- 1Department of Microbiology, Faculty of Medicine, Institute of Medical Sciences, Banaras Hindu University, Varanasi, India
- 2Department of General Surgery, Faculty of Medicine, Institute of Medical Sciences, Banaras Hindu University, Varanasi, India
The recent approval of experimental phage therapies by the FDA and other regulatory bodies with expanded access in cases in the United States and other nations caught the attention of the media and the general public, generating enthusiasm for phage therapy. It started to alter the situation so that more medical professionals are willing to use phage therapies with conventional antibiotics. However, more study is required to fully comprehend phage therapy’s potential advantages and restrictions, which is still a relatively new field in medicine. It shows promise, nevertheless, as a secure and prosperous substitute for antibiotics when treating bacterial illnesses in animals and humans. Because of their uniqueness, phage disinfection is excellent for ready-to-eat (RTE) foods like milk, vegetables, and meat products. The traditional farm-to-fork method can be used throughout the food chain to employ bacteriophages to prevent food infections at all production stages. Phage therapy improves clinical outcomes in animal models and lowers bacterial burdens in numerous preclinical investigations. The potential of phage resistance and the need to make sure that enough phages are delivered to the infection site are obstacles to employing phages in vivo. However, according to preclinical studies, phages appear to be a promising alternative to antibiotics for treating bacterial infections in vivo. Phage therapy used with compassion (a profound understanding of and empathy for another’s suffering) has recently grown with many case reports of supposedly treated patients and clinical trials. This review summarizes the knowledge on the uses of phages in various fields, such as the food industry, preclinical research, and clinical settings. It also includes a list of FDA-approved bacteriophage-based products, commercial phage products, and a global list of companies that use phages for therapeutic purposes.
Introduction
If the crisis of antimicrobial resistance is not addressed, it is anticipated that by 2050, the societal and financial costs will total US$100 trillion, resulting in 70,000 annual deaths (1). This number, although controversial, still emphasizes the severe problem we face regarding therapeutic alternatives for multidrug-resistant (MDR) bacterial infections (2, 3). Significant health risks are posed by pathogens, including methicillin-resistant Staphylococcus aureus (MRSA) and multi-resistant Pseudomonas aeruginosa (MDR-PA). The World Health Organization (WHO) recently published a list of MDR-priority pathogens and called for more research into antimicrobial resistance (4). Equally concerning is the requirement for rapid development of new antibiotics to replace older ones that are losing their efficacy. As a result, many researchers and clinicians are looking at bacteriophage as the most potential substitute for or adjunct to antibiotics to treat bacterial infections in the face of rising antibacterial resistance. Bacteriophages are viruses that uniquely and specifically target and eliminate bacteria. They work cooperatively in microbiological ecosystems in the human body and the environment and do not harm mammalian cells. As natural biological regulators, bacteriophages integrate into the One Health Strategy for animals, humans, and the environment (5). The first phages to be identified were phages against Escherichia coli, Shigella dysenteriae, and Vibrio cholerae (6, 7). Although there are several reports on phage treatment, they are uncontrolled or anecdotal and must adhere to the standards of contemporary evidence-based medicine (8). After the discovery of antibiotics, phages ceased being used as antibacterial agents in Western countries. Given our present in-depth understanding of the biology of bacteriophages, which is crucial in supporting developments in molecular biology, the odds of success the second time around are significantly greater. However, evidence from clinical and animal model studies strongly suggests that phage treatment is secure and may be advantageous (9–11).
This review offers recommendations for clinicians considering experimental phage treatment based on a thorough literature evaluation due to knowledge gaps. The study also intends to give an evidence-based assessment of the situations in which this experimental therapy may be considered and to acquaint clinicians with phage therapy’s preclinical and clinical usage.
Bacteriophages vs antibiotics
Enzybiotics
According to Veiga-Crespo et al. (12), the term “enzybiotics” refers to phages, viruses that target and lyse bacteria and may potentially aid in the treatment of bacterial infections. These can be phage-encoded lytic enzymes, such as lysins, and extracellular polymeric substance (EPS) depolymerase. Even though many EPS depolymerases and certain lysins are connected to virion particles (13), most lysins are endolysins, which are “from-within cell-wall degrading enzymes.” Enzybiotics, however, are added from outside after purification. Gram-positive bacteria’s peptidoglycan is not shielded by an outer membrane, making it susceptible to phage lysins. In order to cross the outer membrane barrier in Gram-negative bacteria, phage lysins often must be altered. Fusion proteins that combine natural lysin with an antibacterial peptide might accomplish this. According to the study by Yang et al. (14), one of these constructs, PlyA, showed good efficacy against A. baumannii and P. aeruginosa and growing cultures but not against cells in the stationary phase unless combined with agents that permeabilize the outer membrane. Due to a lack of awareness and comprehension, engineered bacteriophages could pose a problem in terms of public acceptance and regulatory approvals of engineered bacteriophages could delay their usage (15). In contrast to antibiotics, phages have genomes and can proliferate while parasitizing their host; hence, engineering them could cause additional problems and necessitate the addition of legal clauses addressing the social issues associated with genetic modifications. Additionally, when lysins are used via intravenous administration, neutralizing antibodies are produced, reducing their antibacterial efficacy after multiple administrations (16). However, few endolysin immunogenicity studies have shown that immune serum does slow but is not sufficient to block the antibacterial ability of endolysin (17–19). Since they have a short plasma half-life, are immunogenic and potentially toxic, cause an inflammatory reaction to bacterial debris, and are ineffective in lysing intracellular bacteria, their use as an antibacterial agent in human treatment raises concerns (20).
Delivery system for phage and Endolysin
Despite therapeutic potential of phages and endolysins, these alternative agents must overcome various practical challenges posed by the host system, such as limited bioavailability, loss of action, non-targeted delivery, rapid clearance by the reticuloendothelial system, and antibody-mediated inactivation (21).
Numerous methods for encapsulating phage and endolysin have been discovered recently (21). These delivery methods treat acute and chronic infections in animal models by altering host immunological response to therapeutic entities and increasing pharmacokinetic parameters (22). Many phage encapsulation studies have investigated the possibilities of different drug delivery systems, primarily natural polymers, synthetic polymers, liposomes, and electrospun fibers. GIT infections have been thoroughly researched as a potential polymeric phage encapsulation therapy target. These polymers shield phages from harsh acidic environments that may otherwise result in phage inactivation or loss of phage titer. In addition to safeguarding phages from harsh environments, these polymeric encapsulation materials also give permeability to the mucosal membrane, where bacterium pathogens may live, and get protected from digestive enzymes and bile fluids (23). According to Gondil et al., phage delivery systems are very effective when an infection is still in its early stages and can be effectively eliminated with just one dose of a phage formulation (24). However, late administration of phage formulation necessitates concomitant antibiotic administration or multiple doses of phage formulation to prevent the spread of infection. Different delivery systems of bacteriophage and endolysin are mentioned in Table 1.
Bacteriophage and its applications
Bacteriophages have been used and reported worldwide in various valuable applications. Figure 1: graphically represents the use of phage in clinical, preclinical, agriculture, fishery, and food industries. In addition, the therapeutic efficacy of the phage has been proven using preclinical and clinical settings. Based on these results, many pharmaceutical companies are still running clinical trials using bacteriophage products. However, the FDA has approved various phage products competing with the existing antimicrobials.
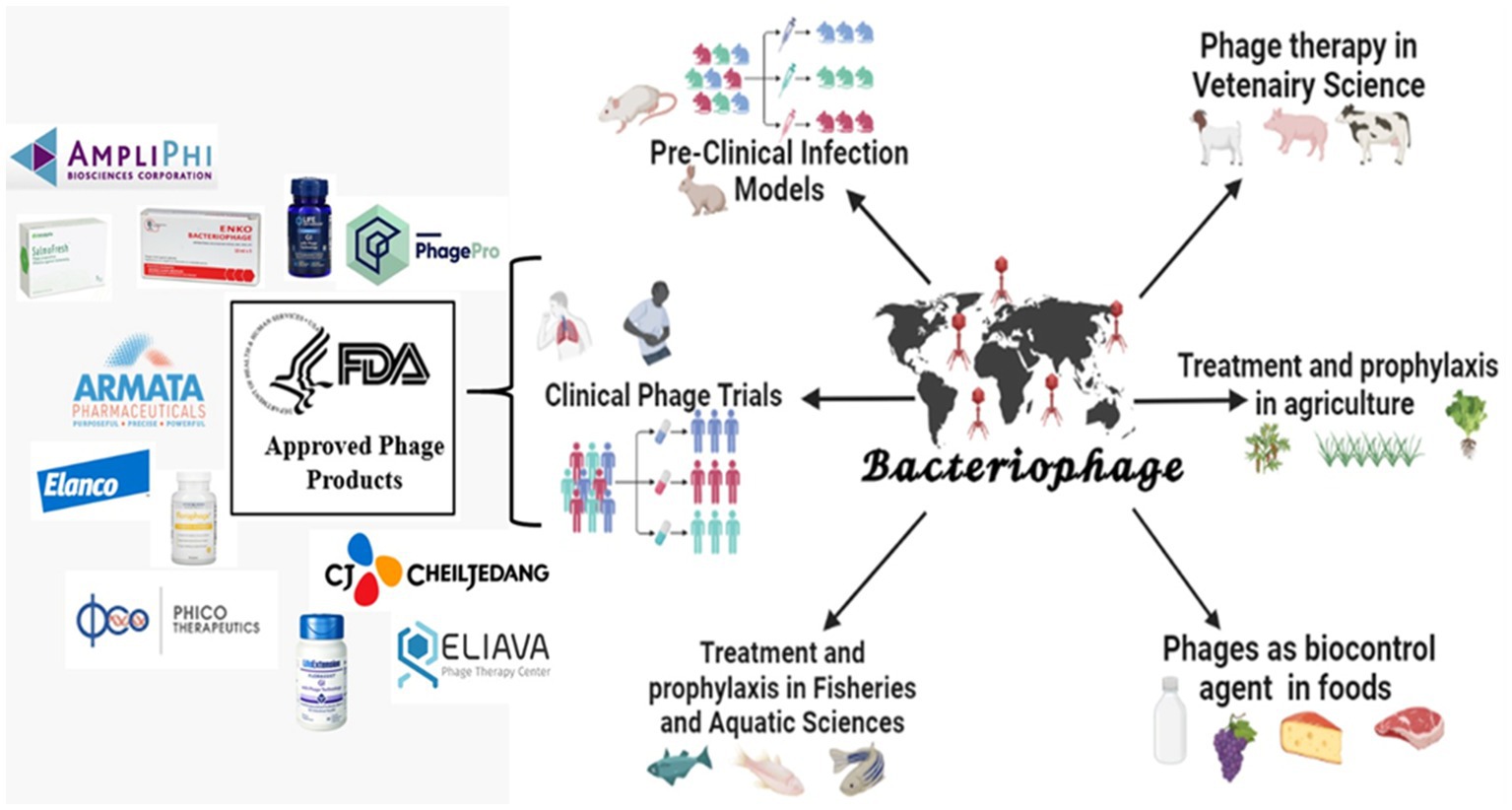
Figure 1. Applications of bacteriophages and its FDA-approved products (created by the help of bio-render tool).
Bacteriophages in preclinical studies
Recent years have seen a resurgence in interest in phage treatment, which uses bacteriophages to treat bacterial illnesses. This is because antibiotic-resistant bacteria are becoming more common. However, before going on to clinical trials in people, preclinical phage application involves evaluating the tolerance and efficacy of phage therapy in animal models.
Preclinical trials of phage therapy in animals typically involve infecting the animals with a specific bacterial pathogen and then administering phages to see if they can reduce bacterial loads and improve clinical outcomes. In addition, the animals are monitored for signs of toxicity or adverse reactions to the phages. Phage therapy improves clinical outcomes in animal models and lowers bacterial burdens in numerous preclinical studies. Nevertheless, there are challenges to using phages in vivo, such as the risk of phage resistance and the requirement to ensure that enough phages are delivered to the infection site.
Pharmacokinetics and pharmacodynamics of bacteriophages
Lytic phages, unlike conventional antibiotics, are unique biological agents capable of replicating within susceptible bacteria. They present complex pharmacokinetic (PK) profiles that encompass various facets, including absorption, distribution, metabolism, and elimination. The bedrock of phage dosage determination lies in the quantity of administered phage particles. Often, the choice of assays for quantifying phages in dosing solutions and biological samples, like blood and urine, is overlooked (35).
The primary method for quantifying phages involves counting visible plaques on agar plates containing susceptible bacteria. However, this approach may not encompass the entire phage population, as it only considers those capable of causing extensive lysis and resulting in visible plaques. Additionally, the concept of “efficiency of plating,” expressed as the ratio of plaque-forming units (pfu) of phages on the target bacterial strain relative to a reference strain, is integral to phage quantification. Using a bacterial host strain with lower plating efficiency can lead to underestimations, necessitating adjustments for an accurate count of infective phages against the target strain.
In the clinical arena, quantitative PCR traditionally monitors the kinetics of viral load for human viruses like HIV, CMV, Hepatitis B & C, and SARS-Cov-2, aiding in disease assessment and evaluating the efficacy of antiviral therapies. Recently, quantitative PCR-based assays have been applied to monitor phage PK in patients with severe bacterial infections undergoing intravenous adjunctive phage therapy. However, these assays face limitations in distinguishing infective phages from non-infective ones or phage DNA/RNA fragments. Therefore, further research is imperative to establish correlations between PCR-based assays and therapeutic outcomes. In conclusion, the pressing need for sensitive and validated methods of quantifying phages remains a paramount concern in the field of phage PK/PD research.
Phage therapy, unlike antibiotics relying on the widely accepted minimum inhibitory concentration (MIC) for evaluating pharmacodynamics (PD), grapples with standardizing antibacterial activity assessment. Traditional approaches encompass agar-based spot tests and efficiency of plating assays, while fluid environments like broth employ planktonic killing assays to assess phage effectiveness. These different testing methods yield divergent results in terms of the host range for the same phage; direct spot tests exhibit the broadest coverage, followed by efficiency of plating and broth killing assays. This variability arises from distinct killing kinetics and the emergence of phage resistance under different testing conditions. Evaluating the strengths and limitations of each method comprehensively is crucial for enhancing phage efficacy assessment (35, 36).
Recognizing that the dynamics of phage resistance observed in controlled laboratory settings may not necessarily mirror what transpires in animals or humans is essential. Initiatives like Clinical Phage Microbiology aim to provide guidance for clinical decision-making, underscoring the significance of incorporating knowledge related to phage pharmacokinetics and pharmacodynamics (PK/PD) to optimize therapy. Developing a robust phage PK/PD framework requires the standardization of PD parameters and the establishment of dependable measurement methods to inform treatment protocols. This endeavor holds immense promise for advancing the field of phage therapy and its application in clinical practice.
However, there are only a few studies that cover the pharmacology of bacteriophage therapy, and even fewer that focus on the pharmacokinetics of phage therapy. Pharmacology focuses on how drugs interact with the body; it is further divided into pharmacokinetics, which evaluates how the body impacts drugs, and pharmacodynamics, which examines how drugs impact the body. In addition, the dosage quantity of a given phage cocktail was crucial for achieving enhanced pharmacokinetics; the high dosage. As it is crucial to produce the pharmacodynamic effects of the treatment, pharmacokinetics explains how well a drug can accumulate in the locality of the targeted tissues and is summarized in absorption, distribution, metabolism, and excretion (36).
According to Abedon et al. (35), drug dilution causes drug densities to decrease during both absorption and distribution, which may result in an increase in drug density in the targeted bodily organ. Different medication delivery methods are used depending on the pharmacokinetics. A variety of criteria are taken into consideration when choosing a drug’s delivery method, including the target tissue, the drug’s sensitivity to body enzymes, the patient’s convenience, and immunity. The formulation of the phage is yet another crucial factor in establishing efficient pharmacokinetics. The stability of phages is improved by using various formulation techniques. When treating various infections in both animal models and humans, it is important to take into account the different pharmacokinetics concepts that are necessary for the phage to penetrate the target bacteria, the achievement of an adequate phage concentration in the target’s locality, and an ample antibacterial response against the target.
Since 2010, all relevant research results regarding phages in vivo in preclinical studies have been compiled (Table 2). The published reports are systematically presented under the headings viz. infection syndrome, the animal used in the study, route of phage administration, microbial organism, clinical outcome, and findings, and reported adverse events, if any. Overall, preclinical studies on phages as an alternative to antibiotics for treating bacterial infections show promise in vivo. However, more investigation is required to completely comprehend the safety and effectiveness of phage therapy and identify the best dosage and delivery techniques for various bacterial infections and animal species (Table 3).
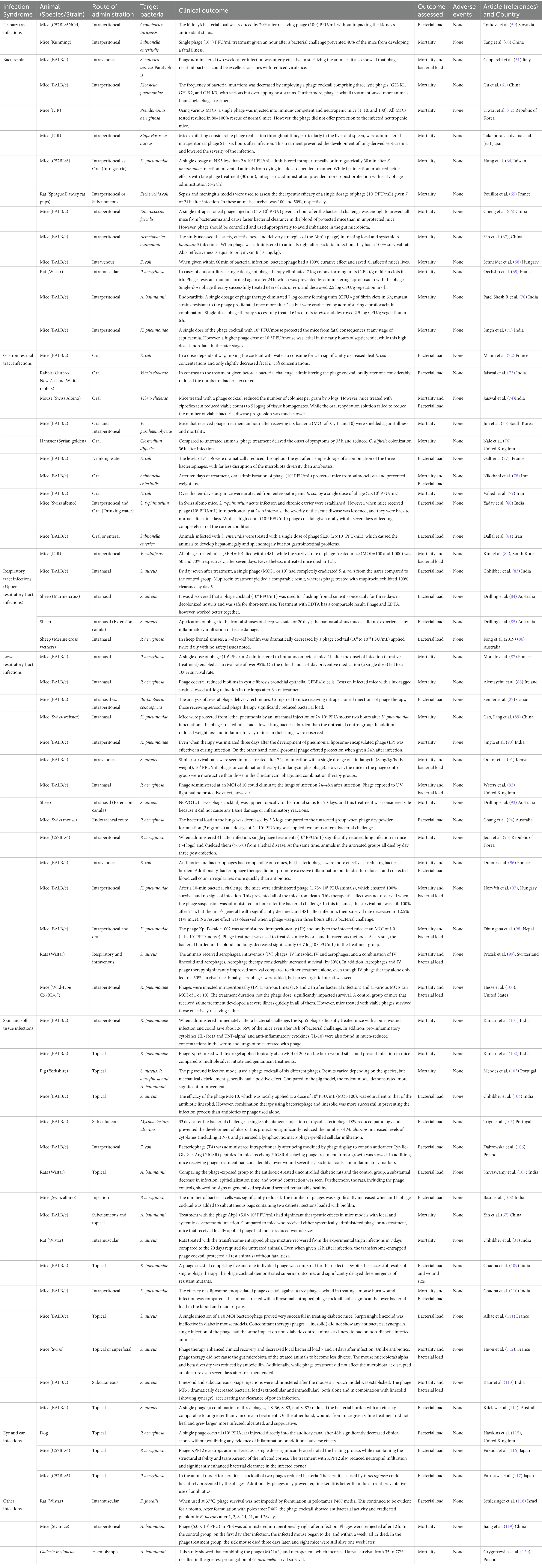
Table 3. A list of recent studies that are pertinent to the use of phages in vivo in preclinical trials.
Bacteriophage therapy in clinical infections
While phage therapy used with compassion (a profound awareness and empathy for another’s suffering) has recently increased with numerous case reports claimed to have cured patients, clinical trials intended to demonstrate its efficacy per current regulatory requirements have officially failed. Given the issue of rising antibiotic resistance, there needs to be an important decision on the role of phage therapy in contemporary medicine. The dramatic surge in case reports of treated patients reflects the growing interest in phage therapy. Additionally, various journals (Front Microbiol., Front Pharmacol., Viruses, Antibiotics, Pathogens, Microorganisms, etc.) have recently published special issues and study subjects on phage therapy (121).
Since their discovery, phages have been widely used in Eastern Europe and the former Soviet Union; as a result, their medical systems now incorporate the therapeutic use of phages. However, a thorough scientific analysis of this potential therapy has recently been conducted (41, 122). Abedon offered a list of essential requirements that should be carefully considered and reported in phage therapy (123). The effectiveness of clinical research depends on the proper characterization and selection of the phages, the participants (humans), and the target microorganisms. Though necessary, additional information like formulations, dosages, and potencies are only helpful when used with clearly defined and well-planned goals. The quality of future studies would increase with more thorough reporting, enabling the replication and expansion of earlier investigations. Choosing suitable disease targets for phage therapy is another factor to consider. Phage mixtures provide a wide range of activity when contemplating monotherapy or combination therapy techniques and lessen the possibility of resistance development. Additionally, it significantly increases the difficulty of determining how each phage in a cocktail would affect inflammation, the possibility of gene transfer, and the emergence of phage resistance (124).
In several world regions, bacteriophage therapy has been utilized for many years to treat bacterial infections. As reviewed by Uyttebroek et al. (125), Kutter et al. (41) Abedo et al. (35) and described in Marza et al. (126), encouraging results have been documented. Although bacteriophage therapy has been used for many years, there are few clinical studies in this field, raising many doubts about its effectiveness against infectious diseases. However, the growing demand for novel antimicrobial therapies drives the development of bacteriophage therapeutics for diverse diseases. These require the completion of extensive clinical trials per United States FDA or European EMA requirements (127). The phage’s bactericidal activity, and concerns about potential toxic shock must also be addressed.
Musculoskeletal infections
Fish et al. (128) presented a continuous case series examining the effectiveness of treating infected and poorly vascularized toe ulcers with the exposed bone when the recommended antibiotic therapy with topically applied S. aureus-specific phages failed. After providing standard wound care, the phage solution was topically given to the ulcerations weekly. All infections responded to the phage therapies, and infected bone debridement resulted in an average healing time of 7 weeks for the ulcers. In order to retain hallux function and treat an ulcer with extremely poor vascularity, 18 weeks of therapy were required.
The case report by Fish et al. (129) successfully treated distal phalangeal osteomyelitis in a 63-year-old diabetic woman with long-term bacteriophage follow-up. In a different study, Fish et al. (130) reported using the commercial formulation of the extensively researched anti-Staphylococcal bacteriophage Sb-1 from Eliava Bio Preparations to cure diabetic toe ulcers successfully. In difficult-to-close toe ulcers containing contaminated/infected bone, topical administration of a single S. aureus-targeting bacteriophage proved successful.
According to a study by Ferry et al. (131) local injections of a bacteriophage mixture during debridement, antibiotics, and implant retention (DAIR) procedures to treat recurrent S. aureus chronic joint prosthesis infection (PJI) were effective, safe, and associated with clinical success.
Cano et al. (132) reported intravenous injections of a single phage (KpJH462) to a 62-year-old diabetic patient with prosthetic infection due to K. pneumoniae complex, resolving local symptoms and signs of infection and restoring function. In addition, a trend toward reduction in biofilm biomass was observed 22 h after exposure to KpJH462.
A case study of a 72-year-old man with a chronic methicillin-resistant S. aureus prosthesis infection was published in 1971 by Doub et al. (133) Bacteriophage therapy was stopped following the third intravenous dose due to a rare, reversible transaminitis. Despite this, the patient’s severe chronic infection was successfully treated and eradicated.
A persistent methicillin-sensitive S. aureus (MSSA) prosthetic knee joint infection in a 61-year-old woman was successfully treated after a second cycle of bacteriophage therapy given during a two-stage replacement procedure, according to Ramirez-Sanchez et al. (134) The study also highlighted the success of bacteriophage therapy with a single lytic phage, the safety and effectiveness of intravenous and intra-articular infusions, and the development of serum neutralization with continued treatment.
The study by Onsea et al. (135) reported the successful use of bacteriophage therapy for patients with severe musculoskeletal infections. There was no recurrence of infection with the causal strains following a single phage therapy with concurrent antibiotics, with follow-up periods ranging from 8 to 16 months. In addition, the phage application protocol has not been associated with harmful side effects.
In a case report, Chan et al. (136) described the therapeutic application of phage OMKO1 to a chronic P. aeruginosa infection of an aortic Dacron graft with a related aortocutaneous fistula. Phage OMKO1 and ceftazidime were administered only once, and the infection seemed to clear up with no signs of recurrence.
Urinary tract infections
Ujmajuridze et al. (137) conducted a prospective cohort study to examine the efficacy of prophylactic bladder instillation in patients undergoing transurethral prostate resection. Nine patients got postoperative phage therapy by bladder instillation after a preoperative examination of asymptomatic individuals for the presence of uropathogens in midstream urine. No adverse events were reported. In six cases, the bacterial burden was also decreased. The outcomes of this two-phase, prospective trial indicated that tailored bacteriophage therapy for treating UTI might be efficient and secure.
A Dutch case study by Kuipers et al. (138) showed how bacteriophage was utilized successfully to treat chronic recurring urinary tract infections in a kidney transplant recipient caused by extended-spectrum lactamase (ESBL)-positive K. pneumoniae.
The study by Corbellino et al. (139) on long-term, multisite colonization by an MDR K. pneumoniae strain in a patient with a single kidney, cutaneous ureterostomy, and permanent ureteral stent resolved after 3 weeks of treatment with personalized BT given both orally and intrarectally.
In refractory P. aeruginosa urinary tract infection accompanied by bilateral ureteric stents and bladder ulcers, Khawaldeh et al. (140) demonstrated the benefit of adjuvant bacteriophage therapy after recurrent failure of antibiotics alone. The dynamics of bacteria and bacteriophages in urine point to a self-perpetuating and self-limiting infection, and no bacteriophage-resistant bacteria formed.
In a clinical trial by Quin et al. (141), phage therapy was utilized to treat a man with multiple urinary tract infections caused by MDR K. pneumoniae. However, three phage therapies were unsuccessful due to polyclonal co-infectious cells in his renal pelvis and bladder. Therefore, a percutaneous nephrostomy was done on the patient (PCN) following analysis. In addition to receiving antibiotic therapy, a mixture of bacteriophages chosen for their ability to attack all 21 diverse isolates were simultaneously irrigated via the kidney and bladder. The patient eventually made a full recovery with an improved bladder.
Other studies investigating the use of bacteriophage therapy in individuals with persistent urinary tract infections have shown its efficacy in overall clinical improvement (symptom alleviation and prevention of UTI recurrence) and bacterial eradication (142–144).
Skin and soft tissue infections
Equal healing rates were observed in the control and test groups of Rhodes D. et al.’s phase I clinical trial on bacteriophage therapy for treating human venous leg ulcers. In the study, 39 patients had their chronic blood vessel leg ulcers treated for 12 weeks with either a bacteriophage product or a placebo. No adverse events or safety issues were recorded (145).
The PhagoBurn Section 1/2 study by Jault P. et al. (5) was another clinical trial that compared standard care (Sulfa-drug silver emulsion cream) with a cocktail of twelve anti-P. aeruginosa lytic phages applied via an alginate dressing for a seven-day treatment of burn wound infection in 25 patients. Unfortunately, bacteriophage therapy has shown to be ineffective due to several factors, including variations in the maximum bacterial load between treatment groups and a significantly lower-than-expected bacteriophage dosage due to a concentration decrease during manufacturing and the fall in titres (during storage) were not checked before using them in the clinical trial.
A study by Patel et al. (146) using a customized bacteriophage preparation in managing chronic nonhealing wounds reported 100% microbiological eradication after 3 months with a healing rate of 81.2%. Additionally, the study suggested that specific phage therapy is equally effective whether a patient has diabetes or not, despite healing being slower in the former cluster. Another study by Gupta P et al. (147) examined the impact of topical bacteriophage therapy on chronic nonhealing wounds infected with E. coli, S. aureus, and P. aeruginosa. They found that after 3 to 5 doses, there was a significant improvement in wound healing with no signs of infection, both clinically and microbiologically.
Both studies offered nearly unambiguous proof that topical phage therapy helped patients who had not responded to conventional therapy completely heal their wounds. Furthermore, the isolates from the research population’s chronic wounds showed significant resistance to the most widely used antibiotics. Neither study’s participants experienced adverse effects, tissue breakdown, or recurrent infections during or after therapy.
In order to determine the impact of bacteriophage on the healing process, Bhartiya et al. (148) conducted a non-randomized, prospective, open-label, blinded, case–control study on infected acute traumatic wounds. The results of this investigation are promising. In patients receiving phage therapy, the average time needed to achieve sterility, complete wound granulation, and primarily intended healing was half that of the control group. The financial analysis also favored bacteriophage therapy (BT), as only 1/3 of the costs were incurred in the BT group compared to the control group.
Marza et al. (126) reported clinical improvement and bacterial elimination after phage therapy in a patient with an infected burn wound. In contrast, Rose T. et al. (149) discovered that phage therapy had no therapeutic effects on burn wounds. Bacteriophage use, however, had no adverse effects.
Ophthalmic infections
Infectious keratitis induced by Vancomycin-resistant S. aureus was described in the study by Fadlallah et al. (150) Phage treatment was given intravenously and topically in the form of eye drops and nasal spray. There were no concurrent antibiotics given. The infection was effectively treated, as evidenced by stabilizing ocular symptoms and observing negative cultures. The six-month follow-up period had showed no adverse effects and no reinfection.
Intensive care for patients with sepsis
Many studies have not been done on patients who received phage therapy for severe sepsis. S. aureus, P. aeruginosa, E. coli, K. pneumoniae, Proteus mirabilis, Morganella morganii, and Enterobacter spp. were the pathogens that were frequently isolated. Phage treatment was given intravenously, intramuscularly, or both intramuscularly and locally. Of the 109 patients, 85 (78%) received antibiotics simultaneously. Adverse incidents were not reported. Follow-up lasted anywhere between 20 days and 4 months. As a result, 90 (83%) of the 109 patients had improved vital signs, and two wholly eradicated their bacteria. Unfortunately, phage therapy proved ineffective in 20 patients; three died despite the initial improvement, and five died shortly after the treatment began (151–153).
Gastrointestinal infections
A 68-year-old diabetic patient with necrotizing pancreatitis was described in a case report by Schooley et al. (154) with an MDR A. baumannii infection. The patient’s clinical course was reversed, the bacteria were cleared up, and they recovered after receiving these bacteriophages intravenously and through percutaneously into the abscess cavities.
Cardiovascular infections
Three studies detail individuals with aortic graft and left ventricular assist system infections that were difficult to cure and were infected by P. aeruginosa or S. aureus (136, 155, 156). Two patients received local phage therapy, while one received intravenous phage therapy. Antibiotics were given concurrently to all patients. After receiving a local phage application, one patient reported nausea. There was a clinical improvement in all cases; one patient showed evidence of bacterial eradication. The follow-up period lasted 7-9 months.
Respiratory infections
Several case studies have been published regarding phage therapy and patients with respiratory infections (157–166). Phage therapy was administered intravenously, orally, and intravenously using a nebulizer. Phage treatment was well tolerated in each case and had no adverse side effects. Furthermore, clinical improvement was observed in most cases, including general improvement and reduced sputum and coughing.
Other infections
Nine patients with chronic rhinosinusitis caused by S. aureus were treated with intranasally given phage therapy in a prospective study by Ooi et al. (167). The treatment was examined for safety and preliminary efficacy. Phage therapy was well tolerated, and the side effects (such as diarrhea, epistaxis, signs of an upper respiratory infection, oropharyngeal discomfort, rhinitis, and a drop in serum bicarbonate) were modest. All nine patients saw improvements in their clinical conditions, and the bacterial load was reduced; however, only two (22%) of the nine patients had their bacteria wholly eradicated. There was a seven-day follow-up period (167).
Despite many positive case studies, there still needs to be robust scientific evidence from well-planned, monitored and regulated clinical trials supporting bacteriophage therapy. However, more recently, a rise in the number of bacteriophage therapy-related articles, books, and reviews and commercial bacteriophage companies focusing on a specific market suggests that the scientific communities and pharmaceutical companies are becoming more eager to integrate bacteriophage therapy into conventional medical practice (127).
Phage therapy may be helpful for bacterial illnesses that are challenging to cure. Additionally, this treatment is generally considered safe because it has a low incidence of side effects and is given via various administration routes. Even though phage therapy appears to be a promising strategy in the fight against untreatable infections and antimicrobial resistance, high-quality studies are desperately needed to advance our understanding of the long-term effects of this treatment. When taken orally and intravenously, getting more knowledge about the pharmacokinetics and pharmacodynamics of the bacteriophage (cocktails) is necessary. Additionally, endotoxin, bacterial, and viral contamination testing for bacteriophage solutions should be done (Table 4).
Worldwide phage development organizations
Phage therapy has been used for many years in certain countries, primarily in Eastern Europe, although it has yet to gain widespread acceptance in Western nations. This is partly due to the need for more commercial phage production, making it difficult to standardize and regulate phage therapy products.
However, several companies have recently been established to develop and commercialize phage-based products. These companies use various methods to produce phages, including isolating phages from environmental samples, genetically engineering phages, and producing phages using fermentation or other bioprocessing techniques. One of the main challenges in commercial phage production is ensuring consistent quality and purity. This is important to ensure that the phages are safe and effective for medical applications. In addition, companies use various methods to standardize and test their phage products, including use of bioassays to measure phage activity and testing for endotoxins and other contaminants. Several companies worldwide are developing and commercializing phage-based therapies for various bacterial infections. Here are some examples, summarized in Table 5, including the name of the company, its location, and the company website.
FDA-approved bacteriophage-based products and regulatory challenges
Phage therapy treatments have only been carried out in Western medicine under the laws of the Helsinki Declaration, which was adopted by the 18th World Medical Association General Assembly (Helsinki, Finland, June 1964) as unproven interventions in clinical practice or out of compassion and with the patient’s informed consent (175). A new legal framework has just been installed in Belgium that permits phages to be used as an active pharmaceutical ingredient in a magistral preparation as long as specific logical requirements are met. A medicinal preparation is described as “any medicinal product prepared in a pharmacy following a medical prescription for an individual patient,” in line with Article 3 of Directive 2001/83 of the European Parliament and Article 6 quarter 3 of the Belgian medicinal law of 25 March 1964 (176, 177). The preparation must be explicitly made by a pharmacist from the various components per current pharmaceutical standards and under a Medical Doctor’s (MD) prescription for a selected patient (177). In 2006, the United States Food and Drug Administration (FDA) approved using a phage as an antibacterial (preventive) agent in “the ready-to-eat food,” The process of developing and marketing phage therapy medical products (PTMPs) can theoretically be completed while adhering to all regulations, but it is exceedingly expensive and time-consuming. Furthermore, given the ongoing evolutionary dynamics between bacteria and phages, it is possible that the phage products that are eventually launched on the market are already obsolete or will do so shortly due to the lengthy development times concerning the divergent evolution of the targeted bacterial populations (178).
The current approach for producing and marketing pharmaceutical products was primarily designed for static chemical drugs like antibiotics and typically includes the following elements: Producing using good manufacturing practices (GMP), Preclinical research, including in vitro and animal pharmacokinetic, pharmacodynamic and toxicological investigations\Phase I to IV clinical trials and centralized marketing authorization (177).
The PhagoBurn study showed that producing PTMPs under the established pathway is very expensive, time-consuming, and only sometimes leads to high-quality and effective products. The licensed GMP research product’s titer was reduced drastically (1000-fold) 15 days after manufacturing, and manufacturing and licensing consumed the majority of the time and money allotted to the study (5). Phage therapy is a form of “experimental treatment” legal in Poland. The modified Act of 5 December 1996 on the Medical Profession, issued in the Polish Law Gazette, 2011, No. 277 item 1,634, and Article 37 of the Declaration of Helsinki, serve as the foundation for this framework. Phage treatment is practically feasible in Poland under specific circumstances, such as informed consent, a doctor’s application, and a bioethics panel’s approval, but only without any other potentially viable and established treatment alternative (177). Under the ‘compassionate use’ regulation, phage therapy may occasionally be used in various nations. Australia and France are examples (140, 179). Despite the European Medicines Agency (EMA)’s advice, each nation appears to employ a unique approach to this treatment.
The U.S. Food and Drug Administration (FDA) has been attempting to create a legal framework for employing bacteriophages as a therapeutic modality. In the United States, phage therapy has been considered an investigational new drug (IND) and regulated under the FDA’s IND program. This program allows for the clinical testing of new drugs and biologics in humans before they are approved for use in the general population. Recently, the FDA has taken steps to establish a more formal regulatory framework for phage therapy. In 2019, the agency released a draft guidance document on developing bacteriophage products for treating bacterial infections. The guidance document provides recommendations for developing and submitting data to support the safety and efficacy of phage products (180).
The FDA’s draft guidance recommends that phage products be evaluated in well-controlled clinical trials designed to demonstrate safety and efficacy. The guidance also recommends manufacturing phage products using appropriate quality control measures to ensure consistency and purity. It can be an essential step in regulating phage therapy. It provides a roadmap for companies developing phage products and clinicians interested in using phage therapy to treat bacterial infections. The guidance also provides a basis for discussion between the FDA and industry stakeholders on developing safe and effective phage products (181). Phage products have also been successfully produced globally in various applications, supporting their safety and efficacy after the approval of the FDA and other regulating bodies. Companies’ names, product details, agency approval, and related applications of phages are compiled in Tables 6, 7.
The information and references provided by the companies mentioned above further explain and support the scientific evidence regarding the potential of phage therapy as an antibiotic substitute and the FDA’s recommendations for creating bacteriophage therapy products.
Limitations and future prospect of implementing phage therapy
While laying out a roadmap of modern phage therapy’s difficulties, it becomes clear that our structured and organized environment brings many obstacles. The first is the uncertain nature of bacteriophages, which are not pure biological macromolecular complexes (like therapeutic proteins) but non-living creatures. As a result, it becomes difficult for regulatory agencies to approve medical therapies or therapeutic substances because legislative approval procedures are intricate, expensive, and time-consuming. Patients may also reject such therapies due to erroneous worries and a lack of knowledge because this approach uses “live viruses” for treatment (207).
Some additional problems attributed to early phage therapy failure and their probable solution and required approach are summarized below:
1. Narrow host range of phages: Due to the high specificity of phages, many negative results may have been acquired due to the inability to select lytic phages for the targeted bacterial species. Before utilizing phages for therapeutic purposes, ascertaining the etiologic agent’s susceptibility to phages (208) and using polyvalent phage cocktails that lyse most etiologic agent strains will be a better approach for favorable results.
2. Insufficient purity of phage preparation: Early therapeutic phages were in crude lysates of the host bacteria and contained a variety of contaminants (including endotoxins) that may have neutralized the phage’s effects. To create phage preparations with a high purity level, ion-exchange chromatography, high-speed centrifugation, and other advanced purification methods should be utilized (208).
3. Poor stability and viability of phage preparations: Commercial phage preparations were supplemented with mercurial, oxidizing agents or heat-treated to ensure bacterial sterility (50). Many of these treatments also may have inactivated the phages, resulting in ineffective phage preparations. Advanced purification techniques can purify phages and ensure they are bacterium-free. The viability and titer of phages should be determined before using them therapeutically.
4. Lack of understanding of the heterogeneity and mode of action of phages (i.e., lytic vs. lysogenic): Some researchers may have used lysogenic phages instead of lytic phages due to their inability to distinguish between them. The temperate phages are not recommended for therapy because they do not “kill” the target bacteria and can cause the transfer of undesired genes. A careful choice should be made when looking for lytic phages. This is essential for preventing potential horizontal gene transfer by lysogenic phages of genes encoding bacterial toxins, antibiotic resistance, etc. (209).
5. Exaggerated claims of the effectiveness of commercial phage preparation: One illustration of this would be the Enterophagos, marketed as applicable against herpes infections, urticaria, and eczema (210), diseases that phages could not possibly be successful against. Phage preparations should be provided with detailed, scientifically backed information regarding their effectiveness against specific bacterial pathogens, potential adverse effects, etc.
6. Failure to establish scientific proof of the efficacy of phage treatment: Most clinical investigations using therapeutic phages lacked placebo controls (211, 212). Highly pure, lytic phages should be used in well-controlled, double-blinded placebo experiments, and outcomes need to be assessed using patient data and meticulous laboratory testing.
7. Development of Phage-neutralizing antibodies: Another issue that could impair the ability of phages to lyse certain bacteria in vivo is the emergence of phage-neutralizing antibodies. Indeed, parenteral phage delivery has been associated with the formation of neutralizing antibodies (213). Nevertheless, it is still being determined how substantial of a concern this might be during phage therapy, particularly when administered orally and/or topically. Since the kinetics of phage activity is significantly faster than the host’s synthesis of neutralizing antibodies, the production of neutralizing antibodies should not, in theory, pose a substantial challenge during the initial treatment of acute infections (208). A study by Archana et al. (214) reported the appearance of neutralizing antibodies after the third week following immunization. Complete neutralization of bacteriophages was detectable between 3 and 5 weeks after immunization.
8. Clearance of phages by reticuloendothelial systems: According to the study by Merril et al. (215), the reticuloendothelial system’s removal of phages from the patient could represent a problem because it could lower the number of phages to a level that is insufficient to combat the infecting bacteria. The authors chose phages with greater capacity to remain in mice’s bloodstreams using a natural selection process they elegantly referred to as the “serial passage” method. Understanding the mechanisms underlying this characteristic of phages will illuminate critical aspects of how they interact with their bacterial hosts.
9. The emergence of resistance: Like antibiotics, bacteria can resist phages over time, leading to the need to develop new phages. Phage therapy’s efficiency may also be constrained by the co-evolution of bacteria and phages, which can result in the emergence of bacterial strains that are resistant to phages (9, 55, 173, 216).
10. Quality control: Ensuring the quality and purity of phage preparations can be challenging, as it requires careful monitoring of phage production and purification processes (217, 218).
11. Delivery methods: The mode of administration can impact the effectiveness of phage therapy since enough phages must be delivered to the infection site for treatment to be effective. This can be challenging in some cases, such as treating deep tissue infections or infections in areas that are difficult to access (22, 24).
Many phages produce virulence factors or toxins, making them ineffective as antimicrobial agents. The precise elimination of potentially dangerous genetic information from viral genomes can tackle this crucial safety concern. The release of bacterial toxins, lipopolysaccharides, and other pathogen-associated molecular patterns (PAMPs) as a result of phage-induced lysis might trigger an innate immune response, increase virulence, or result in additional harm. To lessen these possibly detrimental effects, phage variants that are engineered to destroy target cells without releasing PAMP can be utilized. Phages can be designed to cleave defined nucleotide sequences and genotypes, for example, host cells that carry antibiotic-resistance genes or particular virulence factors, by delivering sequence-specific CRISPR-Cas nucleases via modified genomes or phagemids. Phagemids or modified genomes can be used to deliver sequence-specific CRISPR-Cas nucleases, which can then be programmed to cleave specific genotypes and nucleotide sequences (217).
Phage therapy has not yet been widely adopted in clinical practice, despite increased interest in phages and the collaborative efforts of scientists and clinicians that have led to increased case reports. The fundamental reason for this lack of implementation is the ongoing dearth of reliable clinical evidence on phage therapy, which prevents ethical, actuarial, and governmental authorities from addressing their concerns. To make phage therapy an integral part of clinical practice in the future, a focused and practical regulatory framework for personalized phage therapy techniques and interdisciplinary collaboration between researchers, microbiologists, clinicians, and pharmacists are essential.
Conclusion
Phage therapy holds the promise of unveiling novel approaches to combat bacterial infections. Particularly noteworthy is the potential of phage cocktails, which could revolutionize the treatment landscape by offering solutions for a wide spectrum of bacterial diseases that have proven resistant even to the latest generations of antibiotics. Nevertheless, several pivotal challenges must be surmounted to harness the full potential of phages as antibacterial agents. These challenges encompass ensuring safety, evaluating effectiveness, and assessing the likelihood of immune responses triggered by administered phages. Additionally, refining phage purification techniques and optimizing their growth are essential hurdles that demand attention.
Within clinical settings, phage therapy has emerged as a beacon of promise, despite the obstacles. Ongoing technological advancements and strides in genetic engineering hold the potential to unlock the creation of more precise and potent phages. The synergistic use of phage therapy in conjunction with other therapeutic modalities, such as immunity modifiers or antibiotics, may further enhance its efficacy. Beyond the realm of human healthcare, phage therapy extends its reach into veterinary medicine, agriculture, and food safety, presenting multifaceted applications with vast potential.
In summation, phage therapy stands as a formidable contender in the battle against antibiotic-resistant bacteria, offering a compelling alternative or adjunct to traditional antibiotic treatments. Nevertheless, these prospects are not without their challenges. Continued research and development efforts are imperative to fully exploit the myriad advantages this promising technology offers and to surmount the remaining obstacles that lie in its path. The journey to harness the full potential of phage therapy is still underway, but the destination promises a brighter future in the fight against bacterial infections.
Author contributions
SK, MG, and GN planned to compile phage data systematically. SK, RK, and MG collected and analyzed the data from various authentic resources. SK compiled the data and finalized the review article as per the proposed objectives from the database and the major contributor to writing the manuscript. SK, MG, SB, and GN finalized the draft, and the published data was presented in the review article. All authors have read and approved the final manuscript.
Acknowledgments
The authors express gratitude for the support from the Department of Health Research, Ministry of Health and Family Welfare (Government of India), New Delhi, and the Indian Council of Medical Research (ICMR). This support was instrumental in establishing the State Level Viral Research and Diagnostic Laboratory (VRDL) network under scheme 5066. The scheme has since been updated under the Central sector scheme called the Pradhan Mantri-Ayushman Bharat Health Infrastructure Mission (PM-ABHIM), focusing on strengthening the already operational 80 VRDLs.
Conflict of interest
The authors declare that the research was conducted in the absence of any commercial or financial relationships that could be construed as a potential conflict of interest.
Publisher’s note
All claims expressed in this article are solely those of the authors and do not necessarily represent those of their affiliated organizations, or those of the publisher, the editors and the reviewers. Any product that may be evaluated in this article, or claim that may be made by its manufacturer, is not guaranteed or endorsed by the publisher.
References
1. O’Neill, J. Antimicrobial resistance. Antimicrobial resistance: tackling a crisis for the health and wealth of nations. (2014).
2. De Kraker, MEA, Stewardson, AJ, and Harbarth, S. Will 10 million people die a year due to antimicrobial resistance by 2050? PLoS Med. (2016) 13:e1002184. doi: 10.1371/journal.pmed.1002184
3. Bassetti, M, Poulakou, G, Ruppe, E, Bouza, E, Van Hal, SJ, and Brink, A. Antimicrobial resistance in the next 30 years, humankind, bugs and drugs: a visionary approach. Intensive Care Med. (2017) 43:1464–75. doi: 10.1007/s00134-017-4878-x
4. Tacconelli, E, Carrara, E, Savoldi, A, Harbarth, S, Mendelson, M, Monnet, DL, et al. Discovery, research, and development of new antibiotics: the WHO priority list of antibiotic-resistant bacteria and tuberculosis. Lancet Infect Dis. (2018) 18:318–27. doi: 10.1016/S1473-3099(17)30753-3
5. Jault, P, Leclerc, T, Jennes, S, Pirnay, JP, Que, YA, Resch, G, et al. Efficacy and tolerability of a cocktail of bacteriophages to treat burn wounds infected by Pseudomonas aeruginosa (PhagoBurn): a randomised, controlled, double-blind phase 1/2 trial. Lancet Infect Dis. (2019) 19:35–45. doi: 10.1016/S1473-3099(18)30482-1
6. Bakuradze, N, Merabishvili, M, Makalatia, K, Kakabadze, E, Grdzelishvili, N, Wagemans, J, et al. In vitro evaluation of the therapeutic potential of phage va7 against enterotoxigenic Bacteroides fragilis infection. Viruses. (2021) 13:2044. doi: 10.3390/v13102044
7. Jamal, M, Bukhari, SMAUS, Andleeb, S, Ali, M, Raza, S, Nawaz, MA, et al. Bacteriophages: an overview of the control strategies against multiple bacterial infections in different fields. J Basic Microbiol. (2019) 59:123–33. doi: 10.1002/jobm.201800412
8. Hanlon, GW. Bacteriophages: an appraisal of their role in the treatment of bacterial infections. Int J Antimicrob Agents. (2007) 30:118–28. doi: 10.1016/j.ijantimicag.2007.04.006
9. Górski, A, Międzybrodzki, R, Węgrzyn, G, Jończyk-Matysiak, E, Borysowski, J, and Weber-Dąbrowska, B. Phage therapy: current status and perspectives. Med Res Rev. (2020) 40:459–63. doi: 10.1002/med.21593
10. Hesse, S, and Adhya, S. Phage therapy in the twenty-first century: Facing the decline of the antibiotic era; is it finally time for the age of the phage? Annu Rev Microbiol. (2019) 73:155–74. doi: 10.1146/annurev-micro-090817-062535
11. Łusiak-Szelachowska, M, Weber-Dąbrowska, B, and Górski, A. Bacteriophages and Lysins in biofilm control. Virol Sin. (2020) 35:125–33. doi: 10.1007/s12250-019-00192-3
12. Veiga-Crespo, P, Ageitos, JM, Poza, M, and Villa, TG. Enzybiotics: a look to the future, recalling the past. J Pharm Sci. (2007) 96:1917–24. doi: 10.1002/jps.20853
13. Pires, DP, Oliveira, H, Melo, LDR, Sillankorva, S, and Azeredo, J. Bacteriophage-encoded depolymerases: their diversity and biotechnological applications. Appl Microbiol Biotechnol. (2016) 100:2141–51. doi: 10.1007/s00253-015-7247-0
14. Yang, H, Wang, M, Yu, J, and Wei, H. Antibacterial activity of a novel peptide-modified lysin against Acinetobacter baumannii and Pseudomonas aeruginosa. Front Microbiol. (2015) 6:1471. doi: 10.3389/fmic,b.2015.01471
15. Sybesma, W, Rohde, C, Bardy, P, Pirnay, JP, Cooper, I, Caplin, J, et al. Silk route to the acceptance and re-implementation of bacteriophage therapy–part II. Antibiotics. (2018) 7:35. doi: 10.3390/antibiotics7020035
16. Abdelkader, K, Gerstmans, H, Saafan, A, Dishisha, T, and Briers, Y. The preclinical and clinical progress of bacteriophages and their lytic enzymes: the parts are easier than the whole. Viruses. (2019) 11:96. doi: 10.3390/v11020096
17. Yoong, P, Schuch, R, Nelson, D, and Fischetti, VA. Identification of a broadly active phage lytic enzyme with lethal activity against antibiotic-resistant Enterococcus faecalis and Enterococcus faecium. J Bacteriol. (2004) 186:4808–12. doi: 10.1128/JB.186.14.4808-4812.2004
18. Fischetti, VA. Bacteriophage lytic enzymes: novel anti-infectives. Trends Microbiol. (2005) 13:491–6. doi: 10.1016/j.tim.2005.08.007
19. Fischetti, VA. 12 the use of phage lytic enzymes to control bacterial infections. In: Kutter, E, and Sulakvelidze, A, Bacteriophages: Biology and applications. Boca Raton, FL: CRC Press, p. 321, (2004).
20. Vázquez, R, García, E, and García, P. Phage lysins for fighting bacterial respiratory infections: a new generation of antimicrobials. Front Immunol. (2018) 9:2252. doi: 10.3389/fimmu.2018.02252
21. Loh, B, Gondil, VS, Manohar, P, Khan, FM, Yang, H, and Leptihn, S. Encapsulation and delivery of therapeutic phages. Appl Environ Microbiol. (2021) 87:e01979–20. doi: 10.1128/AEM.01979-20
22. Gondil, VS, and Chhibber, S. Evading antibody mediated inactivation of bacteriophages using delivery systems. J Virol Curr Res. (2017) 1:555–74. doi: 10.19080/JOJIV.2017.01.55557
23. Malik, DJ, Sokolov, IJ, Vinner, GK, Mancuso, F, Cinquerrui, S, Vladisavljevic, GT, et al. Formulation, stabilisation and encapsulation of bacteriophage for phage therapy. Adv Colloid Interf Sci. (2017) 249:100–33. doi: 10.1016/j.cis.2017.05.014
24. Gondil, VS, and Chhibber, S. Bacteriophage and endolysin encapsulation systems: a promising strategy to improve therapeutic outcomes. Front Pharmacol. (2021) 12:675440. doi: 10.3389/fphar.2021.675440
25. Leung, SSY, Parumasivam, T, Gao, FG, Carrigy, NB, Vehring, R, Finlay, WH, et al. Production of inhalation phage powders using spray freeze drying and spray drying techniques for treatment of respiratory infections. Pharm Res. (2016) 33:1486–96. doi: 10.1007/s11095-016-1892-6
26. Mensink, MA, Frijlink, HW, van der Voort Maarschalk, K, and Hinrichs, WLJ. How sugars protect proteins in the solid state and during drying (review): mechanisms of stabilization in relation to stress conditions. Eur J Pharm Biopharm. (2017) 114:288–95. doi: 10.1016/j.ejpb.2017.01.024
27. Semler, DD, Goudie, AD, Finlay, WH, and Dennis, JJ. Aerosol phage therapy efficacy in Burkholderia cepacia complex respiratory infections. Antimicrob Agents Chemother. (2014) 58:4005–13. doi: 10.1128/AAC.02388-13
28. Chang, RY, Wong, J, Mathai, A, et al. Production of highly stable spray dried phage formulations for treatment of Pseudomonas aeruginosa lung infection. Eur J Pharm Biopharm. (2017) 121:1–13. doi: 10.1016/J.EJPB.2017.09.002
29. Ibaraki, H, Kanazawa, T, Chien, WY, Nakaminami, H, Aoki, M, Ozawa, K, et al. The effects of surface properties of liposomes on their activity against Pseudomonas aeruginosa PAO-1 biofilm. J Drug Deliv Sci Technol. (2020) 57:101754. doi: 10.1016/j.jddst.2020.101754
30. Rukavina, Z, and Vanić, Ž. Current trends in development of liposomes for targeting bacterial biofilms. Pharmaceutics. (2016) 8:18. doi: 10.3390/pharmaceutics8020018
31. Chhibber, S, Shukla, A, and Kaur, S. Transfersomal phage cocktail is an effective treatment against methicillin-resistant Staphylococcus aureus-mediated skin and soft tissue infections. Antimicrob Agents Chemother. (2017) 61:e02146. doi: 10.1128/AAC.02146-16
32. Colom, J, Cano-Sarabia, M, Otero, J, Aríñez-Soriano, J, Cortés, P, Maspoch, D, et al. Microencapsulation with alginate/CaCO3: a strategy for improved phage therapy. Sci Rep. (2017) 7:41441. doi: 10.1038/srep41441
33. Rosner, D, and Clark, J. Formulations for bacteriophage therapy and the potential uses of immobilization. Pharmaceuticals. (2021) 14:359. doi: 10.3390/ph14040359
34. Anany, H, Chen, W, Pelton, R, and Griffiths, MW. Biocontrol of listeria monocytogenes and Escherichia coli O157: H7 in meat by using phages immobilized on modified cellulose membranes. Appl Environ Microbiol. (2011) 77:6379–87. doi: 10.1128/AEM.05493-11
35. Nang, SC, Lin, YW, Fabijan, AP, Chang, RYK, Rao, GG, Iredell, J, et al. Pharmacokinetics/pharmacodynamics of phage therapy: a major hurdle to clinical translation. Clin Microbiol Infect. (2023) 29:702–9. doi: 10.1016/j.cmi.2023.01.021
36. Qadir, MI, Mobeen, T, and Masood, A. Phage therapy: Progress in pharmacokinetics. Brazilian. J Pharm Sci. (2018) 54. doi: 10.1590/s2175-97902018000117093
37. Chernomordik, AB. Bacteriophages and their therapeutic-prophylactic use. Med Sestra. (1989) 48:44–7.
38. Gupta, R, and Prasad, Y. Efficacy of polyvalent bacteriophage P-27/HP to control multidrug resistant Staphylococcus aureus associated with human infections. Curr Microbiol. (2011) 62:255–60. doi: 10.1007/s00284-010-9699-x
39. Skurnik, M, Pajunen, M, and Kiljunen, S. Biotechnological challenges of phage therapy. Biotechnol Lett. (2007) 29:995–1003. doi: 10.1007/s10529-007-9346-1
40. Abedon, ST, and Thomas-Abedon, C. Phage therapy pharmacology. Curr Pharm Biotechnol. (2010) 11:28–47. doi: 10.2174/138920110790725410
41. Kutter, E, De Vos, D, Gvasalia, G, Alavidze, Z, Gogokhia, L, Kuhl, S, et al. Phage therapy in clinical practice: treatment of human infections. Curr Pharm Biotechnol. (2010) 11:69–86. doi: 10.2174/138920110790725401
42. Sun, F, Qu, F, Ling, Y, et al. Biofilm-associated infections: antibiotic resistance and novel therapeutic strategies. Future Microbiol. (2013) 8:877–86. doi: 10.2217/fmb.13.58
43. Drulis-Kawa, Z, and Maciejewska, B. Special Issue: “Bacteriophages and biofilms”. Viruses. (2021) 13:257. doi: 10.3390/V13020257
44. Seth, AK, Geringer, MR, Nguyen, KT, Agnew, SP, Dumanian, Z, Galiano, RD, et al. Bacteriophage therapy for Staphylococcus aureus biofilm-infected wounds: a new approach to chronic wound care. Plast Reconstr Surg. (2013) 131:225–34. doi: 10.1097/PRS.0b013e31827e47cd
45. Gurjala, AN, Geringer, MR, Seth, AK, Hong, SJ, Smeltzer, MS, Galiano, RD, et al. Development of a novel, highly quantitative in vivo model for the study of biofilm-impaired cutaneous wound healing. Wound Repair Regen. (2011) 19:400–10. doi: 10.1111/J.1524-475X.2011.00690.X
46. Pires, DP, Melo, LDR, Vilas Boas, D, Sillankorva, S, and Azeredo, J. Phage therapy as an alternative or complementary strategy to prevent and control biofilm-related infections. Curr Opin Microbiol. (2017) 39:48–56. doi: 10.1016/J.MIB.2017.09.004
47. Chegini, Z, Khoshbayan, A, Moghadam, MT, Farahani, I, Jazireian, P, and Shariati, A. Bacteriophage therapy against Pseudomonas aeruginosa biofilms: a review. Ann Clin Microbiol Antimicrob. 19:45.
48. Chang, C, Yu, X, Guo, W, Guo, C, Guo, X, Li, Q, et al. Bacteriophage-mediated control of biofilm: a promising new Dawn for the future. Front Microbiol. (2022) 13:825828. doi: 10.3389/fmicb.2022.825828
49. Hyman, P, and Abedon, ST. Bacteriophage host range and bacterial resistance. Adv Appl Microbiol. (2010) 70:217–48. doi: 10.1016/S0065-2164(10)70007-1
50. Carlton, RM. Phage therapy: past history and future prospects. Arch Immunol Ther Exp. (1999) 47:267–74.
51. Capparelli, R, Nocerino, N, Iannaccone, M, Ercolini, D, Parlato, M, Chiara, M, et al. Bacteriophage therapy of Salmonella enterica: a fresh appraisal of bacteriophage therapy. J Infect Dis. (2010) 201:52–61. doi: 10.1086/648478
52. Skurnik, M, and Strauch, E. Phage therapy: facts and fiction. Int J Med Microbiol. (2006) 296:5–14. doi: 10.1016/j.ijmm.2005.09.002
53. Górski, A, Borysowski, J, and Międzybrodzki, R. Bacteriophages in medicine. Bacteriophage. (2007):125–58.
54. Mann, NH. The potential of phages to prevent MRSA infections. Res Microbiol. (2008) 159:400–5. doi: 10.1016/j.resmic.2008.04.003
55. Loc-Carrillo, C, and Abedon, ST. Pros and cons of phage therapy. Bacteriophage. (2011) 1:111–4. doi: 10.4161/bact.1.2.14590
56. Clokie, MRJ, and Kropinski, A. Methods and protocols, volume 1: isolation, characterization, and interactions methods in molecular biology. Totowa, NJ: Humana Press, pp. 69–81. (2009).
57. Manohar, P, AshokJ, T, Leptihn, S, and Ramesh, N. Pharmacological and immunological aspects of phage therapy. Infect Microb Dis. (2019) 1:34–42. doi: 10.1097/im9.0000000000000013
58. Chopra, I, Hodgson, J, Metcalf, B, and Poste, G. The search for antimicrobial agents effective against bacteria resistant to multiple antibiotics. Antimicrob Agents Chemother. (1997) 41:497–503. doi: 10.1128/AAC.41.3.497
59. Tóthová, Ľ, Celec, P, Bábíčková, J, Gajdošová, J, al-Alami, H, Kamodyova, N, et al. Phage therapy of Cronobacter-induced urinary tract infection in mice. Med Sci Monit. (2011) 17:BR173–8. doi: 10.12659/msm.>16271
60. Tang, F, Zhang, P, Zhang, Q, Xue, F, Ren, J, Sun, J, et al. Isolation and characterization of a broad-spectrum phage of multiple drug resistant Salmonella and its therapeutic utility in mice. Microb Pathog. (2019) 126:193–8. doi: 10.1016/j.micpath.2018.10.042
61. Gu, J, Liu, X, Li, Y, et al. A method for generation phage cocktail with great therapeutic potential. PLoS One. (2012) 7:e31698. doi: 10.1371/journal.pone.0031698
62. Tiwari, BR, Kim, S, Rahman, M, and Kim, J. Antibacterial efficacy of lytic Pseudomonas bacteriophage in normal and neutropenic mice models. J Microbiol. (2011) 49:994–9. doi: 10.1007/s12275-011-1512-4
63. Takemura-Uchiyama, I, Uchiyama, J, Osanai, M, Morimoto, N, Asagiri, T, Ujihara, T, et al. Experimental phage therapy against lethal lung-derived septicemia caused by Staphylococcus aureus in mice. Microbes Infect. (2014) 16:512–7. doi: 10.1016/j.micinf.2014.02.011
64. Hung, CH, Kuo, CF, Wang, CH, Wu, CM, and Tsao, N. Experimental phage therapy in treating Klebsiella pneumoniae-mediated liver abscesses and bacteremia in mice. Antimicrob Agents Chemother. (2011) 55:1358. doi: 10.1128/AAC.01123-10
65. Pouillot, F, Chomton, M, Blois, H, Courroux, C, Noelig, J, Bidet, P, et al. Efficacy of bacteriophage therapy in experimental Sepsis and meningitis caused by a clone O25b:H4-ST131 Escherichia coli strain producing CTX-M-15. Antimicrob Agents Chemother. (2012) 56:3568–75. doi: 10.1128/AAC.06330-11
66. Cheng, M, Liang, J, Zhang, Y, Hu, L, Gong, P, Cai, R, et al. The bacteriophage EF-P29 efficiently protects against lethal vancomycin-resistant enterococcus faecalis and alleviates gut microbiota imbalance in a murine bacteremia model. Front Microbiol. (2017) 8:837. doi: 10.3389/fmicb.2017.00837
67. Yin, S, Huang, G, Zhang, Y, Jiang, B, Yang, Z, Dong, Z, et al. Phage Abp1 rescues human cells and mice from infection by Pan-drug resistant Acinetobacter baumannii. Cell Physiol Biochem. (2018) 44:2337–45. doi: 10.1159/000486117
68. Schneider, G, Szentes, N, Horváth, M, Dorn, A, Cox, A, Nagy, G, et al. Kinetics of targeted phage rescue in a mouse model of systemic Escherichia coli K1. Biomed Res Int. (2018) 2018:7569645. doi: 10.1155/2018/7569645
69. Oechslin, F, Piccardi, P, Mancini, S, et al. Synergistic interaction between phage therapy and antibiotics clears Pseudomonas aeruginosa infection in endocarditis and reduces virulence. J Infect Dis. (2017) 215:703. doi: 10.1093/INFDIS/JIW632
70. Patel, SR, Pratap, CB, and Nath, G. Evaluation of bacteriophage cocktail on septicaemia caused by colistin-resistant Acinetobacter baumannii in immunocompromised mice model. Indian J Med Res. (2021) 154:141. doi: 10.4103/IJMR.IJMR_2271_18
71. Singh, A, Singh, AN, Rathor, N, Chaudhry, R, Singh, SK, and Nath, G. Evaluation of bacteriophage cocktail on septicemia caused by colistin-resistant Klebsiella pneumoniae in mice model. Front Pharmacol. (2022) 13:778676. doi: 10.3389/fphar.2022.778676
72. Maura, D, Galtier, M, le Bouguénec, C, and Debarbieux, L. Virulent bacteriophages can target O104:H4 Enteroaggregative Escherichia coli in the mouse intestine. Antimicrob Agents Chemother. (2012) 56:6235. doi: 10.1128/AAC.00602-12
73. Jaiswal, A, Koley, H, Ghosh, A, Palit, A, and Sarkar, B. Efficacy of cocktail phage therapy in treating Vibrio cholerae infection in rabbit model. Microbes Infect. (2013) 15:152–6. doi: 10.1016/J.MICINF.2012.11.002
74. Jaiswal, A, Koley, H, Mitra, S, Saha, DR, and Sarkar, B. Comparative analysis of different oral approaches to treat Vibrio cholerae infection in adult mice. Int J Med Microbiol. (2014) 304:422–30. doi: 10.1016/J.IJMM.2014.02.007
75. Jun, JW, Shin, TH, Kim, JH, Shin, SP, Han, JE, Heo, GJ, et al. Bacteriophage therapy of a Vibrio parahaemolyticus infection caused by a multiple-antibiotic-resistant O3:K6 pandemic clinical strain. J Infect Dis. (2014) 210:72–8. doi: 10.1093/INFDIS/JIU059
76. Nale, JY, Spencer, J, Hargreaves, KR, Trzepiński, P, Douce, GR, and Clokie, MRJ. Bacteriophage combinations significantly reduce Clostridium difficile growth in vitro and proliferation in vivo. Antimicrob Agents Chemother. (2016) 60:968. doi: 10.1128/AAC.01774-15
77. Galtier, M, de Sordi, L, Maura, D, Arachchi, H, Volant, S, Dillies, MA, et al. Bacteriophages to reduce gut carriage of antibiotic resistant uropathogens with low impact on microbiota composition. Environ Microbiol. (2016) 18:2237–45. doi: 10.1111/1462-2920.13284
78. Nikkhahi, F, Soltan Dallal, MM, Alimohammadi, M, Rahimi Foroushani, A, Rajabi, Z, Fardsanei, F, et al. Phage therapy: assessment of the efficacy of a bacteriophage isolated in the treatment of salmonellosis induced by Salmonella enteritidis in mice. Gastroenterol Hepatol Bed Bench. (2017) 10:131–6.
79. Vahedi, A, Soltan Dallal, MM, Douraghi, M, Nikkhahi, F, Rajabi, Z, Yousefi, M, et al. Isolation and identification of specific bacteriophage against enteropathogenic Escherichia coli (EPEC) and in vitro and in vivo characterization of bacteriophage. FEMS Microbiol Lett. (2018) 365:fny136. doi: 10.1093/FEMSLE/FNY136
80. Yadav, VB, and Nath, G. Bacteriophage therapy of human-restricted Salmonella species—a study in a surrogate bacterial and animal model. Lett Appl Microbiol. (2022) 75:422–30. doi: 10.1111/LAM.13744
81. Dallal, MMS, Nikkhahi, F, Alimohammadi, M, Douraghi, M, Rajabi, Z, Foroushani, AR, et al. Phage therapy as an approach to control Salmonella enterica serotype Enteritidis infection in mice. Rev Soc Bras Med Trop. (2019) 52:e20190290. doi: 10.1590/0037-8682-0290-2019
82. Kim, HJ, Kim, YT, Kim, HB, Choi, SH, and Lee, JH. Characterization of bacteriophage VVP001 and its application for the inhibition of Vibrio vulnificus causing seafood-borne diseases. Food Microbiol. (2021) 94:103630. doi: 10.1016/j.fm.2020.103630
83. Chhibber, S, Gupta, P, and Kaur, S. Bacteriophage as effective decolonising agent for elimination of MRSA from anterior nares of BALB/c mice. BMC Microbiol. (2014) 14:1–13. doi: 10.1186/S12866-014-0212-8/FIGURES/5
84. Drilling, A, Morales, S, Boase, S, Jervis-Bardy, J, James, C, Jardeleza, C, et al. Safety and efficacy of topical bacteriophage and ethylenediaminetetraacetic acid treatment of Staphylococcus aureus infection in a sheep model of sinusitis. Int Forum Allergy Rhinol. (2014) 4:176–86. doi: 10.1002/ALR.21270
85. Drilling, AJ, Ooi, ML, Miljkovic, D, James, C, Speck, P, Vreugde, S, et al. Long-term safety of topical bacteriophage application to the frontal sinus region. Front Cell Infect Microbiol. (2017) 7:49. doi: 10.3389/FCIMB.2017.00049/BIBTEX
86. Fong, SA, Drilling, AJ, Ooi, ML, Paramasivan, S, Finnie, JW, Morales, S, et al. Safety and efficacy of a bacteriophage cocktail in an in vivo model of Pseudomonas aeruginosa sinusitis. Transl Res. (2019) 206:41–56. doi: 10.1016/J.TRSL.2018.12.002
87. Morello, E, Saussereau, E, Maura, D, Huerre, M, Touqui, L, and Debarbieux, L. Pulmonary bacteriophage therapy on Pseudomonas aeruginosa cystic fibrosis strains: first steps towards treatment and prevention. PLoS One. (2011) 6:e16963. doi: 10.1371/journal.pone.0016963
88. Alemayehu, D, Casey, PG, McAuliffe, O, Guinane, CM, Martin, JG, Shanahan, F, et al. Bacteriophages ϕMR299-2 and ϕNH-4 can eliminate Pseudomonas aeruginosa in the murine lung and on cystic fibrosis lung airway cells. MBio. (2012) 3:e00029–12. doi: 10.1128/mBio.00029-12
89. Cao, F, Wang, X, Wang, L, Li, Z, Che, J, Wang, L, et al. Evaluation of the efficacy of a bacteriophage in the treatment of pneumonia induced by multidrug resistance Klebsiella pneumoniae in mice. Biomed Res Int. (2015) 2015:752930. doi: 10.1155/2015/752930
90. Singla, S, Harjai, K, Katare, OP, and Chhibber, S. Bacteriophage-loaded nanostructured lipid carrier: improved pharmacokinetics mediates effective resolution of Klebsiella pneumoniae-induced lobar pneumonia. J Infect Dis. (2015) 212:325–34. doi: 10.1093/INFDIS/JIV029
91. Oduor, JMO, Onkoba, N, Maloba, F, and Nyachieo, A. Experimental phage therapy against haematogenous multi-drug resistant Staphylococcus aureus pneumonia in mice. Afr. J Lab Med. (2016) 5:435. doi: 10.4102/AJLM.V5I1.435
92. Waters, EM, Neill, DR, Kaman, B, Sahota, JS, MRJ, C, Winstanley, C, et al. Phage therapy is highly effective against chronic lung infections with Pseudomonas aeruginosa. Thorax. (2017) 72:666–7. doi: 10.1136/thoraxjnl-2016-209265
93. Drilling, AJ, Ooi, ML, Miljkovic, D, et al. Long-term safety of topical bacteriophage application to the frontal sinus region. Front Cell Infect Microbiol. (2017):7(FEB). doi: 10.3389/FCIMB.2017.00049
94. Chang, RYK, Chen, K, Wang, J, Wallin, M, Britton, W, Morales, S, et al. Proof-of-principle study in a murine lung infection model of antipseudomonal activity of phage PEV20 in a dry-powder formulation. Antimicrob Agents Chemother. (2018) 62:e01714. doi: 10.1128/AAC.01714-17
95. Jeon, J, and Yong, D. Two novel bacteriophages improve survival in Galleria mellonella infection and mouse acute pneumonia models infected with extensively drug-resistant Pseudomonas aeruginosa. Appl Environ Microbiol. (2019) 85:e02900–18. doi: 10.1128/AEM.02900-18
96. Dufour, N, Delattre, R, Chevallereau, A, Ricard, JD, and Debarbieux, L. Phage therapy of pneumonia is not associated with an overstimulation of the inflammatory response compared to antibiotic treatment in mice. Antimicrob Agents Chemother. (2019) 63:e00379. doi: 10.1128/AAC.00379-19
97. Horváth, M, Kovács, T, Koderivalappil, S, Ábrahám, H, Rákhely, G, and Schneider, G. Identification of a newly isolated lytic bacteriophage against K24 capsular type, carbapenem resistant Klebsiella pneumoniae isolates. Sci Rep. (2020) 10:5891. doi: 10.1038/s41598-020-62691-8
98. Dhungana, G, Regmi, M, Paudel, P, Parajuli, A, Upadhyay, E, Gyawali, I, et al. Therapeutic efficacy of bacteriophage therapy to treat carbapenem resistant Klebsiella pneumoniae in mouse model. J Nepal Health Res Council. (2021) 19:76–82. doi: 10.33314/jnhrc.v19i1.3282
99. Prazak, J, Valente, LG, Iten, M, Federer, L, Grandgirard, D, Soto, S, et al. Benefits of aerosolized phages for the treatment of pneumonia due to methicillin-resistant Staphylococcus aureus: an experimental study in rats. J Infect Dis. (2022) 225:1452–9. doi: 10.1093/INFDIS/JIAB112
100. Hesse, S, Malachowa, N, Porter, AR, Freedman, B, Kobayashi, SD, Gardner, DJ, et al. Bacteriophage treatment rescues mice infected with multidrug-resistant Klebsiella pneumoniae ST258. MBio. (2021) 12:1–11. doi: 10.1128/MBIO.00034-21
101. Kumari, S, Harjai, K, and Chhibber, S. Evidence to support the therapeutic potential of bacteriophage Kpn5 in burn wound infection caused by Klebsiella pneumoniae in BALB/c mice. J Microbiol Biotechnol. (2010) 20:935–41. doi: 10.4014/JMB.0909.09010
102. Kumari, S, Harjai, K, and Chhibber, S. Bacteriophage versus antimicrobial agents for the treatment of murine burn wound infection caused by Klebsiella pneumoniae B5055. J Med Microbiol. (2011) 60:205–10. doi: 10.1099/jmm.0.018580-0
103. Mendes, JJ, Leandro, C, Corte-Real, S, Barbosa, R, Cavaco-Silva, P, Melo-Cristino, J, et al. Wound healing potential of topical bacteriophage therapy on diabetic cutaneous wounds. Wound Repair Regen. (2013) 21:595–603. doi: 10.1111/WRR.12056
104. Chhibber, S, Kaur, T, and Kaur, S. Co-therapy using lytic bacteriophage and linezolid: effective treatment in eliminating methicillin resistant Staphylococcus aureus (MRSA) from diabetic foot infections. PLoS One. (2013) 8:56022. doi: 10.1371/journal.pone.0056022
105. Trigo, G, Martins, TG, Fraga, AG, Longatto-Filho, A, Castro, AG, Azeredo, J, et al. Phage therapy is effective against infection by Mycobacterium ulcerans in a murine footpad model. PLoS Negl Trop Dis. (2013) 7:e2183. doi: 10.1371/journal.pntd.0002183
106. Daṃbrowska, K, Kaźmierczak, Z, Majewska, J, Miernikiewicz, P, Piotrowicz, A, Wietrzyk, J, et al. Bacteriophages displaying anticancer peptides in combined antibacterial and anticancer treatment. Future Microbiol. (2014) 9:861–9. doi: 10.2217/fmb.14.50
107. Shivaswamy, VC, Kalasuramath, SB, Sadanand, CK, Basavaraju, AK, Ginnavaram, V, Bille, S, et al. Ability of bacteriophage in resolving wound infection caused by multidrug-resistant Acinetobacter baumannii in uncontrolled diabetic rats. Microb Drug Resist. (2015) 21:171–7. doi: 10.1089/mdr.2014.0120
108. Basu, S, Agarwal, M, and Nath, G. An in vivo wound model utilizing bacteriophage therapy of Pseudomonas aeruginosa biofilms. Ostomy Wound Manage. (2015) 61:16–23.
109. Chadha, P, Katare, OP, and Chhibber, S. In vivo efficacy of single phage versus phage cocktail in resolving burn wound infection in BALB/c mice. Microb Pathog. (2016) 99:68–77. doi: 10.1016/j.micpath.2016.08.001
110. Chadha, P, Katare, OP, and Chhibber, S. Liposome loaded phage cocktail: enhanced therapeutic potential in resolving Klebsiella pneumoniae mediated burn wound infections. Burns. (2017) 43:1532–43. doi: 10.1016/j.burns.2017.03.029
111. Albac, S, Medina, M, Labrousse, D, Hayez, D, Bonnot, D, Anzala, N, et al. Efficacy of bacteriophages in a Staphylococcus aureus nondiabetic or diabetic foot infection murine model. Antimicrob Agents Chemother. (2020) 64:1870. doi: 10.1128/AAC.01870-19
112. Huon, JF, Montassier, E, Leroy, AG, Grégoire, M, Vibet, MA, Caillon, J, et al. Phages versus antibiotics to treat infected diabetic wounds in a mouse model: a microbiological and microbiotic evaluation. Microb Syst. (2020) 5:542. doi: 10.1128/msystems.00542-20
113. Kaur, S, and Chhibber, S. A mouse air pouch model for evaluating the anti-bacterial efficacy of phage MR-5 in resolving skin and soft tissue infection induced by methicillin-resistant Staphylococcus aureus. Folia Microbiol (Praha). (2021) 66:959–72. doi: 10.1007/s12223-021-00895-9
114. Kifelew, LG, Warner, MS, Morales, S, Vaughan, L, Woodman, R, Fitridge, R, et al. Efficacy of phage cocktail AB-SA01 therapy in diabetic mouse wound infections caused by multidrug-resistant Staphylococcus aureus. BMC Microbiol. (2020) 20:204. doi: 10.1186/s12866-020-01891-8
115. Hawkins, C, Harper, D, Burch, D, Änggård, E, and Soothill, J. Topical treatment of Pseudomonas aeruginosa otitis of dogs with a bacteriophage mixture: a before/after clinical trial. Vet Microbiol. (2010) 146:309–13. doi: 10.1016/j.vetmic.2010.05.014
116. Fukuda, K, Ishida, W, Uchiyama, J, Rashel, M, Kato, SI, Morita, T, et al. Pseudomonas aeruginosa keratitis in mice: effects of topical bacteriophage KPP12 administration. PLoS One. (2012) 7:e47742. doi: 10.1371/journal.pone.0047742
117. Furusawa, T, Iwano, H, Hiyashimizu, Y, Matsubara, K, Higuchi, H, Nagahata, H, et al. Phage therapy is effective in a mouse model of bacterial equine keratitis. Appl Environ Microbiol. (2016) 82:5332–9. doi: 10.1128/aem.01166-16
118. Shlezinger, M, Friedman, M, Houri-Haddad, Y, Hazan, R, and Beyth, N. Phages in a thermoreversible sustained-release formulation targeting E. faecalis in vitro and in vivo. PLoS One. (2019) 14:e0219599. doi: 10.1371/journal.pone.0219599
119. Jiang, L, Tan, J, Hao, Y, Wang, Q, Yan, X, Wang, D, et al. Isolation and characterization of a novel myophage Abp9 against pandrug resistant Acinetobacater baumannii. Front Microbiol. (2020) 11:506068. doi: 10.3389/fmicb.2020.506068
120. Grygorcewicz, B, Roszak, M, Golec, P, Śleboda-Taront, D, Łubowska, N, Górska, M, et al. Antibiotics act with vB_AbaP_AGC01 phage against Acinetobacter baumannii in human heat-inactivated plasma blood and galleria mellonella models. Int J Mol Sci. (2020) 21:1–14. doi: 10.3390/IJMS21124390
121. Górski, A, Borysowski, J, and Międzybrodzki, R. Phage therapy: towards a successful clinical trial. Antibiotics. (2020) 9:1–7. doi: 10.3390/antibiotics9110827
122. Villarroel, J, Larsen, MV, Kilstrup, M, and Nielsen, M. Metagenomic analysis of therapeutic PYO phage cocktails from 1997 to 2014. Viruses. (2017) 9:328. doi: 10.3390/v9110328
123. Abedon, ST. Information phage therapy research should report. Pharmaceuticals. (2017) 10:328. doi: 10.3390/ph10020043
124. Harper, DR. Criteria for selecting suitable infectious diseases for phage therapy. Viruses. (2018) 10:177. doi: 10.3390/V10040177
125. Uyttebroek, S, Chen, B, Onsea, J, Ruythooren, F, Debaveye, Y, Devolder, D, et al. Safety and efficacy of phage therapy in difficult-to-treat infections: a systematic review. Lancet Infect Dis. (2022) 22:e208–20. doi: 10.1016/S1473-3099(21)00612-5
126. Marza, JAS, Soothill, JS, Boydell, P, and Collyns, TA. Multiplication of therapeutically administered bacteriophages in Pseudomonas aeruginosa infected patients. Burns. (2006) 32:644–6. doi: 10.1016/j.burns.2006.02.012
127. Parracho, HM, Burrowes, BH, and Enright, MC. The role of regulated clinical trials in the development of bacteriophage therapeutics. J Mol Genet Med. (2012) 6:279:286. doi: 10.4172/1747-0862.1000050
128. Fish, R, Kutter, E, Wheat, G, Blasdel, B, Kutateladze, M, and Kuhl, S. Bacteriophage treatment of intransigent diabetic toe ulcers: a case series. Methods Mol Biol. (2016) 25:S27–33. doi: 10.12968/jowc.2016.25.sup7.s27
129. Fish, R, Kutter, E, Bryan, D, Wheat, G, and Kuhl, S. Resolving digital staphylococcal osteomyelitis using bacteriophage–a case report. Antibiotics. (2018) 7:87. doi: 10.3390/antibiotics7040087
130. Fish, R, Kutter, E, Wheat, G, Blasdel, B, Kutateladze, M, and Kuhl, S. Compassionate use of bacteriophage therapy for foot ulcer treatment as an effective step for moving toward clinical trials. Methods Mol Biol. (2018) 1693:159–70. doi: 10.1007/978-1-4939-7395-8_14
131. Ferry, T, Leboucher, G, Fevre, C, Herry, Y, Conrad, A, Josse, J, et al. Salvage debridement, antibiotics and implant retention (“DAIR”) with local injection of a selected cocktail of bacteriophages: is it an option for an elderly patient with relapsing Staphylococcus aureus prosthetic-joint infection? Open forum. Infect Dis. (2018) 5:ofy269. doi: 10.1093/ofid/ofy269
132. Cano, EJ, Caflisch, KM, Bollyky, PL, van Belleghem, JD, Patel, R, Fackler, J, et al. Phage therapy for limb-threatening prosthetic knee Klebsiella pneumoniae infection: case report and in vitro characterization of anti-biofilm activity. Clin Infect Dis. (2021) 73:e144:–e151. doi: 10.1093/cid/ciaa705
133. Doub, JB, Ng, VY, Johnson, AJ, Slomka, M, Fackler, J, Horne, B’A, et al. Salvage bacteriophage therapy for a chronic MRSA prosthetic joint infection. Antibiotics (Basel). (2020) 9:241. doi: 10.3390/antibiotics9050241
134. Ramirez-Sanchez, C, Gonzales, F, Buckley, M, Biswas, B, Henry, M, Deschenes, MV, et al. Successful treatment of Staphylococcus aureus prosthetic joint infection with bacteriophage therapy. Viruses. (2021) 13:1182. doi: 10.3390/V13061182
135. Onsea, J, Soentjens, P, Djebara, S, Merabishvili, M, Depypere, M, Spriet, I, et al. Bacteriophage application for difficult-to-treat musculoskeletal infections: development of a standardized multidisciplinary treatment protocol. Viruses. (2019) 11:891. doi: 10.3390/V11100891
136. Chan, BK, Turner, PE, Kim, S, Mojibian, HR, Elefteriades, JA, and Narayan, D. Phage treatment of an aortic graft infected with Pseudomonas aeruginosa. Evol Med Public Health. (2018) 2018:60. doi: 10.1093/emph/eoy005
137. Ujmajuridze, A, Chanishvili, N, Goderdzishvili, M, Leitner, L, Mehnert, U, Chkhotua, A, et al. Adapted bacteriophages for treating urinary tract infections. Front Microbiol. (2018) 9:1832. doi: 10.3389/fmicb.2018.01832/full
138. Kuipers, S, Ruth, MM, Mientjes, M, De Sévaux, RGL, and Van Ingen, J. A Dutch case report of successful treatment of chronic relapsing urinary tract infection with bacteriophages in a renal transplant patient. Antimicrob Agents Chemother. (2019) 64:e01281. doi: 10.1128/AAC.01281-19
139. Corbellino, M, Kieffer, N, Kutateladze, M, Balarjishvili, N, Leshkasheli, L, Askilashvili, L, et al. Eradication of a multidrug-resistant, Carbapenemase-producing Klebsiella pneumoniae isolate following Oral and intra-rectal therapy with a custom made, lytic bacteriophage preparation. Rev Infect Dis. (2020) 70:1998–2001. doi: 10.1093/cid/ciz782
140. Khawaldeh, A, Morales, S, Dillon, B, Alavidze, Z, Ginn, AN, Thomas, L, et al. Bacteriophage therapy for refractory Pseudomonas aeruginosa urinary tract infection. J Med Microbiol. (2011) 60:1697–700. doi: 10.1099/JMM.0.029744-0
141. Qin, J, Wu, N, Bao, J, Shi, X, Ou, H, Ye, S, et al. Heterogeneous Klebsiella pneumoniae co-infections complicate personalized bacteriophage therapy. Front Cell Infect Microbiol. (2021) 10:608402. doi: 10.3389/FCIMB.2020.608402
142. Bao, J, Wu, N, Zeng, Y, Chen, L, Li, L, Yang, L, et al. Non-active antibiotic and bacteriophage synergism to successfully treat recurrent urinary tract infection caused by extensively drug-resistant Klebsiella pneumoniae. Emerg Microbes Infect. (2020) 9:771–4. doi: 10.1080/22221751.2020.1747950
143. Rostkowska, OM, Międzybrodzki, R, Miszewska-Szyszkowska, D, Górski, A, and Durlik, M. Treatment of recurrent urinary tract infections in a 60-year-old kidney transplant recipient. The use of phage therapy. Transpl Infect Dis. (2021) 23:e13391. doi: 10.1111/tid.13391
144. Johri, AV, Johri, P, Hoyle, N, Pipia, L, Nadareishvili, L, and Nizharadze, D. Chronic bacterial prostatitis treated with phage therapy after multiple failed antibiotic treatments. Front Pharmacol. (2021) 12:692614. doi: 10.3389/fphar.2021.692614
145. Rhoads, DD, Wolcott, RD, Kuskowski, MA, Wolcott, BM, and Ward, LS. Bacteriophage therapy of venous leg ulcers in humans. J Wound Care. (2009) 18:237–8. doi: 10.12968/jowc.2009.18.6.42801
146. Patel, DR, Bhartiya, SK, Kumar, R, Shukla, VK, and Nath, G. Use of customized bacteriophages in the treatment of chronic nonhealing wounds: a prospective study. Int J Lower Extrem Wounds. (2021) 20:37–46. doi: 10.1177/1534734619881076
147. Gupta, P, Singh, HS, Shukla, VK, Nath, G, and Bhartiya, SK. Bacteriophage therapy of chronic nonhealing wound: clinical study. Int J Lower Extrem Wounds. (2019) 18:171–5. doi: 10.1177/1534734619835115
148. Bhartiya, SK, Prasad, R, Sharma, S, Shukla, V, Nath, G, and Kumar, R. Biological therapy on infected traumatic wounds: a case-control study. Int J Low Extrem Wounds. (2022):727. doi: 10.1177/15347346211072779
149. Rose, T, Verbeken, G, De Vos, D, Merabishvili, M, Vaneechoutte, M, Lavigne, R, et al. Experimental phage therapy of burn wound infection: difficult first steps. Int J burns. Trauma. (2014) 4:66–73.
150. Fadlallah, A, Chelala, E, and Legeais, JM. Corneal infection therapy with topical bacteriophage Administration. Open Ophthalmol J. (2015) 9:167–8. doi: 10.2174/1874364101509010167
151. Jennes, S, Merabishvili, M, Soentjens, P, Pang, KW, Rose, T, Keersebilck, E, et al. Use of bacteriophages in the treatment of colistin-only-sensitive Pseudomonas aeruginosa septicaemia in a patient with acute kidney injury-a case report. Crit Care. (2017) 21:129. doi: 10.1186/S13054-017-1709-Y
152. Duplessis, C, Biswas, B, Hanisch, B, Perkins, M, Henry, M, Quinones, J, et al. Refractory Pseudomonas bacteremia in a 2-year-old sterilized by bacteriophage therapy. J Pediatric Infect Dis Soc. (2018) 7:253–6. doi: 10.1093/jpids/pix056
153. Petrovic Fabijan, A, Lin, RCY, Ho, J, Maddocks, S, Ben Zakour, NL, Iredell, JR, et al. Safety of bacteriophage therapy in severe Staphylococcus aureus infection. Nat Microbiol. (2020) 5:465–72. doi: 10.1038/S41564-019-0634-Z
154. Schooley, RT, Biswas, B, Gill, JJ, Hernandez-Morales, A, Lancaster, J, Lessor, L, et al. Development and use of personalized bacteriophage-based therapeutic cocktails to treat a patient with a disseminated resistant Acinetobacter baumannii infection. Antimicrob Agents Chemother. (2017) 61:e00954. doi: 10.1128/AAC.00954-17
155. Aslam, S, Pretorius, V, Lehman, SM, Morales, S, and Schooley, RT. Novel bacteriophage therapy for treatment of left ventricular assist device infection. J Heart Lung Transplant. (2019) 38:475–6. doi: 10.1016/j.healun.2019.01.001
156. Mulzer, J, Trampuz, A, Trampuz, A, and Potapov, EV. Treatment of chronic left ventricular assist device infection with local application of bacteriophages. Eur J Cardiothorac Surg. (2020) 57:1003–4. doi: 10.1093/ejcts/ezz295
157. Maddocks, S, Fabijan, AP, Ho, J, Lin, RCY, Ben Zakour, NL, Dugan, C, et al. Bacteriophage therapy of ventilator-associated pneumonia and empyema caused by Pseudomonas aeruginosa. Am J Respir Crit Care Med. (2019) 200:1179–81. doi: 10.1164/rccm.201904-0839LE
158. Law, N, Logan, C, Yung, G, Furr, CLL, Lehman, SM, Morales, S, et al. Successful adjunctive use of bacteriophage therapy for treatment of multidrug-resistant Pseudomonas aeruginosa infection in a cystic fibrosis patient. Infection. (2019) 47:665–8. doi: 10.1007/S15010-019-01319-0
159. Aslam, S, Courtwright, AM, Koval, C, Lehman, SM, Morales, S, Furr, CLL, et al. Early clinical experience of bacteriophage therapy in 3 lung transplant recipients. Am J Transplant. (2019) 19:2631–9. doi: 10.1111/ajt.15503
160. Dedrick, RM, Guerrero-Bustamante, CA, Garlena, RA, Russell, DA, Ford, K, Harris, K, et al. Engineered bacteriophages for treatment of a patient with a disseminated drug-resistant Mycobacterium abscessus. Nat Med. (2019) 25:730–3. doi: 10.1038/S41591-019-0437-Z
161. Gainey, AB, Burch, AK, Brownstein, MJ, Brown, DE, Fackler, J, Horne, B'A, et al. Combining bacteriophages with cefiderocol and meropenem/vaborbactam to treat a pan-drug resistant Achromobacter species infection in a pediatric cystic fibrosis patient. Pediatr Pulmonol. (2020) 55:2990–4. doi: 10.1002/ppul.24945
162. Hoyle, N, Zhvaniya, P, Balarjishvili, N, Bolkvadze, D, Nadareishvili, L, Nizharadze, D, et al. Phage therapy against Achromobacter xylosoxidans lung infection in a patient with cystic fibrosis: a case report. Res Microbiol. (2018) 169:540–2. doi: 10.1016/j.resmic.2018.05.001
163. Tan, X, Chen, H, Zhang, M, Zhao, Y, Jiang, Y, Liu, X, et al. Clinical experience of personalized phage therapy against Carbapenem-resistant Acinetobacter baumannii lung infection in a patient with chronic obstructive pulmonary disease. Front Cell Infect Microbiol. (2021) 11:631585. doi: 10.3389/fcimb.2021.631585
164. Dedrick, RM, Freeman, KG, Nguyen, JA, Bahadirli-Talbott, A, Smith, BE, Wu, AE, et al. Potent antibody-mediated neutralization limits bacteriophage treatment of a pulmonary Mycobacterium abscessus infection. Nat Med. (2021) 27:1357–61. doi: 10.1038/S41591-021-01403-9
165. Wu, N, Dai, J, Guo, M, Li, J, Zhou, X, Li, F, et al. Pre-optimized phage therapy on secondary Acinetobacter baumannii infection in four critical COVID-19 patients. Emerg Microbes Infect. (2021) 10:612–8. doi: 10.1080/22221751.2021.1902754
166. Lebeaux, D, Merabishvili, M, Caudron, E, Lannoy, D, Van Simaey, L, Duyvejonck, H, et al. A case of phage therapy against Pandrug-resistant Achromobacter xylosoxidans in a 12-year-old lung-transplanted cystic fibrosis patient. Viruses. (2021) 13:10060. doi: 10.3390/V13010060
167. Ooi, ML, Drilling, AJ, Morales, S, Fong, S, Moraitis, S, Macias-Valle, L, et al. Safety and tolerability of bacteriophage therapy for chronic rhinosinusitis due to Staphylococcus aureus. JAMA Otolaryngol Head Neck Surg. (2019) 145:723–9. doi: 10.1001/jamaoto.2019.1191
168. Tamma, PD, Souli, M, Billard, M, Campbell, J, Conrad, D, Ellison, DW, et al. Safety and microbiological activity of phage therapy in persons with cystic fibrosis colonized with Pseudomonas aeruginosa: study protocol for a phase 1b/2, multicenter, randomized, double-blind, placebo-controlled trial. Trials. 23:1057. doi: 10.1186/s13063-022-07047-5
169. CYPHY. CYstic Fibrosis bacterioPHage Study at Yale (CYPHY)–Study Results. (2023). Available at: https://clinicaltrials.gov/ct2/show/results/NCT04684641 (Accessed June 18, 2023).
170. CTRI. (2023). Available at: https://ctri.nic.in/Clinicaltrials/regtrial.php?modid=1&compid=19&EncHid=37814.12246 (Accessed June 18, 2023).
171. UTI. Phage Therapy for the Treatment of Urinary Tract Infection. (2023). Available at: https://clinicaltrials.gov/ct2/show/NCT05537519 (Accessed June 18, 2023).
172. Huang, Y, Wang, W, Zhang, Z, Gu, Y, Huang, A, Wang, J, et al. Phage products for fighting antimicrobial resistance. Microorganisms. (2022) 10:1324. doi: 10.3390/microorganisms10071324
173. Kropinski, AM. Phage therapy-everything old is new again. Can J Infect Dis Med Microbiol. 17:297–306. doi: 10.1155/2006/329465
174. Endersen, L, O’Mahony, J, Hill, C, Ross, RP, McAuliffe, O, and Coffey, A. Phage therapy in the food industry. Annu Rev Food Sci Technol. (2014) 5:327–49. doi: 10.1146/annurev-food-030713-092415
175. World Medical Association. World Medical Association Declaration of Helsinki: Ethical principles for medical research involving human subjects. JAMA. (2013) 310:2191–4. doi: 10.1001/jama.2013.281053
176. Pirnay, JP, Verbeken, G, Ceyssens, PJ, Huys, I, De Vos, D, Ameloot, C, et al. The magistral phage Viruses. Viruses. (2018) 10:2. doi: 10.3390/V10020064
177. De Vos, D, Verbeken, G, Quintens, J, and Pirnay, JP. Phage therapy in Europe: Regulatory and intellectual property protection issues. D VosDe, Phage Therapy: A Practical Approach. Berlin: Springer, pp. 363–377. (2019).
178. Administration USFD. Food additives permitted for direct addition to food for human consumption; bacteriophage preparation. Fed Regist. (2006) 71:47729–32.
179. Patey, O, McCallin, S, Mazure, H, Liddle, M, Smithyman, A, and Dublanchet, A. Clinical indications and compassionate use of phage therapy: personal experience and literature review with a focus on osteoarticular infections. Viruses. (2019) 11:18. doi: 10.3390/v11010018
180. Voelker, R. FDA approves bacteriophage trial. JAMA. (2019) 321:638. doi: 10.1001/jama.2019.0510
181. US Food and Drug Administration. Draft guidance for industry: Development of bacteriophage therapy products. (2019). Available at: https://www.fda.gov/media/159400/download. (Accessed March 2, 2023).
182. Vaughan, TJ, Williams, AJ, Pritchard, K, Osbourn, JK, Pope, AR, Earnshaw, JC, et al. Human antibodies with sub-nanomolar affinities isolated from a large non-immunized phage display library. Nat Biotechnol. (1996) 14:309–14. doi: 10.1038/nbt0396-309
183. Sarhan, WA, and Azzazy, HME. Phage approved in food, why not as a therapeutic? Expert Rev Anti-Infect Ther. (2015) 13:91–101. doi: 10.1586/14787210.2015.990383
184. Hamzeh-Mivehroud, M, Alizadeh, AA, Morris, MB, Bret Church, W, and Dastmalchi, S. Phage display as a technology delivering on the promise of peptide drug discovery. Drug Discov Today. (2013) 18:1144–57. doi: 10.1016/j.drudis.2013.09.001
185. Morozov, VA, Morozov, AV, Schürmann, D, Jessen, H, and Kücherer, C. Transmembrane protein polymorphisms and resistance to T-20 (Enfuvirtide, Fuzeon) in HIV-1 infected therapy-naive seroconverters and AIDS patients under HAART-T-20 therapy. Virus Genes. (2007) 35:167–74. doi: 10.1007/S11262-007-0098-8
186. Takagi, T, Arisawa, T, Yamamoto, K, Hirata, I, Nakano, H, and Sawada, M. Identification of ligands binding specifically to inflammatory intestinal mucosa using phage display. Clin Exp Pharmacol Physiol. (2007) 34:286–9. doi: 10.1111/J.1440-1681.2007.04563.X
187. Intralytix, Inc. (2023). Available at: http://www.intralytix.com/index.php?page=prod (Accessed March 3, 2023).
188. Alomari, MMM, Dec, M, and Urban-Chmiel, R. Bacteriophages as an alternative method for control of zoonotic and foodborne pathogens. Viruses. (2021) 13:2348. doi: 10.3390/v13122348
189. Soffer, N, Woolston, J, Li, M, Das, C, and Sulakvelidze, A. Bacteriophage preparation lytic for Shigella significantly reduces Shigella sonnei contamination in various foods. PLoS One. (2017) 12:e0175256. doi: 10.1371/journal.pone.0175256
190. Zhang, X, Niu, YD, Nan, Y, Stanford, K, Holley, R, McAllister, T, et al. SalmoFresh™ effectiveness in controlling Salmonella on romaine lettuce, mung bean sprouts and seeds. Int J Food Microbiol. (2019):108250:305. doi: 10.1016/j.ijfoodmicro.2019.108250
191. Moye, ZD, Woolston, J, and Sulakvelidze, A. Bacteriophage applications for Food production and processing. Viruses. (2018) 10:205. doi: 10.3390/v10040205
192. Candidate Products–Pherecydes. (2023). Available at: https://www.pherecydes-pharma.com/en/candidate-products/ (Accessed March 3, 2023).
193. Galtier, M, De Sordi, L, Sivignon, A, De Vallée, A, Maura, D, Neut, C, et al. Bacteriophages targeting adherent invasive Escherichia coli strains as a promising new treatment for Crohn’s disease. J Crohns Colitis. (2017) 11:840–7. doi: 10.1093/ecco-jcc/jjw224
194. Figileco. (2023). Available at: http://www.phageluxagrihealth.com/en/ (Accessed March 3, 2023).
195. Bacteriophages. (2023). Available at: https://www.microgen.ru/en/products/bakteriofagi/ (Accessed March 3, 2023).
196. ELIAVA. (2023). Available at: https://phage.ge/?lang=en (Accessed March 3, 2023).
197. Our Pipeline–Biomx. (2023). https://www.biomx.com/our-pipeline/ (Accessed March 3, 2023).
198. Mai, V, Ukhanova, M, Reinhard, MK, Li, M, and Sulakvelidze, A. Bacteriophage administration significantly reduces Shigella colonization and shedding by Shigella-challenged mice without deleterious side effects and distortions in the gut microbiota. Bacteriophage. (2015) 5:e1088124. doi: 10.1080/21597081.2015.1088124
199. Phage Products BioChimPharm. (2023). https://biochimpharm.ge/en/products/ (Accessed March 3, 2023).
200. Production. (2023). Available at: https://aziyaimmunopreparat.uz/%D0%BF%D1%80%D0%BE%D0%B4%D1%83%D0%BA%D1%86%D0%B8%D1%8F (Accessed March 3, 2023).
201. Produkty Výzkum, vývoj a výroba MB Pharma. (2023). Available at: https://www.mbph.cz/produkty (Accessed March 3, 2023).
202. About RPC Micromir. (2023). Available at: https://micromir.bio/about-eng (Accessed March 3, 2023).
203. Development Pipeline–Adaptive Phage Therapeutics. (2023). Available at: https://aphage.com/science/pipeline/ (Accessed March 3, 2023).
204. Selle, K, Fletcher, JR, Tuson, H, Schmitt, DS, McMillan, L, Vridhambal, GS, et al. In vivo targeting of Clostridioides difficile using phage-delivered CRISPR-Cas3 antimicrobials. MBio. (2020) 11:e00019. doi: 10.1128/MBIO.00019-20
205. Sciphage Products. (2023). Available at: https://sciphage.com/products/ (Accessed March 3, 2023).
206. Ellis Day Skin Science–Balancing Phage Serum. (2023). Available at: https://www.ellisdayskinscience.com/products/balancing-phage-serum (Accessed March 3, 2023).
207. Leptihn, S, and Loh, B. Complexity, challenges and costs of implementing phage therapy. Future Microbiol. (2022) 17:643–6. doi: 10.2217/fmb-2022-0054
208. Sulakvelidze, A, Alavidze, Z, and Morris, JG. Bacteriophage therapy. Antimicrob Agents Chemother. (2001) 45:649–59. doi: 10.1128/AAC.45.3.649-659.2001
209. Alisky, J, Iczkowski, K, Rapoport, A, and Troitsky, N. Bacteriophages show promise as antimicrobial agents. J Infect. (1998) 36:5–15. doi: 10.1016/s0163-4453(98)92874-2
210. Barrow, PA, and Soothill, JS. Bacteriophage therapy and prophylaxis: rediscovery and renewed assessment of potential. Trends Microbiol. (1997) 5:268–71. doi: 10.1016/S0966-842X(97)01054-8
211. Eaton, MD, and Bayne-Jones, S. Bacteriophage therapy: review of the principles and results of the use of bacteriophage in the treatment of infections. J Am Med Assoc. (1934) 103:1769–76. doi: 10.1001/jama.1934.72750490003007
212. Krueger, AP, and Scribner, EJ. The bacteriophage: its nature and its therapeutic use. J Am Med Assoc. (1941) 116:2269–77.
213. Kucharewicz-Krukowska, A, and Slopek, S. Immunogenic effect of bacteriophage in patients subjected to phage therapy. Arch Immunol Ther Exp. (1987) 35:553–61.
214. Archana, A, Patel, PS, Kumar, R, and Nath, G. Neutralizing antibody response against subcutaneously injected bacteriophages in rabbit model. Virus. (2021) 32:38–45. doi: 10.1007/s13337-021-00673-8
215. Merril, CR, Biswas, B, Carlton, R, Jensen, NC, Creed, GJ, Zullo, S, et al. Long-circulating bacteriophage as antibacterial agents. Proc Natl Acad Sci. (1996) 93:3188–92. doi: 10.1073/PNAS.93.8.3188
216. Bhargava, K, Nath, G, Bhargava, A, Aseri, GK, and Jain, N. Phage therapeutics: from promises to practices and prospectives. Appl Microbiol Biotechnol. (2021) 105:9047–67. doi: 10.1007/s00253-021-11695-z
217. Kilcher, S, and Loessner, MJ. Engineering bacteriophages as versatile biologics. Trends Microbiol. (2019) 27:355–67. doi: 10.1016/j.tim.2018.09.006
Keywords: bacteriophage, veterinary medicine, preclinical AND clinical trials, immune response, treatment challenges
Citation: Karn SL, Gangwar M, Kumar R, Bhartiya SK and Nath G (2023) Phage therapy: a revolutionary shift in the management of bacterial infections, pioneering new horizons in clinical practice, and reimagining the arsenal against microbial pathogens. Front. Med. 10:1209782. doi: 10.3389/fmed.2023.1209782
Edited by:
Mark Willcox, University of New South Wales, AustraliaReviewed by:
Vijay Singh Gondil, University of Rochester Medical Center, United StatesUrmi Bajpai, University of Delhi, India
Copyright © 2023 Karn, Gangwar, Kumar, Bhartiya and Nath. This is an open-access article distributed under the terms of the Creative Commons Attribution License (CC BY). The use, distribution or reproduction in other forums is permitted, provided the original author(s) and the copyright owner(s) are credited and that the original publication in this journal is cited, in accordance with accepted academic practice. No use, distribution or reproduction is permitted which does not comply with these terms.
*Correspondence: Gopal Nath, Z29wYWxuYXRoQGdtYWlsLmNvbQ==; Mayank Gangwar, bWF5YW5rZ2FuZ3dhcjIwMDhAZ21haWwuY29t
†These authors have contributed equally to this work