.109364
36. Park, SK, Lee, CW, Park, DI, Woo, HY, Cheong, HS, Shin, HC, et al. Detection of SARS-CoV-2 in fecal samples from patients with asymptomatic and mild COVID-19 in Korea. Clin Gastroenterol Hepatol. (2021) 19:1387–1394.e2. doi: 10.1016/j.cgh.2020.06.005
37. Tian, Y, Rong, L, Nian, W, and He, Y. Review article: gastrointestinal features in COVID-19 and the possibility of faecal transmission. Aliment Pharmacol Ther. (2020) 51:843–51. doi: 10.1111/apt.15731
38. Chiappini, E, Licari, A, Motisi, MA, Manti, S, Marseglia, GL, Galli, L, et al. Gastrointestinal involvement in children with SARS-COV-2 infection: an overview for the pediatrician. Pediatr Allergy Immunol. (2020) 31:92–5. doi: 10.1111/pai.13373
39. Santos, VS, Gurgel, RQ, Cuevas, LE, and Martins-Filho, PR. Prolonged fecal shedding of SARS-CoV-2 in pediatric patients: a quantitative evidence synthesis. J Pediatr Gastroenterol Nutr. (2020) 71:150–2. doi: 10.1097/MPG.0000000000002798
40. Wang, J, Cui, HR, Tang, HB, and Deng, XL. Gastrointestinal symptoms and fecal nucleic acid testing of children with 2019 coronavirus disease: a systematic review and meta-analysis. Sci Rep. (2020) 10:17846. doi: 10.1038/s41598-020-74913-0
41. Xu, Y, Li, X, Zhu, B, Liang, H, Fang, C, Gong, Y, et al. Characteristics of pediatric SARS-CoV-2 infection and potential evidence for persistent fecal viral shedding. Nat Med. (2020) 26:502–5. doi: 10.1038/s41591-020-0817-4
42. Hua, CZ, Miao, ZP, Zheng, JS, Huang, Q, Sun, QF, Lu, HP, et al. Epidemiological features and viral shedding in children with SARS-CoV-2 infection. J Med Virol. (2020) 92:2804–12. doi: 10.1002/jmv.26180
43. Puhach, O, Adea, K, Hulo, N, Sattonnet, P, Genecand, C, Iten, A, et al. Infectious viral load in unvaccinated and vaccinated individuals infected with ancestral, Delta or Omicron SARS-CoV-2. Nat Med. (2022) 28:1491–500. doi: 10.1038/s41591-022-01816-0
44. Jeong, HW, Kim, SM, Kim, HS, Kim, YI, Kim, JH, Cho, JY, et al. Viable SARS-CoV-2 in various specimens from COVID-19 patients. Clin Microbiol Infect. (2020) 26:1520–4. doi: 10.1016/j.cmi.2020.07.020
45. Kim, JM, Kim, HM, Lee, EJ, Jo, HJ, Yoon, Y, Lee, NJ, et al. Detection and isolation of SARS-CoV-2 in serum, urine, and stool specimens of COVID-19 patients from the Republic of Korea. Osong Public Health Res Perspect. (2020) 11:112–7. doi: 10.24171/j.phrp.2020.11.3.02
46. Xiao, F, Sun, J, Xu, Y, Li, F, Huang, X, Li, H, et al. Infectious SARS-CoV-2 in feces of patient with severe COVID-19. Emerg Infect Dis. (2020) 26:1920–2. doi: 10.3201/eid2608.200681
47. Fumian, TM, Malta, FC, Dos Santos, DRL, Pauvolid-Corrêa, A, Fialho, AM, Leite, JPG, et al. SARS-CoV-2 RNA detection in stool samples from acute gastroenteritis cases. Brazil J Med Virol. (2021) 93:2543–7. doi: 10.1002/jmv.26786
48. Zhang, Y, Chen, C, Song, Y, Zhu, S, Wang, D, Zhang, H, et al. Excretion of SARS-CoV-2 through faecal specimens. Emerg Microbes Infect. (2020) 9:2501–8. doi: 10.1080/22221751.2020.1844551
49. Wang, W, Xu, Y, Gao, R, Lu, R, Han, K, Wu, G, et al. Detection of SARS-CoV-2 in different types of clinical specimens. JAMA. (2020) 323:1843–4. doi: 10.1001/jama.2020.3786
50. World health organization. (2021). WHO-2019-nCoV-clinical-2021.2-eng clinical manifestation.Pdf. Accessed 8 May 2023
51. Haddad-Boubaker, S, Joffret, ML, Pérot, P, Bessaud, M, Meddeb, Z, Touzi, H, et al. Metagenomic analysis identifies human adenovirus 31 in children with acute flaccid paralysis in Tunisia. Arch Virol. (2019) 164:747–55. doi: 10.1007/s00705-018-04141-5
52. World health Organization. Manuel for virological investigation of poliomyelitis. Geneva: World Health Organization (2004).
53. Haddad-Boubaker, S, Yahia, AB, Rezig, D, Farès, W, Touzi, H, and Triki, H. Enterovirus detection in stool specimen: relevance for poliovirus and enterovirus surveillance. Arch Inst Pasteur Tunis. (2007) 84:3–9.
54. Chu, DKW, Pan, Y, Cheng, SMS, Hui, KPY, Krishnan, P, Liu, Y, et al. Molecular diagnosis of a novel coronavirus (2019-nCoV) causing an outbreak of pneumonia. Clin Chem. (2020) 66:549–55. doi: 10.1093/clinchem/hvaa029
55. World health Organization. (2020). Available at: https://www.who.int/docs/default-source/coronaviruse/peiris-protocol-16-1-20.pdf. Accessed 8 May 2023
56. World health Organization. (2020). Available at: https://www.who.int/docs/default-source/coronaviruse/real-time-rt-pcr-assays-for-the-detection-of-sars-cov-2-institut-pasteur-paris.pdf Accessed 8 May 2023
57. Gdoura, M, Abouda, I, Mrad, M, Ben Dhifallah, I, Belaiba, Z, Fares, W, et al. SARS-CoV2 RT-PCR assays: in vitro comparison of 4 WHO approved protocols on clinical specimens and its implications for real laboratory practice through variant emergence. Virol J. (2022) 19:54. doi: 10.1186/s12985-022-01784-4
58. Haddad-Boubaker, S, Arbi, M, Souiai, O, Chouikha, A, Fares, W, Edington, K, et al. The Delta variant wave in Tunisia: genetic diversity, spatio-temporal distribution and evidence of the spread of a divergent AY.122 sub-lineage. Front Public Health. (2023) 10:990832. doi: 10.3389/fpubh.2022.990832
59. Molini, U, Coetzee, LM, Engelbrecht, T, de Villiers, L, de Villiers, M, Mangone, I, et al. SARS-CoV-2 in Namibian dogs. Vaccine. (2022) 10:2134. doi: 10.3390/vaccines10122134
60. Di Pasquale, A, Radomski, N, Mangone, I, Calistri, P, Lorusso, A, and Cammà, C. SARS-CoV-2 surveillance in Italy through phylogenomic inferences based on hamming distances derived from pan-SNPs, -MNPs and -InDels. BMC Genomics. (2021) 22:782. doi: 10.1186/s12864-021-08112-0
61. Li, H. Aligning sequence reads, clone sequences and assembly contigs with BWA-MEM. (2014), arXiv, doi: 10.48550/arXiv.1303.3997
62. Bolger, AM, Lohse, M, and Usadel, B. Trimmomatic: a flexible trimmer for Illumina sequence data. Bioinforma Oxf Engl. (2014) 30:2114–20. doi: 10.1093/bioinformatics/btu170
63. Grubaugh, ND, Gangavarapu, K, Quick, J, Matteson, NL, Jesus, JGD, Main, BJ, et al. An amplicon-based sequencing framework for accurately measuring intrahost virus diversity using PrimalSeq and iVar. bioRxiv. (2018):383513. Available at: https://www.biorxiv.org/content/10.1101/383513v1
64. O’Toole, Á, Scher, E, Underwood, A, Jackson, B, Hill, V, McCrone, JT, et al. Assignment of epidemiological lineages in an emerging pandemic using the pangolin tool. Virus Evol. (2021) 7:veab064. doi: 10.1093/ve/veab064
65. Hadfield, J, Megill, C, Bell, SM, Huddleston, J, Potter, B, Callender, C, et al. Nextstrain: real-time tracking of pathogen evolution. Bioinformatics. (2018) 34:4121–3. doi: 10.1093/bioinformatics/bty407
66. Frigge, M, Hoaglin, DC, and Iglewicz, B. Some implementations of the boxplot. Am Stat. (2012) 43:50–4. doi: 10.1080/00031305.1989.10475612
67. The R foundation. R: What is R? (2002). Available at: https://www.r-project.org/about.html
68. Valleriani, F, Mancuso, E, Vincifori, G, Teodori, L, Di Marcantonio, L, Spedicato, M, et al. Neutralization of SARS-CoV-2 variants by serum from BNT162b2 vaccine recipients. Viruses. (2021) 13:2011. doi: 10.3390/v13102011
69. Chouikha, A, Fares, W, Laamari, A, Haddad-Boubaker, S, Belaiba, Z, Ghedira, K, et al. Molecular epidemiology of SARS-CoV-2 in Tunisia (North Africa) through several successive waves of COVID-19. Viruses. (2022) 14:624. doi: 10.3390/v14030624
70. Cai, J, Wang, X, Zhao, J, Ge, Y, Xu, J, Tian, H, et al. Comparison of clinical and epidemiological characteristics of asymptomatic and symptomatic SARS-CoV-2 infection in children. Virol Sin. (2020) 35:803–10. doi: 10.1007/s12250-020-00312-4
71. Jiehao, C, Jin, X, Daojiong, L, Zhi, Y, Lei, X, Zhenghai, Q, et al. A case series of children with 2019 novel coronavirus infection: clinical and epidemiological features. Clin Infect Dis. (2020) 71:1547–51. doi: 10.1093/cid/ciaa198
72. Tam, I, McNamara, C, Dunbar, J, O’Connor, K, Manzano, G, Cabana, MD, et al. SARS-CoV-2 gastrointestinal shedding in hospitalized children. Hosp Pediatr. (2022) 12:e78–85. doi: 10.1542/hpeds.2021-006304
73. Benvari, S, Mahmoudi, S, and Mohammadi, M. Gastrointestinal viral shedding in children with SARS-CoV-2: a systematic review and meta-analysis. World J Pediatr. (2022) 18:582–8. doi: 10.1007/s12519-022-00553-1
74. Holm-Jacobsen, JN, Vonasek, JH, Hagstrøm, S, Donneborg, ML, and Sørensen, S. Prolonged rectal shedding of SARS-CoV-2 in a 22-day-old-neonate: a case report. BMC Pediatr. (2021) 21:506. doi: 10.1186/s12887-021-02976-7
75. Dergham, J, Delerce, J, Bedotto, M, La Scola, B, and Moal, V. Isolation of viable SARS-CoV-2 virus from feces of an immunocompromised patient suggesting a possible fecal mode of transmission. J Clin Med. (2021) 10:2696. doi: 10.3390/jcm10122696
76. Tian, D, Sun, Y, Xu, H, and Ye, Q. The emergence and epidemic characteristics of the highly mutated SARS-CoV-2 Omicron variant. J Med Virol. (2022) 94:2376–83. doi: 10.1002/jmv.27643
77. Kim, KW, Wang, X, Adhikari, A, Yeang, M, Jenkins, F, Naing, Z, et al. Persistent high-level shedding of cultivable SARS-CoV-2 Delta virus 33 days after onset of COVID-19 in a hospitalized patient with pneumonia. J Med Virol. (2022) 94:4043–6. doi: 10.1002/jmv.27832
78. Sun, W, Yang, N, Mao, Y, Yan, D, Song, Q, and Qian, G. Duration of viral shedding of the Omicron variant in asymptomatic and mild COVID-19 cases from Shanghai, China. medRxiv. (2022):2022.12.08.22283272. doi: 10.1101/2022.12.08.22283272v1
79. Pilapil, JD, Notarte, KI, and Yeung, KL. The dominance of co-circulating SARS-CoV-2 variants in wastewater. Int J Hyg Environ Health. (2023) 253:114224. doi: 10.1016/j.ijheh.2023.114224
80. Kim, JY, Bae, JY, Bae, S, Cha, HH, Kwon, JS, Suh, MH, et al. Diagnostic usefulness of subgenomic RNA detection of viable SARS-CoV-2 in patients with COVID-19. Clin Microbiol Infect. (2022) 28:101–6. doi: 10.1016/j.cmi.2021.08.009
Glossary
Keywords: stool, pediatric population, infectious, variants, symptomatic, asymptomatic, SARS-CoV-2
Citation: Khemiri H, Gdoura M, Ben Halima S, Krichen H, Cammà C, Lorusso A, Ancora M, Di Pasquale A, Cherni A, Touzi H, Sadraoui A, Meddeb Z, Hogga N, Ammi R, Triki H and Haddad-Boubaker S (2023) SARS-CoV-2 excretion kinetics in nasopharyngeal and stool samples from the pediatric population. Front. Med. 10:1226207. doi: 10.3389/fmed.2023.1226207
Edited by:
Jawhar Gharbi, King Faisal University, Saudi ArabiaReviewed by:
Manel Ben M'Hadheb, University of Monastir, TunisiaSalma Mhalla, University of Monastir, Tunisia
Kin Israel Notarte, Johns Hopkins University, United States
Copyright © 2023 Khemiri, Gdoura, Ben Halima, Krichen, Cammà, Lorusso, Ancora, Di Pasquale, Cherni, Touzi, Sadraoui, Meddeb, Hogga, Ammi, Triki and Haddad-Boubaker. This is an open-access article distributed under the terms of the Creative Commons Attribution License (CC BY). The use, distribution or reproduction in other forums is permitted, provided the original author(s) and the copyright owner(s) are credited and that the original publication in this journal is cited, in accordance with accepted academic practice. No use, distribution or reproduction is permitted which does not comply with these terms.
*Correspondence: Sondes Haddad-Boubaker, c29uZGVzaGFkZGFkYm91YmFrZXJAZ21haWwuY29t; c29uZGVzLmhhZGRhZGJvdWJha2VyQHBhc3RldXIudG4=; Haifa Khemiri, aGFpZmEua2hlbWlyaUBwYXN0ZXVyLnV0bS50bg==; a2hlbWlyaWhhaWZhMTNAZ21haWwuY29t