- 1Robert’s Program for Sudden Unexpected Death in Pediatrics, Boston Children’s Hospital, Boston, MA, United States
- 2Division of Newborn Medicine, Department of Pediatrics, Boston Children’s Hospital, Boston, MA, United States
- 3Division of Genetics and Genomics, Department of Pediatrics, Boston Children’s Hospital, Boston, MA, United States
- 4Harvard Medical School, Boston, MA, United States
- 5F.M. Kirby Neurobiology Center, Boston Children's Hospital, Boston, MA, United States
- 6Epilepsy Genetics Program, Department of Neurology, Boston Children's Hospital and Harvard Medical School, Boston, MA, United States
- 7Department of Medicine, Brigham and Women's Hospital, Boston, MA, United States
- 8Division of General Pediatrics, Department of Pediatrics, Boston Children's Hospital, Boston, MA, United States
A definitive, authoritative approach to evaluate the causes of unexpected, and ultimately unexplained, pediatric deaths remains elusive, relegating final conclusions to diagnoses of exclusion in the vast majority of cases. Research into unexplained pediatric deaths has focused primarily on sudden infant deaths (under 1 year of age) and led to the identification of several potential, albeit incompletely understood, contributory factors: nonspecific pathology findings, associations with sleep position and environment that may not be uniformly relevant, and the elucidation of a role for serotonin that is practically difficult to estimate in any individual case. Any assessment of progress in this field must also acknowledge the failure of current approaches to substantially decrease mortality rates in decades. Furthermore, potential commonalities with pediatric deaths across a broader age spectrum have not been widely considered. Recent epilepsy-related observations and genetic findings, identified post-mortem in both infants and children who died suddenly and unexpectedly, suggest a role for more intense and specific phenotyping efforts as well as an expanded role for genetic and genomic evaluation. We therefore present a new approach to reframe the phenotype in sudden unexplained deaths in the pediatric age range, collapsing many distinctions based on arbitrary factors (such as age) that have previously guided research in this area, and discuss its implications for the future of postmortem investigation.
Introduction
A substantial proportion of infant and early child mortality remains unexplained. When a postmortem examination fails to identify a specific cause for a child’s death, one of a long list of acronyms may be used to certify the death, with terminology emphasizing its sudden, unexpected nature (Table 1). This list includes sudden unexpected infant death (SUID), referred to as sudden unexpected death in infancy (SUDI) outside the United States (US). SUID and SUDI are intended sometimes to denote a discrete diagnosis used interchangeably with sudden infant death syndrome or “unexplained SUDI,” but at other times they imply a broader category of mortality that includes sudden infant death syndrome (SIDS), undetermined or unclassified cause of death (Undetermined), and accidental strangulation or suffocation in bed (ASSB) (2). SIDS, undetermined, and accidental suffocation are categories used to certify deaths in infants with similar evidence for cause (3), even though the certification of accidental suffocation or positional asphyxia as a cause of death implies objective findings beyond the presence of risk factors (4). When a death without a determined cause occurs in a child over the age of 1 year, the diagnosis of sudden unexplained death in childhood (SUDC) may be used, although the term has not been assigned a formal code in the International Classification of Diseases (ICD). Sometimes the cause of death is left unspecified and is certified as such. Unexplained (unspecified) sudden cardiac death or cardiac arrest and sudden cardiac death, so described are other labels for deaths in children that may be unexplained after autopsy, and similarly deaths may be attributed to epilepsy or a convulsion without a requirement to establish the role or presence of either as a contributor to the lethal event. (Sudden unexplained death in epilepsy, SUDEP, has only recently been given an ICD code.) The above categories comprise 15.9% of deaths in infants and children under the age of 5 years in the United States; between the ages of 1 month and 1 year, they account for nearly half (Figure 1) (1, 5).
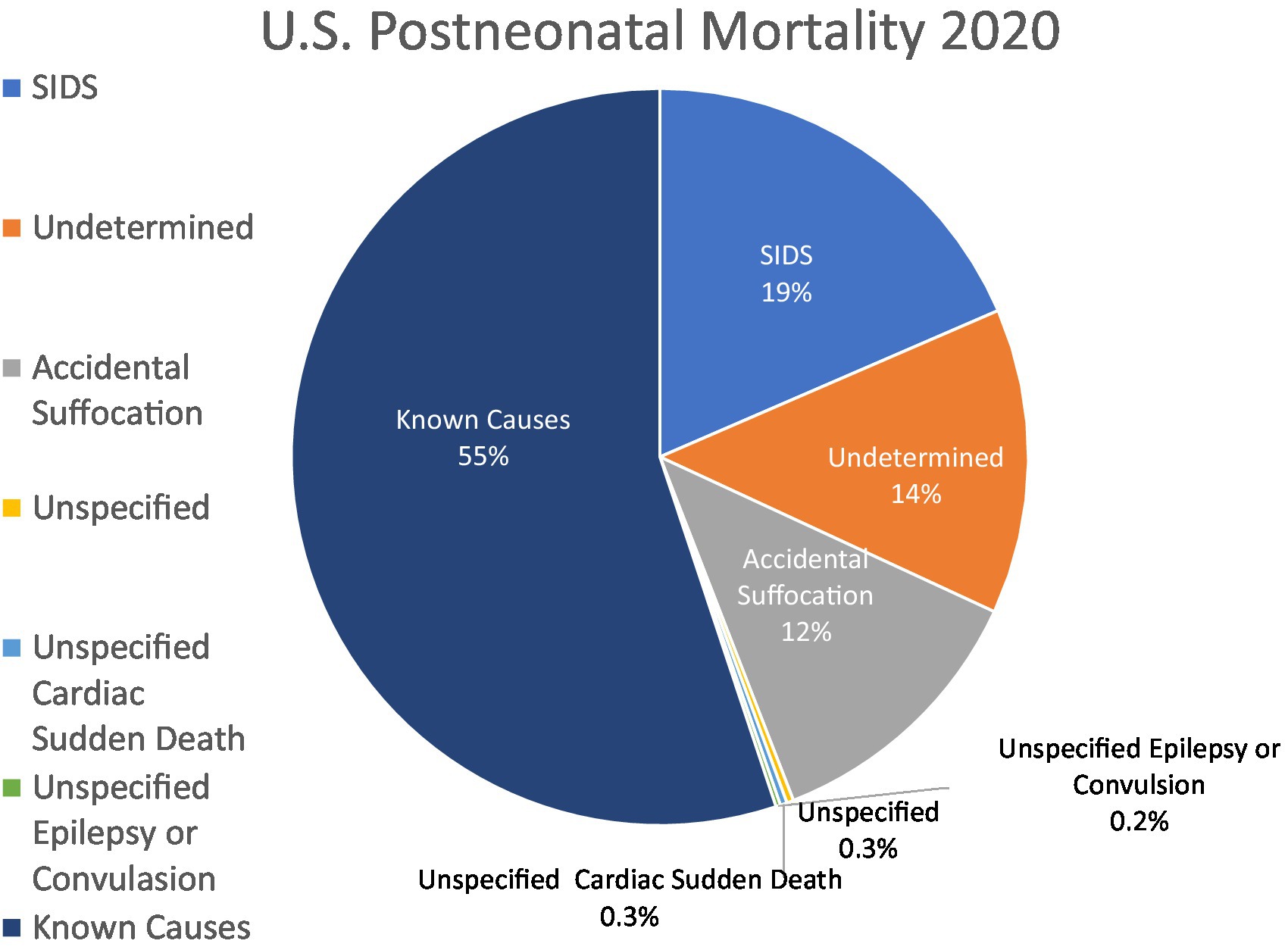
Figure 1. Explained and unexplained US postneonatal mortality, 2020. US postneonatal mortality divided by explained causes of death (55%) and unspecified or other causes of death included in SUID (sudden unexpected infant death; 46%). Data are from Linked Birth/ Infant Deaths Records available through the CDC WONDER database for 2020 (1).
The nosology of sudden, unexpected pediatric deaths reflects the origins of these categories in the pre-genetic, pre-precision medicine era. When initially proposed in 1969, SIDS included all that would now be considered SUID, SUDI, SIDS, undetermined deaths, ASSB, and SUDC. SIDS, although it contained “infant” in the acronym, was originally defined as the sudden death of an infant or young child, which is unexpected by history, in which a thorough post-mortem examination fails to demonstrate an adequate cause for death (6). Essentially, SIDS was meant to label the unexplained death of an infant or child who was not expected to die. The definition of SIDS became restricted to infants under 1 year of age in 1991 as sleep-related factors became implicated (7). Continuing controversies about nomenclature in the field expose fundamental limitations in definitions with little specificity beyond death and age (8). Our program has termed this problem “sudden unexpected death in pediatrics” (SUDP) (9). Hereafter, we will use SUDP as the inclusive umbrella term to incorporate the broader phenomenon of sudden unexpected death in infants and children. We will use SIDS specifically implying the 1991 definition (7), without subtypes (10), and we will use SUID as a term to include SIDS, undetermined deaths and accidental suffocation (unintentional threat to breathing by external cause) under the age of 1 year (11).
The foundations of SIDS research
A greater understanding of factors that contribute to these deaths has been accomplished through the utilization of pathology and epidemiology in the age-based subgroupings. Current preferences for subcategorization, and the avoidance of the term SIDS as originally proposed, reflect a view that the mortality can be sufficiently explained by identified risk factors including age, sleeping position, and sleep environment in sleep-related deaths with a noncontributory medical history. This preference is chiefly not a consequence of the discovery of new biological markers to establish any specific cause, but rather a result of circumstantial observations and their epidemiologic associations.
Pathology
SIDS is a condition that presents catastrophically with death and its evaluation involves investigation by pathologists. Since the first published pathological study of 124 SIDS-like infant deaths, investigators have been typically unable to find conclusive evidence for mechanical suffocation based upon autopsy findings (12). Pathological tissue markers for SIDS have been identified: gliosis of the brainstem in areas crucial to respiratory control, increased hepatic erythropoiesis, abnormal thickening of smooth muscle in the walls of small pulmonary arteries, and abnormal relative retention of periadrenal brown fat (13, 14). These markers continue to be documented as nonspecific but recurrent observations on autopsy and may represent surrogate markers for prior sublethal hypoxic exposures. Intrathoracic petechiae in the thymic, cardiac and pulmonary pleura are also commonly noted, suggesting a terminal event that involves negative intrathoracic pressure, yet their presence is unrelated to sleep position (15). However, no pathognomonic features have been elucidated and no biomarker for asphyxia has been developed (16, 17).
It should be noted that, because SUDP involves an unexpected death in a dependent child, a medicolegal autopsy is mandated around the world. In practical terms, mandated autopsies largely compile evidence about the manner of the child’s death to assist in the investigation for possible homicide or neglect and reveal little pertaining to the causes or mechanisms of death beyond trauma, toxicology, and microbiology/virology. In typical practice, these investigations have been insulated from existing and emerging capabilities in molecular autopsy found in academic medical centers (18, 19) and, at least in the United States, the capabilities of the autopsy have changed very little (20). A review of specialized English-language textbooks and sources addressing practice in sudden unexpected deaths in infants and children (4, 21–24) reveals there are no expectations for a molecular autopsy in the routine assessment of SUDP. Unlike emerging standards for the initiation of family studies in early cardiac deaths in adults (25), elements of the molecular autopsy are rarely incorporated in SUDP (26).
Epidemiology
In contrast, epidemiology directly informs medicine’s approach to SIDS. The initial justification for SIDS as a diagnosis was based on its characteristic pattern of incidence: rare before 1 month of age, peaking between 2 and 4 months, and the major proportion of its mortality occurring before infants reach 6 months of age (Figure 2) (27). This pattern suggests an underlying condition more complicated than developmental vulnerability to airway obstruction and suffocation, since it spares infants whose motor strength and development would be expected to leave them most susceptible to positional asphyxia in the first month of life. A period of concentrated incidence under 6 months is also characteristic of SIDS, and is seen in current data demonstrating that 78.4% of unexplained mortality under the age of 5 years occurred before 6 months of age, followed by continued albeit declining rates of unexplained mortality (5).
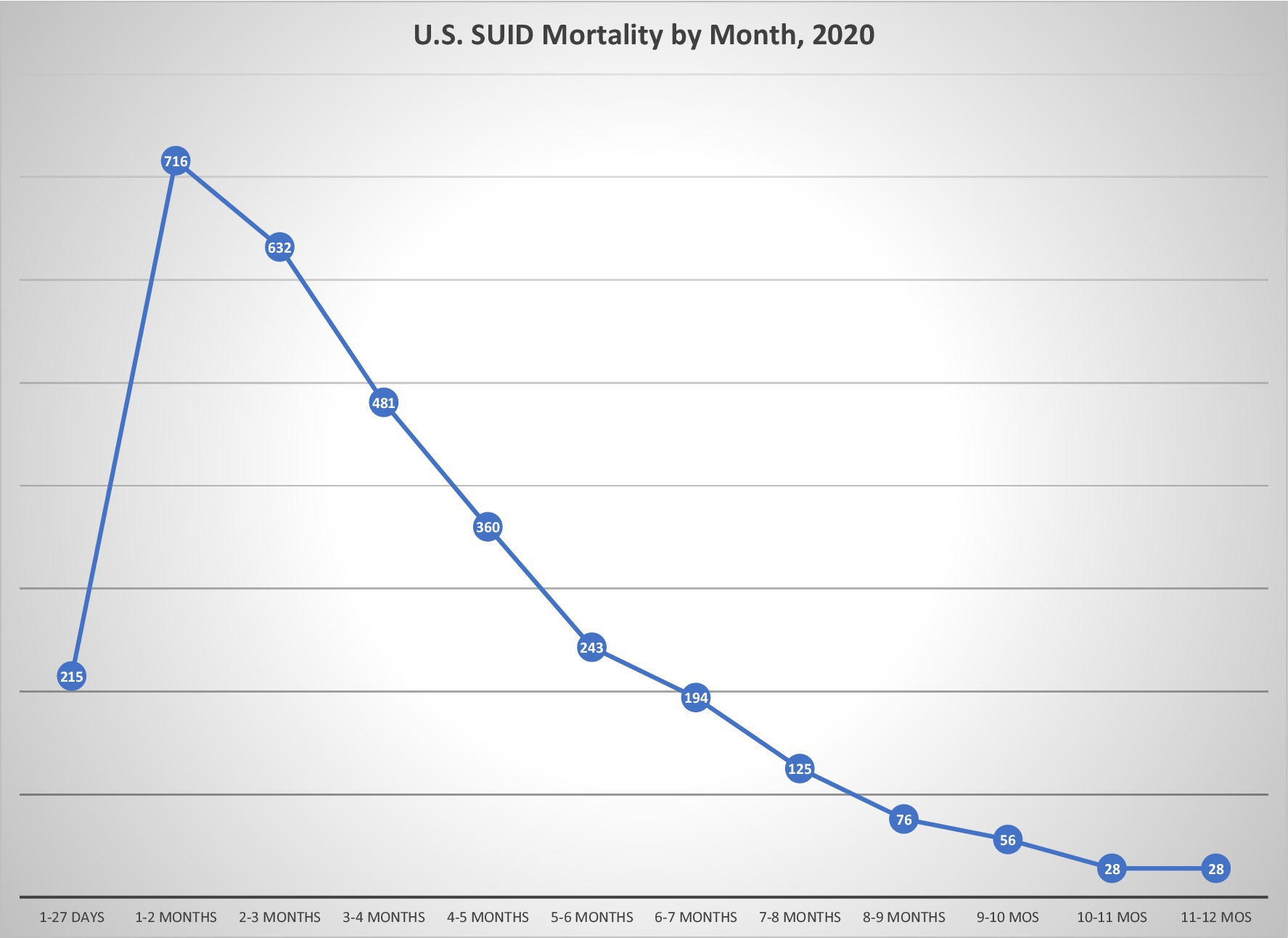
Figure 2. US SUID mortality by age at death. Data of SUID deaths by age of occurrence illustrates relative sparing of youngest neonates, concentrated mortality in ages under 6 months, and continued albeit lesser mortality at older ages. Data are from Linked Birth/ Infant Deaths Records available through the CDC WONDER Online database for 2020 (1).
Epidemiological research has identified associations between sleep position and environment, and the pathogenesis of SIDS (28, 29). The most commonly cited risk factor for SIDS is prone sleep position [odds ratio (OR):2.3–13.1] (30–32), but soft and shared sleep surfaces are also associated with risk (33). Great credence is given to the fact that SIDS rates dropped by nearly half at a time when placing infants to sleep in the supine position was promoted globally. In some quarters, this is interpreted to mean that infants who die from SIDS are children whose vulnerability is due to sleep environment risks that any normally developing infant would be unable to escape, until they develop the ability to roll at 4–5 months of age. However, the mortality decreases observed in infants during the first years of global campaigns advocating supine infant sleep were not isolated to SIDS mortality alone (34), and similar drops were found even in countries that did not promote supine sleep (35).
Brainstem serotonin
Unlike efforts to better describe risk for suffocation, most basic science research in SIDS has endeavored to understand how modest risks in the sleep environment may serve as mechanistic triggers for underlying vulnerabilities. Attention to the age of peak incidence in SIDS directed researchers to identify mechanisms to explain data from infants who had died from SIDS while on cardiorespiratory monitors (36, 37). In some of those cases, the observed terminal event was different than what would be expected in suffocation but, instead, involved a failure of “autoresuscitation” characterized by an uncoupling of terminal gasping from the tachycardia that would be expected to accompany it. In the setting of hypoxia and/or hypercarbia, the infants generated gasps after an apneic pause, but the gasps were not accompanied by a cardiac response (38, 39). The infants did not arouse and there was no struggle at the time of death.
Efforts to explain this phenomenon led to landmark research involving the neurotransmitter serotonin in SIDS (40). Serotonin neurons are carbon dioxide sensors that normally cause arousal from sleep and increase ventilation in response to hypercapnia (41, 42). In numerous studies, researchers have shown that levels of serotonin and its binding sites in key brainstem nuclei involved in this response are altered in SIDS (43, 44). Human tissue research has repeatedly shown that the serotonopathy may be present in as many as 40% of infants dying from SIDS (43), and are observed in prone or supine sleep positions and varied environments (45). Subsequently, a mechanism for this auto-resuscitative failure was demonstrated in experimental animal models at a developmental age consistent with SIDS (46, 47). However, in part due to the insulation of SIDS autopsies (as discussed above), serotonin measurements have not translated into standard practice for the postmortem assessment following SIDS, remaining untested in universal forensic practice. The very limited uptake of testing of this pathway makes it of little relevance to clinical medicine, whatever the fatal mechanism involved. Moreover, though serotonin is a factor, little insight has been gained about what causes the serotonopathy.
Triple risk theory
Taken together, these observations from epidemiology and neuropathology point to a coalescence of factors responsible for death between 1 and 6 months of life, commonly summarized as the “Triple Risk Theory for SIDS” (48). According to the Triple Risk Theory, children who die from SIDS are not “normal” despite having exhibited no overt abnormalities when alive, but they have inapparent underlying vulnerabilities that become fatal when stressed by some external trigger (e.g., hypoxia, hypercarbia, or hyperthermia), and this susceptibility is developmentally or chronologically dependent (48–50). The Triple Risk Theory is commonly held as the working hypothesis for SIDS (33). It may be worth noting that this is the working hypothesis for essentially every disease where the mechanism is unknown.
Factors challenging the status quo
Despite these advancements, our understanding of the etiology of SIDS is generally recognized to be incomplete. Conclusions from epidemiologic research dominate the current approach, but several factors cannot be adequately addressed by identified risk factors related to sleep position and environment in the pathogenesis of SUID. [Though not discussed here, it should be noted that epidemiology and pathology have both demonstrated the role of cigarette smoking in SUID pathogenesis (51, 52).]
As already stated, SUID spares the youngest infants – even though their motor strength and development would be expected to make them especially vulnerable to positional asphyxia or suffocation risks in the sleep environment. Of the substantial mortality occurring in the first month of life—in 2020, over 75% US mortality in children under 18 years occurred between 1 and 27 days of life—SUID mortality amounted to only 2.9% (1). Although the prioritizing of infant sleep advice is frequently justified by the improved mortality rates that occurred when supine sleep was first promoted in the early 1990s, not all children die in the prone position: recent studies of mortality in the era of supine sleep promotion found over 40% of SIDS were placed (53) or found (54) in the supine position. These studies also detail other risk factors in the sleep environment but other meticulous, population-based research reports 8% of its sample was found supine, alone, on a firm surface, with head uncovered (55).
Although the focus is typically placed on data showing that only 1–2% of SUID occurs without any risk factors in their sleep environment, the mortality reductions attributed to infant sleep recommendations largely occurred during the initial years of “Back to Sleep,” when only supine sleep position was promoted and before other modifications in the sleep environment became part of the messaging. There has been very limited improvement in overall SUID rates since the inclusion of environmental risk factors in safe sleep campaigns (11, 56), despite three decades of parent guidance about sleep environment (34) (Figure 3). Yet, in 17.7% of SUID cases where suffocation was implicated, it was attributed to soft bedding in 74% (57). Position and sleep environment remain the most important modifiable risk factors, and further improvements are possible with greater adherence to infant sleep advice, but the argument remains that additional strategies are needed to address SUID.
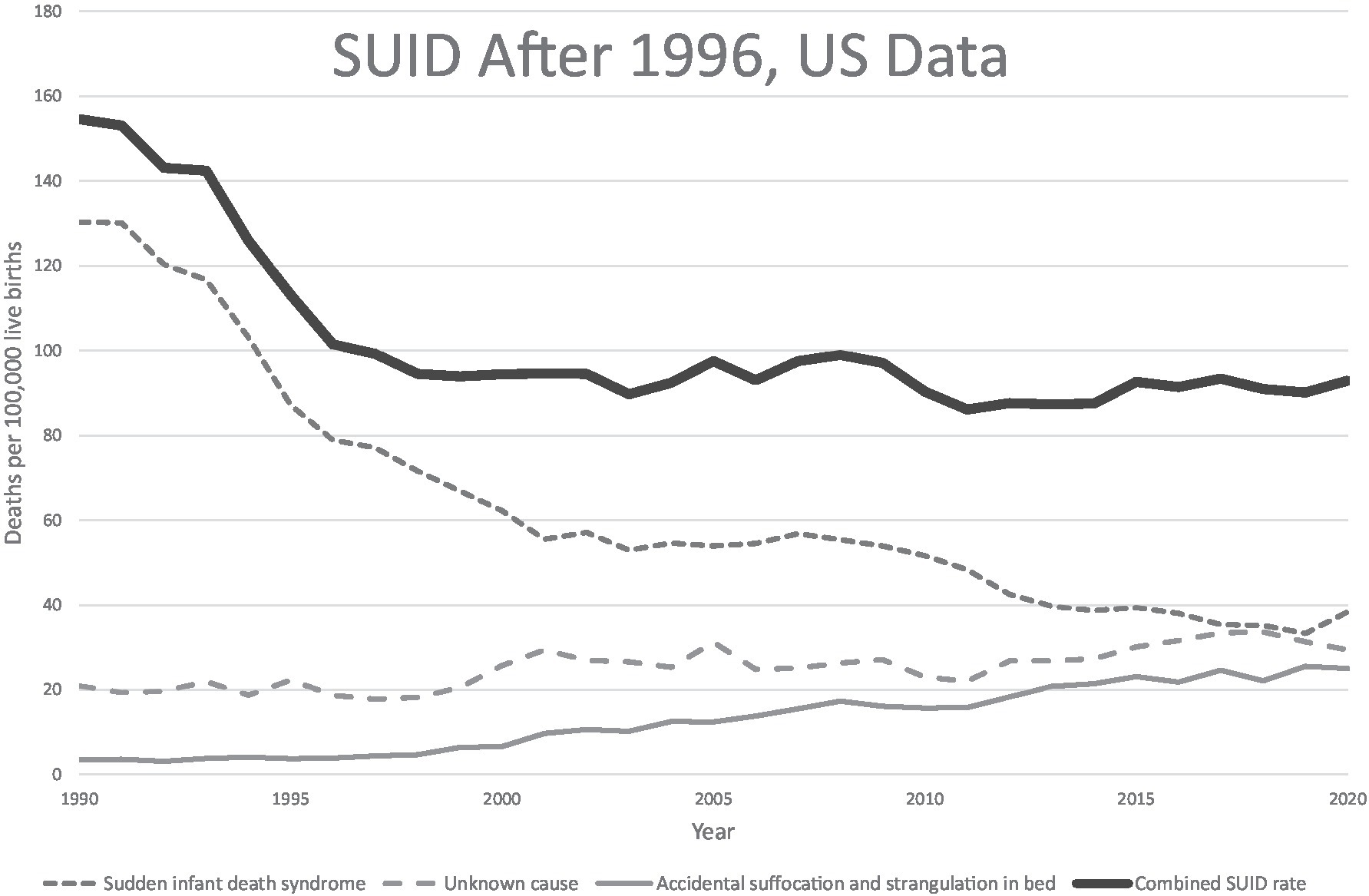
Figure 3. US SUID mortality over the back to sleep era. US mortality rates for SUID and its components in the “Back to Sleep” era. Data from CDC/NCHS, National Vital Statistics System, Mortality Files available at https://www.cdc.gov/sids/data.htm.
Another gap opened with an explanatory model centered on postural competence is its failure to address deaths over the ages of 4–6 months, when children with normal motor development are able to control their head and neck and have the ability to roll (58). Cohorts in research presenting “risk factors for suffocation” include infants well beyond the age when this developmental vulnerability should be relevant (57). Deaths in infants and children over the age of 6 months with normally-developed postural competence have remained a conceptual wasteland, unaddressed by etiologic hypotheses until older ages in childhood when death from diseases like cardiac arrhythmias or epilepsy are suspected or found (Table 1). There is limited recognition of the size and the significance of this unexplained population, and instead there is a tendency to lump the cause of their deaths into other etiologies. For example, cardiac etiologies are sometimes claimed to be responsible for unexplained deaths in children between 1 and 5 years of age with unrevealing autopsies, even in the vast majority for whom genetic testing fails to identify a gene associated with cardiac disease (59). If these cases parallel the 44% of sudden cardiac deaths without an arrhythmic cause in adults (60), then this should more properly be considered an undiagnosed disease. Similarly, sudden unexplained death in epilepsy, the sudden, unexpected, witnessed or unwitnessed, non-traumatic, and non-drowning death in patients with epilepsy (61), requires previously recognized seizures of sufficient complexity to warrant the diagnosis of epilepsy, ignoring the possibility that first seizure events might be lethal. All of this underscores the fact that current practices focus on only some of what are presumed to be many factors in a multifactorial process that leads to death.
Other lines of research have untapped potential in this area. Neuropathologic changes otherwise associated with temporal lobe epilepsy, specifically bilamination in the dentate gyrus of the hippocampus, are found in over 40% of SIDS (62) or SUDC cases (63), in children as young as 6 months up to 16 years (64). Research into SUDEP suggests serotonin mediated mechanisms in common with SIDS and provides new insight into effects on arousal and respiratory control (65). This research suggests that SUDP mortality may occur across a spectrum of diseases with variable ages and severities of presentation, sometimes resembling known disease but at others requiring more than the current approach to diagnosis has to offer.
Genetic diagnoses found in SUID deaths are often published as case reports (66–70) or nested within larger postmortem (18) or disease-specific (59, 66) cohorts. In recent research involving larger cohorts of SUDP cases, the overall pathogenic genomic variation has become clearer. Potentially causative variants have been identified in metabolic and cardiac genes in 20% of SUID (71) and clinically actionable cardiac variants in 4.3% (72). Our group has reported likely contributory variants in 11% of a well-phenotyped cohort of 352 SUDP cases (73). That work also reported an excess burden of rare damaging variants in genes plausibly linked to sudden death and of rare, de novo variants, while others found enrichment in nonsynonymous de novo variants in genes associated with cardiac and seizure disorders and a trend to excess loss of function variants in genes intolerant to such variance (74). Still others have found an increased rate of mitochondrial DNA variants in SUDP, the implications of which remain unclear, but could also be related to prior sublethal hypoxic exposures (75). As barriers to population-based genomic sequencing and analysis of these case are slowly overcome, the prevailing age-based categorization and the idea of a unified cause gives way to heterogeneity among infants and children dying from SUDP and analogs in a spectrum of pediatric diseases (Figure 4). Genetic findings defy the unexpected/unexplained categories and challenge the distinctions upon which the categories of SIDS, SUID and SUDC are based, while also highlighting the limited resolution of the phenotype of SUDP.
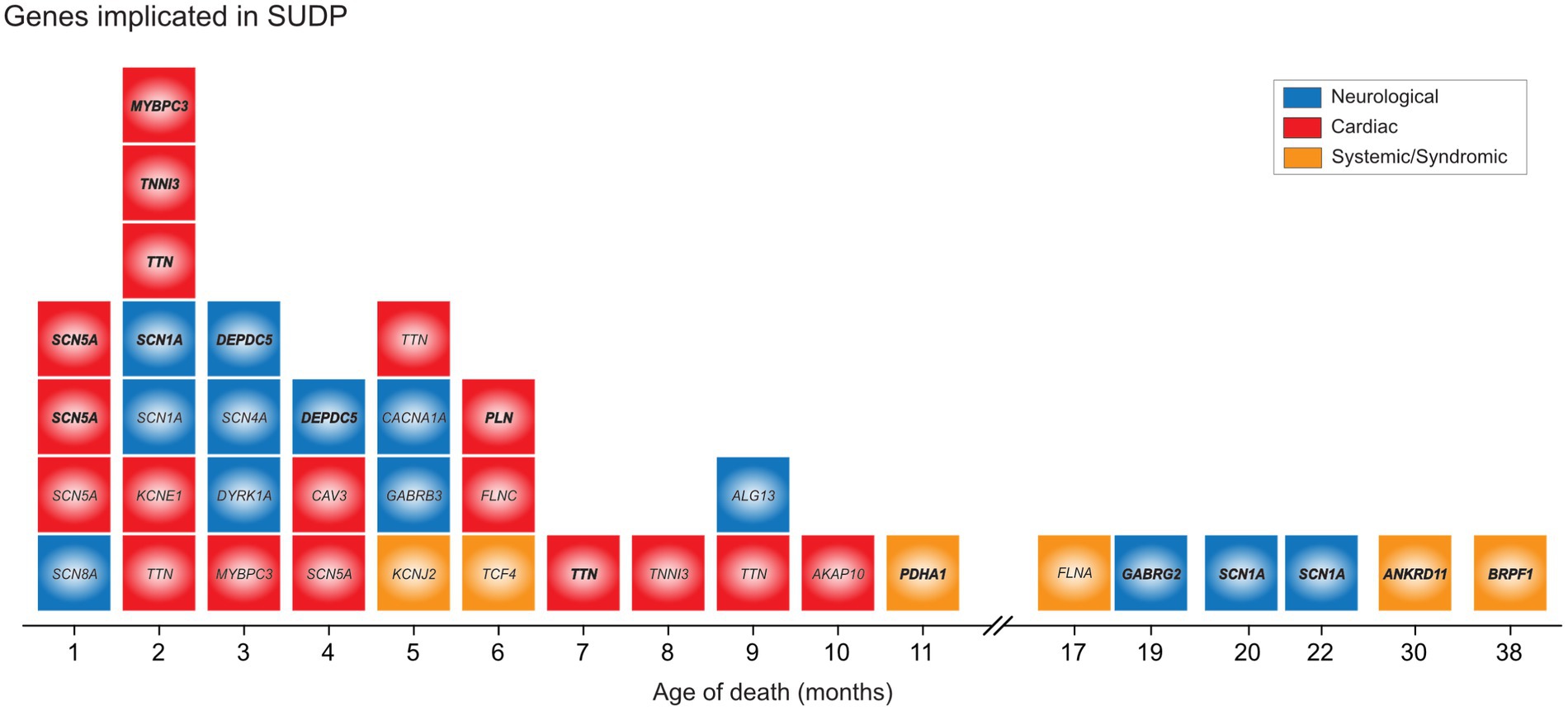
Figure 4. Genetic variants implicated in unexplained deaths in infants and children. Identified pathogenic, likely pathogenic and VUS-leaning pathogenic variants in a cohort illustrate presence of genetic determinant, distribution by age of death mirroring the general epidemiology, and the involvement of common genes that defy the age cut off. By permission, from Koh et al. (73).
Although further investigation into the genotype–phenotype spectrum of SUDP is needed to fully define the breadth and depth of “lethal” pathogenic variation, a large issue that researchers confront is the “phenotype gap,” when deleterious variants are identified in cases where terminal mechanisms are unwitnessed and uncertain. This in contrast to children who, on retrospective review of records in the context of a genetic variant, are found to have multiple abnormalities that had either not prompted medical attention or had not been recognized as a syndrome (68). A review of research in SIDS and long QT syndrome (LQTS) highlights many of the hallmarks of SIDS research conducted without a prevailing phenotype. While LQTS now would be considered a separate cause of death from SIDS, the finding that some SIDS deaths were due to LQTS (76) brought attention to the role of channelopathies and their genetic basis in SIDS. Yet, there has been a reluctance to expand the phenotype from a group of patients living with and surviving disease to now include children who were apparently healthy prior to SUDP, even though disease-associated variants (LQTS) were identified. Recognized, validated adult disease may offer direct links between potential mechanisms and otherwise unexplained death, a conclusion that is supported by growing consensus that variable penetrance is typical in most genetic disorders (77).
This problem goes beyond arrhythmias and also includes cardiomyopathy-associated variants in SUDP. Cardiomyopathy is complicated to diagnose in its early stages, requiring the presentation of symptoms at critical points in its progression. It is virtually unexplored in the pre-diagnostic ages before apparent illness, and extreme first presentations are rarely considered. Yet, clinically actionable cardiomyopathy associated variants made up 70% those found in autopsy-inconclusive sudden cardiac deaths (78). The same might be said about epilepsy associated genes, where deleterious variants in SCN1A have been reported in Dravet’s syndrome, genetic epilepsy with febrile seizures plus (GEFS+), SIDS, and SUDC (69, 70, 73). Since investigation into genetic causes in these cases is not the current standard of practice, it seems reasonable to expect that there are important insights to be gained. One might imagine a panoply of other disorders contributing to SUDP, each recognizable in their fully penetrant forms yet obscurely uniform in early manifestations associated with sudden death.
It is important to note that, while the disease processes may be stochastic and relatively age-agnostic, pediatric disease and genomic risk factors are not unaffected by medical intervention. The true ‘natural history’ of genetic conditions is impossible to determine in the pediatric age range, as survival is highly dependent upon medical intervention. For example, a sudden cardiac and respiratory collapse in an infant previously thought to be healthy may result in death in one clinical context, whereas the same child presenting to another hospital might be resuscitated, undergo a genetic evaluation, and be found to have a chromosomal deletion disorder causing hypotonia and explaining the unexpected collapse; this same child may then survive into childhood and beyond. Indeed, the population of infants who are chronically critically ill, i.e., reliant on technology with life-limiting impairments, may have in different contexts died and been classified as SIDS/SUDC deaths. Further compounding these challenges is the inability to accurately account for deaths attributable to genetic conditions in mortality statistics, with our recent work demonstrating that a substantial proportion of infant mortality confirmed to be due to a genetic disorder is not reflected as such in mortality statistics (79).
Moreover, the current state of clinical genetics and genomics struggles to identify novel genetic conditions with highly negative selection: genes with such a low negative selection pressure (fitness of zero) that they are not known to have a phenotype other than death, as highlighted in a recent genomic investigation of stillbirth that identified variants unique to perinatal lethal populations (80). The challenge thus lies in identifying these genes, when standard postmortem practice rarely includes exome or genome sequencing and at best includes “sudden death panels” that were developed based upon discoveries in individuals surviving long enough to receive the more expansive genetic assessments in modern clinical medicine. Current knowledge regarding lethal genes and phenotypes is flawed and incomplete.
From an undiagnosed diseases perspective, SIDS represents a reservoir of mortality that can be, in some cases, unaffected or only circumstantially affected by extrinsic risk factors. We must also consider evidence that the mechanisms of sudden unexpected deaths may be diverse and not conform to strict categories of organ-based diseases. Children with sudden cardiac death have double the incidence of antecedent febrile seizures than controls (81); personal or family histories of febrile seizures are over-represented in cohorts of children dying from SUDC (82). Second-trimester maternal serum alpha-fetoprotein levels are directly associated with the risk of SIDS, further suggesting unclear mechanisms (83). A focus on mechanisms that seem straightforward in a critical period before rolling in the Triple Risk may miss important aspects of an infant or child’s susceptibility. The current classifications used in unexplained infant deaths have been crucial in establishing recognition for the problem but a continued focus on a phenotype as generic as death considered with another factor providing relatively limited information such as age, has created a syndrome with a false sense of diagnostic completeness that hinders the pursuit of genetic mechanisms while also asserting a bias against the importance of stochastic processes.
A new hypothesis
We propose a new phenotyping strategy to inform future investigation, particularly from birth until the age of 10 years. Our hypothesis is that sudden unexplained deaths in the pediatric age range are the consequence of undiagnosed diseases and their elucidation is amenable to the techniques of precision medicine. The core population comprising this phenotype is demarcated by human survival statistics that distinguish pediatric mortality trends from mortality rates found later in life (Figures 5A,B). The hypothesis underlying this phenotyping approach and its potential utility are supported by discoveries that have resulted in reproducible biomarkers, albeit in restricted subsets of SUDP cases. We believe that any reframing should build on existing insights and observations and uniformly deploy the best modern medical tools to achieve mortality reductions. These tools include important advances in the molecular or genomic autopsy, the uniform inclusion of family history into basic considerations about disease definition, and a deep and systematic evaluation of the family and its environment. This will bring the diagnostic approach to these deaths into alignment with the strengths of precision medicine. The intent of this phenotyping strategy is not merely for classification and population statistics but, rather, for individual case determination and disease discovery.
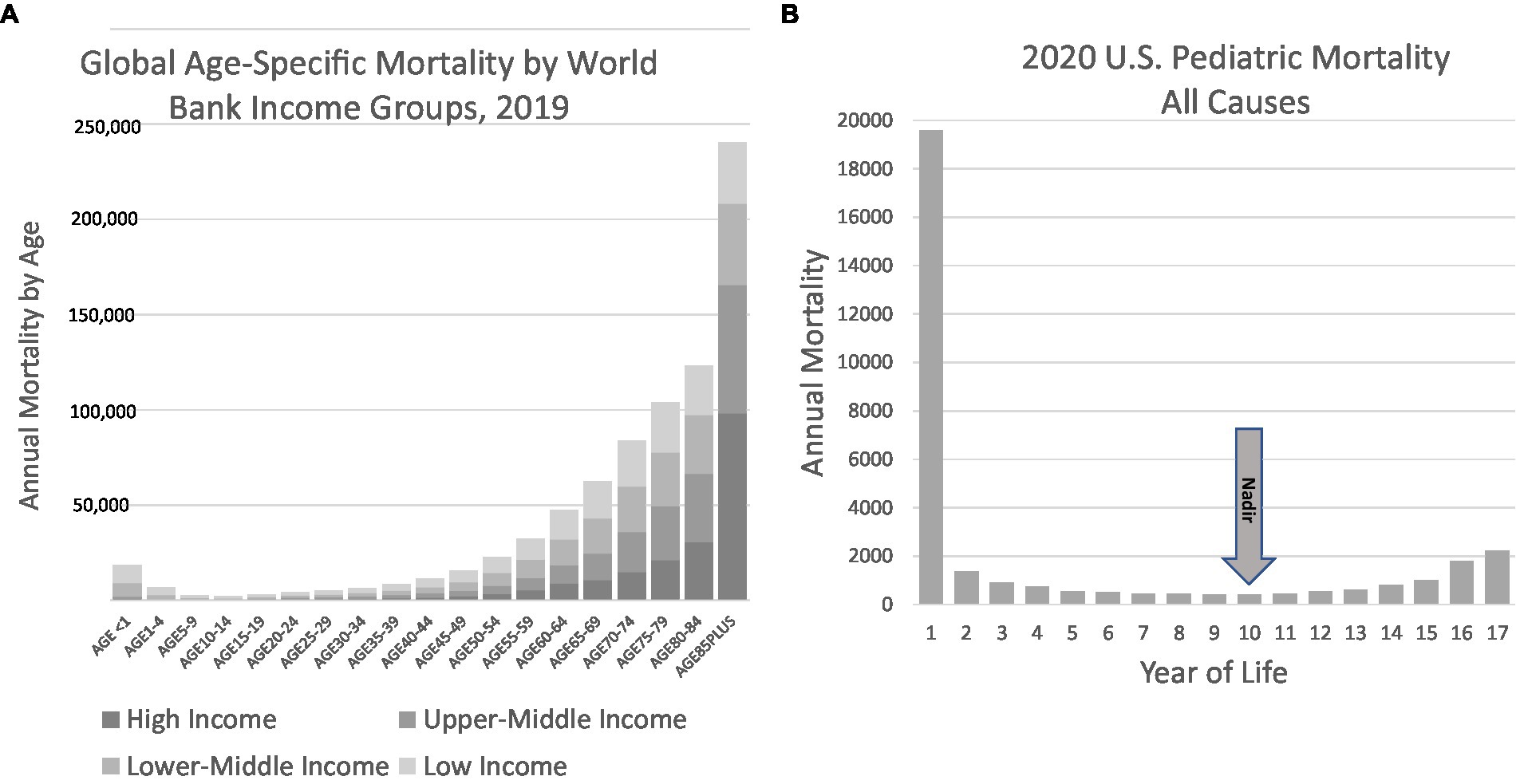
Figure 5. Global mortality and US pediatric mortality by age. (A) Global mortality data illustrate the convex nature of mortality, with decreasing mortality in the first decade followed by increases typically attributed to maturity effect. Data obtained from the World Health Organization Global Health Observatory, Life tables (84). (B) US Pediatric data illustrates the nadir in mortality to occur in the 10th year of life. Data from the CDC WONDER database for 2020 (4).
The proposed phenotyping strategy incorporates all of the diagnostic categories for sudden unexpected pediatric deaths detailed in Table 1. The categories listed in Table 1 are considered false distinctions by implication, except in cases where evidence for the stated cause can be objectively demonstrated and measured. Accidental suffocation, we should mention, is not entirely excluded as a potential cause of death but, instead, is to be regarded as a specific cause of death that requires objective findings at the death scene or on autopsy, and not merely inference from risks in the sleep environment and position. Sudden cardiac death is not excluded as a cause of death, but its diagnosis must be based on genetic, autopsy, or family findings that support its designation.
A phenotype that systematically incorporates all unexplained early child mortality represents a heterogeneous but distinct population of children dying due to (1) etiologies incompatible with survival presenting catastrophically, (2) mechanisms not typically seen in later life or, if seen, manifesting differently in infancy than adulthood, and (3) mechanisms identical to those observed in adult disorders but obscured in pediatrics by incomplete penetrance, variable expressivity or stochastic occurrence over time. It includes presentations of known diseases not routinely ascertained by current postmortem evaluations, expansion of the phenotypes of known diseases, and novel pathological mechanisms revealed by genomic analysis. The diagnostic potential is seen not only in our work (85, 86), but that of others (87). As a working hypothesis, this category exists on a continuum comprising deaths that occur in the setting of stillbirth, congenital malformations, prematurity, SIDS, SUDC, SUDEP and Sudden Cardiac Death. It approximates recognized, validated disease in some case (more frequently in later childhood) when it presents similarly to SUDEP and sudden cardiac death. Age-based distinctions are not without basis but are not central to the definition of this phenotyping approach.
Instead, this phenotypic umbrella integrates multiple noncontiguous endophenotypes. Endophenotypes are biomarkers that are biologically correlated to an outcome of interest or disease vulnerability, and can be measured in all individuals, whether affected or unaffected. They offer the practical advantage of providing greater power to identify disease-related biomarkers and genes than binary disease categories, especially when compared to the vague phenotype of SIDS (84, 88). Endophenotypes serve as intermediaries between genetic risk and ultimate outcome, and gain definition through their combination of multiple axes of objective data. For example, biomarkers of epilepsy and cardiomyopathy can be found on autopsy or in extended family studies, serotonin binding can be quantified through immunohistochemical assays, or personal and family histories of febrile seizures can be identified in medical histories. Once biomarkers are identified, they provide an enhanced method to reveal the genetic architecture of heterogeneous process under the common umbrella of SUDP. The greater challenge is understanding the patterns of disease these structural features represent.
It will be crucial to increase our understanding of heritable risk within affected families and include it into some endophenotypes. In this regard, the lingering influence of “Meadow’s Law” (89) impedes the discovery of genetic diseases. Familial recurrence studies generate odds ratios of 4.84 for SIDS and other or unknown deaths to sibs at any age or an odds ratio of 9.29 (CI 2.62–32.96) in first through third degree relatives (90). Effects of this magnitude are typically seen with Mendelian disorders or conditions with directly causal environmental influences. Discrete endophenotypes might be revealed through a family history of stillbirth, epilepsy, febrile seizures, sleep disorders, or early cardiac death. Similarly, given that preterm infants are at three-to fourfold higher risk for SIDS than term infants, and the gestational age of peak vulnerability for SIDS appears to occur 4 to 6 weeks earlier among preterm than term infants, it may be productive to investigate SUDP deaths when there were siblings with prematurity. A family history of congenital malformations could be considered an endophenotype. Siblings of children who died and were found to harbor serotonin abnormalities might be another. The study of these endophenotypes will require large, carefully detailed cohorts and collaboration.
The rigorous study of a problem with evidence of heritable contributions typically requires a formal kin-cohort study (91), where family members are phenotyped irrespective of their presumed affected status (likely to be assumed non-affected if alive and over 1 year of age) and based simply on relationship to the proband. The current conundrum in SIDS research stems from the forced uniformity of the available dataset (i.e., sudden death with no evident cause), and so cannot be assumed to reflect any particular subset of conditions or mechanisms (genetic or environmental). As an alternative, any systematic approach would ideally require a consistent minimal dataset not conditioned on the mode of presentation. This minimal dataset should include a standard post-mortem sample collection for genomics, metagenomics, proteomics (92), and environmental toxicology. Neurohormonal, metabolic, or ischemia-related biomarkers would also be of broad utility in testing extant hypotheses for SIDS. Whole body post-mortem MRI has the potential to add a substantive and unbiased dataset to the typical forensic autopsy (93, 94), and here too standard tissue sampling protocols for central digital pathology using modern analytics would minimize the subjectivity in these relatively infrequent evaluations. Family members offer the potential for rigorous assessment of multiple anatomic and physiologic endophenotypes in individuals with a high pretest probability of shared abnormalities, including core cardiac and neurological functional evaluations, or sleep studies that include targeted assessment of physiological responses to hypoxia, hypercarbia, or other external perturbations. Rigorous kin-cohort approaches would mandate the study of at least two generations and would include formal transmission probabilities. Segregation analysis also lends remarkable statistical power to this approach and lays the groundwork for formal molecular genetics. The development of functional genomic biomarkers for asphyxia or suffocation would have tremendous utility not only in pediatrics and several candidates already exist including the induction of hypoxia pathways. Such assays, for example RNA sequencing, may also have the advantage of integrating events over the hours prior to the demise of the infant.
Efficient assessment of core endophenotypes across multiple sites would rapidly define whether any potential phenotype is of utility. This necessarily will require investments in collaborations with medical examiner systems, as these efforts might also be rigorously integrated into forensic autopsies accelerating closure in this harrowing process. They will require referral networks with diverse participation; they will be facilitated by centers of excellence that concentrate the resources necessary to conduct the work.
Discussion
The 21st century began as the first in recorded history when mortality rates in infants and children are lower than those for adults. This was achieved through advances in public health, hygiene, and nutrition, and by medicine’s steady progress in transforming diseases and their illnesses from mysteries into scientifically understood mechanisms. Some of medicine’s success applies equally in children and adults. The pathogens of infectious diseases are as relevant in pediatrics as they are in internal medicine. But this is not uniformly the case. Childhood cancers, for example, are mostly distinct from those found later in life and their mechanisms differ. As it goes with known disease, so it goes with the unexplained. Burdens of unexplained disease stand out in relief and, while the extant epidemiologic associations dominating the field are valid, the sole focus on them has not demonstrated continuing effectiveness (Figure 6). We propose, instead, to treat sudden unexplained deaths in pediatrics as a broad phenotype of undiagnosed disease conforming to a distinct pattern in human survival. We believe careful phenotyping and genomic evaluation will shed light on individual causes as well as common mechanisms that will only be understood with this focus.
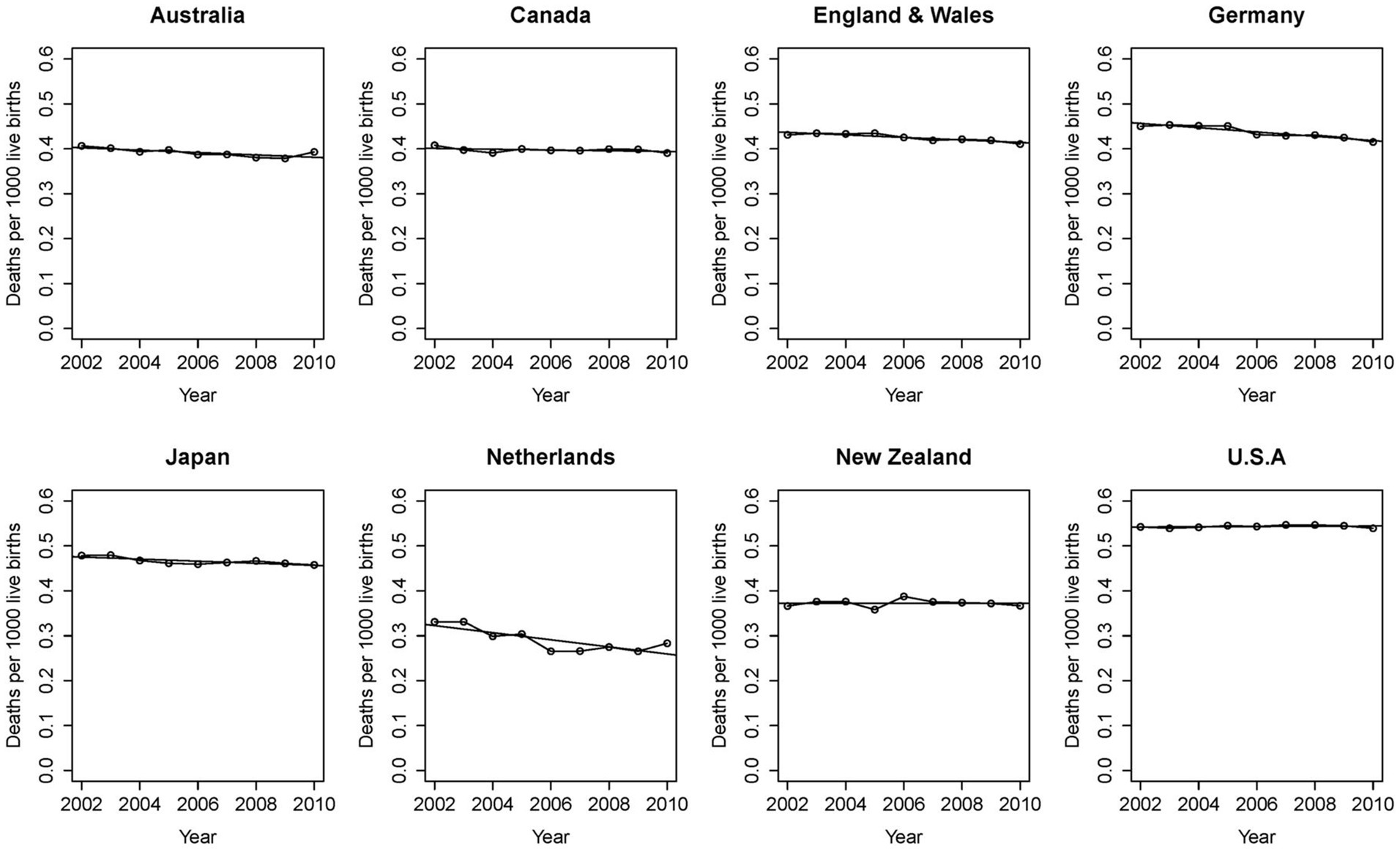
Figure 6. International data shows stalled progress against unexplained mortality. Recommendations for supine, uncluttered, “safer” sleep have been universally embraced for decades. Despite refinements in understanding associated risk in the infant sleep environment, reports from advanced industrial economies fail to demonstrate progress. With permission from Taylor et al. (88).
The same factors leading to the proposed hypothesis also motivate a re-evaluation of the Triple Risk Theory. The convergence of individual vulnerability, environment, and child development can no longer be understood as a uniform mechanism or a continuum balancing the relative contributions of intrinsic vulnerability and extrinsic risk (95). There is no a priori relationship between extrinsic, intrinsic, and developmental risk. The proposed phenotyping approach encompasses an infant discovered prone and wedged on a couch (endophenotypic feature: circumstances for inescapable suffocation without conclusive biomarker), an infant found supine amidst inconclusive sleep environment risks with lowered serotonin binding in medullary raphé nuclei (endophenotypic feature: serotonin), a toddler with a history of febrile seizures found prone with bilamination of the dentate gyrus (endophenotypic feature: epilepsy in situ), and an older child found on autopsy to have an epilepsy-related variant in sodium channels discovered in the prone position (endophenotypic feature: SUDEP without epilepsy). We propose that these deaths are not from the same cause and, moreover, that they present testable hypotheses.
The apparent “mortality gap” reported under 1 month of age also bears scrutiny. The difficult process of labor and delivery followed by an infant’s transition from placenta to independent breathing and circulation—50% of stillbirths occur during labor—may be falsely removed from our considerations. This makes it appear that there is a sparing when in fact the stress of delivery is unlike any challenge an infant or adult will face in their lifetimes and may claim an oversized amount of related mortality. Further complicating the accounting is that once in the NICU, infant deaths are not classified as SIDS, and infants who might otherwise die of SIDS do not. This is not because sudden and unexpected postnatal collapse does not occur; rather, these events either result in survival due to life-saving medical interventions, or in death that is not classified as SIDS. Similarly, possible relationships with preterm deaths and deaths due to congenital anomalies may share commonalities, where deaths occurring in the setting of extreme prematurity or congenital anomalies – which often occur within the first month of life—are not typically thought of or classified as SIDS-related mortality. Indeed, data to understand survival from what otherwise might be considered SIDS is entirely lacking. We point out that dramatic declines in neonatal mortality attributed to advances in neonatal intensive care occurred in parallel with the mortality improvements in SIDS (96).
One additional concept which may be of relevance is that of overall physiologic resilience, where death is a consequence of events that typically would have encountered sufficient homeostatic buffering but in the setting of reduced reserve are unopposed and lethal. This might be imagined to be the mirror image of geriatric vulnerability where the blunting of multiple physiologic responses can lead to unanticipated fatality with apparently minor events or intercurrent illnesses. Importantly, there are some very direct correlates with specific organ systems contributing disproportionately to this reserve. In a pediatric cohort, the failure to build resilience at the relevant rate in these same systems might represent a critical endophenotype, and also is aligned with the observations of SUDP risk in premature infants. What is clear is that current assumptions about the unique attributes of mortality under 10 years leave much to be explored.
This new approach will bring additional metrics for success that go beyond national mortality statistics or parenting practices, if only by incorporating the evaluation of these deaths into clinical medicine, bringing diagnostic and support resources and also accountability to provide an explanation to the child’s family to medicinal practice. Diagnostic yield, the impact of the multidisciplinary evaluation on family health, and the impact on healthcare utilization become newly relevant. Importantly, the uniformity of family assessment may also help destigmatize what is a remarkably challenging investigatory period. The effect of an agreed strategy on the understandable but disproportionate impact of potential infanticide and access to cases may be salutary.
There are several limitations that are unique to genomic analysis and disease discovery in this area. Variant interpretation is a particular challenge, in part due to limited phenotype information. Whereas variants of uncertain significance in living individuals may be resolved through additional clinical investigation or functional evaluation, this is typically not possible for variants identified post-mortem, where samples for functional studies do not exist and it is impossible to know if the decedent would ever develop disease manifestations typically associated with the gene in question. Aside from these phenotyping challenges that limit the clinical interpretation of variants identified, the potential for incomplete penetrance—as seen in genetic cardiomyopathies, cardiac channelopathies, and genetic epilepsy syndromes—presents a barrier to the use of population frequency in attributing variant pathogenicity. For example, multiple individuals in the same family may harbor a pathogenic variant in a cardiomyopathy gene, though the disease manifestation may range from asymptomatic to perinatal lethal. Thus, a traditional approach to Mendelian genomic analysis for early-onset rare disease, where conditions are assumed to be rare and highly penetrant, may not be successful in the majority of cases of SIDS.
A frequently stated concern is the possibility of making postmortem diagnoses we are unable to prevent. There is the additional probability of determining causes for which there is no prevention or treatment. While this reservation can be stated out of concern for families, it must be remembered that in current practice, families after SIDS must make their accommodations to the unknown in the absence of actual facts. If the concerns are for the knowledge base for the sound practice of medicine, the only way to gain information is to do more.
Many diseases remain of unknown cause and, when known, their causes can be complex and involve the interplay of numerous factors, both intrinsic and extrinsic (97). One sobering consequence of the typical approach to SIDS has been to deem it a failure by parents to heed advice. We submit an alternative view: there is a large reservoir of unexplained pediatric mortality that has defied previous efforts by medical science to understand and ameliorate it. Progress will require a different rationale. We therefore introduce a novel approach to phenotyping SUDP, taking into consideration recent genomic advances that have illuminated contributions not previously suspected, while not discounting epidemiologic observations. We believe new objective tools bring us to a threshold for discovery, one promising answers and solace for more families who experience the heartbreaking tragedy of SUDP and must live with evaluations that can seem incomplete. We believe this will provide the critical means to better understand which children are at risk, begin to screen for genetic and/or biological factors, and thus begin a new epoch of prevention.
Data availability statement
The original contributions presented in the study are included in the article/supplementary material, further inquiries can be directed to the corresponding author.
Author contributions
MW and RG drafted the work. MW, AP, IH, CM, and RG made substantial contributions to the conception or design of the work, revised the work critically for important intellectual content, provided approval for publication of the content, and agreed to be accountable for all aspects of the work in ensuring that questions related to the accuracy or integrity of any part of the work are appropriately investigated and resolved. All authors contributed to the article and approved the submitted version.
Funding
MW was supported by K23 HD102589. RG, AP, and IH were supported by the Robert’s Program Fund at Boston Children’s Hospital.
Acknowledgments
We thank the families who have generously participated in our research and who continue to inform and inspire our work.
Conflict of interest
The authors declare that the research was conducted in the absence of any commercial or financial relationships that could be construed as a potential conflict of interest.
Publisher’s note
All claims expressed in this article are solely those of the authors and do not necessarily represent those of their affiliated organizations, or those of the publisher, the editors and the reviewers. Any product that may be evaluated in this article, or claim that may be made by its manufacturer, is not guaranteed or endorsed by the publisher.
Author disclaimer
The content is solely the responsibility of the authors and does not necessarily represent the official views of the National Institutes of Health.
References
1. Underlying Cause of Death 1999-2020, As compiled from data provided by the 57 vital statistics jurisdictions through the vital statistics cooperative program. (2022). Available at: http://wonder.cdc.gov/ucd-icd10.html (Accessed 16 February 2022).
2. Goldstein, RD, Blair, PS, Sens, MA, Shapiro-Mendoza, CK, Krous, HF, Rognum, TO, et al. Inconsistent classification of unexplained sudden deaths in infants and children hinders surveillance, prevention and research: recommendations from the 3rd international congress on sudden infant and child death. Forensic Sci Med Pathol. (2019) 15:622–8. doi: 10.1007/s12024-019-00156-9
3. Malloy, MH, and MacDorman, M. Changes in the classification of sudden unexpected infant deaths: United States, 1992-2001. Pediatrics. (2005) 115:1247–53. doi: 10.1542/peds.2004-2188
4. Bundock, EA, Corey, TS, Andrew, TA, Crandall, LG, Eason, EA, et al. Unexplained Pediatric Deaths: Investigation, Certification, and Family Needs. San Diego (CA): Academic Forensic Pathology. (2019).
5. National Vital Statistics System, Linked birth/infant deaths on CDC WONDER online database. Data are from the linked birth/infant deaths records 2017-2020, as compiled from data provided by the 57 vital statistics jurisdictions the the vital statistics cooperative program. (2022). Available at: http://wonder.cdc.gov/ldb-crrent-expanded.html (Accessed 1 January 2023).
6. Bergman, AB, Beckwith, JB, and Ray, CG. Sudden infant death syndrome: Proceedings of the second international conference on causes of sudden death in infants. Seattle: University of Washington Press (1970).
7. Willinger, M, James, LS, and Catz, C. Defining the sudden infant death syndrome (SIDS): deliberations of an expert panel convened by the National Institute of Child Health and Human Development. Pediatr Pathol. (1991) 11:677–84. doi: 10.3109/15513819109065465
8. Tan, L, and Byard, RW. An analysis of the use of standard SIDS definitions in the English language literature over a three-year period (2019-2021). Acta Paediatr. (2022) 111:1019–22. doi: 10.1111/apa.16266
9. Goldstein, RD, Nields, HM, and Kinney, HC. A new approach to the investigation of sudden unexpected death. Pediatrics. (2017) 140:e20170024. doi: 10.1542/peds.2017-0024
10. Krous, HF, Beckwith, JB, Byard, RW, Rognum, TO, Bajanowski, T, Corey, T, et al. Sudden infant death syndrome and unclassified sudden infant deaths: a definitional and diagnostic approach. Pediatrics. (2004) 114:234–8. doi: 10.1542/peds.114.1.234
11. Shapiro-Mendoza, CK, Tomashek, KM, Anderson, RN, and Wingo, J. Recent national trends in sudden, unexpected infant deaths: more evidence supporting a change in classification or reporting. Am J Epidemiol. (2006) 163:762–9. doi: 10.1093/aje/kwj117
12. Werne, J, and Garrow, I. Sudden deaths of infants allegedly due to mechanical suffocation. Am J Public Health Nations Health. (1947) 37:675–87. doi: 10.2105/AJPH.37.6.675
13. Naeye, RL. Brain-stem and adrenal abnormalities in the sudden-infant-death syndrome. Am J Clin Pathol. (1976) 66:526–30. doi: 10.1093/ajcp/66.3.526
14. Naeye, RL, Whalen, P, Ryser, M, and Fisher, R. Cardiac and other abnormalities in the sudden infant death syndrome. Am J Pathol. (1976) 82:1–8.
15. Krous, HF, Haas, EA, Chadwick, AE, Masoumi, H, and Stanley, C. Intrathoracic petechiae in SIDS: a retrospective population-based 15-year study. Forensic Sci Med Pathol. (2008) 4:234–9. doi: 10.1007/s12024-008-9054-8
16. Rognum, TO, and Saugstad, OD. Hypoxanthine levels in vitreous humor: evidence of hypoxia in most infants who died of sudden infant death syndrome. Pediatrics. (1991) 87:306–10. doi: 10.1542/peds.87.3.306
17. Carpenter, KH, Bonham, JR, Worthy, E, and Variend, S. Vitreous humour and cerebrospinal fluid hypoxanthine concentration as a marker of pre-mortem hypoxia in SIDS. J Clin Pathol. (1993) 46:650–3. doi: 10.1136/jcp.46.7.650
18. Byrne, AB, Arts, P, Ha, TT, Kassahn, KS, Pais, LS, O'Donnell-Luria, A, et al. Genomic autopsy to identify underlying causes of pregnancy loss and perinatal death. Nat Med. (2023) 29:180–9. doi: 10.1038/s41591-022-02142-1
19. Wojcik, MH, Brodsky, D, Stewart, JE, and Picker, J. Peri-mortem evaluation of infants who die without a diagnosis: focus on advances in genomic technology. J Perinatol. (2018) 38:1125–34. doi: 10.1038/s41372-018-0187-7
20. Io, M. Medicolegal death investigation system: Workshop summary. Washington, DC: The National Academies Press (2003). 85 p.
21. Duncan, J. R., and Byard, R. W.. SIDS sudden infant and early childhood death: The past, the present and the future. Adelaide (Aus): University of Adelaide Press. (2018).
22. Cohen, MC. Investigation of sudden infant death syndrome. New York, NY: Cambridge University Press (2019).
23. Sens, MA, and Hughes, R. Diagnostic pathology: Forensic autopsy. 1st ed. Salt Lake City: Elsevier (2020).
24. Government H. Child death review: Statutory and operational guidance (England) London (2018). Available at: https://www.gov.uk/government/publications/child-death-review-statutory-and-operational-guidance-england.
25. Sheppard, MN. Sudden unexpected death: a national programme which will establish genetic testing and cardiological screening of families in the UK. Pathologie. (2022) 43:137–40. doi: 10.1007/s00292-022-01143-8
26. Tang, Y, Stahl-Herz, J, and Sampson, BA. Molecular diagnostics of cardiovascular diseases in sudden unexplained death. Cardiovasc Pathol. (2014) 23:1–4. doi: 10.1016/j.carpath.2013.09.002
27. Hoffman, HJ, Damus, K, Hillman, L, and Krongrad, E. Risk factors for SIDS. Results of the National Institute of Child Health and Human Development SIDS cooperative epidemiological study. Ann N Y Acad Sci. (1988) 533:13–30. doi: 10.1111/j.1749-6632.1988.tb37230.x
29. Engelberts, AC, and de Jonge, GA. Choice of sleeping position for infants: possible association with cot death. Arch Dis Child. (1990) 65:462–7. doi: 10.1136/adc.65.4.462
30. Carpenter, RG, Irgens, LM, Blair, PS, England, PD, Fleming, P, Huber, J, et al. Sudden unexplained infant death in 20 regions in Europe: case control study. Lancet. (2004) 363:185–91. doi: 10.1016/S0140-6736(03)15323-8
31. Fleming, PJ, Blair, PS, Bacon, C, Bensley, D, Smith, I, Taylor, E, et al. Environment of infants during sleep and risk of the sudden infant death syndrome: results of 1993-5 case-control study for confidential inquiry into stillbirths and deaths in infancy. Confidential Enquiry into Stillbirths and Deaths Regional Coordinators and Researchers. BMJ. (1996) 313:191–5. doi: 10.1136/bmj.313.7051.191
32. Hauck, FR, Herman, SM, Donovan, M, Iyasu, S, Merrick Moore, C, Donoghue, E, et al. Sleep environment and the risk of sudden infant death syndrome in an urban population: the Chicago infant mortality study. Pediatrics. (2003) 111:1207–14. doi: 10.1542/peds.111.S1.1207
33. Moon, RY, Carlin, RF, and Hand, I. Evidence base for 2022 updated recommendations for a safe infant sleeping environment to reduce the risk of sleep-related infant deaths. Pediatrics. (2022) 150:e2022057991. doi: 10.1542/peds.2022-057991
34. Goldstein, RD, Trachtenberg, FL, Sens, MA, Harty, BJ, and Kinney, HC. Overall Postneonatal mortality and rates of SIDS. Pediatrics. (2016) 137:e20152298. doi: 10.1542/peds.2015-2298
35. Schlaud, M, Eberhard, C, Trumann, B, Kleemann, WJ, Poets, CF, Tietze, KW, et al. Prevalence and determinants of prone sleeping position in infants: results from two cross-sectional studies on risk factors for SIDS in Germany. Am J Epidemiol. (1999) 150:51–7. doi: 10.1093/oxfordjournals.aje.a009918
36. Poets, CF, and Southall, DP. Recent developments in research into sudden infant death. Thorax. (1994) 49:196–7. doi: 10.1136/thx.49.3.196
37. Sridhar, R, Thach, BT, Kelly, DH, and Henslee, JA. Characterization of successful and failed autoresuscitation in human infants, including those dying of SIDS. Pediatr Pulmonol. (2003) 36:113–22. doi: 10.1002/ppul.10287
38. Kinney, HC, and Thach, BT. The sudden infant death syndrome. N Engl J Med. (2009) 361:795–805. doi: 10.1056/NEJMra0803836
39. Schechtman, VL, Harper, RM, Kluge, KA, Wilson, AJ, Hoffman, HJ, and Southall, DP. Heart rate variation in normal infants and victims of the sudden infant death syndrome. Early Hum Dev. (1989) 19:167–81. doi: 10.1016/0378-3782(89)90077-7
40. Kinney, HC, and Haynes, RL. The serotonin brainstem hypothesis for the sudden infant death syndrome. J Neuropathol Exp Neurol. (2019) 78:765–79. doi: 10.1093/jnen/nlz062
41. Buchanan, GF, and Richerson, GB. Central serotonin neurons are required for arousal to CO2. Proc Natl Acad Sci U S A. (2010) 107:16354–9. doi: 10.1073/pnas.1004587107
42. Buchanan, GF, Smith, HR, MacAskill, A, and Richerson, GB. 5-HT2A receptor activation is necessary for CO2-induced arousal. J Neurophysiol. (2015) 114:233–43. doi: 10.1152/jn.00213.2015
43. Duncan, JR, Paterson, DS, Hoffman, JM, Mokler, DJ, Borenstein, NS, Belliveau, RA, et al. Brainstem serotonergic deficiency in sudden infant death syndrome. JAMA. (2010) 303:430–7. doi: 10.1001/jama.2010.45
44. Paterson, DS, Trachtenberg, FL, Thompson, EG, Belliveau, RA, Beggs, AH, Darnall, R, et al. Multiple serotonergic brainstem abnormalities in sudden infant death syndrome. JAMA. (2006) 296:2124–32. doi: 10.1001/jama.296.17.2124
45. Randall, BB, Paterson, DS, Haas, EA, Broadbelt, KG, Duncan, JR, Mena, OJ, et al. Potential asphyxia and brainstem abnormalities in sudden and unexpected death in infants. Pediatrics. (2013) 132:e1616–25. doi: 10.1542/peds.2013-0700
46. Dosumu-Johnson, RT, Cocoran, AE, Chang, Y, Nattie, E, and Dymecki, SM. Acute perturbation of Pet1-neuron activity in neonatal mice impairs cardiorespiratory homeostatic recovery. elife. (2018) 7:7. doi: 10.7554/eLife.37857
47. Cummings, KJ, Commons, KG, Hewitt, JC, Daubenspeck, JA, Li, A, Kinney, HC, et al. Failed heart rate recovery at a critical age in 5-HT-deficient mice exposed to episodic anoxia: implications for SIDS. J Appl Physiol. (2011) 111:825–33. doi: 10.1152/japplphysiol.00336.2011
48. Filiano, JJ, and Kinney, HC. A perspective on neuropathologic findings in victims of the sudden infant death syndrome: the triple-risk model. Biol Neonate. (1994) 65:194–7. doi: 10.1159/000244052
49. Rognum, TO, and Saugstad, OD. Biochemical and immunological studies in SIDS victims. clues to understanding the death mechanism. Acta Paediatr Suppl. (1993) 82:82–5. doi: 10.1111/j.1651-2227.1993.tb12886.x
50. Guntheroth, WG, and Spiers, PS. The triple risk hypotheses in sudden infant death syndrome. Pediatrics. (2002) 110:e64. doi: 10.1542/peds.110.5.e64
51. Anderson, TM, Lavista Ferres, JM, Ren, SY, Moon, RY, Goldstein, RD, Ramirez, JM, et al. Maternal smoking before and during pregnancy and the risk of sudden unexpected infant death. Pediatrics. (2019) 143:e20183325. doi: 10.1542/peds.2018-3325
52. Iyasu, S, Randall, LL, Welty, TK, Hsia, J, Kinney, HC, Mandell, F, et al. Risk factors for sudden infant death syndrome among northern plains Indians. JAMA. (2002) 288:2717–23. doi: 10.1001/jama.288.21.2717
53. Trachtenberg, FL, Haas, EA, Kinney, HC, Stanley, C, and Krous, HF. Risk factor changes for sudden infant death syndrome after initiation of Back-to-sleep campaign. Pediatrics. (2012) 129:630–8. doi: 10.1542/peds.2011-1419
54. Godoy, M, and Maher, M. A ten-year retrospective case review of risk factors associated with sleep-related infant deaths. Acta Paediatr. (2022) 111:1176–85. doi: 10.1111/apa.16281
55. Krous, HF, Haas, EA, Masoumi, H, Chadwick, AE, and Stanley, C. A comparison of pulmonary intra-alveolar hemorrhage in cases of sudden infant death due to SIDS in a safe sleep environment or to suffocation. Forensic Sci Int. (2007) 172:56–62. doi: 10.1016/j.forsciint.2006.12.005
56. Goldstein, RD, Kinney, HC, and Guttmacher, AE. Only Halfway there with sudden infant death syndrome. N Engl J Med. (2022) 386:1873–5. doi: 10.1056/NEJMp2119221
57. Parks, SE, DeSisto, CL, Kortsmit, K, Bombard, JM, and Shapiro-Mendoza, CK. Risk factors for suffocation and unexplained causes of infant deaths. Pediatrics. (2023) 151:e2022057771. doi: 10.1542/peds.2022-057771
58. Adolph, KE, and Franchak, JM. The development of motor behavior. Wiley Interdiscip rev. Cogn Sci. (2017) 8:e1430. doi: 10.1002/wcs.1430
59. Bagnall, RD, Das, KJ, Duflou, J, and Semsarian, C. Exome analysis-based molecular autopsy in cases of sudden unexplained death in the young. Heart Rhythm. (2014) 11:655–62. doi: 10.1016/j.hrthm.2014.01.017
60. Tseng, ZH, Olgin, JE, Vittinghoff, E, Ursell, PC, Kim, AS, Sporer, K, et al. Prospective countywide surveillance and autopsy characterization of sudden cardiac death: POST SCD study. Circulation. (2018) 137:2689–700. doi: 10.1161/CIRCULATIONAHA.117.033427
61. Nashef, L. Sudden unexpected death in epilepsy: terminology and definitions. Epilepsia. (1997) 38:S6–8. doi: 10.1111/j.1528-1157.1997.tb06130.x
62. Kinney, HC, Cryan, JB, Haynes, RL, Paterson, DS, Haas, EA, Mena, OJ, et al. Dentate gyrus abnormalities in sudden unexplained death in infants: morphological marker of underlying brain vulnerability. Acta Neuropathol. (2015) 129:65–80. doi: 10.1007/s00401-014-1357-0
63. Hefti, MM, Cryan, JB, Haas, EA, Chadwick, AE, Crandall, LA, Trachtenberg, FL, et al. Hippocampal malformation associated with sudden death in early childhood: a neuropathologic study: part 2 of the investigations of the San Diego SUDC research project. Forensic Sci Med Pathol. (2016) 12:14–25. doi: 10.1007/s12024-015-9731-3
64. Kinney, HC, Poduri, AH, Cryan, JB, Haynes, RL, Teot, L, Sleeper, LA, et al. Hippocampal formation Maldevelopment and sudden unexpected death across the pediatric age Spectrum. J Neuropathol Exp Neurol. (2016) 75:981–97. doi: 10.1093/jnen/nlw075
65. Zhan, Q, Buchanan, GF, Motelow, JE, Andrews, J, Vitkovskiy, P, Chen, WC, et al. Impaired serotonergic brainstem function during and after seizures. J Neurosci. (2016) 36:2711–22. doi: 10.1523/JNEUROSCI.4331-15.2016
66. Bonardi, CM, Heyne, HO, Fiannacca, M, Fitzgerald, MP, Gardella, E, Gunning, B, et al. KCNT1-related epilepsies and epileptic encephalopathies: phenotypic and mutational spectrum. Brain. (2021) 144:3635–50. doi: 10.1093/brain/awab219
67. Denti, F, Bentzen, BH, Wojciak, J, Thomsen, NM, Scheinman, M, and Schmitt, N. Multiple genetic variations in sodium channel subunits in a case of sudden infant death syndrome. Pacing Clin Electrophysiol. (2018) 41:620–6. doi: 10.1111/pace.13328
68. Keywan, C, Holm, IA, Poduri, A, Brownstein, CA, Alexandrescu, S, Chen, J, et al. A de novo BRPF1 variant in a case of sudden unexplained death in childhood. Eur J Med Genet. (2020) 63:104002. doi: 10.1016/j.ejmg.2020.104002
69. Halvorsen, M, Petrovski, S, Shellhaas, R, Tang, Y, Crandall, L, Goldstein, D, et al. Mosaic mutations in early-onset genetic diseases. Genet Med. (2015) 18:746–9. doi: 10.1038/gim.2015.155
70. Brownstein, C, Goldstein, RD, Thompson, C, Haynes, RL, Giles, E, Sheidley, B, et al. SCN1A variants associated with sudden infant death syndrome. Epilepsia. (2018) 59:e56–62. doi: 10.1111/epi.14055
71. Neubauer, J, Lecca, MR, Russo, G, Bartsch, C, Medeiros-Domingo, A, Berger, W, et al. Post-mortem whole-exome analysis in a large sudden infant death syndrome cohort with a focus on cardiovascular and metabolic genetic diseases. Eur J Hum Genet. (2017) 25:404–9. doi: 10.1038/ejhg.2016.199
72. Heyne, HO, Singh, T, Stamberger, H, Abou Jamra, R, Caglayan, H, Craiu, D, et al. De novo variants in neurodevelopmental disorders with epilepsy. Nat Genet. (2018) 50:1048–53. doi: 10.1038/s41588-018-0143-7
73. Koh, HY, Haghighi, A, Keywan, C, Alexandrescu, S, Plews-Ogan, E, Haas, EA, et al. Genetic determinants of sudden unexpected death in pediatrics. Genet Med. (2022) 24:839–50. doi: 10.1016/j.gim.2021.12.004
74. Halvorsen, M, Gould, L, Wang, X, Grant, G, Moya, R, Rabin, R, et al. De novo mutations in childhood cases of sudden unexplained death that disrupt intracellular Ca. Proc Natl Acad Sci U S A. (2021) 118:e2115140118. doi: 10.1073/pnas.2115140118
75. Rueda, M, Wagner, JL, Phillips, TC, Topol, SE, Muse, ED, Lucas, JR, et al. Molecular autopsy for sudden death in the young: is data aggregation the key? Front Cardiovasc Med. (2017) 4:72. doi: 10.3389/fcvm.2017.00072
76. Schwartz, PJ, Stramba-Badiale, M, Segantini, A, Austoni, P, Bosi, G, Giorgetti, R, et al. Prolongation of the QT interval and the sudden infant death syndrome. N Engl J Med. (1998) 338:1709–14. doi: 10.1056/NEJM199806113382401
77. Kingdom, R, and Wright, CF. Incomplete penetrance and variable expressivity: from clinical studies to population cohorts. Front Genet. (2022) 13:920390. doi: 10.3389/fgene.2022.920390
78. Isbister, JC, Nowak, N, Yeates, L, Singer, ES, Sy, RW, Ingles, J, et al. Concealed cardiomyopathy in autopsy-inconclusive cases of sudden cardiac death and implications for families. J Am Coll Cardiol. (2022) 80:2057–68. doi: 10.1016/j.jacc.2022.09.029
79. Wojcik, MH, Stadelmaier, R, Heinke, D, Holm, IA, Tan, WH, and Agrawal, PB. The unrecognized mortality burden of genetic disorders in infancy. Am J Public Health. (2021) 111:S156–62. doi: 10.2105/AJPH.2021.306275
80. Stanley, KE, Giordano, J, Thorsten, V, Buchovecky, C, Thomas, A, Ganapathi, M, et al. Causal genetic variants in stillbirth. N Engl J Med. (2020) 383:1107–16. doi: 10.1056/NEJMoa1908753
81. Stampe, NK, Glinge, C, Jabbari, R, Bjune, T, Risgaard, B, Tfelt-Hansen, J, et al. Febrile seizures prior to sudden cardiac death: a Danish nationwide study. Europace. (2018) 20:f192–7. doi: 10.1093/europace/eux335
82. Holm, IA, Poduri, A, Crandall, L, Haas, E, Grafe, MR, Kinney, HC, et al. Inheritance of febrile seizures in sudden unexplained death in toddlers. Pediatr Neurol. (2012) 46:235–9. doi: 10.1016/j.pediatrneurol.2012.02.007
83. Smith, GC, Wood, AM, Pell, JP, White, IR, Crossley, JA, and Dobbie, R. Second-trimester maternal serum levels of alpha-fetoprotein and the subsequent risk of sudden infant death syndrome. N Engl J Med. (2004) 351:978–86. doi: 10.1056/NEJMoa040963
84. Gottesman, II, and Gould, TD. The endophenotype concept in psychiatry: etymology and strategic intentions. Am J Psychiatry. (2003) 160:636–45. doi: 10.1176/appi.ajp.160.4.636
85. Wojcik, MH, Schwartz, TS, Yamin, I, Edward, HL, Genetti, CA, Towne, MC, et al. Genetic disorders and mortality in infancy and early childhood: delayed diagnoses and missed opportunities. Genet Med. (2018) 20:1396–404. doi: 10.1038/gim.2018.17
86. Wojcik, MH, Schwartz, TS, Thiele, KE, Paterson, H, Stadelmaier, R, Mullen, TE, et al. Infant mortality: the contribution of genetic disorders. J Perinatol. (2019) 39:1611–9. doi: 10.1038/s41372-019-0451-5
87. Owen, MJ, Wright, MS, Batalov, S, Kwon, Y, Ding, Y, Chau, KK, et al. Reclassification of the etiology of infant mortality with whole-genome sequencing. JAMA Netw Open. (2023) 6:e2254069. doi: 10.1001/jamanetworkopen.2022.54069
88. Taylor, BJ, Garstang, J, Engelberts, A, Obonai, T, Cote, A, Freemantle, J, et al. International comparison of sudden unexpected death in infancy rates using a newly proposed set of cause-of-death codes. Arch. Dis. Child. (2015) 100:1018–1023. doi: 10.1136/archdischild-2015-308239
89. Meadow, SR. Who's to blame--mothers, Munchausen or medicine? J R Coll Physicians Lond. (1994) 28:332–7.
90. Christensen, ED, Berger, J, Alashari, MM, Coon, H, Robison, C, Ho, HT, et al. Sudden infant death "syndrome"-insights and future directions from a Utah population database analysis. Am J Med Genet A. (2017) 173:177–82. doi: 10.1002/ajmg.a.37994
91. Badner, JA, Sieber, WK, Garver, KL, and Chakravarti, A. A genetic study of Hirschsprung disease. Am J Hum Genet. (1990) 46:568–80.
92. Chatterjee, NA. Prediction of sudden cardiac death: looking beyond ejection fraction. Curr Cardiol Rep. (2023). doi: 10.1007/s11886-023-01871-0
93. Thayyil, S, Sebire, NJ, Chitty, LS, Wade, A, Chong, W, Olsen, O, et al. Post-mortem MRI versus conventional autopsy in fetuses and children: a prospective validation study. Lancet. (2013) 382:223–33. doi: 10.1016/S0140-6736(13)60134-8
94. Leadbetter, KZ, Vesoulis, ZA, White, FV, Schmidt, RE, Khanna, G, Shimony, JS, et al. The role of post-mortem MRI in the neonatal intensive care unit. J Perinatol. (2017) 37:98–103. doi: 10.1038/jp.2016.156
95. Hunt, CE, Darnall, RA, McEntire, BL, and Hyma, BA. Assigning cause for sudden unexpected infant death. Forensic Sci Med Pathol. (2015) 11:283–8. doi: 10.1007/s12024-014-9650-8
96. Horbar, JD, Badger, GJ, Carpenter, JH, Fanaroff, AA, Kilpatrick, S, LaCorte, M, et al. Trends in mortality and morbidity for very low birth weight infants, 1991-1999. Pediatrics. (2002) 110:143–51. doi: 10.1542/peds.110.1.143
Keywords: SUDC, SUEND, SIDS, SUDEP, genetics, molecular autopsy, sudden unexpected death in infancy (SUDI), sudden cardiac death (SCD)
Citation: Wojcik MH, Poduri AH, Holm IA, MacRae CA and Goldstein RD (2023) The fundamental need for unifying phenotypes in sudden unexpected pediatric deaths. Front. Med. 10:1166188. doi: 10.3389/fmed.2023.1166188
Edited by:
Ramon Brugada, University of Girona, SpainReviewed by:
Marta Cohen, Sheffield Children's Hospital, United KingdomCristoforo Pomara, University of Catania, Italy
Copyright © 2023 Wojcik, Poduri, Holm, MacRae and Goldstein. This is an open-access article distributed under the terms of the Creative Commons Attribution License (CC BY). The use, distribution or reproduction in other forums is permitted, provided the original author(s) and the copyright owner(s) are credited and that the original publication in this journal is cited, in accordance with accepted academic practice. No use, distribution or reproduction is permitted which does not comply with these terms.
*Correspondence: Richard D. Goldstein, cmljaGFyZC5nb2xkc3RlaW5AY2hpbGRyZW5zLmhhcnZhcmQuZWR1