- Department of Dermatology, The Second Affiliated Hospital of Chongqing Medical University, Chongqing, China
Mammalian target of rapamycin (mTOR) signaling pathway is a major regulator of cell proliferation and metabolism, playing significant roles in proliferation, apoptosis, inflammation, and illness. More and more evidences showed that the mTOR signaling pathway affects hair follicle circulation and maintains the stability of hair follicle stem cells. mTOR signaling may be a critical cog in Vitamin D receptor (VDR) deficiency-mediated hair follicle damage and degeneration and related alopecia disorders. This review examines the function of mTOR signaling in hair follicles and hair diseases, and talks about the underlying molecular mechanisms that mTOR signaling regulates.
1. Introduction
Mammalian target of rapamycin (mTOR) is a serine/threonine protein kinase that detects and combines a range of external and intracellular signals. It also regulates a number of functions, such as gene transcription, autophagy, mRNA translation, protein synthesis, cell proliferation, and metabolism (1, 2). Target of Rapamycin (TOR), named after its inhibitor rapamycin, was first isolated from soil bacteria in the 1970s. Rapamycin, also known as sirolimus, interacts with fk506-binding protein 12 (FKBP12) to limit the function of mTOR (3). Recent studies have demonstrated that mTOR signaling plays a role in a variety of epidermal homeostasis activities, including hair follicle cycles, skin barrier function, and skin healing (4–8). The incidence of numerous hair diseases is linked with dysregulation of mTOR signaling, such as alopecia areata, androgenetic alopecia, fibrosing alopecia, and hair follicle tumors (9–12). In this review, the function of mTOR signaling in hair follicles and hair diseases was highlighted, and underlying molecular mechanisms regulated by mTOR were discussed. We envision that analyzing these mTOR functional implications during hair follicle cycling will be essential for the development of new treatments for hair diseases.
2. mTOR signaling
The mTOR pathway is a crucial metabolic regulator. The mTOR protein exists in two complexes with distinct structures and functions, called mTOR complex 1 and mTOR complex 2 (1, 13). The proline-rich Akt substrate 40 kDa (PRAS40), an inhibitory subunit, and the regulatory associated protein of mTOR (RAPTOR) are both included in the mTORC1 protein. Numerous growth factor receptors, including the insulin receptor and the epidermal growth factor receptor (EGFR), activate tyrosine kinase adapter molecules at the cell membrane, which attract class I PI3K to the receptor complex. Phosphatidylinositol-3,4,5-trisphosphate (PI [3,4,5] P3), which attracts and stimulates the serine–threonine kinase Akt by phosphorylation on threonine 308, is the pathway through which PI3K activates mTORC1. A major target of Akt is tuberous sclerosis syndrome 2 (TSC2). By phosphorylating TSC2 at threonine 1,462 (Thr1462), Akt prevents the small GTPase RAS homolog enriched in the brain (Rheb) from acting as a GTPase-activating protein (GAP), keeping it in a GTP-bound state and activating mTORC1. The activation of class I PI3K ultimately leads to the activation of mTORC1 by inhibiting TSC2. mTORC1 is activated in organelles like peroxisomes and lysosomes (14). Full activation of mTORC1 requires many nutrients and energy sources, such as glucose, lipids, oxygen, leucine, arginine, and a high ATP/AMP ratio (15, 16) (Figure 1A).
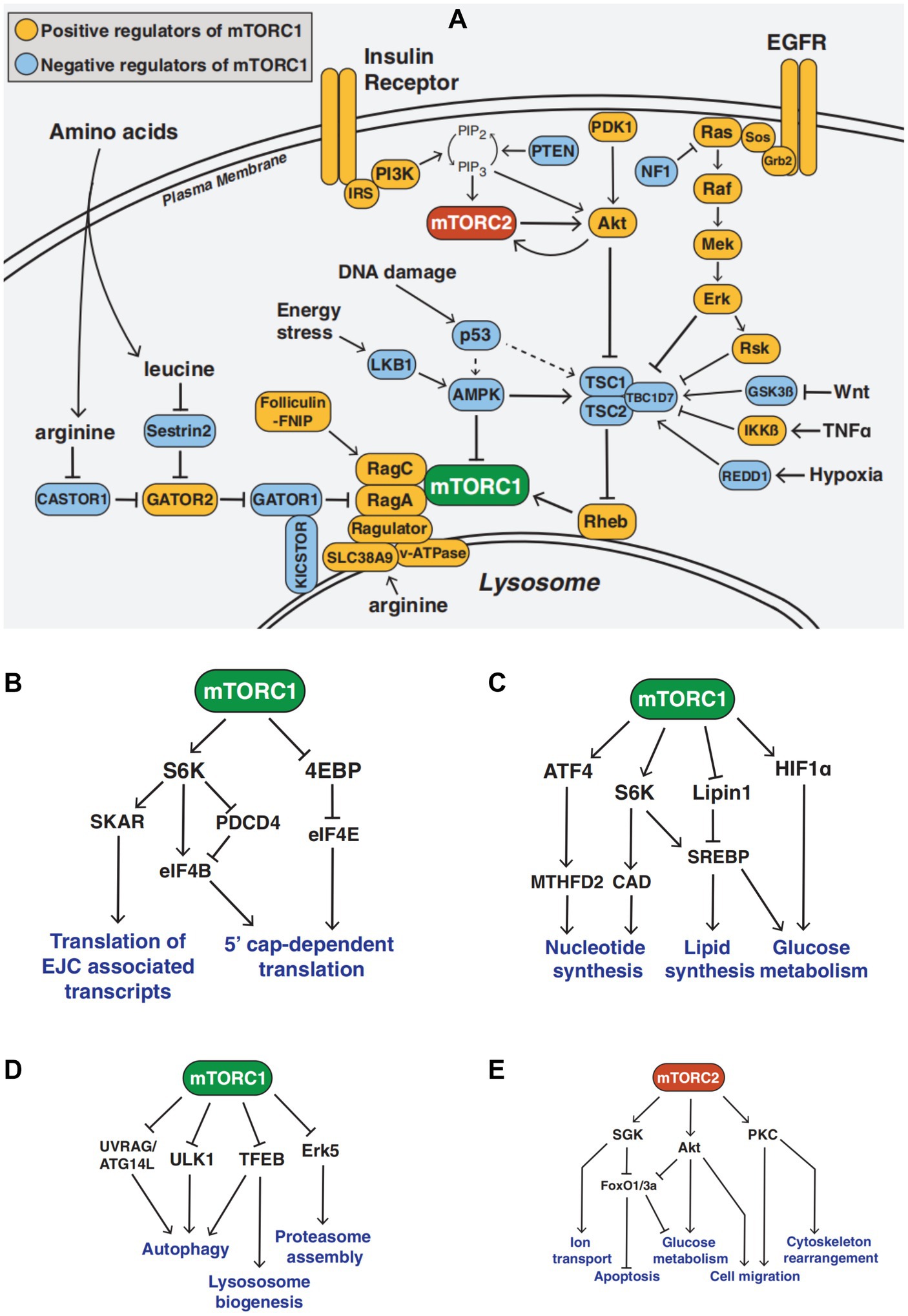
Figure 1. The mTOR Signaling Network (A) Overview of the mTOR signaling pathway (B–D) mTORC1 signaling in mRNA translation, metabolism, and protein turnover (E) mTORC2 signaling function.
The functions of mTORC1 include mRNA translation, metabolism, protein turnover, etc. mRNA translation includes translation of the multi-protein exon junction complex (EJC)-associated transcripts and 5′ cap-dependent translation (17, 18). Metabolism includes nucleotide synthesis, lipid synthesis, and glucose metabolism (19). Protein turnover includes autophagy, lysosome biogenesis, and proteasome assembly (20, 21). Activated mTORC1 can activate s6k, promote protein synthesis, inhibit 4E-BP, regulate translation initiation, phosphorylate ULK1 and ATG, and inhibit the occurrence and development of autophagy. Additionally, mTORC1 inhibition increases ULK1 kinase activity to encourage autophagy (22) (Figures 1B–D).
Rapamycin-insensitive mTOR companion (RICTOR) and mammalian stress-activated Map kinase interacting 1 (mSIN1) are both found in mTORC2. Additionally, PI3K through PI (3–5) p3 activates mTORC2, which phosphorylates Akt on serine 473. The functions of mTORC2 include ion transport, cell migration, apoptosis, glucose metabolism, and cytoskeleton reorganization (23–26). mTORC2 acts downstream of growth factor signaling, activates Akt, PKC, and Sgk1, and stimulates cell growth, proliferation, survival, and cytoskeletal remodeling (27–29). In certain cells and in living animals, chronic rapamycin administration reduces mTORC2 signaling (30, 31) (Figure 1E).
3. Roles of mTOR signaling in hair follicle development and hair regeneration
Hair is a fiber of amazing tensile strength composed primarily of terminally differentiated, dead keratinocytes. The hair follicle is an important structure supporting hair growth, and its periodic structural changes are the basis of hair cycles. The disease and aging of hair follicles will lead to an imbalance of hair cycles, and eventually cause hair loss and other hair diseases. Hair follicles generally go through cycles of growth (anagen), regression (catagen), and rest (telogen) (32). The most active stage of hair follicle development is known as the anagen stage, during which the hair grows quickly and completely forms a hair shaft. Hair follicles typically enter catagen when the hair shaft stops developing, the capacity of cells to proliferate and differentiate starts to wane, cells start to experience apoptosis, and the hair follicles disintegrate quickly. The hair follicle enters telogen after the catagen stage, which is characterized by the lowest biological activity of the hair follicle and the loss of the hair shaft. The development of new hair shafts, the loss of old hair, and changes in dermal papillae structure and morphology are the key changes that take place during the hair cycle (33). Hair follicle stem cells (HFSCs) in the bulge control these transitions of growth cycles (34). HFSCs are mostly in a quiescent state, but they also periodically undergo activities such as cell migration, proliferation, and differentiation (35, 36).
3.1. mTOR signaling in hair follicle cycles and hair follicle stem cells
The regulation mechanism of HFSCs and HF cycles involves the mTOR pathway, the BMP pathway, and the Wnt pathway (37–39). Accumulating evidence suggests mTOR signaling is vitally involved in hair follicle cycles and the stabilization of hair follicle stem cells. Hair cycle beginning was delayed in a pharmacological test in vivo using the particular mTORC1 inhibitor, rapamycin, demonstrating that mTORC1 has a role in anagen entrance. The timing of the anagen beginning phase of the hair cycle may be regulated by mTORC1 (39). The balance between HFSC quiescence and activation during hair regeneration is controlled by mTOR signaling, which is a key regulator in this process. When HFSCs are active at the telogen-to-anagen transition, mTORC1 signaling is also stimulated in these cells. The activation of the HFSC is noticeably delayed and the telogen phase is prolonged in HFs that are unable to react to mTOR signaling. The mTORC1 signaling pathway negatively affects BMP signaling and balances BMP-mediated inhibition of anagen initiation, ultimately promoting HFSC activation and hair growth (40). Interfering with miR-27a causes PIK3R3 to express more, which in turn causes AKT and MTOR to express and be activated more. The proliferation and decreased apoptosis of HFSCs were both boosted by the activation of mTOR signaling (41).
The excessive activation of mTOR was specifically linked to both HF hyperproliferation and HFSC exhaustion. As import signaling pathways, TGF-β adjusts the Akt/mTOR pathways through the Smad2/3 (42). A significant downstream element of the pathway via which Wnt1 stimulation can cause cell proliferation and tissue aging is the mTOR protein. By blocking GSK3, Wnt lessens TSC2’s inhibitory impact on mTOR, enhancing mTOR activity. Wnt1 expression causes HF cells to hyper proliferate and rapidly exhaust their CD34+ stem cells, as shown by the ablation of HF stem cells, which also occurs at the same time as the activation of cell senescence pathways. Rapamycin prevented Wnt1-expressing mice from losing CD34+ stem cells in the bulge area of their hair follicles (43).
A remarkable characteristic of healthy anagen stage hair follicles is the relative immune privilege (IP) that their epithelium displays from the bulge area, the habitat for HF stem cells, downstream to the hair bulb (44). Alopecia areata (AA) and primary cicatricial alopecia (PCA) are the results of an immune attack on the HF when this IP collapses, which is caused by inflammatory infiltrates that collect around the bulge and bulb (45). In the lower follicle ORS and bulb of the hair follicles in the lesional scalps of PCA patients, p-mTOR and p-p70S6K expression rose, whereas p-4EBP1 expression dropped (12). The immunogenicity of differentiated stem cells was markedly reduced by the suppression of mTOR signal pathways (46). These imply that IP collapse may be associated with aberrant mTOR activation in the bulb of the hair follicle. Isolated immune cells are sometimes seen in and around the bulb of an anagen stage HF during the pathological hair development cycle. Dendritic cells, mast cells, NK cells, CD4+T and CD8+T cells, and other inflammatory cells are present in the perifollicular infiltration. Dendritic cells and macrophages are frequently the second kind of cell to enter intra-follicular sites after CD8+T cells. IFN-γ and Substance P, the two known HF IP collapse inducers, are active in both animal and people (44). Inhibition of mTOR signaling reduces the function of many immune cells and may contribute to the maintenance of IP in the hair follicle. mTOR inhibition caused immunosuppression. Damage to the PI3K intracellular signal-transmitting enzymes led to phenotypic abnormalities, a substantial decrease in the number of NK cells in peripheral organs, and decreased cytokine release in other cells (47). Both TGF-β and the mTOR inhibitor rapamycin decreased NK cells’ metabolic activity, proliferation, and abundance of several NK cell receptors, as well as their capacity to carry out cytotoxic action (48). Rapamycin can decrease the proportion and cytotoxic function of CD4+ cytotoxic T lymphocytes (CTLs) in Graves’ orbitopathy (49). When the AKT/mTOR pathway was suppressed, the increases in cytotoxic molecules caused by VEGF-A were considerably decreased. VEGF-A treatment activated the AKT/mTOR pathway in CD4+ CTLs (50). To maintain glucose absorption and glycolysis in CD8+ T cells, mTORC1 activity is essential. The mTORC1-HIF1 pathway, which effectively connects mTORC1 to a multitude of transcriptional processes, is required for effector CTLs to sustain glycolysis and glucose metabolism (51).
Inhibition of mTOR signaling can increase regulatory T cells (Tregs) number, induce immune tolerance, and reduce inflammation, which may help reduce the number of local inflammatory cells around the bulge and bulb and maintain relative IP. As a distinct marker for Tregs, the fork head transcription factor (Foxp3) was discovered. Only T cells expressing Foxp3 were able to prevent transplant rejection in vivo after being exposed to antigen in the presence of TGF-β in vitro, in contrast to their Foxp3-negative counterparts (52). The ratio of Treg to T effector cells (Teff) and the response of mTOR to a variety of microenvironmental stimuli determine whether an individual is tolerant of something or inflammatory. The balance between the quantity of Tregs and Teff is increasingly thought to determine whether the result of an immune response is one of tolerance or inflammation. Activating the GCN2 pathway had no effect on the activation of Foxp3 in naïve CD4+ T cells in the presence of modest doses of TGF-β, while inhibiting the mTOR pathway with rapamycin increased Foxp3 expression. Metabolism and Foxp3 expression are regulated by nutrient and environmental sensing via mTOR. For controlling cell metabolism and Foxp3 expression, mTOR functions as an integrator of signals that come from a variety of cell surface receptors and nutrient-sensing pathways (53).
Inhibition of glutamine metabolism by mTORC2 signaling contributes to progenitor reversibility and reestablishes HFSC niche function (6). Oxidative phosphorylation is activated, and glutamine enters the TCA cycles during the shift from HFSCs to the ORS progenitor state. The HFSC niche is hypoxic, and low pO2 (the partial pressure of oxygen) promotes a stem cell state. Low pO2 inhibits the expression of glutaminase in a way that is reliant on mTORC2-Akt, thereby reducing glutamine hydroxylation to stop the glutamate from entering the Krebs cycles and promoting the return of progenitor cells to the HFSC state, thereby allowing progenitor cells to return to the low oxygen niche, restore stem cell status, and restore bulge to maintain hair follicle stem cells throughout the long run. Loss of mTORC2 impairs niche regeneration of progenitor cells and triggers hair follicle stem cell failure, and for HFSC destiny reversibility and long-term maintenance in vivo, mTORC2 is necessary (Figure 2).
3.2. Factors affecting hair growth by regulating mTOR signaling
Autophagy, heat stress, and Vitamin D are three factors associated with mTOR signaling that play a role in alopecia. Autophagy refers to the starvation-induced degradation of cytoplasmic components under catabolic conditions with limited nutrient supply and is critical for providing substrates for energy production and clearing damaged organelles (54). mTOR signaling is necessary for the activation of hair follicle stem cells and their entry into anagen (39, 40, 43). However, research has also reported that inhibiting mTOR signaling in quiescent telogen hair follicles can activate autophagy and stimulate telogen hair follicles to initiate the anagen phase and accelerate hair regrowth. The metabolites a-ketoglutarate (a-KG) and rapamycin are examples of small compounds that may inhibit mTOR and activate autophagy. Autophagy is also boosted during the anagen phase of the natural hair follicle cycle (55). Activation of autophagy may help to rescue alopecia caused by a shortened anagen phase, a prolonged dormant phase, and/or blocked anagen induction (55). Autophagic activity is inhibited in the HF of AA mice. Compared to untreated AA animals, autophagy induction reduced the severity of AA, but autophagy blocker therapy increased illness (56). Intrafollicular autophagy is crucial for HF growth and plays a fundamental role in HF anagen maintenance (57). Hair matrix keratinocytes of organ-cultured HFs exhibit an active autophagic flux in anagen, but it changes once catagen begins, according to analysis of endogenous lipidated Light Chain 3B and sequestosome 1 proteins as well as ultrastructural visualization of autophagosomes at all stages of the autophagy process. The anagen hair matrix exhibits early catagen and increased keratinocyte death when follicular autophagy is genetically inhibited, suggesting that autophagic flux in this matrix is essential for sustaining this state. Moreover, a hair loss therapy greatly boosts intrafollicular autophagy, which lengthens the anagen phase of human hair development. Intrafollicular autophagy is eminently targetable for therapeutic regulation of human hair growth (57).
The effect of heat stress on phosphorylated mammalian target of rapamycin (p-mTOR) expression occurs in a tissue-specific manner. Heat stress stimulates the mTOR signaling pathway in skeletal muscle, but significantly inhibits the expression of the p-mTOR protein in hair follicle cells and regulates the development of hair follicle cells. By increasing the expression of BMP2 and BMP4, decreasing the mRNA levels of noggin, IGF1, and IGF1R, and increasing the protein level of p-mTOR, heat stress decreased the number of hair follicles (58). Hair follicle mTORC1 signaling can be activated after radiation injury to promote hair follicle regeneration and hair growth (59). By facilitating the activity of the Akt/mTOR signaling pathway and mediating the activation of HFSCs, LGR4 governs the advancement of the hair cycle. The absence of LGR4 prevents the activation of the Akt/mTOR signaling pathway in the HF cycle. In Lgr4 mutant mice, reactivation of Akt signaling reversed its delayed HF cycle, suggesting that Lgr4-regulated HF homeostasis and HFSC activation depend on the activity of the mTOR signaling pathway (60). Micro-current electrical stimulation can increase various growth factors in human hair follicle papilla cells and mouse hair follicles, activate the PI3K/AKT/mTOR pathway, and Wnt/β-catenin pathway significantly, and promote hair growth (61).
Hair follicle damage and degeneration may have a great correlation with mTOR signaling in Vitamin D receptor (VDR) knockout mice. The follicular epithelium of VDR−/− mice is defective in the regression-to-rest morphogenetic stage. VDR−/− hair follicles exhibit DNA damage-inducible transcript 4 (Ddit4) stress compartments with increased Ddit4 during morphogenesis. In VDR−/− mice, abnormalities in Ddit4 signaling impact follicular integrity by disrupting follicular energy balance. In epidermal keratinocytes, the mTOR inhibitor Ddit4 is a direct transcriptional target of the VDR. Ddit4 suppresses mTOR signaling by activating TSC2, a GTP hydrolase activating protein, which subsequently prompts Rheb to inactivate mTOR in a GDP-bound form. In VDR-deficient hair follicles, mTOR signaling is reduced, BMP signaling is increased, the transition from catagen to telogen phase is advanced and prolonged, hair follicle cell differentiation is reduced, and eventually the hair follicle degenerates, which can cause various hair loss diseases (Figure 3) (62).
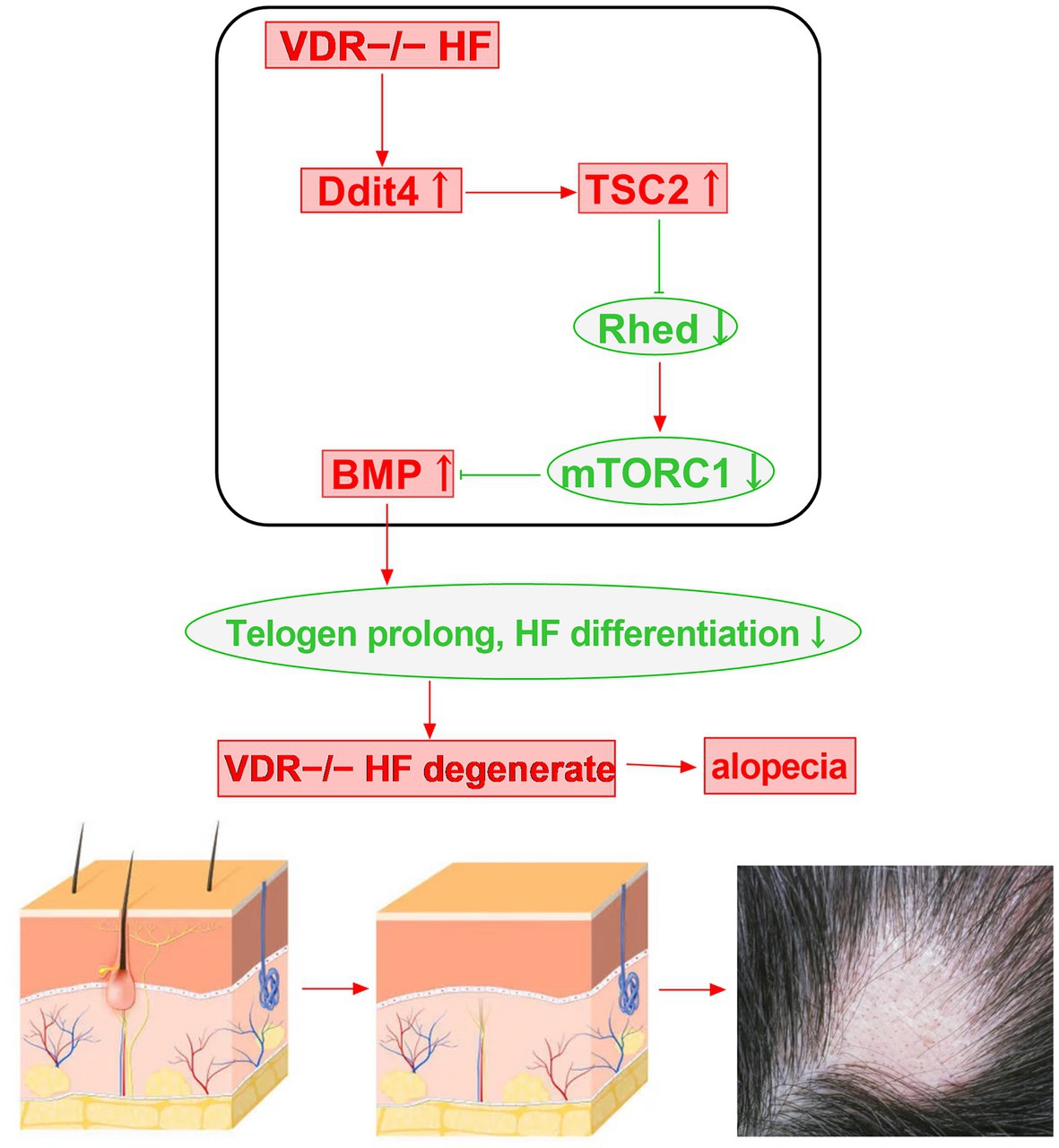
Figure 3. Vitamin D receptor deficiency may induce alopecia through the Ddit4/mTOR signaling pathway.
4. Role of VDR in hair disease
mTOR signaling is an important component of maintaining hair follicle circulation and hair follicle stem cell stability. Once mTOR signaling is out of balance or missing, it can lead to the occurrence of various hair follicle and hair diseases. In addition, 1,25D3 affects mTOR function in the cytoplasm in multiple ways (63), The vitamin D receptor and Ddit4 are important regulators of mTOR signaling and play crucial roles in hair loss diseases.
Alopecia areata is a frequent kind of hair loss that is characterized by well-defined, skin-colored, round to oval, non-scarring patches. Many studies have reported that serum 1,25D3 levels in patients with alopecia areata are lower than those in healthy subjects, some of which are accompanied by compensatory increases in parathyroid hormone levels, and that serum 1,25D3 levels less than 30 ng/ml are associated with the occurrence of alopecia areata (64, 65). In individuals with alopecia areata, vitamin D insufficiency is adversely correlated with the severity and duration of the condition. The tissue and serum VDR levels of patients with alopecia areata were significantly lower than those in the control group. In comparison to healthy skin, alopecia lesions had considerably reduced levels of VDR expression in the hair follicles and epidermis, and the degree of alopecia areata was significantly negatively correlated with VDR in tissues (66). However, another study revealed that reduced VDR expression in alopecia areata was inversely correlated with hair follicle histological inflammation but not with serum vitamin D levels, disease severity, pattern, or duration (10). By modulating mTOR signaling, vitamin D and VDR deficiency may exacerbate alopecia areata and cause severe hair loss. Downregulation of VDR in hair follicles is related to lower hair development.
The most prevalent kind of progressive hair loss is androgenetic alopecia (AGA), which is also known as androgen-related progressive hair thinning. AGA is further classified into male-and female-pattern hair loss. Many studies have confirmed that the serum vitamin D level of AGA patients is lower than that of the control group and that vitamin D deficiency exists (67, 68). AGA patients with high levels of dihydrotestosterone are deficient in all vitamins and trace minerals, including vitamin D, zinc, copper, magnesium, selenium, and vitamin B12 (69). Serum vitamin D levels can be used as an index to judge the incidence and severity of AGA. In addition, the levels of VDR in the scalp and blood of AGA patients were also significantly lower than normal (70). Hair loss occurs in patients with an inherited VDR deficiency, and VDR knockout mice are unable to initiate a new hair cycle. Many studies have found that the serum 1,25D3 concentration in female pattern hair loss patients is lower than that in the control group (71, 72). The average serum 1,25D3 concentration has nothing to do with the course of disease or age, but there is a certain correlation with the severity of the disease. Some studies believe that women with a family history of female pattern hair loss and vitamin D lacking or insufficiency are more likely to suffer from female pattern hair loss, but some studies do not support this view (71). The serum and tissue VDR levels of female patients were higher than those of male patients. Compared with healthy controls, the serum and tissue VDR concentrations of female pattern hair loss patients were significantly lower, but they were not related to the severity of the disease (70). In the study of the correlation between VDR gene polymorphisms (Cdx-1 and Taq-1) and female pattern hair loss, it was found that Taq-1 and Cdx-1 may be risk factors and play a role in the duration of female pattern hair loss (73). Vitamin D and VDR are so closely related to AGA that they may regulate the process of AGA through the Ddit4/mTOR pathway.
5. Roles of mTOR signaling in hair disease
Alopecia areata (AA) and primary cicatricial alopecia (PCA) are the results of an immune attack on the HF when this IP collapses. The relationship between the PI3K/Akt/mTOR pathways and the molecular markers that are specific to AA, such as NK cells, CD4+ and CD8+, MHC classes I and II cells, and migration inhibitory factor (MIF), is indicative of the function that these pathways play in the disease. Immunological mediators such macrophages, Langerhans’ cells, and cytokines, as well as CD4+, CD8+, and other immunological mediators, have all been linked to the onset and progression of AA (74–76). CD8+ T cells, NKG2D+ cells, IFN-γ, and IL-15 have a significant role in the etiology of AA. Since both IFN-γ and IL-15 signal via the Janus kinase (JAK) pathway, JAK inhibitors are promising drugs for managing AA in vivo (77). JAK1 and JAK3 selective inhibitors effectively stimulated hair growth and reduced AA-related inflammation. But a number of JAK2-selective inhibitors failed to reinstate hair growth. The effectiveness of this therapy in reversing autoimmune illnesses like AA may be explained mechanistically by the targeted production of T cell exhaustion utilizing a JAK1/3 inhibitor (78, 79). By promoting the growth of alopecic T cells, exogenous IL-7 hastened the start of AA. In contrast, IL-7 inhibition prevented AA from progressing and reversed early AA in C3H/HeJ mice. The overall number of most T cell subsets was significantly decreased by IL-7R blockage, while regulatory T cells (Tregs) were somewhat spared (80). It was confirmed that the AA risk genes IL2RA, STX17, and TNXB are regulated by miR-30b at particular sites. This study suggests that microRNAs have a role in the development of AA (81). CXCR3-blocking antibodies can stop the development of AA in the graft model and prevent the buildup of CD8+T cells in the skin (82). The care of HF diseases linked to redox imbalance, such as HF graying and HF ageing, androgenetic alopecia and alopecia areata, may benefit from small molecule NRF2 activators. The reduction in early catagen and hair growth inhibition brought on by oxidative stress was decreased by Nrf2 pre-activation, which also reversed the reduction in hair matrix proliferation brought on by reactive oxygen species (83, 84). γδT-cells can cause early catagen, dystrophy, and HF immune privilege breakdown, which become the main reasons for AA (Table 1) (85, 86).
As a type of PCA, Lichen planopilaris (LPP) includes the classical form, frontal fibrosing alopecia (FFA), and Graham-Little syndrome. LPP and FFA can lead to permanent alopecia, which is considered to be related to hair follicle stem cell damage and the mTOR signaling pathway. The mTOR signaling pathway protein is found in all regions of healthy hair follicles, but it has abnormal expression in those of lichen planopilaris and frontal fibrosing alopecia patients. The expression of p-mTOR decreased in the interfollicular epidermis of the patients’ skin lesions, and increased in the lower part of the hair follicle in the ORS and the hair follicle bulb (12). It has been demonstrated that JAK inhibitors are useful for treating LPP (87). In PCA lesions, immune-histological investigations found that the number of mast cells (MC) was elevated. Gene expression analysis identified common PCA-related pathways, particularly those strongly connected with MC (88). Peroxisome proliferator-activated receptor (PPAR)-γ-mediated signaling plays an important role in the LPP/FFA associated with IP collapse (89). Due to its ability to reduce T-lymphocyte activation, proliferation, and antibody generation, sirolimus is another possible therapy for FFA (90).
AGA is distinguished by androgen-related, progressive hair loss in a specific pattern. By reducing the activity of the enzymes 17, 20-lyase of cytochrome P450c17, and 3-hydroxysteroid dehydrogenase, PPAR-γ signaling impacts androgen production, which lowers androgen levels. These results warrant investigating PPAR-γ antagonist therapy for the management of AGA (91). Small molecule NRF2 activators may be helpful in the treatment of HF illnesses including androgenetic alopecia and alopecia areata that are connected to redox imbalance (84). Dihydrotestosterone (DHT) interacts with the dermal papilla cells (DPCs), which line the base of the hair follicle, to cause AGA. The suppression of the hair development cycle in immortalized DPCs may be lessened by DHT’s stimulation of mTOR (92). By triggering the TGF-β signaling pathway, Serenoa repens extracts encourage hair growth and repair in AGA mice models (93). Regenerative stem cell treatment for androgenic alopecia may occur through the Wnt/β-catenin pathway (94).
The mTOR pathway is crucial for treating hair disorders and issues. In rat dermal papilla cells, limonin can promote anagen signaling by activating autophagy by targeting the Wnt/β-catenin and/or PI3K/AKT pathways, suggesting a possible nutrient for hair loss therapy (95). The effect of Eclipta prostrata on promoting hair follicle and hair growth is achieved by increasing the expression of FGF-7 and FGF-5 in human dermal papilla cells and activating the mTOR signaling pathway (96). The molecular mechanism of adenosine promoting hair growth includes regulating the activity of Gsk3β to activate adenosine receptors to mimic the Wnt/β-catenin signaling pathway, Nevertheless, PKA and mTOR activity are necessary for the route to be activated. The Wnt/β-catenin pathway is activated by adenosine mostly through the Gas/cAMP/PKA/mTOR cascade (97). Myristoleic acid induces autophagosome formation by reducing the levels of p-mTOR, Atg7, and LC3II, promoting anagen phase signaling through autophagy and cell cycle progression, which may be useful for alleviating hair loss (98). kin barrier integrity and T cell homeostasis are compromised by RNA stress brought on by SKIV2L loss, which promotes mTORC1 signaling in keratinocytes and T cells. Epidermal hyperplasia and abnormalities in hair formation are caused by the skin-specific deletion of Skiv2l, including skin lesions and hair fragility with nodular alopecia in the hair-hepatic syndrome. Using the mTOR inhibitor rapamycin to treat skiv2l-deficient mice alleviated the cutaneous symptoms that were present (99). Two-pore channels are a class of non-selective cation channels in the lysosome system. ATP can block the activation of two-pore channels (TPCs) through mTOR, resulting in the loss of TPCs, which can affect angiogenesis, autophagy, and human hair pigmentation (100). FLCN is required for the recruitment of mTORC1 to lysosomes upon amino acid stimulation. FLCN gene deficiency can lead to Birt-Hogg-Dubé syndrome, including fibrofolliculoma, lung disease, and kidney disease. Fibrofolliculoma is related to FLCN and mTOR signaling (101). Cronkhite-Canada syndrome can induce alopecia, which may be benefited by the incorporation of sirolimus (102).
6. Summary and remaining problems
The transition of hair follicles between different phases of the hair cycle is regulated by the balance between growth-stimulatory and inhibitory signals, mainly through the mTOR pathway. mTORC1 and mTORC2 exhibit corresponding functions in controlling hair follicle cycles and maintaining the HFSC pool. The activation of mTORC1 is necessary for the activation and entry of hair follicle stem cells into anagen, while the activation of mTORC2 is very important for the maintenance of HFSC and helps the progenitor cells return to the quiescent HFSC state. The mTORC1 signaling pathway balances BMP-mediated inhibition of anagen initiation, ultimately promoting HFSC activation and hair growth. Inhibition of mTOR signaling in quiescent telogen hair follicles can activate autophagy and stimulate telogen hair follicles to initiate the anagen phase and accelerate hair regrowth. VDR−/− hair follicles can up-regulate Ddit4 signaling and down-regulate mTORC1 signaling, then prolong the transition from catagen to telogen, reduce hair follicle cell differentiation, and eventually induce hair follicle degeneration and hair loss. mTOR signaling may be a critical cog signaling in VDR deficiency-mediated hair follicle damage and degeneration and related alopecia disorders such as alopecia areata, androgenetic alopecia, lichen planopilaris, frontal fibrosing alopecia, and even hair repigmentation. The precise interaction between mTOR and other regulating pathways such as BMP and Wnt in hair follicles requires further study. Inhibition of mTOR signaling in quiescent telogen hair follicles can initiate hair growth, but mTOR signaling is vital in anagen and catagen hair follicles for hair follicle cycles and HFSC pool maintenance. How to rationally utilize mTOR signaling to promote hair growth at different growth stages seems worthy of further study.
The etiology of alopecia areata and primary immune alopecia is related to the destruction of immune privilege in the hair follicle bulb and bulge, which leads to the up-regulation of local MHC classes I and II receptors and the accumulation of CD8+ T cells, NK cells, and other immune cells, eventually leading to the occurrence of alopecia. Inhibition of mTOR can induce immunosuppression and immune tolerance, reduce the immunogenicity of hair follicle stem cells, reduce the number of local immune cells, and reduce the function of immune cells, which may help to maintain immune privilege in hair follicles and relieve the clinical symptoms of AA and PCA etiologically. As import signaling pathways of mTOR, Wnt and TGF-β pathways play a critical role in AGA. Therefore, mTOR targeting would be an improvement to the current treatment regimen.
Author contributions
WT created the plot of the manuscript and first draft with the help of Y-WC, MS, and QL. H-GZ critically edited and revised the manuscript. All authors have read and agreed to the published version of the manuscript.
Funding
This work was funded by the National Natural Science Foundation of China for H-GZ (no. 82173440).
Conflict of interest
The authors declare that the research was conducted in the absence of any commercial or financial relationships that could be construed as a potential conflict of interest.
Publisher’s note
All claims expressed in this article are solely those of the authors and do not necessarily represent those of their affiliated organizations, or those of the publisher, the editors and the reviewers. Any product that may be evaluated in this article, or claim that may be made by its manufacturer, is not guaranteed or endorsed by the publisher.
References
1. Saxton, RA, and Sabatini, DM. mTOR signaling in growth, metabolism, and disease. Cells. (2017) 169:361–71. doi: 10.1016/j.cell.2017.03.035
2. Yu, JJ, and Goncharova, EA. mTOR Signaling network in cell biology and human disease. Int J Mol Sci. (2022) 23:85–87. doi: 10.3390/ijms232416142
3. Nasr, AB, Ponnala, D, Sagurthi, SR, Kattamuri, RK, Marri, VK, Gudala, S, et al. Molecular docking studies of Fkbp12-Mtor inhibitors using binding predictions. Bioinformation. (2015) 11:307–15. doi: 10.6026/97320630011307
4. Suzuki, T, Cheret, J, Scala, FD, Akhundlu, A, Gherardini, J, Demetrius, DL, et al. Mtorc1 activity negatively regulates human hair follicle growth and pigmentation. EMBO Rep. (2023) 24:e56574. doi: 10.15252/embr.202256574
5. Wang, J, Cui, B, Chen, Z, and Ding, X. The regulation of skin homeostasis, repair and the pathogenesis of skin diseases by spatiotemporal activation of epidermal mTOR Signaling. Front Cell Dev Biol. (2022) 10:950973. doi: 10.3389/fcell.2022.950973
6. Kim, CS, Ding, X, Allmeroth, K, Biggs, LC, Kolenc, OI, L'Hoest, N, et al. Glutamine metabolism controls stem cell fate reversibility and long-term maintenance in the hair follicle. Cell Metab. (2020) 32:629–642.e8. doi: 10.1016/j.cmet.2020.08.011
7. Ding, X, Willenborg, S, Bloch, W, Wickstrom, SA, Wagle, P, Brodesser, S, et al. Epidermal mammalian target of rapamycin complex 2 controls lipid synthesis and filaggrin processing in epidermal barrier formation. J Allergy Clin Immunol. (2020) 145:283–300.e8. doi: 10.1016/j.jaci.2019.07.033
8. Ding, X, Bloch, W, Iden, S, Ruegg, MA, Hall, MN, Leptin, M, et al. Mtorc1 and Mtorc2 regulate skin morphogenesis and epidermal barrier formation. Nat Commun. (2016) 7:13226. doi: 10.1038/ncomms13226
9. Zubair, Z, Kantamaneni, K, Jalla, K, Renzu, M, Jena, R, Jain, R, et al. Prevalence of low serum vitamin D levels in patients presenting with androgenetic alopecia: a review. Cureus. (2021) 13:e20431. doi: 10.7759/cureus.20431
10. Daroach, M, Narang, T, Saikia, UN, Sachdeva, N, and Sendhil, KM. Correlation of vitamin D and vitamin D receptor expression in patients with alopecia areata: a clinical paradigm. Int J Dermatol. (2018) 57:217–22. doi: 10.1111/ijd.13851
11. Sardina, LA, Rubin, B, Jour, G, Piliang, M, Elston, C, and Bergfeld, WF. Differential expression of Phospho-S6 in hair follicle Tumors: evidence of mammalian target of rapamycin pathway activation. J Cutan Pathol. (2019) 46:256–60. doi: 10.1111/cup.13411
12. Dicle, O, Celik-Ozenci, C, Sahin, P, Pfannes, EKB, Vogt, A, Altinok, BN, et al. Differential expression of mTOR Signaling pathway proteins in lichen Planopilaris and frontal fibrosing alopecia. Acta Histochem. (2018) 120:837–45. doi: 10.1016/j.acthis.2018.09.007
13. Dobrenel, T, Caldana, C, Hanson, J, Robaglia, C, Vincentz, M, Veit, B, et al. Tor Signaling and nutrient sensing. Annu Rev Plant Biol. (2016) 67:261–85. doi: 10.1146/annurev-arplant-043014-114648
14. Benjamin, D, and Hall, MN. Tsc on the peroxisome controls Mtorc1. Nat Cell Biol. (2013) 15:1135–6. doi: 10.1038/ncb2849
15. Carroll, B, Maetzel, D, Maddocks, OD, Otten, G, Ratcliff, M, Smith, GR, et al. Control of Tsc2-Rheb Signaling Axis by arginine regulates Mtorc1 activity. eLife. (2016) 5:5. doi: 10.7554/eLife.11058
16. Jewell, JL, Russell, RC, and Guan, KL. Amino acid signalling upstream of mTOR. Nat Rev Mol Cell Biol. (2013) 14:133–9. doi: 10.1038/nrm3522
17. Cope, CL, Gilley, R, Balmanno, K, Sale, MJ, Howarth, KD, Hampson, M, et al. Adaptation to mTOR kinase inhibitors by amplification of Eif4e to maintain cap-dependent translation. J Cell Sci. (2014) 127:788–800. doi: 10.1242/jcs.137588
18. Le Hir, H, and Seraphin, B. Ejcs at the heart of translational control. Cells. (2008) 133:213–6. doi: 10.1016/j.cell.2008.04.002
19. Mao, Z, and Zhang, W. Role of mTOR in glucose and lipid metabolism. Int J Mol Sci. (2018) 19:126–127. doi: 10.3390/ijms19072043
20. Wang, Y, and Zhang, H. Regulation of autophagy by mTOR Signaling pathway. Adv Exp Med Biol. (2019) 1206:67–83. doi: 10.1007/978-981-15-0602-4_3
21. Morita, M, Gravel, SP, Hulea, L, Larsson, O, Pollak, M, St-Pierre, J, et al. mTOR coordinates protein synthesis, mitochondrial activity and proliferation. Cell Cycle. (2015) 14:473–80. doi: 10.4161/15384101.2014.991572
22. Deleyto-Seldas, N, and Efeyan, A. The mTOR-autophagy Axis and the control of metabolism. Front Cell Dev Biol. (2021) 9:655731. doi: 10.3389/fcell.2021.655731
23. Zhuang, L, Jin, G, Hu, X, Yang, Q, Pei, X, and Zhao, W. Tspan8 alleviates high glucose-induced apoptosis and autophagy via targeting Mtorc2. Cell Biol Int. (2022) 46:1693–703. doi: 10.1002/cbin.11870
24. Orduna-Castillo, LB, Del-Rio-Robles, JE, Garcia-Jimenez, I, Zavala-Barrera, C, Beltran-Navarro, YM, Hidalgo-Moyle, JJ, et al. Calcium sensing receptor stimulates breast Cancer cell migration via the Gbetagamma-Akt-Mtorc2 Signaling pathway. J Cell Commun Signal. (2022) 16:239–52. doi: 10.1007/s12079-021-00662-y
25. Lang, F, and Pearce, D. Regulation of the epithelial Na+ channel by the Mtorc2/Sgk1 pathway. Nephrol Dial Transplant. (2016) 31:200–5. doi: 10.1093/ndt/gfv270
26. Angliker, N, and Ruegg, MA. In vivo evidence for Mtorc2-mediated actin cytoskeleton rearrangement in neurons. BioArchitecture. (2013) 3:113–8. doi: 10.4161/bioa.26497
27. Baffi, TR, Lorden, G, Wozniak, JM, Feichtner, A, Yeung, W, Kornev, AP, et al. Mtorc2 controls the activity of Pkc and Akt by phosphorylating a conserved Tor interaction motif. Sci Signal. (2021) 14:140–141. doi: 10.1126/scisignal.abe4509
28. Yang, G, Murashige, DS, Humphrey, SJ, and James, DE. A positive feedback loop between Akt and Mtorc2 via Sin1 phosphorylation. Cell Rep. (2015) 12:937–43. doi: 10.1016/j.celrep.2015.07.016
29. Masui, K, Tanaka, K, Akhavan, D, Babic, I, Gini, B, Matsutani, T, et al. mTOR complex 2 controls glycolytic metabolism in glioblastoma through Foxo acetylation and upregulation of C-Myc. Cell Metab. (2013) 18:726–39. doi: 10.1016/j.cmet.2013.09.013
30. Lamming, DW, Ye, L, Katajisto, P, Goncalves, MD, Saitoh, M, Stevens, DM, et al. Rapamycin-induced insulin resistance is mediated by Mtorc2 loss and uncoupled from longevity. Science. (2012) 335:1638–43. doi: 10.1126/science.1215135
31. Sarbassov, DD, Ali, SM, Sengupta, S, Sheen, JH, Hsu, PP, Bagley, AF, et al. Prolonged rapamycin treatment inhibits Mtorc2 assembly and Akt/Pkb. Mol Cell. (2006) 22:159–68. doi: 10.1016/j.molcel.2006.03.029
32. Shin, JM, Ko, JW, Choi, CW, Lee, Y, Seo, YJ, Lee, JH, et al. Deficiency of Crif1 in hair follicle stem cells retards hair growth cycle in adult mice. PLoS One. (2020) 15:e0232206. doi: 10.1371/journal.pone.0232206
33. Lin, X, Zhu, L, and He, J. Morphogenesis, growth cycle and molecular regulation of hair follicles. Front Cell Dev Biol. (2022) 10:899095. doi: 10.3389/fcell.2022.899095
34. Hsu, YC, Li, L, and Fuchs, E. Emerging interactions between skin stem cells and their niches. Nat Med. (2014) 20:847–56. doi: 10.1038/nm.3643
35. Mesler, AL, Veniaminova, NA, Lull, MV, and Wong, SY. Hair follicle terminal differentiation is orchestrated by distinct early and late matrix progenitors. Cell Rep. (2017) 19:809–21. doi: 10.1016/j.celrep.2017.03.077
36. Tumbar, T, Guasch, G, Greco, V, Blanpain, C, Lowry, WE, Rendl, M, et al. Defining the epithelial stem cell niche in skin. Science. (2004) 303:359–63. doi: 10.1126/science.1092436
37. Li, KN, and Tumbar, T. Hair follicle stem cells as a skin-organizing signaling center during adult homeostasis. EMBO J. (2021) 40:e107135. doi: 10.15252/embj.2020107135
38. Yi, R. Concise review: mechanisms of quiescent hair follicle stem cell regulation. Stem Cells. (2017) 35:2323–30. doi: 10.1002/stem.2696
39. Kellenberger, AJ, and Tauchi, M. Mammalian target of rapamycin complex 1 (Mtorc1) may modulate the timing of anagen entry in mouse hair follicles. Exp Dermatol. (2013) 22:77–80. doi: 10.1111/exd.12062
40. Deng, Z, Lei, X, Zhang, X, Zhang, H, Liu, S, Chen, Q, et al. mTOR Signaling promotes stem cell activation via counterbalancing bmp-mediated suppression during hair regeneration. J Mol Cell Biol. (2015) 7:62–72. doi: 10.1093/jmcb/mjv005
41. Yu, M, Li, L, Liu, M, Wang, L, Gao, X, Zhou, L, et al. Mir-27a targeting Pik3r3 regulates the proliferation and apoptosis of sheep hair follicle stem cells. Animals (Basel). (2022) 13:194–198. doi: 10.3390/ani13010141
42. Zhao, Z, Zhang, Y, Zhang, C, Zhang, J, Luo, X, Qiu, Q, et al. Tgf-Beta promotes pericyte-myofibroblast transition in subretinal fibrosis through the Smad2/3 and Akt/mTOR pathways. Exp Mol Med. (2022) 54:673–84. doi: 10.1038/s12276-022-00778-0
43. Castilho, RM, Squarize, CH, Chodosh, LA, Williams, BO, and Gutkind, JS. mTOR mediates Wnt-induced epidermal stem cell exhaustion and aging. Cell Stem Cell. (2009) 5:279–89. doi: 10.1016/j.stem.2009.06.017
44. Bertolini, M, McElwee, K, Gilhar, A, Bulfone-Paus, S, and Paus, R. Hair follicle immune privilege and its collapse in alopecia areata. Exp Dermatol. (2020) 29:703–25. doi: 10.1111/exd.14155
45. Anzai, A, Wang, EHC, Lee, EY, Aoki, V, and Christiano, AM. Pathomechanisms of immune-mediated alopecia. Int Immunol. (2019) 31:439–47. doi: 10.1093/intimm/dxz039
46. Wu, CE, Yu, CW, Chang, KW, Chou, WH, Lu, CY, Ghelfi, E, et al. Comparative global immune-related gene profiling of somatic cells, human pluripotent stem cells and their derivatives: implication for human lymphocyte proliferation. Exp Mol Med. (2017) 49:e376. doi: 10.1038/emm.2017.134
47. Mace, EM. Phosphoinositide-3-kinase Signaling in human natural killer cells: new insights from primary immunodeficiency. Front Immunol. (2018) 9:445. doi: 10.3389/fimmu.2018.00445
48. Viel, S, Marcais, A, Guimaraes, FS, Loftus, R, Rabilloud, J, Grau, M, et al. Tgf-Beta inhibits the activation and functions of Nk cells by repressing the mTOR pathway. Sci Signal. (2016) 9:ra19. doi: 10.1126/scisignal.aad1884
49. Zhang, M, Chong, KK, Chen, ZY, Guo, H, Liu, YF, Kang, YY, et al. Rapamycin improves Graves' orbitopathy by suppressing Cd4+ cytotoxic T lymphocytes. JCI. Insight. (2023) 8:334–335. doi: 10.1172/jci.insight.160377
50. Chen, Z, Zhang, M, Liu, Y, Chen, Z, Wang, L, Wang, W, et al. Vegf-a enhances the cytotoxic function of Cd4(+) cytotoxic T cells via the Vegf-receptor 1/Vegf-receptor 2/Akt/Mtor pathway. J Transl Med. (2023) 21:74. doi: 10.1186/s12967-023-03926-w
51. Finlay, DK, Rosenzweig, E, Sinclair, LV, Feijoo-Carnero, C, Hukelmann, JL, Rolf, J, et al. Pdk1 regulation of mTOR and hypoxia-inducible factor 1 integrate metabolism and migration of Cd8+ T cells. J Exp Med. (2012) 209:2441–53. doi: 10.1084/jem.20112607
52. Regateiro, FS, Chen, Y, Kendal, AR, Hilbrands, R, Adams, E, Cobbold, SP, et al. Foxp3 expression is required for the induction of therapeutic tissue tolerance. J Immunol. (2012) 189:3947–56. doi: 10.4049/jimmunol.1200449
53. Waldmann, H, Howie, D, and Cobbold, S. Induction of immunological tolerance as a therapeutic procedure. Microbiol Spectr. (2016) 4:352–365. doi: 10.1128/microbiolspec.MCHD-0019-2015
54. Deretic, V, Saitoh, T, and Akira, S. Autophagy in infection, inflammation and immunity. Nat Rev Immunol. (2013) 13:722–37. doi: 10.1038/nri3532
55. Chai, M, Jiang, M, Vergnes, L, Fu, X, de Barros, SC, Doan, NB, et al. Stimulation of hair growth by small molecules that activate autophagy. Cell Rep. (2019) 27:3413–3421.e3. doi: 10.1016/j.celrep.2019.05.070
56. Gund, R, and Christiano, AM. Impaired autophagy promotes hair loss in the C3h/Hej mouse model of alopecia areata. Autophagy. (2023) 19:296–305. doi: 10.1080/15548627.2022.2074104
57. Parodi, C, Hardman, JA, Allavena, G, Marotta, R, Catelani, T, Bertolini, M, et al. Autophagy is essential for maintaining the growth of a human (Mini-)organ: evidence from scalp hair follicle organ culture. PLoS Biol. (2018) 16:e2002864. doi: 10.1371/journal.pbio.2002864
58. Sun, H, Zhang, Y, Bai, L, Wang, Y, Yang, L, Su, W, et al. Heat stress decreased hair follicle population in rex rabbits. J Anim Physiol Anim Nutr (Berl). (2019) 103:501–8. doi: 10.1111/jpn.13044
59. Wang, WH, Chien, TH, Fan, SM, Huang, WY, Lai, SF, Wu, JT, et al. Activation of Mtorc1 signaling is required for timely hair follicle regeneration from radiation injury. Radiat Res. (2017) 188:681–9. doi: 10.1667/RR14830.1
60. Ren, X, Xia, W, Xu, P, Shen, H, Dai, X, Liu, M, et al. Lgr4 deletion delays the hair cycle and inhibits the activation of hair follicle stem cells. J Invest Dermatol. (2020) 140:1706–1712.e4. doi: 10.1016/j.jid.2019.12.034
61. Hwang, D, Lee, H, Lee, J, Lee, M, Cho, S, Kim, T, et al. Micro-current stimulation has potential effects of hair growth-promotion on human hair follicle-derived papilla cells and animal model. Int J Mol Sci. (2021) 22:436–439. doi: 10.3390/ijms22094361
62. Zhao, H, Rieger, S, Abe, K, Hewison, M, and Lisse, TS. DNA damage-inducible transcript 4 is an innate surveillant of hair follicular stress in vitamin D receptor knockout mice and a regulator of wound re-epithelialization. Int J Mol Sci. (2016) 17:440–456. doi: 10.3390/ijms17121984
63. Quigley, M, Rieger, S, Capobianco, E, Wang, Z, Zhao, H, Hewison, M, et al. Vitamin D modulation of mitochondrial oxidative metabolism and Mtor enforces stress adaptations and anticancer responses. JBMR Plus. (2022) 6:e10572. doi: 10.1002/jbm4.10572
64. Mahamid, M, Abu-Elhija, O, Samamra, M, Mahamid, A, and Nseir, W. Association between vitamin D levels and alopecia areata. Isr Med Assoc J. (2014) 16:367–70.
65. Aksu Cerman, A, Sarikaya Solak, S, and Kivanc, AI. Vitamin D deficiency in alopecia areata. Br J Dermatol. (2014) 170:1299–304. doi: 10.1111/bjd.12980
66. Gerkowicz, A, Chyl-Surdacka, K, Krasowska, D, and Chodorowska, G. The role of vitamin D in non-scarring alopecia. Int J Mol Sci. (2017) 18:502–509. doi: 10.3390/ijms18122653
67. Zhao, J, Sheng, Y, Dai, C, Qi, S, Hu, R, Rui, W, et al. Serum 25 hydroxyvitamin D levels in alopecia areata, female pattern hair loss, and male androgenetic alopecia in a Chinese population. J Cosmet Dermatol. (2020) 19:3115–21. doi: 10.1111/jocd.13396
68. Conic, RRZ, Piliang, M, Bergfeld, W, and Atanaskova-Mesinkovska, N. Vitamin D status in scarring and nonscarring alopecia. J Am Acad Dermatol. (2021) 85:478–80. doi: 10.1016/j.jaad.2018.04.032
69. Kondrakhina, IN, Verbenko, DA, Zatevalov, AM, Gatiatulina, ER, Nikonorov, AA, Deryabin, DG, et al. A cross-sectional study of plasma trace elements and vitamins content in androgenetic alopecia in men. Biol Trace Elem Res. (2021) 199:3232–41. doi: 10.1007/s12011-020-02468-2
70. Fawzi, MM, Mahmoud, SB, Ahmed, SF, and Shaker, OG. Assessment of vitamin D receptors in alopecia areata and androgenetic alopecia. J Cosmet Dermatol. (2016) 15:318–23. doi: 10.1111/jocd.12224
71. Banihashemi, M, Nahidi, Y, Meibodi, NT, Jarahi, L, and Dolatkhah, M. Serum vitamin D3 level in patients with female pattern hair loss. Int J Trichol. (2016) 8:116–20. doi: 10.4103/0974-7753.188965
72. Rasheed, H, Mahgoub, D, Hegazy, R, El-Komy, M, Abdel Hay, R, Hamid, MA, et al. Serum ferritin and vitamin D in female hair loss: do they play a role? Skin Pharmacol Physiol. (2013) 26:101–7. doi: 10.1159/000346698
73. Seleit, I, Bakry, OA, Badr, E, and Mabrouk, M. Vitamin D receptor gene polymorphisms Taq-1 and Cdx-1 in female pattern hair loss. Indian J Dermatol. (2020) 65:259–64. doi: 10.4103/ijd.IJD_482_18
74. Zeberkiewicz, M, Rudnicka, L, and Malejczyk, J. Immunology of alopecia areata. Cent Eur J Immunol. (2020) 45:325–33. doi: 10.5114/ceji.2020.101264
75. Juarez-Rendon, KJ, Rivera Sanchez, G, Reyes-Lopez, MA, Garcia-Ortiz, JE, Bocanegra-Garcia, V, Guardiola-Avila, I, et al. Alopecia areata. Current situation and perspectives. Arch Argent Pediatr. (2017) 115:e404–11. doi: 10.5546/aap.2017.eng.e404
76. Villasante Fricke, AC, and Miteva, M. Epidemiology and burden of alopecia areata: a systematic review. Clin Cosmet Investig Dermatol. (2015) 8:397–403. doi: 10.2147/CCID.S53985
77. Gilhar, A, Schrum, AG, Etzioni, A, Waldmann, H, and Paus, R. Alopecia areata: animal models illuminate autoimmune pathogenesis and novel immunotherapeutic strategies. Autoimmun Rev. (2016) 15:726–35. doi: 10.1016/j.autrev.2016.03.008
78. Dai, Z, Sezin, T, Chang, Y, Lee, EY, Wang, EHC, and Christiano, AM. Induction of T cell exhaustion by Jak1/3 inhibition in the treatment of alopecia areata. Front Immunol. (2022) 13:955038. doi: 10.3389/fimmu.2022.955038
79. Dai, Z, Chen, J, Chang, Y, and Christiano, AM. Selective inhibition of Jak3 Signaling is sufficient to reverse alopecia areata. JCI Insight. (2021) 6:627–630. doi: 10.1172/jci.insight.142205
80. Dai, Z, Wang, EHC, Petukhova, L, Chang, Y, Lee, EY, and Christiano, AM. Blockade of il-7 Signaling suppresses inflammatory responses and reverses alopecia areata in C3h/Hej mice. Sci Adv. (2021) 7:664–669. doi: 10.1126/sciadv.abd1866
81. Tafazzoli, A, Forstner, AJ, Broadley, D, Hofmann, A, Redler, S, Petukhova, L, et al. Genome-wide microRNA analysis implicates Mir-30b/D in the etiology of alopecia areata. J Invest Dermatol. (2018) 138:549–56. doi: 10.1016/j.jid.2017.09.046
82. Dai, Z, Xing, L, Cerise, J, Wang, EH, Jabbari, A, de Jong, A, et al. Cxcr3 blockade inhibits T cell migration into the skin and prevents development of alopecia areata. J Immunol. (2016) 197:1089–99. doi: 10.4049/jimmunol.1501798
83. Haslam, IS, Jadkauskaite, L, Szabo, IL, Staege, S, Hesebeck-Brinckmann, J, Jenkins, G, et al. Oxidative damage control in a human (Mini-) organ: Nrf2 activation protects against oxidative stress-induced hair growth inhibition. J Invest Dermatol. (2017) 137:295–304. doi: 10.1016/j.jid.2016.08.035
84. Jadkauskaite, L, Coulombe, PA, Schafer, M, Dinkova-Kostova, AT, Paus, R, and Haslam, IS. Oxidative stress Management in the Hair Follicle: could targeting Nrf2 counter age-related hair disorders and beyond? BioEssays. (2017) 39:674–676. doi: 10.1002/bies.201700029
85. Uchida, Y, Gherardini, J, Pappelbaum, K, Cheret, J, Schulte-Mecklenbeck, A, Gross, CC, et al. Resident human dermal Gammadeltat-cells operate as stress-sentinels: lessons from the hair follicle. J Autoimmun. (2021) 124:102711. doi: 10.1016/j.jaut.2021.102711
86. Uchida, Y, Gherardini, J, Schulte-Mecklenbeck, A, Alam, M, Cheret, J, Rossi, A, et al. Pro-inflammatory Vdelta1(+)T-cells infiltrates are present in and around the hair bulbs of non-lesional and lesional alopecia areata hair follicles. J Dermatol Sci. (2020) 100:129–38. doi: 10.1016/j.jdermsci.2020.09.001
87. Yang, CC, Khanna, T, Sallee, B, Christiano, AM, and Bordone, LA. Tofacitinib for the treatment of lichen planopilaris: a case series. Dermatol Ther. (2018) 31:e12656. doi: 10.1111/dth.12656
88. Wang, EHC, Monga, I, Sallee, BN, Chen, JC, Abdelaziz, AR, Perez-Lorenzo, R, et al. Primary cicatricial Alopecias are characterized by dysregulation of shared gene expression pathways. PNAS Nexus. (2022) 1:719–722. doi: 10.1093/pnasnexus/pgac111
89. Harries, MJ, Jimenez, F, Izeta, A, Hardman, J, Panicker, SP, Poblet, E, et al. Lichen planopilaris and frontal fibrosing alopecia as model epithelial stem cell diseases. Trends Mol Med. (2018) 24:435–48. doi: 10.1016/j.molmed.2018.03.007
90. Tavakolpour, S, Mahmoudi, H, Abedini, R, Kamyab Hesari, K, Kiani, A, and Daneshpazhooh, M. Frontal fibrosing alopecia: an update on the hypothesis of pathogenesis and treatment. Int J Womens Dermatol. (2019) 5:116–23. doi: 10.1016/j.ijwd.2018.11.003
91. Ramot, Y, Bertolini, M, Boboljova, M, Uchida, Y, and Paus, R. Ppar-gamma signalling as a key mediator of human hair follicle physiology and pathology. Exp Dermatol. (2020) 29:312–21. doi: 10.1111/exd.14062
92. Kang, JI, Kim, SC, Kim, MK, Boo, HJ, Kim, EJ, Im, GJ, et al. Effects of dihydrotestosterone on rat dermal papilla cells in vitro. Eur J Pharmacol. (2015) 757:74–83. doi: 10.1016/j.ejphar.2015.03.055
93. Zhu, HL, Gao, YH, Yang, JQ, Li, JB, and Gao, J. Serenoa Repens extracts promote hair regeneration and repair of hair loss mouse models by activating Tgf-Beta and Mitochondrial Signaling pathway. Eur Rev Med Pharmacol Sci. (2018) 22:4000–8. doi: 10.26355/eurrev_201806_15285
94. Gentile, P, and Garcovich, S. Advances in regenerative stem cell therapy in androgenic alopecia and hair loss: Wnt pathway, growth-factor, and mesenchymal stem cell Signaling impact analysis on cell growth and hair follicle development. Cells. (2019) 8:741–742. doi: 10.3390/cells8050466
95. Kang, JI, Choi, YK, Han, SC, Kim, HG, Hong, SW, Kim, J, et al. Limonin, a component of immature citrus fruits, activates anagen Signaling in dermal papilla cells. Nutrients. (2022) 14:744–746. doi: 10.3390/nu14245358
96. Lee, KH, Choi, D, Jeong, SI, Kim, SJ, Lee, CH, Seo, HS, et al. Eclipta prostrata promotes the induction of anagen, sustains the anagen phase through regulation of Fgf-7 and Fgf-5. Pharm Biol. (2019) 57:105–11. doi: 10.1080/13880209.2018.1561729
97. Kim, J, Shin, JY, Choi, YH, Kang, NG, and Lee, S. Anti-hair loss effect of adenosine is exerted by camp mediated Wnt/Beta-catenin pathway stimulation via modulation of Gsk3beta activity in cultured human dermal papilla cells. Molecules. (2022) 27:27. doi: 10.3390/molecules27072184
98. Choi, YK, Kang, JI, Hyun, JW, Koh, YS, Kang, JH, Hyun, CG, et al. Myristoleic acid promotes anagen Signaling by autophagy through activating Wnt/Beta-catenin and Erk pathways in dermal papilla cells. Biomol Ther (Seoul). (2021) 29:211–9. doi: 10.4062/biomolther.2020.169
99. Lee-Kirsch, MA. Sensing of RNA stress by Mtorc1 drives autoinflammation. J Clin Invest. (2022) 132:759–767. doi: 10.1172/JCI156119
100. Grimm, C, Chen, CC, Wahl-Schott, C, and Biel, M. Two-pore channels: catalyzers of endolysosomal transport and function. Front Pharmacol. (2017) 8:45. doi: 10.3389/fphar.2017.00045
101. Petit, CS, Roczniak-Ferguson, A, and Ferguson, SM. Recruitment of folliculin to lysosomes supports the amino acid-dependent activation of rag Gtpases. J Cell Biol. (2013) 202:1107–22. doi: 10.1083/jcb.201307084
Keywords: mTOR signaling, hair follicle, hair follicle cycles, hair disease, VDR
Citation: Tu W, Cao Y-W, Sun M, Liu Q and Zhao H-G (2023) mTOR signaling in hair follicle and hair diseases: recent progress. Front. Med. 10:1209439. doi: 10.3389/fmed.2023.1209439
Edited by:
Fiorenza Rancan, Charité University Medicine Berlin, GermanyCopyright © 2023 Tu, Cao, Sun, Liu and Zhao. This is an open-access article distributed under the terms of the Creative Commons Attribution License (CC BY). The use, distribution or reproduction in other forums is permitted, provided the original author(s) and the copyright owner(s) are credited and that the original publication in this journal is cited, in accordance with accepted academic practice. No use, distribution or reproduction is permitted which does not comply with these terms.
*Correspondence: Heng-Guang Zhao, emhhb0BjcW11LmVkdS5jbg==