- Hamad Medical Corporation, Doha, Qatar
Tyrosine kinase inhibitors (TKIs) have revolutionized the treatment of chronic myeloid leukemia (CML) but their use was associated with a range of serious cardiopulmonary toxicities including vascular adverse events, QT prolongation, heart failure, pleural effusion, and pulmonary arterial hypertension. Dedicated clinical management guidelines for TKI-induced toxicities are not available. This review aims to discuss TKI-associated cardiopulmonary toxicities and proposes a practical guide for their management.
Introduction
Protein kinases are enzymes involved in different signaling pathways and regulate various cellular functions such as proliferation, differentiation, and death (1, 2). Thus, carcinogenesis can be triggered by disturbances in such processes. Kinase inhibitors have brought about a paradigm shift in the treatment of many diverse malignancies (1). Chronic myeloid leukemia (CML), a rare myeloproliferative disease, is associated with chromosomal translocation (i.e., Philadelphia chromosome), which encodes BCR::ABL1 oncoprotein, through the fusion of BCR and ABL1 genes, with active tyrosine kinase activity (3–6). Thus, tyrosine kinase inhibitors (TKIs) are currently the cornerstone treatment of CML (6). TKIs are categorized into small-molecule TKIs and monoclonal antibody drugs. The latter group inhibits proliferation, angiogenesis, and invasion of tumor cells. The former group is less selective than the other one and may cause more adverse events. Different TKIs, namely imatinib, dasatinib, nilotinib, bosutinib, and ponatinib, are approved in Europe and the United States for the treatment of CML. Although the five TKIs inhibit BCR::ABL1, they differ in their targeted site, mechanisms, efficacy, and safety. Cardiovascular toxicities of TKIs have several manifestations such as heart failure, arrhythmias, and fluid retention (1, 2). However, it is hard to distinguish between the cardiovascular events caused by TKIs from the events that occur due to the patient’s risk factors (7). Vascular adverse events and pulmonary toxicities are also associated with TKI therapy (Figure 1) (3, 8). Herein, this review discusses the TKI-associated cardiopulmonary toxicities, including their mechanisms of toxicity, and proposes a practical guide to their recognition, management, and monitoring.
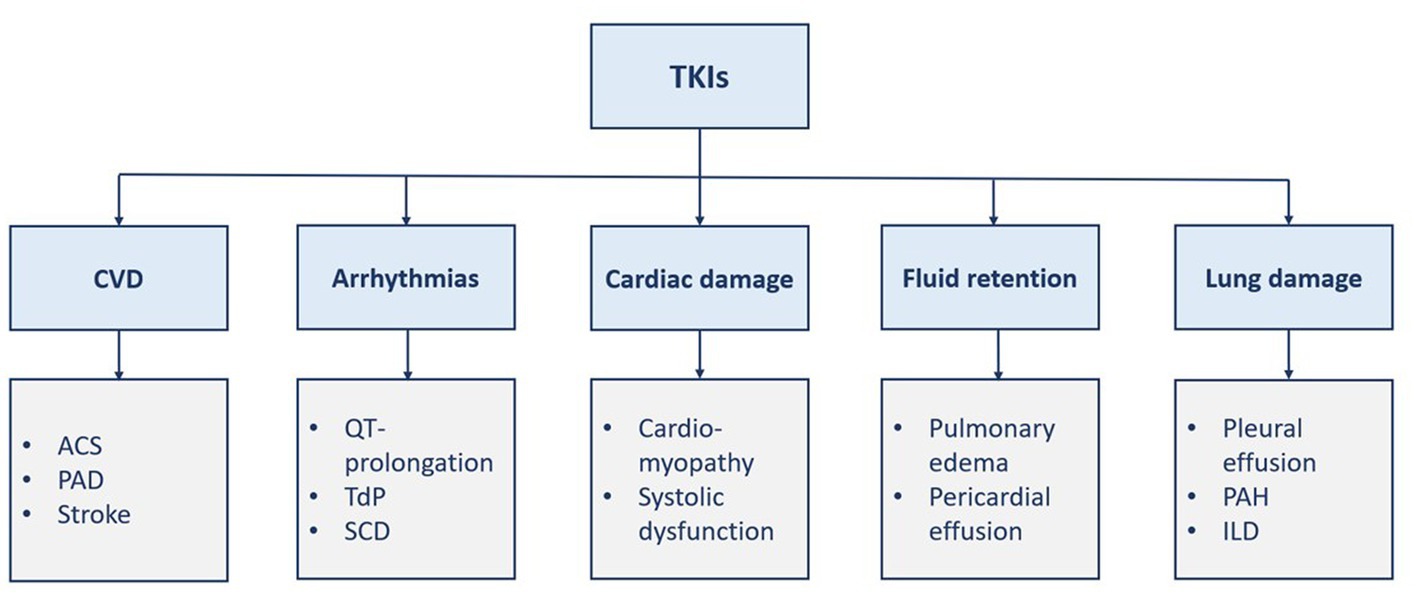
Figure 1. Manifestations of TKI-induced cardiopulmonary toxicities. ACS, acute coronary syndrome; CVD, cardiovascular disease; ILD, interstitial lung disease; PAD, Peripheral arterial disease; PAH, pulmonary arterial hypertension; SCD, sudden cardiac death; TdP, torsade de pointes; TKI, tyrosine kinase inhibitor(s).
Tyrosine kinase inhibitors
Tyrosine kinase inhibitors have revolutionized the treatment of CML with the first-generation agent, imatinib (4, 9). The subsequent more effective second-generation agents (bosutinib, dasatinib, and nilotinib) were developed to overcome imatinib resistance (4, 8, 9). However, both generations are highly resistant in the presence of BCR::ABL1T315I mutation, which leads to rapid disease progression and limited mortality improvement. Consequently, the third-generation ponatinib was developed and approved in patients with BCR::ABL1T315I mutation (6, 9). Table 1 summarizes the general characteristics of TKIs (1–3, 8, 10). The three additional third-generation agents have been investigated in TKI-resistant CML and included olverembatinib, asciminib, and vodobatinib. Olverembatinib (HQP1351) was well-tolerated and effective in TKI-resistant chronic and accelerated phase CML, particularly in the presence of BCR::ABL1T315I mutation (9). It has been granted a fast-track designation for CML in the United States (May 2020) and a breakthrough therapy designation for CML in the European Union and China (November 2021) (11). Although the first approval of asciminib was received in October 2021 (United States) for Philadelphia chromosome-positive CML, there are several ongoing trials investigating new indications as monotherapy in chronic phase CML patients with or without T315I mutation previously treated with two or more TKIs or as add-on therapy to other TKIs (12). In a dose-escalating Phase I study, vodobatinib showed encouraging efficacy in both ponatinib-treated and ponatinib-naïve patients with chronic phase CML (13). An innovative fourth-generation agent, PF-114, that structurally resembles ponatinib can inhibit wild-type and mutated BCR::ABL (14, 15). PF-114, unlike Ponatinib, does not inhibit the vascular endothelial growth factor receptor (VEGFR), hence having the benefit of reducing cardiovascular adverse effects (15). Table 2 presents the general characteristics of the novel TKIs (9, 11, 12, 15).
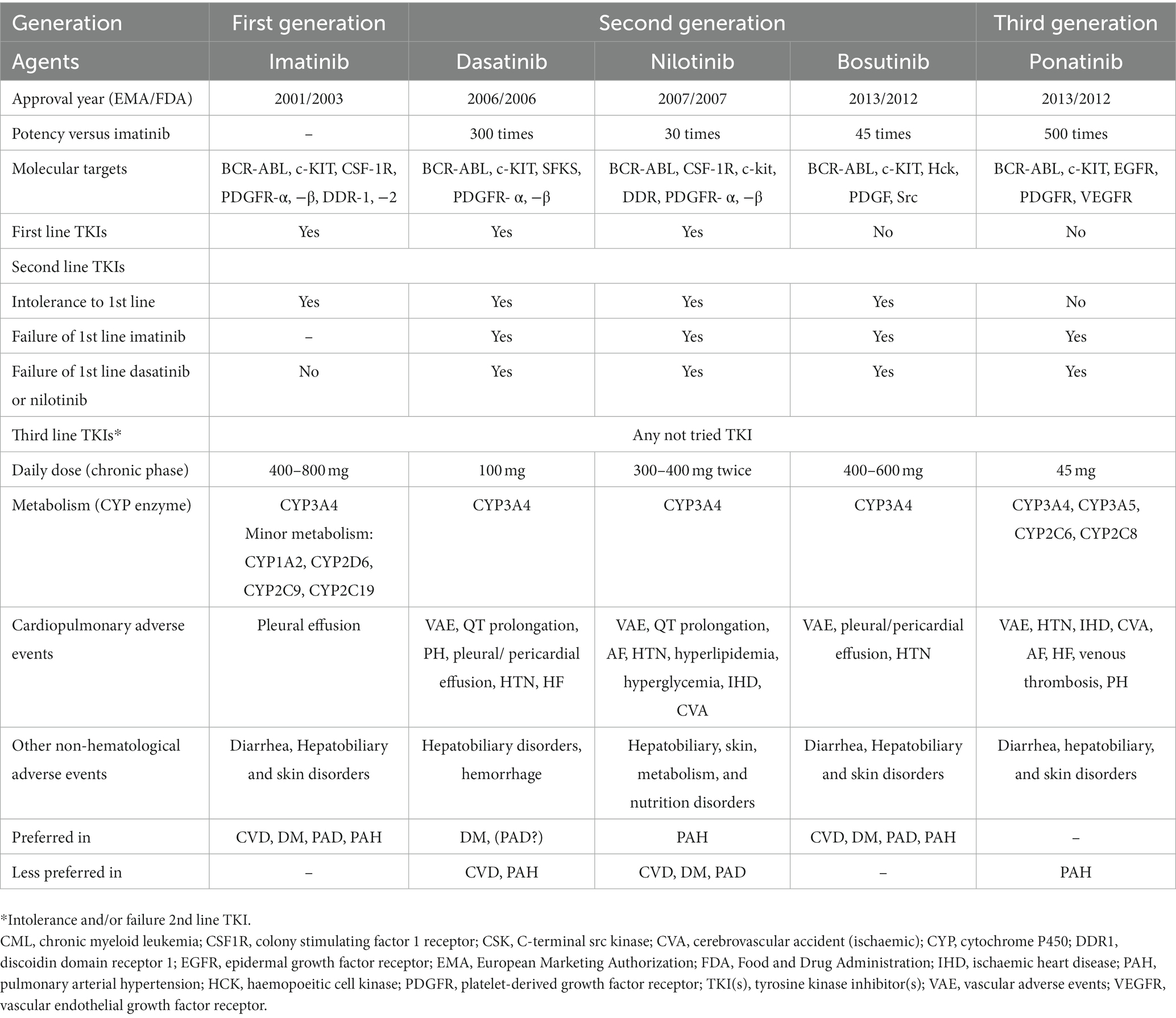
Table 1. The general characteristics of tyrosine kinase inhibitors in chronic phase chronic myeloid leukemia.
Cardiovascular toxicity
Classification and severity of cardiovascular toxicity
The types of cardiotoxicities that have been described earlier are acute, sub-chronic, early-onset chronic, and late-onset chronic cardiotoxicities. A newer classification of chemotherapy-related cardiac dysfunction (CRCD) included Type I (irreversible) and Type II (reversible) CRCD (Table 3) (1). The National Cancer Institute (NCI) developed the Common Terminology Criteria for Adverse Events (CTCAE) to ensure the consistent description and grading of cardiovascular adverse events of therapeutic agents. The severity of TKI-induced cardiovascular adverse events was also graded using NCI CTCAE (Table 4) (16, 17). Cardiovascular and pulmonary toxicities of TKIs are demonstrated in Table 5 (1–3, 5, 8, 18–21). The recent 2022 European cardio-oncology guidelines described consensus definitions for several cancer therapy-related cardiac dysfunction (CTRCD) such as heart failure and cardiomyopathy, myocarditis, cardiac arrhythmias, corrected QT interval (QTc) prolongation, vascular toxicities, and hypertension (22).
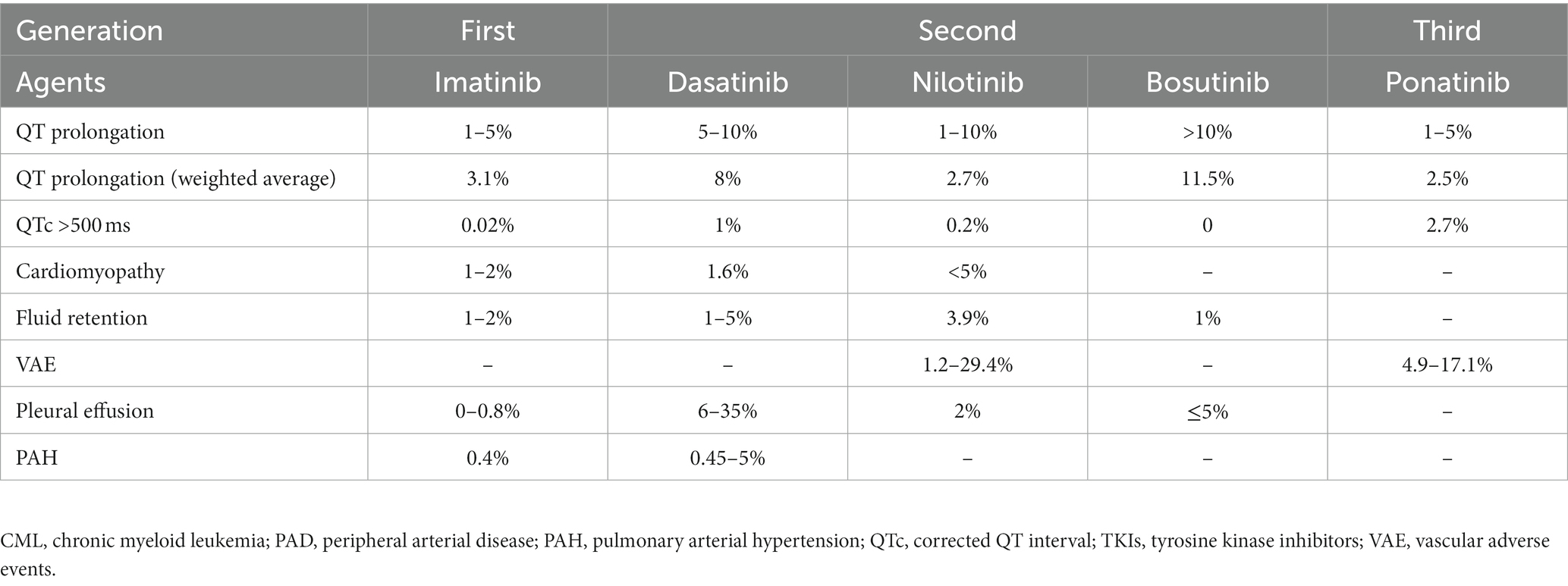
Table 5. Frequencies of cardiopulmonary toxicities of tyrosine kinase inhibitors in chronic myeloid leukemia.
Extent and consequences of TKI-induced cardiovascular toxicity
A case/non-case study using the Food and Drug Administration (FDA) adverse event reporting system (FEARS) database examined the cardiovascular adverse events reports of TKIs for the treatment of CML. Cases referred to reports with one or more of prespecified cardiovascular events such as cardiomyopathy, QT prolongation/torsade de pointes, pulmonary hypertension, or embolic events, whereas non-cases referred to any report for other not specified serious adverse events. FEARS allows the analysis of an enormous number of reports that helps in detecting safety signals. More than 1,300,000 adverse event reports, out of more than 20 million reports, were related to anticancer agents. Finally, 717,163 reports were analyzed after excluding non-serious events, duplicates, and aberrant cases. Cardiovascular events accounted for 64,232 cases, with 3,930 (6.1%) cases related to TKIs. In addition, TKIs were the suspected drugs in 83.2% of the cardiovascular event occurrence. Hospitalization and having fatal issues accounted for 35 and 10.1% of the cases. Of the 3,930 reports related to TKIs, 59, 21.2, 14.4, 4.4, and 1% were related to nilotinib, dasatinib, ponatinib, imatinib, and bosutinib, respectively, with 68.1% of the cases treated for CML. In comparison with other anticancer agents, cardiovascular events were more frequent with TKIs. The adjusted reporting odds ratio was 6.6 (95% confidence interval (95% CI): 5.6–7.8) for QT prolongation/torsade de pointes, 1.7 (95% CI: 1.4–2.1) for cardiac arrhythmias, 2.4 (95% CI: 2.2–2.6) for heart failure, and 3.9 (95% CI: 3.2–4.7) for pulmonary hypertension (6).
Wang et al. investigated the long-term follow-up of TKI use in CML patients (n = 7,119) and its association with cardiovascular toxicity using SEER (surveillance, epidemiology, and end results) program between 1992 and 2011. The program includes 18 cancer registries in the United States and covers up to approximately 28% of the American population. The authors compared patients’ outcomes in the TKI era with that of those in the pre-TKI era. CML diagnosis rate was higher in the TKI era (72.4% versus 27.6%) and was associated with significantly higher overall survival [hazard ratio (HR) 0.22; 95% CI: 0.21–0.24] than in the pre-TKI era. The probability of mortality due to cardiovascular causes was reduced in the TKI era (HR 0.72; 95% CI: 0.59–0.98) (23). Caocci et al. reported survival data of Italian patients with chronic phase CML who were on second- or third-generation TKIs (n = 656) and 15% of them had a history of cardiovascular disease. Following TKI therapy, 7.3 and 1.4% of patients experienced arterial occlusive events and ischemic heart disease, respectively. Peripheral arterial disease rate was higher with nilotinib and ponatinib (7.3 and 5.9%) than dasatinib and bosutinib (1.7 and 1.6%; p = 0.02), whereas stroke rate was higher with bosutinib and ponatinib (5 and 3%) than nilotinib and dasatinib (0.7 and 0%; p = 0.01), respectively. Death was reported in 37 patients of them 12 cases were related to cardiovascular complications. The 15-year overall and cardiovascular mortality free-survival rates were 83.3 and 93%, respectively (24). Interestingly, imatinib, but not dasatinib or nilotinib, caused a significant reduction in the estimated glomerular filtration rate on a long-term follow-up which may have contributed to the occurrence of ischemic events (25).
Mechanisms of TKI-induced cardiovascular toxicity
The mechanisms of cardiovascular toxicity are generally divided into on- and off-target mechanisms. On-target mechanisms refer to the anticancer effect of TKIs through the inhibition of a molecule in the tumor cells but can also adversely affect the function of normal cells. While off-target mechanisms imply the non-selective inhibition of other kinases in normal cells, along with the kinase of the tumor cells that cause cardiotoxicity (1, 2, 7). However, the molecular mechanism of TKI-induced cardiotoxicity is not fully elucidated (1, 2, 19). Examples of target receptors that regulate signaling pathways (e.g., PI3K, MEK, and AKT) include BCR::ABL, VEGFR, epidermal growth factor receptor (EGFR), platelet-derived growth factor receptor (PDGFR), and c-KIT. TKIs are usually multi-target agents and only a few of them have one or two targets such as bosutinib (2, 3). The off-target inhibition spectrum can range from six (nilotinib) to 28 (dasatinib) target receptors. Furthermore, the effects of this inhibition depend on the specific agent and its dosage (3). There is also between-patient variability in cardiotoxicity even when treated with the same TKI, which reflects an interaction between different factors such as TKI targets, genetic predisposition, and cardiovascular risk factors (7). A new study reported a novel mechanism for the cardiovascular toxicity caused by the second- and third-generation BCR::ABL1 TKIs through their ability to activate the Rho-associated coiled-coil-containing kinase (ROCK). ROCK activity may be a prognostic biomarker for cardiovascular adverse events and, therefore, its inhibition may be promising in preventing and/or treating cardiovascular adverse events due to the implicated TKIs (26, 27).
General approach to cardiovascular toxicity
The factors that determine the selection of a TKI comprise the goal of therapy, comorbidities, patient’s age, and the TKI’s toxicity profile (5, 28). Before deciding on the TKI therapy, cardiovascular risk factors should be evaluated. The presence of comorbidities such as coronary artery disease, hypertension, or diabetes should be closely monitored and followed up (2, 18). In addition, except for imatinib, TKIs are associated with serious cardiovascular complications (Table 1). These concerns should prompt cardiovascular risk assessment before starting TKI therapy (1, 18). Figure 2 shows the cardiovascular considerations when selecting a TKI agent (18). Given the absence of biomarkers that predict the cardiovascular risk associated with BCR::ABL1 TKI therapy, traditional risk scores derived from the general population are usually used in CML patients as well. However, such scores may underestimate the risk (27).
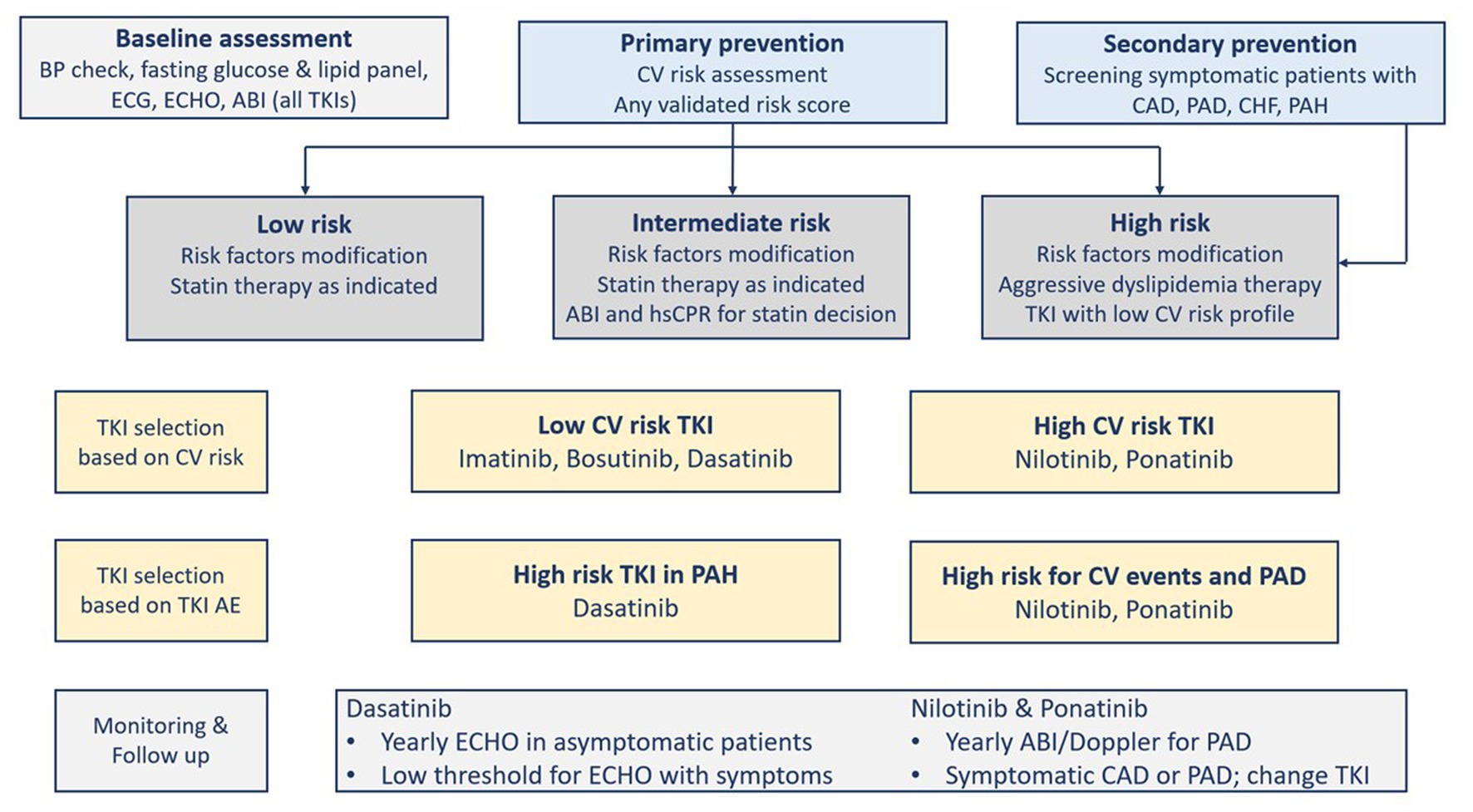
Figure 2. Cardiovascular risk assessment and tyrosine kinase inhibitors selection and monitoring. ABI, Ankle-brachial index; AE, Adverse Events; BP, blood pressure; CAD, coronary artery disease; CHF, congestive heart failure; CV, cardiovascular; ECG, electrocardiogram; ECHO, echocardiography; PAD, Peripheral arterial disease; PAH, pulmonary arterial hypertension; TKI, tyrosine kinase inhibitor(s).
The prevention of cardiovascular diseases starts with the aggressive management of modifiable cardiovascular risk factors in patients with or without CML (7). The “ABCDE” approach lists the classic steps to reduce cardiovascular risk as follows: Assessment of cardiovascular disease signs and symptoms with aspirin use in select patients; Blood pressure control; Cigarette/tobacco cessation and Cholesterol level monitoring; Diabetes control and Diet/weight management; and Exercise (18, 28). International guidelines recommend calculating a risk score (e.g., Framingham, SCORE, QRISK, and Reynolds risk scores), and if the decision to consider statin is uncertain, then other factors can be considered in decision-making, for example, coronary artery calcium score, high-sensitivity C-reactive protein, or ankle-brachial index (ABI). Although such recommendations are not validated in CML patients, they can direct proper aggressive management of the modifiable risk factors. Statin therapy is effective for primary prevention in patients at high cardiovascular risk who can tolerate the therapy, particularly those on ponatinib (7). A systematic review and meta-analysis that used molecular docking to analyze the impact of aspirin and rosuvastatin on hub genes associated with nilotinib cardiotoxicity found that rosuvastatin can be effective in this case (29). Aspirin, in primary prevention, reduces the composite of serious cardiovascular events but is associated with increased gastrointestinal and intracranial bleeding risk. Thus, it needs to balance ischemic and bleeding risks. Aspirin use is more challenging in CML patients using certain TKIs (i.e., dasatinib) because it can inhibit platelet aggregation leading to increased bleeding risk (Table 6) (7). After the baseline assessment (i.e., cardiovascular risk, physical examination, vitals, laboratory tests), there should be follow-up visits at 1, 3, and 6 months (Figure 2) (18, 28). Another approach to reducing adverse events is the use of lower TKI doses and shorter durations (8). The analysis of the German CML-Study IV (Chronic Myeloid Leukemia-Study IV) has concluded that de-escalating a higher dose of imatinib (i.e., 800 mg daily) to 400 mg daily sustained deep molecular remission in 90% of patients (30).
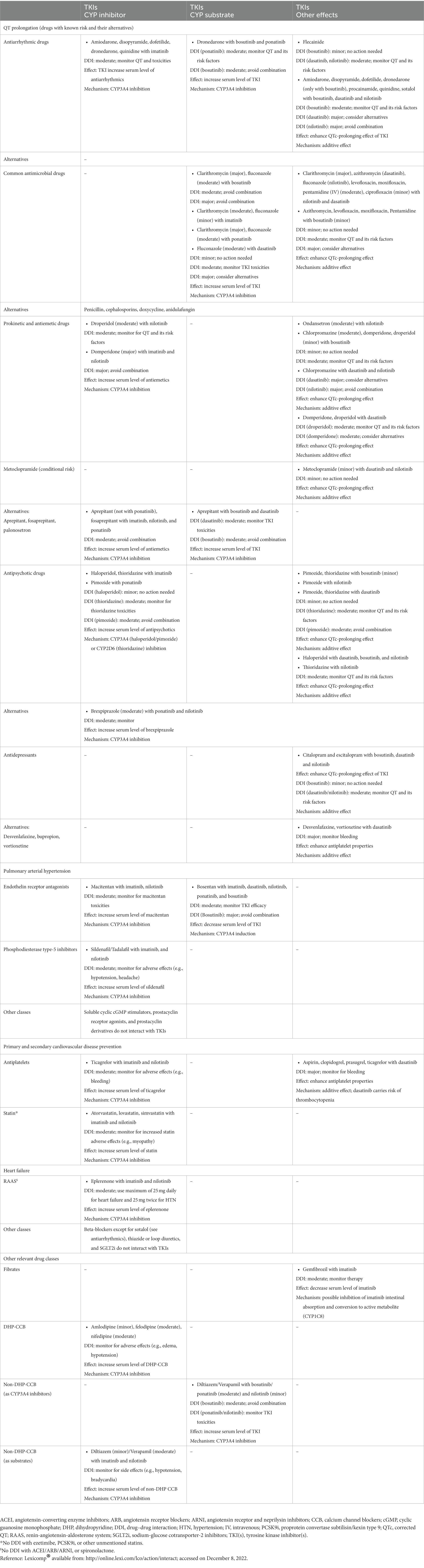
Table 6. Drug–drug interactions between tyrosine kinase inhibitors and potentially interacting drugs.
Vascular adverse events
Occurrence and consequences
TKI-associated vascular events usually involve arterial beds (i.e., cerebral, coronary, and peripheral), which generally arise from various etiologies, for example, atherosclerosis, vasospasm, thrombosis, vasospasm, or vasculitis. Unfortunately, the definition of TKI-associated vascular events has not been consistent among the TKI studies and the vascular events have not been adjudicated in CML studies. A study, for example, defined vascular adverse events as myocardial infarction, ischemic stroke, venous thromboembolism, and arterial occlusive disease (31). The most frequently used oncology-related terminology was peripheral artery (or arterial) occlusive disease (PAOD) (18). PAOD refers to the obstruction or occlusion of large arteries (but not coronary arteries), aortic arch, or the arteries supplying the central nervous system. PAOD shares risk factors similar to those contributing to atherosclerosis such as age, obesity, smoking, male gender, dyslipidemia, diabetes, and hypertension. Kim et al. in their study defined a clinically manifest PAOD as experiencing an acute PAOD event or having typical peripheral ulcerations or imaging-identified lesions. PAOD diagnosis can be established by abnormal ABI measurement (i.e., value <0.9) and the use of imaging modalities such as angiography or ultrasonography can aid in identifying the affected arteries and localizing the related lesions (32). Measuring the ABI in CML patients verified the associated proatherogenic effects, as abnormal ABI is sensitive and specific in detecting peripheral artery disease (8). As such, the mechanism that explains the effect of TKI therapy may be the accumulation of atherosclerosis in numerous arterial beds (18). In a prospective screening for PAOD in chronic phase CML patients on TKIs, ABI was available for 81% of patients and 18.6% of them had pathological or abnormal ABI. The rates of pathological ABI were significantly higher with nilotinib use, either as first (26%; p = 0.0297) or second-line (35.7%; p = 0.0029) therapy than with imatinib first-line therapy (6.3%), accounting for PAOD relative risk of 10.3 with nilotinib first-line therapy. Clinical manifestations of PAOD were only seen in patients on nilotinib. In the first-line nilotinib group, pathological ABI developed after 21–56 months of therapy (32).
Vascular events are clinically important adverse events that were observed with second and third generations TKIs. Earlier, there was little data about the incidence of vascular adverse events with long-term TKI use and the predisposing factors because initial Phase I and II trials did not capture these events (8, 33). However, their frequencies considerably varied in the subsequent reports (e.g., 1–29% over 2 years) (8). Dahlén et al. retrospectively detected vascular events incidence in Swedish CML patients (n = 896) using first- and second-generation TKI therapy with a median follow-up of 4.2 years. The relative risks for arterial and venous events were 1.5 (95% CI: 1.1–2.1) and 2.0 (95% CI: 1.2–3.3), respectively. Myocardial infarction rates were higher with nilotinib or dasatinib than with imatinib use. However, the authors stated that patients may have been prescribed multiple TKI agents during the study (34). The retrospective evidence showed that the vascular events rate (1%) was significantly lower with imatinib than that with nilotinib or ponatinib use. Similarly, the events rate was low with both dasatinib and bosutinib (8). Chen et al. tested the incidence of vascular adverse events in Taiwanese CML patients (n = 1,111) treated with dasatinib, imatinib, or nilotinib and showed that the latter TKI significantly increased the incidence rate compared with imatinib (HR 3.13; 95% CI: 1.30–7.51), while dasatinib was associated with a non-significant numerical rise in events rate (HR 1.71; 95% CI: 0.71–4.26). The identified risk factors included old age, nilotinib use, and previous history of cerebrovascular disease (31). In a meta-analysis of randomized trials, the use of dasatinib [odds ratio (OR) 3.86, 95% CI: 1.33–11.18], nilotinib (OR 3.42, 95% CI: 2.07–5.63), and ponatinib (OR 3.47, 95% CI: 1.23–9.78) was associated with higher vascular occlusive events rate than with imatinib. Only a trend of an increased risk was found with bosutinib use (OR 2.77, 95% CI: 0.39–19.77) (33).
The reported rate of PAOD related to nilotinib ranged from 1.2 to 12.5% (32). Cardiovascular events associated with nilotinib seem to appear at a long-term follow-up (18). For example, at the 12- and 24-month follow-up of the ENESTnd (Evaluating Nilotinib Efficacy and Safety in Clinical Trials–Newly Diagnosed Patients) trial, there was not a safety signal of vascular adverse events (35, 36). Subsequently, PAOD started to be apparent after a minimum of three-year follow-up and did not necessitate drug discontinuation. Most of the patients with PAOD events had baseline risk factors for PAOD and more patients treated with nilotinib experienced ischemic heart adverse events (37). Similar pattern was seen at the 5-year follow-up (38). At a 10-year follow-up, the cumulative cardiovascular events rates continued to increase with nilotinib use for up to 16.5% (300 mg twice daily) and 23.5% (400 mg twice daily) compared with 3.6% for imatinib, even in patients with low Framingham risk score (39). In the ENESTnd extension study, dose escalation from 300 mg to 400 mg twice daily resulted in only a few new adverse episodes (40). The mechanism of vascular adverse events of nilotinib is multifactorial. The direct effect of nilotinib on the vascular cells by exerting proatherogenic and antiangiogenic effects leads to arterial stenosis and inhibition of repair mechanisms, respectively. Other nilotinib-related factors comprise vasospasms and metabolic effects (i.e., elevated cholesterol and glucose levels), all of which can provoke atherosclerosis (8, 18, 33). Ponatinib caused vascular adverse events even in patients without prior treatment with nilotinib, which led to ponatinib transient withdrawal from the market by the FDA in 2013 (8, 18). There is no sufficient data available on the vascular effects of ponatinib, but it may hinder the growth and survival of endothelial cells. Risk factors for TKI-related adverse events comprise those implicated in the process of atherosclerosis such as age, smoking, obesity, diabetes, hypertension, and hypercholesterolemia (8, 18, 33). In a prospective study, the rate of adverse events with ponatinib use was 8.9 and 17.1% after 11 and 24 months, respectively (8).
Management
Preventing vascular adverse events is of paramount importance in the management of CML patients because their prognosis is considered good when treated with TKIs, but vascular adverse events can affect their morbidity and transplant eligibility. Risk factors for PAOD are common in the general population which necessitates individual risk determination using a validated risk score along with aggressive treatment of the underlying comorbidities and modifiable risk factors as discussed above (Figure 2). Individual risk scores can also help in selecting a safe and optimal TKI in CML patients, which is an important initial step in the prevention of adverse events. In patients with multiple risk factors or high-risk scores, nilotinib or ponatinib are not the preferred TKIs if other agents can be considered (8, 33). It was suggested to reserve ponatinib for advanced disease in the presence of a T315I mutation (33). Moreover, the presence of multiple risk factors for PAOD subjects the patient to pleural effusion while on dasatinib. Thus, the aforementioned agents (dasatinib, nilotinib, and ponatinib) are not the optimal choice, particularly in the elderly (8). The claim that bosutinib is a better option should be confirmed by clinical trials. However, the management of vascular adverse events that develop during nilotinib or ponatinib therapy should be individualized. Patients with low-grade PAOD, i.e., grades I and II, should be treated with optimal PAOD therapy and aggressive management of all cardiovascular risk factors, whereas the management of patients with high-grade PAOD, i.e., grades III and IV is more difficult, and some patients need to continue nilotinib or ponatinib based on the mutation status of the CML. Otherwise, the TKI should be stopped or replaced by another agent. Higher doses of TKIs are associated with more clinically relevant vascular adverse events. As a result, lower TKI doses and shorter durations of therapy may reduce the adverse event rates (8). Dorer et al. pooled data from three studies (n = 671) and found a significant correlation between the intensity of ponatinib dose and arterial occlusive events risk (OR 1.71). Ponatinib dose reduction by 15 mg daily decreased arterial occlusive events risk by 33% (41). The OPTIC (Optimizing Ponatinib Treatment in CP-CML) Phase II study explored a dose-reduction approach to examine three ponatinib starting doses (45, 30, and 15 mg daily) on the safety and efficacy in highly resistant CML patients. For 45-mg and 30-mg groups, the dose was reduced to 15 mg daily when treatment response was seen (i.e., BCR::ABL1IS transcript level is 1% or less). The response at 12 months (i.e., primary endpoint) was attained in 44.1, 29, and 23.1%, respectively. The arterial occlusive events of severity grade 3 or above in response to treatment occurred in three patients in the 15-mg group and five patients in each of the other groups. The investigators concluded that optimal efficacy and safety outcome was attained when starting treatment with 45 mg daily and then reducing it to 15 mg daily upon achieving a response (42). Patients with PAOD who require interventional revascularization due to cerebral ischemia or myocardial infarction should consider switching to another TKI therapy, along with the guidelines-recommended antiplatelet and anticoagulation therapies (8).
QT prolongation
Occurrence and consequences
QT prolongation and torsade de pointes are considered acute events. Their incidence was higher with TKIs (6.8%) than that with other anticancer agents (1.2%). The occurrence of QT prolongation was associated with increased polymorphic ventricular arrhythmia and the consequent fatal arrythmias and sudden cardiac death (2). Although the absolute risk is minimal, the risk of torsade de pointes has been associated with prolonged QT intervals, especially when QTc is above 500 milliseconds (ms) (16). There is a 2–3-fold increased risk for torsade de pointes when QTc is 500 ms or above compared with a value of less than 500 ms (22). When quantifying the risk, studies found that each 10-ms increase in QTc interval causes an increase of approximately 5–7% in cardiac events risks such as syncope, cardiac arrest, or death (19). Third-generation TKIs have been associated with QT prolongation (1). QT prolongation warranted a black box warning for nilotinib (18, 19). One of the on-target proposed mechanisms for QT prolongation is the inhibition of the PI3K signaling pathway that led to changes in sodium and potassium current, accounting for more than 70% of the overall prolongation (1, 2, 16). An off-target mechanism occurs through the interference of TKIs with cardiomyocytes potassium channel protein called human Ether-a-go-go (hERG) (2, 19). hERG facilitates ventricular repolarization of potassium current potential during phases 2 and 3 of the action potential. TKIs have variable effects on QT prolongation, with dasatinib being the most implicated agent (2). Potential risk factors that can potentiate the risk of QT prolongation include drug–drug interaction with inhibitors of cytochrome P450 (CYP) 3A4 (CYP3A4) and CYP2D6 enzymes (Table 6), genetic predisposition, electrolyte disturbances, and conditions (e.g., renal or hepatic failure) that reduce the elimination of TKIs (16, 19). Overall, the rate of drug-induced QT prolongation is considered low and patients with malignancies, in comparison with healthy individuals, may be at higher risk for torsade de pointes due to their underlying disease and its treatment. In addition, those with advanced disease may accept the risk of cardiotoxicities of their therapy that prolongs their survival unlike patients with less-advanced cancers who may not (16).
Management
The QT interval is usually measured based on leads II and V5 because they show the earliest onset of the QRS complex and the T-wave offset. There are different formulae to calculate the QT interval which correct the QT interval for the heart rate. The most common ones are the Bazett, Fridericia, and Hodges formulas (19). Although none of them is superior to another (1), the Fridericia formula is the one recommended in cancer patients and compared with other formulas, it has shown fewer errors at high and low heart rates (43). In the general population, the upper 99% limits of normal QTc values are 450 ms for men and 460 ms for women. QTc prolongation to 480 ms or above during cancer therapy is relatively frequent and requires closer monitoring. The change in QT interval of more than 60 ms from baseline does not necessitate an alteration in treatment decision as long as QTc remains below 500 ms (22). There is no risk score to help identify the patient’s risk factors for QTc prolongation while on TKI therapy (19). However, Tisdale et al. developed and validated a risk score for the prediction of QT prolongation in hospitalized patients which may guide monitoring and therapeutic decisions. The risk score classifies patients into three risk categories as low (score < 7), moderate (score 7–10), and high (score ≥ 11), according to a calculated score based on the presence of nine risk factors (age ≥ 68 years, female sex, loop diuretic use, serum potassium ≤3.5 mEq/L, admission QTc ≥ 450 ms, QTc-prolonging drugs, acute myocardial infarction, sepsis, and heart failure) (44).
Before receiving a QT prolonging-TKI, a complete past medical and medication history should be collected (2, 16, 19). There are different reported congenital (e.g., Brugada and congenital long QT syndromes) and acquired reasons for QT prolongation. Acquired causes can be cardiac (e.g., cardiomyopathy and ischemia), metabolic (e.g., hypothyroidism and electrolytes), drug-induced (e.g., antiarrhythmics, psychotropics, and antimicrobials), or others (e.g., hypothermia) (16). Baseline and regular electrocardiogram (ECG) with QTc monitoring are advised during TKI therapy. Other monitoring measurements include blood pressure, electrolytes, thyroid hormones, B-type natriuretic peptide (BNP), and cardiac biomarkers (1, 2, 16). Immediate and careful patient evaluation should be performed upon detecting a prolonged QTc interval as defined (19). General measures comprise correcting and monitoring identifiable causes such as electrolytes imbalance (i.e., hypokalemia, hypomagnesemia, and hypocalcemia) (2, 16), and the presence of QT-prolonging drugs that interact with TKIs. The drug regimen should be modified or discontinued (Table 6). The presence of palpitation, syncope or presyncope, or QT prolongation with new-onset bradycardia of a rate less than 60 beats per minute and high-degree heart block should prompt immediate evaluation with continuous monitoring. The ECG must be repeated on a daily basis until QT prolongation is resolved. Correcting the QT prolongation by using drugs should be considered if there are concerning ECG signs for torsade de pointes. Treatment includes intravenous magnesium sulfate, beta-adrenergic agent (e.g., isoproterenol), lidocaine infusion and temporary pacing for refractory cases, and changing rate setting in patients with implantable cardiac devices (19). A proposed management algorithm for QT interval prolongation surveillance before and during TKI therapy is summarized in Figure 3 (2, 16, 18, 19).
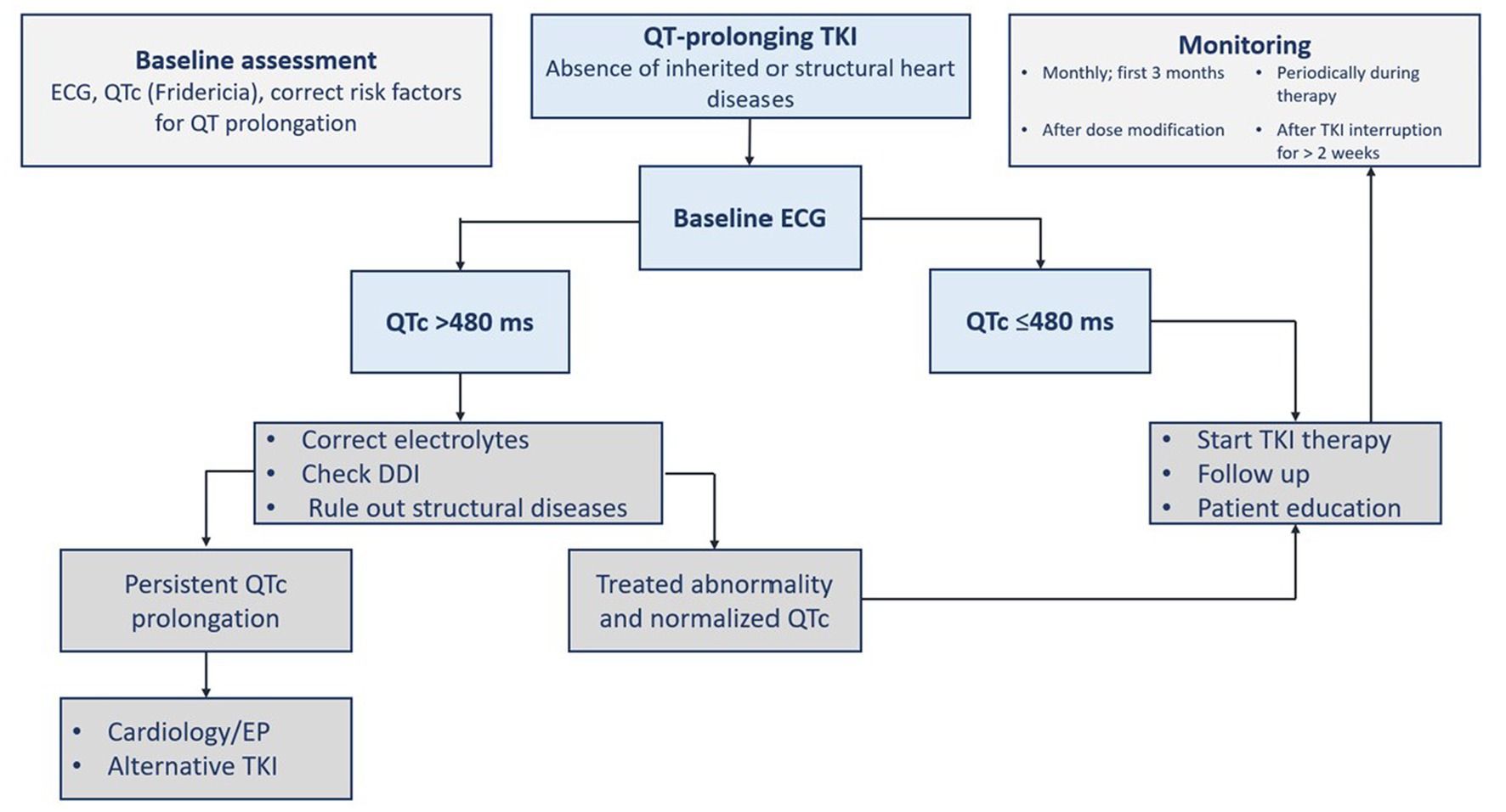
Figure 3. Assessment of QTc prolongation before and during tyrosine kinase inhibitor therapy. ECG, electrocardiogram; DDI, drug-drug interaction; EP, electrophysiology; TKI, tyrosine kinase inhibitor(s); QTc, corrected QT interval.
Heart failure
Occurrence and consequences
Cardiomyopathy or cardiac dysfunction induced by chemotherapy may range from asymptomatic myocardial injury [i.e., evident by a rise in cardiac troponin or decrease in global longitudinal strain (GLS)] to severe heart failure with reduced left ventricular ejection fraction (LVEF). Chemotherapy-induced cardiomyopathy is usually defined as a reduction in LVEF by more than 10% points and reaching a value below the lower limit of normal. Among the other cardiomyopathy types, it has the worse prognosis, adding to that the need for chemotherapy interruption or switching to a less effective alternative, hence leading to reduced survival in cancer patients (31, 45). Heart failure due to cardiomyopathy is a serious adverse event of TKI treatment. Dasatinib caused serious cardiotoxicity including heart failure and cardiomyopathy in 1.6% of patients receiving it. Mitochondrial damage, cardiac energy balance alterations, and contractile protein dysfunction can lead to left ventricular systolic dysfunction and consequent heart failure. It was hypothesized that inhibiting PDGFR and other tyrosine kinase receptors affects the myocytes’ normal response to hypertensive stress. Cardiac damage may result from interfering with the ribosomal S6 kinase family as it inhibits the phosphorylation of apoptosis-activating factors and thus determines the survival of the cardiomyocytes (1).
Management
The risk of systolic dysfunction and heart failure is probably underestimated in the relevant studies (1). TKI-induced cardiotoxicity occurs independently of the dose and at any time throughout therapy (i.e., a few days to months). Early recognition of cardiomyopathy and initiation of heart failure therapy increases the chances of left ventricular function improvement (45). Patients on potentially cardiotoxic agents should be closely monitored for any cardiac dysfunction. Monitoring of patients on a TKI with unclear risk such as imatinib is only recommended in the presence of predisposing comorbidities, or signs and symptoms of cardiac disease. Patients with cancer therapy-induced heart failure should be managed according to the international heart failure guidelines regardless of their cancer status. Before the initiation of TKI therapy, LVEF should be assessed at baseline (1, 45). For patients on TKI, the surveillance strategy is not supported by adequate evidence. The gold standard in chemotherapy-induced cardiomyopathy monitoring is the assessment of LVEF. The utilization of cardiac troponin and BNP has been proposed and supported by evidence for the early detection of subclinical cardiotoxicity (45). For asymptomatic heart failure, the European cardio-oncology guidelines defined the respective CTRCD based on reductions in LVEF and/or changes in GLS (22). Thavendiranathan et al. in their study support the utilization of GLS to guide cardioprotective therapy to prevent CTRCD and reductions in LVEF (46). Earlier, Negishi et al. found that GLS was an early predictor of reductions in LVEF as a consequence of cancer therapy (47). Since chemotherapy-induced heart failure usually improves with guidelines-directed medical therapy, implantable cardiac devices should be reserved for patients with persistent systolic dysfunction or when there is a prognostic mortality benefit. In the absence of dedicated randomized trials, evidence from clinical practice showed a potential long-term efficacy of CML treatment. Patient education about the potential risk, the possibility of prevention, and the management approaches can help benefit from long-term therapy with TKIs (Figure 4) (16).
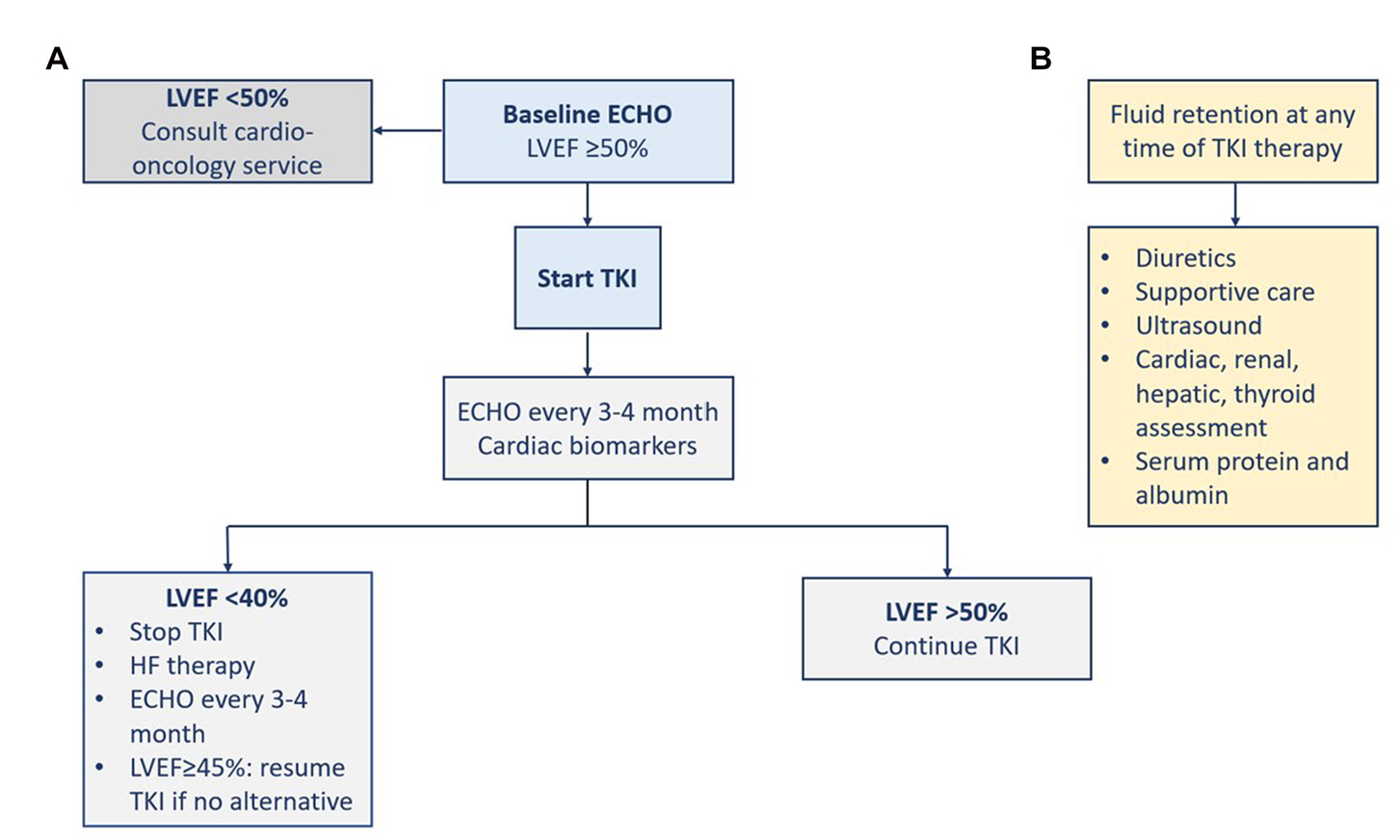
Figure 4. Management of heart failure (A) and fluid retention (B). ECHO, echocardiography; LVEF, left ventricular ejection fraction; TKI, tyrosine kinase inhibitor.
Fluid retention
Occurrence and management
Fluid retention induced by some TKIs can result in pericardial effusion or pulmonary edema (1). At the 5-year follow-up of the ENESTnd trial, severe fluid retention was more frequent with imatinib (23.2%) than with the two doses of nilotinib (11.1 and 14.4%). Severe fluid retention included peripheral edema, pleural effusion, pulmonary edema, pericardial effusion, or cardiac tamponade (38). However, at the 10-year follow-up, severe fluid retention was infrequent (39). Although it has been described with the use of imatinib or bosutinib, heart failure was rarely observed (~1%). Pulmonary edema due to fluid retention was observed with dasatinib use. The inhibition of PDGFR signaling was proposed as a possible mechanism since it regulates the homeostasis of interstitial fluid. The standard management of fluid retention is diuretics and supportive care. The dose of the TKI can be reduced or the TKI can be stopped if needed. There should be an assessment for the cardiac, renal, hepatic, and thyroid functions, in addition to the serum levels of protein and albumin. Ultrasound modality is indicated to diagnose and monitor patients with any sign of pulmonary edema, pericardial or pleural effusion, and ascites (Figure 4) (1).
Pulmonary toxicity
The incidence of TKI-induced pulmonary toxicities is less than 1%. The frequent toxicities included pleural effusion, pulmonary arterial hypertension (PAH), pulmonary edema, interstitial lung disease, pneumonitis, chylothorax, and upper respiratory tract infection (5). The available data about the mechanisms of pulmonary toxicity involves that of dasatinib. The long-term toxicity data for both bosutinib and ponatinib is scarcely given their relatively recent approval. Luckily, the adverse events are usually reversible after drug discontinuation. Although there are various possible overlapping mechanisms for TKI-induced pulmonary toxicities, the strongly implicated ones are, direct cell injury, endothelial cell dysfunction, excess reactive oxygen species at cellular levels, or indirect inflammatory-mediated events. The management of TKI-induced pulmonary toxicity should be individualized considering the severity of respiratory status and hematology service involvement (3).
Pleural effusions and interstitial lung diseases
Occurrence and consequences
The incidence of pleural effusions differs between the TKIs, with dasatinib being the most implicated TKI. Interstitial lung diseases are rarer in occurrence but both conditions should be suspected in TKI-treated patients when experiencing non-specific respiratory symptoms. None of the conditions was reported with ponatinib. An incidence of pleural effusion of less than 5% occurred with bosutinib over 5 years, with more frequent rates in older patients (3). Chylothorax, a subgroup of pleural effusion, is a rare pulmonary toxicity that was reported with dasatinib (5). A retrospective study (n = 212) found that 55% of chronic phase CML patients on dasatinib had adverse events. Pleural effusions were the most frequent ones (25%) and the predominant reason for permanent drug discontinuation. The authors suggested the use of the lowest effective dose particularly in older patients to decrease the risk of pleura effusion occurrence (34, 48). The FDA has announced a warning about the cardiopulmonary risk of dasatinib therapy (18). However, the response to dasatinib therapy and the survival (i.e., both progression-free and overall) did not differ between patients who developed pleural effusion and who did not (3). The significant risk factors for the development of pleural effusion associated with dasatinib included twice daily dasatinib schedule, duration of CML, skin rash, duration of dasatinib therapy, and comorbidities such as cardiac history, hypertension, hypercholesterolemia, and autoimmune disease (3, 5, 8).
Management
A detailed investigation for pleural effusion is warranted given the various possible causes of it. If there is a sufficient effusion volume, diagnostic thoracentesis is indicated to exclude pleural infection and to eliminate other differential diagnoses. The use of diuretics and corticosteroids has been described in the literature but has not been tested in trials. Their use may be dependent on the causative element of the pleural effusion. The size and consequences of the effusion usually dictate specific management. Close clinical and diagnostic monitoring can be sufficient with minimal effusion volume, whereas effusions with moderate or large volumes may necessitate reducing the TKI dose, withdrawing, or changing the used TKI. Since the occurrence of interstitial lung diseases with TKI therapy is rare, other possible causes should be ruled out. TKI-induced pneumonitis resolves spontaneously with TKI discontinuation or corticosteroid use. It has been reported that interstitial lung disease due to imatinib did not occur when changing to nilotinib. However, re-challenging with the use of the offending TKI can be considered based on the risk–benefit assessment for an individual patient (3).
Dasatinib is associated with high occurrence rates of pleural effusion, with the patient’s age being the most significant factor for its incidence. However, it is usually a reversible adverse event in most cases (5). Dose reduction (e.g., 100 mg once daily), dose interruption, TKI discontinuation, and drug therapy have been suggested for the management of dasatinib-induced pleural effusion (3, 5, 7). Naqvi et al. updated the results of their initial study and continued to demonstrate the efficacy and tolerability of dasatinib 50 gm daily for newly diagnosed CML patients in the chronic phase (49, 50), with 6% of patients experiencing pleural effusion and 80% of them needed further dose reduction (50). In the multicenter single-arm DAVLEC (DAsatinib, Very Low-dose, for Elderly CML-CP patients) Phase II Japanese study (n = 52), the standard dasatinib starting dose was reduced by 20% and 20 mg daily was administered in new diagnosed elderly patients above 70 years of age. Pleural effusion, not pulmonary hypertension, was observed in 7.7% of patients. The investigators encouraged dose reduction consideration and conducting more studies on larger and more diverse cohorts (51). However, dasatinib dose reduction did not prevent the recurrence of pleural effusion in all the cases. Drug withdrawal was reported in 22% of patients who developed dasatinib-induced pleural effusion without the need for a therapeutic thoracentesis. Switching from dasatinib to bosutinib resulted in the recurrence of pleural effusion in 30% of the cases. Although not widely used, therapeutic drug monitoring of TKIs may minimize pleural effusion rates (3). There are some reports on the use of diuretics and corticosteroids as mentioned above (3, 7). The use of vasopressin V2-antagonist (tolvaptan) with diuretics, in some reports, improved pleural effusion and allowed continuing or reintroducing dasatinib (3).
Patients with Class 1 pleural effusion do not require intervention. In asymptomatic patients with Class 2 or more, TKI therapy should be interrupted, and diuretics can relieve fluid retention if present. TKI should be resumed after effusion resolution with dose reduction in case of further occurrences. If patients with Class 2 or more are symptomatic or with Class 3 or more but asymptomatic, dasatinib therapy should be interrupted and then resumed after the effusion is resolved. However, it should be discontinued if the pleural effusion recurs. In addition, corticosteroids such as 40 mg of prednisone per day, should be given for 4 days, and the pleural fluid should be examined for other potential causes. Another classification approach defines small effusion as a volume of less than 500 ml with blunting costophrenic angle; medium effusion as with opacity above the costophrenic angle; and large effusion when having more than 30–50% of hemithorax. The respective management and monitoring of each severity are presented in Figure 5 (5).
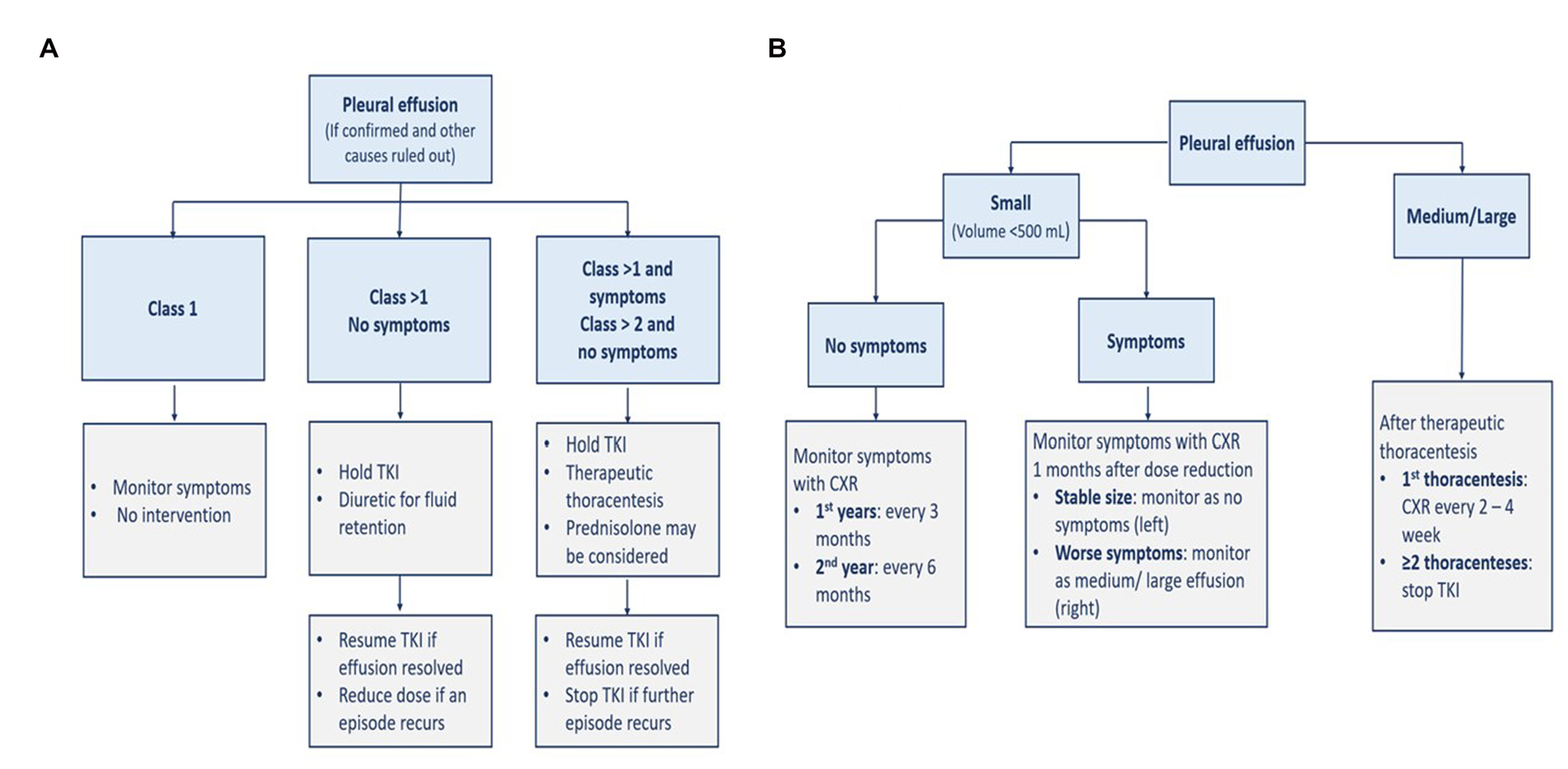
Figure 5. Management (A) and monitoring (B) of pleural effusion. CXR, chest x-ray; TKI, tyrosine kinase inhibitor.
Pulmonary arterial hypertension
Occurrence and consequences
Pulmonary arterial hypertension has been reported with dasatinib therapy, rarely with bosutinib, nilotinib, or ponatinib, whereas no reports with imatinib (3). In PAH, there is usually an increase in pulmonary vascular resistance (PVR) and pulmonary artery pressure (PAP) leading to right ventricular failure (7); mean PAP is above 20 mmHg and PVR is 3 Wood units (WU) or above with normal left cardiac filling pressures (i.e., pulmonary artery wedge pressure (PCWP) ≤15 mmHg) (3, 52). Dasatinib is implicated in increasing the pressure in the pulmonary arterial system, hence causes a pre-capillary PAH (i.e., Clinical Group 1) (7). Earlier, a study using data from the French pulmonary hypertension registry identified nine dasatinib-treated patients with pulmonary hypertension, from dasatinib approval from November 2006 to September 2010. The estimated incidence of dasatinib-related pulmonary hypertension in France was 0.45%. There were not any incidental pulmonary hypertension cases related to other TKIs at the time of diagnosis. Four months after the discontinuation of dasatinib, there were hemodynamic, clinical, and functional improvements. However, there was not a complete recovery in most of the patients after 9 months of follow-up (20). Another study from the same French registry investigated the long-term outcomes of 21 patients with dasatinib-induced PAH which was confirmed by right-heart catheterization. Nineteen patients with CML received dasatinib for a median of 42 months before PAH diagnosis. Dasatinib was discontinued in all patients, and half of them (n = 11) received therapy for PAH such as bosentan, sildenafil, and calcium channel blockers. Despite improvement after drug discontinuation, PAH continued in 37% of patients. Concomitant pleural and pericardial effusions were detected in 62 and 29% of patients, respectively (53). A multicenter study from Australia retrospectively studied 212 patients with chronic phase CML. The study estimated an occurrence of 5% for dasatinib-induced PAH which was frequently associated with pleural effusion. PAH was reversible in most of the cases after dasatinib discontinuation. Permanent discontinuation of dasatinib in immunologically competent patients was not necessary (48).
Cases with reversible PAH may have an intense pulmonary arterial vasoconstriction (3), while the frequently reported cases with the persistence of PAH may suggest an irreversible pulmonary arterial remodeling (3, 7). The latter proved valid with histological examination of explanted lungs from a patient who required lung transplantation. The survival of patients with dasatinib-induced PAH (i.e., 90.5 and 85.7% at 1- and 5-year follow-up, respectively) was similar to that reported in dasatinib randomized trials in CML (3). PAH, however, is considered a life-threatening consequence of dasatinib long-term therapy, particularly when accompanied by pleural effusion. If left untreated, it may cause right ventricular failure (5). Finally, although ponatinib was associated with a 6% increase in venous thromboembolic events, there were no cases reported for thromboembolic PAH due to TKIs use (3).
Management
The incidence of symptomatic PAH is relatively low and does not warrant PAH screening in asymptomatic patients (3). Dasatinib is the TKI with the potential to cause PAH. Early PAH diagnosis and TKI therapy discontinuation are recommended (5). The symptoms of PAH are usually not specific such as dyspnea, fatigue, atypical chest pain, or unexplained syncope. The presence of non-specific symptoms should raise suspicion about PAH diagnosis and prompt investigation using chest X-ray and echocardiogram with Doppler flow, as well as considering referral to cardiology service if warranted. A baseline echocardiogram before dasatinib initiation may be performed, if feasible, to identify a pre-existing PAH (3). If PAH is suspected during TKI therapy, a chest X-ray is performed to rule out pleural effusion, and an echocardiogram is performed to assess PAP and then identify the cause of the elevated PAP (≥25 mmHg). A right-heart catheterization is indicated to confirm PAH diagnosis (5, 7, 18).
When there is a high probability of a new pulmonary hypertension occurrence in a CML patient (i.e., peak tricuspid regurgitation velocity (TRV) is more than 3.4 m/s which is equivalent to an estimated PAP of 50 mmHg or more), TKI therapy should be discontinued until ruled out or confirmed with right-heart catheterization (22). More specifically, if dasatinib-induced PAH is diagnosed, dasatinib therapy should be discontinued (5, 7, 22), and alternative TKI is recommended (22). If dasatinib-treated patients have new asymptomatic peak TRV between 2.9 and 3.4 m/s, the dasatinib dose can be reduced and peak TRV should be monitored every 4 weeks with echocardiography. If peak TRV keeps rising and PAH is confirmed by right-heart catheterization, dasatinib should be stopped (22). Symptoms and PAP usually improve after dasatinib discontinuation (5, 7) but usually without complete recovery of the hemodynamic parameters (5). The overall treatment of pulmonary hypertension should be according to the respective societal guidelines (22). The management of PAH is directed toward the FDA-approved drug classes for PAH treatment and collaboration with the PAH team (5, 7). FDA-approved drug classes include phosphodiesterase type-5 inhibitors (e.g., sildenafil and tadalafil), endothelin receptor-1 antagonists (e.g., bosentan, ambrisentan, and macitentan), prostacyclin derivatives (e.g., systemic epoprostenol, inhaled iloprost, and oral and systemic treprostinil), soluble guanylate cyclase stimulator (e.g., oral riociguat), and prostacyclin receptor agonist (oral selexipag) (5). The steps for the diagnosis and management of TKI-induced PAH are demonstrated in Figure 6 (3, 5, 7, 22). There is not any evidence suggesting the effectiveness of calcium channel blockers in dasatinib-induced PAH (5).
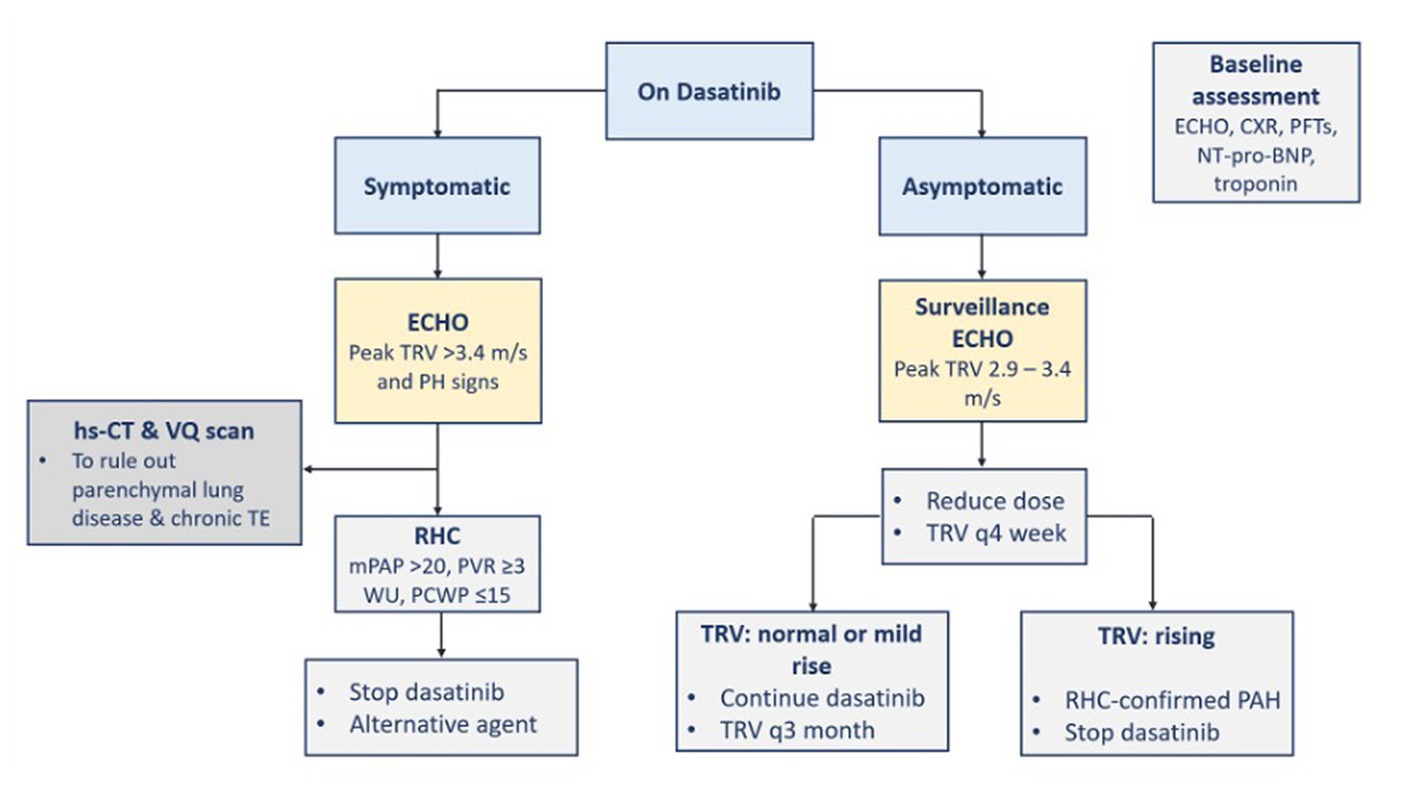
Figure 6. Diagnosis and management of TKI-induced PAH. BNP, B-type natriuretic peptide; CXR, chest x-ray; ECHO, echocardiography; hs-CT, high resolution computed tomography; mPAP, mean pulmonary arterial pressure; PAH, pulmonary arterial hypertension; PCWP, pulmonary artery wedge pressure; PFTs, pulmonary function tests; PH, pulmonary hypertension; PVR, pulmonary vascular resistance; RHC, right heart catheterization; TE, thromboembolism; TKI, tyrosine kinase inhibitor; TRV, tricuspid regurgitation velocity; VQ, ventilation perfusion; WU, Wood units.
Drug–drug interactions
Most of the TKIs are metabolized by CYP enzymes and transported by P-glycoprotein (P-gp) (2, 7). Strong CYP enzyme inhibitors can increase TKIs plasma concentrations (1, 16), and vice versa as TKIs can inhibit CYP enzymes to varying degrees (7). Dasatinib can also influence P-gp, the drug transporter (2). Table 6 lists the drug–drug interactions between TKI agents and the most used drugs in cardiopulmonary conditions (54).
Summary
The introduction of TKIs is considered a milestone in the treatment of CML but their use was associated with a range of serious cardiopulmonary toxicities including vascular adverse events, QT prolongation, heart failure, pleural effusion, and PAH. The current fully approved TKIs (imatinib, bosutinib, dasatinib, nilotinib, and ponatinib) have different efficacy and safety profiles. The exact underlying mechanisms for cardiopulmonary toxicities are not fully known but on- and off-target effects may be partly responsible. Imatinib has the most favorable safety profile among TKIs. Nilotinib and ponatinib were associated with vascular occlusive adverse events, whereas dasatinib was with pleural effusion and PAH. QT prolongation was mostly linked to third-generation agents. TKI-induced adverse events can lead to serious morbidities if left untreated.
The assessment of cardiovascular risk is crucial before starting TKI therapy. The choice of TKI agent should be dictated by its safety profile, disease state, patient comorbidities, and other concurrent therapies considering their interacting tendency. The management of cardiopulmonary adverse events should be integrated with a multidisciplinary collaboration between the respective specialties. Early recognition, frequent monitoring, optimal intervention, and adequate follow-up are essential to managing adverse events while maintaining the long-term TKI therapy benefit. Awareness of the potential TKIs toxicities and balancing the risks and benefits of such therapy should be considered. Future research should fill in the gaps in knowledge about molecular mechanisms, frequencies, definitions, classifications, predictors, and management guidelines of TKIs toxicities.
Author contributions
All authors listed have made a substantial, direct, and intellectual contribution to the work and approved it for publication. All authors contributed equally to the manuscript.
Funding
This work was supported by the Academic Health System, Hamad Medical Corporation.
Conflict of interest
The authors declare that the research was conducted in the absence of any commercial or financial relationships that could be construed as a potential conflict of interest.
Publisher’s note
All claims expressed in this article are solely those of the authors and do not necessarily represent those of their affiliated organizations, or those of the publisher, the editors and the reviewers. Any product that may be evaluated in this article, or claim that may be made by its manufacturer, is not guaranteed or endorsed by the publisher.
Abbreviations
Acronym, Explanation; ABI, Ankle-brachial index; CML, Chronic myeloid leukemia; CRCD, Chemotherapy-related cardiac dysfunction; CTCAE, Common Terminology Criteria for Adverse Events; CTRCD, Cancer therapy-related cardiac dysfunction; CYP, Cytochrome P450; DAVLEC, DAsatinib, very low-dose, for elderly CML-CP patients; ECG, Electrocardiogram; EGFR, Epidermal growth factor receptor; ENESTnd, Evaluating Nilotinib Efficacy and Safety in Clinical Trials–Newly Diagnosed Patients; FDA, Food and Drug Administration; FEARS, Food and Drug Administration adverse event reporting system; GLS, Global longitudinal strain; hERG, Human Ether-a-go-go; LVEF, Left ventricular ejection fraction; NCI, National Cancer Institute; OPTIC, Optimizing Ponatinib Treatment in CP-CML; OR, Odds ratio; PAH, Pulmonary arterial hypertension; PAOD, Peripheral arterial occlusive disease; PAP, Pulmonary artery pressure; PDGFR, Platelet-derived growth factor receptor; QTc, Corrected QT interval; ROCK, Rho-associated coiled-coil containing kinase; TKI(s), Tyrosine kinase inhibitor(s); VEGFR, Vascular endothelial growth factor receptor.
Abbreviations
BNP, B-type natriuretic peptide; CXR, chest x-ray; ECHO, echocardiography; hs-CT, high resolution computed tomography; mPAP, mean pulmonary arterial pressure; PAH, pulmonary arterial hypertension; PCWP, pulmonary artery wedge pressure; PFTs, pulmonary function tests; PH, pulmonary hypertension; PVR, pulmonary vascular resistance; RHC, right heart catheterization; TE, thromboembolism; TKI, tyrosine kinase inhibitor; TRV, tricuspid regurgitation velocity; VQ, ventilation perfusion; WU, wood units.
References
1. Grela-Wojewoda, A, Pacholczak-Madej, R, Adamczyk, A, Korman, M, and Püsküllüoğlu, M. Cardiotoxicity induced by protein kinase inhibitors in patients with Cancer. Int J Mol Sci. (2022) 23:2815. doi: 10.3390/ijms23052815
2. Cheng, M, Yang, F, Liu, J, Yang, D, Zhang, S, Yu, Y, et al. Tyrosine kinase inhibitors-induced arrhythmias: from molecular mechanisms, pharmacokinetics to therapeutic strategies. Front Cardiovasc Med. (2021) 8:758010. doi: 10.3389/fcvm.2021.758010
3. Weatherald, J, Bondeelle, L, Chaumais, MC, Guignabert, C, Savale, L, Jaïs, X, et al. Pulmonary complications of Bcr-Abl tyrosine kinase inhibitors. Eur Respir J. (2020) 56:2000279. doi: 10.1183/13993003.00279-2020
4. Maiellaro, F, Bellino, M, Radano, I, Ciccarelli, M, and Citro, R. Cardiovascular management of patients with chronic myeloid leukemia treated with BCR-ABL tyrosine kinase inhibitors: it is time for a shared management. G Ital Cardiol. (2022) 23:268–77. doi: 10.1714/3766.37536
5. Nekoukar, Z, Moghimi, M, and Salehifar, E. A narrative review on adverse effects of dasatinib with a focus on pharmacotherapy of dasatinib-induced pulmonary toxicities. Blood Res. (2021) 56:229–42. doi: 10.5045/br.2021.2021117
6. Cirmi, S, El Abd, A, Letinier, L, Navarra, M, and Salvo, F. Cardiovascular toxicity of tyrosine kinase inhibitors used in chronic myeloid leukemia: an analysis of the FDA adverse event reporting system database (FAERS). Cancers. (2020) 12:826. doi: 10.3390/cancers12040826
7. Aghel, N, Delgado, DH, and Lipton, JH. Cardiovascular toxicities of BCR-ABL tyrosine kinase inhibitors in chronic myeloid leukemia: preventive strategies and cardiovascular surveillance. Vasc Health Risk Manag. (2017) 13:293–303. doi: 10.2147/VHRM.S108874
8. Valent, P, Hadzijusufovic, E, Schernthaner, GH, Wolf, D, Rea, D, and le Coutre, P. Vascular safety issues in CML patients treated with BCR/ABL1 kinase inhibitors. Blood. (2015) 125:901–6. doi: 10.1182/blood-2014-09-594432
9. Jiang, Q, Li, Z, Qin, Y, Li, Weiming, Xu, Na, Liu, Bingcheng, et al. Olverembatinib (HQP1351), a well-tolerated and effective tyrosine kinase inhibitor for patients with T315I-mutated chronic myeloid leukemia: results of an open-label, multicenter phase 1/2 trial [published correction appears in J Hematol Oncol. 2022 Oct 31;15(1):159]. J Hematol Oncol. (2022); 15:113, doi: 10.1186/s13045-022-01334-z
10. Saydam, G, Ali, R, Demir, AM, Eskazan, AE, Guvenc, B, Haznedaroglu, IC, et al. The effect of comorbidities on the choice of tyrosine kinase inhibitors in patients with chronic myeloid leukemia. Int J Hematol Oncol. (2022) 11:IJH38. doi: 10.2217/ijh-2021-0010
11. Dhillon, S . Olverembatinib: first approval. Drugs. (2022) 82:469–75. doi: 10.1007/s40265-022-01680-9
13. Cortes, JE, Saikia, T, Kim, DW, Alvarado, Y, Nicolini, FE, Khattry, N, et al. Phase 1 trial of vodobatinib, a novel oral BCR-ABL1 tyrosine kinase inhibitor (TKI): activity in CML chronic phase patients failing TKI therapies including ponatinib. Blood. (2020) 136:51–2. doi: 10.1182/blood-2020-139847
14. Ivanova, ES, Tatarskiy, VV, Yastrebova, MA, Khamidullina, AI, Shunaev, AV, Kalinina, AA, et al. PF-114, a novel selective inhibitor of BCR-ABL tyrosine kinase, is a potent inducer of apoptosis in chronic myelogenous leukemia cells. Int J Oncol. (2019) 55:289–97. doi: 10.3892/ijo.2019.4801
15. Andretta, E, Costa, C, Longobardi, C, Damiano, S, Giordano, A, Pagnini, F, et al. Potential approaches versus approved or developing chronic myeloid leukemia therapy. Front Oncol. (2021) 11:801779. doi: 10.3389/fonc.2021.801779
16. Lenihan, DJ, and Kowey, PR. Overview and management of cardiac adverse events associated with tyrosine kinase inhibitors. Oncologist. (2013) 18:900–8. doi: 10.1634/theoncologist.2012-0466
17. Common Terminology Criteria for Adverse Events (CTCAE) . Available at: Common Terminology Criteria for Adverse Events (CTCAE) | Protocol Development | CTEP (cancer.gov) (Accessed November 16, 2022).
18. Barber, MC, Mauro, MJ, and Moslehi, J. Cardiovascular care of patients with chronic myeloid leukemia (CML) on tyrosine kinase inhibitor (TKI) therapy. Hematology Am Soc Hematol Educ Program. (2017) 2017:110–4. doi: 10.1182/asheducation-2017.1.110
19. Porta-Sánchez, A, Gilbert, C, Spears, D, Amir, E, Chan, J, Nanthakumar, K, et al. Incidence, diagnosis, and management of QT prolongation induced by Cancer therapies: a systematic review. J Am Heart Assoc. (2017) 6:e007724. doi: 10.1161/JAHA.117.007724
20. Montani, D, Bergot, E, Günther, S, Savale, L, Bergeron, A, Bourdin, A, et al. Pulmonary arterial hypertension in patients treated by dasatinib. Circulation. (2012) 125:2128–37. doi: 10.1161/CIRCULATIONAHA.111.079921
21. Nodzon, L, Fadol, A, and Tinsley, S. Cardiovascular adverse events and mitigation strategies for chronic myeloid leukemia patients receiving tyrosine kinase inhibitor therapy. J Adv Pract Oncol. (2022) 13:127–42. doi: 10.6004/jadpro.2022.13.2.4
22. Lyon, AR, López-Fernández, T, Couch, LS, Asteggiano, R, Aznar, MC, Bergler-Klein, J, et al. 2022 ESC guidelines on cardio-oncology developed in collaboration with the European Hematology Association (EHA), the European Society for Therapeutic Radiology and Oncology (ESTRO) and the international cardio-oncology society (IC-OS). Eur Heart J. (2022) 43:4229–361. doi: 10.1093/eurheartj/ehac244
23. Wang, Q, Jiang, C, Zhang, Y, Zhang, Y, Yue, B, Zheng-Lin, B, et al. Cardiovascular mortality among chronic myeloid leukemia patients in the pre-tyrosine kinase inhibitor (TKI) and TKI eras: a surveillance, epidemiology and end results (SEER) analysis. Leuk Lymphoma. (2020) 61:1147–57. doi: 10.1080/10428194.2019.1711074
24. Caocci, G, Mulas, O, Annunziata, M, Luciano, L, Abruzzese, E, Bonifacio, M, et al. Long-term mortality rate for cardiovascular disease in 656 chronic myeloid leukaemia patients treated with second- and third-generation tyrosine kinase inhibitors. Int J Cardiol. (2020) 301:163–6. doi: 10.1016/j.ijcard.2019.10.036
25. Molica, M, Scalzulli, E, Colafigli, G, Fegatelli, DA, Massaro, F, Latagliata, R, et al. Changes in estimated glomerular filtration rate in chronic myeloid leukemia patients treated front line with available TKIs and correlation with cardiovascular events. Ann Hematol. (2018) 97:1803–8. doi: 10.1007/s00277-018-3375-9
26. Yu, B, Osman, AEG, Sladojevic, N, Prabhu, N, Tai, HC, Chen, D, et al. Involvement of rho-associated coiled-coil containing kinase (ROCK) in BCR-ABL1 tyrosine kinase inhibitor cardiovascular toxicity. JACC CardioOncol. (2022) 4:371–83. doi: 10.1016/j.jaccao.2022.06.004
27. Upshaw, JN, Travers, R, and Jaffe, IZ. ROCK and rolling towards predicting BCR-ABL kinase inhibitor-induced vascular toxicity. JACC CardioOncol. (2022) 4:384–6. doi: 10.1016/j.jaccao.2022.07.001
28. Ahmed, K, Kaddoura, R, and Yassin, MA. A practical guide to managing hypertension, hyperlipidemia, and hyperglycemia in patients with chronic myeloid leukemia. Front Med. (2022) 9:1025392. doi: 10.3389/fmed.2022.1025392
29. Li, S, He, J, Zhang, X, Cai, Y, Liu, J, Nie, X, et al. Cardiovascular adverse events in chronic myeloid leukemia patients treated with nilotinib or imatinib: a systematic review, meta-analysis and integrative bioinformatics analysis. Front Cardiovasc Med. (2022) 9:966182. doi: 10.3389/fcvm.2022.966182
30. Michel, C, Burchert, A, Hochhaus, A, Saussele, S, Neubauer, A, Lauseker, M, et al. Imatinib dose reduction in major molecular response of chronic myeloid leukemia: results from the German chronic myeloid leukemia-study IV. Haematologica. (2019) 104:955–62. doi: 10.3324/haematol.2018.206797
31. Chen, MT, Huang, ST, Lin, CW, Ko, BS, Chen, WJ, Huang, HH, et al. Tyrosine kinase inhibitors and vascular adverse events in patients with chronic myeloid leukemia: a population-based, propensity score-matched cohort study. Oncologist. (2021) 26:974–82. doi: 10.1002/onco.13944
32. Kim, TD, Rea, D, Schwarz, M, Grille, P, Nicolini, FE, Rosti, G, et al. Peripheral artery occlusive disease in chronic phase chronic myeloid leukemia patients treated with nilotinib or imatinib. Leukemia. (2013) 27:1316–21. doi: 10.1038/leu.2013.70
33. Douxfils, J, Haguet, H, Mullier, F, Chatelain, C, Graux, C, and Dogné, JM. Association between BCR-ABL tyrosine kinase inhibitors for chronic myeloid leukemia and cardiovascular events, major molecular response, and overall survival: a systematic review and Meta-analysis. JAMA Oncol. (2016) 2:625–32. doi: 10.1001/jamaoncol.2015.5932
34. Dahlén, T, Edgren, G, Lambe, M, Höglund, M, Björkholm, M, Sandin, F, et al. Cardiovascular events associated with use of tyrosine kinase inhibitors in chronic myeloid leukemia: a population-based cohort study. Ann Intern Med. (2016) 165:161–6. doi: 10.7326/M15-2306
35. Saglio, G, Kim, DW, Issaragrisil, S, le Coutre, P, Etienne, G, Lobo, C, et al. Nilotinib versus imatinib for newly diagnosed chronic myeloid leukemia. N Engl J Med. (2010) 362:2251–9. doi: 10.1056/NEJMoa0912614
36. Kantarjian, HM, Hochhaus, A, Saglio, G, de Souza, C, Flinn, IW, Stenke, L, et al. Nilotinib versus imatinib for the treatment of patients with newly diagnosed chronic phase, Philadelphia chromosome-positive, chronic myeloid leukaemia: 24-month minimum follow-up of the phase 3 randomised ENESTnd trial. Lancet Oncol. (2011) 12:841–51. doi: 10.1016/S1470-2045(11)70201-7
37. Larson, RA, Hochhaus, A, Hughes, TP, Clark, RE, Etienne, G, Kim, DW, et al. Nilotinib vs imatinib in patients with newly diagnosed Philadelphia chromosome-positive chronic myeloid leukemia in chronic phase: ENESTnd 3-year follow-up. Leukemia. (2012) 26:2197–203. doi: 10.1038/leu.2012.134
38. Hochhaus, A, Saglio, G, Hughes, TP, Larson, RA, Kim, DW, Issaragrisil, S, et al. Long-term benefits and risks of frontline nilotinib vs imatinib for chronic myeloid leukemia in chronic phase: 5-year update of the randomized ENESTnd trial. Leukemia. (2016) 30:1044–54. doi: 10.1038/leu.2016.5
39. Kantarjian, HM, Hughes, TP, Larson, RA, Kim, D-W, Issaragrisil, S, le Coutre, P, et al. Long-term outcomes with frontline nilotinib versus imatinib in newly diagnosed chronic myeloid leukemia in chronic phase: ENESTnd 10-year analysis [published correction appears in leukemia. 2021 Jul;35(7):2142-2143]. Leukemia. (2021) 35:440–53. doi: 10.1038/s41375-020-01111-2
40. Hughes, TP, Hochhaus, A, Kantarjian, HM, Cervantes, F, Guilhot, F, Niederwieser, D, et al. Safety and efficacy of switching to nilotinib 400 mg twice daily for patients with chronic myeloid leukemia in chronic phase with suboptimal response or failure on front-line imatinib or nilotinib 300 mg twice daily. Haematologica. (2014) 99:1204–11. doi: 10.3324/haematol.2013.091272
41. Dorer, DJ, Knickerbocker, RK, Baccarani, M, Cortes, JE, Hochhaus, A, Talpaz, M, et al. Impact of dose intensity of ponatinib on selected adverse events: multivariate analyses from a pooled population of clinical trial patients. Leuk Res. (2016) 48:84–91. doi: 10.1016/j.leukres.2016.07.007
42. Cortes, J, Apperley, J, Lomaia, E, Moiraghi, B, Undurraga Sutton, M, Pavlovsky, C, et al. Ponatinib dose-ranging study in chronic-phase chronic myeloid leukemia: a randomized, open-label phase 2 clinical trial. Blood. (2021) 138:2042–50. doi: 10.1182/blood.2021012082
43. Fradley, MG, and Moslehi, J. QT prolongation and oncology drug development. Card Electrophysiol Clin. (2015) 7:341–55. doi: 10.1016/j.ccep.2015.03.013
44. Tisdale, JE, Jaynes, HA, Kingery, JR, Mourad, NA, Trujillo, TN, Overholser, BR, et al. Development and validation of a risk score to predict QT interval prolongation in hospitalized patients [published correction appears in Circ Cardiovasc Qual outcomes. 2013 Nov;6(6):e57]. Circ Cardiovasc Qual Outcomes. (2013) 6:479–87. doi: 10.1161/CIRCOUTCOMES.113.000152
45. Stone, JR, Kanneganti, R, Abbasi, M, and Akhtari, M. Monitoring for chemotherapy-related Cardiotoxicity in the form of left ventricular systolic dysfunction: a review of current recommendations. JCO Oncol Pract. (2021) 17:228–36. doi: 10.1200/OP.20.00924
46. Thavendiranathan, P, Negishi, T, Somerset, E, Negishi, K, Penicka, M, Lemieux, J, et al. Strain-guided management of potentially cardiotoxic cancer therapy. J Am Coll Cardiol. (2021) 77:392–401. doi: 10.1016/j.jacc.2020.11.020
47. Negishi, K, Negishi, T, Hare, JL, Haluska, BA, Plana, JC, and Marwick, TH. Independent and incremental value of deformation indices for prediction of trastuzumab-induced cardiotoxicity. J Am Soc Echocardiogr. (2013) 26:493–8. doi: 10.1016/j.echo.2013.02.008
48. Fox, LC, Cummins, KD, Costello, B, Yeung, D, Cleary, R, Forsyth, C, et al. The incidence and natural history of dasatinib complications in the treatment of chronic myeloid leukemia. Blood Adv. (2017) 1:802–11. doi: 10.1182/bloodadvances.2016003889
49. Naqvi, K, Jabbour, E, Skinner, J, Yilmaz, M, Ferrajoli, A, Bose, P, et al. Early results of lower dose dasatinib (50 mg daily) as frontline therapy for newly diagnosed chronic-phase chronic myeloid leukemia. Cancer. (2018) 124:2740–7. doi: 10.1002/cncr.31357
50. Naqvi, K, Jabbour, E, Skinner, J, Anderson, K, Dellasala, S, Yilmaz, M, et al. Long-term follow-up of lower dose dasatinib (50 mg daily) as frontline therapy in newly diagnosed chronic-phase chronic myeloid leukemia. Cancer. (2020) 126:67–75. doi: 10.1002/cncr.32504
51. Murai, K, Ureshino, H, Kumagai, T, Tanaka, H, Nishiwaki, K, Wakita, S, et al. Low-dose dasatinib in older patients with chronic myeloid leukaemia in chronic phase (DAVLEC): a single-arm, multicentre, phase 2 trial. Lancet Haematol. (2021) 8:e902–11. doi: 10.1016/S2352-3026(21)00333-1
52. Simonneau, G, and Hoeper, MM. The revised definition of pulmonary hypertension: exploring the impact on patient management. Eur Heart J Suppl. (2019) 21:K4–8. doi: 10.1093/eurheartj/suz211
53. Weatherald, J, Chaumais, MC, Savale, L, Jaïs, X, Seferian, A, Canuet, M, et al. Long-term outcomes of dasatinib-induced pulmonary arterial hypertension: a population-based study. Eur Respir J. (2017) 50:1700217. doi: 10.1183/13993003.00217-2017
54. Interactions . Lexi-Drugs. Hudson, OH: Lexicomp. Available at: http://online.lexi.com/lco/action/interact (Accessed December 8, 2022).
Keywords: chronic myeloid leukemia, tyrosine kinase, heart failure, pulmonary arterial hypertension, QT prolongation, dasatinib, ponatinib, pleural effusion
Citation: Kaddoura R, Dabdoob WA, Ahmed K and Yassin MA (2023) A practical guide to managing cardiopulmonary toxicities of tyrosine kinase inhibitors in chronic myeloid leukemia. Front. Med. 10:1163137. doi: 10.3389/fmed.2023.1163137
Edited by:
Ahmet Emre Eskazan, Istanbul University-Cerrahpasa, TürkiyeReviewed by:
Mario Tiribelli, University of Udine, ItalyDavid Yeung, South Australian Health and Medical Research Institute (SAHMRI), Australia
Copyright © 2023 Kaddoura, Dabdoob, Ahmed and Yassin. This is an open-access article distributed under the terms of the Creative Commons Attribution License (CC BY). The use, distribution or reproduction in other forums is permitted, provided the original author(s) and the copyright owner(s) are credited and that the original publication in this journal is cited, in accordance with accepted academic practice. No use, distribution or reproduction is permitted which does not comply with these terms.
*Correspondence: Rasha Kaddoura, UmFzaGEua2FkZG91cmFAZ21haWwuY29t; Mohamed A. Yassin, eWFzc2lubW9oYUBnbWFpbC5jb20=